Avian Use of Agricultural Areas as Migration Stopover Sites: A Review of Crop Management Practices and Ecological Correlates
- 1School of Biological Sciences, University of Utah, Salt Lake City, UT, United States
- 2Department of Surgery, University of Utah Health, Salt Lake City, UT, United States
- 3College of Sciences, Koç University, Istanbul, Turkey
An estimated 17% of migratory bird species are threatened or near threatened with extinction. This represents an enormous potential loss of biodiversity and cost to human societies due to the economic benefits that birds provide through ecosystem services and ecotourism. Conservation of migratory bird species presents many unique challenges, as these birds rely on multiple geographically distinct habitats, including breeding grounds, non-breeding grounds, and stopover sites during migration. In particular, stopover habitats are seldom studied relative to breeding and non-breeding habitats, despite their importance as refueling stations for migratory birds. In this study, we summarize the current research on the use of temporary primary crops by birds during migration and we assess the species characteristics and agricultural practices most often associated with the use of cropland as stopover habitat. First, we conducted a systematic review of the literature to document the effects various farming practices and crop types have on the abundance and diversity of migratory birds using agricultural areas for stopovers. Second, we analyzed the ecological correlates of bird species in the Northern Hemisphere that predict which species may use these areas while migrating. We ran a GLMM to test whether primary diet, diet breadth, primary habitat, habitat breadth, or realm predicted stopover use of agricultural areas. Our review suggests that particular crop types (principally rice, corn, and sunflower), as well as farming practices that result in higher non-cultivated plant diversity, encourage the use of agricultural areas by migrating birds. We found that cropland is used as stopover habitat by bird species that can utilize a large breadth of habitats, as well as species with preferences for habitat similar in structure to agricultural areas.
Introduction
There are nearly 2,000 long-distance migratory bird species worldwide (Horns and Şekercioğlu, 2018) which exhibit a wide diversity of behaviors, ecological requirements, migratory strategies, and conservation statuses (Rabenold and Rabenold, 1985; Şekercioğlu, 2007; Egevang et al., 2010; Horns and Şekercioğlu, 2018). Although on a global scale migratory birds are less threatened with extinction than are sedentary birds, in recent years the proportion of threatened and near threatened migratory bird species has increased at a much faster rate, from 10% (Şekercioğlu, 2007) to 16% (Horns and Şekercioğlu, 2018), and the number of migratory bird species threatened by global change is likely to be greatly underestimated (Zurell et al., 2018). Conservation of migratory birds is challenging because these species rely on multiple, geographically distinct habitats, including breeding grounds, non-breeding grounds, and stopover sites during migration (Galarza and Tellería, 2003; Newton, 2004; Dänhardt et al., 2010). Migration is increasingly recognized as the most challenging and dangerous period for the adults of migratory species (Carlisle et al., 2009). Consequently, in the last few decades, an increasing number of studies have focused on better understanding the importance of migratory stopover sites (Hutto, 1998; Weber et al., 1999; Carlisle et al., 2009; Greco and Airola, 2018; Weithman et al., 2018).
Migratory birds (hereafter “migrants”) spend up to one-third of each year migrating (Keast and Morton, 1980). Since most species cannot go from breeding to non-breeding grounds in a single flight, individuals alternate between periods of active migration and temporary suspensions of their migration for feeding, resting, recovering, and waiting for favorable weather conditions (hereafter “stopovers”) (Piersma, 1987; Hutto, 1998; Rosenberg et al., 2017; Linscott and Senner, 2021). Migrants rely heavily on stopover sites to quickly replenish fat stores (Péron et al., 2007). Timewise, up to 85% of a bird’s migration is spent at stopover sites (Hedenström and Alerstam, 1997; Green et al., 2002; Schmaljohann et al., 2012; Roques et al., 2020).
Stopovers can be differentiated into three major types of stops: “fire escapes,” “convenience stores,” and “full-service hotels” (Mehlman et al., 2005). “Fire escapes” are used as last minute emergency stops before significant barriers like oceans or deserts. Since the risk of mortality without these stops is high, individuals choose any area before these barriers to refuel, even if these birds are unlikely to fully replenish their resources. “Convenience store” stopover sites are used for brief (under 2 days) rests in suboptimal habitat, usually surrounded by generally inhospitable habitat. Lastly, “full-service hotels” are stops in optimal habitats where the birds can fully replenish their supplies and are generally longer than the “convenience store” stops (Mehlman et al., 2005). The duration of stopovers is positively related to body mass gain (Schmaljohann and Eikenaar, 2017; Roques et al., 2020), but lengthening stopover duration can be detrimental for overall fitness by increasing both migration duration and the likelihood of predation at stopover sites (Lank et al., 2003). Therefore, any loss, change, or degradation of these stopover areas can have large scale negative consequences for a migratory population (Weber et al., 1999; Grishchenko and Prins, 2016). The locations where birds choose to stopover are not random, and particular habitats are selected, while others are avoided (Loria and Moore, 1990; Martin and Karr, 1990; Moore, 1991; Mabey et al., 1993; Moore and Aborn, 2000). Many migrants that have specific habitat requirements on their breeding and non-breeding grounds will make use of a wider range of environments during migration (Petit, 2000). Unfortunately, for most species, the habitats used as stopover sites are understudied relative to the habitats used for breeding and non-breeding grounds (Hutto, 1998; Carlisle et al., 2009).
Anthropogenic land use is a major driver of habitat loss, and increasing levels of human development are correlated with declines in density and diversity for most bird species (Clergeau et al., 1998; Joyce et al., 2018). Agricultural land makes up a substantial share of human land use. Since 1961, the percent of agricultural land has increased from 35.6 to 37.4% of the world’s land area (World Bank, 2020), while agriculture has intensified and traditional agriculture has declined (Marini et al., 2011). Nevertheless, agricultural areas are more suitable habitat than cities or suburbs for many birds (La Sorte et al., 2014), and thus farmland may function as suitable habitat for birds during stopover.
Within agriculture, most crops can be readily categorized into two groups, temporary and permanent. Temporary primary crops are defined by the Food and Agriculture Organization of the United Nations (FAO) as seasonal crops that come directly from the land without any real processing, and include cereals, pulses, roots and tubers, sugar crops, temporary oil-bearing crops, temporary fiber crops, vegetables, tobacco, and fodder crops (FAO, 2021). Permanent crops include orchards, fruits, nuts, permanent oil-bearing crops, spices, and other perennial crops. Since temporary primary crops are usually seasonal or annual, crop type and farm practices can be readily changed from year to year. Furthermore, as of 2014, temporary crops made up 1.3 billion hectares of land use (12.5% of all habitable land) in agricultural areas (Ritchie and Roser, 2013).
One third of all bird species have been recorded in agricultural areas (Şekercioğlu et al., 2007) and avian use of primary temporary crops is well documented, particularly for certain crops such as sugarcane (Alexandrino et al., 2019) and rice (Elphick et al., 2010; Masero et al., 2011; Elphick, 2015). For birds, temporary primary crops can provide food in the form of seeds, the plants themselves, or vertebrates and invertebrates that live among the crops (Stafford et al., 2010). Furthermore, crops provide shelter from predators and places to rest (Hutto, 1998; Linscott and Senner, 2021). Research focusing on rice paddies has revealed extensive use of this habitat by migrating birds in the United States, Europe, and East Asia, even suggesting some of these areas should formally be designated as “Important Bird and Biodiversity Areas” for bird diversity and conservation (Acosta et al., 2010; Masero et al., 2011; Johnson et al., 2012; Yamaguchi et al., 2012; Grishchenko et al., 2019). However, to our knowledge, the use of other dominant crop types by migrating birds, particularly other forms of temporary primary crops, such as corn and sunflower, have yet to be formally reviewed despite the fact that they represent a large percentage of global land cover and are continuing to expand rapidly (Ramankutty and Foley, 1999).
Migratory species differ substantially in their diets, habitat preferences, and migration strategies (Alerstam and Lindström, 1990). The number and duration of stopovers are usually optimized to match with seasonal variation in food availability (Alerstam and Lindström, 1990; Sanz et al., 2003; Baker et al., 2004; Becker et al., 2008; Roques et al., 2020). Given this variation in stopover strategies (Loria and Moore, 1990; Martin and Karr, 1990; Moore, 1991; Mabey et al., 1993; Moore and Aborn, 2000), it is important to understand which traits predict stopover usage. Risk of extinction has been shown to be non-randomly distributed among avian families (Bennett and Owens, 1997), and there are significant relationships between avian ecological traits and extinction risk (Henle et al., 2004; Şekercioğlu et al., 2004; Şekercioğlu, 2011, 2012; Wang et al., 2015, 2018), including migratory behavior (Horns and Şekercioğlu, 2018). Since stopover sites are also not randomly distributed, it is critical to understand the ecological traits and other characteristics of migratory birds that predict their stopover use of agricultural fields.
In this study, we summarize the research on the use of temporary primary croplands by Palearctic and Nearctic birds during migration, and the avian species characteristics and agricultural practices most often associated with the use of croplands as bird stopover habitat. We first synthesize the existing literature on how crop types and management practices can affect the abundance and diversity of species that use temporary primary crops during migration. Our second aim is to understand the ecological correlates, such as habitat and diet preferences, that predict which species may use these areas during migration. This understanding can provide critical guidance for improving migratory bird conservation in agricultural areas.
Materials and Methods
Literature Review
We conducted a systematic search of the peer-reviewed literature published between 1900 and 2020, using search terms related to temporary primary crops and stopover events by avian migrants (Supplementary Table 1). These terms were used as keywords in a search of the ISI Web of Knowledge Complete Collection database. Each article was reviewed to confirm that it discussed temporary primary crops and stopover during avian migration (Supplementary Table 2). We defined the term stopover as a bird being recorded doing activities such as feeding, or roosting in areas between their breeding and non-breeding habitats during migration regardless of duration (Mehlman et al., 2005). All species are listed in Supplementary Table 3.
Data Selection
For our analyses, we compiled a dataset of migratory bird species from the literature and used ecological traits to predict the use of agricultural areas for stopover by these species. We used primary diet, diet breadth, primary habitat, habitat breadth, and biogeographic realm of these bird species to understand which ecological correlates are important in predicting which birds use these sites. We compiled ecological and conservation status data on the world’s birds from Bird Life International (2020) and BirdBase, a dataset of ecological and life-history traits collected from the ornithological literature, including field guides and other ornithological books (e.g., Del Hoyo et al., 1992–2013; Robson, 2014, and the Birds of the World, Billerman et al., 2020; see Şekercioğlu et al., 2004, 2019 for further details on this dataset). We first narrowed our dataset to all migratory species which were considered “Full Migrants” by Bird Life International (2020) or “Full” or “Partial” migrants by BirdBase. The analysis was further restricted to Nearctic and Palearctic migrants, as there is minimal literature outside of these biogeographic realms on migratory bird stopover events in agricultural areas. We also removed all pelagic families from our analyses, as their migrations rarely take them over land.
For each of these migratory species, we collected data on taxonomic family, primary diet, primary habitat, diet breadth, habitat breadth, and threat status (Supplementary Table 4). Taxonomic family was obtained from the Handbook of the Birds of the World and BirdLife International Digital Checklist of the Birds of the World v4 (updated December 2019). Primary diet was categorized from BirdBase into one of nine groups: invertivore, piscivore, granivore, vertivore, generalist herbivore, omnivore, nectarivore, frugivore, or scavenger. If no single food type comprised the majority of a species’ diet, then that species was assigned as a carnivore, herbivore, or omnivore depending on the contents of its diet. Diet breadth is the number of different main food types an individual species is known to consume, such as fruits, seeds, nectar, invertebrates, or fish. Primary habitat is categorized from BirdBase into one of the following main habitat types: wetland, forest, grassland and plains, coastal, woodland, shrub, riparian, savannah, artificial, rocky, and desert. Habitat breadth is the number of habitats from the above groups an individual species is known to inhabit. Threat status was obtained from the IUCN Red List of Threatened Species (IUCN, 2019). We considered threatened species as those with IUCN red list categories of vulnerable (VU), endangered (EN), and critically endangered (CE). Non-threatened species were those with the IUCN red list categories of least concern (LC) and near threatened (NT).
Finally, we determined through an exhaustive literature review whether a species is known to use temporary primary cropland as stopover habitat, i.e., the species was recorded in cropland during the migratory season in at least one of the reviewed studies. This was to ensure that species used in our analyses that were recorded from agricultural fields were in fact stopover migrants and not residents.
Stopover Analyses
We conducted a generalized linear mixed model (GLMM) using a binomial error structure, based on all Nearctic and Palearctic migratory species. The binary response variable denoted whether each species has been reported to stopover in temporary primary croplands. We included primary diet, diet breadth, primary habitat, habitat breadth and biogeographic realm (Nearctic, Palearctic, or both, i.e., Holarctic) as fixed effects, with the family as a random effect to control for similarities among closely related species (Bevilacqua et al., 2012). Next, we used likelihood ratio tests to assess the significance of the categorical variables of the model to test the ability of each predictor variable to independently explain variation.
To assess the usage of cropland by each taxonomic family, primary habitat, habitat breadth, primary diet, diet breadth, and biogeographic realm, we used an exact binomial test to compare the proportion of species in each category that stopover in cropland with the overall proportion of species that stopover in cropland. We also conducted a Welch Two-Sample t-test to understand if the threat status of migrants that used agricultural areas for stopovers was different than those that didn’t use agricultural areas.
To better understand if species within families that spanned both realms differed in their usage of agricultural areas, we performed a post hoc analysis comparing families with more than eight species in both Nearctic and Palearctic realms. For this analysis we used an exact binomial test. We chose to include families with more than eight species in each realm, as this was the smallest sample size for which our other results provided significant results. Eight families were included in the analysis: Anatidae, Rallidae, Scolopacidae, Laridae, Ardeidae, Accipitridae, Turdidae, and Fringillidae.
All statistical analyses were conducted in R (version 4.0.2; R Core Team, 2020) using the packages lme4 (Bates et al., 2015), LmerTest (Kuznetsova et al., 2017), and lmtest (Zeileis and Hothorn, 2002).
Results
Characteristics of Migratory Bird Stopover Sites in Croplands
Crop Type
Rice is one of the most important agricultural crops to migrating birds, providing a valuable food source and habitat (Elphick, 2010). Rice is cultivated worldwide and is one of the most abundant temporary primary crops, constituting over 1% of the world’s ice free lands (Maclean et al., 2002). Almost 86% of rice paddies are flooded, which offers protection from most weeds for the farmers, and adds to the conservation value of the crop (Chang and Luh, 1991; Elphick, 2010). This conservation value is increased in areas where natural wetlands have declined drastically, and rice fields act as the only similar available habitat (Day and Colwell, 1998; Elphick, 2010). After harvest, most rice fields outside of the tropics are left fallow in the non-growing season. These flooded rice fields are similar to wetlands and are used by waterfowl, wading birds, shorebirds, and cranes, as well as non-waterbirds (Elphick, 2004). Postharvest rice paddies provide migrating species with spilled grain, seeds of weeds, rice plants, and aquatic invertebrates to feed on (Stafford et al., 2010). Likewise, leftover stubble from the harvest provides a mosaic of vegetation similar in structure to “hemi-marshes” (Weller and Spatcher, 1965; Kaminski and Prince, 1981; Smith et al., 2004). Rice fields also offer food and shelter in the spring (Ibáñez et al., 2011). Rice paddies have become vital habitat to many migrating bird species by having habitat similar to rapidly declining wetland habitats and by providing a wide variety of foraging and roosting opportunities.
After rice, corn (maize) and sunflower are the temporary primary crop types most often identified as being preferred by migratory birds. These crops are actively selected over other crop types by numerous waterfowl, crane, raptor, and passerine species (Galle et al., 2009; Hagy et al., 2010; Pearse et al., 2011; Cai et al., 2014; Krapu et al., 2014). Corn in particular can constitute up to 90% of ingested material in cranes (Gruidae) and geese (Anatidae), while other crops generally make up less than 10% of ingested material (Krapu et al., 2014, 1995). Both corn and sunflower seeds contain relatively high amounts of metabolizable energy (Joyner et al., 1987; Galle et al., 2009), which may explain this food preference in birds. Besides providing food for migrants, certain crops also retain postharvest three-dimensional structure (e.g., corn and sunflower stalks are left standing after harvest) for use in the fall. These more complex environments are preferred by songbirds (Passeriformes) due to better roosting habitat and protection from predators (Galle et al., 2009).
In some cases, birds may select other crop types, but this preference is limited to a few species. Soybean, for example, is used extensively by waders, such as sandpipers and plovers (Scolopacidae and Charadriidae; Jorgensen et al., 2007; Stodola et al., 2014), for feeding and resting, but is avoided by other groups (LaGrange and Dinsmore, 1989; Krapu, 2004; Galle et al., 2009; Pearse et al., 2010; Krapu et al., 2014). This avoidance by most families may be due to the recent shift toward the use of genetically modified (GM) strains of soybean (Krapu, 2004). GM crops have more efficient means of weed control, which reduces the variety of food types available to migrating birds (Heard et al., 2003), and thereby may lessen the appeal of these GM soybean fields to birds. Canola is strongly preferred by several species of finch (Fringillidae), despite covering a small proportion of the landscape (Lindstrom and Alerstam, 1986; Lindström, 1990, 1989). Meanwhile, curlews (Scolopacidae) and cranes prefer to forage in wheat and alfalfa fields (Reinecke and Krapu, 1986; Leito et al., 2008; Shuford et al., 2013). Both of these groups are known to ingest crop seeds (Reinecke and Krapu, 1986; Shuford et al., 2013; Krapu et al., 2014), although it is likely that a substantial proportion of their time in farmland is also spent foraging on invertebrates that are found in the fields (Reinecke and Krapu, 1986). By planting crops preferred by migrants, like rice, corn, soybean, and canola, and allowing crops to retain their postharvest three-dimensional structure throughout the fall migration period, farmers can increase the utility of their fields to migrating birds by providing both food and shelter.
Farm Management
Migrating and breeding birds prefer farms with a higher diversity of wild plant species (Wilcoxen et al., 2018). Organic agriculture often supports a larger amount of plant diversity both within the fields due to a decrease in herbicide use, and in the surrounding landscape (Beecher et al., 2002). However, when organic farms were directly compared to traditional farms with similar plant diversity in the surrounding landscape, there was no difference in species richness of migrant birds between farm types (Jones et al., 2005; Kirk and Lindsay, 2017). Despite this, there is evidence that some granivores and invertivores are more likely to utilize organic over non-organic fields (Dänhardt et al., 2010). Diverse vegetation provides a broader range of habitat, higher potential for predator avoidance, and a higher diversity of food sources (Schaaf et al., 2008; Robertson et al., 2011; Rosin et al., 2012). Thus, if species are selecting for organic farms, they may be responding to plant heterogeneity both within the crops and in the surrounding landscape.
Retention of edge habitat, i.e., thin bands of native trees or shrubs that line farm borders, is highly associated with crop use by migrant birds (Koford and Best, 1996; Beecher et al., 2002; Rodewald and Brittingham, 2002; Yong and Finch, 2002; Rodewald et al., 2004). The abundance and diversity of food resources is generally higher along field edges (Rodewald and Brittingham, 2002; Foster, 2007), the habitat itself there is more heterogeneous, and therefore supports a higher diversity of species (Farina, 1988; Duckworth, 1994; Sapir et al., 2004). Likewise, farms located near riparian zones or wetlands tend to have higher concentrations of waterfowl, cranes, and waders that use the area to feed and roost (Pearse et al., 2013; Beatty et al., 2014; Bengtsson, 2016; Si et al., 2018). The presence of these habitats appears to allow riparian-dependent species to adequately replenish fat reserves regardless of the intensity of the surrounding agriculture (Janke et al., 2019). For many bird species, use of agricultural areas for stopovers may be due to edges, ecotones and surrounding habitat, and these birds’ association with cropland may be secondary. Retention of native vegetation within croplands not only provides important stopover habitat, but also increases per hectare farm yield through avian and other pollinator ecosystem services (Şekercioğlu, 2006; Pywell et al., 2015; Şekercioğlu et al., 2016).
Furthermore, the use of fallow fields in agriculture is rising (Traba and Morales, 2019). Fallow land is the cultivated land that is not seeded for one or more growing seasons (FAO, 2018). The use of fallow fields within a crop rotation can increase soil nutrients (Mertz et al., 2008). For birds that depend on temporary primary crops for stopovers, a shift from agriculture to fallow fields may have impacts on their migration patterns. For example, after the collapse of the USSR, farmland in northern Russia was mostly abandoned. As the fields transitioned into forests, Greater White-fronted Geese (Anser albifrons) shifted their stopover sites south to locations with more cropland (Grishchenko and Prins, 2016). Shifts away from farmland by geese can occur within 3 years of the fields laying fallow (Grishchenko and Prins, 2016). An increase in fallow fields has contributed to a decline in the numbers of birds using agricultural areas in Spain (Traba and Morales, 2019). However, due to the higher heterogeneity of plants within fallow fields, these practices may be beneficial to other bird species, including birds that do not rely on flooded fields (Wilcoxen et al., 2018). Furthermore, the increase in plant structural diversity within fallow fields compared to tilled fields improves these fields’ suitability for generalist birds (Bryan and Best, 1994; Hultquist and Best, 2001; Galle et al., 2009).
Management of postharvest rice fields can strongly affect their suitability as stopover site. Incomplete postharvest burns prior to flooding maintain a high abundance of waste rice and provide habitat similar to wetlands (Weller and Spatcher, 1965; Kaminski and Prince, 1981; Smith et al., 2004; Kross et al., 2008; Stafford et al., 2010). Likewise, the level of flooding can impact the value of the fields to migrants, with full flooding of the paddies correlating with higher bird diversity than that of dry or puddled fields (Day and Colwell, 1998). In the spring, water management, pesticide use, and field structure all affected the density of birds that utilized the paddies. Avian diversity and density were positively correlated with how long fields were flooded (Ibáñez et al., 2011). However, pesticides, herbicides, and taller, more dense vegetation reduced available food resources for most waterbirds (Ibáñez et al., 2011).
Besides limiting food resources (Ibáñez et al., 2011), pesticides in cropland can also affect migrant bird behavior during stopovers. For example, the ingestion of neonicotinoid insecticides by White-crowned Sparrows (Zonotrichia leucophrys) caused a rapid reduction in food consumption, body mass, and fat levels, and significantly affected their probability of departure (Eng et al., 2019). Birds that were exposed to high doses of the neonicotinoids stayed 3.5 days longer at the site of capture than at control sites (Eng et al., 2019). Furthermore, birds that ingested chlorpyrifos were significantly impaired and had trouble orienting correctly (Eng et al., 2019). This increase in stopover duration may put these birds at a disadvantage in competing for good breeding or non-breeding sites and may decrease their overall fitness. Pesticides may also affect a bird’s ability to fly even after they recommence their migration (Goulson, 2013; Addy-Orduna et al., 2019; Franzen-Klein et al., 2020), further affecting their overall fitness and increasing their risk of mortality.
Stopover Analyses
Our literature review revealed 385 papers that had the potential to contain information about stopover use of agricultural fields by migratory birds. Only 43 papers covered areas outside the Holarctic, and we did not utilize these papers in our study. Of the world’s nearly 2,000 migratory bird species, 1,122 species are non-pelagic migrant species in the Nearctic or Palearctic regions (Supplementary Table 4). There are 375 non-pelagic migratory species of birds that occur only in the Nearctic, and the region was the focus of 161 papers. Additionally, there are 634 non-pelagic migratory species that only occur in the Palearctic, and the region was the focus of 183 papers. Finally, there are 113 non-pelagic migratory species that were found in both realms. Our literature review revealed 335 species that were recorded making a stopover in primary temporary croplands (Supplementary Table 3). Of those, 100 were found in the Palearctic, 175 were found in the Nearctic, and 43 were found in both. The 17 species that were not from Palearctic or Nearctic realms were not included in the analysis. We believe that the small number of species from realms other than the Palearctic or Nearctic was due to limited research conducted in those areas. Including them in our results and expanding the analysis to include birds from all realms would bias our results. The proportion of Nearctic species (Figure 1; 46.6%, p < 0.001) and Holarctic species (Figure 1; 38.1%, p = 0.016) that utilized agricultural areas as stopover sites was significantly higher than average, while the proportion of Palearctic species (Figure 1; 15.8%, p < 0.001) was below average.
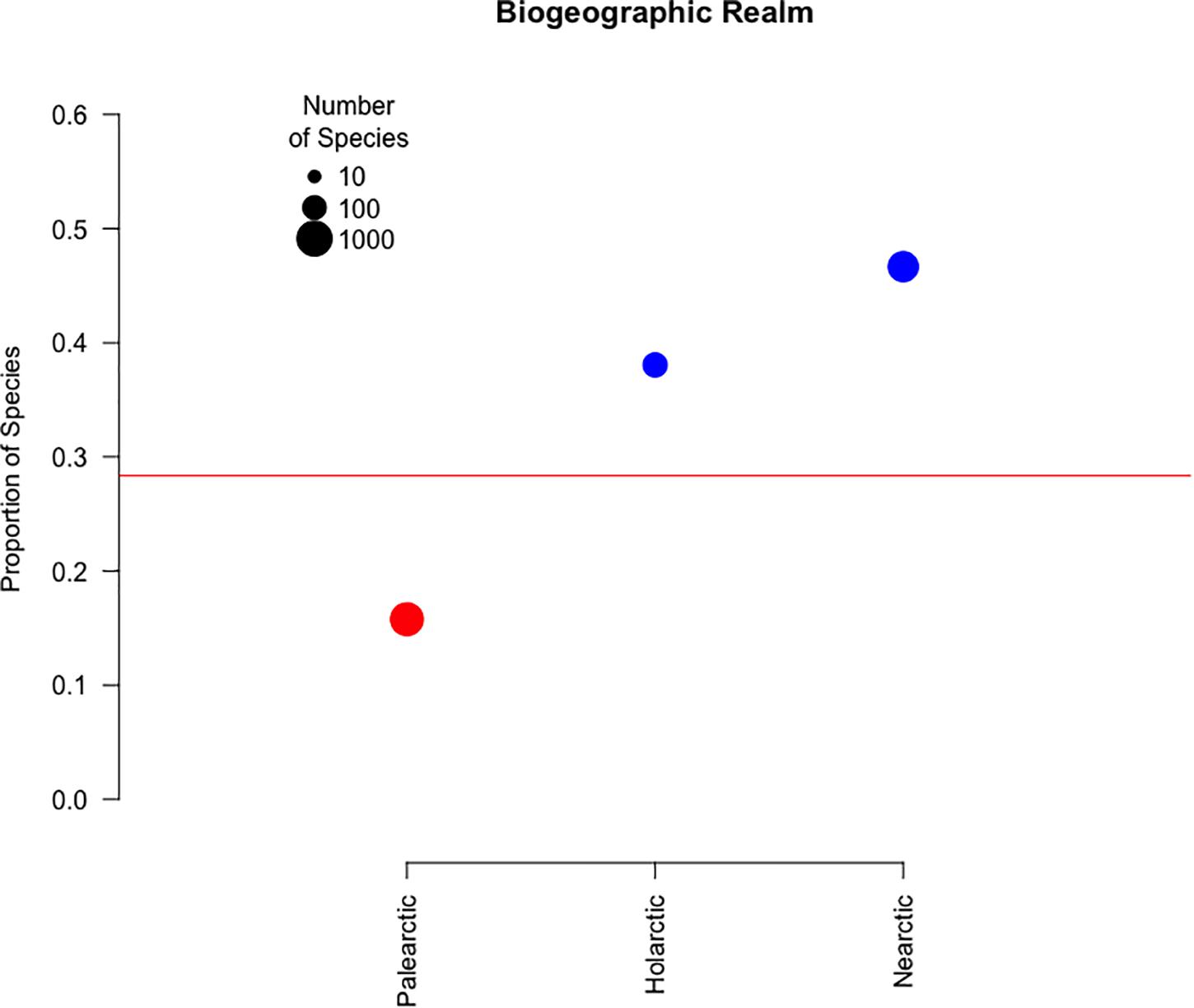
Figure 1. The proportion of species that utilize temporary primary croplands by biogeographic realm. Point size indicates the total number of species within a category. Color represents the proportion of species within each group associated with cropland compared to the average association for migratory birds overall shown as a red line (28.3%): blue indicates a significantly higher than average proportion, red indicates a significantly lower than average proportion, and black indicates non-significant results.
We found that primary habitat (Figure 2A; χ2 = 35.228, p < 0.001), habitat breadth (Figure 2B; 0.341 ± 0.055, z = 6.241, p < 0.001), and biogeographic realm (Figure 1; χ2 = 56.434, p < 0.001) were all significantly associated with the use of temporary primary cropland for stopovers. The proportion of species with stopover events in cropland was highest for species whose primary habitat was woodland (Figure 2A; 40.0%, p = 0.003) or grassland and plains (36.2%, p = 0.018). Conversely, the proportion of species with stopovers recorded in cropland was lowest for species whose primary habitat was defined as coastal (20.0%, p = 0.047), riparian (9.1%, p = 0.007), rocky areas (3.3%, p < 0.001), or desert (0.0%, p = 0.050). Species that can utilize a higher variety of habitats were more likely to be reported with stopovers in temporary primary croplands. Species that used six major habitats were proportionally more likely to utilize temporary primary cropland (Figure 2B; 40.6%, p = 0.005) while habitat specialist species with a habitat breadth of 1 (14.8%, p = 0.010) or 2 (21.1%, p = 0.017) were less likely.
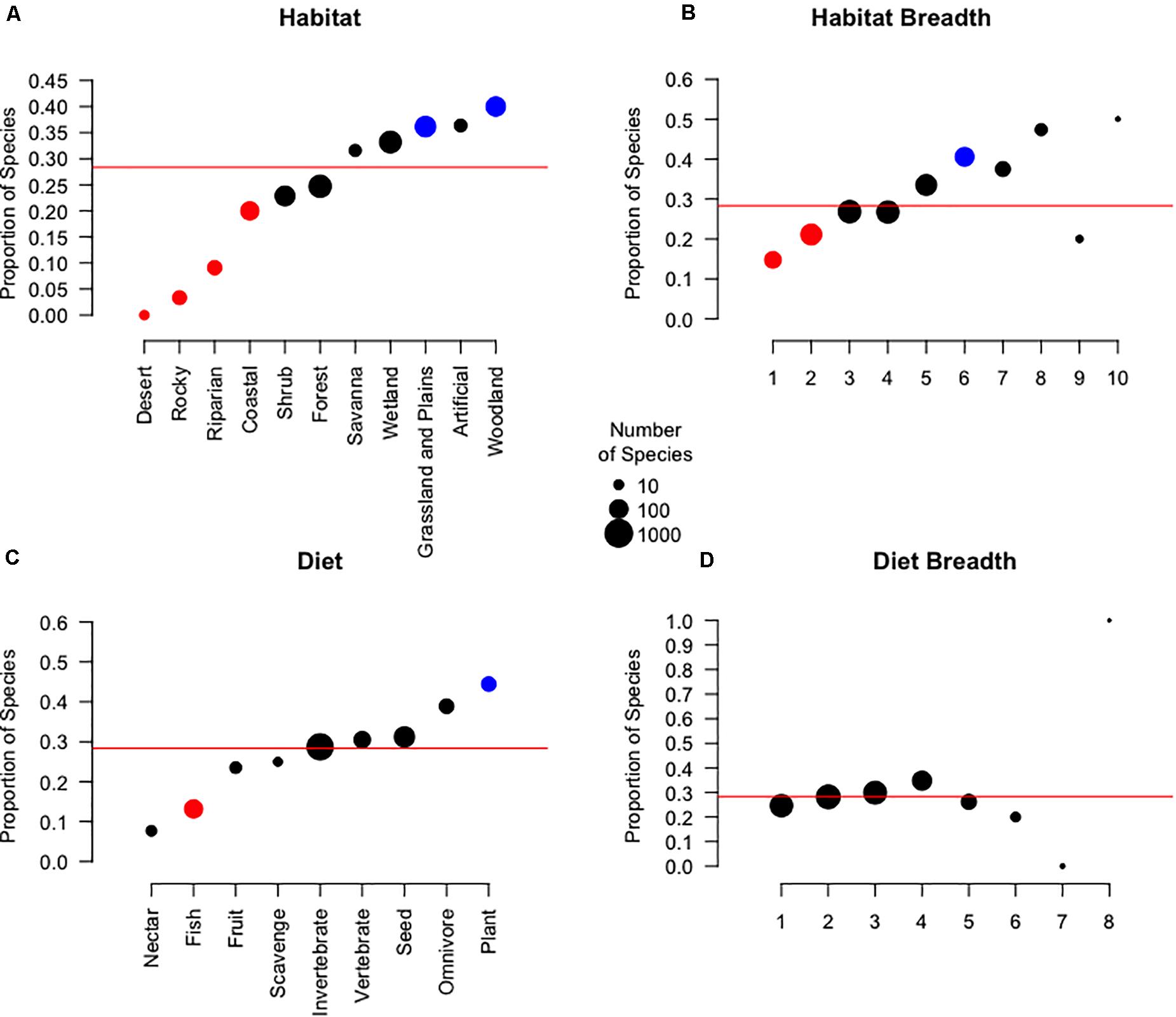
Figure 2. The proportion of species that utilize temporary primary croplands by primary habitat (A), habitat breadth (B), primary diet (C), and diet breadth (D). Point size indicates the total number of species within a category. Color represents the proportion of species within each group associated with cropland compared to the average association for migratory birds overall, shown as a red line (28.3%): blue indicates a significantly higher than average proportion, red indicates a significantly lower than average proportion, and black indicates non-significant results.
Primary diet had a marginally insignificant association with the utilization of temporary primary cropland for stopovers (Figure 2C; χ2 = 18.255, p = 0.051). However, herbivores (Figure 2C; 44.4%, p = 0.029) had significantly higher proportions of stopover migrants than average. Additionally, the proportion of stopover migrants that utilized agricultural areas showed no significant association with diet breadth (Figure 2D). Compared to the average, all diet breadth categories had insignificant proportions of migrants who utilized agricultural areas for stopovers.
Certain families were more likely than others to utilize temporary primary croplands during migration (Figure 3). Families with significantly more species that were recorded having stopovers in crops included Icteridae (76.5%, p < 0.001), Troglodytidae (75.0%, p = 0.008), Cardinalidae (69.2%, p = 0.003), Parulidae (60.0%, p < 0.001), Scolopacidae (59.7%, p < 0.001), Vireonidae (58.3%, p = 0.028), Tyrannidae (56.0%, p = 0.003), Passerellidae (50.0%, p < 0.001), and Charadriidae (48.0%, p = 0.029). Families with significantly fewer species that were recorded having stopovers in cropland included Muscicapidae (16.2%, p = 0.014), Laridae (14.6%, p = 0.020), Emberizidae (7.4%, p = 0.008), Strigidae (6.7%, p = 0.047), Phylloscopidae (2.1%, p < 0.001), Locustellidae (0.0%, p = 0.005), and Acrocephalidae (0.0%, p < 0.001).
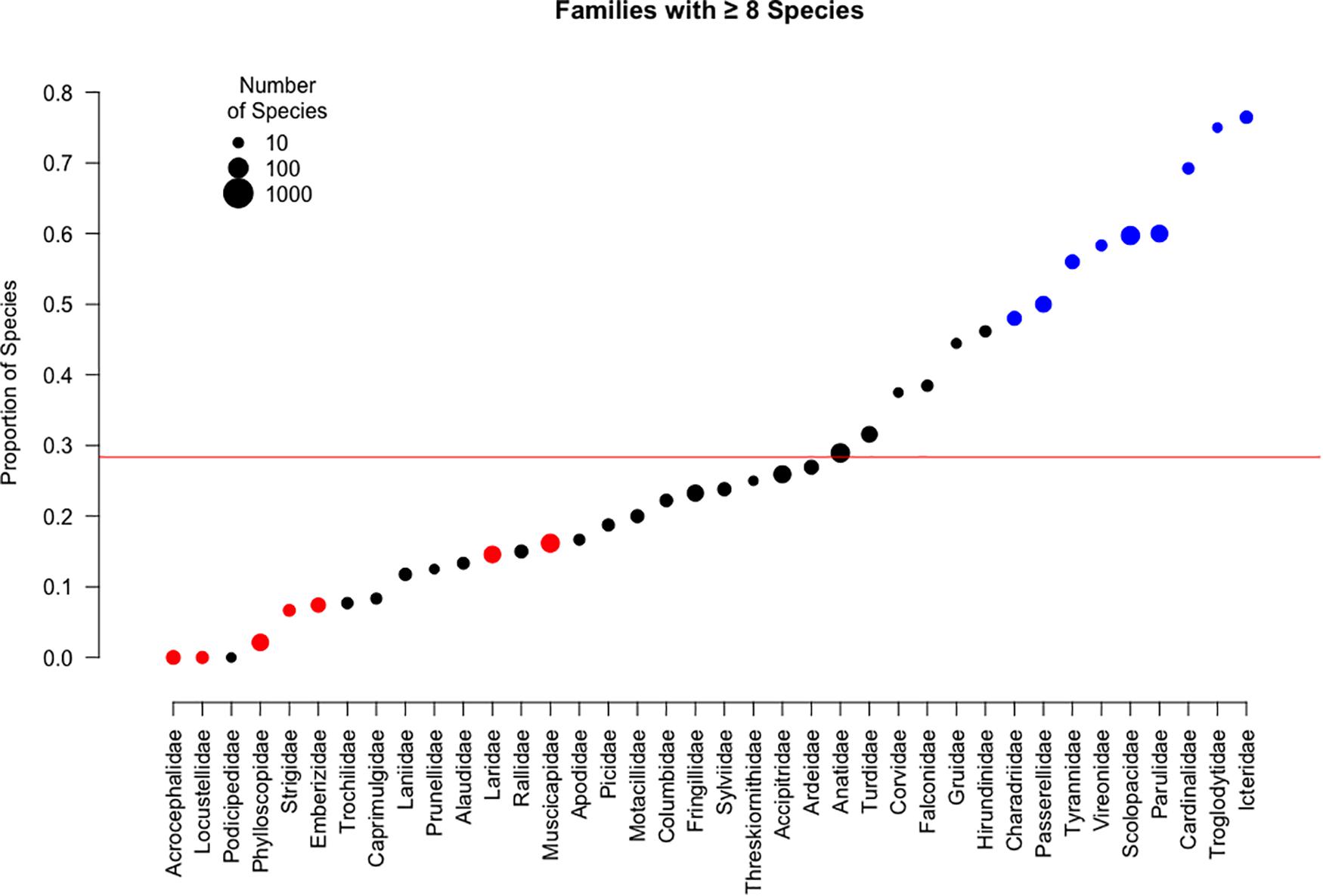
Figure 3. The proportion of species by family that utilize temporary primary croplands. Point size indicates the total number of species within a category. Color represents the proportion of species within each group associated with cropland compared to the average association for migratory birds overall shown as a red line (28.3%): blue indicates a significantly higher than average proportion, red indicates a lower proportion than average, and black indicates non-significant results. No families with fewer than 8 migratory species had significantly higher or lower proportions of species that utilized agriculture for stopovers and were removed from the plot.
We found that there was a significant difference (t1829.2 = −13.079, p < 0.001) between the number of threatened species that were recorded using agricultural areas for stopovers (Mean = 0.022 ± 0.301) and species that did not stopover in agriculture (Mean = 0.101 ± 0.147).
In a post hoc analysis, we explored the differences between families with more than eight species in both Nearctic and Palearctic realms. We found no difference in seven of the eight families. Nearctic Laridae species had a lower proportion (10.0%, p = 0.007) of species that that stopped over in agricultural areas, while there was no significant result in the family within the Palearctic species.
Discussion
As human impact on the planet continues to expand, it is critical to understand how migrant bird species may respond to land use change. By understanding which traits of birds predict whether or not a species utilizes temporary primary croplands for stopovers, we can improve our understanding of which species may benefit from the increase in agricultural areas in the Anthropocene and which species will need conservation intervention. Grassland, plains, and woodland birds, as well as generalist species with wider habitat breadths were more likely to stopover in agricultural areas (Figures 2A,B). Conversely, birds preferring riparian, desert, rocky, or coastal habitats, and habitat specialist species were less likely to stopover in agricultural areas (Figures 2A,B).
Primary habitat and habitat breadth were both significant predictors of whether a species stops in agricultural areas during migration (Figures 2A,B). Our analysis supports previous studies that have shown that birds with preferences for habitat similar to agricultural fields were more likely to use them as stopover sites (Robertson et al., 2011; Fontanilles et al., 2020). Additionally, many agricultural areas assessed in the literature were surrounded by forests, woodlands, or grasslands (e.g., Beecher et al., 2002; Kirk and Lindsay, 2017). This may account for birds with primary habitats that are dissimilar to agriculture fields, like woodlands, being reported in above-average proportions in fields. In areas where natural habitats have been cultivated for other uses, agricultural areas that mimic the primary habitats of a species may alternatively be used (Day and Colwell, 1998). Furthermore, species with wider habitat breadths are more likely to use agricultural areas as stopover sites since they can use more habitats.
We found that neither diet breadth nor primary diet was significantly correlated with agricultural stopover use (Figures 2C,D). This contrasts with other studies that have shown that generalist species, which can forage on a wide range of food types, are more common in these areas (Boutin et al., 1999). Since avian habitat and dietary specialists are more likely to be threatened with extinction (Şekercioğlu, 2011), our results demonstrate that agricultural areas may not be ideal for non-agricultural habitat specialists regardless of their dietary preferences. This corroborates previous research that shows long term declines in bird populations in tropical agricultural areas (Şekercioğlu et al., 2019). Furthermore, we found that disproportionately fewer threatened species were recorded using agricultural areas for stopovers, than compared to species that do not use these areas to stopover.
The proportion of certain dietary guilds associated with cropland may have also been influenced by an inherent bias in the published papers. For example, many geese (Anatidae) and blackbird (Icteridae) species are implicated in causing crop damage (Cummings et al., 1987; Jepsen, 1991). As a result, studies of bird use in agriculture fields have tended to focus on these potential “pests” and similar species given their economic importance (Cummings et al., 1987; Jepsen, 1991; Wallin and Milberg, 1995; Hagy et al., 2008). A review of the literature on avian cropland association will therefore be biased in favor of specific species within certain diet guilds that have received more attention. Even though primary diet was not a significant predicator of stopover use, herbivores were shown to utilize agricultural fields more than other guilds (Figure 2C).
Behavioral differences between families may also account for some of the taxonomic patterns of cropland use. Some species within bird families have been shown at an individual level to learn and adapt to changing conditions (Emery, 2004; Keagy et al., 2009). This adaptability may permit them to exploit altered areas. Behavioral differences may also introduce bias against nocturnal families like true owls (Strigidae), which may have been omitted from the literature as a result of researchers mostly focusing on diurnal species. Though there are fifteen species of migratory owls included within our study there are few radio-tracking studies being conducted on these owls that specifically comment on stopover use. Patterns of cropland use within a family could also be due to similar migratory strategies among related species. There is a positive correlation with body mass and accumulation of fat (per gram of lean body mass) (Alerstam and Lindström, 1990). Consequently, passerines tend to be limited in how far they can fly before replenishing energy reserves, resulting in short migratory “hops” and use of more stopover sites (Alerstam and Lindström, 1990). Conversely, larger species in non-songbird families tend to have longer uninterrupted flight bouts between only a few key stopover locations (Pfister et al., 1992; Atkinson et al., 2007; Littlefield and Johnson, 2013). More frequent use of stopover sites may therefore force songbirds to utilize a higher diversity of habitats, including farmland.
Region was found to have a significant effect on which birds were recorded having stopovers in agricultural areas, with 47.9% of migratory birds in the Nearctic realm being reported in agricultural areas during migration, in contrast to only 16.7% of Palearctic species reported in these areas. Even though our literature review returned a similar number of publications for both regions, almost all of the studies in the Palearctic came from Europe or East Asia, leaving much of Central Asia, South Asia and the Middle East unaccounted for. In this study, we looked at the differences in the proportion of species that stopover in agriculture between realms in eight families that had species in both realms. When we divided species within each family by realm, only one family differed in the proportion of species that utilized agricultural areas for stopovers. Since birds within the same family tend to have similar stopover usage, the differences in usage between realms may not be due to species changing their behavior and preferences to avoid cropland. Instead, this difference in realms may be a result of more Palearctic families not relying on agricultural areas. A lack of literature in much of the southern hemisphere and incomplete coverage in the Middle East, South Asia and Central Asia may bias our results by overestimating the importance of traits in species (i.e., geese and blackbirds) that lead to financial loss to farmers. Research into the cause of this discrepancy between realms may further shed light on how different species in a variety of dietary guilds utilize agriculture.
In addition to avian ecological traits, cropland management regimes also impact the abundance and diversity of birds that stopover. While relatively few prior studies have compared farm types, it is possible to draw several general conclusions about the effects of different farming practices on migration stopover preferences. Rice, corn, and sunflower appear to be preferred over other crops by a diverse set of migrants (Hagy and Bleier, 2007; Hagy et al., 2010; Stafford et al., 2010; Pearse et al., 2011; Cai et al., 2014; Krapu et al., 2014), which may be due to the comparatively high levels of metabolizable energy in these crops (Joyner et al., 1987; Galle et al., 2009; Stafford et al., 2010). All three crops also retain a high degree of spatial complexity, resulting in higher potential for roosting and predator avoidance.
There are many aspects of farm management that can affect whether migrants utilize the area as a stopover. Edge habitat use was positively associated with stopover use, most likely due to the increased plant diversity (Farina, 1988; Duckworth, 1994; Sapir et al., 2004). Crop heterogeneity is highly linked with the likelihood of migrants using the area as a stopover. The increase in vegetative diversity results in a higher abundance and variety of food sources and habitat (Robertson et al., 2011; Rosin et al., 2012). The effect of fallow fields on migrants was inconsistent, being negative for agriculture specialists but positive for non-specialists (Bryan and Best, 1994; Hultquist and Best, 2001; Galle et al., 2009; Wilcoxen et al., 2018). Farm managers can increase the use of temporary primary croplands by a larger breadth of species by maintaining edge habitat and overall plant diversity. Other agricultural practices, like the use of pesticides, may not affect birds initially choosing to stopover in agriculture, but can have lasting effects on birds after they resume their migration (Eng et al., 2019). Furthermore, farm managers can lessen their impact on migrant birds by using herbicides and pesticides with less avian toxicity. Obtaining a better understanding of the effects that common insecticides, herbicides, and fungicides have on migrating species should be considered a priority.
Conclusion
As anthropogenic land use continues to consume more land area, this study aims to shed light on factors that encourage or discourage avian use of agricultural areas, one of the most prevalent land cover types. Our review outlines crop management and selection decisions that can benefit certain birds during migration, a period of heightened vulnerability during a bird’s life cycle. We have also identified a set of factors that help predict which bird species are most inclined to use or avoid agricultural fields. Nevertheless, there is a scarcity of research on avian stopover habitat use in the Southern Hemisphere. Fewer than 6% of species recorded using agricultural areas for stopovers were from realms other than the Palearctic or the Nearctic. We believe this reveals a large gap in our knowledge of where migratory birds from these understudied realms spend up to 85% of their total migration time, which is a major impediment to their effective conservation. With steep declines in migratory bird populations in the Nearctic (Rosenberg et al., 2019) and Palearctic (Sanderson et al., 2006), understanding how to mitigate these declines is of utmost importance for avian conservation. As land is increasingly converted into agricultural use to feed the growing global population, it is imperative that we look for ways to increase the utility of agricultural habitats for birds and other species.
Data Availability Statement
The original contributions presented in the study are included in the article/Supplementary Material, further inquiries can be directed to the corresponding author/s.
Author Contributions
JH and ÇŞ conceived the manuscript idea. JB and JH developed the theory, performed the computations, and wrote the manuscript. KK and MN-C contributed to the analysis and writing of the manuscript. All authors discussed the results and revised the manuscript.
Conflict of Interest
The authors declare that the research was conducted in the absence of any commercial or financial relationships that could be construed as a potential conflict of interest.
Supplementary Material
The Supplementary Material for this article can be found online at: https://www.frontiersin.org/articles/10.3389/fevo.2021.650641/full#supplementary-material
References
Addy-Orduna, L., Brodeur, J., and Mateo, R. (2019). Oral acute toxicity of imidacloprid, thiamethoxam and clothianidin in eared doves: a contribution for the risk assessment of neonicotinoids in birds. Sci. Total Environ. 650, 1216–1223. doi: 10.1016/j.scitotenv.2018.09.112
Acosta, M., Mugica, L., Blanco, D., López-Lanús, B., Dias, R., Doodnath, L., et al. (2010). Birds of rice fields in the Americas. Waterbirds 33, 105–122. doi: 10.2307/40891070
Alerstam, T., and Lindström, Å (1990). “Optimal bird migration: the relative importance of time, energy, and safety,” in Bird Migration, (Berlin: Springer), 331–351.
Alexandrino, E., Forte, Y., Cassiano, C., Ferraz, K., Şekercioğlu, Ç, Buechley, E., et al. (2019). Highly disparate bird assemblages in sugarcane and pastures: implications for bird conservation in agricultural landscapes. Neotropic. Biol. Conserv. 14, 169–194. doi: 10.3897/neotropical.14.e37602
Atkinson, P. W., Baker, A. J., Bennett, K. A., Clark, N. A., Clark, J. A., Cole, K. B., et al. (2007). Rates of mass gain and energy deposition in red knot on their final spring staging site is both time- and condition- dependent. J. Appl. Ecol. 44, 885–895. doi: 10.1111/j.1365-2664.2007.01308.x
Bates, D., Mächler, M., Bolker, B., and Walker, S. (2015). Fitting linear mixed-effects models using lme4. J. Stat. Softw. 67, 1–48. doi: 10.18637/jss.v067.i01
Baker, A. J., González, P. M., Piersma, T., Niles, L. J., do Nascimento, I., Atkinson, P. W., et al. (2004). Rapid population decline in red knots: fitness consequences of decreased refuelling rates and late arrival in Delaware Bay. Proc. Biol. Sci. 271, 875–882. doi: 10.1098/rspb.2003.2663
Beatty, W. S., Kesler, D. C., Webb, E. B., Raedeke, A. H., Naylor, L. W., and Humburg, D. D. (2014). The role of protected area wetlands in waterfowl habitat conservation: implications for protected area network design. Biol. Conserv. 176, 144–152. doi: 10.1016/j.biocon.2014.05.018
Becker, P. H., Dittmann, T., Ludwigs, J.-D., Limmer, B., Ludwig, S. C., Bauch, C., et al. (2008). Timing of initial arrival at the breeding site predicts age at first reproduction in a long-lived migratory bird. Proc. Natl. Acad. Sci.U.S.A. 105, 12349–12352. doi: 10.1073/pnas.0804179105
Beecher, N. A., Johnson, R. J., Brandle, J. R., Case, R. M., and Young, L. J. (2002). Agroecology of birds in organic and nonorganic farmland. Conserv. Biol. 16, 1620–1631. doi: 10.1046/j.1523-1739.2002.01228.x
Bengtsson, D. (2016). Stopover Ecology of Mallards: Where, When and How to do What? Kalmar: Linnaeus University.
Bennett, P., and Owens, I. (1997). Variation in extinction risk among birds: chance or evolutionary predisposition? Proc. R. Soc. B Biol. Sci. 264, 401–408. doi: 10.1098/rspb.1997.0057.
Bevilacqua, S., Terlizzi, A., Claudet, J., Fraschetti, S., and Boero, F. (2012). Taxonomic relatedness does not matter for species surrogacy in the assessment of community responses to environmental drivers. J. Appl. Ecol. 49, 357–366. doi: 10.1111/j.1365-2664.2011.02096.x
Billerman, S. M., Keeney, B. K., Rodewald, P. G., and Schulenberg, T. S. (2020). Birds of the World. Ithaca, NY: Cornell Laboratory of Ornithology.
Bird Life International (2020). Species Populations and Trends. Available online at: http://datazone.birdlife.org/species/spcpop (accessed April 25, 2020).
Boutin, C., Freemark, K. E., and Kirk, D. A. (1999). Spatial and temporal patterns of bird use of farmland in southern Ontario. Can. Field Nat. 113, 430–460.
Bryan, G. G., and Best, L. B. (1994). Avian nest density and success in grassed waterways in Iowa rowcrop fields. Wildlife Soc. Bull. 22, 583–592.
Cai, T., Huettmann, F., and Guo, Y. (2014). Using stochastic gradient boosting to infer stopover habitat selection and distribution of Hooded Cranes Grus monacha during spring migration in Lindian, Northeast China. PLoS One 9:e89913. doi: 10.1371/journal.pone.0089913
Carlisle, J. D., Skagen, S. K., Kus, B. E., van Riper, C., Paxton, K. L., and Kelly, J. F. (2009). Landbird migration in the American west: recent progress and future research directions. Condor 111, 211–225. doi: 10.1525/cond.2009.080096
Chang, T., and Luh, B. S. (1991). “Overview and prospects of rice production,” in Rice Production, ed. B. S. Luh (New York, NY: Van Nostrand Reinhold), 1–11.
Clergeau, P., Savard, J. L., Mennechez, G., and Falardeau, G. (1998). Bird abundance and diversity along an urban-rural gradient: a comparative study between two cities on different continents. Condor 100, 413–425.
Cummings, J. L., Guarino, J. L., Knittle, C. E., and Royall, W. C. Jr. (1987). Decoy plantings for reducing blackbird damage to nearby commercial sunflower fields. Crop Prot. 6, 56–60.
Dänhardt, J., Green, M., Lindström, Å, Rundlöf, M., and Smith, H. G. (2010). Farmland as stopover habitat for migrating birds – effects of organic farming and landscape structure. Oikos 119, 1114–1125. doi: 10.1111/j.1600-0706.2009.18106.x
Day, J. H., and Colwell, M. A. (1998). Waterbird communities in rice fields subjected to different post-harvest treatments. Colonial Waterbirds 21, 118–197.
del Hoyo, J., Elliott, A., and Christie, D. (1992–2013). Handbook of the Birds of the World. Barcelona: Lynx Edicions.
Duckworth, J. W. (1994). Habitat selection by migrant redstarts Phoenicurus phoenicurus and whinchats Saxicola rubetra in lowland english farmland. Ring. Migr. 15, 119–122. doi: 10.1080/03078698.1994.9674084
Egevang, C., Stenhouse, I. J., Phillips, R. A., Petersen, A., Fox, J. W., and Silk, J. R. D. (2010). Tracking of arctic terns (Sterna paradisaea) reveals longest animal migration. Proc. Natl. Acad. Sci. U.S.A. 107, 2078–2081. doi: 10.1073/pnas.0909493107
Elphick, C. S. (2004). Assessing conservation trade-offs: identifying the effects of flooding rice fields for waterbirds on non-target bird species. Biol. Conserv. 117, 105–110.
Elphick, C. S. (2015). A history of ecological studies of birds in rice fields. J. Ornithol. 156, 239–245. doi: 10.1007/s10336-015-1194-5
Elphick, C. S., Taft, O., and Lourenço, P. M. (2010). Management of rice fields for birds during the non-growing season. Waterbirds 33, 181–192. doi: 10.1675/063.033.s114
Emery, N. J. (2004). The mentality of crows: convergent evolution of intelligence in Corvids and Apes. Science 306, 1903–1907. doi: 10.1126/science.1098410
Eng, M. L., Stutchbury, B. J. M., and Morrissey, C. A. (2019). A neonicotinoid insecticide reduces fueling and delays migration in songbirds. Science 365, 1177–1180. doi: 10.1126/science.aaw9419
FAO (2021). Crops Statistics – Concepts, Definitions and Classifications. Available online at: http://www.fao.org/economic/the-statistics-division-ess/methodology/methodology-systems/crops-statistics-concepts-definitions-and-classifications/en/ (accessed March 03, 2021).
FAO (2018). Land Use list. Available online at: www.fao.org/fileadmin/templates/ess/ess_test_folder/Definitions/LandUse_list.xls (accessed October 25, 2018).
Farina, A. (1988). Bird community structure and dynamics during spring migration in selected habitats of northern Italy. Bolletino di Zool. 55, 327–335. doi: 10.1080/11250008809386631
Fontanilles, P., De la Hera, I., Sourdrille, K., Lacoste, F., and Kerbiriou, C. (2020). Stopover ecology of autumn-migrating bluethroats (Luscinia svecica) in a highly anthropogenic river basin. J. Ornithol. 161, 89–101. doi: 10.1007/s10336-019-01717-z
Foster, M. S. (2007). The potential of fruit trees to enhance converted habitats for migrating birds in southern Mexico. Bird Conserv. Int. 17:45. doi: 10.1017/S0959270906000554
Franzen-Klein, D., Jankowski, M., Roy, C. L., Nguyen-Phuc, H., Chen, D., Neuman-Lee, L., et al. (2020). Evaluation of neurobehavioral abnormalities and immunotoxicity in response to oral imidacloprid exposure in domestic chickens (Gallus allus domesticus). J. Toxicol. Environ. Health Part A. 83, 45–65. doi: 10.1080/15287394.2020.1723154
Galarza, A., and Tellería, J. L. (2003). Linking processes: efects of migratory routes on the distribution of abundance of wintering passerines. Anim. Biodivers. Conserv. 26, 19–27.
Galle, A. M., Linz, G. M., Homan, J. H., and Bleier, W. J. (2009). Avian use of harvested crop fields in North Dakota during spring migration. West. N. Am. Nat. 69, 491–500.
Greco, S. E., and Airola, D. A. (2018). The importance of native valley oaks (Quercus lobata) as stopover habitat for migratory songbirds in urban Sacramento, California, USA. Urban For Urban Green 29, 303–311. doi: 10.1016/j.ufug.2018.01.005
Green, M., Alerstam, T., Clausen, P., Drent, R., and Ebbinge, B. S. (2002). Dark-bellied Brent Geese Branta bernicla bernicla, as recorded by satellite telemetry, do not minimize flight distance during spring migration. Ibis 144, 106–121. doi: 10.1046/j.0019-1019.2001.00017.x
Grishchenko, M., and Prins, H. H. T. (2016). Abandoned field succession in Russia and its potential effect on Corncrake Crex crex habitats. Die Vogelwelt 136, 175–184.
Grishchenko, M., Prins, H. H. T., Ydenberg, R. C., Schaepman, M. E., de Boer, W. F., and de Knegt, H. J. (2019). Land use change and the migration geography of Greater White-fronted geese in European Russia. Ecosphere 10:e02754. doi: 10.1002/ecs2.2754
Goulson, D. (2013). An overview of the environmental risks posed by neonicotinoid insecticides. J. Appl. Ecol. 50, 977–987. doi: 10.1111/1365-2664.12111
Hagy, H. M., and Bleier, W. J. (2007). “Are sunflower fields for the birds?,” in Proceedings of the 12th Wildlife Damage Management Conference. Fargo, ND:Google Scholar
Hagy, H. M., Linz, G. M., and Bleier, W. J. (2010). Wildlife conservation sunflower plots and croplands as fall habitat for migratory birds. Am. Midl. Nat. 164, 119–135. doi: 10.1674/0003-0031-164.1.119
Hagy, H. M., Linz, G. M., and Bleier, W. J. (2008). Optimizing the use of decoy plots for blackbird control in commercial sunflower. Crop Prot. 27, 1442–1447. doi: 10.1016/j.cropro.2008.07.006
Heard, M. S., Hawes, C., Champion, G. T., Clark, S. J., Firbank, L. G., Haughton, A. J., et al. (2003). Weeds in fields with contrasting conventional and genetically modified herbicide-tolerant crops. II. Effects on individual species. Philos. Trans. R. Soc. Lond. B Biol. Sci. 358, 846. doi: 10.1098/rstb.2003.1402
Hedenström, A., and Alerstam, T. (1997). Optimum fuel loads in migratory birds: distinguishing between time and energy minimization. J. Theor. Biol. 189, 227–234. doi: 10.1006/jtbi.1997.0505
Henle, K., Davies, K. F., Kleyer, M., Margules, C., and Settele, J. (2004). predictors of species sensitivity to fragmentation. Biodiver. Conserv. 13, 207–251. doi: 10.1023/B:BIOC.0000004319.91643.9e
Horns, J. J., and Şekercioğlu, ÇH. (2018). Conservation of migratory species. Curr. Biol 28, R980–R983. doi: 10.1016/j.cub.2018.06.032
Hultquist, J. M., and Best, L. B. (2001). Bird use of terraces in Iowa rowcrop fields. Am. Midl. Nat. 145, 275–287. doi: 10.1674/00030031(2001)145[0275:buotii]2.0.co;2
Ibáñez, C., Curcó, A., Riera, X., and Ripoll, I. (2011). Influence on birds of rice field management practices during the growing season: a review and an experiment. Waterbirds 33, 167–180. doi: 10.1675/063.033.s113
IUCN (2019). The IUCN Red List of Threatened Species. Version 2019-1. Available online at: https://www.iucnredlist.org (accessed July 25, 2020).
Janke, A. K., Anteau, M. J., and Stafford, J. D. (2019). Prairie wetlands confer consistent migrant refueling conditions across a gradient of agricultural land use intensities. Biol. Conserv. 229, 99–112. doi: 10.1016/j.biocon.2018.11.021
Jepsen, P. U. (1991). Crop damage and management of the pink-footed goose (Anser brachyrhynchus) in Denmark. Ardea 79, 191–194.
Johnson, O. W., Fielding, L., Fisher, J. P., Gold, R. S., Goodwill, R. H., Bruner, A. E., et al. (2012). New insight concerning transoceanic migratory pathways of Pacific Golden-Plovers (Pluvialis fulva): the Japan stopover and other linkages as revealed by geolocators. Wader Study Gr. Bull. 119, 1–8.
Jones, G. A., Sieving, K. E., and Jacobson, S. K. (2005). Avian diversity and functional insectivory on North-Central Florida farmlands. Conserv. Biol. 19, 1234–1245. doi: 10.1111/j.1523-1739.2005.00211.x
Jorgensen, J. G., Mccarty, J. P., and Wolfenbarger, L. L. (2007). Landscape and habitat variables affecting Buff-breasted sandpiper tryngites subruficollis distribution during migratory stopover in the rainwater Basin, Nebraska, USA. Wader Study Gr. Bull. 112, 45–51.
Joyce, M., Barnes, M. D., Possingham, H. P., and Van Rensburg, B. J. (2018). Understanding of avian assemblage change within anthropogenic environments using citizen science data. Landsc. Urban Plan. 179, 81–89. doi: 10.1016/j.landurbplan.2018.07.006
Joyner, D. E., Jacobson, B. N., and Arthur, R. D. (1987). Nutritional characteristics of grains fed to Canada geese. Wildfowl. 38, 89–93.
Kaminski, R. M., and Prince, H. H. (1981). Dabbling duckand aquatic macroinvertebrate responses to manipulated wetland habitat. J. Wildl. Manag. 45, 1–15.
Keagy, J., Savard, J. F., and Borgia, G. (2009). Male satin bowerbird problem-solving ability predicts mating success. Anim. Behav. 78, 809–817. doi: 10.1016/j.anbehav.2009.07.011
Keast, A. E., and Morton, E.S, (eds) (1980). Migrant Birds in the Neotropics. Washington DC: Smithsonian Press.
Kirk, D., and Lindsay, K. (2017). Subtle differences in birds between organic and nonorganic farms in Saskatchewan Prairie Parklands by farm pair and bird functional group. Agric. Ecosyst. Environ. 246, 184–201.
Koford, R. R., and Best, L. B. (1996). Management of Agricultural Landscapes for the Conservation of Neotropical Migratory Birds. Forest Service General Technical Report. Detroit: United States Department of Agriculture, 68–88.
Krapu, G. (2004). Less waste corn, more land in soybeans, and the switch to genetically modified crops: trends with important implications for wildlife management. Wildl. Soc. Bull. 1981, 127–136.
Krapu, G. L., Brandt, D. A., Kinzel, P. J., and Pearse, A. T. (2014). Spring migration ecology of the mid-continent sandhill crane population with an emphasis on use of the Central Platte River Valley, Nebraska. Wildl. Monogr. 189, 1–41. doi: 10.1002/wmon.101
Krapu, G., Reinecke, K., Jorde, D., and Simpson, S. (1995). Spring-staging ecology of midcontinent Greater White-Fronted Geese. J. Wildl. Manag. 59, 736–746. doi: 10.2307/3801950
Kross, J. P., Kaminski, R. M., Reinecke, K. J., and Pearse, A. T. (2008). Conserving waste rice for wintering waterfowl in the Mississippi Alluvial Valley. J. Wildl. Manag. 72, 1383–1387.
Kuznetsova, A., Brockhoff, P. B., and Christensen, R. H. B. (2017). lmertest package: tests in linear mixed effects models. J. Stat. Softw. 82, 1–26. doi: 10.18637/jss.v082.i13
La Sorte, F. A., Tingley, M. W., and Hurlbert, A. H. (2014). The role of urban and agricultural areas during avian migration: an assessment of within-year temporal turnover. Glob. Ecol. Biogeogr. 23, 1225–1234. doi: 10.1111/geb.12199
LaGrange, T. G., and Dinsmore, J. J. (1989). Habitat use by Mallards during spring migration through central Iowa. J. Wildl. Manag. 53, 1076–1081.
Lank, D., Butler, R., Ireland, J., and Ydenberg, R. (2003). Effects of predation on migration strategies of sandpipers. Oikos 103, 303–319. doi: 10.1034/j.1600-0706.2003.12314.x
Leito, A., Truu, J., Ounsaar, M., Sepp, K., Kaasik, A., Ojaste, I., et al. (2008). The impact of agriculture on autumn staging Eurasian cranes (Grus grus) in Estonia. Agric. Food Sci. 17, 53–62.
Lindström, A. (1990). The role of predation risk in stopover habitat selection in migrating bramblings, (Fringilla montifringilla). Behav. Ecol. 1, 102–106.
Lindström, A. (1989). Finch flock size and risk of hawk predation at a migratory stopover site. Auk 106, 225–232.
Lindstrom, A., and Alerstam, T. (1986). The adaptive significance of reoriented migration of Chaffinches (Fringilla coelebs) and Bramblings (Fringilla montifringilla) during autumn in southern Sweden. Behav. Ecol. Sociobiol. 19, 417–424.
Linscott, J., and Senner, N. (2021). Beyond refueling: investigating the diversity of functions of migratory stopover events. Condor 123:duaa074. doi: 10.1093/ornithapp/duaa074
Littlefield, C., and Johnson, D. H. (2013). Migration and habitat preferences of Swainson’s Hawks at an autumn stopover site in northwestern Texas. J. Raptor Res. 47, 54–59.
Loria, D., and Moore, F. (1990). Energy demands of migration on Red-eyed Vireos. Behav. Ecol. 1, 24–35. doi: 10.1093/beheco/1.1.24
Mabey, S. E., McCann, J., Nuns, L. J., Bartlett, C., and Kerlinger, P. (1993). The Migratory Songbird Coastal Corridor Final Report. Report No. NA90AAH-CZ839. Richmond, VA: Virginia Department of Environmental Quality Coastal Resources Management Program.
Maclean, J. L., Dawe, D. C., Hardy, B., and Hettel, G. P. (2002). Rice Almanac: Source Book for the Most Important Economic Activity of Earth. Wallingford: CABI Publishing.
Marini, L., Klimek, S., and Battisti, A. (2011). Mitigating the impacts of the decline of traditional farming on mountain landscapes and biodiversity: a case study in the European Alps. Environ. Sci. Policy 14, 258–267. doi: 10.1016/j.envsci.2010.12.003
Martin, T. E., and Karr, J. R. (1990). Behavioral plasticity of foraging maneuvers of migratory warblers: multiple selection periods for niches? Stud. Avian Biol. 13, 353–359.
Masero, J. A., Santiago-Quesada, F., Sanchez-Guzman, J. M., Villegas, A., Abad-Gomez, J. M., Lopes, R. J., et al. (2011). Long lengths of stay, large numbers, and trends of the Black-tailed Godwit Limosa limosa in rice fields during spring migration. Bird Conserv. 21, 12–24. doi: 10.1017/S0959270910000092
Mehlman, D., Mabey, S., Ewert, D., Duncan, C., Abel, B., Cimprich, D., et al. (2005). Conserving stopover sites for forest-dwelling migratory landbirds. Auk 122, 1281–1290.
Mertz, O., Wadley, R. L., Nielsen, U., Bruun, T. B., Colfer, C. J. P., de Neergaard, A., et al. (2008). A fresh look at shifting cultivation: Fallow length an uncertain indicator of productivity. Agric. Syst. 96, 75–84. doi: 10.1016/j.agsy.2007.06.002
Moore, F. R., and Aborn, D. A. (2000). Mechanisms of en route habitat selection: How do migrants make habitat decisions during stopover? Stud. Avian Biol. 20, 34–42.
Moore, E. R. (1991). Ecophysiological and behavioral response to energy demand during migration. Acta XX Congr. Int. Ornithol. 20, 753–760.
Newton, I. (2004). Population limitation in migrants. Ibis 146, 197–226. doi: 10.1111/j.1474-919X.2004.00293.x
Pearse, A. T., Krapu, G., and Cox, R. R. (2013). Comparative spring-staging ecology of sympatric arctic-nesting geese in south-central Nebraska. Am. Midl. Nat. 169, 371–381. doi: 10.1674/0003-0031-169.2.371
Pearse, A. T., Krapu, G. L., Brandt, D. A., and Kinzel, P. J. (2010). Changes in agriculture and abundance of Snow Geese affect carrying capacity of Sandhill Cranes in Nebraska. J. Wildl. Manage. 74, 479–488. doi: 10.2193/2008-539
Pearse, A. T., Krapu, G. L., Cox, R. R., and Bruce, E. (2011). Spring-migration ecology of Northern Pintails in south-central Nebraska. Waterbirds 34, 10–18.
Péron, G., Henry, P. Y., Provost, P., Dehorter, O., and Julliard, R. (2007). Climate changes and post-nuptial migration strategy in two reedbed passerines. Clim. Res. 35, 147–157. doi: 10.3354/cr00721
Petit, D. R. (2000). Habitat use by landbirds along Neartic-Neotropical migration routes: Implications for conservation of stopover habitats. Stud. Avian Biol. 20, 15–33.
Pfister, C., Harrington, B., and Lavine, M. (1992). The impact of human disturbance on shorebirds at a migration staging area. Biol. Conserv. 60, 115–126.
Piersma, T. (1987). Hop, skip, or jump? Constraints on migration of arctic waders by feeding, fattening, and flight speed. Limosa 60, 185–194.
Pywell, R. F., Heard, M. S., Woodcock, B. A., Hinsley, S., Ridding, L., and Nowakowski, M. et al. (2015). Wildlife-friendly farming increases crop yield: evidence for ecological intensification. Proc. Roy. Soc. B. 282, 20151740.
R Core Team (2020). R: A Language and Environment for Statistical Computing. Vienna: R Foundation for Statistical Computing.
Rabenold, K. N., and Rabenold, P. P. (1985). Variation in altitudinal migration, winter segregation, and site tenacity in two subspecies of Dark-eyed Juncos in the southern Appalachians. Auk 102, 805–819.
Ramankutty, N., and Foley, J. A. (1999). Estimating historical changes in global land cover: Croplands historical have converted areas. Glob. Biogeochem. Cycles 13, 997–1027. doi: 10.1029/1999GB900046
Reinecke, K. J., and Krapu, G. L. (1986). Feeding ecology of sandhill cranes during spring migration in Nebraska. J. Wildl. Manag. 50, 71–79. doi: 10.2307/3801490
Ritchie, H., and Roser, M. (2013). Land Use. Available online at: https://ourworldindata.org/land-use (accessed Novemeber 01, 2020).
Robertson, B. A., Doran, P. J., Loomis, E. R., Robertson, J. R., and Schemske, D. W. (2011). Avian use of perennial biomass feedstocks as Post-breeding and migratory stopover habitat. PLoS One 6:e16941. doi: 10.1371/journal.pone.0016941
Rodewald, P. G., and Brittingham, M. C. (2002). Habitat use and behavior of mixed species landbird flocks during fall migration. Wilson Bull. 114, 87–98.
Rodewald, P. G., Brittingham, M. C., and Moore, F. (2004). Stopover habitats of landbirds during fall: use of edge-dominated and early-successional forests. Auk 121, 1040–1055. doi: 10.1642/0004-80382004121
Rosenberg, K. V., Dokter, A. M., Blancher, P. J., Sauer, J. R., Smith, A. C., Smith, P. A., et al. (2019). Decline of the North American avifauna. Science 366, 120–124. doi: 10.1126/science.aaw1313
Rosenberg, K. V., Blancher, P. J., Stanton, J. C., and Panjabi, A. O. (2017). Use of North American Breeding Bird Survey data in avian conservation assessments. Condor 119, 594–606. doi: 10.1650/CONDOR-17-57.1
Rosin, Z. M., Skórka, P., Wylegała, P., Kra̧kowski, B., Tobolka, M., and Myczko, Ł́ et al. (2012). Landscape structure, human disturbance and crop management affect foraging ground selection by migrating geese. J. Ornithol. 153, 747–759. doi: 10.1007/s10336-011-0791-1
Roques, S., Henry, P. Y., Guyot, G., Bargain, B., Cam, E., and Pradel, R. (2020). When to depart from a stopover site? Time-since-arrival matters more than weather conditions. BioRxiv [Preprint] doi: 10.1101/2020.02.05.933788
Sanderson, F., Donald, P., Pain, D., Burfield, I., and Bommel, F. (2006). Long-term declines in Afro-Palearctic migrant birds. Biol. Conserv. 131, 93–105. doi: 10.1016/j.biocon.2006.02.008
Sanz, J., Potti, J., Moreno, J., Merino, S., and Frías, Ó. (2003). Climate change and fitness components of a migratory bird breeding in the Mediterranean region. Glob. Change Biol. 9, 461–472. doi: 10.1046/j.1365-2486.2003.00575.x
Sapir, N., Abramsky, Z., Shochat, E., and Izhaki, I. (2004). Scale-dependent habitat selection in migratory frugivorous passerines. Naturwissenschaften 91, 544–547. doi: 10.1007/s00114-004-0564-2
Schaaf, D., Linz, G. M., Doetkott, C., Lutman, M. W., and Bleier, W. J. (2008). Non-blackbird avian occurrence and abundance in North Dakota sunflower fields. Prairie Nat. 40, 73–86.
Schmaljohann, H., Fox, J. W., and Bairlein, F. (2012). Phenotypic response to environmental cues, orientation and migration costs in songbirds flying halfway around the world. Anim. Behav. 84, 623–640. doi: 10.1016/j.anbehav.2012.06.018
Schmaljohann, H., and Eikenaar, C. (2017). How do energy stores and changes in these affect departure decisions by migratory birds? A critical view on stopover ecology studies and some future perspectives. J. Comp. Physiol. A. 203, 411–429. doi: 10.1007/s00359-017-1166-8
Şekercioğlu, ÇH. (2012). Bird functional diversity in tropical forests, agroforests and open agricultural areas. J. Ornithol. 153, 153–161.
Şekercioğlu, ÇH. (2007). Conservation ecology: area trumps mobility in fragment bird extinctions. Curr. Biol. 17, 283–286. doi: 10.1016/j.cub.2007.02.018
Şekercioğlu, ÇH. (2006). “Ecological significance of bird populations,” in Handbook of the Birds of the World Alive, (Barcelona: Lynx Edicions), 15–51.
Şekercioğlu, ÇH. (2011). Functional extinctions of bird pollinators cause plant declines. Science 331, 1019–1020.
Şekercioğlu, ÇH., Daily, G. C., and Ehrlich, P. R. (2004). Ecosystem consequences of bird declines. Proc. Natl. Acad. Sci.U.S.A. 101, 18042–18047. doi: 10.1073/pnas.0408049101
Şekercioğlu, ÇH., Loarie, S. R., Oviedo Brenes, F., Ehrlich, P. R., and Daily, G. C. (2007). Persistence of forest birds in the Costa Rican agricultural countryside. Conserv. Biol. 21, 482–494. doi: 10.1111/j.1523-1739.2007.00655.x
Şekercioğlu, ÇH., Wenny, D. G., and Whelan, C. J. (2016). Why Birds Matter: Avian Ecological Function and Ecosystem Services. Chicago, IL: University of Chicago Press.
Şekercioğlu, ÇH., Mendenhall, C. D., Brenes, F. O., Horns, J. J., Ehrlich, P. R., and Daily, G. C. (2019). Long-term declines in bird populations in tropical agricultural countryside. PNAS 116, 9903–9912.
Shuford, W. D., Page, G. W., Langham, G. M., and Hickey, C. M. (2013). Long-billed Curlews in California’s central valley in fall. West. Birds 44, 196–205.
Si, Y., Xu, Y., Xu, F., Li, X., Zhang, W., Wielstra, B., et al. (2018). Spring migration patterns, habitat use, and stopover site protection status for two declining waterfowl species wintering in China as revealed by satellite tracking. Ecol. Evol. 8, 6280–6289. doi: 10.1002/ece3.4174
Smith, L. M., Haukos, D. A., and Prather, R. M. (2004). Avianresponse to a vegetative pattern in playa wetlands dur-ing winter. Wildl. Soc. Bull. 32, 474–480.
Stafford, J. D., Kaminski, R. M., and Reinecke, K. J. (2010). Avian foods, foraging and habitat conservation in world rice fields. Waterbirds Int. J. Waterbird Biol. 33, 133–150. doi: 10.1675/063.033.s110
Stodola, K. W., O’Neal, B. J., Alessi, M. G., Deppe, J. L., Dallas, T. R., Beveroth, T. A., et al. (2014). Stopover ecology of American Golden-Plovers (Pluvialis dominica) in Midwestern agricultural fields. Condor 116, 162–172. doi: 10.1650/CONDOR-13-114.1
Traba, J., and Morales, M. B. (2019). The decline of farmland birds in Spain is strongly associated to the loss of fallowland. Sci. Rep. 9:9473. doi: 10.1038/s41598-019-45854-0
Wallin, E., and Milberg, P. (1995). Effect of bean geese (Anser fabalis) grazing on winter wheat during migration stopover in southern Sweden. Agric. Ecosyst. Environ. 54, 103–108.
Wang, Y., Thornton, D. H., Ge, D., Wang, S., and Ding, P. (2015). Ecological correlates of vulnerability to fragmentation in forest birds on inundated subtropical land-bridge islands. Biol. Conserv. 191, 251–257. doi: 10.1016/j.biocon.2015.06.041
Wang, Y., Si, X., Bennett, P. M., Chen, C., Zeng, D., Zhao, Y., et al. (2018). Ecological correlates of extinction risk in Chinese birds. Ecography 41, 782–794. doi: 10.1111/ecog.03158
Weber, T. P., Houston, A. I., and Ens, B. J. (1999). Consequences of habitat loss at migratory stopover sites: a theoretical investigation consequences of habitat loss at migratory stopover sites: a theoretical investigation. J. Avian Biol. 30, 416–426.
Weller, M. W., and Spatcher, C. S. (1965). Role of Habitat in the Distribution and Abundance of Marsh Birds. Agriculture and Home Economics Experiment Station Special Report. Ames: Iowa State University, 43.
Weithman, C. E., Gibson, D., Walker, K. M., Maddock, S. B., Fraser, J. D., Karpanty, S. M., et al. (2018). Discovery of an important stopover location for migratory Piping Plovers (Charadrius melodus) on South Point, Ocracoke Island, North Carolina, USA. Waterbirds 41, 56–62. doi: 10.1675/063.041.0107
Wilcoxen, C. A., Walk, W., and Ward, M. P. (2018). Agriculture, ecosystems and environment use of cover crop fields by migratory and resident birds. Agric. Ecosyst. Environ. 252, 42–50. doi: 10.1016/j.agee.2017.09.039
Yamaguchi, N. M., Hupp, J. W., Flint, P. L., Pearce, J. M., Shigeta, Y., Shimada, T., et al. (2012). Habitat use and movement patterns of Northern Pintails during spring in northern Japan: the importance of agricultural lands. J. F. Ornithol. 83, 141–153. doi: 10.1111/j.1557-9263.2012.00364.x
Yong, W., and Finch, D. M. (2002). Stopover Ecology of Landbirds Migrating Along the Middle Rio Grande in Spring and Falling Genetic Technical Report RMRS-GTR-99. Ogden, UT: U.S. Department of Agriculture, Forest Service, Rocky Mountain Research Station, 52.
Zeileis, A., and Hothorn, T. (2002). Diagnostic checking in regression relationships. R News 2, 7–10.
Keywords: crop science, land use, landscape ecology, habitat, diet, nearctic, palearctic, conservation ecology
Citation: Blount JD, Horns JJ, Kittelberger KD, Neate-Clegg MHC and Şekercioğlu ÇH (2021) Avian Use of Agricultural Areas as Migration Stopover Sites: A Review of Crop Management Practices and Ecological Correlates. Front. Ecol. Evol. 9:650641. doi: 10.3389/fevo.2021.650641
Received: 07 January 2021; Accepted: 12 April 2021;
Published: 14 May 2021.
Edited by:
Orsolya Valkó, Hungarian Academy of Science, HungaryReviewed by:
Judit Kriszta Szabo, Federal University of Bahia, BrazilFederico Morelli, Czech University of Life Sciences Prague, Czechia
Copyright © 2021 Blount, Horns, Kittelberger, Neate-Clegg and Şekercioğlu. This is an open-access article distributed under the terms of the Creative Commons Attribution License (CC BY). The use, distribution or reproduction in other forums is permitted, provided the original author(s) and the copyright owner(s) are credited and that the original publication in this journal is cited, in accordance with accepted academic practice. No use, distribution or reproduction is permitted which does not comply with these terms.
*Correspondence: J. David Blount, David.Blount@utah.edu