- 1Department of Biomedical Sciences, School of Medicine and Health Sciences, University of North Dakota, Grand Forks, ND, United States
- 2Institut Pasteur, Paris, France
The Lyme disease causing bacterium Borrelia burgdorferi has an affinity for the central nervous system (CNS) and has been isolated from human cerebral spinal fluid by 18 days following Ixodes scapularis tick bite. Signaling from resident immune cells of the CNS could enhance CNS penetration by B. burgdorferi and activated immune cells through the blood brain barrier resulting in multiple neurological complications, collectively termed neuroborreliosis. The ensuing symptoms of neurological impairment likely arise from a glial-driven, host inflammatory response to B. burgdorferi. To date, however, the mechanism by which the bacterium initiates neuroinflammation leading to neural dysfunction remains unclear. We hypothesized that dead B. burgdorferi and bacterial debris persist in the CNS in spite of antibiotic treatment and contribute to the continuing inflammatory response in the CNS. To test our hypothesis, cultures of primary human microglia were incubated with live, antibiotic-killed and antibiotic-killed sonicated B. burgdorferi to define the response of microglia to different forms of the bacterium. We demonstrate that primary human microglia treated with B. burgdorferi show increased expression of pattern recognition receptors and genes known to be involved with cytoskeletal rearrangement and phagocytosis including MARCO, SCARB1, PLA2, PLD2, CD14, and TLR3. In addition, we observed increased expression and secretion of pro-inflammatory mediators and neurotrophic factors such as IL-6, IL-8, CXCL-1, and CXCL-10. Our data also indicate that B. burgdorferi interacts with the cell surface of primary human microglia and may be internalized following this initial interaction. Furthermore, our results indicate that dead and sonicated forms of B. burgdorferi induce a significantly larger inflammatory response than live bacteria. Our results support our hypothesis and provide evidence that microglia contribute to the damaging inflammatory events associated with neuroborreliosis.
Introduction
The bacterium B. burgdorferi causes Lyme disease, which affects many tissues and organs, including the CNS. Lyme neuroborreliosis may manifest as meningitis, cranial neuritis, facial nerve palsy, encephalitis, or peripheral nerve disease (Rupprecht et al., 2008; Ramesh et al., 2013; Halperin, 2015). Additional rare complications can include CNS vasculitis and hemorrhagic stroke (Fallon et al., 2010; Back et al., 2013). While most patients respond well to antibiotics, some experience long-term sequelae including depressive states, decreases in verbal fluency, fatigue, sleep disruptions, emotional lability, and short-term memory problems (Fallon and Nields, 1994; Gustaw et al., 2001; Fallon et al., 2008, 2010; Ljostad and Mygland, 2010). Indeed, approximately 20% of patients treated for Lyme disease experience PTLDS. However, the cause of persistent, lingering neurological symptoms in patients following antibiotic treatment is unknown.
Examination of tissues from patients with neuroborreliosis is limited, but there is evidence for inflammatory changes in the brains of patients, as well as from rhesus macaque models (Sumiya et al., 1997; Ramesh et al., 2013, 2015). Indeed, post-mortem pathological analysis from patients with clinically diagnosed neuroborreliosis revealed lymphocytic infiltrates in the CNS along with widespread microglial activation (Bertrand et al., 1999). While the cause of neurologic symptoms following antibiotic treatment is unclear, dead spirochetes and/or their debris can provide a constant immune stimulus that contributes to inflammatory pathogenic processes (Bockenstedt et al., 2012; Embers et al., 2012, 2017; Wormser et al., 2012; Parthasarathy et al., 2013). Dead spirochetes and spirochetal antigens have been found to persist within injured tissues in human patients with Lyme disease, and spirochetal antigens persist in the joints of mice following antibiotic treatment (Steere et al., 1988; Nanagara et al., 1996; Bockenstedt et al., 2012). Whether such material persists in the CNS of patients is unknown; however, in vitro studies demonstrate that non-viable B. burgdorferi can stimulate the secretion of inflammatory mediators from human CNS cells such as oligodendrocytes (Parthasarathy et al., 2013). Collectively, these reports indicate that the presence of spirochetal debris following antibiotic treatment may drive persistent inflammation.
Microglia are resident CNS cells that respond to injury. These dynamic cells of myeloid origin possess immune receptors such as Fc receptors and TLRs, and as such are poised to infections with microbes such as B. burgdorferi (Lee et al., 2013). Indeed, upon stimulation with B. burgdorferi lysate, expression of TLR1 and TLR2 increases leading to the activation of TLR-linked intracellular signaling pathways (Cassiani-Ingoni et al., 2006; Parthasarathy and Philipp, 2015). Microglia are also facultative phagocytes and, in addition to secreting pro-inflammatory cytokines, produce neurotropic and neuroprotective factors. Hence, microglia are recognized as innate immune cells of the CNS but also have roles in brain homeostasis and tissue repair (Blaylock, 2013). Inflammatory and homeostatic responses must be balanced to eliminate pathogens and repair wounds while preserving brain tissue and minimizing neurological impairment. Continuous stimulation of microglia drives a pro-inflammatory profile in the CNS and disrupt homeostasis.
We hypothesized that dead B. burgdorferi and debris persist in the CNS following antibiotic treatment and promote a chronic inflammatory response in the CNS through interaction with microglial cells. In this study, we compared the innate immune and phagocytic responses of highly pure cultures of primary human microglia to live or killed B. burgdorferi. We demonstrate that primary human microglia treated with B. burgdorferi increase expression of genes involved with innate immunity, cytoskeletal rearrangement, and phagocytosis, and secrete pro-inflammatory mediators and neurotrophic factors. We also visualized B. burgdorferi–microglia interactions and demonstrate that the spirochete adheres to the cell surface and may be internalized.
Materials and Methods
Microglial Culture
Primary human microglial cells were purchased from ScienCell Research Laboratories (Carlsbad, CA, United States; catalog #1901). Microglial cells were maintained on T75 flasks coated with poly-L-lysine (Corning, 2 μg/cm2, T-75) in microglia medium (ScienCell, catalog # 1901) supplemented with 100 units/mL of penicillin and 100 μg/mL of streptomycin. In addition, cultures contained microglia growth supplement (MGS, catalog # 1952). Prior to B. burgdorferi stimulation, medium was replaced with antibiotic-free medium. Microglia were used at passages 2 or 3 at >85% confluence. B. burgdorferi at a MOI of 10:1 bacteria:cells was used to stimulate microglia for 24 or 72 h. Cell purity was determined at all passages using immunocytochemical labeling of parallel cultures grown on 12 mm glass coverslips (Deutsch Deckglaser-NeuVita Inc., Germany) coated with poly-L-lysine (MilliporeSigma, St. Louis, MO, United States) with a specific marker for microglia rabbit anti IBA-1 (Wako Chemicals, Richmond, VA, United States). 97% of cells were Iba-1 positive at passage 2 as were 95% of cells at passage 3, indicating high levels of culture purity as determined by counting the number of IBA-1 expressing cells vs. DAPI stained nuclei (Figure 1). Experimental protocols and commercially available primary cell cultures utilized in these studies followed the University of North Dakota IRB guidelines as stated in section 2.19 “Commercially Available Human Biological Specimens (45 CFR 46.102, 46.103, and 46.116)” and do not require IRB review.
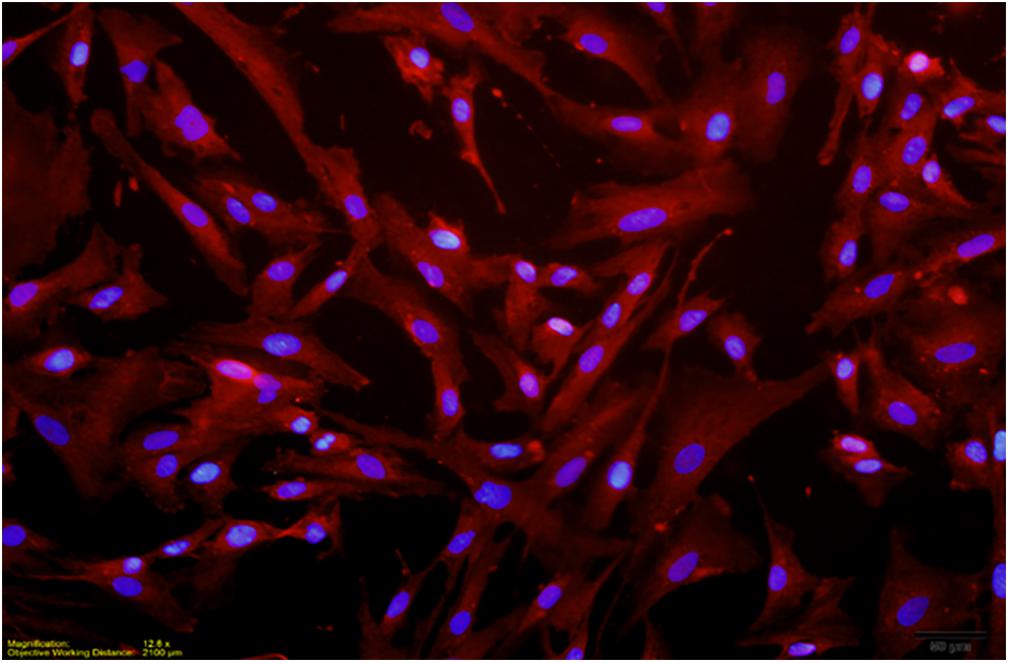
FIGURE 1. Dual localization of DAPI stained nuclei and Texas Red labeled IBA-1 (red) in passage 3 culture. Two independent immunocytochemistry experiments were performed, with two replicate coverslips per experiment. One count was made per quadrant of coverslip to give a total of eight counts for each independent experiment. Cell purity in a passage 3 culture was revealed to be >95%.
Borrelia burgdorferi Culture
Virulent B. burgdorferi strain B31 MI-16 (Fraser et al., 1997; Casjens et al., 2000; Miller et al., 2003), avirulent derivative e2 (Babb et al., 2004), or gfp-expressing B. burgdorferi (kind gift of M.A. Motaleb, Eastern Carolina University; Sultan et al., 2015) were grown at 34°C to cell densities of approximately 1 × 108/mL in modified Barbour-Stoenner-Kelly II (BSK-II) medium (Zückert, 2007). For stimulation of microglial cultures, B. burgdorferi were centrifuged at 6,000 ×g, washed 3X with PBS, and resuspended in microglia medium containing no antibiotics. Bacterial counts were determined using a Petroff-Hausser chamber and viewed using dark field microscopy. For bacterial killing, 1 × 108 B. burgdorferi/mL were treated with ceftriaxone (MilliporeSigma) for 72 h at 5X the minimum inhibitory concentration (Veinovic et al., 2013). Ceftriaxone was chosen as the antibiotic as it is the preferred treatment for neurological symptoms according to both United States and European guidelines for human treatment of Lyme disease (Mygland et al., 2010; Hu, 2012). Complete killing of B. burgdorferi was confirmed by darkfield microscopy and subculture into fresh BSKII medium (data not shown). For stimulation experiments performed with B. burgdorferi sonicate, cultures were subjected to five pulses (amplitude 4 for 12–15 s each) with a probe sonicator (Fisher Scientific, Pittsburgh, PA, United States; Model 120). A 10:1 MOI was used to stimulate microglia cells with live B. burgdorferi. An equivalent of 10:1 MOI was used to stimulate cell cultures with antibiotic-killed B. burgdorferi. The amount of sonicate that was used to stimulate cultures of microglia was equivalent to 10:1 MOI and was determined by the concentration of B. burgdorferi in media prior to spirochetal disruption. In this manner, stimulation experiments were performed at a 10:1 MOI of live or dead bacteria, or the amount of sonicate that would have resulted in a 10:1 MOI had it not been sonicated.
Nucleic Acid Isolation and Synthesis
Total RNA was isolated via the RNeasy kit (Qiagen, Valencia, CA, United States). After aspiration of media from stimulated cultures, adherent cells were washed 3X with warm, sterile PBS. Cells were suspended with trypsin-EDTA (ScienCell) and all enzymatic activity neutralized with the addition of microglial medium containing 5% fetal bovine serum. Buffer RLT (Qiagen) was used to lyse cells. Genomic DNA was then removed by on-column DNA digestion with RNase-Free DNase Set (catalog # 79254, Qiagen). RNA was then concentrated using a the Qiagen RNeasy MinElute Cleanup Kit (catalog # 74204). RNA quality and concentration was assessed by spectrometry (Epoch, Bio-Tek, Winooski, VT, United States). cDNA was synthesized with Qiagen RT2 First Strand Kit (Catalog # 330401) following manufacturer’s instructions.
Gene Expression Analysis by Quantitative Real-Time RT-PCR (qPCR)
The expression of chemokine, cytokine, and neurotrophin genes, as well as those associated with phagocytosis, was determined first with RT2 Profiler PCR arrays (Qiagen) on a Bio-Rad myIQ2 Real-Time PCR instrument. The manufacturer’s software was used to analyze the data. Alterations in individual genes were confirmed using individual PCR primer sets for qRT-PCR (Qiagen; Table 1). Individual reactions contained 5.5 μl nuclease-free water, 2 μl primer mix (both forward and reverse) at 10 μM, and 12.5 μl Bio-Rad Sybr Green Supermix ±5 μl template DNA or no template control (nuclease-free water). Forty cycles of PCTR were performed following an initial 10 min denaturation at 95°C. For each cycle, a 1 min annealing step at 60°C preceded a 15-s melting interval at 95°C. Melting curves were obtained using a stepped temperature gradient of 0.5°C × 10 s starting at 60°C. Transcript expression levels were then compared to standard housekeeping genes (β-actin and GAPDH) and to those of untreated cells using the 2-ΔΔCT method, where CT (threshold cycle). This method was used on each individual example with the untreated sample as the comparator (Schmittgen and Livak, 2008). Triplicate samples were analyzed from a minimum of three independent biological replicates for each time point.
Enzyme-Linked Immunosorbent Assay (ELISA)
Following microglial stimulation, culture supernatants were collected, aliquoted for single use and stored at -80°C. ELISA kits were purchased from R&D Systems (Minneapolis, MN, United States) and were used following manufacturer’s instructions. Briefly, plates were coated overnight with 100 μl of specific capture antibody (e.g., anti-IL-8) followed by multiple wash cycles. Sample standards and controls (100 μl) were then added to individual wells and incubated for 2 h at room temperature. Plates were then washed 3X and 100 μl of antibody conjugate was added to each well, and incubated for 2-h at room temperature and finally chromogenic detection of desired host cell factor. Plates reads were performed at an optical density of 450 nm on a BioTek Epoch plate reader. Triplicate samples from each treatment group were collected and pooled. Data represent the means and standard errors from at least two independent biological replicates analyzed in triplicate per time point.
Immunocytochemistry
Microglia were maintained on poly-L-lysine coated glass coverslips (Neurovita, Germany) in microglia medium (seeding density of 20,000 cells/coverslip). Microglia were stimulated by exposure to B. burgdorferi MOI 10:1 for 24 or 72 h. Microglia were fixed for 15 min in buffered 4% paraformaldehyde and washed 3X with PBS. The cells were given three 10 min PBS plus 0.1% Triton x100 (PBS-T) washes and were incubated for 1 h at room temperature in blocking serum. Blocking serum consisted of 4% normal serum of the species of the secondary antibody. The cells were probed with rabbit anti-IBA-1; 1:500 dilution (Wako Chemicals) diluted in blocking serum and incubated overnight at 4°C. Cells were washed 3X with PBS-T and incubated for 1 h in a solution of biotinylated goat anti-rabbit IgG, (1:500 dilution; Jackson Immunoresearch, West Grove, PA, United States) diluted in blocking serum. Following three more washes with PBS-T, the cells were incubated in a solution of streptavidin-AlexaFluor 594 for 1 h (1:1000 dilution; Thermo Fisher, Grand Island, NY, United States; cat #S11227). Cells were then washed in PBS 3X and coverslipped with Vectashield containing DAPI (Vector Laboratories, Burlingame, CA, United States). Immunostained coverslips were visualized with fluorescent microscopy using an Olympus BX-51 fluorescence microscope with attached DP-71 digital camera and dedicated CellSens Standard software (Olympus, Waltham, MA, United States).
Confocal Microscopy
Coverslips were coated with collagen at 5 μg/cm2 using a ScienCell Collagen I-Cell Culture Surface Coating Kit (Cat #8188) according to manufacturer’s instructions. Microglia were maintained on collagen-coated glass coverslips in microglia medium (seeding density of 20,000 cells/coverslip). Microglia were stimulated by exposure to B. burgdorferi MOI 10:1 for 24 h, or with 5 × 106 beads per well (∼100 ± 25 beads per cell) of green Fluoresbrite Plain YG 1.0 micron microspheres with excitation maxima and emission maxima of 441 nm and 485 nm, respectively (Polysciences Inc., Warrington, PA, United States; Cat# 17154). Cells were fixed for 15 min in buffered 4% paraformaldehyde and washed three times with PBS. The cells were blocked in PBS buffer until labeled with IBA-1 as described above. Images were acquired on an Olympus FV300 confocal microscope. Z stacks were taken at 0.225 μm/image with a Kallman of 3. Images were viewed and edited with Imaris software.
Statistical Analysis
Enzyme-linked immunosorbent assay experiments were carried out two times (24-h samples) or three times (72-h samples and control samples) in biologically independent experiments with triplicate replicates. Chemokine and cytokine qPCR experiments were carried out three or four times in independent experiments with triplicate replicates. Phagocytosis experiments were carried out between four and seven times in biologically independent experiments with triplicate replicates. Statistical significance for ELISAs was determined by running a one-way ANOVA with a post hoc Student–Newman–Keuls (SNK) test. Statistical significance for qPCR experiments was determined by running a one-way ANOVA with a post hoc Dunn’s test.
Results
Characterization of Microglia Culture Purity
To verify the purity of our primary human microglial cultures, cultures of passage 2–3 microglia were incubated with anti-IBA-1 antibody and counterstained with DAPI. Culture purity was determined by dividing the number of cells immunoreactive for IBA-1 staining (microglia) with the total number of nuclei (DAPI). At passage 2, primary human microglial cultures were >97% pure; at passage 3, primary human microglial cultures were >95% pure (Figure 1). No changes in morphological characteristics were observed through the passages.
Differential Chemokine Gene Expression in Human Microglia Cells Stimulated With B. burgdorferi
Primary cultures of human microglia cells were stimulated with virulent, non-virulent, and antibiotic killed B. burgdorferi for 24–72 h. RNA was extracted from stimulated and control cells, cDNA synthesized for real-time PCR array analysis. There was very little variation between technical replicates across the array, with standard deviations averaging less than 1 threshold cycle (data not shown). Several genes were differentially upregulated more than threefold by non-virulent B. burgdorferi, virulent B. burgdorferi, and antibiotic-killed bacteria, including neutrophil chemoattractants (IL-8), growth/differentiation factors (CSF-1, GDF-5, LIF), and cytokines IL-6, IL-15, and VEGF (Table 2).
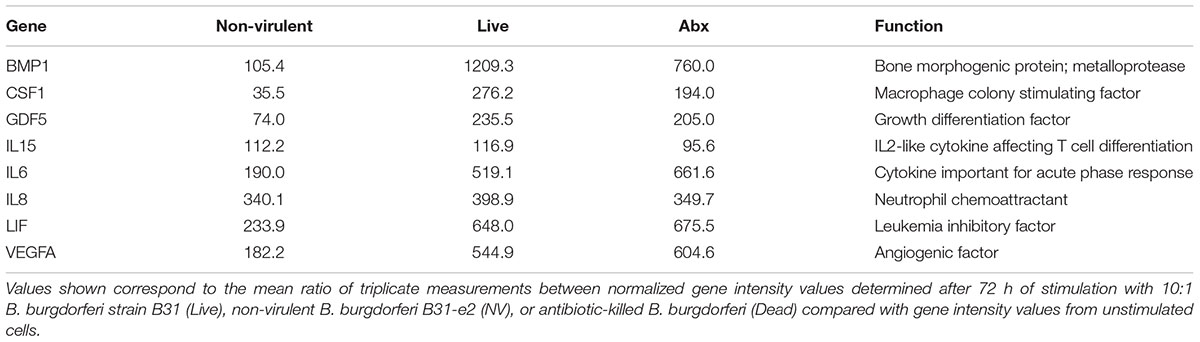
TABLE 2. Transcriptional profiling of chemokine gene expression in microglial cells upon stimulation with B. burgdorferi.
Transcription of selected genes from the PCR array were confirmed at 24 and 72 h after stimulation with live virulent, non-virulent, antibiotic-killed, and antibiotic-killed sonicated B. burgdorferi. A similar trend was observed for several transcripts at 24 h, including IL-6, IL-8, IL-15, LIF, and VEGF (Figure 2).
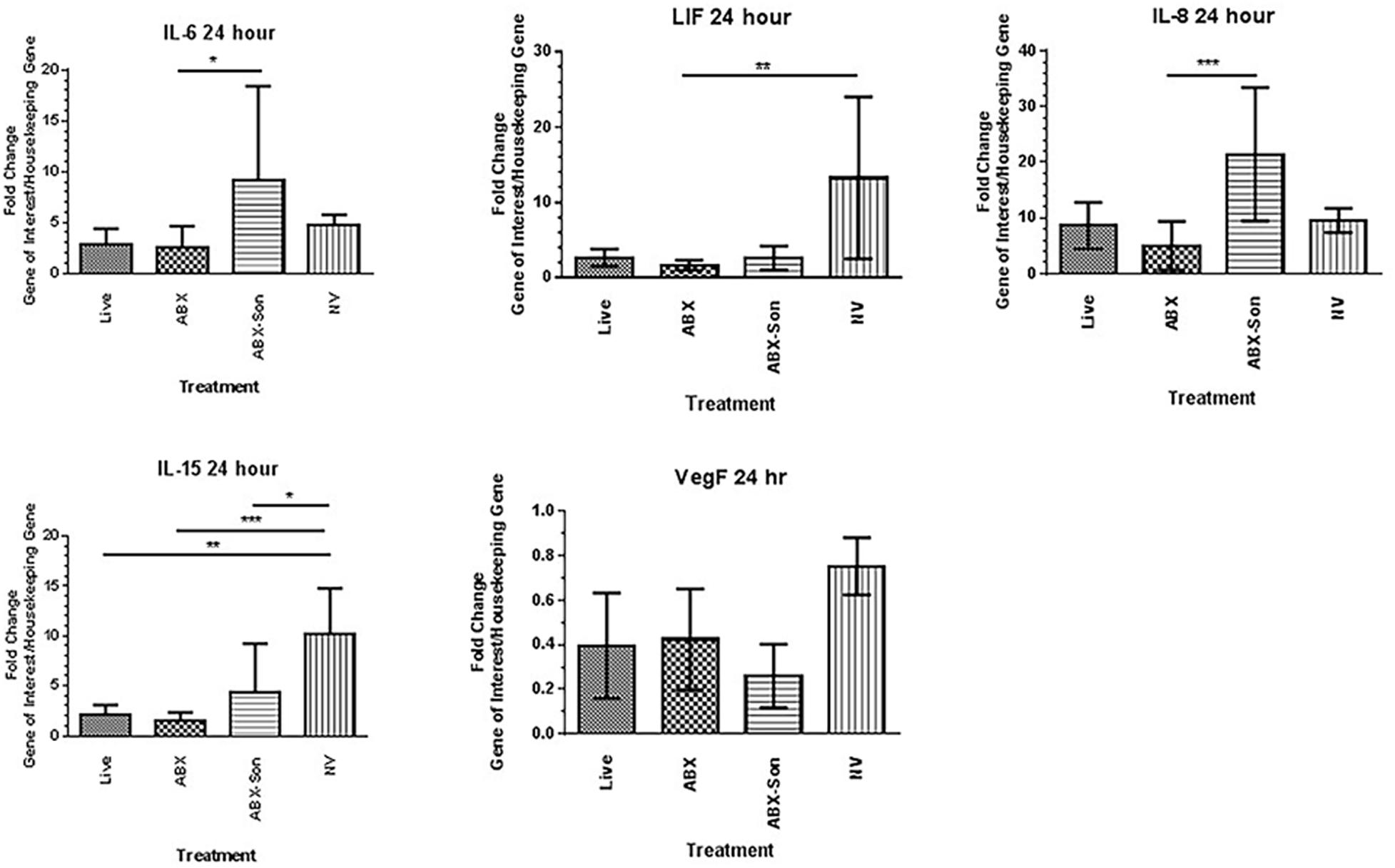
FIGURE 2. Validation of differentially regulated genes by qPCR array analysis. Primary human microglia were stimulated with live virulent (V), non-virulent (NV), antibiotic-killed (Abx), or antibiotic-treated and sonicated (Abx/Son) B. burgdorferi for 24 or 72 h. Data are representative of two biological replicates per time point, with each PCR reaction run in triplicate. Data were normalized to transcription levels of two independent housekeeping genes and are expressed as fold change versus no spirochete control. Error bars represent SEM. Statistical significance for qPCR experiments was determined by running a one-way ANOVA with a post hoc Dunn’s test (∗p < 0.05, ∗∗p < 0.01, ∗∗∗p < 0.001).
To determine if these transcriptional changes were biologically relevant, we measured the production of cytokines, chemokines, and neurotrophins secreted from microglia stimulated with live virulent, non-virulent, and antibiotic-killed and antibiotic-killed sonicated spirochetes for 24–72 h. We quantified supernatant protein concentrations of IL-6, IL-8, LIF, CXCL-1, and CXCL-10 by ELISA. Our results demonstrate elevated protein levels for all six proteins examined when compared to non-treatment controls with the highest concentrations of protein associated with the antibiotic-treated and sonicated B. burgdorferi. Chemokines IL-8, CXCL-1, and CXCL-10 and the cytokine IL-6 were generally secreted in a temporal pattern, with highest secretion occurring 72 h after cell stimulation when compared to the 24 h time point (Figure 3).
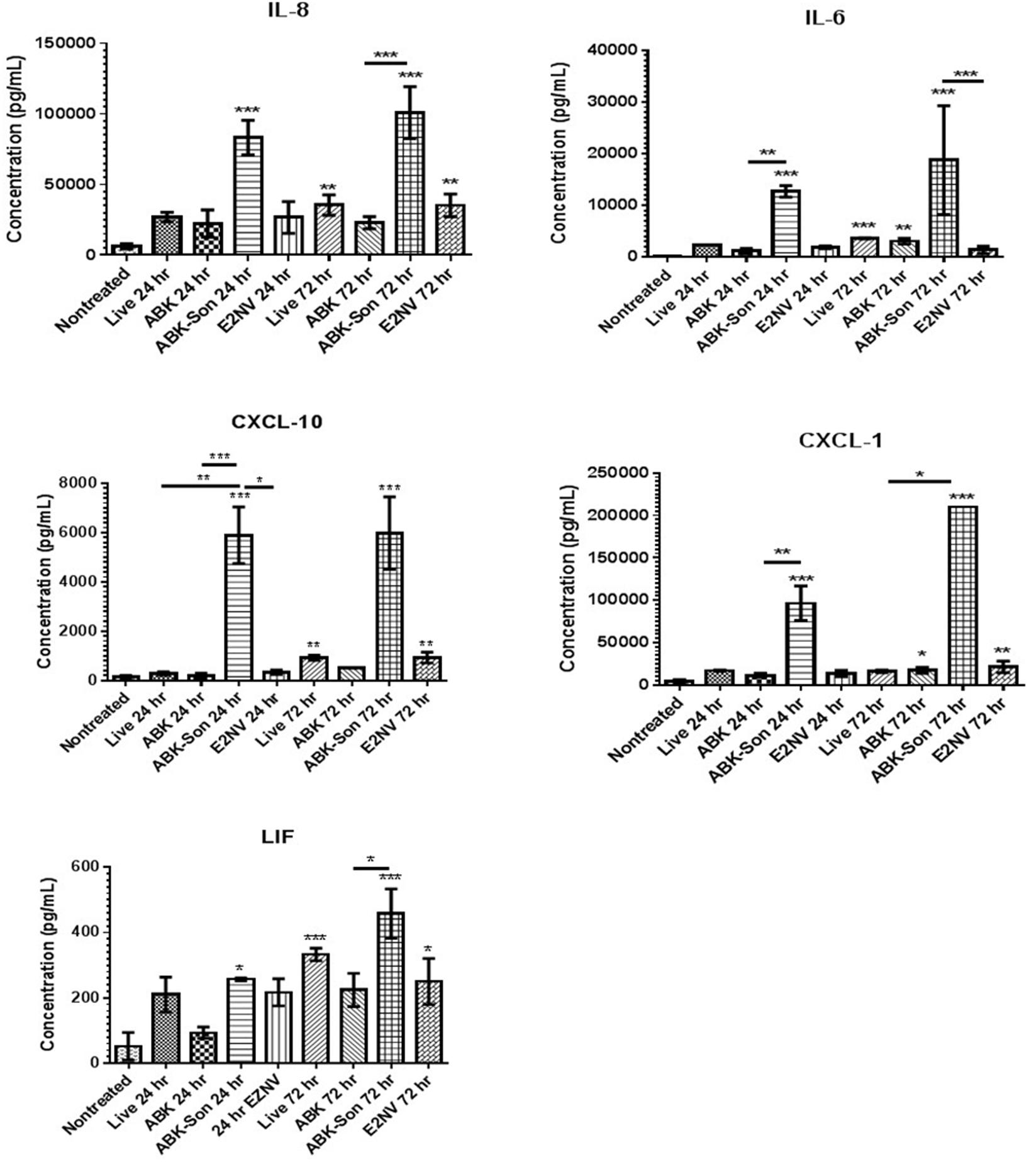
FIGURE 3. B. burgdorferi-stimulated microglia protein expression. Primary human microglia were stimulated with live virulent, non-virulent, antibiotic-killed or antibiotic-treated and sonicated B. burgdorferi for 24 or 72 h as described in “Materials and Methods.” Cytokine and chemokine concentrations were measured using ELISA analysis (R&D Systems). Data represent two biological replicates per time point, with each sample run in triplicate. Error bars represent SD. Statistical significance for ELISAs was determined by running a one-way ANOVA with a post hoc Student–Newman–Keuls (SNK) test (∗p < 0.05, ∗∗p < 0.01, ∗∗∗p < 0.001).
Differential Phagocytosis-Related Gene Expression in Human Microglia Cells in Response to B. burgdorferi
Primary cultures of human microglia cells were stimulated with exponential phase live, antibiotic-killed and antibiotic-killed sonicated spirochetes for 24 h. Again, we utilized commercial qPCR arrays to quantify the transcription of a panel of human phagocytosis-related genes. Surprisingly, no genes were upregulated when stimulated with live B. burgdorferi (data not shown). Because antibiotic-killed B. burgdorferi had elicited robust chemokine expression, we investigated whether primary human microglia would respond to dead B. burgdorferi. Bacteria were either killed with antibiotics or killed with antibiotics and disrupted by sonication. In contrast to live B. burgdorferi, several genes were upregulated more than threefold by dead B. burgdorferi, including scavenger receptors (MARCO, SCARB1), PLAs (PLA2, PLD2), and pattern recognition receptors (CD14, TLR3) (Table 3).
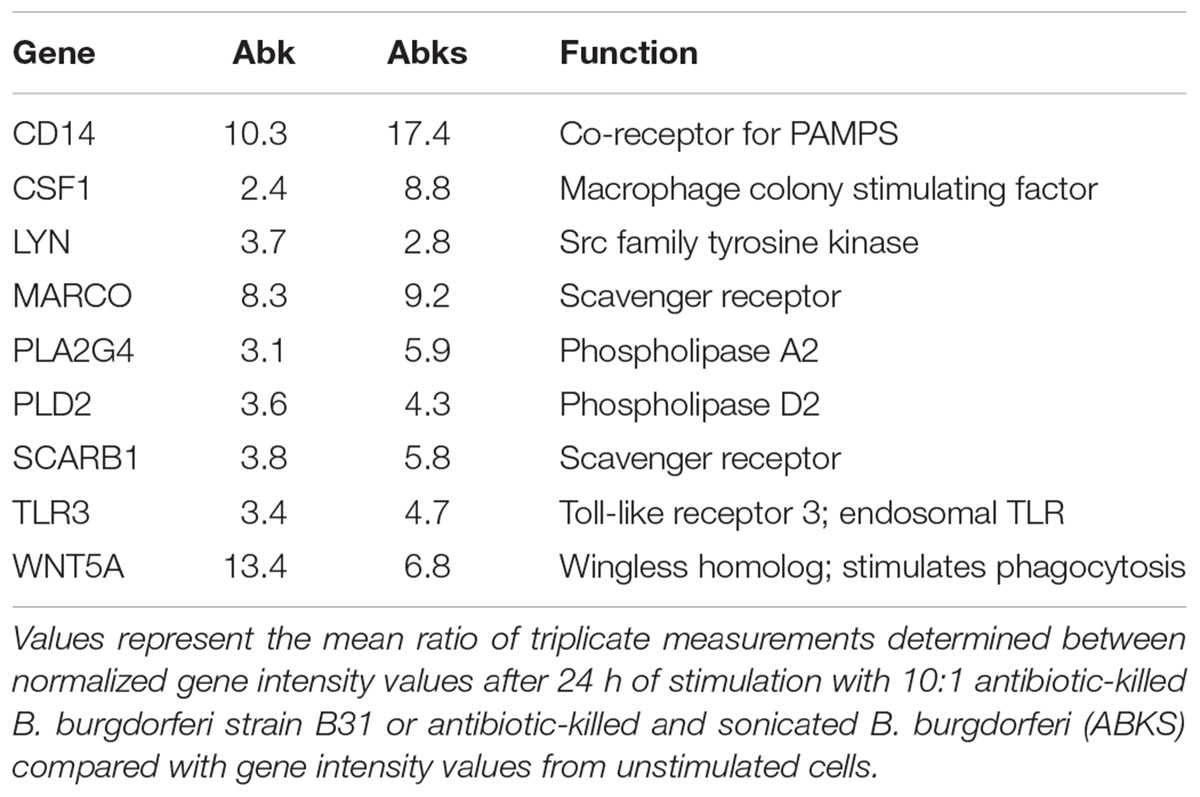
TABLE 3. Transcriptional profiling of phagocytosis-related gene expression in microglial cells in response to B. burgdorferi.
We then validated the expression of selected differentially regulated genes by qPCR array analysis. The mRNA expression of selected genes from the phagocytosis array was analyzed at 24 h after B. burgdorferi stimulation. Transcriptional induction by antibiotic-killed and antibiotic-killed sonicated was observed by qRT-PCR and that seen in the commercial real-time PCR arrays for several transcripts, including MARCO and CD14 (Figure 4). In contrast to the array results, live B. burgdorferi also upregulated several of these transcripts, including CD14 and MARCO. No significant differences were seen between live and antibiotic-killed spirochete induction of transcripts, with the exception of TLR3.
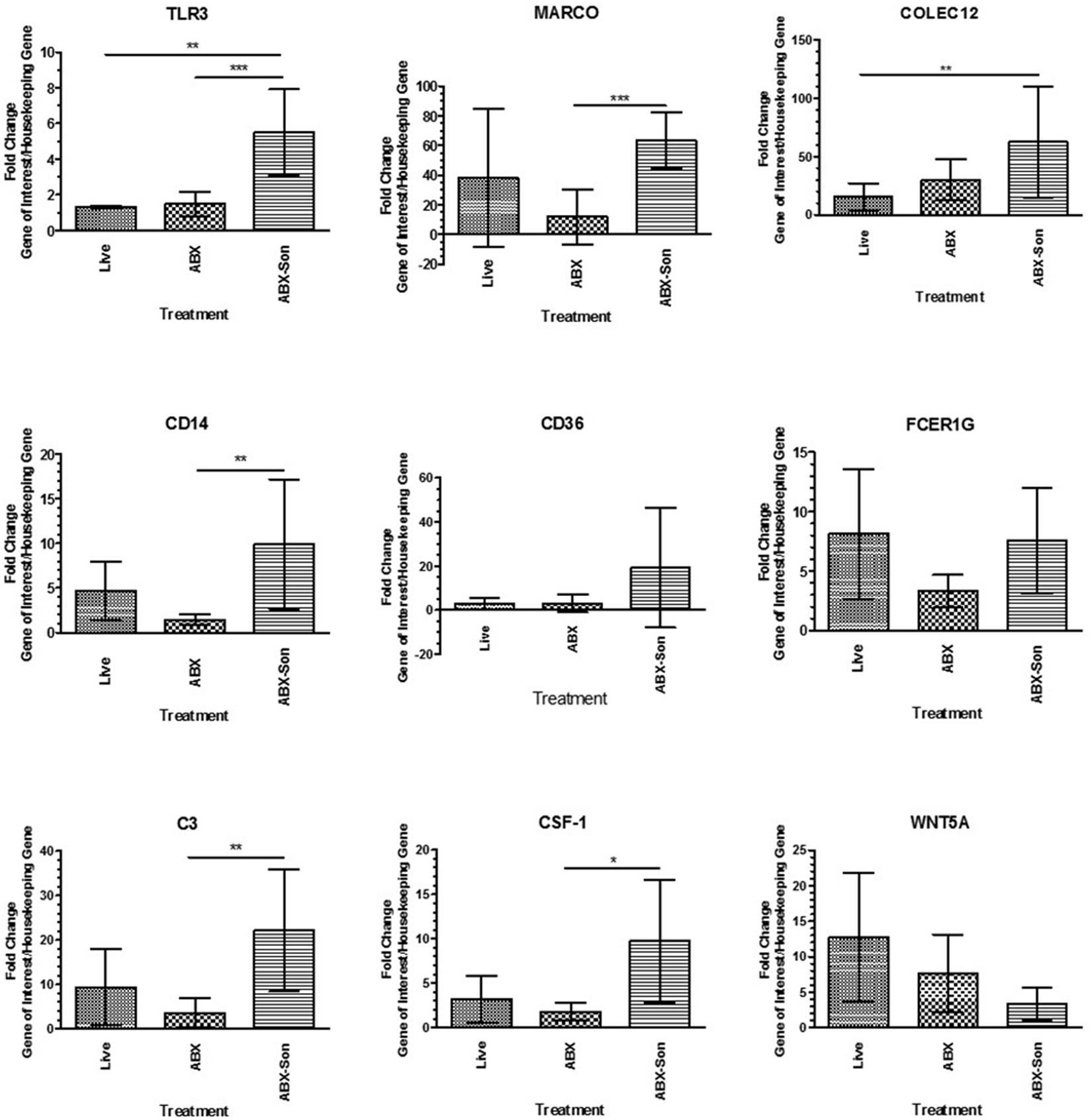
FIGURE 4. Validation of selected phagocytosis-related gene expression. Primary human microglia were stimulated with live virulent (V), non-virulent (NV), antibiotic-killed (Abx), or antibiotic-treated and sonicated (Abx/Son) B. burgdorferi for 24 h followed by RNA purification and cDNA synthesis. Individual primer sets (SABiosciences) were used to amplify transcripts of interest by qPCR. Data represent two biological replicates per time point, with each PCR reaction run in triplicate. Data were normalized to transcription levels of two independent housekeeping genes and are expressed as fold change compared to no spirochete control. Error bars represent SEM. Statistical significance for qPCR experiments was determined by running a one-way ANOVA with a post hoc Dunn’s test (∗p < 0.05, ∗∗p < 0.01, ∗∗∗p < 0.001).
Confocal Microscopic Analysis of Microglial Phagocytosis of B. burgdorferi
In order to confirm whether the results of commercial phagocytosis arrays were the result of internalization or solely the aftermath of cell-surface receptor cascades, confocal microscopy was used to visualize B. burgdorferi in microglia cells. Primary cultures of human microglia were left un-stimulated, stimulated with green fluorescent control beads, or stimulated for 24 h with fluorescent gfp-expressing B. burgdorferi. After fixation, cells were stained with Texas Red 594 for IBA-1. Images of non-treated control cells demonstrate Texas Red 594 bound to IBA-1 without the co-localization of gfp-expressing B. burgdorferi (Figure 5). Fluorescent beads are useful probes for measuring phagocytic response among cells. Indeed, our cultures of primary human microglia are capable of internalizing or engulfing fluorescent control beads and confocal analysis of microglia treated with live B. burgdorferi suggested interaction on the cell surface in addition to internalization as well (Figure 5).
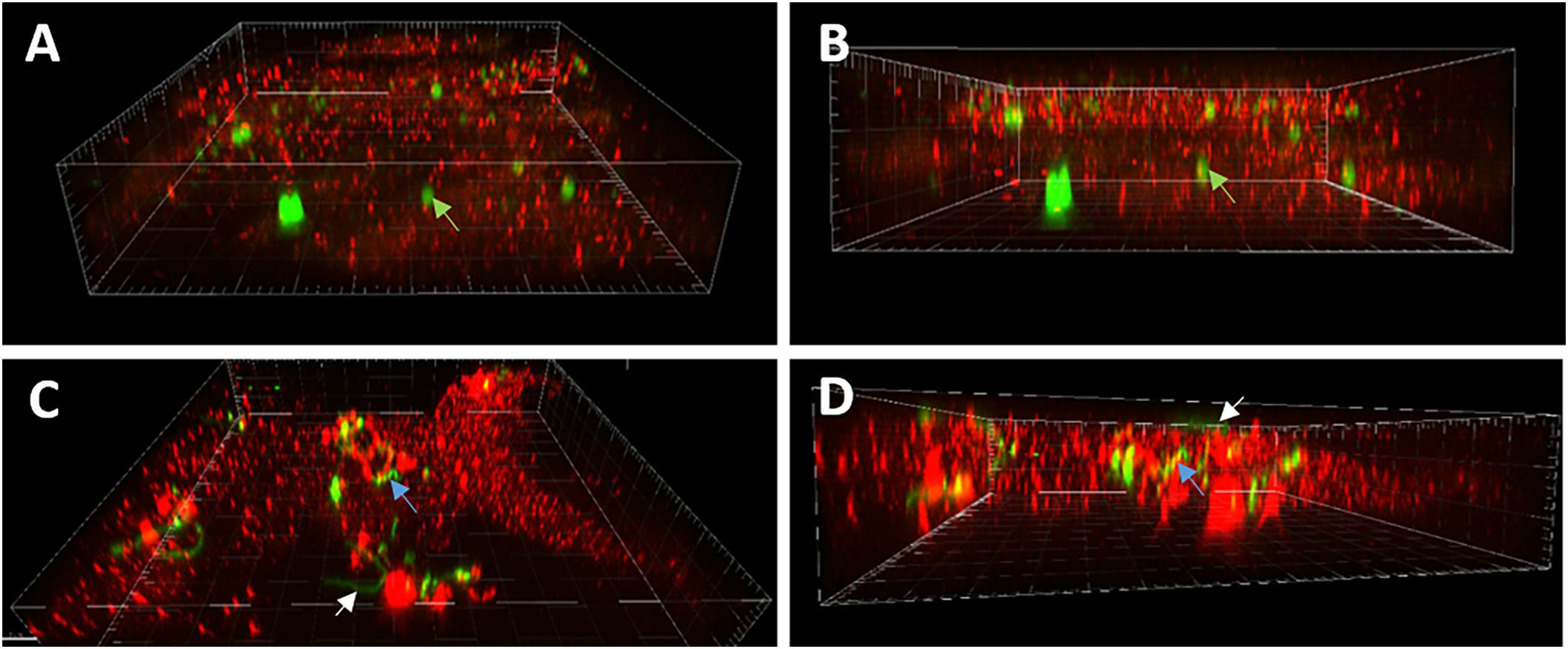
FIGURE 5. Confocal microscopy of B. burgdorferi interactions with primary human microglia. (A) Oblique view showing 1 μM green fluorescent beads associated with microglial cell (green arrows, 60X). (B) Demonstrates internalization of beads. Green arrows denote internalized bead in (A,B). (C) White arrows illustrate intact spirochete associated with surface of microglial cell with no indication of internalization. (D) Blue arrows demonstrate internalization of Borrelia particles (C,D) suggesting internalization is associated with prior bacterial cell death.
Discussion
Previous work on murine microglia and B. burgdorferi indicates that both live B. burgdorferi as well as spirochetal antigen induces IL-6 and TNF-α secretion from these cells. Furthermore, B. burgdorferi antigen induces increased expression of several innate immune molecules and signaling factors such as NOD2, TLR2, and CD14 by murine microglia (Rasley et al., 2002; Sterka and Marriott, 2006; Sterka et al., 2006; Tauber et al., 2011). Likewise, after 24 h of incubation with live B. burgdorferi, primary rhesus microglia respond with increased transcription of chemokines and cytokines including IL-6 and IL-8. Among other products, primary rhesus microglia elaborate the proteins IL-6 and IL-8 (Myers et al., 2009; Ramesh et al., 2009). As with murine microglia, TLRs and their signaling pathways are involved in the response of rhesus microglia to B. burgdorferi (Bernardino et al., 2008; Parthasarathy and Philipp, 2013). Taken together, previous studies characterizing the response of both murine and primary rhesus microglia to B. burgdorferi indicate an upregulation of innate immune receptors such as toll like receptors along with the secretion of chemokines and cytokines including IL-6, IL-8, and TNF-α.
Our results demonstrate the robust transcription and protein secretion of both chemotaxic and potentially neuroinflammatory proteins by primary human microglial cells in response to B. burgdorferi in vitro, consistent with previous studies in mouse and monkey microglia (Rasley et al., 2002; Myers et al., 2009). CXCL-1 and IL-8 (potent neutrophil chemoattractants) were among the most upregulated, although secretion of CXCL-10 (T cell chemoattractant) and IL-6 were also observed. IL-6 can play both inflammatory and neurotrophic roles in CNS disorders (Suzuki et al., 2009), and whether it potentiates B. burgdorferi-induced damage or protects neurons in Lyme neuroborreliosis is unknown. The strongest protein response was observed in antibiotic-killed and sonicated spirochete treated cultures indicating that elevated protein expression can occur in response to dead or viable B. burgdorferi. These data suggest even after antibiotic killing, B. burgdorferi debris and dead bacteria could potentiate an inflammatory response in the absence of live organisms. This concept is supported by work by Parthasarathy et al. (2013) demonstrating inflammatory responses to non-viable B. burgdorferi in human oligodendrocytes, as well as studies in which inflammation remained after antibiotic treatment of B. burgdorferi-infected rhesus macaques (Parthasarathy et al., 2013; Crossland et al., 2017; Embers et al., 2017).
We also saw increased transcription and protein secretion of growth factors and neuroprotective factors including LIF. LIF is a neurotrophic cytokine that shares a receptor subunit with IL-6 and ciliary neurotrophic factor (Suzuki et al., 2009), and was recently shown to be required to prevent the propagation of secondary neurodegeneration after brain injury (Goodus et al., 2016). Microglial activation can be beneficial or detrimental, depending on the balance of pro-inflammatory factors, anti-inflammatory molecules, neurotrophins, and the magnitude of response. Factors involved in promoting glial inflammation in response to infection and injury include more than the usual suspects (e.g., inflammatory cytokines). Indeed, recent work supports roles for the neuropeptide substance P in inflammatory CNS disorders, including infection with B. burgdorferi (Chauhan et al., 2008; Johnson et al., 2016; Burmeister et al., 2017; Martinez et al., 2017). In light of recent work on the connection of glia to persistent pain (Loggia et al., 2015; Ji et al., 2016), the contribution of neurotrophins, growth factors, and neurotransmitters to cellular responses to B. burgdorferi may offer clues to chronic pain often reported by patients with post-treatment Lyme sequelae. Interestingly, we saw upregulation of CSF-1 in response to B. burgdorferi, and this factor has been recently linked to microglia proliferation (Guan et al., 2016).
We also investigated whether primary human microglia could effectively phagocytize B. burgdorferi. Phagocytosis of B. burgdorferi can occur via one of three mechanisms: opsonic mediated phagocytosis (through interaction with complement receptors), conventional phagocytosis mediated by integrins and C-type lectins, or coiling phagocytosis, which involves filopodial protrusion and actin rearrangement (reviewed in Cervantes et al., 2014). Many of the factors involved in phagocytosis of B. burgdorferi were substantially upregulated in primary human microglia, such as CD14 and MARCO, but only in the presence of dead spirochetes. These results were somewhat surprising, as murine microglia incubated with live B. burgdorferi can efficiently phagocytize and kill the bacteria, even in the absence of opsonizing antibodies (Kuhlow et al., 2005). The cell surface component CD14 is key to the recognition of bacteria such as B. burgdorferi. More specifically, CD14 has been shown to cooperate with complement receptor 3 in promoting phagocytosis of B. burgdorferi in both murine macrophages and human monocytes (Hawley et al., 2012). The scavenger receptor MARCO is upregulated in response to B. burgdorferi in murine macrophages, and the lack of this receptor decreases the ability of these cells to internalize B. burgdorferi (Petnicki-Ocwieja et al., 2013). In the only other published report investigating responses of human microglia to B. burgdorferi, cells stimulated with B. burgdorferi lysate also upregulate transcription of MARCO (Cassiani-Ingoni et al., 2006).
Consistent with these results, we saw increased expression of MARCO only in antibiotic-killed and antibiotic-killed sonicated B. burgdorferi-treated cells. MARCO and other scavenger receptors may play a role in Alzheimer’s and other neurodegenerative diseases, where the receptors facilitate uptake of amyloid beta and induce an inflammatory response that could contribute to microglial neurotoxicity (Yu and Ye, 2015). Future experiments are required to confirm the expression of MARCO in microglia in the rhesus macaque model of neuroborreliosis, and its function in B. burgdorferi clearance in non-murine models.
Conclusion
We have demonstrated that primary human microglia treated with B. burgdorferi experience increased expression of pattern recognition receptors and genes known to be involved with cytoskeletal rearrangement and phagocytosis, in addition to the expected increased expression as well as secretion of pro-inflammatory molecules and neurotrophic factors. Our data also indicate that B. burgdorferi interacts with the cell surface of primary human microglia and may be internalized following this initial interaction. Our futures studies will use scanning and transmission electron microscopy to gather high-resolution images of the cell surface interacting with B. burgdorferi to confirm B. burgdorferi internalization by human microglia.
Author Contributions
JG performed the experiments and this work represents a portion of his UND Undergraduate Honors Thesis. RG provided training for JG and assisted in the execution of the experiments. CB contributed to the training of JG and RG, as well as the design and conception of the experiments, statistical analysis, and preparation of the manuscript. JW also contributed to the training of JG and the conception, design, and execution of the experiments and preparation of the manuscript.
Funding
This work was supported in part by the National Institutes of Health grant P20GM113123-01, UND Faculty Seed Grants to CB through grant 21418-4010-02501 and 21418-4010-02403 to JW, and ND EPSCoR funds to CB through grant UND0014140.
Conflict of Interest Statement
The authors declare that the research was conducted in the absence of any commercial or financial relationships that could be construed as a potential conflict of interest.
Acknowledgments
The authors thank members of the Brissette and Watt laboratories for helpful discussion and critical reading and evaluation of the manuscript.
Abbreviations
CCL-5, chemokine C-C motif ligand; CD-14, cell differentiation factor 14; CNS, central nervous system; CSF, cerebral spinal fluid; CSF-1, macrophage colony stimulating factor-1; CXCL, chemokine C-X-C motif; ELISA, enzyme linked immunosorbent assay; GDF-5, growth differentiation factor-5; gfp, green fluorescent protein; IL-8, interleukin 8; LIF, leukemia inhibitory factor; MARCO, macrophage receptor with collagenous structure; MOI, multiplicity of infection; PBS, phosphate buffered saline; PCR, polymerase chain reaction; PLA, phospholipase; PTLDS, post-treatment Lyme disease syndrome; SCARB1, human scavenger receptor class B; TLR, toll-like receptor; VEGF, vascular epithelial growth factor.
References
Babb, K., McAlister, J. D., Miller, J. C., and Stevenson, B. (2004). Molecular characterization of Borrelia burgdorferi erp promoter/operator elements. J. Bacteriol. 186, 2745–2756. doi: 10.1128/JB.186.9.2745-2756.2004
Back, T., Grunig, S., Winter, Y., Bodechtel, U., Guthke, K., Khati, D., et al. (2013). Neuroborreliosis-associated cerebral vasculitis: long-term outcome and health-related quality of life. J. Neurol. 260, 1569–1575. doi: 10.1007/s00415-013-6831-4
Bernardino, A. L., Myers, T. A., Alvarez, X., Hasegawa, A., and Philipp, M. T. (2008). Toll-like receptors: insights into their possible role in the pathogenesis of Lyme neuroborreliosis. Infect. Immun. 76, 4385–4395. doi: 10.1128/IAI.00394-08
Bertrand, E., Szpak, G. M., Pilkowska, E., Habib, N., Lipczynska-Lojkowska, W., Rudnicka, A., et al. (1999). Central nervous system infection caused by Borrelia burgdorferi. Clinico-pathological correlation of three post-mortem cases. Folia Neuropathol. 37, 43–51.
Blaylock, R. L. (2013). Immunology primer for neurosurgeons and neurologists part 2: innate brain immunity. Surg. Neurol. Int. 4:118. doi: 10.4103/2152-7806.118349
Bockenstedt, L. K., Gonzalez, D. G., Haberman, A. M., and Belperron, A. A. (2012). Spirochete antigens persist near cartilage after murine Lyme borreliosis therapy. J. Clin. Invest. 122, 2652–2660. doi: 10.1172/JCI58813
Burmeister, A. R., Johnson, M. B., Chauhan, V. S., Moerdyk-Schauwecker, M. J., Young, A. D., Cooley, I. D., et al. (2017). Human microglia and astrocytes constitutively express the neurokinin-1 receptor and functionally respond to substance P. J. Neuroinflammation 14:245. doi: 10.1186/s12974-017-1012-5
Casjens, S., Palmer, N., van Vugt, R., Huang, W. M., Stevenson, B., Rosa, P., et al. (2000). A bacterial genome in flux: the twelve linear and nine circular extrachromosomal DNAs of an infectious isolate of the Lyme disease spirochete Borrelia burgdorferi. Mol. Microbiol. 35, 490–516. doi: 10.1046/j.1365-2958.2000.01698.x
Cassiani-Ingoni, R., Cabral, E. S., Lunemann, J. D., Garza, Z., Magnus, T., Gelderblom, H., et al. (2006). Borrelia burgdorferi Induces TLR1 and TLR2 in human microglia and peripheral blood monocytes but differentially regulates HLA-class II expression. J. Neuropathol. Exp. Neurol. 65, 540–548. doi: 10.1097/00005072-200606000-00002
Cervantes, J. L., Hawley, K. L., Benjamin, S. J., Weinerman, B., Luu, S. M., and Salazar, J. C. (2014). Phagosomal TLR signaling upon Borrelia burgdorferi infection. Front. Cell. Infect. Microbiol. 4:55. doi: 10.3389/fcimb.2014.00055
Chauhan, V. S., Sterka, D. G. Jr., Gray, D. L., Bost, K. L., and Marriott, I. (2008). Neurogenic exacerbation of microglial and astrocyte responses to Neisseria meningitidis and Borrelia burgdorferi. J. Immunol. 180, 8241–8249. doi: 10.4049/jimmunol.180.12.8241
Crossland, N. A., Alvarez, X., and Embers, M. E. (2017). Late disseminated Lyme disease: associated pathology and spirochete persistence post-treatment in rhesus macaques. Am. J. Pathol. 188, 672–682. doi: 10.1016/j.ajpath.2017.11.005
Embers, M. E., Barthold, S. W., Borda, J. T., Bowers, L., Doyle, L., Hodzic, E., et al. (2012). Persistence of Borrelia burgdorferi in rhesus macaques following antibiotic treatment of disseminated infection. PLoS One 7:e29914. doi: 10.1371/journal.pone.0029914
Embers, M. E., Hasenkampf, N. R., Jacobs, M. B., Tardo, A. C., Doyle-Meyers, L. A., Philipp, M. T., et al. (2017). Variable manifestations, diverse seroreactivity and post-treatment persistence in non-human primates exposed to Borrelia burgdorferi by tick feeding. PLoS One 12:e0189071. doi: 10.1371/journal.pone.0189071
Fallon, B. A., Keilp, J. G., Corbera, K. M., Petkova, E., Britton, C. B., Dwyer, E., et al. (2008). A randomized, placebo-controlled trial of repeated IV antibiotic therapy for Lyme encephalopathy. Neurology 70, 992–1003. doi: 10.1212/01.WNL.0000284604.61160.2d
Fallon, B. A., Levin, E. S., Schweitzer, P. J., and Hardesty, D. (2010). Inflammation and central nervous system Lyme disease. Neurobiol. Dis. 37, 534–541. doi: 10.1016/j.nbd.2009.11.016
Fallon, B. A., and Nields, J. A. (1994). Lyme disease: a neuropsychiatric illness. Am. J. Psychiatry 151, 1571–1583. doi: 10.1176/ajp.151.11.1571
Fraser, C. M., Casjens, S., Huang, W. M., Sutton, G. G., Clayton, R., Lathigra, R., et al. (1997). Genomic sequence of a Lyme disease spirochaete, Borrelia burgdorferi. Nature 390, 580–586. doi: 10.1038/37551
Goodus, M. T., Kerr, N. A., Talwar, R., Buziashvili, D., Fragale, J. E., Pang, K. C., et al. (2016). Leukemia inhibitory factor haplodeficiency desynchronizes glial reactivity and exacerbates damage and functional deficits after a concussive brain injury. J. Neurotrauma 33, 1522–1534. doi: 10.1089/neu.2015.4234
Guan, Z., Kuhn, J. A., Wang, X., Colquitt, B., Solorzano, C., Vaman, S., et al. (2016). Injured sensory neuron-derived CSF1 induces microglial proliferation and DAP12-dependent pain. Nat. Neurosci. 19, 94–101. doi: 10.1038/nn.4189
Gustaw, K., Beltowska, K., and Studzinska, M. M. (2001). Neurological and psychological symptoms after the severe acute neuroborreliosis. Ann. Agric. Environ. Med. 8, 91–94.
Halperin, J. J. (2015). Nervous system Lyme disease. Clin. Lab. Med. 35, 779–795. doi: 10.1016/j.cll.2015.07.002
Hawley, K. L., Olson, C. M. Jr., Iglesias-Pedraz, J. M., Navasa, N., Cervantes, J. L., and Caimano, M. J. (2012). CD14 cooperates with complement receptor 3 to mediate MyD88-independent phagocytosis of Borrelia burgdorferi. Proc. Natl. Acad. Sci. U.S.A. 109, 1228–1232. doi: 10.1073/pnas.1112078109
Ji, R. R., Chamessian, A., and Zhang, Y. Q. (2016). Pain regulation by non-neuronal cells and inflammation. Science 354, 572–577. doi: 10.1126/science.aaf8924
Johnson, M. B., Young, A. D., and Marriott, I. (2016). The therapeutic potential of targeting substance P/NK-1R interactions in inflammatory CNS disorders. Front. Cell. Neurosci. 10:296. doi: 10.3389/fncel.2016.00296
Kuhlow, C. J., Garcia-Monco, J. C., Coleman, J. L., and Benach, J. L. (2005). Murine microglia are effective phagocytes for Borrelia burgdorferi. J. Neurimmunol. 168, 183–187. doi: 10.1016/j.jneuroim.2005.06.030
Lee, H., Lee, S., Cho, I. H., and Lee, S. J. (2013). Toll-like receptors: sensor molecules for detecting damage to the nervous system. Curr. Protein Pept. Sci. 14, 33–42. doi: 10.2174/1389203711314010006
Ljostad, U., and Mygland, A. (2010). Remaining complaints 1 year after treatment for acute Lyme neuroborreliosis; frequency, pattern and risk factors. Eur. J. Neurol. 17, 118–123. doi: 10.1111/j.1468-1331.2009.02756.x
Loggia, M. L., Chonde, D. B., Akeju, O., Arabasz, G., Catana, C., Edwards, R. R., et al. (2015). Evidence for brain glial activation in chronic pain patients. Brain 138(Pt 3), 604–615. doi: 10.1093/brain/awu377
Martinez, A. N., Burmeister, A. R., Ramesh, G., Marriott, I., and Philipp, M. T. (2017). Aprepitant limits in vivo neuroinflammatory responses in a rhesus model of Lyme neuroborreliosis. J. Neuroinflammation 14:37. doi: 10.1186/s12974-017-0813-x
Miller, J. C., von Lackum, K., Babb, K., McAlister, J. D., and Stevenson, B. (2003). Temporal analysis of Borrelia burgdorferi Erp protein expression throughout the mammal-tick infectious cycle. Infect. Immun. 71, 6943–6952. doi: 10.1128/IAI.71.12.6943-6952.2003
Myers, T. A., Kaushal, D., and Philipp, M. T. (2009). Microglia are mediators of Borrelia burgdorferi-induced apoptosis in SH-SY5Y neuronal cells. PLoS Pathog. 5:e1000659. doi: 10.1371/journal.ppat.1000659
Mygland, A., Ljostad, U., Fingerle, V., Rupprecht, T., Schmutzhard, E., and Steiner, I. (2010). EFNS guidelines on the diagnosis and management of European Lyme neuroborreliosis. Eur. J. Neurol. 8–16. doi: 10.1111/j.1468-1331.2009.02862.x
Nanagara, R., Duray, P. H., and Schumacher, H. R. Jr. (1996). Ultrastructural demonstration of spirochetal antigens in synovial fluid and synovial membrane in chronic Lyme disease: possible factors contributing to persistence of organisms. Hum. Pathol. 27, 1025–1034. doi: 10.1016/S0046-8177(96)90279-8
Parthasarathy, G., Fevrier, H. B., and Philipp, M. T. (2013). Non-viable Borrelia burgdorferi induce inflammatory mediators and apoptosis in human oligodendrocytes. Neurosci. Lett. 556, 200–203. doi: 10.1016/j.neulet.2013.10.032
Parthasarathy, G., and Philipp, M. T. (2013). The MEK/ERK pathway is the primary conduit for Borrelia burgdorferi-induced inflammation and P53-mediated apoptosis in oligodendrocytes. Apoptosis 19, 76–89. doi: 10.1007/s10495-013-0913-8
Parthasarathy, G., and Philipp, M. T. (2015). Inflammatory mediator release from primary rhesus microglia in response to Borrelia burgdorferi results from the activation of several receptors and pathways. J. Neuroinflammation 12:60. doi: 10.1186/s12974-015-0274-z
Petnicki-Ocwieja, T., Chung, E., Acosta, D. I., Ramos, L. T., Shin, O. S., Ghosh, S., et al. (2013). TRIF mediates toll-like receptor 2-dependent inflammatory responses to Borrelia burgdorferi. Infect. Immun. 81, 402–410. doi: 10.1128/IAI.00890-12
Ramesh, G., Borda, J. T., Gill, A., Ribka, E. P., Morici, L. A., Mottram, P., et al. (2009). Possible role of glial cells in the onset and progression of Lyme neuroborreliosis. J. Neuroinflammation 6:23. doi: 10.1186/1742-2094-6-23
Ramesh, G., Didier, P. J., England, J. D., Santana-Gould, L., Doyle-Meyers, L. A., Martin, D. S., et al. (2015). Inflammation in the pathogenesis of Lyme neuroborreliosis. Am. J. Pathol. 185, 1344–1360. doi: 10.1016/j.ajpath.2015.01.024
Ramesh, G., Santana-Gould, L., Inglis, F. M., England, J. D., and Philipp, M. T. (2013). The Lyme disease spirochete Borrelia burgdorferi induces inflammation and apoptosis in cells from dorsal root ganglia. J. Neuroinflammation 10:88. doi: 10.1186/1742-2094-10-88
Rasley, A., Anguita, J., and Marriott, I. (2002). Borrelia burgdorferi induces inflammatory mediator production by murine microglia. J. Neuroimmunol. 130, 22–31.
Rupprecht, T. A., Koedel, U., Fingerle, V., and Pfister, H. W. (2008). The pathogenesis of lyme neuroborreliosis: from infection to inflammation. Mol. Med. 14, 205–212.
Schmittgen, T. D., and Livak, K. J. (2008). Analyzing real-time PCR data by the comparative C(T) method. Nat. Protoc. 3, 1101–1108. doi: 10.1038/nprot.2008.73
Steere, A. C., Duray, P. H., and Butcher, E. C. (1988). Spirochetal antigens and lymphoid cell surface markers in Lyme synovitis. Comparison with rheumatoid synovium and tonsillar lymphoid tissue. Arthritis Rheum. 31, 487–495. doi: 10.1002/art.1780310405
Sterka, D. Jr., and Marriott, I. (2006). Characterization of nucleotide-binding oligomerization domain (NOD) protein expression in primary murine microglia. J. Neuroimmunol. 179, 65–75. doi: 10.1016/j.jneuroim.2006.06.009
Sterka, D. Jr., Rati, D. M., and Marriott, I. (2006). Functional expression of NOD2, a novel pattern recognition receptor for bacterial motifs, in primary murine astrocytes. Glia 53, 322–330. doi: 10.1002/glia.20286
Sultan, S. Z., Sekar, P., Zhao, X., Manne, A., Liu, J., Wooten, R. M., et al. (2015). Motor rotation is essential for the formation of the periplasmic flagellar ribbon, cellular morphology, and Borrelia burgdorferi persistence within Ixodes scapularis tick and murine hosts. Infect. Immun. 83, 1765–1777. doi: 10.1128/IAI.03097-14
Sumiya, H., Kobayashi, K., Mizukoshi, C., Aoki, T., Koshino, Y., Taki, J., et al. (1997). Brain perfusion SPECT in Lyme neuroborreliosis. J. Nucl. Med. 38, 1120–1122.
Suzuki, S., Tanaka, K., and Suzuki, N. (2009). Ambivalent aspects of interleukin-6 in cerebral ischemia: inflammatory versus neurotrophic aspects. J. Cereb. Blood Flow Metab. 29, 464–479. doi: 10.1038/jcbfm.2008.141
Tauber, S. C., Ribes, S., Ebert, S., Heinz, T., Fingerle, V., Bunkowski, S., et al. (2011). Long-term intrathecal infusion of outer surface protein C from Borrelia burgdorferi causes axonal damage. J. Neuropathol. Exp. Neurol. 70, 748–757. doi: 10.1097/NEN.0b013e3182289acd
Veinovic, G., Cerar, T., Strle, F., Lotric-Furlan, S., Maraspin, V., Cimperman, J., et al. (2013). In vitro susceptibility of European human Borrelia burgdorferi sensu stricto strains to antimicrobial agents. Int. J. Antimicrob. Agents 41, 288–291. doi: 10.1016/j.ijantimicag.2012.11.016
Wormser, G. P., Nadelman, R. B., and Schwartz, I. (2012). The amber theory of Lyme arthritis: initial description and clinical implications. Clin. Rheumatol. 31, 989–994. doi: 10.1007/s10067-012-1964-x
Yu, Y., and Ye, R. D. (2015). Microglial Abeta receptors in Alzheimer’s disease. Cell. Mol. Neurobiol. 35, 71–83. doi: 10.1007/s10571-014-0101-6
Keywords: Borrelia burgdorferi, microglia, neuroborreliosis, Lyme disease, phagocytosis
Citation: Greenmyer JR, Gaultney RA, Brissette CA and Watt JA (2018) Primary Human Microglia Are Phagocytically Active and Respond to Borrelia burgdorferi With Upregulation of Chemokines and Cytokines. Front. Microbiol. 9:811. doi: 10.3389/fmicb.2018.00811
Received: 19 January 2018; Accepted: 10 April 2018;
Published: 25 April 2018.
Edited by:
Amy Rasley, Lawrence Livermore National Laboratory (DOE), United StatesReviewed by:
Maryam Dadar, Razi Vaccine and Serum Research Institute, IranMarisa Mariel Fernandez, Instituto de Estudios de la Inmunidad Humoral, Argentina
Copyright © 2018 Greenmyer, Gaultney, Brissette and Watt. This is an open-access article distributed under the terms of the Creative Commons Attribution License (CC BY). The use, distribution or reproduction in other forums is permitted, provided the original author(s) and the copyright owner are credited and that the original publication in this journal is cited, in accordance with accepted academic practice. No use, distribution or reproduction is permitted which does not comply with these terms.
*Correspondence: Catherine A. Brissette, catherine.brissette@med.und.edu John A. Watt, john.watt@med.und.edu