- 1The First Affiliated Hospital, Anhui University of Chinese Medicine, Hefei, China
- 2Key Laboratory of Xin'an Medicine of the Ministry of Education, Anhui University of Chinese Medicine, Hefei, China
- 3Anhui Province Key Laboratory of Modern Chinese Medicine Department of Internal Medicine Application Foundation Research and Development, Hefei, China
- 4Institute of Rheumatology, Anhui Academy of Chinese Medicine, Hefei, China
Ankylosing spondylitis (AS) is a type of arthritis that primarily affects the spine and involves disorders of the immune and skeletal systems. However, the exact pathogenesis of AS is not fully understood. Non-coding RNAs (ncRNAs), particularly, long non-coding RNAs (lncRNAs), circular RNAs (circRNAs), and micro RNAs (miRNAs) and their interactions have been shown to influence many biological processes such as inflammatory responses, osteogenic differentiation and apoptosis, pyroptosis, and proliferation. In addition, ncRNAs reflect the disease activity of AS. In this review, we discuss the regulatory roles of ncRNAs in AS cell functions (inflammatory responses, cellular osteogenic differentiation and apoptosis, pyroptosis, and proliferation) and their potential applications in AS diagnosis and treatment. Understanding the role of ncRNAs in the pathogenesis of AS will lay the foundation for exploring potential new therapeutic approaches for AS.
1 Introduction
Ankylosing spondylitis (AS) is a chronic autoimmune disease characterized by systemic inflammation and osteogenesis (1, 2), and is a highly disabling and destructive type of arthritis (3). AS affects 0.09%-0.3% of the global population (4) and occurs mostly in young people (5). It causes severe back pain and stiffness, which leads to decreased function and eventually spinal and pelvic fusion, imposing a significant burden on patients and the society (6, 7). In recent years, multiple factors contributing to the development of AS have been identified, including infections, environmental triggers, and genetic susceptibility, especially immune disorders (8–10).
Inflammation and stiffness are the primary manifestations of AS (11). Treatment with non-steroidal anti-inflammatory drugs (NSAIDs) and biologics, including tumor necrosis factor inhibitors (TNFi), interleukin-17 inhibitors (IL-17i), and more recently, Janus kinase inhibitors, has led to significant improvements in clinical symptoms and quality of life (12–14). In addition, recent studies have shown that biological agents have the potential to inhibit new bone formation in a sustained manner (11). However, for patients with a high disease activity or in whom the process of bone remodeling has already started, inhibition of inflammation will not be sufficient. Surgical intervention is inevitable to improve pain and joint motion (15). Despite recent advances in AS drug development, the functional outcome for many AS patients remains unsatisfactory. In particular, the development of new therapies is challenged by the fact that the pathogenesis of AS is still unclear (16). Therefore, new molecular targets must be identified.
Non-coding RNAs (ncRNAs) have recently attracted considerable attention caused by their critical role in biology (17). They are divided into housekeeping ncRNAs (such as transfer RNA (tRNA) and ribosomal RNA (rRNA)) and regulatory ncRNAs. Regulatory ncRNAs include micro RNAs (miRNAs), long non-coding RNAs (lncRNAs), and circular RNAs (circRNAs) (18). The competing endogenous RNA (ceRNA) hypothesis, proposed in 2011 based on experimental and theoretical studies, states that RNAs can communicate with each other and post-transcriptionally regulate gene expression by binding to miRNA binding sites (19). Different RNAs will form multiple regulatory relationships, which will eventually form a ceRNA network. Currently, studies are being conducted on the pathogenesis of ceRNA-related diseases, and various ncRNAs (e.g., lncRNAs, miRNAs, circRNAs) and ceRNA networks constructed from differentially expressed ncRNAs have been shown to be related to the pathogenesis of various autoimmune diseases (20–23), further illustrating the interconnection between different RNA molecules and the regulation of gene expression. The construction of ceRNA regulatory networks is of great importance to further understand the functions of ncRNAs. The interconnection and altered regulatory expression of different RNA molecules may also be involved in the pathogenesis of AS.
In this review, we focus on the roles and possible mechanisms of three ncRNAs (lncRNAs, miRNAs, and circRNAs) in AS (Table 1) and discuss the role of ceRNA networks associated with lncRNAs/circRNAs in the pathogenesis of AS (Figure 1). This will help in the diagnosis and treatment of AS.
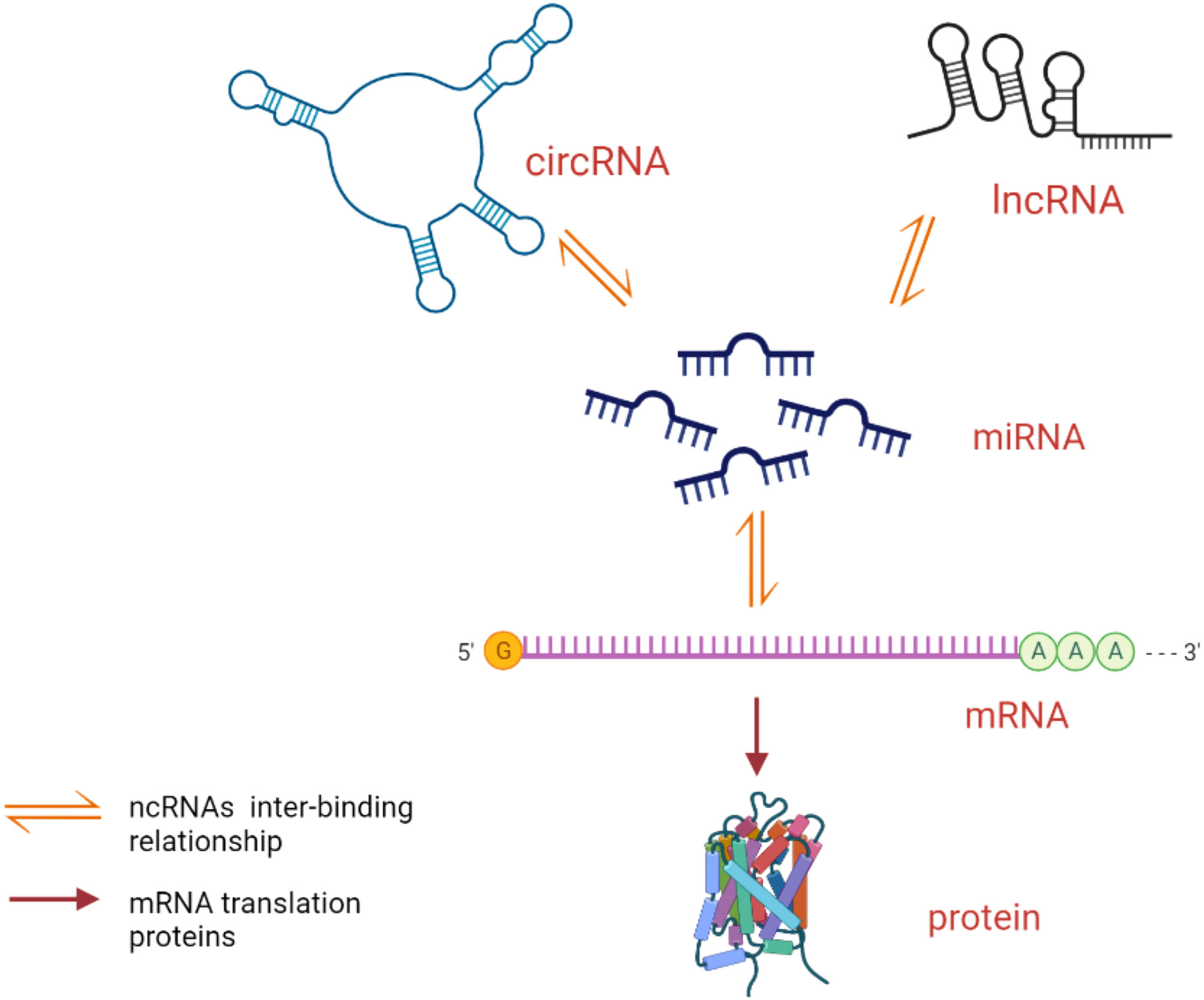
Figure 1 Mode of action of ceRNA network. lncRNAs/circRNAs competitively bind miRNAs to form ceRNAs to play the role of translating proteins.
2 Role of ncRNAs
ncRNAs are widely present in cells and are involved in many physiological and pathological processes by regulating gene expression, cell cycle, chromatin remodeling, and epigenetic modifications (41, 42). Although ncRNAs lack the ability to encode proteins, they not only play important physiological regulatory roles in various cellular activities, but their aberrant expression and regulation are also important links in the pathogenesis of many diseases. ncRNAs are usually expressed in response to external signals, during differentiation or at specific stages of development. Their differential expression can regulate the transcription or translation of other genes or can directly interfere with signaling pathways (43).
2.1 miRNAs
miRNAs are endogenous ncRNAs (approximately 22 nucleotides long) that are widely found in eukaryotic organisms. They can regulate gene expression by directly targeting the promoters of bound genes or by binding to the non-protein translation region (3’-UTR) of the target messenger RNA (mRNA) to induce degradation of the target mRNA or translational repression, thereby achieving transcriptional or post-transcriptional level regulation of genes (44).
2.2 lncRNAs
lncRNAs are a class of ncRNAs longer than 200 nucleotides; depending on their intracellular localization, lncRNAs can play different roles (45). Cytoplasmic lncRNAs mainly play ceRNA roles by regulating the degradation or translation of target mRNAs or competitively binding miRNAs to regulate gene expression at the post-transcriptional level; cytosolic lncRNAs mainly play regulatory roles by controlling the epigenetic state of specific genes, directly participating in transcriptional regulation and variable splicing or constituting nuclear structural domains (46, 47).
2.3 circRNAs
circRNAs are a class of covalently closed loops without a 5’ cap and 3’ poly A tail, unlike traditional linear RNAs. circRNAs are present in high abundance and have high diversity, spatio-temporal specific expression, and highly conserved sequences; in particular, the covalent closed-loop structure protects them from nucleic acid exonuclease shearing. They have higher stability than linear RNAs (48). The molecular biological functions of circRNAs in genetic or epigenetic regulation are becoming clear, including competitive binding of miRNA, regulation of transcription and variable splicing, and interaction with RNA-binding proteins (49).
3 Regulatory functions of ncRNAs in AS cells
3.1 AS cell inflammatory responses
Immune-mediated inflammatory response is a key aspect of AS, with the IL-23 and TNF pathways being the main effector pathways (50). The inflammatory response in AS is reflected by the dysregulation of inflammatory cytokines in blood and tissues in vivo. High expression of cytokines activates inflammatory signaling pathways, causing an inflammatory response in the body leading to AS-related joint pain, and ncRNAs may be involved in regulating the inflammatory response in AS cells.
Overexpression of miR-451 suppressed macrophage migration inhibitory factor (MIF) and levels of inflammatory cytokines (24). Yu et al. (32) found that LOC645166 expression was downregulated in T cells from AS patients, which upregulated NF-κB activation by reducing the recruitment of polyubiquitin chains that block the IKK complex to K63 linkage, making AS patients more sensitive to stimulation by pro-inflammatory cytokines or TLR ligands. Li et al. (33) showed that lncRNA maternally expressed gene 3 (MEG3) plays a partial anti-inflammatory role in AS by targeting miR-146a to regulate the expression of IL-1β, IL-6, and TNF-α. Fang et al. (34) showed that the expression level of hsa_circ_0003307 correlated with the inflammatory response in AS. hsa_circ_0003307 knockdown could reduce the inflammatory response in AS by regulating the PI3K/AKT pathway. Zhang et al. (36) showed that lncRNA H19 in peripheral blood mononuclear cells of AS could form ceRNA with miR22-5p/miR675-5p- vitamin D receptor (VDR) to regulate the IL-17A/IL-23 signaling pathway expression, which has an important role in the pathogenesis of AS. The results of another study showed that hsa_circ_0000652 in peripheral blood mononuclear cells (PBMCs) of AS patients promoted macrophage proliferation and cytokine production and inhibited apoptosis and may act as a pro-inflammatory factor for macrophages and a positive regulator of OX40/OX40L through sponge hsa-miR-1179 (38). Thus, ncRNAs may be involved in regulating the cellular immune inflammatory response in AS, but do not act in the same way.
3.2 Osteogenic differentiation of AS cells
The typical pathology of AS involves the progression of inflammation into ossification and ankylosis (51). Pathological osteogenesis can cause progressive ankylosis of the spine and peripheral joints, leading to motor impairment and even permanent loss of mobility, which severely affects patients’ life and work and is the main cause of disability in AS patients (52). Fibroblasts have the potential to differentiate into osteoblasts, as shown by the expression of osteogenic marker genes alkaline phosphatase (ALP) and osteocalcin (OC). However, their activation requires specific cytokine stimulation, the most important one being bone morphogenetic proteins (BMPs) (53). Several studies have shown that ncRNAs regulate the differentiation of fibroblastogenic cells into osteoblasts in AS hip capsule specimens (40, 54). Low expression of miR-150-5p and miR-204-5p in fibroblasts from AS patient ligaments inhibited osteogenesis via VDR and Notch2, respectively (25, 26), while high expression of miR-148a-3p exacerbated the osteogenic differentiation of fibroblasts by inhibiting the expression of the downstream target gene Dickkopf homologue 1 (DKK1) and activating the Wnt pathway, leading to increased calcified nodules and mineralization (27). A study of animal experiments showed that miR‐96 was expressed at a high level in proteoglycan-induced AS mice, and overexpression of miR‐96 led to stimulation of osteoblast differentiation and bone formation through activation of the Wnt pathway in AS, which may provide novel aspects for AS treatments in the future (28). A study of osteoblast-specific miR-214 knockout mice (CKO: Ocn-cre; miR-214fl/fl mice) showed that miR-214, the production of which is stimulated by IL-17A in osteoblasts, was an important inhibitor of bone formation in AS. Osteoblast-derived miR-214 stimulated by IL-17A can be transferred into osteoclasts to promote their activity and thus inhibit bone formation (29). Another study revealed that circ_0070562 was significantly upregulated in bone marrow mesenchymal stem cells (BMSCs) from AS patients, which may play an important role in AS-BMSC osteogenesis in combination with miR-424-5p and miR-133b of the TGF-beta pathway (35). Furthermore, in AS hip capsule specimens, circ_0018168 overexpression elevated DKK1 through adsorption of miR-330-3p and inhibited AS fibroblast proliferation and osteogenic differentiation; the results suggested that circ RNA-related ceRNA could play a regulatory role in the osteogenic differentiation of AS cells (39).
3.3 AS cell proliferation, apoptosis, and pyroptosis
Excessive proliferation and insufficient apoptosis of many kinds of cells, including T cells, fibroblast-like synoviocytes (FLS), and fibroblasts, lead to the pathogenesis of AS (30, 31, 40, 55). Pyroptosis is an inflammatory type of regulated cell death that occurs following inflammasome activation (56, 57). In AS patients, activated pyroptosis leads to inflammatory responses, which cause various inflammatory diseases (58, 59).
Li et al. (30) found that miR-130a-3p was downregulated in T cells from AS patients, and an miR-130a-3p inhibitor could inhibit T cell proliferation and induce apoptosis by upregulating the downstream target gene HOXB1. Similarly, miR-204 expression was decreased and Gasdermin D (GSDMD) was elevated in the FLS of AS patients. miR-204 mimics inhibited FLS pyroptosis in AS cells by suppressing the expression of GSDMD (31). In addition, ceRNAs are involved in cellular regulation in AS. Li et al. (40) showed that competitive binding of hsa_circ_0056558 and cyclin-dependent kinase 6 (CDK6) to miR-1290 inhibits cell proliferation and differentiation while promoting apoptosis, a process that may be mediated through the PI3K/AKT/NF-κB pathway. Another study showed that lncRNA MALAT1 and GSDMD expression was upregulated in AS chondrocytes, but that of miR-558 was downregulated. Downregulation of lncRNA MALAT1 expression increased miR-558 activity by suppressing GSDMD and inhibited inflammation, apoptosis, and pyroptosis in AS chondrocytes, thus acting as a potential suppressor of AS (37).
4 ncRNA applications in AS diagnosis and treatment
The stable and tissue-specific expression of ncRNAs makes them promising diagnostic markers for diseases such as AS; they could help in reflecting the activity of AS, monitoring the effect of treatment, and predicting the occurrence and recurrence rate of AS.
Recent studies have shown that a variety of ncRNAs may have potential diagnostic value and are closely related to AS disease activity. Tan et al. (60) found that miR-146a/miR-125a-5p/miR-125b-5p/miR-499a/miR-155a combination (area under the curve (AUC)=0.824, 95% confidence interval (CI) = 0.727-0.921) had high sensitivity and specificity for the diagnosis of AS. C-reactive protein (CRP) levels were positively correlated with miR-125a-5p (r= 0.438, p = 0.005) and miR-155a (r= 0.414, p = 0.006) expression, suggesting that miR-125a-5p and miR-155a may exacerbate AS-induced inflammation. Another study showed that lnc-ITSN1-2 expression was elevated in patients with AS, and lnc-ITSN1-2 was positively correlated with levels of CRP and interleukin (IL)-1β, Bass Ankylosing Spondylitis Disease Activity Index (BASDAI), and ankylosing spondylitis disease activity with c-reactive protein (ASDASCRP) score (61). Similarly, Tang et al. (62) found that hsa_circRNA_012732 was downregulated during inflammation, and negatively correlated with the BASDAI, high-sensitivity C-reactive protein (hs-CRP), and globulin (GLOB), and positively correlated with lymphocyte count (LY), mean red blood cell volume, and albumin (ALB). ROC curve analysis showed that hsa_circRNA_001544 (95% CI=0.610-0.831, P<0.05) was statistically significant, and its AUC values was 0.720. hsa_circRNA_001544 and hsa_circRNA_012732 have potential to be molecular markers for AS diagnosis and disease activity, respectively. High-throughput sequencing of PBMC samples from five AS patients and healthy controls was performed. NONHSAT118801.2, ENST00000444046, and NONHSAT183847.1 were found to be significantly upregulated in AS patient samples, and the expression of NONHSAT118801.2 and NONHSAT183847.1 was positively correlated with disease severity (63). All these findings suggest that ncRNAs may serve as diagnostic markers for AS.
With respect to therapy, the therapeutic potential of ncRNAs is gradually being revealed as translational medicine research continues to advance. miR-21 can induce the proliferation and differentiation of MSCs to promote bone formation. miR-21-exosome injection may help alleviate spinal osteoporosis in patients with AS, which is characterized by an increase in bone mineral content and bone density and a decrease in osteoclast number (64). However, current studies have mainly focused on animal experiments, and further clinical trials are needed to confirm this in the future.
ncRNAs may predict the occurrence and recurrence rate of AS. Han et al. (65) revealed that lncRNA-adjacent FOXA2 enhancer (lncRNA-NEF) expression was upregulated in synovial fluid samples from AS patients and was associated with ASDAS, BASDAI, erythrocyte sedimentation rate (ESR), and CRP levels (P<0.05). NSAID treatment significantly downregulated lncRNA-NEF expression. Three-year follow-up showed a high relapse rate in patients with high lncRNA-NEF levels (hazard ratio=2.266). These results suggest that lncRNA-NEF upregulation predicts relapse and poor treatment outcome in AS and has great potential as a predictive biomarker for AS relapse. Similarly, Zhong et al. (66) found that LINC00311 was upregulated in AS patients, which positively correlated with disease activity. At 2-year follow-up, patients with high LINC00311 levels had significantly higher rehospitalization rates. Thus, LINC00311 is overexpressed in AS and predicts treatment outcome and recurrence. These results suggest that ncRNA may have an impact on the prognosis of AS.
5 Conclusions and future perspectives
With the continuous development of molecular biology techniques, our understanding of disease mechanisms has gradually advanced to include genetic and epigenetic processes. As one of the key research areas in epigenetics, ncRNA plays an important regulatory role in diseases including cancers, cardiovascular diseases, and neurological pathologies. ncRNA is an important part of rheumatic disease research to study the pathogenesis and identify new targets for the diagnosis and treatment. Recently, ncRNAs have been studied in association with inflammatory response in AS and bone formation (Figure 2); key molecules regulating the developmental process of AS will be identified in the future, providing new entry points for the study of AS etiology and molecular targeting therapy. However, exploring the specific mechanisms of ncRNAs in inflammation and bone formation in AS is challenging due to the wide variety of ncRNAs. Currently, it is difficult to determine which ncRNAs can be targeted for the most effective intervention. In addition, the specific mechanism to intervene in inflammatory responses and bone formation targets via ncRNAs is still unclear and needs further investigation.
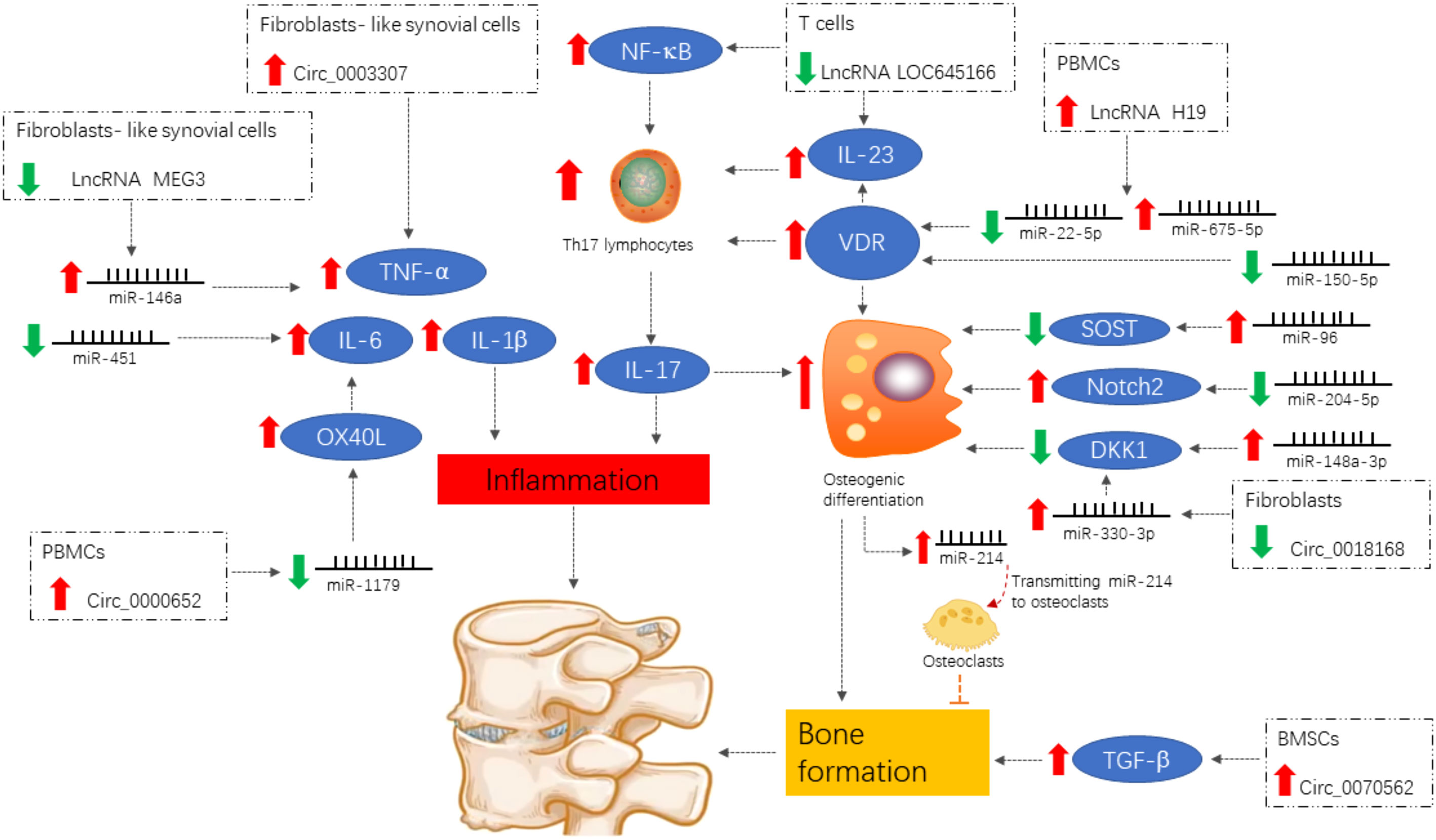
Figure 2 Differentially expressed ncRNAs of functional importance in ankylosing spondylitis. Signals are focused on the induction of the pro-inflammatory cytokines IL-1β, TNF-α, and IL-6 and the enhancement of the IL-23/IL-17 axis, both of which contribute to inflammation and abnormal bone formation.
Author contributions
YF conceptualized and designed the study. JL supervised the project and contributed to manuscript revision. Both authors contributed to the article and approved the submitted version.
Funding
This work was supported by grants from the National Nature Fund Program (No. 82104817); the Key Laboratory of Xin’an Medicine of the Ministry of Education, Anhui University of Chinese Medicine (2020xayx08); the 2021 open fund of Anhui Key Laboratory of Applied Basic and Development Research of Modern Internal Medicine of Traditional Chinese Medicine (2021AKLMCM003); Scientific Research Project of Anhui Provincial Health Commission (AHWJ2021b036); Anhui Famous Traditional Chinese Medicine Liu Jian Studio Construction Project (Traditional Chinese Medicine Development Secret (2018) No. 11); Anhui Provincial Laboratory of Applied Basic and Development Research of Modern Internal Medicine of Traditional Chinese Medicine (2016080503B041); Anhui Province Traditional Chinese Medicine Leading Talent Project (Traditional Chinese Medicine Development Secret (2018) No. 23); and the 12th batch of “115” Innovation team of Anhui Province (Anhui Talent Office (2019) No. 1).
Conflict of interest
The authors declare that the research was conducted in the absence of any commercial or financial relationships that could be construed as a potential conflict of interest.
Publisher’s note
All claims expressed in this article are solely those of the authors and do not necessarily represent those of their affiliated organizations, or those of the publisher, the editors and the reviewers. Any product that may be evaluated in this article, or claim that may be made by its manufacturer, is not guaranteed or endorsed by the publisher.
References
1. Mauro D, Thomas R, Guggino G, Lories R, Brown MA, Ciccia F. Ankylosing spondylitis: An autoimmune or autoinflammatory disease? Nat Rev Rheumatol (2021) 17(7):387–404. doi: 10.1038/s41584-021-00625-y
2. Xie Z, Yu W, Zheng G, Li J, Cen S, Ye G, et al. TNF-α-mediated m6A modification of ELMO1 triggers directional migration of mesenchymal stem cell in ankylosing spondylitis. Nat Commun (2021) 12(1):5373. doi: 10.1038/s41467-021-25710-4
3. Fang Y, Liu J, Xin L, Wen J, Guo J, Huang D, et al. Exploration of the immuno-inflammatory potential targets of xinfeng capsule in patients with ankylosing spondylitis based on data mining, network pharmacology, and molecular docking. Evid Based Complement Alternat Med (2022) 2022:5382607. doi: 10.1155/2022/5382607
4. Zhang Q, Jin D, Mou X, Ye H. PBMC CDC42 reveals the disease activity and treatment efficacy of TNF inhibitor in patients with ankylosing spondylitis. J Clin Lab Anal (2022) 36(3):e24267. doi: 10.1002/jcla.24267
5. Han Q, Zheng Z, Zhang K, Ding J, Baraliakos K, Zhu P, et al. A comprehensive assessment of hip damage in ankylosing spondylitis, especially early features. Front Immunol (2021) 12:668969. doi: 10.3389/fimmu.2021.668969
6. Garrido-Mesa J, Brown MA. T Cell repertoire profiling and the mechanism by which HLA-B27 causes ankylosing spondylitis. Curr Rheumatol Rep (2022) 24(12):398–410. doi: 10.1007/s11926-022-01090-6
7. Ben-Shabat N, Shabat A, Watad A, Kridin K, Bragazzi NL, McGonagle D, et al. Mortality in ankylosing spondylitis according to treatment: A nationwide retrospective cohort study of 5,900 patients from Israel. Arthritis Care Res (Hoboken) (2022) 74(10):1614–22. doi: 10.1002/acr.24616
8. Sternes PR, Brett L, Phipps J, Ciccia F, Kenna T, de Guzman E, et al. Distinctive gut microbiomes of ankylosing spondylitis and inflammatory bowel disease patients suggest differing roles in pathogenesis and correlate with disease activity. Arthritis Res Ther (2022) 24(1):163. doi: 10.1186/s13075-022-02853-3
9. Kenyon M, Maguire S, Rueda Pujol A, O'Shea F, McManus R. The genetic backbone of ankylosing spondylitis: How knowledge of genetic susceptibility informs our understanding and management of disease. Rheumatol Int (2022) 42(12):2085–95. doi: 10.1007/s00296-022-05174-5
10. Casadó-Llombart S, Velasco-de Andrés M, Català C, Leyton-Pereira A, Gutiérrez-Cózar R, Suárez B, et al. Experimental and genetic evidence for the impact of CD5 and CD6 expression and variation in inflammatory bowel disease. Front Immunol (2022) 13:966184. doi: 10.3389/fimmu.2022.966184
11. Kusuda M, Haroon N, Nakamura A. Complexity of enthesitis and new bone formation in ankylosing spondylitis: Current understanding of the immunopathology and therapeutic approaches. Mod Rheumatol (2022) 32(3):484–92. doi: 10.1093/mr/roab057
12. Deodhar A, Kruzikas D, Zhou L, Biljan A, Saffore CD. Geographic variations in diagnosis and treatment of ankylosing spondylitis in the united states: A real-world study. Rheumatol Ther (2022) 9(2):447–63. doi: 10.1007/s40744-021-00406-9
13. Tam HKJ, Robinson PC, Nash P. Inhibiting IL-17A and IL-17F in rheumatic disease: Therapeutics help to elucidate disease mechanisms. Curr Rheumatol Rep (2022) 24(10):310–20. doi: 10.1007/s11926-022-01084-4
14. Lee YH. Comparative efficacy and safety of janus kinase inhibitors and secukinumab in patients with active ankylosing spondylitis: A systematic review and meta-analysis. Pharmacology (2022) 107(11-12):537–44. doi: 10.1159/000525627
15. Liu C, Hu F, Hu W, Zhang Z, Zheng G, Song K, et al. Clinical results of utilizing the satellite rod technique in treating ankylosing spondylitis kyphosis. Orthop Surg (2022) 14(9):2180–7. doi: 10.1111/os.13427
16. Rong T, Jia N, Wu B, Sang D, Liu B. New insights into the regulatory role of ferroptosis in ankylosing spondylitis via consensus clustering of ferroptosis-related genes and weighted gene Co-expression network analysis. Genes (Basel) (2022) 13(8):1373. doi: 10.3390/genes13081373
17. Zhuo L, Song B, Liu Y, Li Z, Fu X. Predicting ncRNA-protein interactions based on dual graph convolutional network and pairwise learning. Brief Bioinform (2022) 23(5):bbac339. doi: 10.1093/bib/bbac339
18. Gao L, Jiang Z, Han Y, Li Y, Yang X. Regulation of pyroptosis by ncRNA: A novel research direction. Front Cell Dev Biol (2022) 10:840576. doi: 10.3389/fcell.2022.840576
19. Salmena L, Poliseno L, Tay Y, Kats L, Pandolfi PP. A ceRNA hypothesis: the Rosetta stone of a hidden RNA language? Cell (2011) 146(3):353–8. doi: 10.1016/j.cell.2011.07.014
20. Huang Z, Kuang N. Construction of a ceRNA network related to rheumatoid arthritis. Genes (Basel) (2022) 13(4):647. doi: 10.3390/genes13040647
21. Hao Y, He M, Fu Y, Zhao C, Xiong S, Xu X. Identification of novel key genes and pathways in multiple sclerosis based on weighted gene coexpression network analysis and long noncoding RNA-associated competing endogenous RNA network. Oxid Med Cell Longev (2022) 2022:9328160. doi: 10.1155/2022/9328160
22. Song W, Qiu J, Yin L, Hong X, Dai W, Tang D, et al. Integrated analysis of competing endogenous RNA networks in peripheral blood mononuclear cells of systemic lupus erythematosus. J Transl Med (2021) 19(1):362. doi: 10.1186/s12967-021-03033-8
23. Chen X, Cheng Q, Du Y, Liu L, Wu H. Differential long non-coding RNA expression profile and function analysis in primary sjogren's syndrome. BMC Immunol (2021) 22(1):47. doi: 10.1186/s12865-021-00439-3
24. Park MC, Kwon OC, Lee SW, Song JJ, Park YB. MiR-451 suppresses inflammatory responses in ankylosing spondylitis by targeting macrophage migration inhibitory factor. Clin Exp Rheumatol (2020) 38(2):275–81. doi: 10.55563/clinexprheumatol/2l5emt
25. Li Y, Qi W, Shi Y. miR-150-5p inhibits osteogenic differentiation of fibroblasts in ankylosing spondylitis by targeting VDR. Exp Ther Med (2022) 23(4):283. doi: 10.3892/etm.2022.11213
26. Zhao J, Zhang Y, Liu B. MicroRNA−204−5p inhibits the osteogenic differentiation of ankylosing spondylitis fibroblasts by regulating the Notch2 signaling pathway. Mol Med Rep (2020) 22(3):2537–44. doi: 10.3892/mmr.2020.11303
27. Sheng W, Jiang H, Yuan H, Li S. miR−148a−3p facilitates osteogenic differentiation of fibroblasts in ankylosing spondylitis by activating the wnt pathway and targeting DKK1. Exp Ther Med (2022) 23(5):365. doi: 10.3892/etm.2022.11292
28. Ma S, Wang DD, Ma CY, Zhang YD. microRNA-96 promotes osteoblast differentiation and bone formation in ankylosing spondylitis mice through activating the wnt signaling pathway by binding to SOST. J Cell Biochem (2019) 120(9):15429–42. doi: 10.1002/jcb.28810
29. Liu Z, Huang F, Luo G, Wang Y, Du R, Sun W, et al. miR-214 stimulated by IL-17A regulates bone loss in patients with ankylosing spondylitis. Rheumatol (Oxford) (2020) 59(5):1159–69. doi: 10.1093/rheumatology/kez594
30. Li F, Si D, Guo X, Guo N, Li D, Zhang L, et al. Aberrant expression of miR−130a−3p in ankylosing spondylitis and its role in regulating t−cell survival. Mol Med Rep (2019) 20(4):3388–94. doi: 10.3892/mmr.2019.10573
31. Gu G, Huo Y, Xu G, Li L, Yu J, Sheng L, et al. MicroRNA-204-GSDMD interaction regulates pyroptosis of fibroblast-like synoviocytes in ankylosing spondylitis. Int Immunopharmacol (2021) 91:107227. doi: 10.1016/j.intimp.2020.107227
32. Yu HC, Huang KY, Lu MC, Huang Tseng HY, Liu SQ, Lai NS, et al. Down-regulation of LOC645166 in T cells of ankylosing spondylitis patients promotes the NF-κB signaling via decreasingly blocking recruitment of the IKK complex to K63-linked polyubiquitin chains. Front Immunol (2021) 12:591706. doi: 10.3389/fimmu.2021.591706
33. Li Y, Zhang S, Zhang C, Wang M. LncRNA MEG3 inhibits the inflammatory response of ankylosing spondylitis by targeting miR-146a. Mol Cell Biochem (2020) 466(1-2):17–24. doi: 10.1007/s11010-019-03681-x
34. Fang Y, Liu J, Long Y, Wen J, Huang D, Xin L. Knockdown of circular RNA hsa_circ_0003307 inhibits synovial inflammation in ankylosing spondylitis by regulating the PI3K/AKT pathway. Adv Clin Exp Med (2022) 31(7):781–8. doi: 10.17219/acem/146830
35. Wang S, Chen F, Zeng C, Gu H, Wang Z, Yu W, et al. RNA Sequencing reveals the expression profiles of circRNAs and indicates Hsa_circ_0070562 as a pro-osteogenic factor in bone marrow-derived mesenchymal stem cells of patients with ankylosing spondylitis. Front Genet (2022) 13:947120. doi: 10.3389/fgene.2022.947120
36. Zhang X, Ji S, Cai G, Pan Z, Han R, Yuan Y, et al. H19 increases IL-17A/IL-23 releases via regulating VDR by interacting with miR675-5p/miR22-5p in ankylosing spondylitis. Mol Ther Nucleic Acids (2020) 19:393–404. doi: 10.1016/j.omtn.2019.11.025
37. Chen W, Wang F, Wang J, Chen F, Chen T. The molecular mechanism of long non-coding RNA MALAT1-mediated regulation of chondrocyte pyroptosis in ankylosing spondylitis. Mol Cells (2022) 45(6):365–75. doi: 10.14348/molcells.2022.2081
38. Song M, Gao J, Yan T, Bi E, An T, Wang X, et al. Hsa_circ_0000652 aggravates inflammation by activation of macrophages and enhancement of OX40/OX40L interaction in ankylosing spondylitis. Front Cell Dev Biol (2021) 9:737599. doi: 10.3389/fcell.2021.737599
39. Zhao L, Jiao J, Yan G, Wei W, Fang G, Yu T. Circ_0018168 inhibits the proliferation and osteogenic differentiation of fibroblasts in ankylosing spondylitis via regulating miR-330-3p/DKK1 axis. Regener Ther (2022) 21:175–84. doi: 10.1016/j.reth.2022.06.005
40. Li X, Zhou W, Li Z, Guan F. Hsa_circ_0056558 regulates cyclin-dependent kinase 6 by sponging microRNA-1290 to suppress the proliferation and differentiation in ankylosing spondylitis. Autoimmunity (2021) 54(2):114–28. doi: 10.1080/08916934.2021.1894417
41. Cech TR, Steitz JA. The noncoding RNA revolution-trashing old rules to forge new ones. Cell (2014) 157(1):77–94. doi: 10.1016/j.cell.2014.03.008
42. Gong C, Maquat LE. lncRNAs transactivate STAU1-mediated mRNA decay by duplexing with 3' UTRs via alu elements. Nature (2011) 470(7333):284–8. doi: 10.1038/nature09701
43. Della Bella E, Koch J, Baerenfaller K. Translation and emerging functions of non-coding RNAs in inflammation and immunity. Allergy (2022) 77(7):2025–37. doi: 10.1111/all.15234
44. Rani V, Sengar RS. Biogenesis and mechanisms of microRNA-mediated gene regulation. Biotechnol Bioeng (2022) 119(3):685–92. doi: 10.1002/bit.28029
45. Chen LL. Towards higher-resolution and in vivo understanding of lncRNA biogenesis and function. Nat Methods (2022) 19(10):1152–5. doi: 10.1038/s41592-022-01626-9
46. Nojima T, Proudfoot NJ. Mechanisms of lncRNA biogenesis as revealed by nascent transcriptomics. Nat Rev Mol Cell Biol (2022) 23(6):389–406. doi: 10.1038/s41580-021-00447-6
47. Zhang P, Wu S, He Y, Li X, Zhu Y, Lin X, et al. LncRNA-mediated adipogenesis in different adipocytes. Int J Mol Sci (2022) 23(13):7488. doi: 10.3390/ijms23137488
48. Liang L, Zhu Y, Li J, Zeng J, Wu L. ALKBH5-mediated m6A modification of circCCDC134 facilitates cervical cancer metastasis by enhancing HIF1A transcription. J Exp Clin Cancer Res (2022) 41(1):261. doi: 10.1186/s13046-022-02462-7
49. Liu CX, Chen LL. Circular RNAs: Characterization, cellular roles, and applications. Cell (2022) 185(12):2016–34. doi: 10.1016/j.cell.2022.04.021
50. Brown MA, Kenna T, Wordsworth BP. Genetics of ankylosing spondylitis–insights into pathogenesis. Nat Rev Rheumatol (2016) 12(2):81–91. doi: 10.1038/nrrheum.2015.133
51. Zeng Y, He R, Liu Y, Luo T, Li Q, He Y, et al. HDAC1 regulates inflammation and osteogenic differentiation of ankylosing spondylitis fibroblasts through the wnt-smad signaling pathway. J Orthop Surg Res (2022) 17(1):343. doi: 10.1186/s13018-022-03224-z
52. Cui H, Li Z, Chen S, Li X, Chen D, Wang J, et al. CXCL12/CXCR4-Rac1-mediated migration of osteogenic precursor cells contributes to pathological new bone formation in ankylosing spondylitis. Sci Adv (2022) 8(14):eabl8054. doi: 10.1126/sciadv.abl8054
53. Jiang N, Liu HX, Liang HY, Feng XH, Liu BY, Zhou YY. Osteogenic differentiation characteristics of hip joint capsule fibroblasts obtained from patients with ankylosing spondylitis. Ann Transl Med (2021) 9(4):331. doi: 10.21037/atm-20-7817
54. Ding L, Yin Y, Hou Y, Jiang H, Zhang J, Dai Z, et al. microRNA-214-3p suppresses ankylosing spondylitis fibroblast osteogenesis via BMP-TGFβ axis and BMP2. Front Endocrinol (Lausanne) (2021) 11:609753. doi: 10.3389/fendo.2020.609753
55. Ma C, Wen B, Zhang Q, Shao PP, Gu W, Qu K, et al. Emodin induces apoptosis and autophagy of fibroblasts obtained from patient with ankylosing spondylitis. Drug Des Devel Ther (2019) 13:601–9. doi: 10.2147/DDDT.S182087
56. McKenzie BA, Dixit VM, Power C. Fiery cell death: Pyroptosis in the central nervous system. Trends Neurosci (2020) 43(1):55–73. doi: 10.1016/j.tins.2019.11.005
57. Zou YC, Yang XW, Yuan SG, Zhang P, Ye YL, Li YK. Downregulation of dickkopf-1 enhances the proliferation and osteogenic potential of fibroblasts isolated from ankylosing spondylitis patients via the wnt/β-catenin signaling pathway in vitro. Connect Tissue Res (2016) 57(3):200–11. doi: 10.3109/03008207.2015.1127916
58. Spel L, Martinon F. Inflammasomes contributing to inflammation in arthritis. Immunol Rev (2020) 294(1):48–62. doi: 10.1111/imr.12839
59. Kong R, Sun L, Li H, Wang D. The role of NLRP3 inflammasome in the pathogenesis of rheumatic disease. Autoimmunity (2022) 55(1):1–7. doi: 10.1080/08916934.2021.1995860
60. Tan H, Ren R, Zhang J, Huang Z, Niu Q, Yang B. Analysis of inflammation-related microRNA expression in patients with ankylosing spondylitis. Immunol Res (2022) 70(1):23–32. doi: 10.1007/s12026-021-09249-6
61. Li M, Zhou X. Long noncoding RNA intersectin 1-2 gradually declines during adalimumab treatment, and its reduction correlates with treatment efficacy in patients with ankylosing spondylitis. Inflammopharmacology (2021) 29(5):1371–8. doi: 10.1007/s10787-021-00854-3
62. Tang YP, Zhang QB, Dai F, Liao X, Dong ZR, Yi T, et al. Circular RNAs in peripheral blood mononuclear cells from ankylosing spondylitis. Chin Med J (Engl) (2021) 134(21):2573–82. doi: 10.1097/CM9.0000000000001815
63. Huang D, Liu J, Wan L, Fang Y, Long Y, Zhang Y, et al. Identification of lncRNAs associated with the pathogenesis of ankylosing spondylitis. BMC Musculoskelet Disord (2021) 22(1):272. doi: 10.1186/s12891-021-04119-6
64. Hu L, Guan Z, Tang C, Li G, Wen J. Exosomes derived from microRNA-21 overexpressed adipose tissue-derived mesenchymal stem cells alleviate spine osteoporosis in ankylosing spondylitis mice. J Tissue Eng Regener Med (2022) 16(7):634–42. doi: 10.1002/term.3304
65. Han D, Ouyang G, Pan P, Yuan Y. Upregulated lncRNA-NEF predicts recurrence and poor treatment outcomes of ankylosing spondylitis. Immun Inflammation Dis (2022) 10(8):e627. doi: 10.1002/iid3.627
Keywords: lncRNAs, circRNAs, miRNAs, ceRNA, ankylosing spondylitis
Citation: Fang Y and Liu J (2023) Novel regulatory role of non-coding RNAs in ankylosing spondylitis. Front. Immunol. 14:1131355. doi: 10.3389/fimmu.2023.1131355
Received: 24 December 2022; Accepted: 14 February 2023;
Published: 24 February 2023.
Edited by:
Mark Hwang, University of Texas Health Science Center at Houston, United StatesReviewed by:
Esraah Alharris, University of Al-Qadisiyah, IraqCopyright © 2023 Fang and Liu. This is an open-access article distributed under the terms of the Creative Commons Attribution License (CC BY). The use, distribution or reproduction in other forums is permitted, provided the original author(s) and the copyright owner(s) are credited and that the original publication in this journal is cited, in accordance with accepted academic practice. No use, distribution or reproduction is permitted which does not comply with these terms.
*Correspondence: Jian Liu, liujianahzy@126.com