- 1Instituto de Investigación en Biomedicina de Buenos Aires (IBioBA)-CONICET-Partner Institute of the Max Planck Society, Buenos Aires, Argentina
- 2Laboratory of Inflammation, Oswaldo Cruz Institute, Oswaldo Cruz Foundation (FIOCRUZ), Rio de Janeiro, Brazil
- 3Pulmonary and Critical Care Medicine Division, Department of Medicine, Brigham and Women’s Hospital and Harvard Medical School, Boston, MA, United States
- 4Laboratory of Respiratory Virus and Measles, Instituto Oswaldo Cruz, FIOCRUZ, Rio de Janeiro, Brazil
- 5Instituto René Rachou, Fundação Oswaldo Cruz, Belo Horizonte, Brazil
Influenza is one of the most relevant respiratory viruses to human health causing annual epidemics, and recurrent pandemics. Influenza disease is principally associated with inappropriate activation of the immune response. Chemokine receptor 5 (CCR5) and its cognate chemokines CCL3, CCL4 and CCL5 are rapidly induced upon influenza infection, contributing to leukocyte recruitment into the airways and a consequent effective antiviral response. Here we discuss the existing evidence for CCR5 role in the host immune responses to influenza virus. Complete absence of CCR5 in mice revealed the receptor’s role in coping with influenza via the recruitment of early memory CD8+ T cells, B cell activation and later recruitment of activated CD4+ T cells. Moreover, CCR5 contributes to inflammatory resolution by enhancing alveolar macrophages survival and reprogramming macrophages to pro-resolving phenotypes. In contrast, CCR5 activation is associated with excessive recruitment of neutrophils, inflammatory monocytes, and NK cells in models of severe influenza pneumonia. The available data suggests that, while CCL5 can play a protective role in influenza infection, CCL3 may contribute to an overwhelming inflammatory process that can harm the lung tissue. In humans, the gene encoding CCR5 might contain a 32-base pair deletion, resulting in a truncated protein. While discordant data in literature regarding this CCR5 mutation and influenza severity, the association of CCR5delta32 and HIV resistance fostered the development of different CCR5 inhibitors, now being tested in lung inflammation therapy. The potential use of CCR5 inhibitors to modulate the inflammatory response in severe human influenza infections is to be addressed.
Introduction
Aside from the onset of Corona Virus Disease 19 (COVID-19) pandemics in 2020, influenza virus is the most relevant respiratory virus for the healthcare system, causing millions of infections worldwide annually with estimates of up to 650 thousand deaths (1, 2). Although it is unpredictable when and to which extent the circulation of influenza among humans will return to pre-COVID-19 levels, the threat is perpetual due to the high genetic variability of the virus and the existence of multiple reservoirs (3). Influenza virus belongs to the Orthomixoviridae family of segmented, negative sense, single stranded RNA viruses (4). The infection of host respiratory epithelial cells occurs through the recognition of glycoconjugates with terminal N-acetylneuraminic acid (sialic acid) in the cell membrane by the viral protein hemagglutinin (HA). The multivalent attachment to sialic acid structures triggers the endocytosis of the virus (5). Influenza A and B are the most medically relevant types among the family causing annual epidemics, whereas only Influenza A might also give rise to pandemics such as the 1918 Spanish Flu and 2009 Swine Flu, both caused by H1N1 strains subtype, that occasioned more than 50 million and 363 thousand deaths respectively (6, 7). Some influenza A avian subtypes, including H5N1 and H7N9, are highly pathogenic to humans and, although human-to-human transmission is still limited, they have been closely monitored as potential new pandemic strains (8). Despite antivirals and vaccine availability, the emergence of pandemic strains is an imminent threat due to the high genetic variability of the virus, the ability to infect birds and swine that act as reservoirs, and a decreased population immunity to new strains (9–12). Therefore, comprehending the disease mechanisms involved in respiratory virus infections and continuous viral surveillance are badly needed as they set the basis for new therapeutics.
Dysfunctional inflammation triggered by influenza infection is related to the clinical manifestations and is orchestrated by different mediators (e.g: leukotrienes, cytokines and chemokines) and cell types (e.g: leukocytes, epithelial and endothelial cells) (13). However, a regulated well-controlled response ensures a proper viral clearance with restoration to tissue homeostasis. Therefore, inflammation has a dual role during influenza infection and disease. Although the chemokine receptor CCR5 does not actively participate in the infection process of influenza, after its activation by the chemokines CCL3/MIP-1α, CCL4/MIP-1β, and CCL5/RANTES, it becomes a key player in the inflammatory milieu that contributes to infection restraint. However, it might also be associated with inflammatory bystander lung damage. Here we discuss this duality of CCR5 activation during influenza infection.
Expression of CCR5 and CCR5 Ligands Upon Influenza Infection
One of the first reactions of the host after influenza infection is the production of CCR5 ligands by lung resident cells, especially alveolar macrophages and epithelial cells (14–16). CCL5 can be detected in human bronchoalveolar lavage fluid (BALF) samples after 7 days of symptoms onset (17). In vitro infection of type 2 human alveolar epithelial cells (hAECII) with either H1N1 or H5N1 virus leads to a significant production of CCL5 by those cells, showing that they may be a principal source of CCL5 during influenza pneumonia. In addition, human alveolar macrophages exposed to both H1N1 and H5N1 viruses produced CCL5. Interestingly, the infection with the H5N1 virus, a more pathogenic subtype, led to stronger CCL5 production in both cell types (15, 18). CCL3 and CCL4, the other CCR5 ligands, are also expressed in response to experimental influenza infection in human volunteers (19). A study of over 15 critically ill patients showed that CCL3 is augmented in lung aspirates of patients, and notably, at the serum level, there was an increment of CCL3 and CCL4 in comparison with mild cases of influenza infection (20). In addition, there is recent evidence showing, at the mRNA level, that peripheral blood monocytes derived from hospitalized patients diagnosed with influenza A or Severe Acute Respiratory Syndrome Virus-2(SARS-CoV-2) infection overexpress CCL3 (21).
In murine models, all CCR5 ligands are produced in lung tissue in response to influenza infection (22, 23). This contributes to the acute recruitment of leukocytes from the innate immunity to the lungs, mainly inflammatory monocytes and neutrophils, but also NK cells, which can induce CCR5 expression in response to the infection (24, 25). The latter recruitment of cells from adaptive immunity is also mediated by CCR5. Indeed, effector cytotoxic Th1 lymphocytes, memory CD8 T cells, and also B lymphocytes express CCR5. Moreover, there is evidence pointing that CCL5:CCR5 interaction contributes to the formation of inducible bronchus-associated lymphoid tissue iBALT in mice (14, 23, 26).
The Role for CCR5 in Inflammation and Immunity to Influenza Virus
The immune responses that follow influenza infection are crucial to control virus proliferation and for the development of memory responses; however, uncontrolled, or exaggerated activation of the many components of the immune system is associated with severe pulmonary damage and contributes to flu mortality (13). Thus, the immune responses must be finely regulated and coordinated to ensure viral clearance and restoration of lung homeostasis, with minimum bystander damage. As part of the host immune circuits for resistance to infection, CCR5 mediates the recruitment and activation of leukocytes during influenza. Interestingly, CCR5 plays contrasting roles in different inflammatory and infectious diseases leading to protection against certain pathogens or immunopathology triggered by exacerbated inflammation (27, 28). In this regard, CCR5 activation during different phases of influenza infection might also lead to different outcomes. Indeed, CCR5 activation during the initial stages of influenza infection ensure the proper recruitment of leukocytes and activation of antiviral pathways in the epithelial cells (Figure 1 left panel) (14, 23, 29, 30). However, sustained or exaggerated CCR5 activation during severe/exacerbated influenza infection might fuel the inflammatory responses leading to increase pulmonary damage and dysfunction (Figure 1 right panel). The cellular expression of CCR5 dictates what cell type can be recruited by mediators such as CCL3, CCL4 and CCL5, the classical CCR5-associated chemokines.
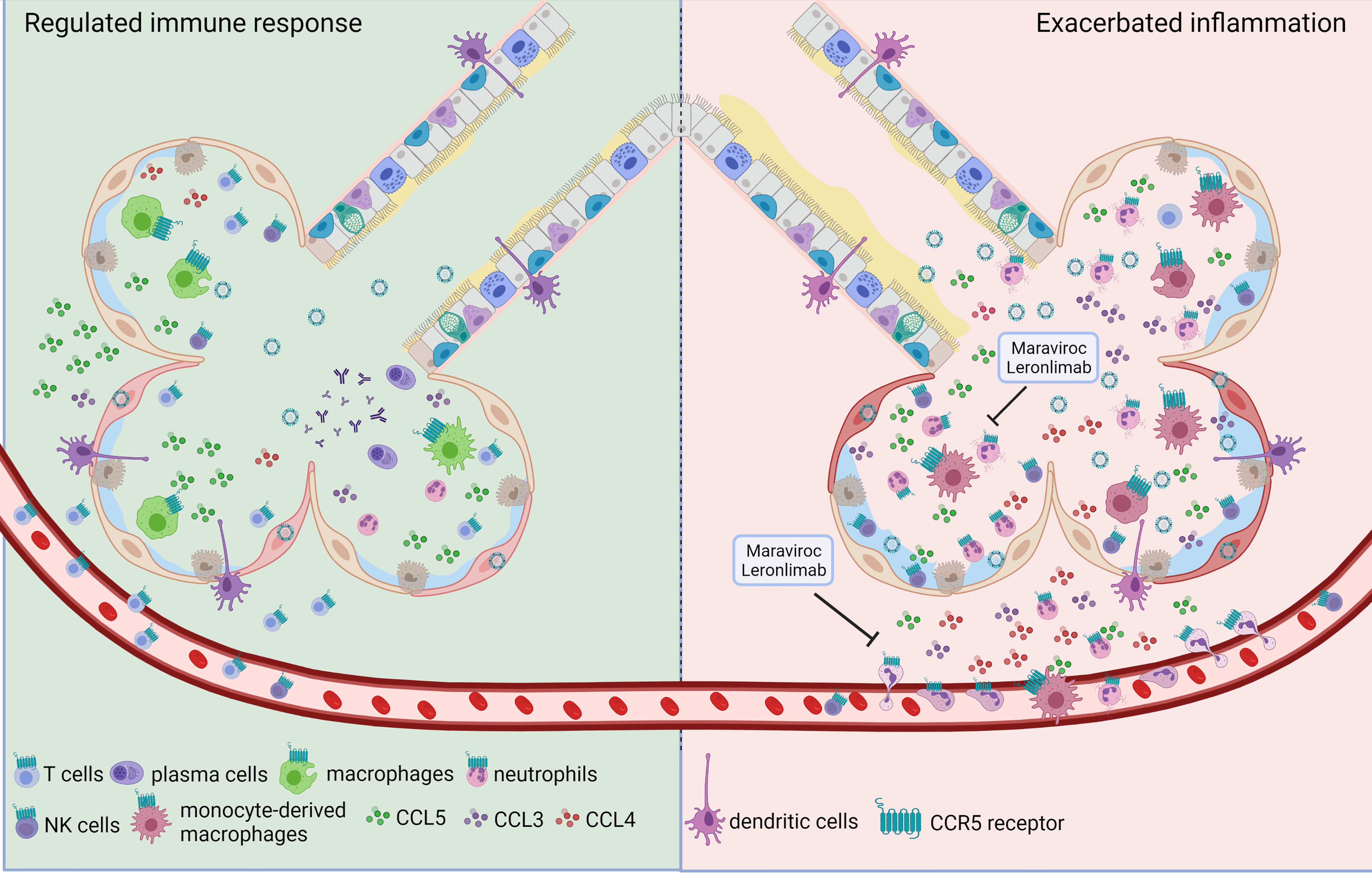
Figure 1 The dual role of CCR5 during influenza infection. Triggered by influenza infection, one of the first reactions of epithelial cells and resident alveolar macrophages is the production of CCR5 ligands. CCL5:CCR5 interaction is necessary for the development of a proper immune response (left side) to restrain viral expansion since it favors resident macrophages survival, promotes reprogramming of macrophages to pro-resolving phenotypes, mediates the recruitment of T lymphocytes and the establishment of iBALT contributing to immunological memory. However, uncontrolled activation of many components of the immune system after influenza infection is associated with severe pulmonary damage (right side). In this scenario, increased recruitment of neutrophils, inflammatory monocytes and natural killer cells can be mediated by CCR5 expression on those cells, and the actual evidence shows that CCL3 may be related to this exacerbated response. In this situation CCR5 inhibition by Maraviroc or Leronlimab, might represent an interesting therapeutic alternative.
CCR5 and its cognate chemokines are rapidly induced post-influenza infection in both humand and mice and ensure the prompt recruitment of leukocytes to the airways for an effective response (31). Indeed, the development and use of CCR5 knockout mice shed light on the mechanistic role for CCR5 mediating host protection to influenza. CCR5 deficient mice are highly susceptible to influenza infections and present increased neutrophilic inflammation and lung dysfunction in comparison to wild type mice (23). During influenza infection in mice, neutrophil expression of CCR5 is significantly increased and promotes different ex vivo cell functions (25). Whether CCR5 signaling in vivo directly regulates neutrophil activation or recruitment during influenza is yet to be explored; nevertheless, CCL5:CCR5 was shown to promote reprogramming of murine macrophages to pro-resolving phenotypes contributing to resolution of inflammation (32). In addition, CCR5:CCL5 was shown to prevent virus-induced apoptosis of human and mouse macrophages during influenza infection (33). Alveolar macrophages are crucial cells for viral and apoptotic cell clearance during infections preventing further unnecessary inflammatory responses in the lungs and tissue damage (34). Therefore, CCR5 signaling aids to the regulation of macrophage regulatory responses to guarantee restoration of tissue homeostasis during influenza infections.
NK cells are also recruited by CCR5 (24) and play a role in immunity to influenza infections in humans and mice (35, 36). NK cells can interact with influenza-infected cells and with the virus itself leading to secretion of cytokines and cytotoxic granules that restrain viral replication within the early stages of infection (36, 37). On the other hand, influenza virus can directly impair NK function to evade this innate layer of host immunity (38) and exaggerated NK cell activation, rather than being protective, might contribute to lung damage during severe influenza infections (39). Whether CCR5 activation transduces a protective or pathological NK cell response during influenza is yet to be determined. In parallel, the recruitment and activation of γδ T cells, mainly by CCR5, are important components of the potent antiviral responses to influenza infection in humans (40, 41).
In addition to the above mentioned role in innate immunity to influenza, CCR5 signaling is also necessary for the recruitment and effective response of the components of the adaptive immune system (42). Indeed, the increased pathology and lung dysfunction of CCR5 deficient mice are associated with decreased recruitment of CD8+ T cells during infection (23). Moreover, CCR5 was shown to be important for the development of early CD8+ T cell memory leading to control of virus replication during a secondary infectious challenge in mice (14). Furthermore, CCR5 might also impact B cell activation and recruitment during influenza. Secretion of CCL3 and CCL4 by B cells can lead to the recruitment of activated follicular CCR5+ CD4+ T cells, which enhances interaction between these two cell types and improves humoral immunity (43). Akin with that, mice lacking the CCL5 scavenger Atypical Chemokine Receptor 2 (ACKR2) present increased CCL5 levels, CCR5+CD4+ lymphocyte recruitment to the airways and augmented levels of IgA in the BALF during influenza. The specific phenotype of the T CD4+ recruited via CCR5 during influenza is yet to be defined (23). Noteworthy, the antagonism of CCR5 using maraviroc has not impaired the humoral response of HIV patients to the 2009 pandemic influenza A-H1N1 adjuvanted vaccine (44).
Pulmonary epithelial cells, in addition to the leukocytes, are important players driving antiviral responses to influenza (29). More recently, the direct role of CCL5:CCR5 in epithelial antiviral responses was uncovered. CCL5:CCR5 was shown to reduce influenza A replication in human epithelial cells by inducting the antiviral restriction factor SAM domain and HD domain-containing protein 1 (SAMHD1) (30). Keeping with that, the CCR5 agonist gp120 was shown to reduce A(H1N1)pdm09 replication in vitro in an IFITM3-dependent manner in human macrophages and human epithelial cervical cancer (HeLa) cells (45). Therefore, CCR5 signaling can impact the antiviral responses mediated by both epithelial and immune cells and, this should be taken into consideration when developing therapeutic strategies targeting this receptor for other inflammatory diseases. Interestingly, a recent study provided strong evidence for the protective role of CCR5 antagonism during Chronic Obstructive Pulmonary Disease (COPD) exacerbations caused by influenza in which CCL3 levels but no CCL5, correlated with an exacerbated inflammatory process (46). Maraviroc treatment during COPD viral exacerbations protected mice from the lethal pulmonary inflammation without affecting viral replication (46). In this regard, understanding the response to the virus and distinguishing between harmful and protective inflammation is crucial. The most relevant findings regarding CCR5 role during influenza infection are summarized on Table 1.
CCR5delta32 and Disease Severity
The gene encoding CCR5 might contain a 32 base pair deletion within the exon 3 resulting in a truncated protein that cannot be expressed on cell surface and therefore is non-functional (47). This deletion is present at different frequencies on populations around the world, which is related to ancestry. Whereas the allele frequency (AF) of the deletion is more than 15% in some European countries like Norway, Estonia and Latvia, some Asian and African countries present CCR5delta32 AF lower than 1% (48). Delta32 deletion was discovered in individuals multiply-exposed to HIV that were resistant to the infection and carried two alleles of CCR5-delta32 (49). This resistance was observed in CCR5-tropic HIV strains which depend on CCR5 as a co-receptor for cell entry. This process is avoided when a non-functional CCR5 is present in every cell on CCR5-delta32 homozygousity and act as a dominant-negative on the expression of wild type CCR5 and also C-X-C Motif Chemokine Receptor 4 (CXCR4), the other co-receptor of HIV (50).
While CCR5-delta32 homozygosis confers protection to HIV, meta-analysis have shown that HIV susceptibility or perinatal infection are not affected by CCR5-delta32 heterozygosity (51–53). Upon this findings on HIV resistance, CCR5 blockers or antagonists started to be tested against Acquired Immune Deficiency Syndrome (AIDS) and currently the CCR5 antagonist Maraviroc is clinically used (54). Moreover, a patient with acute myeloid leukemia and HIV had the infection controlled by the transplant of stem cells from a homozygous delta32 donor (55).
Besides HIV, CCR5delta32 has been associated with susceptibility (56, 57) or protection to different diseases, including COVID-19 (58–60). Regarding influenza, discordant data are present in literature. After the 2009 H1N1 pandemics, studies on distinct populations evaluated the CCR5delta32 allele frequencies on influenza patients with different outcomes. The first published study, assessing only 20 cases in Canada, found that the CCR5delta32 was a risk factor for the severity of H1N1 infection in white patients (61). In 2013 one Spanish study comparing a mild and a fatal case of the pandemic H1N1 infection found that the fatal case was homozygous for the CCR5Δ32 allele (62). Another Spanish study from 2015, assessing a larger population of 171 influenza patients found a correlation of CCR5Δ32 and mortality (63). On the other hand, three studies, one with 29 European (mostly Italian), other with 330 Brazilians and another with 432 Brazilian influenza patients with different clinical manifestations found no association between CCR5Δ32 and H1N1 severity (64–66). The conflict data might be explained by the global distribution of CCR5Δ32 allele. CCR5delta32 AF in countries where associations with influenza outcomes were found – Canada and Spain – are higher (8.1% and 7%, respectively) than in countries where no association was found – Italy (6.27%) and Brazil (4-5.44%) (48, 67).
CCR5 as Potential Target to Modulate Inflammation in Lung
Severe pneumonia following viral infection is principally associated with an overwhelmed production of inflammatory mediators and leukocyte recruitment to lung tissue. For that reason, chemokine receptors are interesting therapeutic candidates for lung inflammation. As aforementioned, CCR5 contribution during influenza infection appears to be crucial for the development of an antiviral response and the proper induction of immunologic memory. On the other hand, CCR5 activation is associated with excessive recruitment of neutrophils, inflammatory monocytes and NK cells in models of severe influenza pneumonia (24, 46, 68). This dual role of a chemokine receptor in the context of lung diseases is not an exclusive characteristic of CCR5 (69). Currently, the information obtained by the use of animal models suggests that while some CCR5 ligands, like CCL5, can play a protective role in influenza infection (23, 33) others, like CCL3, may contribute to an overwhelming inflammatory process which can harm the lung tissue (46, 70). Many pharmacological strategies that aim to impair CCR5 activity and endocytosis have been developed to fight HIV infection and were already tested in humans showing good safety profiles and effective antagonism properties. Nowadays, repositioning strategies based on the well-established CCR5-inhibitory capacities of drugs like Maraviroc, the only CCR5 inhibitor approved for clinical use, and Leronlimab, a CCR5-specific human IgG4 monoclonal antibody, succeed at presenting a good anti-inflammatory performance in the context of lung inflammatory conditions. Indeed, it was recently published that Leronlimab treatment reduced plasma IL-6 and viral load in critical COVID-19 patients (71). Besides, new CCR5 antagonists like cenicriviroc, which also present CCR2 inhibition, and GRL-117C, arise opportunities for the discovery of novel anti-inflammatory treatments focusing on CCR5 in the near future (72, 73). Currently, there is no disclosed clinical trial attempting to assess whether CCR5 antagonism can improve patient outcome during severe influenza pneumonia. However, as the current COVID-19 pandemics brought up challenging times while also emphazised a pre-existing demand for novel treatments to control the inflammatory response in the lungs, at least five clinical trials are being conducted to study CCR5 as potential drug target to treat lung inflammation during SARS-CoV-2 infection (NCT04441385, NCT04475991, NCT04710199, NCT04901676 NCT04901689). Either by CCR5 antagonism with Maraviroc or by its blockage with Leronlimab, these trials attempt to control the excessive inflammatory response by decreasing leukocyte accumulation in the lungs and inflammatory mediators in plasma of COVID-19 patients which is expected to improve patients outcome. By the moment, four of these clinical trials are on recruiting phase and the only completed study has no posted results yet (NCT04710199).
Conclusions
CCR5 plays important roles during influenza infection (Figure 1) by contributing to a suitable immune response via CCL5 to cope with the viral infection, but also subsidizing excessive inflammation and tissue damage by mechanisms associated with increased CCL3 production. This ambivalent character of CCR5 on influenza infection is not unique to this chemokine receptor but observed for many others in the dispute between pathological lung inflammation and restoration of physiological state. Thus, the correct use of CCR5 inhibitors as potential anti-inflammatory drugs in severe influenza infections requires a profound knowledge of the different phases in the inflammatory processes to be modulated.
Author Contributions
MF, LT, and CG contributed to the conception and design of the study; wrote the manuscript and discussed the content. All authors contributed to the article and approved the submitted version.
Funding
This work was supported by Fundação Oswaldo Cruz/Fiocruz.
Conflict of Interest
The authors declare that the research was conducted in the absence of any commercial or financial relationships that could be construed as a potential conflict of interest.
Publisher’s Note
All claims expressed in this article are solely those of the authors and do not necessarily represent those of their affiliated organizations, or those of the publisher, the editors and the reviewers. Any product that may be evaluated in this article, or claim that may be made by its manufacturer, is not guaranteed or endorsed by the publisher.
Acknowledgments
The authors thank Conselho Nacional de Desenvolvimento Científico e Tecnológico (CNPq); Consejo Nacional de Investigaciones Científicas y Técnicas (CONICET) Programa Fiocruz de Fomento a Inovação (INOVA-FIOCRUZ) and Fundação Carlos Chagas de Amparo à Pesquisa do Estado do Rio de Janeiro (FAPERJ) for support.
Abbreviations
CCR5, Chemokine receptor 5; CCL3, C-C Motif Chemokine Ligand 3; CCL4, C-C Motif Chemokine Ligand 4; CCL5, C-C Motif Chemokine Ligand 5; COVID-19, Corona Virus Disease 19; BALF, the bronchoalveolar lavage fluid; hAECII, human alveolar epithelial cells; SARS-CoV-2, Severe Acute Respiratory Syndrome Virus-2; NK, Natural Killer; ACKR2, Atypical Chemokine Receptor 2; HIV, Human immunodeficiency virus; SAMHD1, SAM domain and HD domain-containing protein 1; COPD, chronic obstructive pulmonary disease, AF, allele frequency, CXCR4, C-X-C Motif Chemokine Receptor 4; AIDS, Acquired Immune Deficiency Syndrome.
References
1. Iuliano AD, Roguski KM, Chang HH, Muscatello DJ, Palekar R, Tempia S, et al. Estimates of Global Seasonal Influenza-Associated Respiratory Mortality: A Modelling Study. Lancet (2018) 391(10127):1285–300. doi: 10.1016/S0140-6736(17)33293-2
2. Piroth L, Cottenet J, Mariet AS, Bonniaud P, Blot M, Tubert-Bitter P, et al. Comparison of the Characteristics, Morbidity, and Mortality of COVID-19 and Seasonal Influenza: A Nationwide, Population-Based Retrospective Cohort Study. Lancet Respir Med (2021) 9(3):251–9. doi: 10.1016/S2213-2600(20)30527-0
3. Choi YK. Emerging and Re-Emerging Fatal Viral Diseases. Exp Mol Med (2021) 53(5):711–2. doi: 10.1038/s12276-021-00608-9
4. Payne S. Family Orthomyxoviridae. Cambridge, Massachusetts, Estados Unidos: Academic Press (2017). pp. 197–208. doi: 10.1016/B978-0-12-803109-4.00023-4
5. Dou D, Revol R, Ostbye H, Wang H, Daniels R. Influenza A Virus Cell Entry, Replication, Virion Assembly and Movement. Front Immunol (2018) 9:1581. doi: 10.3389/fimmu.2018.01581
6. Zambon MC. Epidemiology and Pathogenesis of Influenza. J Antimicrob Chemother (1999) 44 Suppl B:3–9. doi: 10.1093/jac/44.suppl_2.3
7. Taubenberger JK, Kash JC, Morens DM. The 1918 Influenza Pandemic: 100 Years of Questions Answered and Unanswered. Sci Transl Med (2019) 11(502):1946–6234. doi: 10.1126/scitranslmed.aau5485
8. Widdowson MA, Bresee JS, Jernigan DB. The Global Threat of Animal Influenza Viruses of Zoonotic Concern: Then and Now. J Infect Dis (2017) 216(suppl_4):S493–8. doi: 10.1093/infdis/jix331
9. Beard KR, Brendish NJ, Clark TW. Treatment of Influenza With Neuraminidase Inhibitors. Curr Opin Infect Dis (2018) 31(6):514–9. doi: 10.1097/QCO.0000000000000496
10. Grohskopf LA, Alyanak E, Broder KR, Walter EB, Fry AM, Jernigan DB. Prevention and Control of Seasonal Influenza With Vaccines: Recommendations of the Advisory Committee on Immunization Practices - United State-20 Influenza Season. MMWR Recomm Rep (2019) 68(3):1–21. doi: 10.15585/mmwr.rr6803a1
11. O’Hanlon R, Shaw ML. Baloxavir Marboxil: The New Influenza Drug on the Market. Curr Opin Virol (2019) 35:14–8. doi: 10.1016/j.coviro.2019.01.006
12. Harrington WN, Kackos CM, Webby RJ. The Evolution and Future of Influenza Pandemic Preparedness. Exp Mol Med (2021) 53(5):737–49. doi: 10.1038/s12276-021-00603-0
13. Tavares LP, Teixeira MM, Garcia CC. The Inflammatory Response Triggered by Influenza Virus: A Two Edged Sword. Inflammation Res (2017) 66(4):283–302. doi: 10.1007/s00011-016-0996-0
14. Kohlmeier JE, Miller SC, Smith J, Lu B, Gerard C, Cookenham T, et al. The Chemokine Receptor CCR5 Plays a Key Role in the Early Memory CD8+ T Cell Response to Respiratory Virus Infections. Immunity (2008) 29(1):101–13. doi: 10.1016/j.immuni.2008.05.011
15. Yu WC, Chan RW, Wang J, Travanty EA, Nicholls JM, Peiris JS, et al. Viral Replication and Innate Host Responses in Primary Human Alveolar Epithelial Cells and Alveolar Macrophages Infected With Influenza H5N1 and H1N1 Viruses. J Virol (2011) 85(14):6844–55. doi: 10.1128/JVI.02200-10
16. Wang J, Nikrad MP, Travanty EA, Zhou B, Phang T, Gao B, et al. Innate Immune Response of Human Alveolar Macrophages During Influenza A Infection. PloS One (2012) 7(3):e29879. doi: 10.1371/journal.pone.0029879
17. Reynolds D, Vazquez Guillamet C, Day A, Borcherding N, Vazquez Guillamet R, Choreno-Parra JA, et al. Comprehensive Immunologic Evaluation of Bronchoalveolar Lavage Samples From Human Patients With Moderate and Severe Seasonal Influenza and Severe COVID-19. J Immunol (2021) 207(5):1229–38. doi: 10.4049/jimmunol.2100294
18. Chan MC, Cheung CY, Chui WH, Tsao SW, Nicholls JM, Chan YO, et al. Proinflammatory Cytokine Responses Induced by Influenza A (H5N1) Viruses in Primary Human Alveolar and Bronchial Epithelial Cells. Respir Res (2005) 6:135. doi: 10.1186/1465-9921-6-135
19. Fritz RS, Hayden FG, Calfee DP, Cass LM, Peng AW, Alvord WG, et al. Nasal Cytokine and Chemokine Responses in Experimental Influenza A Virus Infection: Results of a Placebo-Controlled Trial of Intravenous Zanamivir Treatment. J Infect Dis (1999) 180(3):586–93. doi: 10.1086/314938
20. Arankalle VA, Lole KS, Arya RP, Tripathy AS, Ramdasi AY, Chadha MS, et al. Role of Host Immune Response and Viral Load in the Differential Outcome of Pandemic H1N1 (2009) Influenza Virus Infection in Indian Patients. PloS One (2010) 5(10):e13099. doi: 10.1371/journal.pone.0013099
21. Lee JS, Park S, Jeong HW, Ahn JY, Choi SJ, Lee H, et al. Immunophenotyping of COVID-19 and Influenza Highlights the Role of Type I Interferons in Development of Severe COVID-19. Sci Immunol (2020) 5(49):eabd1554. doi: 10.1126/sciimmunol.abd1554
22. Wareing MD, Lyon AB, Lu B, Gerard C, Sarawar SR. Chemokine Expression During the Development and Resolution of a Pulmonary Leukocyte Response to Influenza A Virus Infection in Mice. J Leukoc Biol (2004) 76(4):886–95. doi: 10.1189/jlb.1203644
23. Tavares LP, Garcia CC, Goncalves APF, Kraemer LR, Melo EM, Oliveira FMS, et al. ACKR2 Contributes to Pulmonary Dysfunction by Shaping CCL5:CCR5-Dependent Recruitment of Lymphocytes During Influenza A Infection in Mice. Am J Physiol Lung Cell Mol Physiol (2020) 318(4):L655–70. doi: 10.1152/ajplung.00134.2019
24. Carlin LE, Hemann EA, Zacharias ZR, Heusel JW, Legge KL. Natural Killer Cell Recruitment to the Lung During Influenza A Virus Infection Is Dependent on CXCR3, CCR5, and Virus Exposure Dose. Front Immunol (2018) 9:781. doi: 10.3389/fimmu.2018.00781
25. Rudd JM, Pulavendran S, Ashar HK, Ritchey JW, Snider TA, Malayer JR, et al. Neutrophils Induce a Novel Chemokine Receptors Repertoire During Influenza Pneumonia. Front Cell Infect Microbiol (2019) 9:108. doi: 10.3389/fcimb.2019.00108
26. Moyron-Quiroz JE, Rangel-Moreno J, Kusser K, Hartson L, Sprague F, Goodrich S, et al. Role of Inducible Bronchus Associated Lymphoid Tissue (iBALT) in Respiratory Immunity. Nat Med (2004) 10(9):927–34. doi: 10.1038/nm1091
27. Marques RE, Guabiraba R, Russo RC, Teixeira MM. Targeting CCL5 in Inflammation. Expert Opin Ther Targets (2013) 17(12):1439–60. doi: 10.1517/14728222.2013.837886
28. Vangelista L, Vento S. The Expanding Therapeutic Perspective of CCR5 Blockade. Front Immunol (2017) 8:1981. doi: 10.3389/fimmu.2017.01981
29. Ramos I, Smith G, Ruf-Zamojski F, Martinez-Romero C, Fribourg M, Carbajal EA, et al. Innate Immune Response to Influenza Virus at Single-Cell Resolution in Human Epithelial Cells Revealed Paracrine Induction of Interferon Lambda 1. J Virol (2019) 93(20):e00559–19. doi: 10.1128/JVI.00559-19
30. Silva T, Temerozo JR, do Vale G, Ferreira AC, Soares VC, Dias SSG, et al. The Chemokine CCL5 Inhibits the Replication of Influenza A Virus Through SAMHD1 Modulation. Front Cell Infect Microbiol (2021) 11:549020. doi: 10.3389/fcimb.2021.549020
31. Sprenger H, Meyer RG, Kaufmann A, Bussfeld D, Rischkowsky E, Gemsa D. Selective Induction of Monocyte and Not Neutrophil-Attracting Chemokines After Influenza A Virus Infection. J Exp Med (1996) 184(3):1191–6. doi: 10.1084/jem.184.3.1191
32. Aswad M, Assi S, Schif-Zuck S, Ariel A. CCL5 Promotes Resolution-Phase Macrophage Reprogramming in Concert With the Atypical Chemokine Receptor D6 and Apoptotic Polymorphonuclear Cells. J Immunol (2017) 199(4):1393–404. doi: 10.4049/jimmunol.1502542
33. Tyner JW, Uchida O, Kajiwara N, Kim EY, Patel AC, O’Sullivan MP, et al. CCL5-CCR5 Interaction Provides Antiapoptotic Signals for Macrophage Survival During Viral Infection. Nat Med (2005) 11(11):1180–7. doi: 10.1038/nm1303
34. Watanabe S, Alexander M, Misharin AV, Budinger GRS. The Role of Macrophages in the Resolution of Inflammation. J Clin Invest (2019) 129(7):2619–28. doi: 10.1172/JCI124615
35. Weiss ID, Shoham H, Wald O, Wald H, Beider K, Abraham M, et al. Ccr5 Deficiency Regulates the Proliferation and Trafficking of Natural Killer Cells Under Physiological Conditions. Cytokine (2011) 54(3):249–57. doi: 10.1016/j.cyto.2011.01.011
36. Schultz-Cherry S. Role of NK Cells in Influenza Infection. Curr Top Microbiol Immunol (2015) 386:109–20. doi: 10.1007/82_2014_403
37. Mendelson M, Tekoah Y, Zilka A, Gershoni-Yahalom O, Gazit R, Achdout H, et al. NKp46 O-Glycan Sequences That are Involved in the Interaction With Hemagglutinin Type 1 of Influenza Virus. J Virol (2010) 84(8):3789–97. doi: 10.1128/JVI.01815-09
38. Mao H, Tu W, Liu Y, Qin G, Zheng J, Chan PL, et al. Inhibition of Human Natural Killer Cell Activity by Influenza Virions and Hemagglutinin. J Virol (2010) 84(9):4148–57. doi: 10.1128/JVI.02340-09
39. Abdul-Careem MF, Mian MF, Yue G, Gillgrass A, Chenoweth MJ, Barra NG, et al. Critical Role of Natural Killer Cells in Lung Immunopathology During Influenza Infection in Mice. J Infect Dis (2012) 206(2):167–77. doi: 10.1093/infdis/jis340
40. Qin G, Mao H, Zheng J, Sia SF, Liu Y, Chan PL, et al. Phosphoantigen-Expanded Human Gammadelta T Cells Display Potent Cytotoxicity Against Monocyte-Derived Macrophages Infected With Human and Avian Influenza Viruses. J Infect Dis (2009) 200(6):858–65. doi: 10.1086/605413
41. Qin G, Liu Y, Zheng J, Ng IH, Xiang Z, Lam KT, et al. Type 1 Responses of Human Vgamma9Vdelta2 T Cells to Influenza A Viruses. J Virol (2011) 85(19):10109–16. doi: 10.1128/JVI.05341-11
42. Klein RS. A Moving Target: The Multiple Roles of CCR5 in Infectious Diseases. J Infect Dis (2008) 197(2):183–6. doi: 10.1086/524692
43. Damdinsuren B, Zhang Y, Khalil A, Wood WH 3rd, Becker KG, Shlomchik MJ, et al. Single Round of Antigen Receptor Signaling Programs Naive B Cells to Receive T Cell Help. Immunity (2010) 32(3):355–66. doi: 10.1016/j.immuni.2010.02.013
44. Canestri A, Krivine A, Assoumou L, Le Corre M, Rozenberg F, Marcelin AG, et al. Maraviroc Does Not Affect Humoral Response to the Pandemic Influenza A-H1N1v 2009 Adjuvanted Vaccine in HIV-1-Infected Patients. AIDS (2010) 24(18):2887–9. doi: 10.1097/QAD.0b013e3283402bc1
45. Mesquita M, Fintelman-Rodrigues N, Sacramento CQ, Abrantes JL, Costa E, Temerozo JR, et al. HIV-1 and its Gp120 Inhibits the Influenza A(H1N1)pdm09 Life Cycle in an IFITM3-Dependent Fashion. PloS One (2014) 9(6):e101056. doi: 10.1371/journal.pone.0101056
46. Ferrero MR, Garcia CC, Dutra de Almeida M, Torres Braz da Silva J, Bianchi Reis Insuela D, Teixeira Ferreira TP, et al. CCR5 Antagonist Maraviroc Inhibits Acute Exacerbation of Lung Inflammation Triggered by Influenza Virus in Cigarette Smoke-Exposed Mice. Pharmaceuticals (Basel) (2021) 14(7):620. doi: 10.3390/ph14070620
47. Samson M, Libert F, Doranz BJ, Rucker J, Liesnard C, Farber CM, et al. Resistance to HIV-1 Infection in Caucasian Individuals Bearing Mutant Alleles of the CCR-5 Chemokine Receptor Gene. Nature (1996) 382(6593):722–5. doi: 10.1038/382722a0
48. Solloch UV, Lang K, Lange V, Bohme I, Schmidt AH. Frequencies of Gene Variant CCR5-Delta32 in 87 Countries Based on Next-Generation Sequencing of 1.3 Million Individuals Sampled From 3 National DKMS Donor Centers. Hum Immunol (2017) 78(11-12):710–7. doi: 10.1016/j.humimm.2017.10.001
49. Liu R, Paxton WA, Choe S, Ceradini D, Martin SR, Horuk R, et al. Homozygous Defect in HIV-1 Coreceptor Accounts for Resistance of Some Multiply-Exposed Individuals to HIV-1 Infection. Cell (1996) 86(3):367–77. doi: 10.1016/s0092-8674(00)80110-5
50. Agrawal L, Lu X, Qingwen J, VanHorn-Ali Z, Nicolescu IV, McDermott DH, et al. Role for CCR5Delta32 Protein in Resistance to R5, R5X4, and X4 Human Immunodeficiency Virus Type 1 in Primary CD4+ Cells. J Virol (2004) 78(5):2277–87. doi: 10.1128/jvi.78.5.2277-2287.2004
51. Contopoulos-Ioannidis DG, O’Brien TR, Goedert JJ, Rosenberg PS, Ioannidis JP. Effect of CCR5-Delta32 Heterozygosity on the Risk of Perinatal HIV-1 Infection: A Meta-Analysis. J Acquir Immune Defic Syndr (2003) 32(1):70–6. doi: 10.1097/00126334-200301010-00010
52. Liu S, Kong C, Wu J, Ying H, Zhu H. Effect of CCR5-Delta32 Heterozygosity on HIV-1 Susceptibility: A Meta-Analysis. PloS One (2012) 7(4):e35020. doi: 10.1371/journal.pone.0035020
53. Ni J, Wang D, Wang S. The CCR5-Delta32 Genetic Polymorphism and HIV-1 Infection Susceptibility: A Meta-Analysis. Open Med (Wars) (2018) 13:467–74. doi: 10.1515/med-2018-0062
54. Veljkovic N, Vucicevic J, Tassini S, Glisic S, Veljkovic V, Radi M. Preclinical Discovery and Development of Maraviroc for the Treatment of HIV. Expert Opin Drug Discov (2015) 10(6):671–84. doi: 10.1517/17460441.2015.1041497
55. Hutter G, Nowak D, Mossner M, Ganepola S, Mussig A, Allers K, et al. Long-Term Control of HIV by CCR5 Delta32/Delta32 Stem-Cell Transplantation. N Engl J Med (2009) 360(7):692–8. doi: 10.1056/NEJMoa0802905
56. Kindberg E, Mickiene A, Ax C, Akerlind B, Vene S, Lindquist L, et al. A Deletion in the Chemokine Receptor 5 (CCR5) Gene is Associated With Tickborne Encephalitis. J Infect Dis (2008) 197(2):266–9. doi: 10.1086/524709
57. Lim JK, Louie CY, Glaser C, Jean C, Johnson B, Johnson H, et al. Genetic Deficiency of Chemokine Receptor CCR5 is a Strong Risk Factor for Symptomatic West Nile Virus Infection: A Meta-Analysis of 4 Cohorts in the US Epidemic. J Infect Dis (2008) 197(2):262–5. doi: 10.1086/524691
58. Pokorny V, McQueen F, Yeoman S, Merriman M, Merriman A, Harrison A, et al. Evidence for Negative Association of the Chemokine Receptor CCR5 D32 Polymorphism With Rheumatoid Arthritis. Ann Rheum Dis (2005) 64(3):487–90. doi: 10.1136/ard.2004.023333
59. Cuesta-Llavona E, Gomez J, Albaiceta GM, Amado-Rodriguez L, Garcia-Clemente M, Gutierrez-Rodriguez J, et al. Variant-Genetic and Transcript-Expression Analysis Showed a Role for the Chemokine-Receptor CCR5 in COVID-19 Severity. Int Immunopharmacol (2021) 98:107825. doi: 10.1016/j.intimp.2021.107825
60. Hubacek JA, Dusek L, Majek O, Adamek V, Cervinkova T, Dlouha D, et al. CCR5Delta32 Deletion as a Protective Factor in Czech First-Wave COVID-19 Subjects. Physiol Res (2021) 70(1):111–5. doi: 10.33549/physiolres.934647
61. Keynan Y, Juno J, Meyers A, Ball TB, Kumar A, Rubinstein E, et al. Chemokine Receptor 5 Big Up Tri, Open32 Allele in Patients With Severe Pandemic (H1N1) 2009. Emerg Infect Dis (2010) 16(10):1621–2. doi: 10.3201/eid1610.100108
62. Rodriguez A, Falcon A, Cuevas MT, Pozo F, Guerra S, Garcia-Barreno B, et al. Characterization In Vitro and In Vivo of a Pandemic H1N1 Influenza Virus From a Fatal Case. PloS One (2013) 8(1):e53515. doi: 10.1371/journal.pone.0053515
63. Falcon A, Cuevas MT, Rodriguez-Frandsen A, Reyes N, Pozo F, Moreno S, et al. CCR5 Deficiency Predisposes to Fatal Outcome in Influenza Virus Infection. J Gen Virol (2015) 96(8):2074–8. doi: 10.1099/vir.0.000165
64. Sironi M, Cagliani R, Pontremoli C, Rossi M, Migliorino G, Clerici M, et al. The CCR5Delta32 Allele is Not a Major Predisposing Factor for Severe H1N1pdm09 Infection. BMC Res Notes (2014) 7:504. doi: 10.1186/1756-0500-7-504
65. Maestri A, dos Santos MC, Ribeiro-Rodrigues EM, de Mello WA, Sousa RC, dos Santos SE, et al. The CCR5Delta32 (Rs333) Polymorphism is Not a Predisposing Factor for Severe Pandemic Influenza in the Brazilian Admixed Population. BMC Res Notes (2015) 8:326. doi: 10.1186/s13104-015-1299-1
66. Matos AR, Martins J, Oliveira MLA, Garcia CC, Siqueira MM. Human CCR5Delta32 (Rs333) Polymorphism has No Influence on Severity and Mortality of Influenza A(H1N1)pdm09 Infection in Brazilian Patients From the Post Pandemic Period. Infect Genet Evol (2019) 67:55–9. doi: 10.1016/j.meegid.2018.10.024
67. Silva-Carvalho WH, de Moura RR, Coelho AV, Crovella S, Guimaraes RL. Frequency of the CCR5-Delta32 Allele in Brazilian Populations: A Systematic Literature Review and Meta-Analysis. Infect Genet Evol (2016) 43:101–7. doi: 10.1016/j.meegid.2016.05.024
68. Alon R, Sportiello M, Kozlovski S, Kumar A, Reilly EC, Zarbock A, et al. Leukocyte Trafficking to the Lungs and Beyond: Lessons From Influenza for COVID-19. Nat Rev Immunol (2021) 21(1):49–64. doi: 10.1038/s41577-020-00470-2
69. Tomankova T, Kriegova E, Liu M. Chemokine Receptors and Their Therapeutic Opportunities in Diseased Lung: Far Beyond Leukocyte Trafficking. Am J Physiol Lung Cell Mol Physiol (2015) 308(7):L603–618. doi: 10.1152/ajplung.00203.2014
70. Cook DN. The Role of MIP-1 Alpha in Inflammation and Hematopoiesis. J Leukoc Biol (1996) 59(1):61–6. doi: 10.1002/jlb.59.1.61
71. Patterson BK, Seethamraju H, Dhody K, Corley MJ, Kazempour K, Lalezari J, et al. CCR5 Inhibition in Critical COVID-19 Patients Decreases Inflammatory Cytokines, Increases CD8 T-Cells, and Decreases SARS-CoV2 RNA in Plasma by Day 14. Int J Infect Dis (2021) 103:25–32. doi: 10.1016/j.ijid.2020.10.101
72. Nakata H, Maeda K, Das D, Chang SB, Matsuda K, Rao KV, et al. Activity and Structural Analysis of GRL-117C: A Novel Small Molecule CCR5 Inhibitor Active Against R5-Tropic HIV-1s. Sci Rep (2019) 9(1):4828. doi: 10.1038/s41598-019-41080-w
Keywords: influenza, chemokine receptor 5, CCR5delta32, CCL5, CCL3
Citation: Ferrero MR, Tavares LP and Garcia CC (2022) The Dual Role of CCR5 in the Course of Influenza Infection: Exploring Treatment Opportunities. Front. Immunol. 12:826621. doi: 10.3389/fimmu.2021.826621
Received: 01 December 2021; Accepted: 31 December 2021;
Published: 20 January 2022.
Edited by:
Joel Henrique Ellwanger, Federal University of Rio Grande do Sul, BrazilReviewed by:
Takayuki Uematsu, Kitasato University, JapanLaila N. Abudulai, University of Western Australia, Australia
Copyright © 2022 Ferrero, Tavares and Garcia. This is an open-access article distributed under the terms of the Creative Commons Attribution License (CC BY). The use, distribution or reproduction in other forums is permitted, provided the original author(s) and the copyright owner(s) are credited and that the original publication in this journal is cited, in accordance with accepted academic practice. No use, distribution or reproduction is permitted which does not comply with these terms.
*Correspondence: Maximiliano Ruben Ferrero, maximiliano-ferrero@hotmail.com