The External Exposome and Allergies: From the Perspective of the Epithelial Barrier Hypothesis
- 1Clinic of Immunology and Allergic Diseases, Ankara City Hospital, Ankara, Turkey
- 2Department of Nutrition and Diet, Ankara University, Ankara, Turkey
- 3Division of Immunology and Allergic Diseases, Department of Internal Medicine, School of Medicine, Ankara University, Ankara, Turkey
- 4Division of Immunology and Allergic Diseases, Department of Chest Diseases, School of Medicine, Ankara University, Ankara, Turkey
- 5Clinic of Immunology and Allergic Diseases, Eskisehir City Hospital, Eskisehir, Turkey
- 6Clinic of Immunology and Allergic Diseases, Kayseri City Hospital, Kayseri, Turkey
- 7Swiss Institute of Allergy and Asthma Research (SIAF), University of Zurich, Davos, Switzerland
- 8Division of Immunology and Allergic Diseases, Department of Chest Diseases, Erciyes University, Kayseri, Turkey
- 9Department of Pediatric Basic Sciences, Institute of Child Health, Istanbul University, Istanbul, Turkey
- 10Division of Pediatric Allergy and Immunology, Department of Pediatrics, Istanbul Faculty of Medicine, Istanbul University, Istanbul, Turkey
- 11Christine Kühne-Center for Allergy Research and Education (CK-CARE), Davos, Switzerland
Introduction: In the last decades, we have seen a rapid increase in the prevalence of allergic diseases such as asthma, allergic rhinitis, atopic dermatitis, and food allergies. The environmental changes caused by industrialization, urbanization and modernization, including dramatic increases in air pollutants such as particulate matter (PM), diesel exhaust, nitrogen dioxide (NO2), ozone (O3), alarming effects of global warming, change and loss of biodiversity, affect both human health and the entire ecosystem.
Objective: In this review, we aimed to discuss the effects of the external exposome on epithelial barriers and its relationship with the development of allergic diseases by considering the changes in all stakeholders of the outer exposome together, in the light of the recently proposed epithelial barrier hypothesis.
Method: To reach current, prominent, and comprehensive studies on the subject, PubMed databases were searched. We included the more resounding articles with reliable and strong results.
Results: Exposure to altered environmental factors such as increased pollution, microplastics, nanoparticles, tobacco smoke, food emulsifiers, detergents, and household cleaners, and climate change, loss and change in microbial biodiversity, modifications in the consumption of dietary fatty acids, the use of emulsifiers, preservatives and the decrease in the antioxidant content of the widely consumed western diet may disrupt the epithelial barriers of the skin, respiratory and gastrointestinal tracts, making us more vulnerable to exogeneous allergens and microbes. Epithelial cell activation, microbial dysbiosis and bacterial translocation disrupt the immune balance and a chronic Th2 inflammation ensues.
Conclusion: Dramatic increases in air pollution, worrisome effects of global warming, dysbiosis, changing dietary habits and the complex interactions of all these factors affect the epithelial barriers and local and systemic inflammation. We want to draw attention to the emerging health effects of environmental changes and to motivate the public to influence government policies for the well-being of humans and the nature of the earth and the well-being of future generations.
Introduction
The concept of the exposome covers all environmental exposures throughout an individual's life span (1, 2). These exposures can be sub-grouped into three categories: general external environment (climate, biodiversity, urban environment, social and economic factors), specific external environment (allergens, microbes, diet, tobacco, pollutants, and toxic substances), and host-dependent internal environment (metabolic factors, inflammation, and oxidative stress) (1, 3). The bidirectional effect of the environment on human beings and the effect of humans on all other living systems and genomes has been recently referred to as the meta-exposome (4).
A high biodiversity has been proposed to enrich the human microbiome, maintain immune homeostasis, and protect against allergic and inflammatory diseases (5). After the industrial revolution in the 19th century, air pollutants increased significantly in all areas with industrial sectors, especially in urban areas. These contribute to global warming, and loss of biodiversity not only in human microbiota but also in the ecosystem (3, 6, 7). Westernized (modern urban life) diets are characterized by low antioxidants and fiber intake and high fatty acid content. In addition, processed foods with additives, such as preservatives, enzymes, and emulsifiers have become an important part of the diet (8, 9). The “epithelial barrier hypothesis” has been recently proposed to explain the detrimental effect of all these factors on human health, attributing it to the damage of these substances to the epithelial barriers of the skin, respiratory and gastrointestinal tracts and reshaping of the microbiome, leading to initiation of peri epithelial inflammation (8).
In this review, we discuss how these environmental and lifestyle changes including climate change, microbial dysbiosis, altered diet preferences, processed food additives, and increased exposure to environmental insults and pollutants (detergents, airborne allergens, particulate matter (PM), ozone, microplastics, nanoparticles, and tobacco smoke) affect the development of allergic diseases in relation with the recently proposed “epithelial barrier hypotheses” (Figure 1).
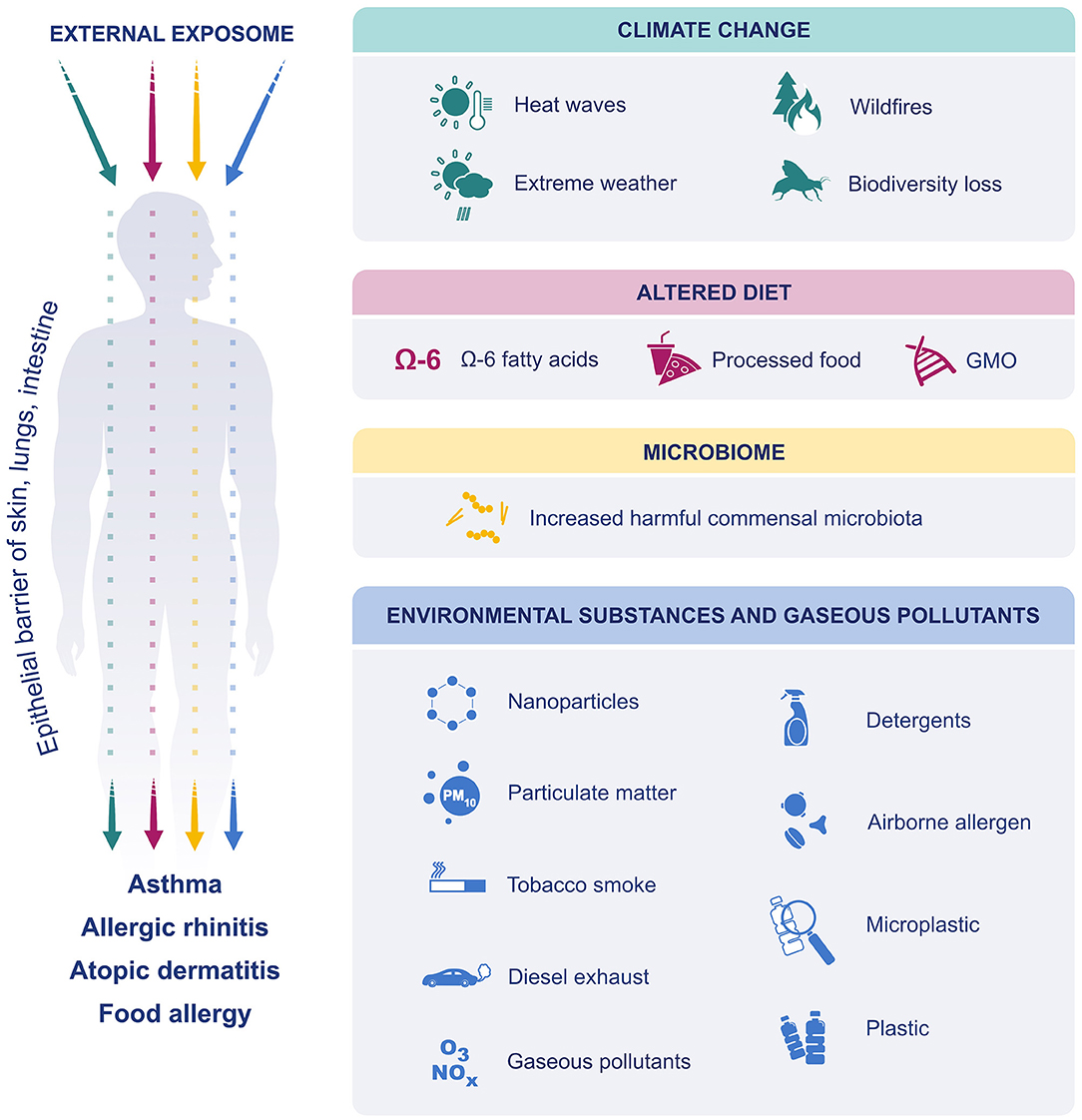
Figure 1. The effect of external exposome on epithelial barriers of skin lung and intestine. Due to climate change, extreme weather events have become more frequent and more intense. The air was polluted, and biodiversity was lost. Dietary preferences have shifted toward increased consumption of processed foods, n-6 fatty acids and GM foods. Exposure to environmental substances such as, detergents, PM, ozone, diesel exhaust, nanoparticles, microplastics, environmental tobacco smoke and airborne allergens were increased. Finally, the microbiome was affected, and increase in harmful commensals resulted in dysbiosis. All these factors affect and disrupt the epithelial barriers of skin, lung and gastrointestinal system and cause allergic diseases.
Climate Change
Anthropogenic activities are increasing the emissions of carbon dioxide (CO2), methane (CH4), nitrogen oxides (NOx), fluorinated gases and PM in the atmosphere and cause global warming by trapping heat on Earth, the greenhouse effect, which is the biggest threat to our planet including all ecosystems (9). As a result, natural disasters are becoming more frequent and severe as observed in recent years with record numbers of heat waves, wildfires, thunderstorms, sandstorms, droughts, floods, blizzards, and hurricanes (10, 11). A warmer and more humid environment changes the cycle and seasonal nature of plants in some regions, prolongs the pollen season, increases the quantity of pollen, and alters the distribution, timing, dispersion of some pollen species (i.e., ragweed in Europe), which may lead to increase in the prevalence and severity of allergies (3, 9, 11). In addition, rising levels of CO2 and other air pollutants may increase pollen outputs and allergenicity (3, 12–14). Besides increased urbanization, global warming has created warm and humid conditions, which provide a suitable environment for the growth of both indoor allergens and outdoor molds (15, 16). The protease activities of house dust mites and Aspergillus fumigatus have been shown to damage the epithelial barriers (17). There are many unknowns on the impact of climate change on human health and further research is warranted to elucidate the causative relationship between climate change and different types of allergies or other inflammatory conditions. For instance, it has been shown that pollen, independent of allergens, alters innate immunity and especially impairs antiviral defense by changing the immunological barrier functions of airway epithelial cells, interfering with NF-κB signaling in dendritic cells, reducing interferon-λ and proinflammatory chemokine responses (22, 23).
The prevalence of peanut and tree nut allergies has increased during the last few decades, but there is insufficient evidence to link this phenomenon solely to climate change or an increase in CO2 concentration in the atmosphere (24). Elevated CO2 concentrations may alter the allergenic potential of peanuts, possibly by increasing the amount of the main peanut allergen, Ara h 1 (25).
Global warming and air pollution are closely linked with inflammation in the upper and lower airways, exacerbating allergic airway diseases (12, 18). The association between exposure to air pollutants early in life and asthma is well-established (19–21). A recent study has suggested that almost 11% of childhood asthma cases are preventable if the recommended precautionary measures are taken (22, 23). It has been proposed that thunderstorms, due to heavy rain, wind gusts, static electricity, and lightning strikes might cause the pollen grains to be ruptured by osmotic shock and form small-sized allergenic particles that can penetrate deep into the lower airways, thereby triggering asthma attacks (3, 25, 26). Recently, there have been multiple acute asthma attacks triggered by thunderstorms during pollen seasons in cities and regions around the world, disrupting the health system (27). Moreover, wildfire smoke induces epithelial barrier dysfunction, causing T cell skewing to T helper (Th) 2 cells, in turn inducing asthma exacerbations (28, 29). Albeit being larger in size, particles in dust storms enhance inflammatory responses and worsen respiratory symptoms (30).
With the effect of climate change, stratospheric ozone is decreasing and the more harmful UVB reaches the surface of the Earth, while increased cloud cover, dust and smoke reduce UV light penetration (31, 32). Ultraviolet (UV) light, cold and dry weather, and floods may aggravate atopic dermatitis (AD). Floods are thought to affect the exacerbations of childhood AD by increasing the levels of indoor molds and contaminated water (33). Moreover, increased concentrations of air pollutants and pollen counts aggravate the severity of AD symptoms (34, 35).
Epithelial Barrier Hypothesis
To date, several hypotheses have been proposed to explain the complex interplay between the immune system, allergens, environmental triggers, and epigenetics. Damage to the epithelial barriers of the skin, airways and gastrointestinal tract by exposure to harmful substances has been brought to light by the recently proposed “epithelial barrier hypothesis” (8). Our journey toward understanding the role of the epithelial barrier in allergic diseases began in 1998 with the first demonstration of keratinocyte apoptosis induced by activated T cells in the pathogenesis of atopic dermatitis (36), asthma and chronic rhinosinusitis (37, 38). In common, these diseases were characterized by the death of highly activated local tissue epithelial cells by apoptosis, which is an important finding in terms of the tissue responses in the physiopathology of these diseases. We demonstrated a chronic epithelial barrier defect, such as spongiosis in the skin, epithelial desquamation in asthma and sinusitis (36–38). Interestingly, highly activated epithelial cells were dying, thereby decreasing the local tissue inflammatory burden. The epithelial barrier was opening and local subepithelial inflammation was draining to the lumens of mucosal surfaces to reduce the epithelial barrier burden. Similarly, dermal inflammation was being drained away from the skin due to spongiotic morphology. Transepidermal water passage, the so-called transepidermal water loss (TEWL), increased in eczema lesions of AD, which contained all the inflammatory cells, cytokines, and chemokines. All these events were decreasing the epithelial inflammatory burden leading to the chronicity of the diseases with a continuous exacerbation and healing process. The opening of the epithelial barrier as an extrusion mechanism also allows for the concomitant entry of allergens, microbes, and toxic substances into deeper tissues (8, 39).
Keep away, wash away, and suppress constitute the main barrier functions of the mucosal tissue, which include multiple immune and tissue cell-related mechanisms (39). First, keep away factors are related to allergen ignorance: increased basement membrane (lamina reticularis) thickness that acts as a physical barrier between allergens and the immune system cells (40), mucosal secretory IgA production against allergens (41), and mucus production in physiological quantities (42). The wash away function involves the clearance of inflammatory cells and cytokines. Mechanisms include opening of the epithelial barriers and clearance of airway tissue inflammatory cells by migration cells, debris and proinflammatory cytokines and chemokines toward the lumen (43), and induction of bronchial epithelial cell apoptosis and their shedding (44). The third function is immune suppression, which includes cells and cytokines that are related to the suppression of inflammation, such as the generation of regulatory dendritic cells (DCs), Treg and Breg cells and their suppressor cytokines (45).
A defective epithelial barrier and the dysregulated interaction between the epithelium, immune cells and microbiota is implicated in the development of multiple allergic conditions (46, 47). The epithelial barrier plays a critical role in maintaining homeostasis through various physiological functions and it acts as the first-line of physical defense and immune response. A leaky epithelium results in dysbiosis of microbial contents which are translocated into interepithelial and subepithelial compartments inducing local tissue inflammation (8). IL-25, IL-33 and thymic stromal lymphopoietin (TSLP) secreted by epithelial cells in response to various stimuli activate local dendritic cells, group 2 innate lymphoid cells and T cells and cause a skew in immune response to Th2 type (48). Th2 cells and their cytokines IL-4 and IL-13, and ILC2s, disrupt epithelial barrier integrity through IL-13 (49, 50).
Both genetic and environmental factors are implicated in the epithelial barriers function. The epidermal barrier in the skin consists of the stratum corneum and TJs. Decreased expression of TJ proteins, such as claudins and occludins, and changes in the composition and dysfunction of lipids (ceramides, free fatty acids and cholesterol) and structural proteins (filaggrin), high skin pH and loss of microbiome diversity may contribute to the epidermal barrier defect observed in patients with atopic dermatitis (51, 52). Loss-of-function mutations in filaggrin also pose risks for the development of food allergy, AR, and asthma. The development of sensitivity to various foods in infants with severe atopic dermatitis, prior to introduction of complementary foods, indicates that this may be associated with an inflamed and impaired epithelial barrier (53).
Damage of the epithelial barrier integrity by toxic substances initiates a cascade of events that includes changes in the microbial composition and colonization of opportunistic pathogens (Figure 2). A continuous expulsion response develops against microbiota translocating below the epithelium such as the expulsion response to parasites in Loffler's pneumonia (8, 54), which results in microbial dysbiosis and decreased biodiversity. Airway epithelial cells not only form a physical barrier against inhaled particles, but also recruit and activate other effector cells by producing antimicrobial peptides, chemokines, and cytokines to scavenge pathogenic insults (55). Studies have shown that the expression of TJ proteins such as claudin-1, occludin and ZO-1 is significantly reduced in patients with asthma and upper respiratory diseases such as nasal polyps and chronic rhinosinusitis (56, 57). Claudin-18 expression, a TJ protein required for maintaining the integrity of the airway epithelial permeability, was also lower in asthmatics compared to healthy controls (58, 59).
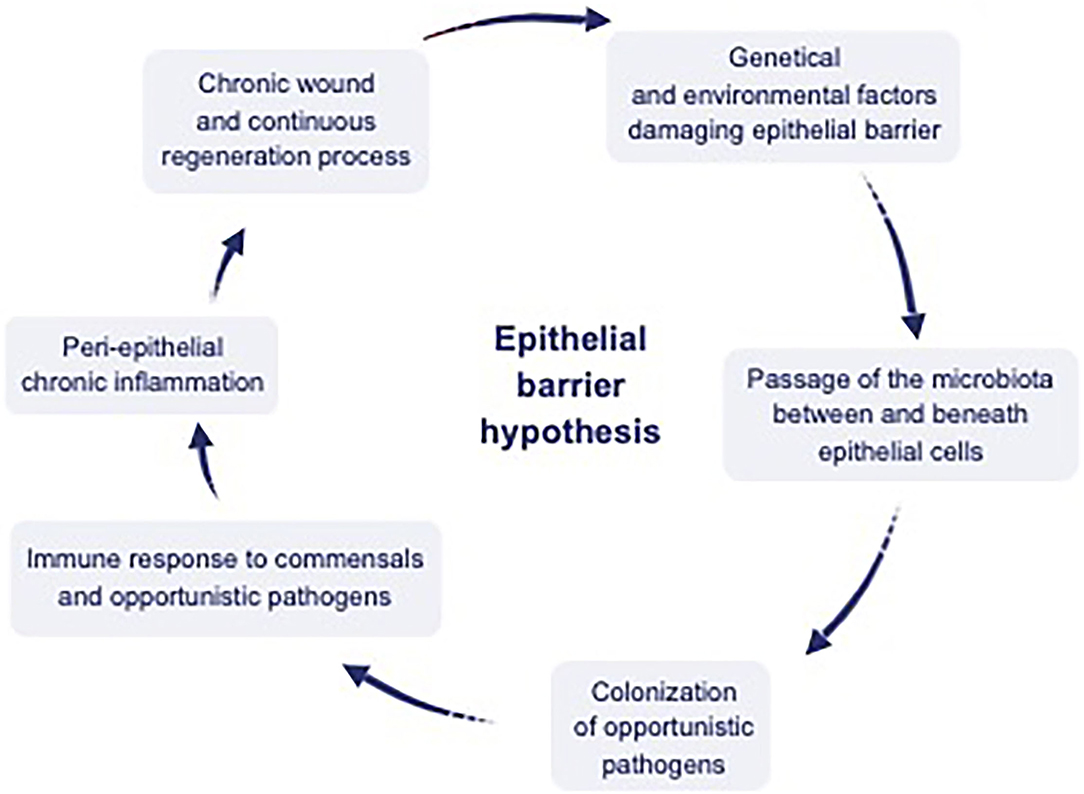
Figure 2. Interaction between environmental factors, epithelium, microbiota, and the immune system. Dysregulation of the epithelial barrier by genetic and environmental factors has been hypothesized to cause a leaky epithelium, which causes dysbiosis of microbial content, including commensals and opportunistic pathogens. Translocation of this content to interepithelial and subepithelial compartments and colonization of the opportunistic pathogens inducing peri-epithelial chronic inflammation. Finally chronic wound and continuous regeneration process ensues [adapted from reference (10)].
The gastrointestinal epithelial barrier is comprised of desmosomes, adherence junctions and TJs. It represents the largest mucosal surface in contact with foreign antigens and toxins, acting as a selective barrier that absorbs and exchanges nutrients, water, and electrolytes (60). Oral intolerance to foods has been demonstrated to be associated with an impaired intestinal barrier function in various experimental models and clinical trials (61, 62).
Environmental Substances
There is a long list of toxic substances that directly damage the respiratory, skin, and gut epithelium. Over 200 000 chemicals have been introduced since the 1960s with insufficient data on their toxicity. Our studies have demonstrated that many of these substances damage the epithelial barriers in various tissues, such as those present in cigarette smoke, diesel exhaust, detergents and surfactants, cleaning products, microplastics, nanoparticles, enzymes and emulsifiers in processed food, as well as particulate matter and ozone (63–71). Along with these factors and climate changes, the increasing concentrations and allergenicity of a set of airborne pollens (i.e., oak, ragweed) also needs to be considered (13, 14).
Particulate matter (PM2.5 and PM10) are one of the most common pollutants, disrupt the epithelial barriers by degrading tight junction proteins (TJ) downregulating occludin and claudin-1, suppressing E-cadherin levels, decreasing transepithelial electrical resistance and increasing paracellular permeability (65, 72). They also disrupt structural proteins such as cytokeratin, filaggrin and cause an increase in lysosomal membrane permeability, lipid peroxidation, and FOXP3 methylation (73). Black carbon produced by incomplete combustion of fuel is an important component of atmospheric PM. Many studies have shown a relationship between exposure to black carbon and the risk of developing asthma (74, 75). In addition, exposure to black carbon has been shown to trigger the development of AR by increasing oxidative stress and inducing the expression of interleukin IL-1β in human nasal epithelial cells (76, 77).
An important traffic-related pollutant, NO2, can deeply penetrate and disrupt the epithelial barriers of the upper and lower airways and increase the risk of developing respiratory diseases (74, 75). As another air pollutant, nanoparticles pass the alveolocapillary membrane and can enter the systemic circulation. They directly stimulate epithelial cells, macrophages, and fibroblasts to secrete proinflammatory and profibrotic mediators. Various nanoparticles induce overexpression of immature neurotrophins, destroy phospholipid membranes, endothelial cell junctions and even lysosomal membranes by interacting with lipid-rich structures resulting in epithelial cell death (76). They also disrupt the integrity of the skin and intestinal epithelial barrier with cytotoxic effects by altering cell junctions, inducing proinflammatory cytokines and causing mitochondrial and lysosomal dysfunction (77).
Exposure to ozone can aggravate respiratory diseases by passing through the lower respiratory tract and even to the capillary endothelium, leading to cell stress, desquamation, and cell death by oxidative damage via reactive oxygen species (66, 71, 78). In chronic exposure, ozone induces collagen deposition in epithelial and subepithelial areas causing peribronchial fibrosis (78).
There are extensive in-vivo and ex-vivo studies demonstrating the health hazards of tobacco smoking and e-cigarettes. The toxic chemical substances present induce rapid lipid peroxidation, airway epithelial cell death, impairment of macrophage functions, alter bronchial epithelial cell cytokine secretion patterns and disrupt bronchial epithelial barrier integrity (79, 80).
Direct contact or inhalation of detergents disrupt the epithelial barriers of the skin and respiratory tract, even at very high dilutions or in contact with residues remaining on the laundry after rinsing (7). Surfactants damage the epithelial barrier integrity directly by insulting TJs and related molecules (63, 64) facilitating transepidermal water loss, decreasing stratum corneum hydration and inducing Th2 inflammation (63, 81). Dietary emulsifiers have been demonstrated to alter the microbiota composition in the gut promoting bacteria with an inflammatory potential and facilitates bacterial penetration though the intestinal mucus layer (82).
Microplastics and nanoplastics derived from petroleum can easily infiltrate tissues and interact with cellular structural molecules (83). Microplastics were first solely considered as a pollutant of the oceans. There is increasing evidence indicating high levels of daily human exposure to micro- and nano-plastics through inhalation and ingestion. Once in our respiratory system, nanoplastics can modify the cell lipid membrane structure and secondary structures of proteins, up-regulate pro-inflammatory cytokines, alter the expression of cell cycle-associated proteins, induce inflammatory gene transcription and apoptosis (84).
Overall, particulate air pollutants specially damage the respiratory tract epithelium but also disrupt the skin and gastrointestinal barriers triggering various allergic and inflammatory diseases. Finally, protease activities of airborne allergens such as molds, pollens, cockroaches, and house dust mites have detrimental effects on the TJ molecules in the airway epithelium. In addition, food cysteine proteases also impair the intestinal barrier and increase intestinal permeability (85). Disruption of epithelial barrier integrity facilitates the presentation of allergens and initiates type 2 inflammation (86–88).
Microbial Dysbiosis
After the development of peri-epithelial inflammation, microbial dysbiosis and decreased biodiversity in the affected tissues are taking place. This has been predominantly attributed to the colonization of opportunistic pathogens in leaky barrier tissues and the mounted immune response against these pathogens. Fundamental changes in modern environments, hygiene and lifestyles reduce microbial biodiversity, leading to more unstable and less resilient microbiota (89). This condition, known as dysbiosis, alters the balance maintained by the gut, skin, and respiratory microbiomes, impairs immune homeostasis and is implicated in many chronic inflammatory diseases, including asthma, allergic diseases such as allergic rhinitis, AD, and food allergy (8, 89–92).
Early exposure to protective commensals contributes to the development of tolerogenic responses (93, 94). The fetal immune system is being influenced and shaped during intrauterine and postnatal periods by maternal infections, microbiota, diet, antibiotics, mode of delivery, breastfeeding, and environmental exposures. These factors may alter the development of lung immunity associated with dysbiosis, contributing to the development of asthma (89, 90, 95). Continuous contact with farm animals increases indoor endotoxin concentrations and this increased exposure promotes an immune regulatory stimulus (96). In addition, microbiota-host interactions have been suggested to play a role in the development of chronic lung diseases. Gut-derived microbiota produce metabolites that reach the lungs (gut-lung axis) and shift the Th2, Treg (Th2-Treg) balance toward Tregs, thereby protecting the host from the development of asthma (89). In a study following 10 years of atopic sensitization and allergic diseases in 180 children aged 7–11 years from Finland and Russia Karelia (both with similar climates, the former is a more Westernized region, the second is a non-Westernized region), Finnish Karelian children were found to be 10 times more likely to develop allergies. Additionally, the bacterial and fungal populations in the nasal mucosa of these participants were less and less diverse than their Russian counterparts. In conclusion, it was stated in this study that exposure to environmental microbiota at an early age may be biologically related to allergic symptoms at younger ages (97). In a different study of young adults in the Karelian region, the number of allergic individuals was found to be lower on the Russian side compared to the Finnish side, which could not be explained by the genotypic difference. This difference was found to be associated with the diversity in the skin and nasal epithelial microbiota of the Russian side and the suppression of the innate immunity by the abundance of acinetobacter on their skin (98, 99)).
Skin microbiome composition and diversity differs between patients with eczema and healthy individuals. There is a decrease in numbers of commensal bacteria in atopic skin, and increased pathogen density in patients with AD is positively correlated with the severity of skin lesions and disease (100). Moreover, intestinal bacterial dysbiosis affects the skin microbiota via the gut-skin axis and leads to a systemic imbalance in the Th2-Treg lymphocyte ratio (101).
The gut microbiome is continuously reshaped by external factors such as diet and dysbiosis of the gut microbiome has been associated with the development of food allergy (102). Poor microbiota richness is associated with increased food sensitivity in infants up to 1-year-old (103). Diversity and different taxa were also found to be higher in early life gut microbiota of children with egg allergy (104). This supports the role of specific microbiota in food allergies, opening an avenue for microbiota-targeted therapies.
Dietary Preferences
There is mounting evidence suggesting that the transition from a traditional diet to a Western diet and increased ratio of n-6/n-3 polyunsaturated fatty acid consumption are associated with the development of allergic diseases. Arachidonic acid (AA), one of the n-6 fatty acid metabolites, aggravates allergic responses through eicosanoids (thromboxane A2, prostaglandin E2 and leukotriene B4) (105). On the other hand, n-3 fatty acids compete with AA and prevent the formation of inflammatory agents through resolvins (especially resolvin E1) (106). Increased consumption of fast food and n-6 fatty acid-derived vegetable oils are the primary dietary insults associated with allergic diseases (107). Although there are limited and some conflicting data, consumption of processed/fast food has been reported to increase the risk of AD (108). A high n-6/n-3 fatty acid ratio induces asthma by causing airway inflammation and bronchoconstriction (109). Contrary to n-6 fatty acids, n-3 fatty acids mainly exert a protective effect on allergic diseases by reducing the inflammatory response, mostly through pro-resolving mediators (110). For example, n-3 fatty acid-derived Protectin D1, generated from docosahexaenoic acid in asthma, ameliorates airway inflammation (111). Administration of n-3 fatty acid supplementation has been found to reduce disease severity in patients with AD (112). In contrast, a different study did not find an association between n-3 fatty acid intake and AD (113). The effect of n-3 fatty acids on food allergy during pregnancy and/or lactation is contradictory. However, n-3 fatty acid supplementation in early pregnancy has been shown to reduce allergic sensitivity to food proteins in the infants (114). In addition, high fecal butyrate levels were associated with a reduced risk of atopic sensitization and the development of asthma and food allergy (115). Moreover, butyrate and propionate have been shown to restore epithelial barrier functions in eosinophilic esophagitis (116).
Oxidative stress is well-recognized to contribute to the pathophysiology of allergic diseases (117). Systemic oxidative stress can aggravate inflammatory responses. Dietary antioxidants and vitamin supplementation have been suggested for reducing the incidence or morbidity of allergic diseases (118). Processed foods contain low levels of antioxidants such as vitamin A, vitamin C and vitamin E and have been suggested to increase the susceptibility to allergic diseases (119). The increase in reactive oxygen species due to insufficient intake of antioxidant vitamins makes individuals susceptible to asthma, AR and AD (120–122). Contrary to these results, there are also studies that don't show any beneficial effects of vitamin supplementation in allergic diseases (123, 124). A diet high in fruits and vegetables, which are good sources of vitamins A, C and E, during lactation and pregnancy and in the infant's diet are protective against the development of food allergy (125). In contrast, high-sugar and fat diets and diet-induced low fecal SCFA levels have been associated with the development of FA (126).
There has been recent controversy on the allergenicity of Genetically Modified (GM) products (127, 128). Some studies have suggested that transgenic crops may have allergic effects, but some do not (129, 130). Long-term studies are warranted to evaluate the risks of consumption of GM foods on the development of food allergies.
In recent years, artificial sweeteners and food additives used to improve taste, color and stability of food products have been increasingly consumed and this may be associated with and increased risk of food allergy, AD, childhood asthma and AR (129–132).
Conclusion
The external exposome concept is a collective model that not only considers all exposures but also takes into account all of the proven or potential interactions between these environmental factors. Data obtained shows that the rapid increase in the prevalence of allergic diseases and other immune-mediated conditions can be explained by the concept of external exposomes. However, there are gaps in our scientific knowledge on this subject and these need to be answered. For now, it is imperative that we raise awareness of the effects of environmental changes on health to motivate people that will influence governmental policies, for our own well-being and that of future generations.
Author Contributions
ZC, DM, and CA conceptualized the scope of the article, decided the headings and subheadings and distributed the tasks among all authors. BO and ZC contributed to the part of environmental substances. PC and SA contributed the part of the effects of climate change. MT and IY contributed the part of microbiome. BG and ÜÖ contributed the part of the nutritional factors. CO contributed the part of the epithelial barrier hypothesis. ZC reviewed and combined all parts. ZC, MA, CO, DM, and CA reviewed the article and figures, contributed different sections, and harmonized the final version. All authors contributed to the article and approved the submitted version.
Conflict of Interest
The authors declare that the research was conducted in the absence of any commercial or financial relationships that could be construed as a potential conflict of interest.
Publisher's Note
All claims expressed in this article are solely those of the authors and do not necessarily represent those of their affiliated organizations, or those of the publisher, the editors and the reviewers. Any product that may be evaluated in this article, or claim that may be made by its manufacturer, is not guaranteed or endorsed by the publisher.
References
1. Vrijheid M. The exposome: a new paradigm to study the impact of environment on health. Thorax. (2014) 69:876–8. doi: 10.1136/thoraxjnl-2013-204949
2. Agache I, Miller R, Gern JE, Hellings PW, Jutel M, Muraro A, et al. Emerging concepts and challenges in implementing the exposome paradigm in allergic diseases and asthma: a practall document. Allergy. (2019) 74:449–63. doi: 10.1111/all.13690
3. Cecchi L, D'Amato G, Annesi-Maesano I. External exposome and allergic respiratory and skin diseases. J Allergy Clin Immunol. (2018) 141:846–57. doi: 10.1016/j.jaci.2018.01.016
4. Renz H, Holt PG, Inouye M, Logan AC, Prescott SL, Sly PD. An exposome perspective: early-life events and immune development in a changing world. J Allergy Clin Immunol. (2017) 140:24–40. doi: 10.1016/j.jaci.2017.05.015
6. Cecchi L, D'Amato G, Annesi-Maesano I. Climate change and outdoor aeroallergens related to allergy and asthma: taking the exposome into account. Allergy. (2020) 75:2361–3.
7. Sözener ZC, Cevhertas L, Nadeau K, Akdis M, Akdis CA. Environmental factors in epithelial barrier dysfunction. J Allergy Clin Immunol. (2020) 145:1517–28.
8. Akdis CA. Does the epithelial barrier hypothesis explain the increase in allergy, autoimmunity and other chronic conditions? Nat Rev Immunol. (2021) 21:739–51.
9. Eguiluz-Gracia I, Mathioudakis AG, Bartel S, Vijverberg SJ, Fuertes E, Comberiati P, et al. The need for clean air: the way air pollution and climate change affect allergic rhinitis and asthma. Allergy. (2020) 75:2170–84. doi: 10.1111/all.14177
10. D'Amato G, Akdis CA. Global warming, climate change, air pollution and allergies. Allergy. (2020) 75:2158–60. doi: 10.1111/all.14527
11. Gao YD, Ding M, Dong X, Zhang JJ, Kursat Azkur A, Azkur D, et al. Risk factors for severe and critically ill Covid-19 patients: a review. Allergy. (2021) 76:428–55. doi: 10.1111/all.14657
12. D'Amato G, Chong-Neto HJ, Monge Ortega OP, Vitale C, Ansotegui I, Rosario N, et al. The effects of climate change on respiratory allergy and asthma induced by pollen and mold allergens. Allergy. (2020) 75:2219–28. doi: 10.1111/all.14476
13. Ziska LH, Makra L, Harry SK, Bruffaerts N, Hendrickx M, Coates F, et al. Temperature-related changes in airborne allergenic pollen abundance and seasonality across the northern hemisphere: a retrospective data analysis. Lancet Planet Health. (2019) 3:e124–31. doi: 10.1016/S2542-5196(19)30015-4
14. Zhao F, Elkelish A, Durner J, Lindermayr C, Winkler JB, Ruëff F, et al. Common ragweed (Ambrosia Artemisiifolia L): allergenicity and molecular characterization of pollen after plant exposure to elevated No2. Plant Cell Environ. (2016) 39:147–64. doi: 10.1111/pce.12601
15. Paudel B, Chu T, Chen M, Sampath V, Prunicki M, Nadeau KC. Increased duration of pollen and mold exposure are linked to climate change. Sci Rep. (2021) 11:12816. doi: 10.1038/s41598-021-92178-z
16. Acevedo N, Zakzuk J, Caraballo L. House dust mite allergy under changing environments. Allergy Asthma Immunol Res. (2019) 11:450–69. doi: 10.4168/aair.2019.11.4.450
17. Van Cleemput J, Poelaert KCK, Laval K, Impens F, Van den Broeck W, Gevaert K, et al. Pollens destroy respiratory epithelial cell anchors and drive alphaherpesvirus infection. Sci Rep. (2019) 9:4787. doi: 10.1038/s41598-019-41305-y
18. Smith AM, Ramirez RM, Harper N, Jimenez F, Branum AP, Meunier JA, et al. Large-Scale provocation studies identify maladaptive responses to ubiquitous aeroallergens as a correlate of severe allergic rhinoconjunctivitis and asthma. Allergy. (2021). doi: 10.1111/all.15124
19. Bowatte G, Lodge C, Lowe AJ, Erbas B, Perret J, Abramson MJ, et al. The influence of childhood traffic-related air pollution exposure on asthma, allergy and sensitization: a systematic review and a meta-analysis of birth cohort studies. Allergy. (2015) 70:245–56. doi: 10.1111/all.12561
20. Jacquemin B, Siroux V, Sanchez M, Carsin AE, Schikowski T, Adam M, et al. Ambient air pollution and adult asthma incidence in six European cohorts (Escape). Environ Health Perspect. (2015) 123:613–21. doi: 10.1289/ehp.1408206
21. Rice MB, Rifas-Shiman SL, Litonjua AA, Gillman MW, Liebman N, Kloog I, et al. Lifetime air pollution exposure and asthma in a pediatric birth cohort. J Allergy Clin Immunol. (2018) 141:1932–4 e7. doi: 10.1016/j.jaci.2017.11.062
22. Khreis H, Cirach M, Mueller N, de Hoogh K, Hoek G, Nieuwenhuijsen MJ, et al. Outdoor air pollution and the burden of childhood asthma across Europe. Eur Respir J. (2019) 54:1802194. doi: 10.1183/13993003.02194-2018
23. Organization WH. Who Global Air Quality Guidelines: Particulate Matter (Pm2. 5 and Pm10), Ozone, Nitrogen Dioxide, Sulfur Dioxide and Carbon Monoxide: Executive Summary. Organization WH (2021).
24. Fiorito S, Soligo M, Gao Y, Ogulur I, Akdis CA, Bonini S. Is epithelial barrier hypothesis the key to understanding the higher incidence and excess mortality during Covid-19 Pandemic? the case of northern Italy. Allergy. (2022) 77:1408–17. doi: 10.1111/all.15239
25. Senechal H, Visez N, Charpin D, Shahali Y, Peltre G, Biolley JP, et al. A review of the effects of major atmospheric pollutants on pollen Grains, Pollen Content, and Allergenicity. Sci World J. (2015) 2015:940243. doi: 10.1155/2015/940243
26. D'Amato G, Annesi-Maesano I, Cecchi L, D'Amato M. Latest news on relationship between thunderstorms and respiratory allergy, severe asthma, and deaths for asthma. Allergy. (2019) 74:9–11. doi: 10.1111/all.13616
27. Hew M, Lee J, Susanto NH, Prasad S, Bardin PG, Barnes S, et al. The 2016 melbourne thunderstorm asthma epidemic: risk factors for severe attacks requiring hospital admission. Allergy. (2019) 74:122–30. doi: 10.1111/all.13609
28. Prunicki M, Kelsey R, Lee J, Zhou X, Smith E, Haddad F, et al. The impact of prescribed fire vs. wildfire on the immune and cardiovascular systems of children. Allergy. (2019) 74:1989–91. doi: 10.1111/all.13825
29. Arriagada NB, Horsley JA, Palmer AJ, Morgan GG, Tham R, Johnston FH. Association between fire smoke fine particulate matter and asthma-related outcomes: systematic review and meta-analysis. Environ res. (2019) 179:108777. doi: 10.1016/j.envres.2019.108777
30. Lorentzou C, Kouvarakis G, Kozyrakis GV, Kampanis NA, Trahanatzi I, Fraidakis O, et al. Extreme desert dust storms and copd morbidity on the Island of crete. Int J Chron Obstruct Pulmon Dis. (2019) 14:1763–8. doi: 10.2147/COPD.S208108
31. Lieberman JA, Gupta RS, Knibb RC, Haselkorn T, Tilles S, Mack DP, et al. The global burden of illness of peanut allergy: a comprehensive literature review. Allergy. (2021) 76:1367–84. doi: 10.1111/all.14666
32. Beggs PJ, Walczyk NE. Impacts of climate change on plant food allergens: a previously unrecognized threat to human health. Air Quality, Atmosphere Health. (2008) 1:119–23.
33. Chen NT, Chen MJ, Wu CD, Guo YL. Emergency room visits for childhood atopic dermatitis are associated with floods? Sci Total Environ. (2021) 773:145435. doi: 10.1016/j.scitotenv.2021.145435
34. Patella V, Florio G, Palmieri M, Bousquet J, Tonacci A, Giuliano A, et al. Atopic dermatitis severity during exposure to air pollutants and weather changes with an artificial neural network (Ann) analysis. Pediatr Allergy Immunol. (2020) 31:938–45. doi: 10.1111/pai.13314
35. Bais AF, Lucas RM, Bornman JF, Williamson CE, Sulzberger B, Austin AT, et al. Environmental effects of ozone depletion, Uv radiation and interactions with climate change: unep environmental effects assessment panel, update 2017. Photochem Photobiol Sci. (2018) 17:127–79. doi: 10.1039/c7pp90043k
36. Trautmann A, Akdis M, Kleemann D, Altznauer F, Simon H-U, Graeve T, et al. T cell–mediated fas-induced keratinocyte apoptosis plays a key pathogenetic role in eczematous dermatitis. J Clin Invest. (2000) 106:25–35. doi: 10.1172/JCI9199
37. Basinski TM, Holzmann D, Eiwegger T, Zimmermann M, Klunker S, Meyer N, et al. Dual nature of T cell–epithelium interaction in chronic rhinosinusitis. J Allergy Clin Immunol. (2009) 124:74–80. e8. doi: 10.1016/j.jaci.2009.04.019
38. Solarewicz-Madejek K, Basinski T, Crameri R, Akdis M, Akkaya A, Blaser K, et al. T cells and eosinophils in bronchial smooth muscle cell death in asthma. Clin Exp Allergy. (2009) 39:845–55. doi: 10.1111/j.1365-2222.2009.03244.x
39. Akdis CA. Allergy and hypersensitivity: mechanisms of allergic disease. Curr Opin Immunol. (2006) 18:718–26. doi: 10.1016/j.coi.2006.09.016
40. Laitinen L, Laitinen A, Haahtela T. Airway mucosal inflammation even in patients with newly diagnosed asthma. Am Rev Respir Dis. (1993) 147:697–704. doi: 10.1164/ajrccm/147.3.697
41. Böttcher M, Häggström P, Björkstén B, Jenmalm M. Total and allergen-specific immunoglobulin a levels in saliva in relation to the development of allergy in infants up to 2 years of age. Clin Exp Allergy. (2002) 32:1293–8. doi: 10.1046/j.1365-2222.2002.01470.x
42. McMillan SJ, Xanthou G, Lloyd CM. Manipulation of allergen-induced airway remodeling by treatment with Anti-Tgf-B antibody: effect on the smad signaling pathway. J Immunol. (2005) 174:5774–80. doi: 10.4049/jimmunol.174.9.5774
43. Erjefält J, Uller L, Malm-Erjefält M, Persson C. Rapid and efficient clearance of airway tissue granulocytes through transepithelial migration. Thorax. (2004) 59:136–43.
44. Trautmann A, Krüger K, Akdis M, Müller-Wening D, Akkaya A, Bröcker E-B, et al. Apoptosis and loss of adhesion of bronchial epithelial cells in asthma. Int Arch Allergy Immunol. (2005) 138:142–50. doi: 10.1159/000088436
45. Akdis CA, Akdis M. Advances in allergen immunotherapy: aiming for complete tolerance to allergens. Sci Trans Med. (2015) 7:280ps6. doi: 10.1126/scitranslmed.aaa739
46. Sugita K, Soyka MB, Wawrzyniak P, Rinaldi AO, Mitamura Y, Akdis M, et al. Outside-in hypothesis revisited: the role of microbial, epithelial, and immune interactions. Ann Allergy Asthma Immunol. (2020) 125:517–27. doi: 10.1016/j.anai.2020.05.016
47. Simon D, Page B, Vogel M, Bussmann C, Blanchard C, Straumann A, et al. Evidence of an abnormal epithelial barrier in active, untreated and corticosteroid-treated eosinophilic esophagitis. Allergy. (2018) 73:239–47. doi: 10.1111/all.13244
48. Akdis CA, Arkwright PD, Bruggen MC, Busse W, Gadina M, Guttman-Yassky E, et al. Type 2 Immunity in the skin and lungs. Allergy. (2020) 75:1582–605. doi: 10.1111/all.14318
49. Sugita K, Steer CA, Martinez-Gonzalez I, Altunbulakli C, Morita H, Castro-Giner F, et al. Type 2 innate lymphoid cells disrupt bronchial epithelial barrier integrity by targeting tight junctions through Il-13 in asthmatic patients. J Allergy Clin Immunol. (2018) 141:300–10. e11. doi: 10.1016/j.jaci.2017.02.038
50. Wawrzyniak P, Wawrzyniak M, Wanke K, Sokolowska M, Bendelja K, Ruckert B, et al. Regulation of bronchial epithelial barrier integrity by type 2 cytokines and histone deacetylases in asthmatic patients. J Allergy Clin Immunol. (2017) 139:93–103. doi: 10.1016/j.jaci.2016.03.050
51. Hon KL, Leung AK, Barankin B. Barrier repair therapy in atopic dermatitis: an overview. Am J Clin Dermatol. (2013) 14:389–99. doi: 10.1007/s40257-013-0033-9
52. Rinaldi AO, Korsfeldt A, Ward S, Burla D, Dreher A, Gautschi M, et al. Electrical impedance spectroscopy for the characterization of skin barrier in atopic dermatitis. Allergy. (2021) 76:3066–79. doi: 10.1111/all.14842
53. Nowak-Wegrzyn A, Chatchatee P. Mechanisms of tolerance induction. Ann Nutr Metab. (2017) 70 (Suppl. 2):7–24. doi: 10.1159/000457915
55. Heijink IH, Kuchibhotla VN, Roffel MP, Maes T, Knight DA, Sayers I, et al. Epithelial cell dysfunction, a major driver of asthma development. Allergy. (2020) 75:1902–17. doi: 10.1111/all.14421
56. Soyka MB, Wawrzyniak P, Eiwegger T, Holzmann D, Treis A, Wanke K, et al. Defective epithelial barrier in chronic rhinosinusitis: the regulation of tight junctions by Ifn-Gamma and Il-4. J Allergy Clin Immunol. (2012) 130:1087–96 e10. doi: 10.1016/j.jaci.2012.05.052
57. Velasco E, Delicado-Miralles M, Hellings PW, Gallar J, Van Gerven L, Talavera K. Epithelial and Sensory Mechanisms of Nasal Hyperreactivity. Allergy.
58. Sweerus K, Lachowicz-Scroggins M, Gordon E, LaFemina M, Huang X, Parikh M, et al. Claudin-18 deficiency is associated with airway epithelial barrier dysfunction and asthma. J Allergy Clin Immunol. (2017) 139:72–81. e1. doi: 10.1016/j.jaci.2016.02.035
59. Zhou J., Zhou X-d, Xu R, Du X-z, Li Q, Li B, et al. The degradation of airway epithelial tight junctions in asthma under high airway pressure is probably mediated by Piezo-1 Front Physiol. (2021) 12:427. doi: 10.3389/fphys.2021.637790 eCollection 2021
60. Groschwitz KR, Hogan SP. Intestinal barrier function: molecular regulation and disease pathogenesis. J Allergy Clin Immunol. (2009) 124:3–20. doi: 10.1016/j.jaci.2009.05.038
61. Samadi N, Klems M, Untersmayr E. The role of gastrointestinal permeability in food allergy. Ann Allergy Asthma Immunol. (2018) 121:168–73. doi: 10.1016/j.anai.2018.05.010
62. Dupont C, Barau E, Molkhou P, Raynaud F, Barbet JP, Dehennin L. Food-induced alterations of intestinal permeability in children with cow's milk-sensitive enteropathy and atopic dermatitis. J Pediatr Gastroenterol Nutr. (1989) 8:459–65. doi: 10.1097/00005176-198905000-00007
63. Wang M, Tan G, Eljaszewicz A, Meng Y, Wawrzyniak P, Acharya S, et al. Laundry detergents and detergent residue after rinsing directly disrupt tight junction barrier integrity in human bronchial epithelial cells. J Allergy Clin Immunol. (2019) 143:1892–903. doi: 10.1016/j.jaci.2018.11.016
64. Xian M, Wawrzyniak P, Ruckert B, Duan S, Meng Y, Sokolowska M, et al. Anionic surfactants and commercial detergents decrease tight junction barrier integrity in human keratinocytes. J Allergy Clin Immunol. (2016) 138:890–3 e9. doi: 10.1016/j.jaci.2016.07.003
65. Xian M, Ma S, Wang K, Lou H, Wang Y, Zhang L., et al. Particulate matter 25 causes deficiency in barrier integrity in human nasal epithelial cells. Allergy Asthma Immunol Res. (2020) 12:56–71. doi: 10.4168/aair.2020.12.1.56
66. Michaudel C, Mackowiak C, Maillet I, Fauconnier L, Akdis CA, Sokolowska M, et al. Ozone exposure induces respiratory barrier biphasic injury and inflammation controlled by Il-33. J Allergy Clin Immunol. (2018) 142:942–58. doi: 10.1016/j.jaci.2017.11.044
67. Aghapour M, Raee P, Moghaddam SJ, Hiemstra PS, Heijink IH. Airway epithelial barrier dysfunction in chronic obstructive pulmonary disease: role of cigarette smoke exposure. Am J Respir Cell Mol Biol. (2018) 58:157–69.
68. Caraballo JC, Yshii C, Westphal W, Moninger T, Comellas AP. Ambient particulate matter affects occludin distribution and increases alveolar transepithelial electrical conductance. Respirology. (2011) 16:340–9. doi: 10.1111/j.1440-1843.2010.01910.x
69. Vita AA, Royse EA, Pullen NA. Nanoparticles and danger signals: oral delivery vehicles as potential disruptors of intestinal barrier homeostasis. J Leukoc Biol. (2019) 106:95–103. doi: 10.1002/JLB.3MIR1118-414RR
70. Jin Y, Lu L, Tu W, Luo T, Fu Z. Impacts of polystyrene microplastic on the gut barrier, microbiota and metabolism of mice. Sci Total Environ. (2019) 649:308–17. doi: 10.1016/j.scitotenv.2018.08.353
71. Sokolowska M, Quesniaux VFJ, Akdis CA, Chung KF, Ryffel B, Togbe D. Acute respiratory barrier disruption by ozone exposure in mice. Front Immunol. (2019) 10:2169. doi: 10.3389/fimmu.2019.02169
72. Chuang HC, Ho KF, Cao JJ, Chuang KJ, Ho SS, Feng PH, et al. Effects of non-protein-type amino acids of fine particulate matter on E-cadherin and inflammatory responses in mice. Toxicol Lett. (2015) 237:174–80. doi: 10.1016/j.toxlet.2015.06.013
73. Prunicki M, Stell L, Dinakarpandian D, de Planell-Saguer M, Lucas RW, Hammond SK., et al. Exposure to No(2), Co, and Pm(25) is linked to regional DNA methylation differences in asthma. Clin Epigenetics. (2018) 10:2. doi: 10.1186/s13148-017-0433-4
74. Belanger K, Holford TR, Gent JF, Hill ME, Kezik JM, Leaderer BP. Household levels of nitrogen dioxide and pediatric asthma severity. Epidemiology. (2013) 24:320.
75. Carson JL, Collier AM, Hu S, Devlin RB. Effect of nitrogen dioxide on human nasal epithelium. Am J Respir Cell Mol Biol. (1993) 9:264–70. doi: 10.1165/ajrcmb/9.3.264
76. Urbancic I, Garvas M, Kokot B, Majaron H, Umek P, Cassidy H, et al. Nanoparticles can wrap epithelial cell membranes and relocate them across the epithelial cell layer. Nano Lett. (2018) 18:5294–305. doi: 10.1021/acs.nanolett.8b02291
77. Meng H, Leong W, Leong KW, Chen C, Zhao Y. Walking the line: the fate of nanomaterials at biological barriers. Biomaterials. (2018) 174:41–53. doi: 10.1016/j.biomaterials.2018.04.056
78. Michaudel C, Fauconnier L, Jule Y, Ryffel B. Functional and morphological differences of the lung upon acute and chronic ozone exposure in mice. Sci Rep. (2018) 8:10611. doi: 10.1038/s41598-018-28261-9
79. Ghosh B, Reyes-Caballero H, Akgun-Olmez SG, Nishida K, Chandrala L, Smirnova L, et al. Effect of sub-chronic exposure to cigarette smoke, electronic cigarette and waterpipe on human lung epithelial barrier function. BMC Pulm Med. (2020) 20:216. doi: 10.1186/s12890-020-01255-y
80. Ween MP, Hamon R, Macowan MG, Thredgold L, Reynolds PN, Hodge SJ. Effects of E-cigarette E-liquid components on bronchial epithelial cells: demonstration of dysfunctional efferocytosis. Respirology. (2020) 25:620–8. doi: 10.1111/resp.13696
81. Leoty-Okombi S, Gillaizeau F, Leuillet S, Douillard B, Fresne-Languille S, Carton T, et al. Effect of Sodium Lauryl Sulfate (Sls) applied as a patch on human skin physiology and its microbiota. Cosmetics. (2021) 8:6. doi: 10.3390/cosmetics8010006
82. Viennois E, Chassaing B. First victim, later aggressor: how the intestinal microbiota drives the pro-inflammatory effects of dietary emulsifiers? Gut Microbes. (2018) 9:1–4. doi: 10.1080/19490976.2017.1421885
83. Yee MS-L, Hii L-W, Looi CK, Lim W-M, Wong S-F, Kok Y-Y, et al. Impact of microplastics and nanoplastics on human health. Nanomaterials. (2021) 11:496. doi: 10.3390/nano11020496
84. Xu M, Halimu G, Zhang Q, Song Y, Fu X, Li Y, et al. Internalization and toxicity: a preliminary study of effects of nanoplastic particles on human lung epithelial cell. Sci Total Environ. (2019) 694:133794. doi: 10.1016/j.scitotenv.2019.133794
85. Grozdanovic MM, Cavić M, Nešić A, Andjelković U, Akbari P, Smit JJ, et al. Kiwifruit cysteine protease actinidin compromises the intestinal barrier by disrupting tight junctions. Biochim Biophys Acta. (2016) 1860:516–26. doi: 10.1016/j.bbagen.2015.12.005
86. Wan H, Winton HL, Soeller C, Tovey ER, Gruenert DC, Thompson PJ, et al. Der P 1 facilitates transepithelial allergen delivery by disruption of tight junctions. J Clin Invest. (1999) 104:123–33. doi: 10.1172/JCI5844
87. Namvar S, Warn P, Farnell E, Bromley M, Fraczek M, Bowyer P, et al. Aspergillus fumigatus proteases, Asp F 5 and Asp F 13, are essential for airway inflammation and remodelling in a murine inhalation model. Clin Exp Allergy. (2015) 45:982–93. doi: 10.1111/cea.12426
88. Vinhas R, Cortes L, Cardoso I, Mendes VM, Manadas B, Todo-Bom A, et al. Pollen proteases compromise the airway epithelial barrier through degradation of transmembrane adhesion proteins and lung bioactive peptides. Allergy. (2011) 66:1088–98. doi: 10.1111/j.1398-9995.2011.02598.x
89. Ruff WE, Greiling TM, Kriegel MA. Host-Microbiota interactions in immune-mediated diseases. Nat Rev Microbiol. (2020) 18:521–38. doi: 10.1038/s41579-020-0367-2
90. Sbihi H, Boutin RC, Cutler C, Suen M, Finlay BB, Turvey SE. Thinking bigger: how early-life environmental exposures shape the gut microbiome and influence the development of asthma and allergic Disease. Allergy. (2019) 74:2103–15. doi: 10.1111/all.13812
91. Lynch SV, Wood RA, Boushey H, Bacharier LB, Bloomberg GR, Kattan M, et al. Effects of early-life exposure to allergens and bacteria on recurrent wheeze and atopy in urban children. J Allergy Clin Immunol. (2014) 134:593–601 e12. doi: 10.1016/j.jaci.2014.04.018
92. Lunjani N, Satitsuksanoa P, Lukasik Z, Sokolowska M, Eiwegger T, O'Mahony L. Recent developments and highlights in mechanisms of allergic diseases: microbiome. Allergy. (2018) 73:2314–27. doi: 10.1111/all.13634
93. Naik S, Bouladoux N, Linehan JL, Han SJ, Harrison OJ, Wilhelm C, et al. Commensal-dendritic-cell interaction specifies a unique protective skin immune signature. Nature. (2015) 520:104–8. doi: 10.1038/nature14052
94. Linehan JL, Harrison OJ, Han SJ, Byrd AL, Vujkovic-Cvijin I, Villarino AV, et al. Non-Classical Immunity controls microbiota impact on skin immunity and tissue repair. Cell. (2018) 172:784–96 e18. doi: 10.1016/j.cell.2017.12.033
95. Siracusa A, De Blay F, Folletti I, Moscato G, Olivieri M, Quirce S, et al. Asthma and exposure to cleaning products–a European academy of allergy and clinical immunology task force consensus statement. Allergy. (2013) 68:1532–45. doi: 10.1111/all.12279
96. Waser M, Schierl R, von Mutius E, Maisch S, Carr D, Riedler J, et al. Determinants of endotoxin levels in living environments of farmers' children and their peers from rural areas. Clin Exp Allergy. (2004) 34:389–97. doi: 10.1111/j.1365-2222.2004.01873.x
97. Choi CH, Poroyko V, Watanabe S, Jiang D, Lane J., deTineo M, et al. Seasonal allergic rhinitis affects sinonasal microbiota. Am J Rhinol Allergy. (2014) 28:281–6. doi: 10.2500/ajra.2014.28.4050
98. Lee JJ, Kim SH, Lee MJ, Kim BK, Song WJ, Park HW, et al. Different upper airway microbiome and their functional genes associated with asthma in young adults and elderly individuals. Allergy. (2019) 74:709–19. doi: 10.1111/all.13608
99. Ruokolainen L, Paalanen L, Karkman A, Laatikainen T, von Hertzen L, Vlasoff T, et al. Significant disparities in allergy prevalence and microbiota between the young people in Finnish and Russian Karelia. Clin Exp Allergy. (2017) 47:665–74. doi: 10.1111/cea.12895
100. Paller AS, Kong HH, Seed P, Naik S, Scharschmidt TC, Gallo RL, et al. The Microbiome in patients with atopic dermatitis. J Allergy Clin Immunol. (2019) 143:26–35. doi: 10.1016/j.jaci.2018.11.015
101. Stefanovic N, Irvine AD, Flohr C. The role of the environment and exposome in atopic dermatitis. Curr Treat Options Allergy. (2021). 8:222–41. doi: 10.1007/s40521-021-00289-9
102. Savage JH, Lee-Sarwar KA, Sordillo J, Bunyavanich S, Zhou Y, O'Connor G, et al. A prospective microbiome-wide association study of food sensitization and food allergy in early childhood. Allergy. (2018) 73:145–52. doi: 10.1111/all.13232
103. Azad MB, Konya T, Guttman DS, Field CJ, Sears MR, HayGlass KT, et al. Infant gut microbiota and food sensitization: associations in the first year of life. Clin Exp Allergy. (2015) 45:632–43. doi: 10.1111/cea.12487
104. Fazlollahi M, Chun Y, Grishin A, Wood RA, Burks AW, Dawson P, et al. Early-life gut microbiome and egg allergy. Allergy. (2018) 73:1515–24. doi: 10.1111/all.13389
105. Julia V, Macia L, Dombrowicz D. The impact of diet on asthma and allergic diseases. Nat Rev Immunol. (2015) 15:308–22. doi: 10.1038/nri3830
106. Kytikova O, Novgorodtseva T, Denisenko Y, Antonyuk M, Gvozdenko T. Pro-Resolving lipid mediators in the pathophysiology of asthma. Medicina (Kaunas). (2019) 55:284. doi: 10.3390/medicina55060284
107. Adams S, Lopata AL, Smuts CM, Baatjies R, Jeebhay MF. Relationship between serum omega-3 fatty acid and asthma endpoints. Int J Environ Res. (2019) 16:43.
108. Park S, Bae J-H. Fermented food intake is associated with a reduced likelihood of atopic dermatitis in an adult population (Korean National Health and Nutrition Examination Survey 2012-2013). Nutr Res. (2016) 36:125–33. doi: 10.1016/j.nutres.2015.11.01
109. Hogenkamp A, Ehlers A, Garssen J, Willemsen LEM. Allergy modulation by N-3 long chain polyunsaturated fatty acids and fat soluble nutrients of the mediterranean diet. Front Pharmacol. (2020) 11:1244. doi: 10.3389/fphar.2020.01244
110. Maslova E, Rifas-Shiman SL, Oken E, Platts-Mills TAE, Gold DR. Fatty acids in pregnancy and risk of allergic sensitization and respiratory outcomes in childhood. Ann Allergy Asthma Immunol. (2019) 122:120–2 e3. doi: 10.1016/j.anai.2018.09.450
111. Levy BD, Kohli P, Gotlinger K, Haworth O, Hong S, Kazani S, et al. Protectin D1 is generated in asthma and dampens airway inflammation and hyperresponsiveness. J Immunol. (2007) 178:496–502. doi: 10.4049/jimmunol.178.1.496
112. Koch C, Dolle S, Metzger M, Rasche C, Jungclas H, Ruhl R, et al. Docosahexaenoic acid (Dha) supplementation in atopic eczema: a randomized, double-blind, controlled trial. Br J Dermatol. (2008) 158:786–92. doi: 10.1111/j.1365-2133.2007.08430.x
113. Søyland E, Funk J, Rajka G, Sandberg M, Thune P, Rustad L, et al. Dietary supplementation with very long-chain N-3 fatty acids in patients with atopic dermatitis. a double-blind, multicentre study. Br J Dermatol. (1994) 130:757–64. doi: 10.1111/j.1365-2133.1994.tb03414.x
114. Hoppenbrouwers T, Cvejic Hogervorst JH, Garssen J, Wichers HJ, Willemsen LEM. Long chain polyunsaturated fatty acids (Lcpufas) in the prevention of food allergy. Front Immunol. (2019) 10:1118. doi: 10.3389/fimmu.2019.01118
115. Roduit C, Frei R, Ferstl R, Loeliger S, Westermann P, Rhyner C, et al. High levels of butyrate and propionate in early life are associated with protection against atopy. Allergy. (2019) 74:799–809. doi: 10.1111/all.13660
116. Kleuskens MT, Haasnoot ML, Herpers BM, Ampting MTv, Bredenoord AJ, Garssen J, et al. Butyrate and propionate restore interleukin 13-compromised esophageal epithelial barrier function. Allergy (2021).
117. Sahiner UM, Birben E, Erzurum S, Sackesen C, Kalayci O. Oxidative stress in asthma. World Allergy Organ J. (2011) 4:151–8. doi: 10.1097/WOX.0b013e318232389e
118. Moreno-Macias H, Romieu I. Effects of antioxidant supplements and nutrients on patients with asthma and allergies. J Allergy Clin Immunol. (2014) 133:1237–44. doi: 10.1016/j.jaci.2014.03.020
119. van Neerven R, Savelkoul H. Nutrition and allergic diseases. Nutrients. (2017) 9:762. doi: 10.3390/nu9070762
120. Wang SY, Wang YF, Pan CC, Sun JW. Serum level and clinical significance of vitamin E in children with allergic rhinitis. BMC Pediatr. (2020) 20:362. doi: 10.1186/s12887-020-02248-w
121. Qu J, Li Y, Zhong W, Gao P, Hu C. Recent developments in the role of reactive oxygen species in allergic asthma. J Thorac Dis. (2017) 9:E32–43. doi: 10.21037/jtd.2017.01.05
122. Oh SY, Chung J, Kim MK, Kwon SO, Cho BH. Antioxidant nutrient intakes and corresponding biomarkers associated with the risk of atopic dermatitis in young children. Eur J Clin Nutr. (2010) 64:245–52. doi: 10.1038/ejcn.2009.148
123. Tongtako W, Klaewsongkram J, Mickleborough TD, Suksom D. Effects of aerobic exercise and vitamin C supplementation on rhinitis symptoms in allergic rhinitis patients. Asian Pac J Allergy Immunol. (2018) 36:222–31. doi: 10.12932/AP-040417-0066
124. Dunstan J, Breckler L, Hale J, Lehmann H, Franklin P, Lyons G, et al. Supplementation with vitamins C, E, B-carotene and selenium has no effect on anti-oxidant status and immune responses in allergic adults: a randomized controlled trial. Clin Exp Allergy. (2007) 37:180–7. doi: 10.1111/j.1365-2222.2007.02657.x
125. Grimshaw KE, Maskell J, Oliver EM, Morris RC, Foote KD, Mills EN, et al. Diet and food allergy development during infancy: birth cohort study findings using prospective food diary data. J Allergy Clin Immunol. (2014) 133:511–9. doi: 10.1016/j.jaci.2013.05.035
126. Sandin A, Braback L, Norin E, Bjorksten B. Faecal short chain fatty acid pattern and allergy in early childhood. Acta Paediatr. (2009) 98:823–7. doi: 10.1111/j.1651-2227.2008.01215.x
127. Nica-Badea D, Udristioiu A, Andriţoiu C. Allergenicity risk assessment of foods derived from genetically modified crop plants. Rev Fr Allergol. (2018) 58:29–34.
128. Dunn SE, Vicini JL, Glenn KC, Fleischer DM, Greenhawt MJ. The allergenicity of genetically modified foods from genetically engineered crops: a narrative and systematic review. Ann Allergy Asthma Immunol. (2017) 119:214–22 e3. doi: 10.1016/j.anai.2017.07.010
129. Anil H, Harmanci K. Evaluation of contact sensitivity to food additives in children with atopic dermatitis. Postepy Dermatol Alergol. (2020) 37:390–5. doi: 10.5114/ada.2020.96112
130. Yamashita H, Matsuhara H, Miotani S, Sako Y, Matsui T, Tanaka H, et al. Artificial sweeteners and mixture of food additives cause to break oral tolerance and induce food allergy in murine oral tolerance model for food allergy. Clin Exp Allergy. (2017) 47:1204–13. doi: 10.1111/cea.12928
131. Park S, Choi HS, Bae JH. Instant noodles, processed food intake, and dietary pattern are associated with atopic dermatitis in an adult population (Knhanes 2009-2011). Asia Pac J Clin Nutr. (2016) 25:602–13. doi: 10.6133/apjcn.092015.23
Keywords: air pollution, climate change, epithelial barrier, microbiome, nutrition, exposome
Citation: Celebi Sozener Z, Özbey Yücel Ü, Altiner S, Ozdel Oztürk B, Cerci P, Türk M, Gorgülü Akin B, Akdis M, Yilmaz I, Ozdemir C, Mungan D and Akdis CA (2022) The External Exposome and Allergies: From the Perspective of the Epithelial Barrier Hypothesis. Front. Allergy 3:887672. doi: 10.3389/falgy.2022.887672
Received: 01 March 2022; Accepted: 29 April 2022;
Published: 08 July 2022.
Edited by:
Athanasios Damialis, Aristotle University of Thessaloniki, GreeceReviewed by:
Letty De Weger, Leiden University Medical Center, NetherlandsNicolas Bruffaerts, Sciensano, Belgium
Copyright © 2022 Celebi Sozener, Özbey Yücel, Altiner, Ozdel Oztürk, Cerci, Türk, Gorgülü Akin, Akdis, Yilmaz, Ozdemir, Mungan and Akdis. This is an open-access article distributed under the terms of the Creative Commons Attribution License (CC BY). The use, distribution or reproduction in other forums is permitted, provided the original author(s) and the copyright owner(s) are credited and that the original publication in this journal is cited, in accordance with accepted academic practice. No use, distribution or reproduction is permitted which does not comply with these terms.
*Correspondence: Zeynep Celebi Sozener, zeynepsozener@gmail.com; orcid.org/0000-0003-4188-0959; Cezmi A. Akdis, akdisac@siaf.uzh.ch; orcid.org/0000-0001-8020-019X