Comparison of Risk of Target Organ Damage in Different Phenotypes of Arterial Stiffness and Central Aortic Blood Pressure
- 1Department of Geriatrics, Ruijin Hospital, Shanghai Jiao Tong University School of Medicine, Shanghai, China
- 2Department of Biomedical Sciences, Faculty of Medicine, Health and Human Sciences, Macquarie (University) Medical School, Sydney, NSW, Australia
Objectives: The aim of this study was to explore the risk of target organ damage (TOD) in different groups based on carotid-femoral pulse wave velocity (cfPWV) and central aortic blood pressure (CBP) in different populations.
Methods: The study cohort was divided into four groups according to the status of cfPWV and CBP [Group (cfPWV/CBP): high cfPWV and high CBP; Group (cfPWV): high cfPWV and normal CBP; Group (CBP): normal cfPWV and high CBP; Group (control): normal cfPWV and normal CBP]. TOD was determined by the assessment of carotid intima-media thickness (CIMT) abnormality, chronic kidney disease (CKD), microalbuminuria, and left ventricular hypertrophy (LVH).
Results: A total of 1,280 patients (mean age 53.14 ± 12.76 years, 64.1% male patients) were recruited in this study. Regarding Group (control) as reference, LVH was significantly higher in Group (cfPWV) and Group (CBP) [OR 2.406, 95% CI (1.301–4.452), P < 0.05; OR 2.007, 95% CI (1.335–3.017), P < 0.05]; microalbuminuria was significantly higher in Group (cfPWV/CBP) and Group (CBP) [OR 3.219, 95% CI (1.630–6.359), P < 0.05; OR 3.156, 95% CI (1.961–5.079), P < 0.05]. With age stratified by 60 years, the risk of CKD was significantly higher in Group (cfPWV/CBP) [OR 4.019, 95% CI (1.439–11.229), P < 0.05].
Conclusion: Different phenotypes based on the status of cfPWV and CBP were associated with different TOD. Individuals with both cfPWV and CBP elevated have a higher risk of microalbuminuria.
Introduction
Various studies have demonstrated that arterial stiffness is associated with target organ damage (TOD), such as carotid intima-medium thickness (CIMT) abnormality, left ventricular hypertrophy (LVH), chronic kidney disease(CKD), and microalbuminuria (1–4). At present, the gold standard for evaluating arterial stiffness is carotid-femoral pulse wave velocity (cfPWV). cfPWV is one of the most frequently applied PWV measurements, which has a prognostic value not only for TOD but also for cardiovascular events.
Central aortic pressure is more closely associated with markers of vascular function and incidence of cardiovascular events compared with peripheral pressure (4). In 2019, the Taiwan Society of Cardiology (TSOC) and the Taiwan Society of Hypertension (THS) developed the consensus of clinical application of central blood pressure (CBP) in patients with hypertension, and CBP ≥ 130/90 mmHg was defined as hypertension. CBP is more predictive of TOD than peripheral blood pressure (PBP) (5). However, the potential clinical use of central aortic hemodynamic indices as markers of TOD has not been fully established (5, 6), and few studies have been conducted to elucidate the associations of TOD with cfPWV combined with CBP (1, 2). Based on this, we conducted this study aiming to risk stratify patients for TOD based on vascular risk parameters (cfPWV, CBP). Through this research, we hope to promote individualized diagnostic and therapeutic management of patients and to avoid overtreatment or insufficient treatment of patients by considering only a single biomarker (such as only cfPWV or CBP) in clinical practice.
Methods
Study Population
A total of 1,335 patients from Ruijin Hospital affiliated to Shanghai Jiao Tong University School of Medicine between December 2017 and August 2020 were included in this study. The inclusion criteria were health assessment population and age ≥18 years and ≤ 85 years. All patients gave written informed consent and accepted cfPWV and CBP examinations. Exclusion criteria were clinical or laboratory evidence confirming acute cardiovascular and cerebrovascular disease within the previous 3 months before enrollment or any life-threatening disease, including severe arrhythmias, such as atrial flutter, atrial fibrillation, ventricular premature beats, and ventricular tachycardia. Of the 1,335 patients, 11 cases and 44 cases were excluded due to missing data and data duplication, respectively. Finally, 1,280 patients were recruited in this study. Patients were divided into four groups according to the status of cfPWV and CBP: Group (cfPWV/CBP) (high cfPWV and high CBP), Group (cfPWV) (high cfPWV and normal CBP), Group (CBP) (normal cfPWV and high CBP), and Group (control) (normal cfPWV and normal CBP). cfPWV >10 m/s was defined as “high cfPWV,” and cfPWV ≤ 10 m/s was defined as “normal cfPWV” for cfPWV >10 m/s is a high-risk factor of asymptomatic hypertensive target organ damage (7). “High CBP” refers to central systolic blood pressure (cSBP) ≥130 mmHg and/or central diastolic blood pressure (cDBP) ≥90 mmHg, and “normal CBP” refers to cSBP < 130 mmHg and cDBP < 90 mmHg (8). Medical records, including age, sex, height, body mass index (BMI), smoking history (yes or no), antihypertensive drugs (yes or no), antilipidemic drugs (yes or no), heart rate (HR), and cfPWV, were collected. A sample of venous blood was drawn, and a sample of urine was collected after obtaining informed consent. Serum fasting glucose, hemoglobinA1c (HbA1c), creatinine (Cr), uric acid, triglyceride and total, low-density lipoprotein (LDL), and high-density lipoprotein (HDL) cholesterol were measured with standard methods on the venous blood sample; urinary albumin and creatinine were measured from the urine sample. Body mass index (BMI) was calculated as body weight in kilograms divided by the square of body height in meters; body surface area (BSA) was calculated using the formula: BSA (m2) = 0.0061 × body height (m) + 0.0128 × body weight (kg)−0.1529 (9). The study protocol was reviewed and approved by the Ethics Committee of Ruijin Hospital (Ethics No. 2011-30), Shanghai Jiao Tong University School of Medicine. All patients provided written informed consent.
Indices of Central and Peripheral Hemodynamics
Radial waveforms and pulse wave analysis measurements were obtained by applanation tonometry using a high-fidelity SPT-304 micromanometer (Millar Instruments, Houston, TX) interfaced with a laptop computer. Central aortic pressure waveforms were derived from the radial waveforms with a validated transfer function using the SphygmoCor software, version 8.0 (AtCor Medical, Sydney, Australia) (10), and central hemodynamics indices, including central systolic blood pressure (cSBP), central diastolic blood pressure (cDBP), central mean arterial blood pressure (cMAP), central augmentation index (cAIx), and cAIx adjusted to heart rate of 75 bpm (beats per minute) (AIx@HR75), were generated. Radial waveforms were calibrated with the average of the peripheral systolic blood pressure (pSBP) and peripheral diastolic blood pressure (pDBP) measured 3 times at the left brachial artery with a validated Omron 705CP oscillometric device (Omron, Kyoto, Japan) (11), following at least 10 min of rest, and all measurements were performed in a quiet room with stable temperature with the subject in a supine position, avoiding smoking, caffeine, and exercise for 30 min (12). Peripheral mean arterial blood pressure (pMAP) was calculated for further study. Recordings were discarded when systolic or diastolic variability of consecutive waveforms exceeded 5% or when the amplitude of the pulse wave signal was <80 mV. All recordings met the manufacturer's quality control standards integrated into the software package.
Carotid-Femoral Pulse Wave Velocity
Carotid-femoral pulse wave velocity was calculated as the measured distance from the suprasternal notch to the femoral artery subtracted by the distance from the suprasternal notch to the carotid artery and then divided by the pulse transit time. The fiducial point at the foot of the pulse for the measurement of transit time was determined by the intersecting tangent method, where a linear fit is performed on the late diastolic portion of the pulse and the early systolic rise of the subsequent pulse. The pulse transit time between the two arterial sites was determined as the difference between the R-wave of the electrocardiogram and the diastolic foot at the respective sites averaged over 10 consecutive heartbeats. Following the measurement of office blood pressure, carotid and femoral arterial waveforms at the patient's right side were obtained by applanation tonometry sequentially a short time apart. Patients fasted overnight, and no caffeine beverage or smoking was allowed within 3 h of the measurement. A single high-fidelity applanation tonometer SphygmoCor V8.0 device (AtCor Medical, Sydney, Australia) was used for the PWV measurements. cfPWV > 10 m/s was defined as “high cfPWV,” and cfPWV ≤ 10 m/s was defined as “normal cfPWV” in our study. We also estimated the cardio-ankle vascular index (CAVIo) using the same cfPWV value (13, 14).
Target Organ Damage
Carotid Intima-Media Thickness
Carotid intima-media thickness was examined bilaterally using high-resolution Doppler ultrasound (HD11EX Ultrasound; Philips Medical Systems, Andover, MA, USA) with a broadband linear array transducer (multiple frequencies: 4–12 MHz). Three recordings were taken from the bulb origin (common carotid artery starting ~1.5 cm proximal to the carotid artery bulb) of both left and right carotid arteries during the diastolic portion of the cardiac cycle, and the average value of the three recordings was calculated for each side. Finally, CIMT was calculated as the average of the left CIMT and the right CIMT ([Left CIMT + Right CIMT]/2). CIMT > 1.3 mm was diagnosed with carotid plaque. CIMT abnormality was diagnosed as CIMT ≥0.9 mm and/or the presence of carotid plaque.
Left Ventricular Hypertrophy
Cardiac dimensions were measured based on a standardized reading protocol, and all indices were evaluated by an experienced sonographer or cardiologist. Cardiac dimensions were quantified using digital images and the leading-edge technique as recommended by the American Society of Echocardiography. M-mode echocardiography was used to obtain linear measurements of the left ventricular (LV) cavity [LV end-diastolic diameter and LV end-systolic diameter (LVESD), interventricular septum thickness (IVST), and posterior wall thickness (PWT)]. Left ventricular mass (LVM) was calculated according to the American Society of Echocardiography guidelines (15). LVM was calculated with the formula: LVM (g) = 0.8 × [1.04 × [(IVST + PWT + LVDD)3-(LVDD)3] + 0.6. Body surface area (BSA) was calculated using the formula: BSA (m2) = 0.0061 × body height (m) + 0.0128 × body weight (kg)−0.1529. Left ventricular mass index (LVMI) = LVM/BSA (g/m2). We defined LVH as LVMI >95 g/m2 and >115 g/m2 for women and men, respectively.
Renal Abnormalities
Urinary albumin-creatinine ratio (ACR) was used to screen patients with urinary albuminuria. ACR was measured from spot morning urine samples obtained from participants. ACR values have been shown to identify kidney disease that occurs as a complication with hypertension. Abnormal albuminuria was defined as a urine ACR >3.5 mg/mmol in female patients and >2.5 mg/mmol in male patients. The definition and the diagnostic criteria for chronic kidney disease were proposed in the K/DOQI guidelines (16): estimated glomerular filtration rate (eGFR <60 ml/min/1.73 m2) calculated by the MDRD formula: eGFR (ml/min/1.73 m2) = 175 × Cr (mg/dl)−1.234 × age (years old)−0.179 × 0.79 (if female patient).
Statistical Analysis
All analyses were performed using SPSS 24.0 for Windows (SPSS Inc, Chicago, IL, USA). A two-sided P < 0.05 was considered statistically significant. Quantitative and qualitative parameters were presented as mean ± standard deviation and numbers with the percentage in parentheses, compared among groups by one-way ANOVA and chi-squared test, respectively. Pearson's correlation analysis was applied to investigate the correlation of central and peripheral hemodynamic indices with TOD. Furthermore, the relative odds ratios by multivariate stepwise linear or logistic regressions analysis [forward likelihood ratio (LR)] were conducted to compare the associations of risk factors with TOD among different groups after adjusting for age, sex, BMI, height, smoking history, antihypertensive drugs (yes or no), HDL-c, LDL-c, FBG, HR, and pMAP. Only variables staying in the final model were presented.
Results
Baseline Characteristics of the Studied Population
A total of 1,280 patients (mean age 53.14 ± 12.76 years, 64.06% male patients) were recruited in this study; 30.8% of patients were taking antihypertensive drugs; and 16.8% of patients had a smoking history. ACR was skewed so Log ACR was used for the logistic regression. Mean values of CIMT, LVMI, eGFR, and LogACR were significantly different in the four groups (P < 0.05). The percentages of TOD were significantly different among Group (cfPWV/CBP) to Group (control) (P < 0.001). Age, BMI, triglycerides, cholesterol, FBG, HbA1c, HR, cfPWV, and CAVIo were all significantly different among groups (P < 0.05). As for central and peripheral blood hemodynamic indices, patients in Group (cfPWV/CBP) had significantly higher levels of pSBP and cSBP than the other three groups (P < 0.05). Patients in Group (CBP) had higher levels of pDBP, cDBP, and cAIx than Group (cfPWV) and Group (control) (P < 0.05) (Table 1).
Correlation Between Central or Peripheral Hemodynamic Indices and TOD
In the overall studied population, cfPWV and cSBP were positively correlated with CIMT (r = 0.283, P < 0.01; r = 0.186, P < 0.01) (Figures 1A,B). cfPWV and cSBP were negatively correlated with eGFR (r = −0.235, P < 0.01; r = −0.122, P < 0.01) (Figures 1C,D). Both cfPWV and cSBP were positively correlated with LVMI(r = 0.325, P < 0.01; r = 0.281, P < 0.01) (Figures 1E,F), and LogACR (r = 0.185, P < 0.01; r = 0.185, P < 0.01) (Figures 1G,H).
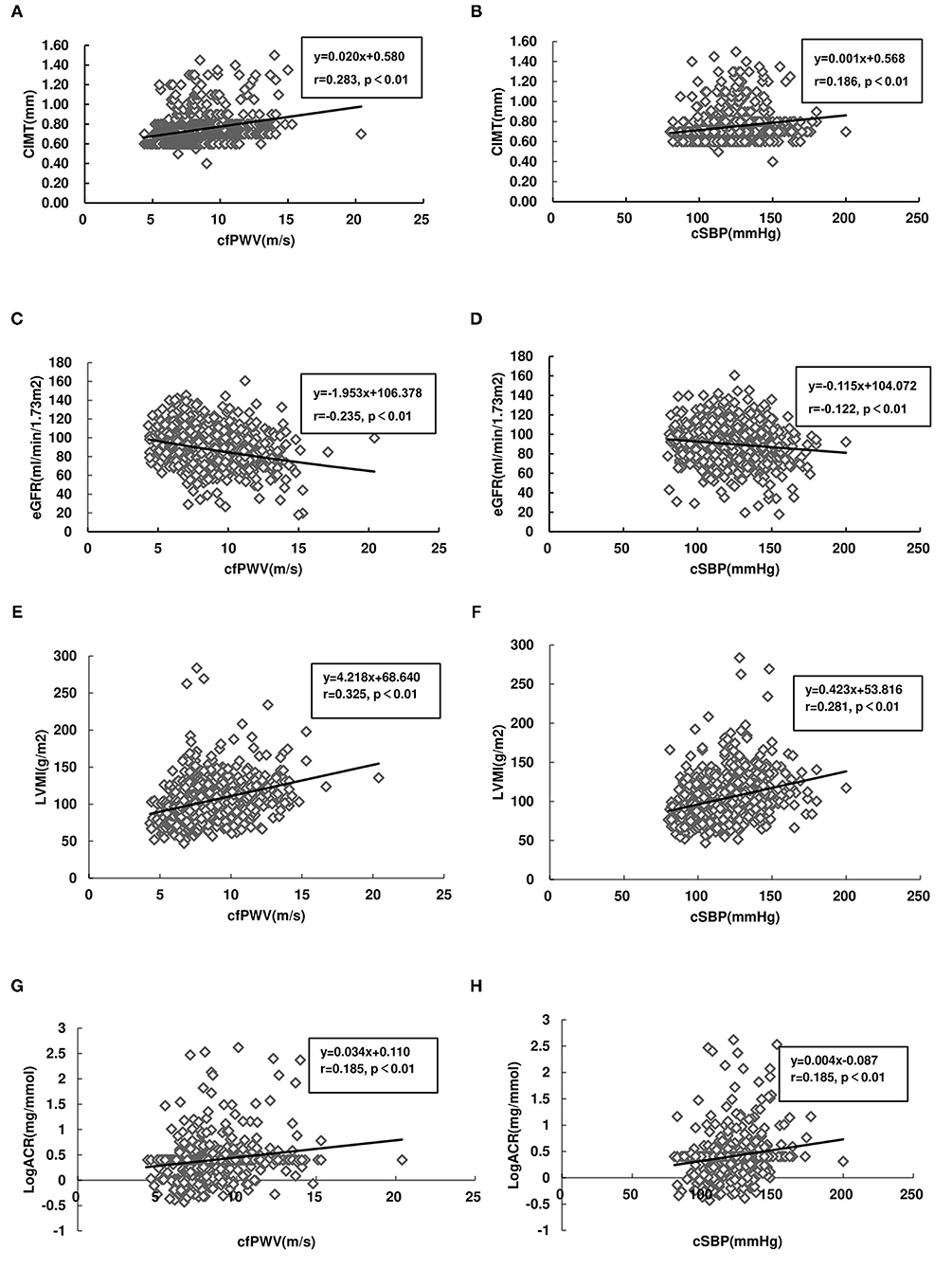
Figure 1. Correlations between target organ damage (TOD) and carotid-femoral pulse wave velocity (cfPWV), as well as central systolic blood pressure (cSBP), carotid intima-media thickness (CIMT), and cfPWV (A); CIMT and cSBP (B); estimated glomerular filtration rate (eGFR) and cfPWV (C); eGFR and cSBP (D); left ventricular mass index (LVMI) and cfPWV (E); LVMI and cSBP (F); Log (urinary albumin-creatinine ratio, ACR) (LogACR) and cfPWV (G); and LogACR and cSBP (H). Linear regression lines for (x) and (y) variables are shown with correlation coefficients and P-values.
Multivariate Stepwise Linear and Logistic Regression Analysis of the Relationship Between Arterial Stiffness Indices or CBP With the Presence of TOD
In the overall study population, according to multiple stepwise linear regression analysis, cSBP was positively correlated with CIMT (β = 0.095, P = 0.009), cSBP and cfPWV were positively correlated with LVMI (β = 0.105, P = 0.008; β = 0.137, P = 0.003), cDBP was negatively correlated with eGFR (β = −0.103, P = 0.001), whereas cAIx@HR75 was positively correlated with eGFR (β = 0.080, P = 0.017) and cSBP was positively correlated with LogACR (β = 0.186, P < 0.001) after adjusting for age, sex, height, BMI, smoking history, antihypertensive drugs (yes or no), HDL-c, LDL-c, FBG, pMAP, and HR. Age was the main independent influence factor of CIMT, eGFR, and LVMI, while cSBP affected LogACR more compared with age and FBG (Table 2).
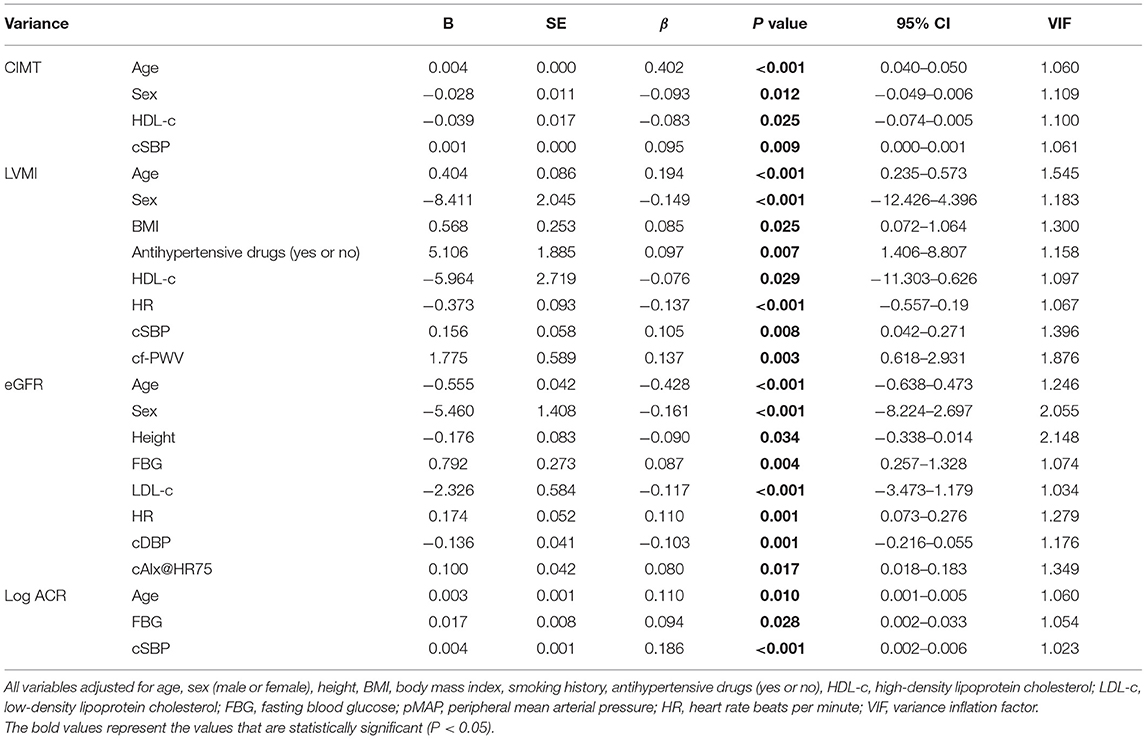
Table 2. Multiple stepwise linear regression of risk factors of target organ damage in the overall studied population.
According to multiple stepwise logistic regression, we found that cAIx@HR75 was significantly associated with LVH [OR 0.959, 95% CI (0.936–0.983), P = 0.001], cfPWV was significantly associated with CKD [OR 1.303, 95% CI (1.096–1.550), P = 0.003], and cSBP was significantly associated with ACR abnormality [OR 1.034, 95% CI (1.020–1.048), P < 0.001] after adjusting for age, sex, height, BMI, smoking history, antihypertensive drugs (yes or no), HDL-c, LDL-c, FBG, pMAP, and HR (Table 3).
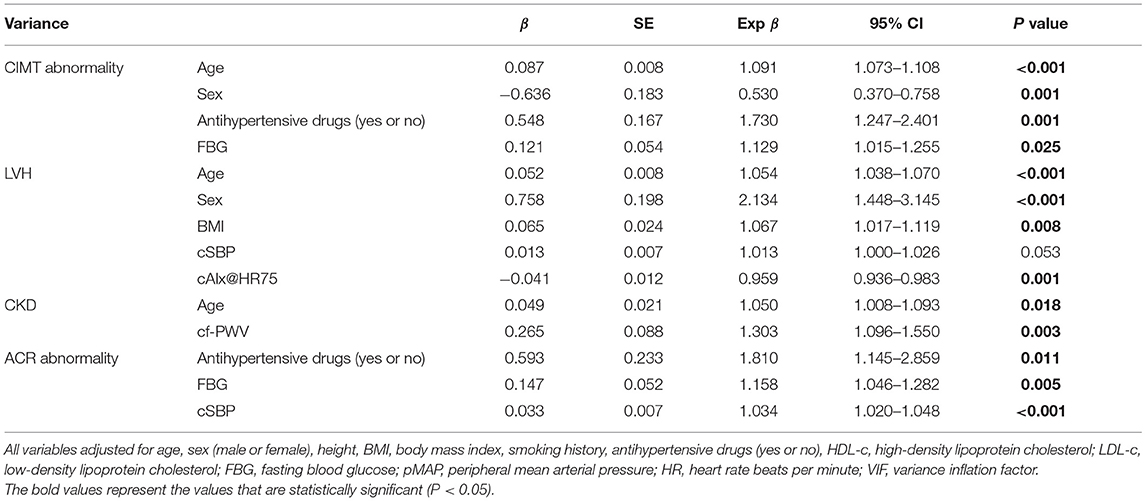
Table 3. Multiple stepwise logistic regression of risk factors of target organ damage in the overall studied population.
Comparing the Risk of TOD in Different Groups Based on the Status of cfPWV and CBP by Multiple Stepwise Logistic Regression Analysis
Without adjusting for covariates, regarding Group (control) as reference, CIMT abnormality was significantly higher in Group (cfPWV/CBP) and Group (cfPWV) [OR 3.182, 95% CI (1.854–5.460), P < 0.01; OR 4.544, 95% CI (2.487–8.302), P < 0.01]. LVH was significantly higher in Group (cfPWV/CBP), Group (cfPWV), and Group (CBP) [OR 1 3.012, 95% CI (1.787–5.077), P < 0.01; OR 2 3.920, 95% CI (2.330–6.597), P < 0.01; OR 3 2.101, 95% CI (1.456–3.032), P < 0.01]. CKD had a significantly higher prevalence in Group (cfPWV/CBP) [OR 6.667, 95% CI (2.901–15.321), P < 0.01]. ACR abnormality was significantly higher in Group (cfPWV/CBP) and Group (CBP) [OR 5.157, 95% CI (2.724–9.762), P < 0.01; OR 3.848, 95% CI (2.437–6.075), P < 0.01] (Figure 2).
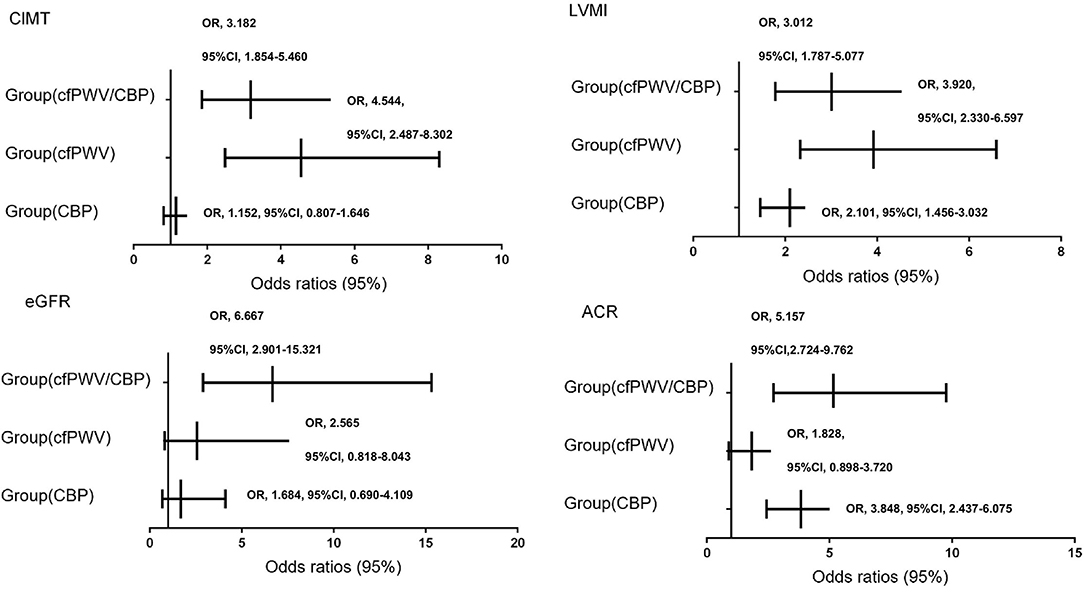
Figure 2. Multiple stepwise logistic regression of target organ damage among groups by cf-PWV and CBP without adjusted factors. Groups by cfPWV and CBP status: Group (cfPWV/CBP): high cfPWV and high CBP; Group (cfPWV): high cfPWV and normal CBP; Group (CBP): normal cfPWV and high CBP; Group (control): normal cfPWV and normal CBP.
After adjusting for covariates, such as age, sex, height, BMI, smoking history, antihypertensive drugs (yes or no), HDL-c, LDL-c, FBG, pMAP, and HR, we found that LVH was significantly higher in Group (cfPWV) and Group (CBP) [OR 2.406, 95% CI (1.301–4.452), P = 0.005; OR 2.007, 95% CI (1.335–3.017), P = 0.001]. ACR abnormality was significantly higher in Group (cfPWV/CBP) and Group (CBP) [OR 3.219, 95% CI (1.630–6.359), P = 0.001; OR 3.156, 95 % CI (1.961–5.079), P < 0.001] (Table 4). As age was the main susceptibility factor of CKD and CIMT abnormality, we performed the subgroup analysis of eGFR abnormality and CIMT abnormality in different groups by stratifying age by 60 years according to the international standards for the definition of elderly at 60 years (17). Furthermore, we found that CKD was significantly higher in Group (cfPWV/CBP) [OR 4.019, 95% CI(1.439–11.229), P = 0.008] (Table 5), and CIMT abnormality was significantly lower in Group (CBP) [OR 0.466, 95% CI (0.231–0.941), P = 0.033] (Table 6).
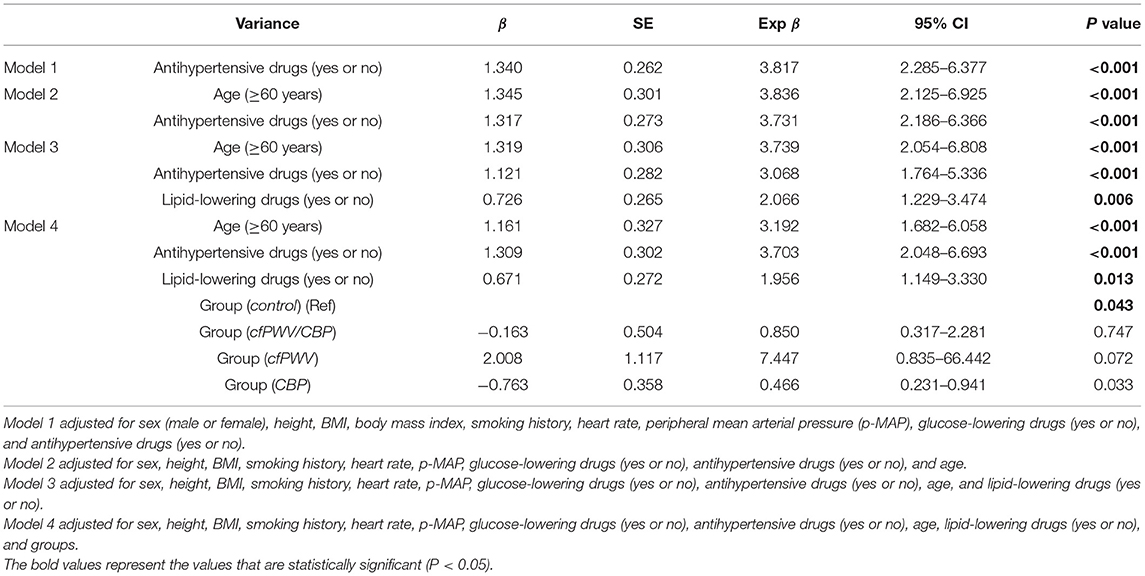
Table 6. Subgroup analysis of CIMT abnormalities adjusting for age among different groups by cfPWV and CBP.
Discussion
Through multiple stepwise linear or logistic regression analysis, we found that CBP parameters and cfPWV were significantly correlated with cardiovascular and renal damage indexes. By group comparison, Group (cfPWV/CBP), individuals with high cf-PWV and high CBP, had an increased risk of microalbuminuria; Group (cfPWV), individuals with high cf-PWV and low CBP, had an increased risk of LVH, while Group (CBP) of low cf-PWV and high CBP had an increased risk of both microalbuminuria and LVH, with OR values lower than the first two groups. The prevalence of CKD and CIMT abnormality in the three groups was not significantly different from that in the control group.
cf-PWV, identified by meta-analyses as a predictor of future CVD events and all-cause mortality independent of blood pressure (6, 18), has also been associated with a decreased renal function (19–21). On the contrary, a number of studies have shown that central aortic blood pressure could better reflect the load on the heart and central vasculature (22, 23) and is associated with cardiovascular outcomes and mortality independent of peripheral brachial arterial pressure (24). In this study, CBP parameters and cf-PWV were found to be significantly correlated with cardiovascular and renal damage indexes, consistent with previous studies.
Although there is a strong relationship between blood pressure and vascular stiffness, the correlation between various blood pressure parameters and cf-PWV could be different (25) and varies according to the age range of the population studied (26–28), not to mention that cf-PWV is influenced by many other factors, such as gender, heart rate, salt intake, or genetic factors. Therefore, it is not surprising that CBP and cf-PWV are partly inconsistent in the study population. We found discordant CBP and cf-PWV status in 378 of 1,280 participants (29.5%): 92 with normal CBP and high cf-PWV and 286 with high CBP and normal cf-PWV. We questioned if the population was divided into different subgroups based on cf-PWV and CBP, whether the groups would show inconsistency in screening TOD?
A small number of studies showed apparent inconsistencies between CBP and cf-PWV. In a sub-study of the Framingham Heart Study, a similar grouping method was used to investigate the relative predictive value of central pulse pressure and cf-PWV for LVH and CVD, and it was concluded that in the community-dwelling middle-aged population, disagreement between pulse pressure and cf-PWV is common, with the highest prevalence of LVH and highest risk of CVD in people with both being elevated (29). However, in our study, regarding Group (control) as a reference, LVH was significantly higher in Group (cfPWV/CBP), Group (cfPWV), and Group (CBP) without adjusting covariates. After adjusting for covariates, Group (cfPWV/CBP) was not associated with LVH [OR = 1.762, P = 0.064]. In accordance with “Framingham Heart Study” (29), we additionally adjusted for height and pMAP in multiple logistic regression analysis. By screening covariates, we found that age was the main factor. Hence, this result illustrates the significant role of adjusting potential confounding variables that influence the risk factors.
In our study, individuals with only cf-PWV elevated had the highest risk of LVH, while the risk of microalbuminuria was not significantly different from the control group. In contrast, individuals with only CBP elevated had a significantly increased risk of both TOD. This suggests that cf-PWV may be a more important driver of CVD than CBP, but inferior to CBP in screening for early manifestations of kidney damage, which may be because cf-PWV is more a measure of large artery stiffness and does not fully reflect the stiffness or function of smaller arteries. However, with the two combined, we found that individuals with both high cf-PWV and high CBP had an even greater risk of microalbuminuria than individuals with elevated CBP alone, which may suggest that the conjoint effect of cf-PWV and CBP on screening for early renal damage is additive.
Multiple stepwise linear regression analysis showed that cSBP was positively significantly associated with CIMT after adjusting for covariates, and age was the main independent influence factor of CIMT, eGFR, and LVMI, while cSBP affected LogACR more compared with age and FBG in our study. As age was a main susceptibility factor of CIMT (30), we stratified age by 60 years and then we found that CIMT abnormality was significantly lower in Group (CBP) compared with Group (control). A previous study suggested that each SD increase in pPP and cPP was associated with an increased risk of carotid IMT >0.9 mm (31). Various studies have illustrated that cf-PWV was significantly related to CIMT and arterial plaque (3, 32). However, there were few studies conducted to compare associations of CBP with CIMT in a certain level of cfPWV. For the sample size limitation and unclassified CIMT and carotid plaque of our study, further research could be carried out to investigate the relationship between CBP and CIMT in individuals with different degrees of arterial stiffness.
In our study, cAIx@HR75 was significantly associated with LVH [OR = 0.959, P = 0.001] after adjusting for covariates. Previous studies showed that pulse pressure amplification (PPA) was tightly associated with LVH (32); higher MAP and central pulse pressure were associated with incident LVH (4). However, opinion on the influence of cAIx@HR75 on LVH varies. In Obayashia's study (33), central AIx was lower in men than in women with hypertension, but the central AIx was not independently associated with the LVMI. It has been shown that in patients with never-treated hypertension, female sex, and shorter height are the important risk factors of elevated radial AIx 75 (34). In our study, cAIx@HR75 was significantly higher in female patients (28.51 ± 10.68 vs. 23.43 ± 12.09, P < 0.01). However, multiple logistical regression with age stratified by 60 years showed that cAIx@HR75 was not significantly associated with LVH. Aix was associated with arterial stiffness; however, it has been shown that central SBP, but not the central AIx, was an independent determinant of LVH in hypertensive patients in general (33). Further research needs to be conducted to explore the varying relationship of cAIx@HR75 and LVH.
Therefore, each subtype seems to show different associations with certain TOD, and we propose that this is an interesting study direction for further investigation. With larger sample size and prospective studies, by exploring the development trend of diseases in different subtypes of patients, more evidence might be provided for clinical work to guide the focus of screening for TODs and related therapy.
The use of measured systolic and diastolic pressure of brachial artery for calibration of the radial waveform is a conventional method that is applied to the SphygmoCor device and has been widely used. The effect of waveform morphology affecting the relationship between central and peripheral pulse pressure is taken into account by the mathematical transfer function in the device. This accounts for differences in pulse pressure, and the mean pressure is equivalent between central and peripheral locations. However, limitations still remain. Validation of data, as well as the clinical utility of these devices, varies (35); when the radial waveform was calibrated with the oscillometric brachial pressures, the SphygmoCor system could not provide an accurate estimation of central BPs measured invasively. The inherent error in the sphygmomanometric measurement of cuff pressure was the major limiting factor and not the use of the transfer function in clinical settings (36) when compared with invasive values. Recent studies have shown that among different calibration approaches of central aortic blood pressure, including systo-diastolic (SD), calculated mean (CM), and oscillometric mean (OscM) (37–39), CM and OscM were preferred. Further studies, such as validation of a novel method to derive central aortic systolic pressure from the radial pressure waveform using an N-Point moving average method, have been explored as well (13, 40). Hence, the results of this study should be considered in the context of the conventional measurement of BP using a brachial cuff sphygmomanometer when the radial pulse wave is calibrated using brachial SBP and DBP.
Arterial stiffness is influenced by BP, and cfPWV is BP dependent, which has hampered its use in clinical practice. To overcome the limitations, different approaches and parameters have been proposed. The cardio-ankle vascular index (CAVI) is essentially a BP independent index of arterial stiffness, overcoming the limitation of cfPWV. However, CAVI is not equal to the actual, intrinsic stiffness index of the pressure–diameter relationship (β0) but instead varies with BP. A straightforward modification of the formula for calculating CAVI yields a pressure independent version, that is, CAVIo. Actually, PWV in the formula is not equal to cfPWV but PWV measured between the aortic valve and the ankle. Even though some studies have shown that the CAVI formula can equally well be applied to any PWV measurement in pulsatile pressure vessels, including the carotid-femoral PWV (41, 42), we still would expect further studies on the accurate formula of cfPWV. Furthermore, our study calculated CAVIo as a derivation using cfPWV rather than estimating it directly; therefore, we focused on examining the associations of cfPWV with other hemodynamic parameters.
Nevertheless, there are still some limitations in our research. As a cross-sectional study with a small sample size, the results need to be further confirmed in prospective studies. The study was conducted in an Asian population, and it is not known whether the results will hold true for other ethnic groups. To account for possible measurement variations, in future studies consideration could be given to using the average value of the three measurements of cfPWV. In our study, although 10 m/s was chosen as the cutoff of cfPWV based on previous guidelines (7, 43), some limitations still remain. Arterial stiffness was age-related, distributing homogeneously. The age-related reduction in the central-to-periphery stiffness gradient is associated with adverse clinical outcomes (44). For the first time, the study of Bia et al. (42) gave the definition of population-based reference values for PWV, suggesting that age-related stiffness changes were less marked in middle-aged adults and became gradually greater after ~60 years. The majority of the studied population in our study was middle aged, so that cutoff 10 m/s of cfPWV might not adequately characterize the real variations of TOD in different groups in young and older adults. Therefore, additional information might be obtained by accounting for age- and sex-related reference intervals (RIs) for regional and local PWV.
In conclusion, different phenotypes based on the status of cf-PWV and CBP are associated with different TOD. For patients with both elevated cfPWV and CBP, screening for microalbuminuria facilitates the detection of early renal damage. Patients with elevated cfPWV or CBP are recommended to accept echocardiograph Doppler examination for the early detection of risks of LVH. Furthermore, patients with only elevated CBP have an increased risk of ACR abnormality, and central aortic blood pressure evaluation probably helps to assess the risk of early renal impairment. Patients with age over 60 years with both elevated cfPWV and CBP had an increased risk of CKD. The combination of cfPWV and CBP grouping has limited significance for the evaluation of CIMT abnormalities.
Data Availability Statement
The original contributions presented in the study are included in the article/supplementary material, further inquiries can be directed to the corresponding authors.
Ethics Statement
The studies involving human participants were reviewed and approved by Ethics Committee of Ruijin Hospital. The patients/participants provided their written informed consent to participate in this study.
Author Contributions
JZ: conceptualization and funding acquisition. YB, QW, DC, YH, and HC: data curation. YB, QW, BT, and JZ: formal analysis. YB, DC, and JZ: methodology. YB and QW: writing (original draft). AA, BT, and JZ: writing (review and editing). All authors contributed to the article and approved the submitted version.
Funding
This project was supported by the National Natural Science Foundation of China (Grant No. 81500190), Clinical Science and Shanghai Municipal Hospital New Frontier Technology Joint Project (SHDC12019X20), and Shanghai Municipal Commission of Health and Family Planning (Grant No. 2020YJZX0124).
Conflict of Interest
The authors declare that the research was conducted in the absence of any commercial or financial relationships that could be construed as a potential conflict of interest.
Publisher's Note
All claims expressed in this article are solely those of the authors and do not necessarily represent those of their affiliated organizations, or those of the publisher, the editors and the reviewers. Any product that may be evaluated in this article, or claim that may be made by its manufacturer, is not guaranteed or endorsed by the publisher.
Acknowledgments
We gratefully acknowledge the invaluable assistance of the physicians of the Department of Geriatric Medicine Ruijin Hospital, Shanghai Jiaotong University School of Medicine. This study would not have been possible without their support.
References
1. Kaess BM, Rong J, Larson MG, Hamburg NM, Vita JA, Cheng S, et al. Relations of central hemodynamics and aortic stiffness with left ventricular structure and function: the Framingham heart study. J Am Heart Assoc. (2016) 5: e002693. doi: 10.1161/JAHA.115.002693
2. Kollias A, Lagou S, Zeniodi ME, Boubouchairopoulou N, Stergiou GS. Association of central vs. brachial blood pressure with target-organ damage: systematic review and meta-analysis. Hypertension. (2016) 67: 183–90. doi: 10.1161/HYPERTENSIONAHA.115.06066
3. Lu Y, Zhu M, Bai B, Chi C, Yu S, Teliewubai J, et al. Comparison of carotid-femoral and brachial-ankle pulse-wave velocity in association with target organ damage in the community-dwelling elderly Chinese: the Northern Shanghai study. J Am Heart Assoc. (2017) 6:e004168. doi: 10.1161/JAHA.116.004168
4. Vasan RS, Short MI, Niiranen TJ, Xanthakis V, DeCarli C, Cheng S, et al. Interrelations between arterial stiffness, target organ damage, and cardiovascular disease outcomes. J Am Heart Assoc. (2019) 8:e012141. doi: 10.1161/JAHA.119.012141
5. Zuo J, Chang G, Tan I, Butlin M, Chu SL, Avolio A. Central aortic pressure improves prediction of cardiovascular events compared to peripheral blood pressure in short-term follow-up of a hypertensive cohort. Clin Exp Hypertens. (2020) 42:16–23. doi: 10.1080/10641963.2018.1557682
6. Ben-Shlomo Y, Spears M, Boustred C, May M, Anderson SG, Benjamin EJ, et al. Aortic pulse wave velocity improves cardiovascular event prediction: an individual participant meta-analysis of prospective observational data from 17,635 subjects. J Am Coll Cardiol. (2014) 63: 636–46. doi: 10.1016/j.jacc.2013.09.063
7. Williams B, Mancia G, Spiering W, Agabiti Rosei E, Azizi M, Burnier M, et al. 2018 Esc/Esh guidelines for the management of arterial hypertension. Eur Heart J. (2018) 39:3021–104. doi: 10.1093/eurheartj/ehy339
8. Hao-Min Cheng, Shao-Yuan Chuang, Shih-Hsien Sung, Chih-Cheng Wu, Jiun-Jr Wang, Pai-Feng Hsu, et al. 2019 Consensus of the Taiwan hypertension society and Taiwan society of cardiology on the clinical application of central blood pressure in the management of hypertension. Acta Cardiol Sin. (2019) 35:234–43. doi: 10.6515/acs.201905_35(3).20190415b
9. Stevenson PH. Height-weight-surface formula for the estimation of surface area in Chinese subjects. Chin J Physiol. (1937) 3: 327–330.
10. Chen CH, Nevo E, Fetics B, Pak PH, Yin FC, Maughan WL, et al. Estimation of central aortic pressure waveform by mathematical transformation of radial tonometry pressure. Validation of generalized transfer function. Circulation. (1997) 95:1827–36. doi: 10.1161/01.CIR.95.7.1827
11. Laurent S, Cockcroft J, van Bortel L, Boutouyrie P, Giannattasio C, Hayoz D, et al. Expert consensus document on arterial stiffness: methodological issues and clinical applications. Eur Heart J. (2006) 27: 2588–605. doi: 10.1093/eurheartj/ehl254
12. Unger T, Borghi C, Charchar F, Khan NA, Poulter NR, Prabhakaran D, et al. 2020 International society of hypertension global hypertension practice guidelines. Hypertension. (2020) 75: 1334–57. doi: 10.1161/HYPERTENSIONAHA.120.15026
13. Spronck B, Avolio AP, Tan I, Butlin M, Reesink KD, Delhaas T. Arterial stiffness index beta and cardio-ankle vascular index inherently depend on blood pressure but can be readily corrected. J Hypertens. (2017) 35: 98–104. doi: 10.1097/HJH.0000000000001132
14. Spronck B, Delhaas T, Butlin M, Reesink KD, Avolio AP. Options for dealing with pressure dependence of pulse wave velocity as a measure of arterial stiffness: an update of cardio-ankle vascular index (Cavi) and Cavi0. Pulse. (2018) 5:106–14. doi: 10.1159/000479322
15. Nunan D, Wassertheurer S, Lasserson D, Hametner B, Fleming S, Ward A, et al. Assessment of central haemomodynamics from a brachial cuff in a community setting. BMC Cardiovasc Disord. (2012) 12:48. doi: 10.1186/1471-2261-12-48
16. Ma YC, Zuo L, Chen JH, Luo Q, Yu XQ, Li Y, et al. Modified glomerular filtration rate estimating equation for Chinese patients with chronic kidney disease. J Am Soc Nephrol. (2006) 17:2937–44. doi: 10.1681/ASN.2006040368
17. WHO. World Health Day 2012: Ageing and Health: Toolkit for Event Organizers. Geneva: World Health Organization (2012).
18. Vlachopoulos C, Aznaouridis K, Stefanadis C. Prediction of cardiovascular events and all-cause mortality with arterial stiffness: a systematic review and meta-analysis. J Am Coll Cardiol. (2010) 55:1318–27. doi: 10.1016/j.jacc.2009.10.061
19. Elias MF, Robbins MA, Budge MM, Abhayaratna WP, Dore GA, Elias PK. Arterial pulse wave velocity and cognition with advancing age. Hypertension. (2009) 53:668–73. doi: 10.1161/HYPERTENSIONAHA.108.126342
20. Kearney-Schwartz A, Rossignol P, Bracard S, Felblinger J, Fay R, Boivin JM, et al. Vascular structure and function is correlated to cognitive performance and white matter hyperintensities in older hypertensive patients with subjective memory complaints. Stroke. (2009) 40:1229–36. doi: 10.1161/STROKEAHA.108.532853
21. Triantafyllidi H, Arvaniti C, Lekakis J, Ikonomidis I, Siafakas N, Tzortzis S, et al. Cognitive impairment is related to increased arterial stiffness and microvascular damage in patients with never-treated essential hypertension. Am J Hypertens. (2009) 22:525–30. doi: 10.1038/ajh.2009.35
22. Oliveras A, García-Ortiz L, Segura J, Banegas JR, Martell-Claros N, Vigil L, et al. Association between urinary albumin excretion and both central and peripheral blood pressure in subjects with insulin resistance. J Hypertens. (2013) 31:103–8. doi: 10.1097/HJH.0b013e32835ac7b5
23. Roman MJ, Okin PM, Kizer JR, Lee ET, Howard BV, Devereux RB. Relations of central and brachial blood pressure to left ventricular hypertrophy and geometry: the strong heart study. J Hypertens. (2010) 28:384–8. doi: 10.1097/HJH.0b013e328333d228
24. Sharman JE, Marwick TH, Gilroy D, Otahal P, Abhayaratna WP, Stowasser M. Randomized trial of guiding hypertension management using central aortic blood pressure compared with best-practice care: principal findings of the Bp guide study. Hypertension. (2013) 62:1138–45. doi: 10.1161/HYPERTENSIONAHA.113.02001
25. Kim EJ, Park CG, Park JS, Suh SY, Choi CU, Kim JW, et al. Relationship between blood pressure parameters and pulse wave velocity in normotensive and hypertensive subjects: invasive study. J Hum Hypertens. (2007) 21:141–8. doi: 10.1038/sj.jhh.1002120
26. Nürnberger J, Dammer S, Opazo Saez A, Philipp T, Schäfers RF. Diastolic blood pressure is an important determinant of augmentation index and pulse wave velocity in young, healthy males. J Hum Hypertens. (2003) 17:153–8. doi: 10.1038/sj.jhh.1001526
27. Franklin SS, Gustin Wt, Wong ND, Larson MG, Weber MA, Kannel WB, et al. Hemodynamic patterns of age-related changes in blood pressure. The Framingham heart study. Circulation. (1997) 96:308–15. doi: 10.1161/01.CIR.96.1.308
28. Khattar RS, Swales JD, Dore C, Senior R, Lahiri A. Effect of aging on the prognostic significance of ambulatory systolic, diastolic, and pulse pressure in essential hypertension. Circulation. (2001) 104:783–9. doi: 10.1161/hc3201.094227
29. Niiranen TJ, Kalesan B, Mitchell GF, Vasan RS. Relative contributions of pulse pressure and arterial stiffness to cardiovascular disease. Hypertension. (2019) 73:712–7. doi: 10.1161/HYPERTENSIONAHA.118.12289
30. Song P, Fang Z, Wang H, Cai Y, Rahimi K, Zhu Y, et al. Global and regional prevalence, burden, and risk factors for carotid atherosclerosis: a systematic review, meta-analysis, and modelling study. Lancet Glob Health. (2020) 8:e721–9. doi: 10.1016/S2214-109X(20)30117-0
31. Zuo J, Chu S, Tan I, Butlin M, Zhao J, Avolio A. Association of haemodynamic indices of central and peripheral pressure with subclinical target organ damage. Pulse. (2018) 5:133–43. doi: 10.1159/000484441
32. Bai B, Teliewubai J, Lu Y, Yu S, Xiong J, Chi C, et al. Comparison of pulse wave velocity and pulse pressure amplification in association with target organ damage in community-dwelling elderly: the Northern Shanghai study. Hypertens Res. (2018) 41:372–81. doi: 10.1038/s41440-018-0027-3
33. Obayashi M, Kobayashi S, Nanno T, Hamada Y, Yano M. Relation between oscillometric measurement of central hemodynamics and left ventricular hypertrophy in hypertensive patients. Pulse. (2021) 9:116–24. doi: 10.1159/000520006
34. Ahn KT, Park KI, Kim MJ, Oh JK, Han JH, Kwon HJ, et al. Height and sex is strongly associated with radial augmentation index in Korean patients with never-treated hypertension. Clin Interv Aging. (2016) 11:415–22. doi: 10.2147/CIA.S101302
35. Butlin M, Qasem A. Large artery stiffness assessment using sphygmocor technology. Pulse. (2017) 4:180–92. doi: 10.1159/000452448
36. Zuo JL, Li Y, Yan ZJ, Zhang RY, Shen WF, Zhu DL, et al. Validation of the central blood pressure estimation by the Sphygmocor system in Chinese. Blood Press Monit. (2010) 15:268–74. doi: 10.1097/MBP.0b013e3283386866
37. Díaz A, Bia D, Zócalo Y. Impact of methodological and calibration approach on the association of central and peripheral systolic blood pressure with cardiac structure and function in children, adolescents and adults. High Blood Press Cardiovasc Prev. (2019) 26:509–34. doi: 10.1007/s40292-019-00346-0
38. Díaz A, Bia D. Association between central-peripheral blood pressure amplification and structural and functional cardiac properties in children, adolescents, and adults: impact of the amplification parameter, recording system and calibration scheme. High Blood Press Cardiovasc Prev. (2021) 28:185–249. doi: 10.1007/s40292-021-00440-2
39. Negishi K, Yang H, Wang Y, Nolan MT, Negishi T, Pathan F, et al. Importance of calibration method in central blood pressure for cardiac structural abnormalities. Am J Hypertens. (2016) 29:1070–6. doi: 10.1093/ajh/hpw039
40. Williams B, Lacy PS, Yan P, Hwee CN, Liang C, Ting CM. Development and validation of a novel method to derive central aortic systolic pressure from the radial pressure waveform using an n-point moving average method. J Am Coll Cardiol. (2011) 57:951–61. doi: 10.1016/j.jacc.2010.09.054
41. Wohlfahrt P, Krajčoviechová A, Seidlerová J, Mayer O, Bruthans J, Filipovský J, et al. Arterial stiffness parameters: how do they differ? Atherosclerosis. (2013) 231:359–64. doi: 10.1016/j.atherosclerosis.2013.10.006
42. Bia D, Zócalo Y. Physiological age- and sex-related profiles for local (Aortic) and regional (Carotid-Femoral, Carotid-Radial) pulse wave velocity and center-to-periphery stiffness gradient, with and without blood pressure adjustments: reference intervals and agreement between methods in healthy subjects (3–84 years). J Cardiovasc Dev Dis. (2021) 8:3. doi: 10.3390/jcdd8010003
43. Mancia G, Fagard R, Narkiewicz K, Redón J, Zanchetti A, Böhm M, et al. 2013 Esh/Esc guidelines for the management of arterial hypertension: the task force for the management of arterial hypertension of the european society of hypertension (Esh) and of the european society of cardiology (Esc). Am J Hypertens. (2013) 31:1281–357. doi: 10.1097/01.hjh.0000431740.32696.cc
Keywords: arterial stiffness, carotid-femoral pulse wave velocity, central blood pressure, target organ damage, risk factors
Citation: Bai Y, Wang Q, Cheng D, Hu Y, Chao H, Avolio A, Tang B and Zuo J (2022) Comparison of Risk of Target Organ Damage in Different Phenotypes of Arterial Stiffness and Central Aortic Blood Pressure. Front. Cardiovasc. Med. 9:839875. doi: 10.3389/fcvm.2022.839875
Received: 20 December 2021; Accepted: 14 March 2022;
Published: 14 April 2022.
Edited by:
Tzung Hsiai, University of California, Los Angeles, United StatesReviewed by:
Daniel Bia, Universidad de la República, UruguayMedine Cüre, Private Tanfer Hospital, Turkey
Constance G. Weismann, Lund University, Sweden
Copyright © 2022 Bai, Wang, Cheng, Hu, Chao, Avolio, Tang and Zuo. This is an open-access article distributed under the terms of the Creative Commons Attribution License (CC BY). The use, distribution or reproduction in other forums is permitted, provided the original author(s) and the copyright owner(s) are credited and that the original publication in this journal is cited, in accordance with accepted academic practice. No use, distribution or reproduction is permitted which does not comply with these terms.
*Correspondence: Biwen Tang, rossi120222@163.com; Junli Zuo, zuo-junli@163.com
†These authors have contributed equally to this work