Individual Variability in Migration Timing Can Explain Long-Term, Population-Level Advances in a Songbird
- 1Department of Biological Sciences, University of Manitoba, Winnipeg, MB, Canada
- 2Purple Martin Conservation Association, Erie, PA, United States
Migratory animals may be particularly at-risk due to global climate change, as they must match their timing with asynchronous changes in suitable conditions across broad, spatiotemporal scales. It is unclear whether individual long-distance migratory songbirds can flexibly adjust their timing to varying inter-annual conditions. Longitudinal data for individuals sampled across migration are ideal for investigating phenotypic plasticity in migratory timing programs, but remain exceptionally rare. Using the largest, repeat-tracking data set available to date for a songbird (n = 33, purple martin Progne subis), we investigated individual variability in migration timing across 7,000–14,000 km migrations between North American breeding sites and South American overwintering sites. In contrast to previous studies of songbirds, we found broad, within-individual variability between years in the timing of spring departure (0–20 days), spring crossing of the Gulf of Mexico (0–20 days), and breeding site arrival (0–18 days). Spring departure and arrival dates were fairly repeatable across years (depart r = 0.39; arrive r = 0.32). Fall migration timing was more variable at the individual level (depart range = 0–19 days; gulf crossing range = 1–15 days; arrive range = 0–24 days) and less repeatable, with fall crossing of the Tropic of Cancer being the least repeatable (r = 0.0001). In this first, repeat-tracking study of a diurnal migratory songbird, the high within-individual variability in timing that we report may reflect the greater influence of environmental and social cues on migratory timing, as compared to the migration of more solitary, nocturnally migrating songbirds. Further, large, within-individual variability in migration dates (0–24 days) suggest that advances in spring arrival dates with climate change that have been reported for multiple songbird species (including purple martins) could potentially be explained by intra-individual flexibility in migration timing. However, whether phenotypic plasticity will be sufficient to keep up with the pace of climate change remains to be determined.
Introduction
Phenotypic plasticity in animal migration timing could provide the means for rapid acclimation to environmental change, as compared to adaptive responses through genetic change (Charmantier and Gienapp, 2014). To what extent phenotypic plasticity and/or micro-evolution are the mechanisms responsible for population-level advances in the spring migration timing of some landbirds has been hotly debated (Knudsen et al., 2011; Charmantier and Gienapp, 2014). Steep population declines among migratory species (Both et al., 2010), lends urgency to determining whether constraints on adaptive timing are a contributing factor.
Longitudinal data are ideal for research on these themes because they provide the opportunity to investigate phenotypic variation within individuals in response to varying environmental conditions across years (Charmantier and Gienapp, 2014). For songbird migration, most previous studies have focused on the use of observational data to determine the individual repeatability (r) of spring migration departure and arrival dates. These studies report broad variation (r = 0.04–0.51), both within and among migratory species (Potti, 1998; Brown and Brown, 2000; Moller, 2001; Ninni et al., 2004; Cooper et al., 2009; Studds and Marra, 2011). Direct-tracking technologies (Stutchbury et al., 2009) provide the means to examine complete annual migration tracks, but studies where multiple migrations by the same individual are monitored are still rare (Both et al., 2016). In a Neotropical songbird (wood thrush, Hylocichla mustelina), within-individual spring migration timing was remarkably repeatable, with a mean difference of just 3 days in spring arrival date between years, suggesting limited plasticity (n = 10; Stanley et al., 2012). In a Palearctic example (red-backed shrikes, Lanius collurio), within-individual variability was similarly low, where mean within-individual differences were 3–12 days, and breeding arrival date (n = 2) varied by only 1–4 days (Pedersen et al., 2018). Thus, repeatability of migration timing of songbirds using direct tracking is generally reported to be high, particularly in spring. However, studies to date have relied on low sample size (<20 individuals), are thinly spread across species and migratory systems, and have focused on nocturnally migrating species (Both et al., 2016).
In many migratory species, population-level advances in spring migration timing have been observed over decadal scales and linked to temperature increase with climate change (Butler, 2003; Mayor et al., 2017; Lehikoinen et al., 2019). Across European and North American migratory landbird systems, the mean advance over several decades in spring migration timing was 1 week (Lehikoinen et al., 2019); with advances within some species reported to be >2 weeks (Butler, 2003). There is much debate as to whether these timing advances are the result of phenotypic plasticity, micro-evolutionary change, or both (Knudsen et al., 2011). For species with high, within-individual repeatability in migration timing, these rapid population-level advances in timing are difficult to reconcile. In Icelandic black-tailed godwits (Limosa limosa islandica), population-level arrival dates advanced by approximately 17 days over 20 years (Gunnarsson and Tomasson, 2011) but over this same time span individual arrival dates were highly consistent (r = 0.51; Gill et al., 2014). These results for black-tailed godwits suggest that advances were not driven by individual plasticity of adult migrants, but rather ontogenetic effects during development could underlie these rapid advances in timing (Gill et al., 2014). Such comparisons of individual variability vs. population-level advances in timing remain rare, and need to be further explored in other species and systems. There is currently a deficit of knowledge about the mechanisms of adaptive change (microevolutionary and/or phenotypic plasticity) in response to environmental conditions during migration (Pulido and Berthold, 2004) and the impact of longer-scale climatic effects on the flexibility of migration patterns (Knudsen et al., 2011), especially for long-distance migratory songbirds.
We investigated the phenotypic plasticity of migration timing by using a diurnal, long distance, Neotropical migratory songbird (purple martin, Progne subis) that travels 10, 000–20, 000 km annually between North American breeding sites and South American overwintering sites (Fraser et al., 2012, 2013a,b). Purple martins are aerial insectivores, and like other swallows, are thought to use a fly-and-forage strategy during their diurnal migration (Brown and Tarof, 2013). At the population-level, this species has advanced spring migration timing by 8–20 days over the last 100 years (Arab and Courter, 2015). However, arrival dates did not advance in response to a record-setting, early spring in 2012 (Fraser et al., 2013a). Data on the variation in individual timing across multiple migrations are therefore required to further investigate the potential for phenotypic plasticity in purple martins in response to environmental conditions and to determine whether this can explain long-term advances in timing. We used the largest repeat-track data set available for a songbird, comprised of 33 individuals tracked across 2 years by using light-level geolocators. Our objectives were to (1) determine within-individual variation and repeatability (r) in timing across both spring and fall migration, and (2) assess whether the degree of within-individual variability provides a potential mechanism to explain population-level advances in spring timing reported for martins and whether this species can serve as a model for similar investigations in other songbirds.
Methods
Geolocator Analysis Methods
Light-level geolocators were deployed on adult purple martins at 8 North American breeding sites (latitudinal range 38.36°N to 53.02°N; Supplementary Table 1) using a leg-loop backpack-style harness made of Teflon ribbon (Rappole and Tipton, 1991; Stutchbury et al., 2009) and retrieved in the following year (or subsequent year, n = 2) at the same locations (2009–2016). This study was conducted in accordance with the recommendations of the Ornithological Council's Guidelines to the Use of Wild Birds in Research' and was approved by the University of Manitoba and York University Animal Care Committees (2009- 2 W, F14-009/1–3).
We defined sunrises and sunsets (twilights) from the raw geolocator light data using the preprocessLight function in the R-package BAStag version 0.1.3 (Wotherspoon et al., 2016). We used a light intensity threshold of 32 to define the separation of day and night. Events that influenced the geolocator's light sensor outside of sunrise and sunset times (e.g., shading during day, light during night) indicated false twilights. We used the initiation of heavy shading (false twilights during daylight periods) in spring, that clearly indicated entrance and exits of nest cavities, to identify breeding arrival date. After defining arrival date, all false twilights were removed.
The twilight dataset was used to define daily locations and movement periods by using the R-package GeoLight version 2.0 (Lisovski and Hahn, 2012). We used the coord function to determine spatial coordinates throughout entire migratory tracks. We calculated an appropriate sun elevation angle using twilight data at each bird's known breeding location, before fall migration. Latitudes impacted by spring and fall equinox periods were omitted by using a tolerance level of 0.13 (Lisovski and Hahn, 2012). The resulting data were used to determine daily coordinates and movement periods and to identify spring and fall arrival and departure dates, as well as the date individuals crossed the Tropic of Cancer (23.4°N). We used the changeLight function to determine residency and movement periods, and shifts in latitudinal and longitudinal coordinates, to identify departure and fall arrival dates. We defined overwintering locations as a tenure of >7 days within the known non-breeding range. Most stopovers in this region were <7 days (Van Loon et al., 2017), thus this provided a conservative estimate of when birds had completed migration and arrived at their overwintering destination.
Repeatability of Spring and Fall Migration Phenology
To investigate if individuals are consistent in their migration timing between years, repeatability was examined for birds tracked for at least 2 years (individuals = 33, geolocator tracks = 67). We examined the repeatability of migration departure date (fall tracks = 66, spring tracks = 67), date passing the Tropic of Cancer (23.4°N; fall tracks = 34, spring tracks = 61), and date of arrival at the breeding grounds or overwintering grounds (fall tracks = 67, spring tracks = 67). We also included 144 single-tracked (1 year only) individuals in the analysis to better account for population level variability in the analysis, resulting in a total of 5–57 tracks per breeding location (Supplementary Table 1). Repeatability was calculated as the fraction of variation in behavior between individuals, as compared to the sum of phenotypic plasticity and measurement error (Nakagawa and Schielzeth, 2010). Repeatability is a proportion between 0 and 1, where low values indicate most of the variation is due to plasticity and error. The adjusted repeatability (value of repeatability calculated after controlling for confounding effects) of aspects of migration timing were calculated in linear, mixed-effects models using the package MCMCglmm (Hadfield, 2010). In this case the confounding effects of sex and age were set as fixed effects (males and older birds may have earlier timing) with year, individual, and breeding colony as random effects to control for repeated measures. We did not include temperature or other weather factors in our analysis owing to limitations in the number of repeat-track birds per site. Confidence intervals for repeatability were estimated by parametric bootstrapping with 1,000 replications. Results were replicated with an uninformed prior which produced quantitatively similar results (Table 1) with overlapping 95% credibility intervals. All analyses were done in R version 3.5.3 (R Core Team, 2018).
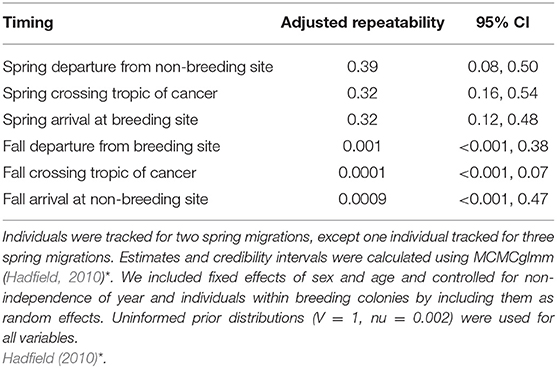
Table 1. Adjusted repeatability estimates and 95% credibility intervals for spring and fall migration (2008–2016) including departure dates (n = 67; n = 66), spring and fall crossing the Tropic of Cancer (n = 61, n = 34), and spring and fall arrival dates (n = 67; n = 67).
Results
We found that spring migration timing (departure, crossing 23.4°N, arrival) was more repeatable between years at the individual level than timing during fall migration (spring range, r = 0.32–0.39; fall range, r = 0.0001–0.001; Table 1, Figure 1). The timing of spring departure was the most consistent across years (r = 39, CI = 0.08–0.50), perhaps owing to strong endogenous control of migration initiation. Spring crossing of the Tropic of Cancer (23.4°N) and breeding arrival date were also fairly consistent across years (cross r = 0.32; arrive r = 0.32). Fall arrival date was much less repeatable than breeding arrival date (0.0009 vs. 0.32) (Figure 1). Variance explained by the random factors of colony and year ranged from 32.47 to 90.76 and 3.09–30.94, respectively (Supplementary Table 2). Age had a significant effect on timing across both spring and fall migration, with ASY birds departing and arriving earlier by 5.52–7.18 days as compared to SY birds. Sex impacted the timing of spring departure and spring cross only, with males migrating 6.46 and 6.08 days earlier than females (Supplementary Table 3).
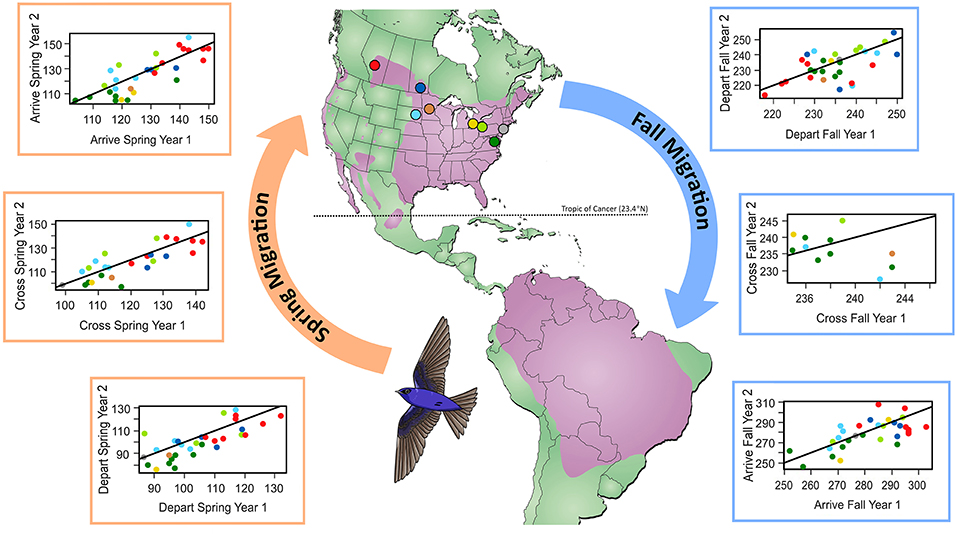
Figure 1. Comparison of migration timing (day of year) at the individual level for the first vs. second year of tracking. Colors show breeding locations where geolocators were retrieved and correspond to points indicated on the map. Line indicates 1:1, where points below the line indicate earlier migration in year two. The map shows the year-round purple martin range in purple (Brown and Tarof, 2013).
Within-individual variability between the first and second year of tracking was broad (0–24 days), with individual timing earlier (1–24 days), or later (1–23 days) in the second year of tracking (Figure 2). In spring, departure date varied by 0–20 days, spring crossing of 23.4°N by 0–20 days, and arrival at the breeding site by 0–18 days. Fall migration timing was generally more variable at the individual level (depart range = 0–19 days; cross range = 1–15 days; arrive range = 0–24 days). Overall within-individual variability over the year was therefore up to 24 days, which spans the 8–20 day population-level advance in spring arrival date over 100 years, reported for purple martins (Arab and Courter, 2015) (Figure 2).
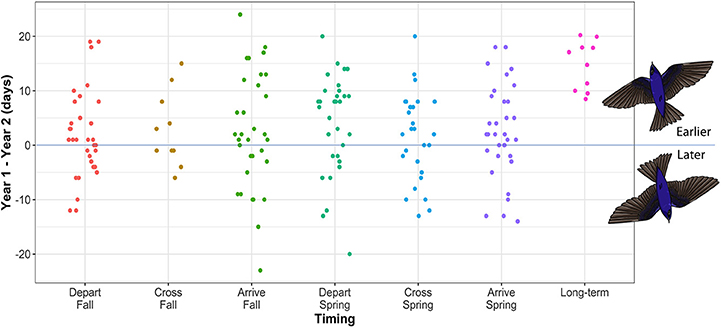
Figure 2. The difference in migration timing between year one and year two for individual purple martins tracked between North American breeding and South American overwintering sites. A positive value indicates earlier timing in the second year of tracking. Sample sizes for each timing category are as follows: depart fall = 32, cross fall = 10, arrive fall = 32, depart spring = 32, cross spring = 28, arrive spring = 33. Data show timing at migration start and end points, as well as the timing of crossing the Tropic of Cancer (23.4°N), in both spring and fall. Long-term data for mean breeding arrival date in spring across the range (from Arab and Courter, 2015) are included to enable comparison of these longer-term advances in timing over 100 years with short-term differences at the individual level (this study).
Discussion
Individual patterns of migration timing across years provide invaluable clues regarding the phenotypic plasticity of migration timing. We show that spring migration timing of a long-distance migratory songbird was more repeatable, from start-to-finish, than fall migration, with spring departure date being the most consistent between years. We found broad, within-individual variability in migration timing (year 1 as compared to year 2) at key points around the annual cycle (start and stop dates, and approximate midway points). We show that broad, intra-individual variability (up to 24 days earlier or 23 days later in the second year of tracking), was a feature of both spring and fall migration. Our results therefore suggest individual plasticity as a potential mechanism to account for population-level advances in spring arrival date (8–20 days) reported for purple martin (Arab and Courter, 2015). The degree of plasticity we show in individual martins also exceed mean population-level advancements (~1 week) for spring migration reported for North American and European migratory landbird systems, including several species of long-distance aerial insectivores (Lehikoinen et al., 2019).
It has been difficult to reconcile the decadal-scale advances in spring migration timing at the population level, with intra-individual data that show high consistency of migration timing (e.g., Gill et al., 2014). It is debated whether strong selection for advanced timing and rapid micro-evolution could be responsible for population-level change (Knudsen et al., 2011) because advances via these mechanisms are generally predicted to take much longer. For example, using quantitative genetic models an observed 14-days advance in laying date in great tits (Parus major) was estimated to require more than two centuries to attain via micro-evolution (Charmantier and Gienapp, 2014). The predicted time period is considerably longer than the scale of the 10–100-years advances reported for multiple landbird species across North American and European migration systems (Lehikoinen et al., 2019). If micro-evolutionary responses cannot occur this quickly (Charmantier and Gienapp, 2014), and individuals do not exhibit a high level of phenotypic plasticity in spring timing, then how do we explain population-level advances in timing over short timescales? Our results show, that at least in purple martins, individual variation is a potential explanation for the kinds of advances reported in spring timing (Arab and Courter, 2015). We found within individual variation of up to 24 days whereas population level advancements for this species over 100 years are between 8 and 20 days (Arab and Courter, 2015). Our results contrast those for Icelandic godwit, where consistency in individual timing precluded individual plasticity of adult birds as a viable explanation for population-level advances in timing (Gill et al., 2014). Further studies are required across species and systems to determine whether individual plasticity is a potential explanation for observed advances in timing. It would also be valuable for future studies to investigate the influence of additional factors on individual plasticity, such as nocturnal vs. diurnal migratory strategies, foraging guild, short vs. long-distance migration (Lehikoinen et al., 2019), nest strategy, and within-species patterns.
We found moderate repeatability of spring migration timing (range: r = 0.32–0.39) that is lower than reported in other studies of nocturnally migrating songbirds (e.g., range: r = 0.49–0.71, Stanley et al., 2012) and many long-distance migrants generally (Both et al., 2016). We found the highest repeatability of timing at spring departure from the non-breeding grounds as has been shown for red-backed shrikes, Icelandic whimbrels, and black-tailed godwits; perhaps owing to the strong role of endogenous cues in migration initiation (Gwinner, 1996; Pedersen et al., 2018; Carneiro et al., 2019; Senner et al., 2019). Sex and age were also important factors influencing spring departure timing, but differences between the sexes diminished by the time of arrival at breeding areas, whereas older birds were consistently earlier than younger ones. Relatively high repeatability of spring arrival date may reflect strong selection on timing at the breeding ground. In martins, high competition for nest cavities may further contribute to higher repeatability of spring arrival dates (Brown and Tarof, 2013). In contrast, fall timing was much less repeatable (r = 0.0001–0.001). Particularly low repeatability of fall migration arrival date (r = 0.0009), may reflect relaxed selection on this trait in purple martins; a species that is non-territorial in winter and joins large communal roosts, in contrast to a songbird that is territorial in winter, where repeatability was relatively high (r = 0.62; Stanley et al., 2012). Intra-individual variation in nest success may also have contributed to low fall repeatability values in our study, if birds with failed nests depart earlier than birds attending young from successful broods. The overall, lower repeatability of migratory timing in our study of a diurnal migrant as compared to results for some nocturnally migrating songbirds (Both et al., 2016) may be influenced by migratory strategy (diurnal vs. nocturnal). Phenological and repeatability studies of songbirds have tended to focus more on nocturnally migrating species and comparisons of short and long-distance migrants (Both et al., 2016; Lehikoinen et al., 2019), however, diurnal and nocturnal migrants may exhibit different amounts of plasticity in timing to environmental change and should be further investigated.
We found higher repeatability in spring than fall for crossing of the Tropic of Cancer (23.4°N), which is generally associated with crossing of the Gulf of Mexico in martins, as most individuals make a >800 km open-water crossing of this “barrier” during spring and fall migration (Fraser et al., 2013a,b). Lower repeatability in fall may indicate greater, population-level synchronization of the timing of crossing in this season. In wood thrushes, crossing of the gulf showed low repeatability during both spring and fall (r = 0.12, Stanley et al., 2012). In spring, the timing of gulf crossing in martins is not largely impacted by weather conditions (Abdulle and Fraser, 2018), thus we infer that higher consistency in individual timing at this barrier is a result of a carry-over effect of spring departure timing, rather than an influence of conditions at this stage of migration.
We speculate that the generally larger, intra-individual variation (up to 24 days) that we found for martins as compared to other songbirds (Stanley et al., 2012; Both et al., 2016; Pedersen et al., 2018), may reflect the nature of migration and social behavior in martins, and possibly other swallows. The intra-individual variability we found for purple martins is more similar to broad, within-individual variation recently shown for some shorebirds (Senner et al., 2019; Verhoeven et al., 2019), than to data reported for nocturnally migrating songbirds. Purple martins are diurnal migrants that roost in large flocks during stopovers across migration (Brown and Tarof, 2013), and may use island-like habitats for stopover (Fraser et al., 2017; Fournier et al., 2019). Large social aggregations and suitable stopover habitat are unevenly distributed across a migratory landscape, thus martin stopover decisions may be influenced by these social factors which could contribute to variation in their individual timing. In contrast, diurnal songbirds that migrate singly during the night may not require social stopover cues to the same degree, which may favor more independent and consistent migration schedules. While it has been demonstrated that short-distance migrants may exhibit swifter phenological shifts in response to environmental change than long-distance migrants (Hurlbert and Liang, 2012; Kullberg et al., 2015; Takuji et al., 2017), whether diurnal, long-distance migrants exhibit greater phenotypic plasticity than nocturnal ones would be valuable to determine.
Our data did not provide the opportunity to examine variation in repeatability across populations breeding at different latitudes, but such within-species investigations are an important frontier. Such research would be particularly important for purple martins and other aerial insectivores, where strong north-south patterns of population decline are reported (Nebel et al., 2010), and where relative limitations in behavioral plasticity between populations could be playing a role. More northern breeding populations may exhibit larger advances in spring arrival dates than more southern ones (Arab and Courter, 2015), which should be investigated in concert with individual-level patterns.
Conclusion
In an era of rapid, global environmental change, it is critical that we address the degree to which migratory birds can mount phenotypic responses to change. In this first investigation using a diurnal migrant and the largest repeat-tracking data set for a songbird, we show that phenotypic plasticity in migration timing is a potential mechanism to explain decadal-scale, population-level advancement in spring migration timing. It remains to be determined whether the degree of individual plasticity we show is connected to, or cued by, temperature and whether any advances in migration timing are sufficient to match advances in seasonal phenology of lower trophic levels. Future research should also investigate the role of environmental cues and other mechanisms contributing to within-individual variation in migration timing.
Data Availability
All datasets generated for this study are included in the manuscript and/or the Supplementary Files.
Ethics Statement
This study was conducted in accordance with the recommendations of the Ornithological Council's Guidelines to the Use of Wild Birds in Research' and was approved by the University of Manitoba and York University Animal Care Committees (2009- 2W, F14-009/1-3).
Author Contributions
KF, AS, and JS conducted fieldwork. KF, AS, EG, and CG analyzed the data. KF, AS, EG, JS, and CG wrote the manuscript.
Conflict of Interest Statement
The authors declare that the research was conducted in the absence of any commercial or financial relationships that could be construed as a potential conflict of interest.
Acknowledgments
For field assistance and support we thank Nanette Mickle, Paul Mammenga, Tim Shaheen, Kelly Applegate, Michael North, Larry Leonard, Edward Cheskey, Megan McIntosh, Pat Kramer, Cassandra Silverio, Lee Bakewell, Richard Doll, Myrna Pearman, Alisha Ritchie, Bridget Stutchbury, and John Tautin. Funding and support were provided by University of Manitoba, NSERC, Nature Canada, Central Lakes College, Minnesota Audubon, Minnesota Ornithologists' Union, Brainerd Lakes Audubon Society, Mille Lacs Band of Ojibwe, and Ellis Bird Farm.
Supplementary Material
The Supplementary Material for this article can be found online at: https://www.frontiersin.org/articles/10.3389/fevo.2019.00324/full#supplementary-material
References
Abdulle, S. A., and Fraser, K. C. (2018). Does wind speed and direction influence timing and route of a trans-hemispheric migratory songbird (purple martin) at a migration barrier? Anim. Migrat. 5, 49–58. doi: 10.1515/ami-2018-0005
Arab, A., and Courter, J. R. (2015). Spatio-temporal trend analysis of spring arrival data for migratory birds. Commun. Stat. Simul. Comput. 44, 2535–2547. doi: 10.1080/03610918.2013.809100
Both, C., Bijlsma, R. G., and Ouwehand, J. (2016). Repeatability in spring arrival dates in pied flycatchers varies among year and sexes. Ardea 104, 3–21. doi: 10.5253/arde.v104i1.a1
Both, C., Van Turnhout, C. A., Bijlsma, R. G., Siepel, H., and Van Strien, A. J. Foppen R. P. (2010). Avian population consequences of climate change are most severe for long-distance migrants in seasonal habitats. Proc. Biol. Sci. 277, 1259–1266. doi: 10.1098/rspb.2009.1525
Brown, C. R., and Brown, M. B. (2000). Weather-mediated natural selection on arrival time in cliff swallows. Behav. Ecol. Sociobiol. 47, 339–345. doi: 10.1007/s002650050674
Brown, C. R., and Tarof, S. (2013). “Purple martin (Progne subis),” in The Birds of North America, ed P. G. Rodewald. Ithaca: Cornell Lab of Ornithology.
Butler, C. (2003). The disproportionate effect of global warming on the arrival dates of short-distance migratory birds in North America. Ibis 145, 484–496. doi: 10.1046/j.1474-919X.2003.00193.x
Carneiro, C., Gunnarsson, T. G., and Alves, J. A. (2019). Why are whimbrels not advancing their arrival dates into Iceland? Exploring seasonal and sex-specific variation in consistency of individual timing during the annual cycle. Front. Ecol. Evol. 7:248. doi: 10.3389/fevo.2019.00248
Charmantier, A., and Gienapp, P. (2014). Climate change and timing of avian breeding and migration: evolutionary versus plastic changes. Evol. Appl. 7, 15–28. doi: 10.1111/eva.12126
Cooper, N. W., Murphy, M. T., and Redmond, L. J. (2009). Age- and sex-dependent spring arrival dates of eastern kingbirds. J Field Ornithol. 80, 35–41. doi: 10.1111/j.1557-9263.2009.00203.x
Fournier, A. M. V., Shave, A., Fischer, J., Siegrist, J., Ray, J., et al. (2019). Precise direct tracking and remote sensing reveal the use of forest islands as roost sites by Purple Martins during migration. J. Field Ornithol. doi: 10.1111/jofo.12298
Fraser, K. C., Shave, A., Savage, A., Ritchie, A., Bell, K., Siegrist, J., et al. (2017). Determining fine-scale migratory connectivity and habitat selection for a migratory songbird by using new GPS technology. J. Avian Biol. 48, 339–45. doi: 10.1111/jav.01091
Fraser, K. C., Silverio, C., Kramer, P., Mickle, N., Aeppli, R., and Stutchbury, B. J. (2013a). A trans-hemispheric migratory songbird does not advance spring schedules or increase migration rate in response to record-setting temperatures at breeding sites. PLoS ONE 8:e64587. doi: 10.1371/journal.pone.0064587
Fraser, K. C., Stutchbury, B. J., Silverio, C., Kramer, P. M., Barrow, J., Newstead, D., et al. (2012). Continent-wide tracking to determine migratory connectivity and tropical habitat associations of a declining aerial insectivore. Proc. Biol. Sci. 279, 4901–4906. doi: 10.1098/rspb.2012.2207
Fraser, K. C., Stutchbury, B. J. M., Kramer, P., Silverio, C., Barrow, J., Newstead, D., et al. (2013b). Consistent range-wide pattern in fall migration strategy of purple martin (Progne subis), despite different migration routes at the Gulf of Mexico. Auk 130, 291–296. doi: 10.1525/auk.2013.12225
Gill, J. A., Alves, J. A., Sutherland, W. J., Appleton, G. F., Potts, P. M., and Gunnarsson, T. G. (2014). Why is timing of bird migration advancing when individuals are not? Proc. R. Soc. B-Biol. Sci. 281:20132161. doi: 10.1098/rspb.2013.2161
Gunnarsson, T. G., and Tomasson, G. (2011). Flexibility in spring arrival of migratory birds at northern latitudes under rapid temperature changes. Bird Study. 58, 1–12. doi: 10.1080/00063657.2010.526999
Gwinner, E. (1996). Circadian and circannual programmes in avian migration. J. Exp. Biol. 199, 39–48.
Hadfield, J. D. (2010). MCMC methods for multi-response generalized linear mixed models: the MCMCglmm R package. J. Stat. Softw. 33, 1–22. doi: 10.18637/jss.v033.i02
Hurlbert, A. H., and Liang, Z. (2012). Spatiotemporal variation in avian migration phenology: citizen science reveals effects of climate change. PLoS ONE 7:e31662. doi: 10.1371/journal.pone.0031662
Knudsen, E., Linden, A., Both, C., Jonzen, N., Pulido, F., Saino, N., et al. (2011). Challenging claims in the study of migratory birds and climate change. Biol. Rev. Camb. Philos. Soc. 86, 928–946. doi: 10.1111/j.1469-185X.2011.00179.x
Kullberg, C., Fransson, T., Jonzén, N., Langvall, O., Nilsson, J., and Bolmgren, K. (2015). Change in spring arrival of migratory birds under an era of climate change, Swedish data from the last 140 years. Ambio 44, 69–77. doi: 10.1007/s13280-014-0600-1
Lehikoinen, A., Lindén, A., Karlsson, M., Andersson, A., Crewe, T. L., Dunn, E. H., et al. (2019). Phenology of the avian spring migratory passage in Europe and North America: asymmetric advancement in time and increase in duration. Ecol. Indicat. 101, 985–991. doi: 10.1016/j.ecolind.2019.01.083
Lisovski, S., and Hahn, S. (2012). GeoLight – processing and analysing light-based geolocator data in R. Methods Ecol. Evol. 3, 1055–59. doi: 10.1111/j.2041-210X.2012.00248.x
Mayor, S. J., Guralnick, R. P., Tingley, M. W., Otegui, J., Withey, J. C., Elmendorf, S. C., et al. (2017). Increasing phenological asynchrony between spring green-up and arrival of migratory birds. Sci. Rep. 7:1902. doi: 10.1038/s41598-017-02045-z
Moller, A. P. (2001). Heritability of arrival date in a migratory bird. Proc. Biol. Sci. 268, 203–6. doi: 10.1098/rspb.2000.1351
Nakagawa, S., and Schielzeth, H. (2010). Repeatability for Gaussian and non-Gaussian data: a practical guide for biologists. Biol. Rev. Camb. Philos. Soc. 85, 935–956. doi: 10.1111/j.1469-185X.2010.00141.x
Nebel, S., Mills, A., McCracken, J. D., and Taylor, P. D. (2010). Declines of aerial insectivores in North America follow a geographic gradient. Avian Conserv. Ecol. 5:1. doi: 10.5751/ACE-00391-050201
Ninni, P., Saino, N., Haussy, C., and Moller, A. P. (2004). Antioxidants and condition-dependence of arrival date in a migratory passerine. Oikos 105, 55–64. doi: 10.1111/j.0030-1299.2004.12516.x
Pedersen, L., Jackson, K., Thorup, K., and Tottrup, A. P. (2018). Full-year tracking suggests endogenous control of migration timing in a long-distance migratory songbird. Behav. Ecol. Sociobiol. 72, 72–139. doi: 10.1007/s00265-018-2553-z
Potti, J. (1998). Arrival time from spring migration in male pied flycatchers: individual consistency and familial resemblance. Condor 100, 702–08. doi: 10.2307/1369752
Pulido, F., and Berthold, P. (2004). Microevolutionary response to climate change. Adv. Ecol. Res. 35, 151–83. doi: 10.1016/S0065-2504(04)35008-7
R Core Team (2018) R: A Language and Environment for Statistical Computing. Vienna: R Foundation for Statistical Computing. Available online at: https://www.R-project.org
Rappole, J. H., and Tipton, A. R. (1991). New harness design for attachment of radio transmitters to small passerines. J. Field Ornithol. 62, 335–37.
Senner, N. R., Verhoeven, M., Abad-Gómez, A., Alves, J. A., Hooijmeijer, J. C. E. W, Howson, R. A., et al. (2019). High migratory survival and highly variable migratory behavior in black-tailed godwits. Front. Ecol. Evol. 7:96. doi: 10.3389/fevo.2019.00096
Stanley, C. Q., MacPherson, M., Fraser, K. C., McKinnon, E. A., Stutchbury, B. J., et al. (2012). Repeat tracking of individual songbirds reveals consistent migration timing but flexibility in route. PLoS ONE 7:e40688. doi: 10.1371/journal.pone.0040688
Studds, C. E., and Marra, P. P. (2011). Rainfall-induced changes in food availability modify the spring departure programme of a migratory bird. Proc. Biol. Sci. 278, 3437–3443. doi: 10.1098/rspb.2011.0332
Stutchbury, B. J., Tarof, S. A., Done, T., Gow, E., Kramer, P. M., Tautin, J., et al. (2009). Tracking long-distance songbird migration by using geolocators. Science 323:896. doi: 10.1126/science.1166664
Takuji, U. S., Butchart, H. M., and Phillimore, A. B. (2017). Temporal shifts and temperature sensitivity of avian spring migratory phenology: a phylogenetic meta-analysis. Anim. Ecol. J. 86, 250–261. doi: 10.1111/1365-2656.12612
Van Loon, A., Ray, J. D., Savage, A., Mejeur, J., Moscar, L., Pearson, M., et al. (2017). Migratory stopover timing is predicted by breeding latitude, not environmental variability, in a long-distance migratory songbird. J. Ornithol. 158, 745–752. doi: 10.1007/s10336-017-1435-x
Verhoeven, M. A., Loonstra, A. H. J., Senner, N. R., McBride, A. D., Both, C., and Piersma, T. (2019). Variation from an unknown source: large inter-individual differences in migrating black-tailed godwits. Front. Ecol. Evol. doi: 10.3389/fevo.2019.00031
Keywords: phenotypic plasticity, spring phenology, repeatability, climate change, avian, long-distance migration, songbird
Citation: Fraser KC, Shave A, de Greef E, Siegrist J and Garroway CJ (2019) Individual Variability in Migration Timing Can Explain Long-Term, Population-Level Advances in a Songbird. Front. Ecol. Evol. 7:324. doi: 10.3389/fevo.2019.00324
Received: 27 March 2019; Accepted: 13 August 2019;
Published: 06 September 2019.
Edited by:
Brett K. Sandercock, Norwegian Institute for Nature Research (NINA), NorwayReviewed by:
Jason Courter, Malone University, United StatesKristen Covino, Loyola Marymount University, United States
Copyright © 2019 Fraser, Shave, de Greef, Siegrist and Garroway. This is an open-access article distributed under the terms of the Creative Commons Attribution License (CC BY). The use, distribution or reproduction in other forums is permitted, provided the original author(s) and the copyright owner(s) are credited and that the original publication in this journal is cited, in accordance with accepted academic practice. No use, distribution or reproduction is permitted which does not comply with these terms.
*Correspondence: Kevin C. Fraser, kevin.fraser@umanitoba.ca