High Performance Copper Based Metal Organic Framework for Removal of Heavy Metals From Wastewater
- 1Department of Chemistry, College of Natural and Computational Science, Energy and Environment Research Center, Dilla University, Dilla, Ethiopia
- 2Department of Chemistry, College of Science, Bahir Dar University, Bahir Dar, Ethiopia
The biggest challenge of this century is the generation of wastewater which is released to the environment due to industrial expansion. Industrial development has caused the release of various pollutants including heavy metals such as Cd, Pb, and Cr into the environment. In this study, copper diphenylamine metal-organic framework (Cu-DPA MOF) has been synthesized via hydrothermal method and its adsorption capacity toward the removal of heavy metals from wastewater was examined. The removal efficiency of heavy metals by Cu-DPA MOF was tested at optimized adsorption parameters such as optimal adsorbent dosage, pH, initial metals concentration, and adsorption time. The heavy metals concentration in the wastewater before treated with the as-synthesized MOF was determined to be 0.3027, 0.0098, and 0.1021 mg/L for Cr, Cd, and Pb, respectively. The corresponding concentrations of heavy metals in wastewater were reduced to 0.0015, 0.00024, and 0.00016 mg/L when treated with the as-synthesized MOF. As a result, a maximum removal efficiency of 97.6%, 99.5%, and 99.5% was achieved for Cd, Cr, and Pb metals, respectively. This is possibly due to the high porous nature and huge surface area of the as-synthesized MOF. The adsorption data were best fitted with Freundlich isotherm throughout this study. The study sheds light on the design of adsorbents with high removal efficiency of pollutants found in the environment.
Introduction
Heavy metals contamination in water is a major environmental concern. Heavy metals from heavy industry, and agricultural and other human activities could enter the water body and cause danger to the environment and human health. The release of toxic chemicals from industrial wastewater degrades water quality and is hazardous for human beings and other living organisms. Heavy metals such as lead (Pb), cadmium (Cd), chromium (Cr), arsenic (As), copper (Cu), mercury (Hg), and nickel (Ni) are commonly found in the discharged wastewater from industries. The toxicity of heavy metals leads to obstructive lung disease, reduced growth and development, causes cancer, organ damage, nervous system damage, and death in extreme cases (Aksu, 2005; Ahluwalia and Goyal, 2007; WHO, 2011; Madadrang et al., 2012; Tesfaye, 2016). Heavy metals are classified as hazardous pollutants due to their severe toxicity. The presence of these harmful heavy metal elements, even at low concentrations, affects the health of humans and other living organisms (Bakhtiari and Azizian, 2015; Cui et al., 2015).
The development of the industrial sector in developing countries is the primary cause of heavy metals being released into water, air, and soil in all countries across the world (Majeed et al., 2014). Most industries, e.g., electroplating, chemical and petrochemical, metal and mining, leather, textile, ceramic, cement, fertilizer, tanneries, batteries, paper, and pesticides, release their wastewater containing various harmful heavy metals without treatment into the environment. Toxic heavy metals such as cadmium, lead, and chromium do not break down in the environment, contributing to their bioaccumulation in the kidneys and livers of vertebrates and invertebrates. These toxic heavy metals cause cancers of the brain, kidney, bladder, colon, and rectum. The maximum permitted value for cadmium, chromium, and lead in water is 0.003 mg/L, 0.05 mg/L, and 0.01 mg/L, respectively (WHO 2011).
Nowadays, the growth of industrial development for one country is a backbone for the economy, but side by side has an effect, mainly releasing wastewater that contains heavy metal ions (Sharma and Bhattacharya 2017) and other pollutants (Mahmoodi et al., 2014; Mahmoodi and Saffar-Dastgerdi 2019; Hoseinzadeh et al., 2021). Proper wastewater management should be applied by different wastewater management policies to prevent harm to the environment and to human health. The industrial sectors comply with environmental regulations to treat their wastewater.
Thus far, various treatment technologies including precipitation, ion exchange, membrane filtration, reversible osmosis, ultrafiltration, coagulation-flocculation, and flotation have been widely used to remove heavy metals from wastewater. However, these methods are time-consuming and expensive (Qasem et al., 2021). On the other hand, adsorption or sorbent-based treatment strategies have been found to be promising and effective for the treatment of heavy metals contamination (Rajasulochana and Preethy, 2016). It is reported that zeolites, commercial activated carbon and biomass derived adsorbent such as saw dust, orange peel, rice husk, tea and coffee waste, peanut shells, dry tree leaves and barks, papaya seed, egg shell, and coconut leaf powder, have been used as adsorbent for the removal of heavy metals from various wastewater sources (Nguyen et al., 2013; Hussain and sheriff, 2014; Jayaweera et al., 2018) However, most of the existing adsorbents are limited by some factors such as requirement of higher calcination temperature, longer time of reaction, relatively low surface area and porosity, low stability, reusability, and others. Therefore, it is critically important to develop low cost adsorbent with high surface area and porosity using simple and scalable synthesis strategies.
Recently, metal-organic frameworks (MOFs), a class of porous material with inherent large surface areas, uniform but tunable cavities, tailorable chemistry and useful physical and chemical properties, have obtained broad and comprehensive applications than activated carbon and zeolites (Dehghankar et al., 2021). Although MOFs have been explored for a wide range of applications in catalysis, gas storage, chemical sensing, and drug delivery (Zhuang et al., 2014; Yang and Xu, 2017; Xie et at., 2018), there have been only a few reports on using MOFs for removal of heavy metals from wastewater (Efome et al., 2018; Asghar et al., 2021). Among all the reported MOFs, Cu-based MOFs are attractive because the high stability and earth-abundance of the Cu element makes them ideal sacrificial templates to prepare adsorbent with high surface area and porosity. Moreover, copper can preferably form many geometric complexes with a number of ligands (Burg and Meyerstein, 2012). To the best of our knowledge, no study has been conducted toward the removal of heavy metal from wastewater using the as-synthesized copper diphenylamine metal-organic framework.
Herein, we have successfully synthesized a highly porous nature Cu-DPA MOF via the hydrothermal method from copper nitrate trihydrate and diphenylamine linker. The use of as-synthesized MOF was investigated for the removal of heavy metals from wastewater. The adsorbents reported before for removal of heavy metals are time consuming, expensive, and are not stable. In this study, we reported highly porous copper-based MOF (Cupper-diphenylamine combination) for the first time for removal of Pb, Cd, and Cr. The linker (i.e., diphenylamine) used in this study was not reported before and is by far much cheaper than other linkers. The percentage removal by the synthesized MOF is much higher (97.5%, 99.5%, and 99.5% toward Cr, Cd, and Pb metals, respectively, from wastewater sample) when compared with the literature. This indicates the adsorbent in this study reveals very good potential for simultaneous removal of toxic metals from high-volume contaminated wastewaters. Therefore, the technology reported in this study can also be used as an adsorbent instead of more expensive and ineffective materials.
Experimental Section
Chemicals and Reagents
All the reagents were commercially available and were used as received without further purification. Copper nitrate (Cu(NO3)2·3H2O, 98%), diphenylamine (99%), N, N-Dimethyl formamide (DMF, 99%), methanol (CH3OH, 99.5%), and ethanol (EtOH) were purchased from Shanghai Chemical Reagent Co. Ltd.
Instruments and Apparatus
Wastewater samples were digested on a hot plate heating apparatus using round bottom flasks. Borosilicate volumetric flasks (25, 50, and 100 ml), pipettes (Pyrex, United States), measuring cylinders (Duran, Germany), and micropipettes (Dragonmed, 1–10 μl, 100–1,000 μl, Shanghai, China) were used for measuring different quantities of sample solution, acid reagents, and standard metal solutions. Graphite furnace atomic absorption spectroscopy (GFAAS) equipped with a deuterium background corrector was employed to quantify the heavy metals before and after absorption; iodine number analysis, X-ray diffraction (XRD), and Fourier transform infrared radiation (FTIR) were used to characterize the adsorbent.
Wastewater Sampling and Study Area Description
A wastewater sample was collected from Gamo Gofa zone, Arba Minch town, from the car wash labajo area. The sampling area is defined geographically as 6°01′–7°12′ N, and 37°32′–38° 02′ E. Composite samples were collected in 2-a-week intervals during March–April 2017 and analyzed for Pb, Cd, and Cr levels. The concentrations of these heavy metals were determined using GFAAS before and after treatment.
Digestion of the Wastewater Sample
Wastewater samples were digested with HNO3 and HClO4 in the ratio of 5:3. Digestion was done on a hot plate at a temperature of 93 °C for 1 h following a method reported by Fanta et al. (2019).
Instrument Calibration and Method Detection Limit
To determine the concentrations of metals in the untreated and treated effluent sample solutions, calibration curves were created. The GFAAS instrument was calibrated using four series of working standards (0.1, 0.5, 1.0, 1.5 mg/L). A correlation coefficient of 0.999 and above was obtained for the three metals. The detection limits for the metals tested in this study were calculated by multiplying the weighted average of standard deviations of the reagent blank by three. The wavelengths at which analysis was done, the correlation coefficients of the calibration curve for each of the metals, and the method detection limits of each metal are given in Table 1. The results clearly show that the calibration curves with good correlation coefficients and lower method detection limits were obtained during the analysis.
Method Validation
The validity of the above-optimized digestion procedure toward analyzing the wastewater samples concerning each of the selected metals (Pb, Cr, and Cd) could be made possible via conducting spiking experiments. Accordingly, the efficiency of the optimized procedure was checked by adding fixed volumes of standard solutions containing 5 ml of 0.5 mg/L of each metal into 50 ml wastewater. The spiked samples were then digested in the same manner as the original sample. Then the digests were diluted to 25 ml with deionized water. Finally, the solutions were analyzed for metal concentration with GFAAS. As used for the original samples, triplicate spiked samples were prepared, and the readings were recorded, and recovery was calculated using Eq. 1.
where
Synthesis of Copper Based Metal Organic Framework
Copper metal-organic framework (Cu-DPA MOF) was prepared from Cu(NO3)2·3H2O and diphenylamine ligand following a solution-based method. Briefly, 1 g of diphenylamine was dissolved in 5 ml of ethanol, and 1 g of copper nitrate trihydrate was dissolved in 5 ml of distilled water. The two solutions were mixed and stirred by a magnetic stirrer on a hot plate for 1 h until a homogeneous mixture was observed. The mixture was then kept for 3 days until a blue precipitate was formed. The resulting precipitates were isolated by centrifugation, washed four times with de-ionized water, DMF, and ethanol, respectively, and dried at 40°C for 24 h. The obtained precipitate, named Cu-DPA MOF afterward, was kept for further characterization and experimentation.
Optimization of Metal to Ligand Ratio
The metal to ligand ratio of the copper metal-organic framework was optimized by taking a different metal to ligand ratio (1:1, 2:1, 3:1, and 4:1). The synthesized MOFs were then subjected to an optical microscope, and the iodine number was determined following the American Society for Testing and Materials methods. The one with the smallest particle size and largest iodine number value was taken as the optimum ratio.
Characterization
The prepared Cu-DPA MOF was characterized by XRD, FTIR, GFAAS, and iodine number analysis. XRD measurements were taken on a D2 phaser XRD-300 W using Cu K
Batch Adsorption Experiment
Batch adsorption experiments were carried out to determine the effect of the adsorbent dose, contact time, pH, initial metal ion concentration, and time of mixing to reach equilibrium for Pb, Cd, and Cr adsorbed by Cu-DPA MOF. About 0.5–3.5 g of adsorbent (as synthesized Cu-DPA MOF) was added into a synthetic solution of each metal and stirred for 4 h, and then kept for 24 h until equilibrium could be achieved and filtered. The experiment was conducted three times. The optimum mass of the adsorbent was obtained by plotting the percentage removal vs the mass of the adsorbent. The effect of contact time in the removal of Pb, Cd, and Cr from wastewater using Cu-DPA MOF is studied in a contact time range of 20–120 min. Removal of metal ions was also carried at a different initial concentration of metals (5–80 mg/L) and pH (2–10). Following the optimized procedure, 1.5 g of Cu-DPA MOF was added to 50 ml of the composite wastewater containing the Cd (0.0098 mg/L), Cr (0.3027 mg/L), and Pb (0.1021 mg/L) metals (at pH 2 for Cr and 6 for Pb and Cd). The solution was shaken for 80 min in the case of Cd and Cr (60 min for Pb), filtered using Whatman filter paper stored in refrigeration prior to GFAAS analysis. The adsorption capacity and removal efficiency were calculated using Eqs 3, 4, respectively:
where qe is heavy metal ions concentration adsorbed on adsorbent at equilibrium (mg of metal ion/g of adsorbent), Co and Ce are the initial and equilibrium concentration or final concentration of metal ions in the solution (mg/L), V is the initial volume of metal ions solution used (in L) and m is the mass of adsorbent (in g). The removal efficiency was calculated using Eq. (4):
where C0 and Ce are the initial concentration of heavy metals (mg/L) and the equilibrium concentration of heavy metals (mg/L), respectively.
Results and Discussion
Iodine Number Analysis for the Prepared Cu-DPA MOF
The synthetic process was conducted at five different (i.e., for 1, 2, 3, 4 and 5 days) times of reaction. The copper nitrate trihydrate and diphenylamine precursors were completely dissolved in ethanol and distilled water, respectively prior to mixing and stirring. The two solutions with 1:1 M ration were then mixed, stirred for 1 h, and allowed to settle for 1, 2, 3, 4, and 5 days. The separated yield (based on metal precursor) increases from 38 to 92% as the reaction time increases from 1 d to 3 days. However, further increase in reaction time up to 5 days did not show significant improvement in yield, indicating the saturation level of the synthetic route. Analysis of iodine adsorption number is used to investigate the pore nature (e.g., microporous, mesoporous, and macroporous) of most adsorbents. The type of pores is an indication of adsorption capacity. Furthermore, the iodine adsorption number is used to evaluate the level of activation (a higher degree indicates stronger activation), and it is commonly provided in mg/g (with a typical range of 500–1,200 mg/g). The metal to ligand precursor ratio and their corresponding iodine number and isolated yield are shown in Table 2. The 1:1 metal to ligand ratio provided maximum iodine value (635 mg/g) and yield (92%), indicating good adsorption capacity with high porosity and high surface area. The adsorption capacity of Cu-DPA MOF decreases with increasing metal to ligand precursor ratio from 1:1 to 4:1. This is because the macropore structure of Cu-MOF deteriorated due to excessive copper metal that leads to the formation of a macropore in the MOF and basically due to the effect of pore widening that leads to the destruction of pore walls between neighboring pores, which decreases adsorption performance (Gupta et al., 2003). The iodine number value obtained for the 1:1 Cu-DPA MOF is comparable with zeolite-based adsorbents (641.7 mg/g) and twofold higher than activated carbon-based adsorbent from tamarind seeds (300 mg/g) reported before (Mopoung et al., 2015; Fanta et al., 2019). On the other hand, relatively higher iodine number (895 mg/g) was reported for activated carbon obtained from bean husk (Evwierhoma et al., 2018). Based on the determined iodine value and isolated yield, the 1:1 ratio is considered to be the optimal combination ratio. Fascinated by this observation, we fully characterized the prepared Cu-DPA MOF using a 1:1 ratio of metal to ligand combination.
Crystallinity Studies of Adsorbent
The crystal structure of the as-synthesized Cu-DPA MOF was examined using XRD measurements of the samples. Figure 1 displays XRD patterns of as-prepared Cu-DPA MOF. As visibly evidenced in Figure 1A, a strong diffraction peak observed at 2 Theta values of 12.8°, 18.6°, and 20.2° are characteristics of Cu-DPA MOF and have good similarity with the diffraction patterns of other Cu-based MOF (Loera-Serna et al., 2012), indicating the profound crystalline nature of the prepared MOF. The calculated relative grain size (i.e., using the Scherer equation) was found to be 22.3 nm, indicating a mesoporous characteristic nature of the prepared MOF. This agrees well with the iodine adsorption number analysis. To examine the synthesized MOF’s structural information, we also measured FT-IR spectra of the prepared Cu-DPA MOF in the region from 400 cm−1 to 4,000 cm−1 in KBr using an FTIR spectrometer. Figure 1B shows the FTIR spectra of Cu-DPA MOF. As clearly displayed in Figure 1B, the presence of a broad peak at 3,384 cm−1 in the as-prepared Cu-DPA MOF sample can be assigned to the N–H stretching. The diminished feature of this peak is a solid indicative for the linkage formed between copper and diphenylamine ligand. The peaks located at 1,497 and 1,319 cm−1 are assigned to the N–H stretching vibrations of the aromatic ring. The Cu-diphenylamine ligand asymmetric stretching is observed at 749 cm−1. The existence of NH functional group could act as a chemical binding agent via its dissociation into a negatively charged surface which could attract positively charged ions like metals. It can be seen that the IR-spectra indicated the presence of ionizable functional groups; their ionization leaves vacant sites which can be replaced by metal ions. This gives an indication that this material could be used as adsorbents for heavy metals removal (Aziz et al., 2005).
Method of Detection Limit and Method Validation
Before quantifying the metals in the wastewater samples, the MDL of the GFAAS was evaluated by analyzing six blank samples. It was found that the MDL of Pb, Cd, and Cr was 0.0615 mg/L, 0.0231 mg/L, and 0.0535 mg/L, respectively. By adding known concentrations of each element to the wastewater sample, the efficiency of the digestion technique and analytical method (GFAAS) was tested. In the same way, the spiked and non-spiked samples were digested and examined. By spiking 2.0 ml of 12.5 mg/L of each metal into a 50 ml wastewater sample, the efficiency of the optimized procedure was tested. As shown in Table 3, the calculated percent recoveries for studied metals in the wastewater sample (i.e., 93.26–98.2%) lie within the acceptable range (Mico et al., 2006).
Optimization Parameters for Removal of Heavy Metals (Using Synthetic Solution)
Effect of pH
The initial pH of the metal ion solution is a significant factor in controlling the adsorption process. In this study, the effect of pH on removal efficiency of heavy metals was studied from a range of 2–10 at a contact time of 30 min, metal ion solution of 20 mg/L, Cu-DPA based adsorbents of 1.5 g, temperature of 25 °C, and at an agitation speed of 150 rpm (Figure 2). As shown in Figure 2, both the adsorption capacity (Figure 2A) and the percentage removal of Pb and Cd (Figure 2B) increase as the pH increases from 2 to 6. However, a decrease in adsorption capacity and removal percentages were observed when the pH values ranged from 6 to 10. The maximum percentage removal of Cd (92.4%) and Pb (94.05%) were obtained at pH 6. This is in good agreement with the findings obtained by Rashed et al. (2018) using modified physically activated sewage sludge adsorbents. The removal of Cd and Pb at lower pH (pH < 3 was low because protons could occupy most of the adsorption), however, at moderate pH, the proton linked to the adsorbent surface gets released from the active sites of the adsorbent resulting in increased adsorbed metal ions. The low percentage removal efficiency of Cu-DPA at a higher pH value (pH > 6) might be due to the formation of metal precipitate that decreases adsorption. Increasing the adsorption capacity of metals with increasing pH is attributed to two possible mechanisms; cation exchange and surface complexation. The slow adsorption at high pH is probably due to electrostatic hindrance between adsorbed positively charged adsorbate into the surface of adsorbent and cationic adsorbate species in the solution, and slow pore diffusion into the bulk of the adsorbent.
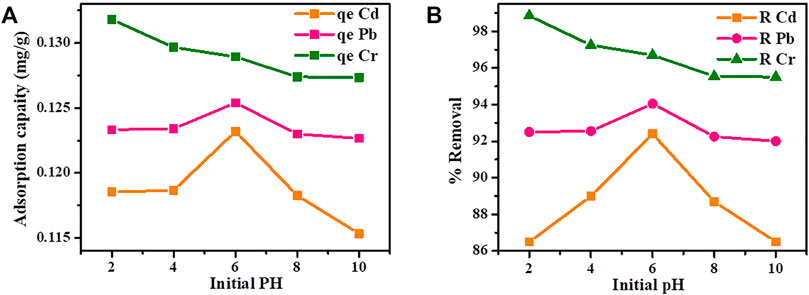
FIGURE 2. Effect of initial PH on (A) adsorption capacity and (B) removal efficiency of Cd, Pb and Cr by Cu-DPA MOF.
On the other hand, as seen in Figure 2B, the percentage removal for Cr decreases as the pH increases from 2–10, and a maximum percentage removal (98%) was attained at pH 2. The finding in this study was in good agreement with the literature report. For instance, at pH 2, the study conducted by Liu et al. (2014) revealed a removal efficiency of 87% Cr from wastewater using Zizania caduciflora adsorbent. Mubarak et al. (2014) used manganese oxide as adsorbent to remove chromium from wastewater and obtained maximum removal efficiency of 85% at pH 2. The greater removal effectiveness of Cr at low pH is attributable to the neutralization of negative charges on the adsorption surface by abundant hydrogen ions, allowing hydrogen chromate ions to diffuse more easily (HCrO4−) and their subsequent adsorption (Dula et al., 2014). At basic pH conditions Cr adsorption onto Cu-DPA MOF decreases due to the formation of hydrolysis and polymeric products such as CrOH2+, Cr2(OH)24+, and Cr3(OH)45+. This can be best explained on the basis of the lower extent protonated hydroxyl groups with rising pH. In view of the aforementioned results, the optimum pH for Cd, Pb, and Cr adsorption is taken to be 6, 6, and 2, respectively, in the following experiments.
Effect of Adsorbent Dosage
The effect of adsorbent dose was studied by varying the amount of Cu-DPA MOF from 0.5–3.5 g/20 ml (Figure 3). As seen in Figure 3A, the adsorption capacity of Cd, Pb, and Cr decreases with an increase in Cu-DPA MOF amount from 0.5–3.5 g. Here the response from Cd and Pb was observed to be the same. The percentage removal of Pb, Cd, and Cr were increased from 71.4 to 88.5%, 91–94% and 92.5–94.5%, respectively, when the Cu-DPA MOF dose increased from 0.5 to 1.5 g (Figure 3B) and remained nearly constant at adsorbent doses higher than 1.5 g. The constant removal efficiency with increasing dose of adsorbent is attributed to adsorption sites remaining unsaturated during the adsorption reaction which is a result of the larger number of available adsorption sites favoring the enhanced uptake of the metal ions (Liu et al., 2007; Zou et al., 2016).
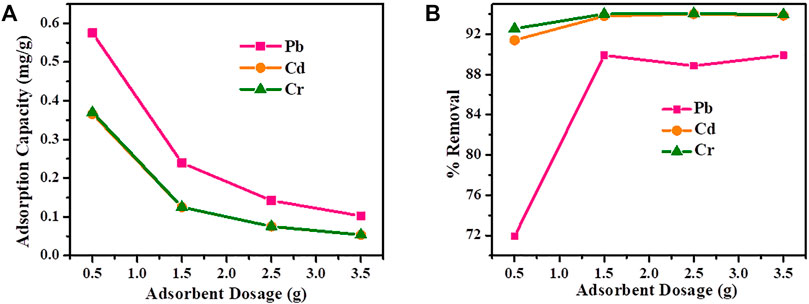
FIGURE 3. Effect of adsorbent dose on (A) adsorption Capacity and (B) removal efficiency of Cd. Pb and Cr.
Effect of Initial Metals Concentration and Contact Time
We also carried out intensive optimization experiments revealing the effect of initial metal concentration and contact time. To a different 50 ml size Erlenmeyer’s flask, 25 ml sample of 10, 20, 40, 60, and 80 mg/L concentrations of Pb, Cd, and Cr were added. The optimum dose of Cu-DPA MOF (1.5 g for each metal ion) was added to each of the above metal concentrations, and the mixture was mechanically stirred for their optimum time. After filtration, the concentration of Pb, Cd, and Cr were analyzed using atomic absorption spectroscopy. Figure 4 shows the effect of initial metal ion concentration of Cr, Pb, and Cd and contact time on Pb, Cd, and Cr removal efficiency by Cu-DPA MOF over Cu-DPA MOF under optimum pH and adsorbent dose. As shown in Figure 4A, the percentage removal efficiency increased from 48 to 95%, 35–85%, and 32–83% for Cr, Cd, and Pb, respectively, with increasing initial concentration from 5 to 20 mg/L. It is revealed that Cu-DPA MOF exhibited higher removal efficiency of metal ions in the order of Cr > Cd > Pb at all initial concentration value. The difference in percent removal of distinct heavy metal ions at the same initial metal ion concentration, adsorbent dose, and contact time could be related to differences in chemical affinity and ion exchange capacity with respect to the chemical functional group on the adsorbent’s surface (Hermes et al., 2007). The maximum removal efficiency of the Cu-DPA MOF was found to be 83.3, 89.7, and 95.0% for Pb, Cd, and Cr, respectively, and the removal efficiency slightly decreased or reached the saturation point at higher initial concentrations. The increase in adsorption or removal capacities of adsorbents with rising metal ion concentration might be explained by an acceleration of metal ion diffusion into adsorbents due to an increase in concentration gradient with increasing initial metal ion concentration (Li et al., 2012). As a result, the extent of each metal ion uptake by the adsorbents increases significantly with the increase of contact time. The slight decrease in the removal capacity or remaining constant after the saturation point of the adsorbents at higher initial metal ion concentration could be due to a higher probability of collision between the adsorbent particle (Cu-DPA MOF) and the metal ions; and decrease in the available active sites for adsorption due to their saturation.
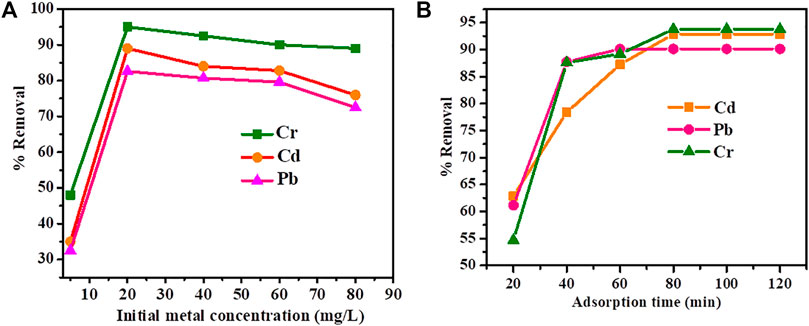
FIGURE 4. Effect of (A) initial metals ion concentration and (B) contact time on removal efficiency of Pb, Cd and Cr.
The effect of contact time on percentage removal of each metal ion by Cu-DPA MOF was also studied at various intervals (20–120 min) using optimum pH, adsorbent dosage, and initial metal concentration. As shown in Figure 4B, increasing the contact time increases the percentage removal of each metal until the system reaches the adsorption equilibrium. The adsorption was very fast from the beginning and with further increase of adsorption time. In this study, it is found that the optimum contact time revealing maximum percentage removal of Cd (92.9%) and Cr (93.8%) was 80 min. While in the case of Pb, high removal efficiency of 90.13% was attained within 60 min. This finding is in good agreement with the result obtained by Kowsura et al. (2017) on Pb removal from water using Zn3(BTC)2. Liu et al. (2007) reported chromium removal with zero-valent iron decorant on a graphene nanosheet by using 1 g adsorbent dosage within 90 min has a removal efficiency of 70%. It is best known that the rate of metal ion uptake was higher at the initial stage of adsorption; however, a slower adsorption would follow as the available adsorption site is gradually decreased. This can be best explained by the fact that initially, the rate of ion uptake was higher because all sites on the adsorbent were vacant and ion concentration was high, but remain constant since all sites occupied hence as it does not take up the additional adsorbate.
Adsorption Experiment for Real Sample
Fascinated by the above findings, we also carried out investigation of removal efficiency of the as prepared Cu-DPA MOF toward Cd, Pb, and Cr in real wastewater samples collected from the car wash Labajo area located at Gamo Gofa zone, Arba Minch town, Ethiopia. The experiments were conducted under optimized conditions shown in the previous section. As clearly demonstrated in Table 4, the concentration of Pb (0.1021 mg/L), Cd (0.0098 mg/L), and Cr (0.3027 mg/L) in the wastewater before treatment was much higher than the permissible limit set by the World Health Organization. However, the corresponding concentration of Pb, Cd, and Cr was reduced to 0.0056, 0.0024, and 0.0015 mg/L after the treatment with the Cu-DPA MOF adsorbent. As a result, the removal efficiency of Cu-DPA MOF adsorbent for heavy metals in a real sample was calculated to be 97.6%, 99.5%, and 99.5% for Cd, Pb, and Cr, respectively. This indicates the as-prepared metal organic framework is very powerful and promising toward treatment of wastewater. Motivated by these findings, we also studied the regeneration of the Cu-DPA MOF after adsorption experiment. Herein a methanol solution followed by irradiation of the sample with low power UV was used to regenerate the used Cu-DPA MOF (Gupta et al., 2021). We first submerged and stirred the used MOF in 10 ml of methanol for 24 h at 500 rpm, followed by centrifugation and drying at ambient temperature for 24 h. Then, the dried MOF was illuminated with a low power UV lamp using a Pyrex tube fitted in an acryl reactor. About 91%, 94%, and 93.5% of the Cd, Cr, and Pb, respectively, could be removed from the used Cu-DPA MOF based on GFAAS analysis from the supernatant solution. Next, we reuse the regenerated Cu-DPA MOF under the same condition, the corresponding Cd, Cr, and Pb removal efficiency was reduced to 81%, 86%, and 87%, respectively, indicating that the MOF still exhibits efficient removal efficiency after regeneration. We further conducted scanning electron microscopy measurements to examine the morphology and microstructure of the 1:1 Cu-DPA MOF. As clearly displayed in Figure 5A, the as-prepared MOF before adsorption experiment exhibited a bright surface with octahedral and triangular particle features. However, when the MOF was taken to the adsorption experiment (Figure 5B), the small particles are observed over the octahedral and triangular structures of the MOF, indicating the adsorption of the metals over the MOF surface. Regardless of the coverage of the MOF surface with a small particle, there was no significant change in morphology after adsorption experiment.

TABLE 4. Removal of Pb, Cd, and Cr from real water samples collected from the car wash Labajo area using Cu-DPA MOF at optimized conditions.
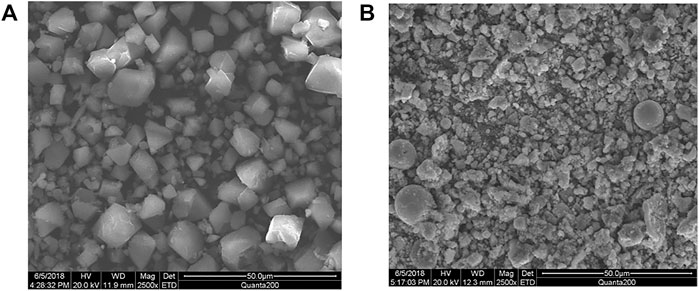
FIGURE 5. SEM images of the as prepared Cu-DPA MOF (A) before adsorption and (B) after adsorption experiment.
Adsorption Isotherms
To further investigate the relationship between adsorbent and adsorbate, we also underwent analysis of the adsorption process via the most commonly used models such as Langmuir and Freundlich isotherms. Adsorption happens at homogeneous locations, forming a monolayer, according to the Langmuir isotherm. In other words, once an adsorbate has been linked to a site, no additional adsorption can occur. Mathematically, the Langmuir isotherm is given by the following equation:
where qm is to the adsorption capacity (mg/g), qe is the equilibrium concentration of metals in the adsorbed phase (mg/g), Ce is the equilibrium concentration in the liquid phase (mg/L), and Langmuir constants, which are related to the adsorption capacity (qm) and energy of adsorption (
where Co is initial concentration of adsorbate (mg/L),
where qe is the amount adsorbed at equilibrium (mg/g),
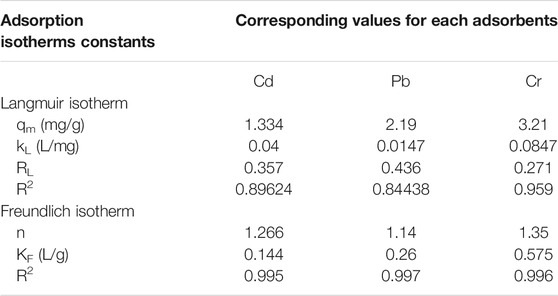
TABLE 5. Calculated isotherm parameters for the adsorption of Pb, Cd, and Cr on Cu-DPA MOF at 300 K.
The adsorption capacity of the Cu-DPA MOF after optimizing all the parameters was estimated to be 2.19, 1.33, and 3.21 mg/g for Pb, Cd, and Cr, respectively. More interestingly, the obtained adsorption capacity is much higher than the reported values Cr (0.5–0.6 mg/g). Pb (1.9 mg/g) and Cd (0.63 mg/g) used activated carbon made from bamboo materials and carbon nanotube (Atieh et al., 2010; Lo et al., 2012). Furthermore, these adsorption capacity values are also comparable with activated carbon made from maize cob, 1.89 and 1.98 mg/g for Pb2+ and Cd2+, respectively (Igwe and Abia, 2007). On the other hand, the adsorption capacity obtained in the present study is relatively lower than the reported values for adsorbents such as natural zeolite (>9.0 mg/g). Synthetic zeolite (500 mg/g) and activated carbon made from the coffee husk and rice husk (3.0–38 mg/g) (Oliveira et al., 2008; Srivastava et al., 2008; Hamidpour et al., 2010; Agarwal and Singh, 2017).
Conclusion
We have successfully synthesized a highly porous nature Cu-DPA MOF via hydrothermal method from copper nitrate trihydrate and diphenylamine linker. The use of as-synthesized MOF was investigated for the removal of heavy metals from wastewater. The Cu-DPA MOF was characterized by XRD, FTIR, and iodine number analysis that shows the material is capable of adsorbing the adsorbate. Our material revealed a maximum removal efficiency of 97.5%, 99.5%, and 99.5% toward Cr, Cd, and Pb metals, respectively, from wastewater sample. The adsorption parameters are found to be important factors for heavy metals removal, i.e., as initial metals concentration, pH value, contact time, and Cu-MOF dosage exhibited significant effect on the adsorption process. The adsorption process fit well with Freundlich isotherm with R2 (0.995, 0.997, 0.996) for Cd, Pb, and Cr, respectively. This indicates the formation of a multilayer on the surface of the adsorbent. The adsorbent in this study reveals very good potentials for simultaneous removal of toxic metals from high-volume contaminated wastewaters. It can also be used as an adsorbent instead of more expensive and ineffective materials.
Data Availability Statement
The original contributions presented in the study are included in the article/Supplementary Material, further inquiries can be directed to the corresponding author.
Author Contributions
HWH performed the experiment; HWH and MAC collected the data and drafted the manuscript; MA validated the data; AAD designed the study and supervision; AAD and MA edited the manuscript. All authors read and approved the final manuscript.
Conflict of Interest
The authors declare that the research was conducted in the absence of any commercial or financial relationships that could be construed as a potential conflict of interest.
Publisher’s Note
All claims expressed in this article are solely those of the authors and do not necessarily represent those of their affiliated organizations, or those of the publisher, the editors, and the reviewers. Any product that may be evaluated in this article, or claim that may be made by its manufacturer, is not guaranteed or endorsed by the publisher.
Acknowledgments
The authors would like to gratefully acknowledged Dilla University for financial support and provision of laboratory facilities.
References
Agarwal, M., and Singh, K. (2017). Heavy Metal Removal from Wastewater Using Various Adsorbents: a Review. J. Water Reuse Desalin. 7 (4), 387–419. doi:10.2166/wrd.2016.104
Ahluwalia, S. S., and Goyal, D. (2007). Microbial and Plant Derived Biomass for Removal of Heavy Metals from Wastewater. Bioresour. Tech. 98 (12), 2243–2257. doi:10.1016/j.biortech.2005.12.006
Aksu, Z. (2005). Application of Biosorption for the Removal of Organic Pollutants: a Review. Process. Biochem. 40 (3-4), 997–1026. doi:10.1016/j.procbio.2004.04.008
Asghar, A., Bello, M. M., and Raman, A. A. A. (2021). “Metal-Organic Frameworks for Heavy Metal Removal,” in Applied Water Science Volume 2, ed(s). I. Inamuddin, M. I. Ahamed, R. Boddula, and T. A. Rangreez (John Wiley & Sons), 321–356. doi:10.1002/9781119725282.ch9
Atieh, M. A., Bakather, O. Y., Tawabini, B. S., Bukhari, A. A., Khaled, M., Alharthi, M., et al. (2010). Removal of Chromium (III) from Water by Using Modified and Nonmodified Carbon Nanotubes. J. Nanomater. 2010, 1–9. doi:10.1155/2010/232378
Aziz, H. A., Adlan, M. N., Hui, C. S., Zahari, M. S. M., and Hameed, B. H. (2005). Removal of Ni, Cd, Pb, Zn and Colour from Aqueous Solution Using Potential Low Cost Adsorbent. Indian J. Eng. Mater. Sci. 12 (3), 248–258.
Bakhtiari, N., and Azizian, S. (2015). Adsorption of Copper Ion from Aqueous Solution by Nanoporous MOF-5: a Kinetic and Equilibrium Study. J. Mol. Liquids 206, 114–118. doi:10.1016/j.molliq.2015.02.009
Burg, A., and Meyerstein, D. (2012). “The Chemistry of Monovalent Copper in Aqueous Solutions,” in Advances in Inorganic Chemistry, ed (s). R. Eldik, and I. Ivanović-Burmazović (Academic Press), 219–261. doi:10.1016/B978-0-12-396462-5.00007-6
Cui, L., Wang, Y., Gao, L., Hu, L., Yan, L., Wei, Q., et al. (2015). EDTA Functionalized Magnetic Graphene Oxide for Removal of Pb(II), Hg(II) and Cu(II) in Water Treatment: Adsorption Mechanism and Separation Property. Chem. Eng. J. 281, 1–10. doi:10.1016/j.cej.2015.06.043
Dehghankar, M., Mohammadi, T., Moghadam, M. T., and Tofighy, M. A. (2021). Metal-organic Framework/zeolite Nanocrystal/polyvinylidene Fluoride Composite Ultrafiltration Membranes with Flux/antifouling Advantages. Mater. Chem. Phys. 260, 124128. doi:10.1016/j.matchemphys.2020.124128
Dula, T., Siraj, K., and Kitte, S. A. (2014). Adsorption of Hexavalent Chromium from Aqueous Solution Using Chemically Activated Carbon Prepared from Locally Available Waste of Bamboo (Oxytenanthera Abyssinica). Int. Sch. Res. Notices 2014, 1–9. doi:10.1155/2014/438245
Efome, J. E., Rana, D., Matsuura, T., and Lan, C. Q. (2018). Metal-organic Frameworks Supported on Nanofibers to Remove Heavy Metals. J. Mater. Chem. A. 6 (10), 4550–4555. doi:10.1039/c7ta10428f
Esrafili, L., Safarifard, V., Tahmasebi, E., Esrafili, M. D., and Morsali, A. (2018). Functional Group Effect of Isoreticular Metal-Organic Frameworks on Heavy Metal Ion Adsorption. New J. Chem. 42 (11), 8864–8873. doi:10.1039/C8NJ01150H
Evwierhoma, E. T., Madubiko, O. D., and Jaiyeola, A. (2018). Preparation and Characterization of Activated Carbon from Bean Husk. Nig. J. Tech. 37 (3), 674–678. doi:10.4314/njt.v37i3.17
Fanta, F. T., Dubale, A. A., Bebizuh, D. F., and Atlabachew, M. (2019). Copper Doped Zeolite Composite for Antimicrobial Activity and Heavy Metal Removal from Waste Water. BMC Chem. 13 (1), 44–12. doi:10.1186/s13065-019-0563-1
Gupta, V. K., Jain, C. K., Ali, I., Sharma, M., and Saini, V. K. (2003). Removal of Cadmium and Nickel from Wastewater Using Bagasse Fly Ash-A Sugar Industry Waste. Water Res. 37 (16), 4038–4044. doi:10.1016/s0043-1354(03)00292-6
Gupta, N. K., Kim, S., Bae, J., and Kim, K. S. (2021). Chemisorption of Hydrogen Sulfide Over Copper-Based Metal-Organic Frameworks: Methanol and UV-Assisted Regeneration. RSC Adv. 11, 4890–4900. doi:10.1039/D0RA09017D
Hamidpour, M., Afyuni, M., Kalbasi, M., Khoshgoftarmanes, A. H., and Inglezakis, V. J. (2010). Mobility and Plant-Availability of Cd(II) and Pb(II) Adsorbed on Zeolite and Bentonite. Appl. Clay Sci. 48 (3), 342–348. doi:10.1016/j.clay.2010.01.004
Hermes, S., Zacher, D., Baunemann, A., Wöll, C., and Fischer, R. A. (2007). Selective Growth and MOCVD Loading of Small Single Crystals of MOF-5 at Alumina and Silica Surfaces Modified with Organic Self-Assembled Monolayers. Chem. Mater. 19 (9), 2168–2173. doi:10.1021/cm06285410.1021/cm062854++
Hoseinzadeh, H., Hayati, B., Shahmoradi Ghaheh, F., Seifpanahi-Shabani, K., and Mahmoodi, N. M. (2021). Development of Room Temperature Synthesized and Functionalized Metal-Organic Framework/graphene Oxide Composite and Pollutant Adsorption Ability. Mater. Res. Bull. 142, 111408. doi:10.1016/j.materresbull.2021.111408
Hussain, A. Z., and Sheriff, K. M. (2014). Removal of Heavy Metals from Wastewater Using Low Cost Adsorbents. Arch. Appl. Sci. Res. 6 (6), 52–54. doi:10.5772/intechopen.95841
Igwe, J. C., and Abia, A. A. (2007). Adsorption Isotherm Studies of Cd (II), Pb (II) and Zn (II) Ions Bioremediation from Aqueous Solution Using Unmodified and EDTA-Modified maize Cob. Eclet. Quím. 32 (1), 33–42. doi:10.1590/S0100-46702007000100005
Jayaweera, H. D. A. C., Siriwardane, I., de Silva, K. M. N., and de Silva, R. M., (2018). Synthesis of Multifunctional Activated Carbon Nanocomposite Comprising Biocompatible Flake Nano Hydroxyapatite and Natural Turmeric Extract for the Removal of Bacteria and lead Ions from Aqueous Solution. Chem. Cent. J. 12 (1), 18–14. doi:10.1186/s13065-018-0384-7
Kowsura, C., Pangkumhang, B., Pangkumhang, B., Jutaporn, P., and Tanboonchuy, V. (2017). Isotherm Models of Heavy Metal Sorption onto Zinc-Tricarboxylic. Ijcea 8 (3), 179–183. doi:10.18178/ijcea.2017.8.3.652
Li, S., Lu, X., Li, X., Xue, Y., Zhang, C., Lei, J., et al. (2012). Preparation of Bamboo-like PPy Nanotubes and Their Application for Removal of Cr(VI) Ions in Aqueous Solution. J. Colloid Interf. Sci. 378 (1), 30–35. doi:10.1016/j.jcis.2012.03.065
Liu, H., Liang, S., Gao, J., Ngo, H. H., Guo, W., Guo, Z., et al. (2014). Enhancement of Cr(VI) Removal by Modifying Activated Carbon Developed from Zizania Caduciflora with Tartaric Acid during Phosphoric Acid Activation. Chem. Eng. J. 246, 168–174. doi:10.1016/j.cej.2014.02.046
Liu, Y., Xiao, D., and Li, H. (2007). Kinetics and Thermodynamics of lead (II) Adsorption on Vermiculite. Sep. Sci. Tech. 42 (1), 185–202. doi:10.1080/01496390600998045
Lo, S.-F., Wang, S.-Y., Tsai, M.-J., and Lin, L.-D. (2012). Adsorption Capacity and Removal Efficiency of Heavy Metal Ions by Moso and Ma Bamboo Activated Carbons. Chem. Eng. Res. Des. 90 (9), 1397–1406. doi:10.1016/j.cherd.2011.11.020
Loera-Serna, S., Oliver-Tolentino, M. A., de Lourdes López-Núñez, M., Santana-Cruz, A., Guzmán-Vargas, A., Cabrera-Sierra, R., et al. (2012). Electrochemical Behavior of [Cu3(BTC)2] Metal-Organic Framework: The Effect of the Method of Synthesis. J. Alloys Comp. 540, 113–120. doi:10.1016/j.jallcom.2012.06.030
Madadrang, C. J., Kim, H. Y., Gao, G., Wang, N., Zhu, J., Feng, H., et al. (2012). Adsorption Behavior of EDTA-Graphene Oxide for Pb (II) Removal. ACS Appl. Mater. Interfaces 4 (3), 1186–1193. doi:10.1021/am201645g
Mahmoodi, N. M., Arabloo, M., and Abdi, J. (2014). Laccase Immobilized Manganese Ferrite Nanoparticle: Synthesis and LSSVM Intelligent Modeling of Decolorization. Water Res. 67, 216–226. doi:10.1016/j.watres.2014.09.011
Mahmoodi, N. M., and Saffar-Dastgerdi, M. H. (2019). Zeolite Nanoparticle as a superior Adsorbent with High Capacity: Synthesis, Surface Modification and Pollutant Adsorption Ability from Wastewater. Microchemical J. 145, 74–83. doi:10.1016/j.microc.2018.10.018
Majeed, M. R., Muhammed, A. S., and Rasheed, K. A. (2014). The Removal of Zinc, Chromium and Nickel from Industrial Waste Water Using Rice Husk. Iraqi J. Sci. 55 (2A), 411–418.
Mico, C., Peris, M., Sánchez, J., and Recatalá, L. (2006). Heavy Metal Content of Agricultural Soils in a Mediterranean Semiarid Area: the Segura River Valley (Alicante, Spain). Span.J. Agric. Res. (4), 363–372.
Mopoung, S., Moonsri, P., Palas, W., and Khumpai, S. (2015). Characterization and Properties of Activated Carbon Prepared from Tamarind Seeds by KOH Activation for Fe(III) Adsorption from Aqueous Solution. Scientific World J. 2015, 1–9. doi:10.1155/2015/415961
Mubarak, N. M., Sahu, J. N., Abdullah, E. C., and Jayakumar, N. S. (2014). Removal of Heavy Metals from Wastewater Using Carbon Nanotubes. Sep. Purif. Rev. 43 (4), 311–338. doi:10.1080/15422119.2013.821996
Nguyen, T. A. H., Ngo, H. H., Guo, W. S., Zhang, J., Liang, S., Yue, Q. Y., et al. (2013). Applicability of Agricultural Waste and By-Products for Adsorptive Removal of Heavy Metals from Wastewater. Bioresour. Tech. 148, 574–585. doi:10.1016/j.biortech.2013.08.124
Oliveira, W. E., Franca, A. S., Oliveira, L. S., and Rocha, S. D. (2008). Untreated Coffee Husks as Biosorbents for the Removal of Heavy Metals from Aqueous Solutions. J. Hazard. Mater. 152 (3), 1073–1081. doi:10.1016/j.jhazmat.2007.07.085
Qasem, N. A. A., Mohammed, R. H., and Lawal, D. U. (2021). Removal of Heavy Metal Ions from Wastewater: a Comprehensive and Critical Review. Npj Clean. Water 4, 36. doi:10.1038/s41545-021-00127-0
Rajasulochana, P., and Preethy, V. (2016). Comparison on Efficiency of Various Techniques in Treatment of Waste and Sewage Water - A Comprehensive Review. Resource-Efficient Tech. 2 (4), 175–184. doi:10.1016/j.reffit.2016.09.004
Rashed, N. M., Soltan, M. E., Ahmed, M. M., and Abdou, A. (2018). Heavy Metals Removal from Wastewater by Adsorption on Modified Physically Activated Sewage Sludge. Arc Org. Inorg. Chem. Sci. 1 (1), 1–8. doi:10.32474/aoics.2018.01.000102
Sharma, S., and Bhattacharya, A. (2017). Drinking Water Contamination and Treatment Techniques. Appl. Water Sci. 7 (3), 1043–1067. doi:10.1007/s13201-016-0455-7
Srivastava, V. C., Mall, I. D., and Mishra, I. M. (2008). Removal of Cadmium (II) and Zinc (II) Metal Ions from Binary Aqueous Solution by rice Husk Ash. Colloids Surf. A: Physicochem. Eng.Asp 312 (2-3), 172–184. doi:10.1016/j.colsurfa.2007.06.048
Tesfaye, D. (2016). Removal of Lead from Wastewater Using Corncob Activated Carbon as an Adsorbent. M.Sc Thesis. Addis Ababa: Addis Ababa University
Thamilarasu, P., and Karunakaran, K. (2013). Kinetic, Equilibrium and Thermodynamic Studies on Removal of Cr (VI) by Activated Carbon Prepared from Ricinus communis Seed Shell. Can. J. Chem. Eng. 91 (1), 9–18. doi:10.1002/cjce.20675
WHO (2011). Guidelines for Drinking Water Quality. 4th edn. Geneva: World Health Organization ( WHO. ISBN 978 92 4 154815 1.
Xie, M.-H., Cai, W., Chen, X., Guan, R.-F., Wang, L.-M., Hou, G.-H., et al. (2018). Novel CO2 Fluorescence Turn-On Quantification Based on a Dynamic AIE-Active Metal-Organic Framework. ACS Appl. Mater. Interfaces 10 (3), 2868–2873. doi:10.1021/acsami.7b17793
Yang, X., and Xu, Q. (2017). Bimetallic Metal-Organic Frameworks for Gas Storage and Separation. Cryst. Growth Des. 17 (4), 1450–1455. doi:10.1021/acs.cgd.7b00166
Zhuang, J., Kuo, C.-H., Chou, L.-Y., Liu, D.-Y., Weerapana, E., and Tsung, C.-K. (2014). Optimized Metal-Organic-Framework Nanospheres for Drug Delivery: Evaluation of Small-Molecule Encapsulation. ACS nano 8 (3), 2812–2819. doi:10.1021/nn406590q
Keywords: wastewater, heavy metals, adsorption, removal efficiency, metal-organic framework
Citation: Haso HW, Dubale AA, Chimdesa MA and Atlabachew M (2022) High Performance Copper Based Metal Organic Framework for Removal of Heavy Metals From Wastewater. Front. Mater. 9:840806. doi: 10.3389/fmats.2022.840806
Received: 21 December 2021; Accepted: 24 January 2022;
Published: 08 March 2022.
Edited by:
Daniel Manaye Kabtamu, National Taiwan University of Science and Technology, TaiwanReviewed by:
Niyaz Mohammad Mahmoodi, Institute for Color Science and Technology (ICST), IranYuni Krisnandi, Universitas indonesia, Indonesia
Mona Elsayed Ossman, Pharos University in Alexandria, Egypt
Copyright © 2022 Haso, Dubale, Chimdesa and Atlabachew. This is an open-access article distributed under the terms of the Creative Commons Attribution License (CC BY). The use, distribution or reproduction in other forums is permitted, provided the original author(s) and the copyright owner(s) are credited and that the original publication in this journal is cited, in accordance with accepted academic practice. No use, distribution or reproduction is permitted which does not comply with these terms.
*Correspondence: Amare Aregahegn Dubale, amare2122@gmail.com