- Key Laboratory of Biorheological Science and Technology, Ministry of Education, College of Bioengineering, Chongqing University, Chongqing, China
A2A receptors (A2AR), a typical GPCR with a high affinity for adenosine, was expressed in many immune cells, such as regulatory T cells, cytotoxic T cells, macrophages, etc. Adenosine binding to the A2AR receptor activates the typical G protein and triggers the cAMP/PKA/CREB pathway. The adenosine-A2AR pathway plays an important role in protecting normal organs and tissues from the autoimmune response of immune cells. However, many solid tumors hijack the adenosine-A2AR pathway by promoting adenosine accumulation. The activation of the A2AR pathway inhibited the immune response of immune cells and then promotes the immune escape of tumor cells in the tumor microenvironment. Recently, both animal experiments and clinical trials indicated that blocking the adenosine pathway can inhibit the progression of a variety of solid tumors. In addition, it is encouraging that A2AR blockade combined with CAR T cells therapy showed better anti-tumor efficacy. Therefore, this review will discuss the role of the adenosine-A2AR pathway in the tumor microenvironment and summarize recent advances of A2AR-cancer related studies.
Introduction
Targeting the adenosine-A2AR pathway is one of the few novel approaches that holds great promise for saving all patients with tumors that are refractory to other therapies, including PD1 blockade, and recent clinical evidence suggests that this approach is effective (1), although much work remains to be done to improve it. Adenosine is an important intermediate for the synthesis of adenosine triphosphate (ATP), adenine, and adenylate (2).In addition, extracellular adenosine also acts as a signal molecule to activate adenosine receptors and plays a more extensive physiological role (3). Adenosine receptor, a GPCR, has been found in four categories: A1R, A2AR, and A3R with high affinity for adenosine, and A2BR with low affinity for adenosine (4–6). Among them, the A2AR is widely distributed on the surface of most immune cells (7–9). The discovery and development of the Hypoxia-A2-adenosinergic Pathway in cancer immunotherapy goes through three phases.
Phase I, the discovery and demonstration of the principle of adenosine -A2AR pathway mediated immunosuppression. Extracellular adenosine binds to A2AR on the surface of immune cells and activates the downstream cAMP pathway, which could lead to inhibition of T cells activation and expansion (10). The adenosine-A2AR pathway is critical and non-redundant in controlling inflammatory damage to overactive anti-pathogen immune cells (11), yet the axis also reduces damage to cancer tissue by anti-tumor killer cells. Studies have shown that the solid tumor microenvironment often presents with transient or chronic hypoxia (12, 13), which facilitates the accumulation of extracellular adenosine (14). Ohta’s team first propose to target the hypoxia-adenosine-A2AR pathway as a cancer immunotherapy strategy and demonstrated that pharmacological or genetic elimination of the extracellular adenosine-A2AR-cAMP axis significantly improves T cell rejection of tumors in an in vivo model of tumor immunotherapy (15).
Phase II, the confirmations, and extensions to clinical trials. CD39 (also known as ENTPD1) being upstream of CD73 in the CD39/CD73 tandem of ectoenzyme, is the dominant ectoenzyme that controls extracellular nucleotide concentrations, which is expressed on the surface of T regulatory cells and generates adenosine to T effector cells with A2AR expression (16–18). Robson’s team demonstrated for the first time that inhibition of extracellular adenosine-mediated signal transduction in CD39-deficient mice affected angiogenesis and tumor growth (19). In addition, Cd39 deletion of bone marrow-derived cells can enhance NK cell-mediated antitumor immunity and inhibit liver metastasis of melanoma (20). Zhang Bin’s team confirmed that CD73-generated adenosine prevents tumor destruction by inhibiting antitumor immunity and CD73 expression in tumor cells negatively regulates T cell response, and knockdown of CD73 expression can prolong the survival of tumor-bearing mice and enhance adoptive T-cell therapy (21). In the same year team of Mark Smyth demonstrated that anti-CD73 antibody therapy inhibits breast tumor growth and metastasis in a mouse model (22). However, it’s worth noting that the blockade of CD73 therapy not only requires adaptive immunity (15, 23) but also requires A2A adenosine receptors on immune cells (22, 24). In addition, anti-hypoxic oxygenation agents or hyperoxic breathing can also act as an “Anti-A2-adenosinergic drug” by inhibiting the expression of HIF-1α, which is upstream of CD39 and CD73 (25–27).
Phase III, the current clinical transformation. The following are some of the methods for blocking or reducing the immunosuppression caused by the Hypoxia/HIF-1α -A2-Adenosinergic pathway. ①Blocking Hypoxia agents, ②Blocking CD39 agents ③Blocking CD73 agents ④A2AR/A2BR antagonist ⑤Block PKA agents (28). Preliminary clinical results have reported that some agents [including anti-CD73 antibody, BMS-986179 (29) and CPI-006 (30); A2AR antagonist NIR178 (PBF-509) (31), inupadenant (EOS-850) (32) and Ciforadenant (CPI-444) (1, 33); A2AR/A2BR dual antagonist, AB928 (34, 35)] show good human tolerability and encouraging cancer treatment effects.
1 Hypoxia and Extracellular Adenosine Accumulation in Cancer
Multiple cell types release adenine nucleotides in the form of ATP, ADP, and AMP when exposed to hypoxia. In addition, intratumoral hypoxia can result in HIF-1α overexpression (36), which promotes the transcription of genes [including CD39 and CD73 which represent the major source of extracellular adenosine in the tumor microenvironment (25)] implicated in important aspects of cancer biology (37).
Here, we summarize the metabolic patterns of extracellular adenosine. There are three ways to produce extracellular adenosine: ① ATP generates ADP under the catalysis of CD39, and ADP continues to generate amp under the catalysis of CD39; AMP generates adenosine under the catalysis of CD73, which is also the most important way of adenosine production. ② nicotinamide adenine dinucleotide (NAD+) generates ADPR through CD38, ADPR generates amp under the catalysis of CD203a, and amp generates adenosine under the action of CD73 ③ S-adenosylhomocysteine (SAH) generates adenosine under S-adenosylhomocysteine hydrolase (SAHH). This enzymatic reaction process is reversible. The metabolism and utilization of adenosine mainly include: ① adenosine is transformed into AMP through adenosine kinase (AK). ② Adenosine is converted to inosine by adenosine deaminase (ADA).
Under the environmental induction of hypoxia and high H +, many tumor cells, such as GBM, LGG, PAAD, and STAD (Figures 1A, B), upregulate the expression of CD39 and CD73. The increased expression of CD73 and CD39 on the tumor surface will lead to the production of adenosine. In addition, hypoxia can also reduce the expression of adenosine kinase and inhibit the transformation of adenosine (38). A high concentration of adenosine further reduced the expression of ADA/CD26 complex, formed positive feedback, and promoted adenosine accumulation (39, 40) Moreover, a large number of experiments have proved that the concentration of adenosine in the tumor microenvironment is much higher than that in normal tissues (41). The accumulation of adenosine was thought to be a major cause of tumor immunosuppression and escape. The median survival time of patients with high CD73 expression in 31 cancers (the median overall survival time is 74.3 months) is significantly lower than that of patients with low CD73 expression (the median overall survival time is 98.3 months), according to survival analysis of TCGA data. Patients with low CD39 expression did not have a better survival prognosis than patients with high CD39 expression, according to the overall survival analysis of CD39 in 31 cancers. However, when the median overall survival time of CD39-high patients was compared to that of CD39-low patients in eight cancers with high CD73 expression, it was discovered that the median overall survival time of CD39-high patients was significantly shorter than that of CD39-low patients (the median overall survival 42.4 months VS 58.6 months). As a result, we believe that CD73, rather than CD39, was a more critical component in adenosine buildup and tumor immunosuppression. Although CD39 is a crucial step in the synthesis of adenosine, its role was redundant, and the CD38-CD203a-CD73 pathway might partially replace CD39’s activity (42).
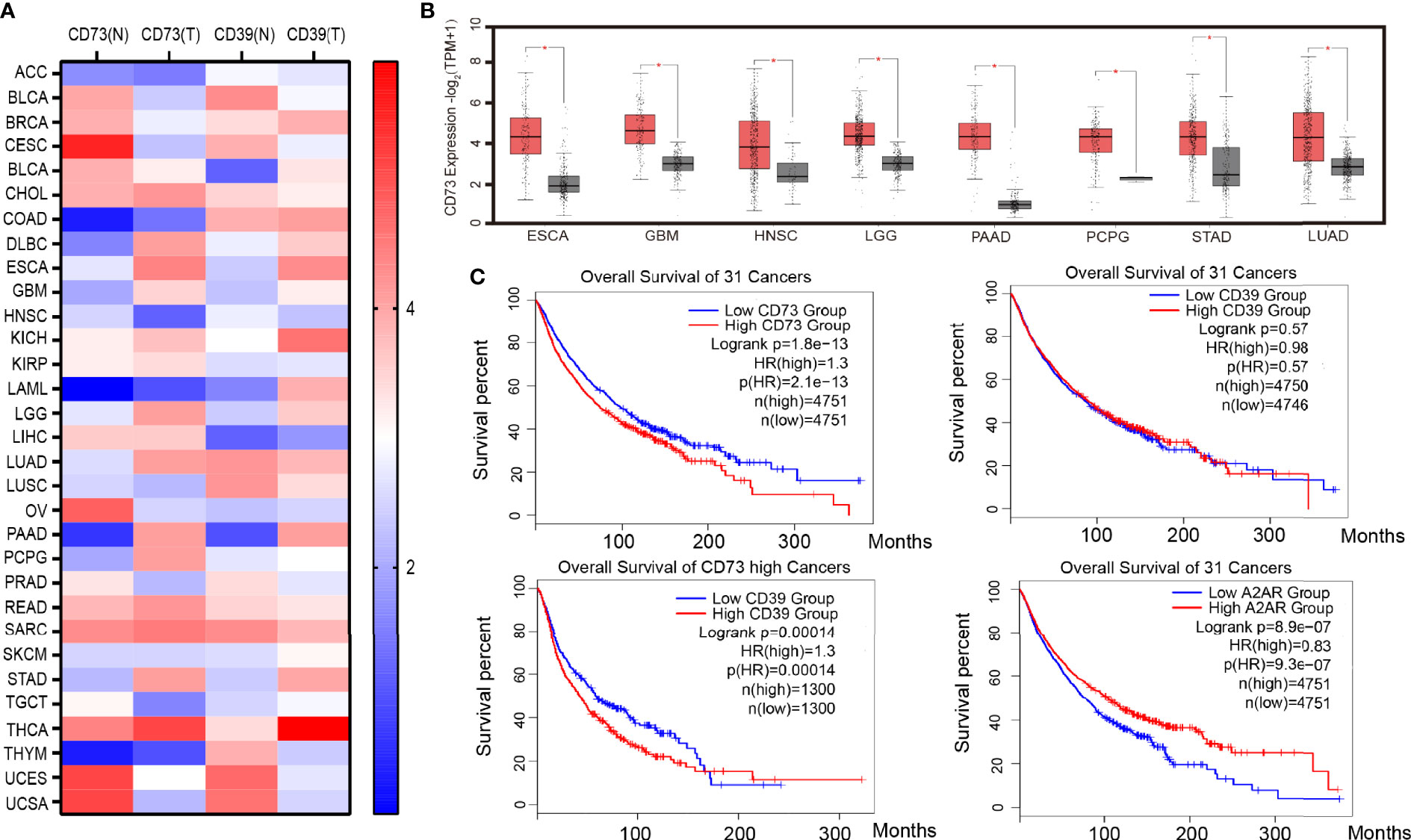
Figure 1 CD39, CD73, and A2AR expression and survival in cancer. (A) Heat map of CD39 and CD73 genes expressed in 31 kinds of tumor tissue and corresponding normal tissue. (B) Cancers with significantly high expression of the CD73 gene. (C) Survival analysis of cancer patients with high or low CD39, CD73 and A2AR genes expression. Data from the TCGA and GTEx studies on RNA sequencing expression in 9,736 cancers and 8,587 normal samples. ACC (Adrenocortical carcinoma), BLCA (Bladder Urothelial Carcinoma), BRCA (Breast invasive carcinoma), CESC (Cervical squamous cell carcinoma and endocervical adenocarcinoma), CHOL (Cholangio carcinoma), COAD (Colon adenocarcinoma), DLBC (Lymphoid Neoplasm Diffuse Large B-cell Lymphoma), ESCA (Esophageal carcinoma), GBM (Glioblastoma multiforme), HNSC (Head and Neck squamous cell carcinoma), KICH(Kidney Chromophobe), KIRC (Kidney renal clear cell carcinoma), KIRP (Kidney renal papillary cell carcinoma), LAML (Acute Myeloid Leukemia), LGG (Brain Lower Grade Glioma), LIHC (Liver hepatocellular carcinoma), LUAD (Lung adenocarcinoma), LUSC (Lung squamous cell carcinoma), MESO (Mesothelioma), OV (Ovarian serous cystadenocarcinoma), PAAD (Pancreatic adenocarcinoma), PCPG (Pheochromocytoma and Paraganglioma), PRAD (Prostate adenocarcinoma), READ (Rectum adenocarcinoma), SARC (Sarcoma), SKCM (Skin Cutaneous Melanoma), STAD (Stomach adenocarcinoma), TGCT (Testicular Germ Cell Tumors), THCA (Thyroid carcinoma), THYM (Thymoma), UCEC (Uterine Corpus Endometrial Carcinoma), UCS (Uterine Carcinosarcoma).
2 A2AR-Adenosine Pathway in Cancer
2.1 Adenosine- A2AR Signaling in Tumor Cells
CD73 promotes the accumulation of adenosine, which activates the A2AR signal pathway in tumor cells, and A2AR signal activation leads to the activation of Rap1, which recruits P110 to the plasma membrane and triggers PIP3 production. After that, the PIK3/AKT signaling pathway is turned on (43). The activation of the PIK3/AKT signal promotes tumor cell EMT (Epithelial-Mesenchymal Transition) and anti-apoptosis, which promote tumor cell growth and metastasis (44). Furthermore, a TCGA survival analysis revealed that tumor patients with high A2AR expression had a worse prognosis (median overall survival was 77.8 months versus 104.6 months) (Figure 1C), implying that tumor cells’ adenosine-A2AR pathway promotes tumor progression
2.2 Adenosine- A2AR Signaling in Immune Cells
Immune cells recognize tumor cell surface antigens and then initiate cellular immunity and kill tumor cells by secreting anti-tumor-related cytokines (INF-γ, TNF-α, and IL-6, etc.) and cell phagocytosis. Most immune cells express A2AR on the surface. As GPCR, A2AR on the surface of the immune cell together with intracellular Gα (mainly Gαs subunit) and Gβ-Gγ form a complex. Gαs dissociated with Gβ-Gγ after adenosine binding to A2AR. Dissociated Gαs activates AC enzymes (such as ADCY1 and ADCY9), AC decomposes intracellular ATP to produce diphosphate and cAMP. PKA is activated by cAMP, an intracellular second messenger. Under the action of PKA, CREB gets phosphorylated. Cre (cAMP response element) is bound by phosphorylated CREB and other proteins such as the CBP-p300 complex, and then IL-10, Foxp3, and other factors can begin to be expressed. Furthermore, suppression of phosphorylated CREB, can trigger the expression of cytokines such as TNF-α, IL-1β, IL-6, and NOS2 (Figure 2).
2.2.1 T Cells Respond to the Adenosine - A2AR Signaling
Cellular immunity mediated by T-lymphocytic subsets such as CD4+ T and CTL is an important way for the body to eliminate the threat of tumor cells. Antigen activation of TCR on CD4+ T cells surface resulted in up-regulation of INF-γ, CD25, and CD69 expression (45), Adenosine inhibits TCR and IL-2 receptor triggered signal transduction by activating (46). In addition, in vitro experiments showed that the gene dose effect affected the inhibition degree. The maximum inhibition degree of A2AR-/+ CD4+ T lymphocytes by A2AR agonist was 50 percent, and the maximum inhibition degree of A2AR-/- CD4+ T lymphocytes was 100 percent (45). Naïve T cell is called Th0, it could develop into various CD4+ T cell subsets in response to various cytokines. CD4+ T cells can be classified into THL, Th2, Th17, Treg, Th9, and Th22 subsets based on the secreted cytokine spectrum. Among them, Th1 (secreting cytokines such as INF-γ, IL-2, TNF, and IL12) mainly mediates the cellular immune response. It is worth noting that INF-γ and IL-2 can not only promote the development and proliferation of Th1 but also inhibit the differentiation of Th0 into Th2 and Th17 (47). Activation of the adenosine -A2AR pathway leads to the reduction of Th1 induced by decreasing number of cytokines like INF-γ and IL-2. Adenosine and A2AR agonists(For example ATL146e, ATL370, and ATL1223)have been shown to enhance the TGFβ-induced generation of FoxP3+ Tregs (48). In addition to protecting the host from pathogen invasion, the human immune system is responsible for inhibiting the immune response to self-antigens and preventing an excessive immune response from causing damage to the host. And this activity is primarily mediated by regulatory T cells (Tregs) that specialize in immunosuppression (49, 50). However, the increase of CD4+ Foxp3+ Treg inhibited the antitumor effect (51). Blocking A2AR in vivo using the antagonist SCH58261 inhibited tumor growth, induced the decrease of CD4+ Foxp3+ Treg cells, and enhanced the antitumor response of T cells (52).
The main way of adenosine immunosuppression is to block the cytotoxic function of CD8+ T cells through the A2AR signal. This promotes the immune escape of tumor cells. In B16F10 and SM1WT1 tumor models of A2AR deficient mice, the infiltration of CD8+ T cells into tumors was enhanced, which inhibited the growth and metastasis of tumors (53). In an HNSCC mouse tumor model, it was found that blocking A2AR with SCH58261, a small molecule inhibitor could increase the number of tumor-infiltrating CD8+ T cells, at the same time, the function of CD8+ T cells was enhanced (INF-γ and TNF-α expression increased) (52). By analyzing adenosine-mediated the immunosuppression on CD8+ T cells at different differentiation stages (T central memory-TCM, T effector memory-TEM, T terminally differentiated-TEMRA), it was found that the expression level of A2AR in CD8+ T cells determined the sensitivity to adenosine, TCM was more sensitive to adenosine, and its cytokine secretion was more inhibited than TEM and TEMRA subgroups (54). However, the inhibition degree of adenosine on T cell’s chemotaxis did not depend on the expression level of A2AR. The increased sensitivity of CD8+ T cells to adenosine was associated with the decrease of KCa3.1 potassium channel activity, but not with adenosine receptor expression or signal transduction. KCa3.1 channel agonist treatment can restore the migration of CD8+ T cells in the presence of adenosine (55). Moreover, the adenosine-A2AR signaling regulates PKA and mTORC1 activation and impairs the metabolic fitness of CD8+ T cells (56).
2.2.2 NK Cells Respond to the Adenosine - A2AR Signaling
Natural killer (NK) cells, which can destroy target cells without antigen pre sensitization, have no MHC restriction and fast response speed in tumor immunity. Adenosine-mediated A2AR signal is an internal negative regulator of NK cell maturation and antitumor immune response. The adenosine- A2AR signaling limits NK cell maturation and proliferation. NK cell-specific A2AR-deletion, which promotes the maturation and proliferation of NK cells (7). The adenosine- A2AR signaling not only reduces the expression of proinflammatory factors (such as IFN-γ, IL-2, TNF-α, GM-GSF, and MIP-1α), it can also reduce the expression of perforin, which is essential for NC cells to play a cytotoxic role (57, 58). In addition, the cleavage ability of NK cells dependent on the FASL pathway was also significantly reduced during this process (59).
2.2.3 Macrophages Respond to the Adenosine - A2AR Signaling
It is generally believed that macrophages are derived from monocytes. And macrophages will differentiate into M1 cells, mainly playing roles in promoting inflammation, sterilization, and anti-tumor, stimulated by factors such as TLR, TNF-α, IFN-γ, CSF2. Stimulated by cytokines like IL4, IL-10, IL-13, TGF-β, and PGE2, macrophages will differentiate into M2 subtype, which could inhibit inflammation and promote wound repair, but also promote tumor proliferation and metastasis (60). Macrophages polarize into an M2 phenotype due to increased IL-10 and decreased TLR, TNF-α, IFN-γ, which is caused by the activation of the adenosine-A2AR pathway (61). Macrophages are the key mediators of ADCP (antibody-mediated cellular phagocytosis) and can be observed in a large number in the tumor microenvironment (62, 63). Adenosine signaling can damage macrophage antibody-mediated ADCP, acting as a “don’t eat me signal”. In a xenograft lymphoma model, A2AR antagonist drug inhibition overcomes adenosine-mediated negative regulation of ADCP (64). In conclusion, the adenosine-A2AR signaling leads to the low pro-inflammatory and defensive immune activity of macrophages under tumor conditions, showing more phenotypic and functional characteristics of M2 type, and abnormally secreting growth factors such as VEGF. All these promote the proliferation and metastasis of tumors.
3 A2AR Antagonist and Clinical Progress
The discovery of the important role of the adenosine-A2AR pathway in the human body [not only in cancer but also in other diseases such as Parkinson’s disease (67, 68)], has made the development of effective and selective A2A adenosine receptor antagonists an appealing research field. Adenosine receptor antagonists can be divided into two groups: xanthine derivatives and nitrogen poliheterocyclic systems (69). However, some of these compounds have issues that prevent them from being used in clinical trials, such as low water solubility (SCH58261, and xanthine derivatives) or high affinity for the A2B adenosine receptor subtype (ZM241385) (69).
Currently, clinically tested A2AR antagonists include CPI-444, NIR178, AZD4635, etc (Table 1). The first clinical report demonstrating antagonistic effects of the adenosine pathway in cancer immunotherapy was published in 2020. In this clinical trial, 68 renal cell cancer patients (most of them resistant or refractory to anti-PD-1/PD-L1 antibodies and had predominantly PD-L1-negative tumors) received either ciforadenant or ciforadenant plus atezolizumab therapy. This study demonstrated the antitumor activity of both monotherapy and anti-PD-L1 combination therapy in refractory renal cell cancer patients. Median progression-free survival 4.1 months with monotherapy vs 5.8 months with combination and overall survival >69% at 16 months vs >90% at 25 months were observed. In many other trials, A2AR antagonists immunotherapy also has shown activity in many types of cancer patients (31, 33, 35). These encouraging findings support the adenosine axis as a feasible immunotherapy target, but further research is needed to establish which drug or combination would be most effective.
Here, we list some other high affinity and selective adenosine A2AR inhibitors with potential clinical application in Figure 3. With a Ki value of 1.4 nM, ZM241385 is a strong, high affinity, and specific adenosine A2AR antagonist (70, 71). Istradefylline, with a Ki of 2.2 nM, is an extremely strong, selective, and orally active adenosine A2A receptor antagonist (72).. SCH58261 is a potent, selective, and competitive antagonist of adenosine A2A receptor with an IC50 of 15 nM, and displays 323-, 53- and 100-fold more selective for A2A receptor than A1, A2B, and A3 receptors, respectively (73–75). With a Ki of 1.1 nM and over 1000-fold selectivity over other adenosine receptors, Preladenant (SCH-420814) is a powerful and competitive antagonist of the human adenosine A2A receptor (76). With a Ki of 0.048 for human A2AR, SCH442416 is a strong, selective, and brain-penetrant antagonist of A2AR. It is 23145-, 208333-, and 208333-fold more selective for A2A receptor than A1, A2B, and A3 (77). With Ki values of 4 nM, A2A receptor antagonist 1 (CPI-444 analog) is a selective antagonist of adenosine A2A receptor and has over 66-fold selectivity over adenosine A1 receptor (78).
Conclusion
Hypoxia-induced adenosine accumulation in the tumor microenvironment is common in a variety of tumors. The activation of the adenosine A2AR signaling pathway leads to immunosuppression and tumor EMT and anti-apoptosis, which promote the process of tumor development. A2AR antagonist blocking, or A2AR knockout of immune cells, indicates that blocking the adenosine-A2AR signaling pathway can significantly improve the antitumor effect. At present, A2AR inhibitors have shown gratifying effects in many clinical trials of cancer. However, for some patients, the lack of immune cells, or the mutation or deletion of cancer cell antigen leads to the unrecognition of T cell surface receptor TCR. As a result, A2AR inhibitors cannot exert their effect. The combination of A2AR inhibitors and CAR T therapy or other drugs can effectively improve the antitumor effect and greatly expand the application scenario of A2AR antagonists. Future combinations of A2AR inhibitors and CAR T may become the most effective and highly regarded cancer therapies.
Author Contributions
All authors listed have made a substantial, direct, and intellectual contribution to the work and approved it for publication.
Funding
This work was funded by the National Natural Science Foundation of China (NO. 11972099).
Conflict of Interest
The authors declare that the research was conducted in the absence of any commercial or financial relationships that could be construed as a potential conflict of interest.
Publisher’s Note
All claims expressed in this article are solely those of the authors and do not necessarily represent those of their affiliated organizations, or those of the publisher, the editors and the reviewers. Any product that may be evaluated in this article, or claim that may be made by its manufacturer, is not guaranteed or endorsed by the publisher.
References
1. Fong L, Hotson A, Powderly JD, Sznol M, Heist RS, Choueiri TK, et al. Adenosine 2a Receptor Blockade as an Immunotherapy for Treatment-Refractory Renal Cell Cancer. Cancer Discov (2020) 10(1):40–53. doi: 10.1158/2159-8290.Cd-19-0980
2. Pelleg A, Porter RS. The Pharmacology of Adenosine. Pharmacotherapy (1990) 10(3):157–74. doi: 10.1002/j.1875-9114.1990.tb02573.x
3. Leiva A, Guzman-Gutierrez E, Contreras-Duarte S, Fuenzalida B, Cantin C, Carvajal L, et al. Adenosine Receptors: Modulators of Lipid Availability That are Controlled by Lipid Levels. Mol Aspects Med (2017) 55:26–44. doi: 10.1016/j.mam.2017.01.007
4. Robeva AS, Woodard RL, Jin XW, Gao ZH, Bhattacharya S, Taylor HE, et al. Molecular Characterization of Recombinant Human Adenosine Receptors. Drug Dev Res (1996) 39(3-4):243–52. doi: 10.1002/(Sici)1098-2299(199611/12)39:3/4<243::Aid-Ddr3>3.0.Co;2-R
5. Muller-Haegele S, Muller L, Whiteside TL. Immunoregulatory Activity of Adenosine and its Role in Human Cancer Progression. Expert Rev Clin Immunol (2014) 10(7):897–914. doi: 10.1586/1744666X.2014.915739
6. Olah ME, Stiles GL. Adenosine Receptor Subtypes: Characterization and Therapeutic Regulation. Annu Rev Pharmacol Toxicol (1995) 35:581–606. doi: 10.1146/annurev.pa.35.040195.003053
7. Young A, Ngiow SF, Gao YL, Patch AM, Barkauskas DS, Messaoudene M, et al. A2AR Adenosine Signaling Suppresses Natural Killer Cell Maturation in the Tumor Microenvironment. Cancer Res (2018) 78(4):1003–16. doi: 10.1158/0008-5472.Can-17-2826
8. Halpin-Veszeleiova K, Hatfield SM. Oxygenation and A2AR Blockade to Eliminate Hypoxia/HIF-1alpha-Adenosinergic Immunosuppressive Axis and Improve Cancer Immunotherapy. Curr Opin Pharmacol (2020) 53:84–90. doi: 10.1016/j.coph.2020.07.005
9. Ohta A, Sitkovsky M. The Adenosinergic Immunomodulatory Drugs. Curr Opin Pharmacol (2009) 9(4):501–6. doi: 10.1016/j.coph.2009.05.005
10. Huang S, Apasov S, Koshiba M, Sitkovsky M. Role of A2a Extracellular Adenosine Receptor-Mediated Signaling in Adenosine-Mediated Inhibition of T-Cell Activation and Expansion. Blood (1997) 90(4):1600–10. doi: 10.1182/blood.V90.4.1600.1600_1600_1610
11. Ohta A, Sitkovsky M. Role of G-Protein-Coupled Adenosine Receptors in Downregulation of Inflammation and Protection From Tissue Damage. Nature (2001) 414(6866):916–20. doi: 10.1038/414916a
12. Harris AL. Hypoxia - A Key Regulatory Factor in Tumour Growth. Nat Rev Cancer (2002) 2(1):38–47. doi: 10.1038/nrc704
13. Giatromanolaki A, Sivridis E, Kouskoukis C, Gatter KC, Harris AL, Koukourakis MI. Hypoxia-Inducible Factors 1 Alpha and 2 Alpha are Related to Vascular Endothelial Growth Factor Expression and a Poorer Prognosis in Nodular Malignant Melanomas of the Skin. Melanoma Res (2003) 13(5):493–501. doi: 10.1097/00008390-200310000-00008
14. Decking UKM, Schlieper G, Kroll K, Schrader J. Hypoxia-Induced Inhibition of Adenosine Kinase Potentiates Cardiac Adenosine Release. Circ Res (1997) 81(2):154–64. doi: 10.1161/01.Res.81.2.154
15. Ohta A, Gorelik E, Prasad SJ, Ronchese F, Lukashev D, Wong MK, et al. A2A Adenosine Receptor Protects Tumors From Antitumor T Cells. Proc Natl Acad Sci USA (2006) 103(35):13132–7. doi: 10.1073/pnas.0605251103
16. Kaczmarek E, Koziak K, Sevigny J, Siegel JB, Anrather J, Beaudoin AR, et al. Identification and Characterization of CD39 Vascular ATP Diphosphohydrolase. J Biol Chem (1996) 271(51):33116–22. doi: 10.1074/jbc.271.51.33116
17. Deaglio S, Dwyer KM, Gao W, Friedman D, Usheva A, Erat A, et al. Adenosine Generation Catalyzed by CD39 and CD73 Expressed on Regulatory T Cells Mediates Immune Suppression. J Exp Med (2007) 204(6):1257–65. doi: 10.1084/jem.20062512
18. Dwyer KM, Deaglio S, Gao W, Friedman D, Strom TB, Robson SC. CD39 and Control of Cellular Immune Responses. Purinerg Signal (2007) 3(1-2):171–80. doi: 10.1007/s11302-006-9050-y
19. Jackson SW, Hoshi T, Wu Y, Sun X, Enjyoji K, Cszimadia E, et al. Disordered Purinergic Signaling Inhibits Pathological Angiogenesis in Cd39/Entpd1-Null Mice. Am J Pathol (2007) 171(4):1395–404. doi: 10.2353/ajpath.2007.070190
20. Sun XF, Wu Y, Gao WD, Enjyoji K, Csizmadia E, Muller CE, et al. CD39/ENTPD1 Expression by CD4(+)Foxp3(+) Regulatory T Cells Promotes Hepatic Metastatic Tumor Growth in Mice. Gastroenterology (2010) 139(3):1030–40. doi: 10.1053/j.gastro.2010.05.007
21. Jin D, Fan J, Wang L, Thompson LF, Liu A, Daniel BJ, et al. CD73 on Tumor Cells Impairs Antitumor T-Cell Responses: A Novel Mechanism of Tumor-Induced Immune Suppression. Cancer Res (2010) 70(6):2245–55. doi: 10.1158/0008-5472.CAN-09-3109
22. Stagg J, Divisekera U, McLaughlin N, Sharkey J, Pommey S, Denoyer D, et al. Anti-CD73 Antibody Therapy Inhibits Breast Tumor Growth and Metastasis. Proc Natl Acad Sci USA (2010) 107(4):1547–52. doi: 10.1073/pnas.0908801107
23. Sitkovsky MV. Sufficient Numbers of Anti-Tumor T Cells is a Condition of Maximal Efficacy of Anti-Hypoxia-A2-Adenosinergic Drugs During Cancer Immunotherapy. Curr Opin Pharmacol (2020) 53:98–100. doi: 10.1016/j.coph.2020.07.011
24. Armstrong JM, Chen JF, Schwarzschild MA, Apasov S, Smith PT, Caldwell C, et al. Gene Dose Effect Reveals No Gs-Coupled A2A Adenosine Receptor Reserve in Murine T-Lymphocytes: Studies of Cells From A2A-Receptor-Gene-Deficient Mice. Biochem J (2001) 354(Pt 1):123–30. doi: 10.1042/0264-6021:3540123
25. Hatfield SM, Kjaergaard J, Lukashev D, Belikoff B, Schreiber TH, Sethumadhavan S, et al. Systemic Oxygenation Weakens the Hypoxia and Hypoxia Inducible Factor 1alpha-Dependent and Extracellular Adenosine-Mediated Tumor Protection. J Mol Med (Berl) (2014) 92(12):1283–92. doi: 10.1007/s00109-014-1189-3
26. Hatfield SM, Kjaergaard J, Lukashev D, Schreiber TH, Belikoff B, Abbott R, et al. Immunological Mechanisms of the Antitumor Effects of Supplemental Oxygenation. Sci Transl Med (2015) 7(277):277ra30. doi: 10.1126/scitranslmed.aaa1260
27. Hatfield SM, Sitkovsky MV. Antihypoxic Oxygenation Agents With Respiratory Hyperoxia to Improve Cancer Immunotherapy. J Clin Invest (2020) 130(11):5629–37. doi: 10.1172/Jci137554
28. Sitkovsky MV. Lessons From the A2A Adenosine Receptor Antagonist-Enabled Tumor Regression and Survival in Patients With Treatment-Refractory Renal Cell Cancer. Cancer Discov (2020) 10(1):16–9. doi: 10.1158/2159-8290.Cd-19-1280
29. Siu LL, Burris H, Le DT, Hollebecque A, Steeghs N, Delord JP, et al. Preliminary Phase 1 Profile of BMS-986179, an Anti-CD73 Antibody, in Combination With Nivolumab in Patients With Advanced Solid Tumors. Cancer Res (2018) 78(13):CT180. doi: 10.1158/1538-7445.Am2018-Ct180
30. Luke J, Merchan J, Harshman L, Marron T, Powderly J, Barve M, et al. Immunobiology and Clinical Activity of CPI-006, an Anti-CD73 Antibody With Immunomodulating Properties in a Phase 1/1b Trial in Advanced Cancers. J Immunother Cancer (2019) 37(15_suppl):2505. doi: 10.1200/JCO.2019.37.15_suppl.2505
31. Chiappori A, Williams CC, Creelan B, Tanvetyanon T, Gray JE, Haura EB, et al. Phase I/II Study of the A2AR Antagonist NIR178 (PBF-509), an Oral Immunotherapy, in Patients (Pts) With Advanced NSCLC. J Clin Oncol (2018) 36(15_suppl):9089. doi: 10.1200/JCO.2018.36.15_suppl.9089
32. Buisseret L, Rottey S, De Bono JS, Migeotte A, Delafontaine B, Manickavasagar T, et al. Phase 1 Trial of the Adenosine A2A Receptor Antagonist Inupadenant (EOS-850): Update on Tolerability, and Antitumor Activity Potentially Associated With the Expression of the A2A Receptor Within the Tumor. J Clin Oncol (2021) 39(15_suppl):2562. doi: 10.1200/JCO.2021.39.15_suppl.2562
33. Harshman LC, Chu M, George S, Gordon B, Hughes M, Carthon BC, et al. Adenosine Receptor Blockade With Ciforadenant Plus /- Atezolizumab in Advanced Metastatic Castration-Resistant Prostate Cancer (mCRPC). J Clin Oncol (2020) 38(6_suppl):129. doi: 10.1200/JCO.2020.38.6_suppl.129
34. Seitz L, Jin LX, Leleti M, Ashok D, Jeffrey J, Rieger A, et al. Safety, Tolerability, and Pharmacology of AB928, a Novel Dual Adenosine Receptor Antagonist, in a Randomized, Phase 1 Study in Healthy Volunteers. Invest New Drug (2019) 37(4):711–21. doi: 10.1007/s10637-018-0706-6
35. Powderly J, Spira A, Gutierrez R, DiRenzo D, Udyavar A, Karakunnel JJ, et al. Phase I Evaluation of AB928, a Novel Dual Adenosine Receptor Antagonist, Combined With Chemotherapy or AB122 (Anti-PD-1) in Patients (Pts) With Advanced Malignancies. Ann Oncol (2019) 30(5_suppl). doi: 10.1093/annonc/mdz253.032
36. Wang GL, Jiang BH, Rue EA, Semenza GL. Hypoxia-Inducible Factor-1 Is a Basic-Helix-Loop-Helix-Pas Heterodimer Regulated by Cellular O-2 Tension. Proc Natl Acad Sci USA (1995) 92(12):5510–4. doi: 10.1073/pnas.92.12.5510
37. Semenza GL. Targeting HIF-1 for Cancer Therapy. Nat Rev Cancer (2003) 3(10):721–32. doi: 10.1038/nrc1187
38. Morote-Garcia JC, Rosenberger P, Kuhlicke J, Eltzschig HK. HIF-1-Dependent Repression of Adenosine Kinase Attenuates Hypoxia-Induced Vascular Leak. Blood (2008) 111(12):5571–80. doi: 10.1182/blood-2007-11-126763
39. Linden J. Adenosine Metabolism and Cancer. Focus on "Adenosine Downregulates DPPIV on HT-29 Colon Cancer Cells by Stimulating Protein Tyrosine Phosphatases and Reducing ERK1/2 Activity via a Novel Pathway". Am J Physiol-Cell Ph (2006) 291(3):C405–C6. doi: 10.1152/ajpcell.00242.2006
40. Clayton A, Al-Taei S, Webber J, Mason MD, Tabi Z. Cancer Exosomes Express CD39 and CD73, Which Suppress T Cells Through Adenosine Production. J Immunol (2011) 187(2):676–83. doi: 10.4049/jimmunol.1003884
41. Hatfield SM, Kjaergaard J, Lukashev D, Belikoff B, Schreiber TH, Sethumadhavan S. Systemic Oxygenation Weakens the Hypoxia and Hypoxia Inducible Factor 1alpha-Dependent and Extracellular Adenosine-Mediated Tumor Protection. J Mol Med (Berl) (2014) 92(12):1283–92. doi: 10.1007/s00109-014-1189-3
42. Horenstein AL, Chillemi A, Zaccarello G, Bruzzone S, Quarona V, Zito A, et al. A CD38/CD203a/CD73 Ectoenzymatic Pathway Independent of CD39 Drives a Novel Adenosinergic Loop in Human T Lymphocytes. Oncoimmunology (2013) 2(9):e26246. doi: 10.4161/onci.26246
43. Ma XL, Shen MN, Hu B, Wang BL, Yang WJ, Lv LH, et al. CD73 Promotes Hepatocellular Carcinoma Progression and Metastasis via Activating PI3K/AKT Signaling by Inducing Rap1-Mediated Membrane Localization of P110 and Predicts Poor Prognosis. J Hematol Oncol (2019) 12(1):37. doi: 10.1186/s13045-019-0724-7
44. Chen B, Zeng X, He Y, Wang XX, Liang ZW, Liu JJ, et al. STC2 Promotes the Epithelial-Mesenchymal Transition of Colorectal Cancer Cells Through AKT-ERK Signaling Pathways. Oncotarget (2016) 7(44):71400–16. doi: 10.18632/oncotarget.12147
45. Lappas CM, Rieger JM, Linden J. A2A Adenosine Receptor Induction Inhibits IFN-Gamma Production in Murine CD4+ T Cells. J Immunol (2005) 174(2):1073–80. doi: 10.4049/jimmunol.174.2.1073
46. Newick K, O’Brien S, Sun J, Kapoor V, Maceyko S, Lo A, et al. Augmentation of CAR T-Cell Trafficking and Antitumor Efficacy by Blocking Protein Kinase A Localization. Cancer Immunol Res (2016) 4(6):541–51. doi: 10.1158/2326-6066.CIR-15-0263
47. Berthelot JM, Maugars Y. Role for Suppressor T Cells in the Pathogenesis of Autoimmune Diseases (Including Rheumatoid Arthritis). Facts and Hypotheses. Joint Bone Spine (2004) 71(5):374–80. doi: 10.1016/j.jbspin.2003.11.004
48. Han KL, Thomas SV, Koontz SM, Changpriroa CM, Ha SK, Malech HL, et al. Adenosine a(2)A Receptor Agonist-Mediated Increase in Donor-Derived Regulatory T Cells Suppresses Development of Graft-Versus-Host Disease. J Immunol (2013) 190(1):458–68. doi: 10.4049/jimmunol.1201325
49. Sakaguchi S, Yamaguchi T, Nomura T, Ono M. Regulatory T Cells and Immune Tolerance. Cell (2008) 133(5):775–87. doi: 10.1016/j.cell.2008.05.009
50. Jones KR, Kang EM. Graft Versus Host Disease: New Insights Into A(2A) Receptor Agonist Therapy. Comput Struct Biotec (2015) 13:101–5. doi: 10.1016/j.csbj.2014.12.003
51. Sitkovsky MV. T Regulatory Cells: Hypoxia-Adenosinergic Suppression and Re-Direction of the Immune Response. Trends Immunol (2009) 30(3):102–8. doi: 10.1016/j.it.2008.12.002
52. Ma SR, Deng WW, Liu JF, Mao L, Yu GT, Bu LL, et al. Blockade of Adenosine A2A Receptor Enhances CD8(+) T Cells Response and Decreases Regulatory T Cells in Head and Neck Squamous Cell Carcinoma. Mol Cancer (2017) 16(1):99. doi: 10.1186/s12943-017-0665-0
53. Young A, Ngiow SF, Barkauskas DS, Sult E, Hay C, Blake SJ, et al. Co-Inhibition of CD73 and A2AR Adenosine Signaling Improves Anti-Tumor Immune Responses. Cancer Cell (2016) 30(3):391–403. doi: 10.1016/j.ccell.2016.06.025
54. Mastelic-Gavillet B, Navarro Rodrigo B, Decombaz L, Wang H, Ercolano G, Ahmed R, et al. Adenosine Mediates Functional and Metabolic Suppression of Peripheral and Tumor-Infiltrating CD8(+) T Cells. J Immunother Cancer (2019) 7(1):257. doi: 10.1186/s40425-019-0719-5
55. Chimote AA, Balajthy A, Arnold MJ, Newton HS, Hajdu P, Qualtieri J, et al. A Defect in KCa3.1 Channel Activity Limits the Ability of CD8(+) T Cells From Cancer Patients to Infiltrate an Adenosine-Rich Microenvironment. Sci Signal (2018) 11(527):eaaq1616. doi: 10.1126/scisignal.aaq1616
56. Chang CH, Pearce EL. Emerging Concepts of T Cell Metabolism as a Target of Immunotherapy. Nat Immunol (2016) 17(4):364–8. doi: 10.1038/ni.3415
57. Vigano S, Alatzoglou D, Irving M, Menetrier-Caux C, Caux C, Romero P, et al. Targeting Adenosine in Cancer Immunotherapy to Enhance T-Cell Function. Front Immunol (2019) 10:925. doi: 10.3389/fimmu.2019.00925
58. Antonioli L, Blandizzi C, Pacher P, Hasko G. Immunity, Inflammation and Cancer: A Leading Role for Adenosine. Nat Rev Cancer (2013) 13(12):842–57. doi: 10.1038/nrc3613
59. Raskovalova T, Huang X, Sitkovsky M, Zacharia LC, Jackson EK, Gorelik E. Gs Protein-Coupled Adenosine Receptor Signaling and Lytic Function of Activated NK Cells. J Immunol (2005) 175(7):4383–91. doi: 10.4049/jimmunol.175.7.4383
60. Jayasingam SD, Citartan M, Thang TH, Zin AAM, Ang KC, Ch’ng ES. Evaluating the Polarization of Tumor-Associated Macrophages Into M1 and M2 Phenotypes in Human Cancer Tissue: Technicalities and Challenges in Routine Clinical Practice. Front Oncol (2020) 9:1512. doi: 10.3389/fonc.2019.01512
61. Ludwig N, Yerneni SS, Azambuja JH, Gillespie DG, Menshikova EV, Jackson EK, et al. Tumor-Derived Exosomes Promote Angiogenesis via Adenosine A(2B) Receptor Signaling. Angiogenesis (2020) 23(4):599–610. doi: 10.1007/s10456-020-09728-8
62. Scott DW, Gascoyne RD. The Tumour Microenvironment in B Cell Lymphomas. Nat Rev Cancer (2014) 14(8):517–34. doi: 10.1038/nrc3774
63. Keane C, Vari F, Hertzberg M, Le Cao KA, Green MR, Han E, et al. Ratios of T-Cell Immune Effectors and Checkpoint Molecules as Prognostic Biomarkers in Diffuse Large B-Cell Lymphoma: A Population-Based Study. Lancet Haematol (2015) 2(10):E445–E55. doi: 10.1016/S2352-3026(15)00150-7
64. Nakamura K, Casey M, Oey H, Vari F, Stagg J, Gandhi MK, et al. Targeting an Adenosine-Mediated "Don’t Eat Me Signal" Augments Anti-Lymphoma Immunity by Anti-CD20 Monoclonal Antibody. Leukemia (2020) 34(10):2708–21. doi: 10.1038/s41375-020-0811-3
65. Willingham S, Hotson A, Ho P, Choy C, Laport G, McCaffery I, et al. CPI-444: A Potent and Selective Inhibitor of A2AR Induces Antitumor Responses Alone and in Combination With Anti-PD-L1 in Preclinical and Clinical Studies. Cancer Immunol Res (2016) 4(11_suppl):PR04. doi: 10.1158/2326-6066.Imm2016-Pr04
66. Borodovsky A, Wang YJ, Ye MW, Shaw JC, Sachsenmeier KF, Deng NH, et al. Preclinical Pharmacodynamics and Antitumor Activity of AZD4635, a Novel Adenosine 2A Receptor Inhibitor That Reverses Adenosine Mediated T Cell Suppression. Cancer Res (2017) 77 (13_suppl):5580. doi: 10.1158/1538-7445.Am2017-5580
67. Armentero MT, Pinna A, Ferre S, Lanciego JL, Muller CE, Franco R. Past, Present and Future of A(2A) Adenosine Receptor Antagonists in the Therapy of Parkinson’s Disease. Pharmacol Ther (2011) 132(3):280–99. doi: 10.1016/j.pharmthera.2011.07.004
68. Moreira-de-Sa A, Lourenco VS, Canas PM, Cunha RA. Adenosine A(2A) Receptors as Biomarkers of Brain Diseases. Front Neurosci-Switz (2021) 15:702581. doi: 10.3389/fnins.2021.702581
69. Cacciari B, Pastorin G, Spalluto G. Medicinal Chemistry of A2A Adenosine Receptor Antagonists. Curr Top Med Chem (2003) 3(4):403–11. doi: 10.2174/1568026033392183
70. Wang ZY, Che PL, Du JA, Ha B, Yarema KJ. Static Magnetic Field Exposure Reproduces Cellular Effects of the Parkinson’s Disease Drug Candidate Zm241385. PloS One (2010) 5(11):e13883. doi: 10.1371/journal.pone.0013883
71. Poucher SM, Keddie JR, Singh P, Stoggall SM, Caulkett PW, Jones G, et al. The In Vitro Pharmacology of ZM 241385, a Potent, non-Xanthine A2a Selective Adenosine Receptor Antagonist. Br J Pharmacol (1995) 115(6):1096–102. doi: 10.1111/j.1476-5381.1995.tb15923.x
72. Chen JF, Xu K, Petzer JP, Staal R, Xu YH, Beilstein M, et al. Neuroprotection by Caffeine and A(2A) Adenosine Receptor Inactivation in a Model of Parkinson’s Disease. J Neurosci (2001) 21(10):RC143. doi: 10.1523/JNEUROSCI.21-10-j0001.2001
73. Zocchi C, Ongini E, Ferrara S, Baraldi PG, Dionisotti S. Binding of the Radioligand [3H]-SCH 58261, a New non-Xanthine A2A Adenosine Receptor Antagonist, to Rat Striatal Membranes. Br J Pharmacol (1996) 117(7):1381–6. doi: 10.1111/j.1476-5381.1996.tb15296.x
74. Varani K, Gessi S, Dalpiaz A, Borea PA. Pharmacological and Biochemical Characterization of Purified A2a Adenosine Receptors in Human Platelet Membranes by [3H]-CGS 21680 Binding. Br J Pharmacol (1996) 117(8):1693–701. doi: 10.1111/j.1476-5381.1996.tb15341.x
75. Xi J, McIntosh R, Shen X, Lee S, Chanoit G, Criswell H, et al. Adenosine A2A and A2B Receptors Work in Concert to Induce a Strong Protection Against Reperfusion Injury in Rat Hearts. J Mol Cell Cardiol (2009) 47(5):684–90. doi: 10.1016/j.yjmcc.2009.08.009
76. Hodgson RA, Bertorelli R, Varty GB, Lachowicz JE, Forlani A, Fredduzzi S, et al. Characterization of the Potent and Highly Selective A2A Receptor Antagonists Preladenant and SCH 412348 [7-[2-[4-2,4-Difluorophenyl]-1-Piperazinyl]Ethyl]-2-(2-Furanyl)-7H-Pyrazolo[4,3-E ][1,2,4]Triazolo[1,5-C]Pyrimidin-5-Amine] in Rodent Models of Movement Disorders and Depression. J Pharmacol Exp Ther (2009) 330(1):294–303. doi: 10.1124/jpet.108.149617
77. Todde S, Moresco RM, Simonelli F, Baraldi PG, Cacciari B, Spalluto G, et al. Design, Radiosynthesis, and Biodistribution of a New Potent and Selective Ligand for In Vivo Imaging of the Adenosine A(2A) Receptor System Using Positron Emission Tomography. J Med Chem (2000) 43(23):4359–62. doi: 10.1021/jm0009843
78. Gillespie RJ, Cliffe IA, Dawson CE, Dourish CT, Gaur S, Jordan AM, et al. Antagonists of the Human Adenosine A2A Receptor. Part 3: Design and Synthesis of Pyrazolo[3,4-D]Pyrimidines, Pyrrolo[2,3-D]Pyrimidines and 6-Arylpurines. Bioorg Med Chem Lett (2008) 18(9):2924–9. doi: 10.1016/j.bmcl.2008.03.072
Keywords: adenosine, A2AR, antagonist, immunosuppression, tumor microenvironment
Citation: Sun C, Wang B and Hao S (2022) Adenosine-A2A Receptor Pathway in Cancer Immunotherapy. Front. Immunol. 13:837230. doi: 10.3389/fimmu.2022.837230
Received: 17 December 2021; Accepted: 28 February 2022;
Published: 21 March 2022.
Edited by:
Silvia Deaglio, University of Turin, ItalyReviewed by:
Luiz Eduardo Baggio Savio, Federal University of Rio de Janeiro, BrazilMichael Sitkovsky, Northeastern University, United States
Copyright © 2022 Sun, Wang and Hao. This is an open-access article distributed under the terms of the Creative Commons Attribution License (CC BY). The use, distribution or reproduction in other forums is permitted, provided the original author(s) and the copyright owner(s) are credited and that the original publication in this journal is cited, in accordance with accepted academic practice. No use, distribution or reproduction is permitted which does not comply with these terms.
*Correspondence: Bochu Wang, wangbc2000@126.com; Shilei Hao, shilei_hao@cqu.edu.cn