Bioprinting in cardiovascular tissue engineering: a review
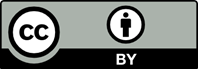
Fabrication techniques for cardiac tissue engineering have been evolving around scaffold-based and scaffold-free approaches. Conventional fabrication approaches lack control over scalability and homogeneous cell distribution. Bioprinting provides a technological platform for controlled deposition of biomaterials, cells, and biological factors in an organized fashion. Bioprinting is capable of alternating heterogeneous cell printing, printing anatomical relevant structure and microchannels resembling vasculature network. These are essential features of an engineered cardiac tissue. Bioprinting can potentially build engineered cardiac construct that resembles native tissue across macro to nanoscale.
1. Braunwald E, 2013, Heart failure. JACC: Heart Failure, vol.1(1): 1–20. http://dx.doi.org/10.1016/j.jchf.2012.10.002
2. Go A S, Mozaffarian D, Roger V L, et al., 2014, Heart disease and stroke statistics—2014 update: a report from the American Heart Association. Circulation, vol.129: e28–e292. http://dx.doi.org/10.1161/01.cir.0000441139.02102.80
3. Adler C P, Friedburg H, Herget G W, et al., 1996, Varia-bility of cardiomyocyte DNA content, ploidy level and nuclear number in mammalian hearts. Virchows Archiv, vol.429: 159–164. http://dx.doi.org/10.1007/bf00192438
4. Laflamme M A and Murry C E, 2011, Heart regenera-tion. Nature, vol.473: 326–335. http://dx.doi.org/10.1038/nature10147
5. Hunter P, 2009, Cardiovascular mechanics, in Binder M, Hirokawa N and Windhorst U (eds), Encyclopedia of Neuroscience, Springer, Berlin Heidelberg, 564–567. http://dx.doi.org/10.1007/978-3-540-29678-2_824
6. Ferreira F P, Kilner P J, McGill L A, et al., In vivo car-diovascular magnetic resonance diffusion tensor imaging shows evidence of abnormal myocardial laminar orientations and mobility in hypertrophic cardiomyopathy. Journal of Cardiovascular Magnetic Resonance, vol.16(87). http://dx.doi.org/10.1186/s12968-014-0087-8
7. Tillman B, Hardin-Young J, Shannon W, et al., 2013, Meeting the need for regenerative therapies: translation-focused analysis of U.S. regenerative medicine op-portunities in cardiovascular and peripheral vascular medicine using detailed incidence data. Tissue Engi-neering Part B: Reviews, vol.19(2): 99–115. http://dx.doi.org/10.1089/ten.teb.2011.0678
8. Makkar R R, Smith R R, Cheng K, et al., 2012, Intra-coronary cardiosphere-derived cells for heart regenera-ion after myocardial infarction (CADUCEUS): A pros-pective, randomised phase 1 trial. The Lancet, vol.379(9819): 895–904. http://dx.doi.org/10.1016/S0140-6736(12)60195-0
9. Bolli R, Chugh A R, D'Amario D, et al., 2011, Cardiac stem cells in patients with ischaemic cardiomyopathy (SCIPIO): initial results of a randomised phase 1 trial. Lancet, vol.378(9806): 1847–1857. http://dx.doi.org/10.1016/S0140-6736(11)61590-0
10. Lin Y D, Yeh M L, Yang Y J, et al., 2010, Intramyocar-dial peptide nanofiber injection improves postinfarction ventricular remodeling and efficacy of bone marrow cell therapy in pigs. Circulation, vol.122: S132–S141. http://dx.doi.org/10.1161/CIRCULATIONAHA.110.939512
11. Malliaras K, Makkar R R, Smith R R, et al., 2014, Intracoronary cardiosphere-derived cells after myocar-dial infarction: evidence of therapeutic regeneration in the final 1-year results of the CADUCEUS trial (CAr-diosphere-Derived aUtologous stem CElls to reverse ventricUlar dySfunction). Journal of the American Col-lege of Cardiology, vol.63(2): 110–122. http://dx.doi.org/10.1016/j.jacc.2013.08.724
12. Bolli R, Chugh A R, D'Amario D, et al., 2011, Cardiac stem cells in patients with ischaemic cardiomyopathy (SCIPIO): initial results of a randomised phase 1 trial. The Lancet, vol.378(9806): 1847–1857. http://dx.doi.org/10.1016/S0140-6736(11)61590-0
13. Cheng K, Blusztajn A, Shen D, et al., 2012, Functional performance of human cardiosphere-derived cells deli-vered in an in situ polymerizable hyaluronan-gelatin hydrogel. Biomaterials, vol.33(21): 5317–5324. http://dx.doi.org/10.1016/j.biomaterials.2012.04.006
14. Wiria F E, Leong K F, Chua C K, et al., 2007, Poly- ε-caprolactone/hydroxyapatite for tissue engineering scaffold fabrication via selective laser sintering. Acta Biomaterialia, vol.3(1): 1–12. http://dx.doi.org/10.1016/j.actbio.2006.07.008
15. Langer R and Vacanti J P, 1993, Tissue engineering. Science, vol.260(5510): 920–926. http://dx.doi.org/10.1126/science.8493529
16. Yeong W Y, Chua C K, Leong K F, et al., 2004, Rapid
prototyping in tissue engineering: challenges and poten-tial. Trends in Biotechnology, vol.22(12): 643–652. http://dx.doi.org/10.1016/j.tibtech.2004.10.004
17. Tillman B, Hardin-Young J, Shannon W, et al., 2013, Meeting the need for regenerative therapies: transla-tion-focused analysis of U.S. regenerative medicine op-portunities in cardiovascular and peripheral vascular medicine using detailed incidence data. Tissue Engi-neering Part B: Reviews, vol.19(2): 99–115. http://dx.doi.org/10.1089/ten.teb.2011.0678
18. Sing S L, An J, Yeong W Y, et al., 2016, Laser and elec-tron-beam powder-bed additive manufacturing of metal-lic implants: a review on processes, materials and de-signs. Journal of Orthopaedic Research, vol.34(3): 369–385. http://dx.doi.org/10.1002/jor.23075
19. Sing S L, Yeong W Y, Wiria F E, et al., 2016, Characte-rization of titanium lattice structures fabricated by selec-tive laser melting using an adapted compressive test method. Experimental Mechanics, vol.56(5): 735–748. http://dx.doi.org/10.1007/s11340-015-0117-y
20. Yeong W Y, Sudarmadji N, Yu H Y, et al., 2010, Porous polycaprolactone scaffold for cardia tissue engineering fabricated by selective laser sintering. Acta Biomateria-lia, vol.6(6): 2028–2034. http://dx.doi.org/10.1016/j.actbio.2009.12.033
21. Jawad H, Ali N N, Lyon A R, et al., 2007, Myocardial tissue engineering: a review. Journal of Tissue Engi-neering and Regenerative Medicine, vol.1(5): 327–342. http://dx.doi.org/10.1002/term.46
22. Iyer R K, Chiu L L, Reis L A, et al., 2011, Engineered cardiac tissues. Current Opinion in Biotechnology, vol.22(5): 706–714. http://dx.doi.org/10.1016/j.copbio.2011.04.004
23. Kohl P, Camelliti P, Burton F L, et al., 2005, Electrical coupling of fibroblasts and myocytes: relevance for car-diac propagation. Journal of Electrocardiology, vol.38(4): 45–50. http://dx.doi.org/10.1016/j.jelectrocard.2005.06.096
24. Camelliti P, Green C R, LeGrice I, et al., 2004, Fibrob-last network in rabbit sinoatrial node: structural and functional identification of homogeneous and heteroge-neous cell coupling. Circulation Research, vol.94: 828–835. http://dx.doi.org/10.1161/01.RES.0000122382.19400.14
25. Hirsch E, Nagai R and Thum T, 2014, Heterocellular signalling and crosstalk in the heart in ischaemia and heart failure. Cardiovascular Research, vol.102(2): 191–193. http://dx.doi.org/10.1093/cvr/cvu073
26. Nakatani S, 2011, Left ventricular rotation and twist: why should we learn? Journal of Cardiovascular Ul-trasound, vol.19(1): 1–6. http://dx.doi.org/10.4250/jcu.2011.19.1.1
27. Vunjak-Novakovic G, Lui K O, Tandon N, et al., 2011, Bioengineering heart muscle: a paradigm for regenera-tive medicine. Annual Review of Biomedical Engineer-ing, vol.13: 245–267. http://dx.doi.org/10.1146/annurev-bioeng-071910-124701
28. LeBlon C E, Pai R, Fodor C R, et al., 2013, In vitro comparative biodegradation analysis of salt-leached porous polymer scaffolds. Journal of Applied Polymer Science, vol.128(5): 2701–2712. http://dx.doi.org/10.1002/app.38321
29. Sin D, Miao X G, Liu G, et al., 2010, Polyurethane (PU) scaffolds prepared by solvent casting/particulate leach-ing (SCPL) combined with centrifugation. Materials Science & Engineering C: Materials for Biological Applications, vol.30(1): 78–85. http://dx.doi.org/10.1016/j.msec.2009.09.002
30. Pego A P, Siebum B, Van Luyn M J A, et al., 2003, Preparation of degradable porous structures based on 1,3-trimethylene carbonate and D,L-lactide (co)poly-mers for heart tissue engineering. Tissue Engineering, vol.9(5): 981–994. http://dx.doi.org/10.1089/107632703322495628
31. Neal R A, Jean A, Park H, et al., 2013, Three-dimensional elastomeric scaffolds designed with cardiac-mimetic structural and mechanical features. Tissue Engineering Part A, vol.19(5-6): 793–807. http://dx.doi.org/10.1089/ten.tea.2012.0330
32. Kharaziha M, Nikkhah M, Shin S R, et al., 2013, PGS: Gelatin nanofibrous scaffolds with tunable mechanical and structural properties for engineering cardiac tissues. Biomaterials, vol.34(27): 6355–6366. http://dx.doi.org/10.1016/j.biomaterials.2013.04.045
33. Orlova Y, Magome N, Liu L, et al., 2011, Electrospun nanofibers as a tool for architecture control in engineered cardiac tissue. Biomaterials, vol.32(24): 5615– 5624. http://dx.doi.org/10.1016/j.biomaterials.2011.04.042
34. Xu B, Li Y, Fang X, et al., 2013, Mechanically tissue-like elastomeric polymers and their potential as a vehicle to deliver functional cardiomyocytes. Journal of the Mechanical Behavior of Biomedical Materials, vol.28: 354–365. http://dx.doi.org/10.1016/j.jmbbm.2013.06.005
35. Prabhakaran M P, Nair A S, Kai D, et al., 2012, Electrospun composite scaffolds containing poly(octanediol-co-citrate) for cardiac tissue engineering. Biopolymers, vol.97(7): 529–538. http://dx.doi.org/10.1002/bip.22035
36. Kai D, Prabhakaran M P, Jin G, et al., 2011, Guided orientation of cardiomyocytes on electrospun aligned nanofibers for cardiac tissue engineering. Journal of Biomedical Materials Research Part B: Applied Biomaterials, vol.98(2): 379–386. http://dx.doi.org/10.1002/jbm.b.31862
37. Shevach M, Maoz B M, Feiner R, et al., 2013, Nanoengineering gold particle composite fibers for cardiac tissue engineering. Journal of Materials Chemistry B, vol.1(39): 5210–5217. http://dx.doi.org/10.1039/c3tb20584c
38. Fleischer S, Feiner R, Shapira A, et al., 2013, Spring-like fibers for cardiac tissue engineering. Biomaterials, vol.34(34): 8599-8606. http://dx.doi.org/10.1016/j.biomaterials.2013.07.054
39. Engelmayr G C Jr, Cheng M, Bettinger C J, et al., 2008, Accordion-like honeycombs for tissue engineering of cardiac anisotropy. Nature Materials, vol.7: 1003–1010. http://dx.doi.org/10.1038/nmat2316
40. Zhang D, Shadrin I Y, Lam J, et al., 2013, Tissue-engineered cardiac patch for advanced functional maturation of human ESC-derived cardiomyocytes. Biomaterials, vol.34(23): 5813–5820. http://dx.doi.org/10.1016/j.biomaterials.2013.04.026
41. Zimmermann W H, Fink C, Kralisch D, et al., 2000, Three-dimensional engineered heart tissue from neonatal rat cardiac myocytes. Biotechnology and Bioengineering, vol.68(1): 106–114. http://dx.doi.org/10.1002/(SICI)1097-0290(20000405)68:1%3C106::AID-BIT13%3E3.0.CO;2-3
42. Zimmermann W H, Melnychenko I, Wasmeier G, et al., 2006, Engineered heart tissue grafts improve systolic and diastolic function in infarcted rat hearts. Natural Medicine, vol.12: 452–458. http://dx.doi.org/10.1038/nm1394
43. Dvir T, Timko B P, Brigham M D, et al., 2011, Nano-wired three-dimensional cardiac patches. Nature Nanotechnology, vol.6: 720–725. http://dx.doi.org/10.1038/nnano.2011.160
44. Dohmen P M, Scheckel M, Stein-Konertz M, et al., 2002, In vitro hydrodynamics of a decellularized pulmonary porcine valve, compared with a glutaraldehyde and polyurethane heart valve. International Journal of Artificial Organs, vol.25: 1089–1094.
45. Juthier F, Vincentelli A, Gaudric J, et al., 2006, Decellularized heart valve as a scaffold for in vivo recellularization: deleterious effects of granulocyte colony-stimulating factor. Journal of Thoracic and Cardiovascular Surgery, vol.131(4): 843–852. http://dx.doi.org/10.1016/j.jtcvs.2005.11.037
46. Ott H C, Matthiesen T S, Goh S K, et al., 2008, Perfusion-decellularized matrix: using nature's platform to engineer a bioartificial heart. Nature Medicine, vol.14: 213–221. http://dx.doi.org/10.1038/nm1684
47. Song J J and Ott H C, 2011, Organ engineering based on decellularized matrix scaffolds. Trends in Molecular Medicine, vol.17(8): 424–432. http://dx.doi.org/10.1016/j.molmed.2011.03.005
48. Li Z and Guan J, 2011, Hydrogels for cardiac tissue engineering. Polymers, vol.3(2): 740–761. http://dx.doi.org/10.3390/polym3020740
49. Chua C K, Yeong W Y and Leong K F, 2005, Rapid prototyping in tissue engineering: a state-of-the-art re-port, in Virtual Modelling and Rapid Manufacturing: Advanced Research in Virtual and Rapid Prototyping. Proceedings of the 2nd International Conference on Advanced Research in Virtual and Rapid Prototyping, Leiria, Portugal, 28 September–1 October, 2005, 19–27.
50. Yeong W Y, Chua C K and Leong K F, et al., 2005, Development of scaffolds for tissue engineering using a 3D inkjet model maker, in Virtual Modelling and Rapid Manufacturing: Advanced Research in Virtual and Rapid Prototyping. Proceedings of the 2nd International Conference on Advanced Research in Virtual and Rapid Prototyping, Leiria, Portugal, 28 September–1 October, 2005, 115–118.
51. Shimizu T, 2014, Cell sheet-based tissue engineering for fabricating 3-dimensional heart tissues. Circulation Journal, vol.78(11): 2594–2603. http://doi.org/10.1253/circj.CJ-14-0973
52. Yasui H, Lee J K, Yoshida A, et al., 2014, Excitation propagation in three-dimensional engineered hearts using decellularized extracellular matrix. Biomaterials, vol.35(27): 7839–7850. http://dx.doi.org/10.1016/j.biomaterials.2014.05.080
53. Shimizu T, Yamato M, Kikuchi A, et al., 2003, Cell sheet engineering for myocardial tissue reconstruction. Biomaterials, vol.24(13): 2309–2316. http://dx.doi.org/10.1016/S0142-9612(03)00110-8
54. Hata H, Bar A, Dorfman S, et al., 2010, Engineering a novel three-dimensional contractile myocardial patch with cell sheets and decellularised matrix. European Journal of Cardio-Thoracic Surgery, vol.38(4): 450–455. http://dx.doi.org/10.1016/j.ejcts.2010.02.009
55. Miki K, Uenaka H, Saito A, et al., 2012, Bioengineered myocardium derived from induced pluripotent stem cells improves cardiac function and attenuates cardiac remodeling following chronic myocardial infarction in rats. Stem Cells Translational Medicine, vol.1(5): 430– 437. http://dx.doi.org/10.5966/sctm.2011-0038
56. Hasegawa A, Haraguchi Y, Shimizu T, et al., 2015, Rapid fabrication system for three-dimensional tissues using cell sheet engineering and centrifugation. Journal of Biomedical Materials Research Part A, vol.103(12): 3825–3833. http://dx.doi.org/10.1002/jbm.a.35526
57. Sakaguchi K, Shimizu T, Horaguchi S, et al., 2013, In Vitro engineering of vascularized tissue surrogates. Scientific Reports, vol.3: 1316. http://dx.doi.org/10.1038/srep01316
58. Guillemot F, Mironov V and Nakamura M, 2010, Bio-printing is coming of age: report from the International
Conference on Bioprinting and Biofabrication in Bor-deaux (3B'09). Biofabrication, vol.2(1): 010201. http://dx.doi.org/10.1088/1758-5082/2/1/010201
59. Ozbolat I T and Hospodiuk M, 2016, Current advances and future perspectives in extrusion-based bioprinting. Biomaterials, vol.76: 321–343. http://dx.doi.org/10.1016/j.biomaterials.2015.10.076
60. Xu T, Zhao W, Zhu J M, et al., 2013, Complex heterogeneous tissue constructs containing multiple cell types prepared by inkjet printing technology. Biomaterials, vol.34(1): 130–139. http://dx.doi.org/10.1016/j.biomaterials.2012.09.035
61. Guillemot F, Guillotin B, Fontaine A, et al., 2011, Laser-assisted bioprinting to deal with tissue complexity in regenerative medicine. MRS Bulletin, vol.36(12): 1015– 1019. http://dx.doi.org/10.1557/mrs.2011.272
62. Lee H, Ahn S, Bonassar L J, et al., 2013, Cell-laden poly(varepsilon-caprolactone)/alginate hybrid scaffolds fabricated by an aerosol cross-linking process for obtaining homogeneous cell distribution: fabrication, seeding efficiency, and cell proliferation and distribution. Tissue Engineering Part C: Methods, vol.19(10): 784– 793. http://dx.doi.org/10.1089/ten.tec.2012.0651
63. Billiet T, Gevaert E, De Schryver T, et al., 2014, The 3D printing of gelatin methacrylamide cell-laden tissue-engineered constructs with high cell viability. Biomaterials, vol.35(1): 49–62. http://dx.doi.org/10.1016/j.biomaterials.2013.09.078
64. Duan B, Hockaday L A, Kang K H, et al., 2013, 3D bioprinting of heterogeneous aortic valve conduits with alginate/gelatin hydrogels. Journal of Biomedical Materials Research: Part A, vol.101(5): 1255–1264. http://dx.doi.org/10.1002/jbm.a.34420
65. Fedorovich N E, Wijnberg H M, Dhert W J et al., 2011, Distinct tissue formation by heterogeneous printing of osteo- and endothelial progenitor cells. Tissue Engineering Part A, vol.17(15–16): 2113–2121. http://dx.doi.org/10.1089/ten.TEA.2011.0019
66. Huang Y, He K and Wang X, 2013, Rapid prototyping of a hybrid hierarchical polyurethane-cell/hydrogel construct for regenerative medicine. Materials Science and Engineering: C. Materials for Biological Applications, vol.33(6): 3220–3229. http://dx.doi.org/10.1016/j.msec.2013.03.048
67. Ozbolat I T, Chen H and Yu Y, 2014, Development of ‘Multi-arm Bioprinter’ for hybrid biofabrication of tissue engineering constructs. Robotics and Computer-Integrated Manufacturing, vol.30(3): 295–304. http://dx.doi.org/10.1016/j.rcim.2013.10.005
68. Shim J M, Lee J S, Kim J Y, et al., 2012, Bioprinting of a mechanically enhanced three-dimensional dual cell-laden construct for osteochondral tissue engineering using a multi-head tissue/organ building system. Journal of Micromechanics and Microengineering, vol.22(8): 085014. http://dx.doi.org/10.1088/0960-1317/22/8/085014
69. Snyder J E, Hamid Q, Wang C, et al., 2011, Bioprinting cell-laden matrigel for radioprotection study of liver by pro-drug conversion in a dual-tissue microfluidic chip. Biofabrication, vol.3(3): 034112. http://dx.doi.org/10.1088/1758-5082/3/3/034112
70. Wang X H, Yan Y N, Pan Y Q, et al., 2006, Generation of three-dimensional hepatocyte/gelatin structures with rapid prototyping system. Tissue Engineering, vol.12(1): 83–90.
71. Skardal A, Zhang J and Prestwich G D, 2010, Bioprinting vessel-like constructs using hyaluronan hydrogels crosslinked with tetrahedral polyethylene glycol tetracrylates. Biomaterials, vol.31(24): 6173–6181. http://dx.doi.org/10.1016/j.biomaterials.2010.04.045
72. Visser J, Peters B, Burger T J, et al., 2013, Biofabrication of multi-material anatomically shaped tissue constructs. Biofabrication, vol.5(3): 035007. http://dx.doi.org/10.1088/1758-5082/5/3/035007
73. Lee W, Lee V, Polio S, et al., 2009, Three-dimensional cell-hydrogel printer using electromechanical micro-valve for tissue engineering. in TRANSDUCERS 2009 — 2009 International Solid-State Sensors, Actuators and Microsystems Conference, 2230–2233. http://dx.doi.org/10.1109/SENSOR.2009.5285591
74. Gaetani R, Doevendans P A, Metz C H, J. et al., 2012, Cardiac tissue engineering using tissue printing technology and human cardiac progenitor cells. Biomaterials, vol.33(6): 1782–1790. http://dx.doi.org/10.1016/j.biomaterials.2011.11.003
75. Kolesky D B, Truby R L, Gladman A S, et al., 2014, 3D bioprinting of vascularized, heterogeneous cell-laden tissue constructs. Advanced Materials, vol.26(19): 3124–3130. http://dx.doi.org/10.1002/adma.201305506
76. Norotte C, Marga F S, Niklason L E, et al., 2009, Scaffold-free vascular tissue engineering using bioprinting. Biomaterials, vol.30(30): 5910–5917. http://dx.doi.org/10.1016/j.biomaterials.2009.06.034
77. Hinton T J, Jallerat Q, Palchesko R N, et al., 2015, Three-dimensional printing of complex biological structures by freeform reversible embedding of suspended hydrogels. Science Advances, vol.1: e1500758. http://dx.doi.org/10.1126/sciadv.1500758
78. Gaebel R, Ma N, Liu J, et al., 2011, Patterning human stem cells and endothelial cells with laser printing for cardiac regeneration. Biomaterials, vol.32(35): 9218– 9230.
http://dx.doi.org/10.1016/j.biomaterials.2011.08.071
79. Xu T, Baicu C, Aho M, et al., 2009, Fabrication and characterization of bio-engineered cardiac pseudo tis-sues. Biofabrication, vol.1(3): 035001. http://dx.doi.org/10.1088/1758-5082/1/3/035001
80. Vunjak-Novakovic G, Eschenhagen T and Mummery C, 2014, Myocardial tissue engineering: in vitro models. Cold Spring Harbor Perspectives in Medicine, vol.4.(3): a014076. http://dx.doi.org/10.1101/cshperspect.a014076
81. Chanthakulchan A, Koomsap P, Parkhi A A, et al., 2015, Environmental effects in fibre fabrication using electrospinning-based rapid prototyping. Virtual and Physical Prototyping, vol.10(4): 227–237. http://dx.doi.org/10.1080/17452759.2015.1112411
82. Sooppan R, Paulsen S J, Han J, et al., 2016, In vivo anastomosis and perfusion of a three-dimensionally-printed construct containing microchannel networks. Tissue Engineering Part C: Methods, vol.22(1): 1–7. http://dx.doi.org/10.1089/ten.TEC.2015.0239
83. Liu L B and Wang X H, 2015, Creation of a vascular system for organ manufacturing. International Journal of Bioprinting, vol.1(1): 77–86. http://dx.doi.org/10.18063/IJB.2015.01.009
84. Dvir T, Timko B P, Brigham M D, et al., 2011, Nano-wired three-dimensional cardiac patches. Nature Nanotechnology, vol.6(11): 720–725. http://dx.doi.org/10.1038/nnano.2011.160
85. Xu L, Gutbrod S R, Bonifas A P, et al., 2014, 3D multi-functional integumentary membranes for spatiotemporal cardiac measurements and stimulation across the entire epicardium. Nature Communications, vol.5: 3329. http://dx.doi.org/10.1038/ncomms4329
86. Wang S, Lee J M and Yeong W Y, 2015, Smart hydrogels for 3D bioprinting. International Journal of Bioprinting, vol.1(1): 3–14. http://dx.doi.org/10.18063/IJB.2015.01.005
87. Dixon J E, Shah D A, Rogers C, et al., 2014, Combined hydrogels that switch human pluripotent stem cells from self-renewal to differentiation. Proceedings of the National Academy of Sciences, vol.111(15): 5580–5585. http://dx.doi.org/10.1073/pnas.1319685111
88. Lee H Y, Kim H W, Lee J H, et al., 2015, Controlling oxygen release from hollow microparticles for pro-longed cell survival under hypoxic environment. Biomaterials, vol.53: 583–591. http://dx.doi.org/10.1016/j.biomaterials.2015.02.117
89. Farris A L, Rindone A N, Grayson W L, 2016, Oxygen delivering biomaterials for tissue engineering. Journal of Materials Chemistry B, vol.4(20): 3422–3432. http://dx.doi.org/10.1039/C5TB02635K