- 1Department of Food Biotechnology, Branch for Northwest and West Region, Agricultural Biotechnology Research Institute, Education and Extension Organization (AREEO), Tabriz, Iran
- 2Dietary Supplements and Probiotic Research Center, Alborz University of Medical Sciences, Karaj, Iran
The present study focused on probiotic characterization and safety evaluation of Enterococcus isolates from different artisanal dairy products. All the isolates exhibited inhibitory activity against several food spoilage bacteria and food-borne pathogens, including Shigella flexneri, Staphylococcus aureus, Listeria monocytogenes, Yersinia enterocolitica, Klebsiella pneumoniae, Escherichia coli, and Bacillus subtilis. The PCR results indicated the presence of at least one enterocin structural gene in all the tested strains. The Enterococcus isolates were further evaluated regarding their safety properties and functional features. The isolates were susceptible to vancomycin, gentamycin, and chloramphenicol. The results of PCR amplification revealed that all the tested isolates harbored none of the tested virulence genes except E. faecalis (ES9), which showed the presence of esp gene. The Enterococcus isolates showed cholesterol lowering properties. The selected isolates showed a high tolerance to low pH, and toward bile salts. They also demonstrated hydrophobicity activity, auto-aggregation, and adhesion ability to the human intestinal Caco-2 cell line. These properties may contribute the bacteria colonizing the gut. This study revealed that the Enterococcus isolates, especially E. durans ES11, ES20 and ES32, might be excellent candidates for production of functional foods to promote health benefits.
Introduction
Enterococci are belonging to genera of lactic acid bacteria (LAB). They are Gram-positive, catalase negative, cocci-shaped, facultative anaerobe, and non-spore forming bacteria (Haghshenas et al., 2016). Based on phylogenetic evidence and molecular studies (16S-rDNA sequencing or DNA–DNA hybridization), more than 26 species were classified in this genus. These microorganisms are ubiquitous bacteria which present as common microbiota in the intestine of humans, mammals, and other animals gastrointestinal tracts, but they are also exist in soil, water, vegetable products, meats, fermented and cooked meat and dairy products (Li et al., 2018; Zommiti et al., 2018). This is due to their high tolerance to harsh conditions such as high temperatures, low pH and high salinity. Significant role of E. faecium, E. faecalis, and E. durans in the ripening of traditional cheeses have indicated that enterococci play an important role in the ripening of these cheeses, probably through proteolysis, lipolysis, and citrate breakdown, hence contributing to their typical taste and flavor. A majority of works specify that Enterococcus isolates play a vital role in the development of the sensory properties of fermented foods like olives (they break down oleuropein in fermented olives), sausages and cheese (Moreno et al., 2006).
There are different arrays of probiotics, mostly Lactobacillus and Bifidobacteria groups and Enterococcus genera in recent years, are used in functional foods (Haghshenas et al., 2017). The claimed advantageous of probiotic enterococci are: (i) diarrhea or diarrhea treatment in association with antibiotic medication, viral contaminations, chemotherapy and diseases originated from food-borne pathogens (Lau and Chamberlain, 2016); (ii) curbing the pathogenic bacteria growth (Zorriehzahra et al., 2016); (iii) anti-mutagenic and anti-carcinogenic features; (iv); increased intestinal mucosal barrier (Ahl et al., 2016); (v) stimulation of the immune system (Sheikhi et al., 2016); (vi) prevention of ulcers related to Helicobacter pylori infection (Oh et al., 2016) and (vii) cholesterol assimilation in food and human intestine (Kobyliak et al., 2016). These microorganisms have antagonistic activities through pathogens by different antimicrobial compounds production comprises bacteriocins, lactic and acetic acids and hydrogen peroxide.
However, due to association of some enterococci with human infections, like urinary tract infections, bloodstream infections, bacteraemia, endocarditis and diarrhea and surgical site infections; concerns about the safety of these bacteria have raised the attention of health organizations to use as probiotic bacteria because their virulence aspects contribute in human infections (Brandão et al., 2010; Zommiti et al., 2018). Generally, vancomycin-resistant enterococci (VRE) in nosocomial infections is considered as a major problem (Arias and Murray, 2012). Furthermore, the action of many virulence genes have been elucidated in Enterococcus isolates (Carlos et al., 2010). The most important virulence factors are cylA, cylB and cylM, esp, agg, gelE, cpd, ccf, and cad genes. The gene cylA is responsible for the cytosilin transportation and activation. The genes cylB and cylM have an application in modification of post-translational, while a cell wall protein concerned in the immune evasion is associated to esp gene. Adherence to eukaryotic cells is associated to an aggregation protein which is encoded by agg gene. gelE is responsible for the production of toxin which hydrolyzes gelatin, and finally sex pheromones which are responsible for facilitating conjugation are encoded by cpd, ccf, and cad genes (Belgacem et al., 2010; Liu et al., 2015). The aim of this study was to isolate and identify Enterococcus isolates from traditional dairy products, evaluation of their safety, probiotic aptitudes, and antimicrobial properties due to high potential roles of enterococci in health and food.
Materials and Methods
Sampling and Culture Conditions
Artisanal dairy products (yogurt, cheese, and curd) were collected from domestic producers (Table 1). The samples were transported to the laboratory in ice boxes and stored at 4°C. For better separation of bacteria from solid particles of yogurt and cheese, initial homogenization took place by vortexing. To prepare the bacterial suspension of yogurt, 10 g of yogurt was transferred to 100 mL of sterile physiological peptone water and shaken gently. To prepare the bacterial suspension of cheese and curd, 20 g of each sample were suspended in 180 mL of tri-sodium citrate sterile solution, and after half an hour, 10 mL of prepared solution were added to 200 mL de Man Rogosa Sharpe (MRS) broth in order to enrich and enhance the initial bacterial population in anaerobic conditions and incubated at 37°C for 24 h (Haghshenas et al., 2017).
Isolation of Enterococcus Isolates
Enterococcus isolates were isolated by the streak-plate method on MRS agar and incubated aerobically at 37°C for 24 h. The single colonies were routinely checked for purity by microscopic examination. The pure colonies were used to characterize Gram staining and catalase test. The colonies which were Gram-positive and catalase-negative were selected and inoculated in MRS broth containing 30% glycerol as cryo-protectant and stored at -80°C (El Soda et al., 2003). The purified cultures were activated by sub-culturing twice in MRS broth before use.
Assessment of Probiotic Properties
Acid and Bile Salts Tolerance
To determine acid tolerance, 10 mL of bacterial culture of each sample were incubated for 24 h in MRS broth. Selected colonies were transferred into mineral medium phosphate-buffered saline (PBS, pH 2.5). The samples were incubated aerobically for 3 h at 37°C. Afterward, the cells were diluted up to 10 times using sterile saline (sodium chloride: 5.8 g/L) and each dilution of 100 μL for MRS agar surface in culture medium was cultured. The samples were incubated aerobically for 48–72 h at 37°C (Haghshenas et al., 2015).
Tolerance to bile salts was analyzed based on the method used previously by Nami et al. (2015b). Briefly, MRS broth culture medium, as a control, and MRS with 0.3% bile oxgall, used as a test medium (treatments), were inoculated simultaneously with 1% of active bacterial culture at 37°C for 4 h. Optical densities of the control and treated cultures growth were measured by a spectrophotometer (Eppendorf, Germany) at 600 nm. The percentage of growth suppression was measured by using the following formula:
Antimicrobial Activity and Bacteriocin Detection
Well diffusion method was performed to conclude and recognize the inhibitory metabolites produced by Enterococcus isolates (Nami et al., 2015a). Overnight cultures of the selected isolates were cultured in MRS agar at 37°C for 24 h. Indicator bacteria used in this study were Shigella flexneri PTCC 1234, Staphylococcus aureus ATCC 25923, Listeria monocytogenes ATCC 13932, Yersinia enterocolitica ATCC 23715, Klebsiella pneumoniae PTCC 1053, Escherichia coli PTCC, 1276 and Bacillus subtilis ATCC 19652. These pathogenic organisms were purchased from the Persian Type Culture Collection (PTCC) to detect the antagonistic substances. Half McFarland indicator bacteria (1.5 × 108 CFU/mL) were poured on Mueller-Hinton agar and the wells were cut on plates. Then, each well was filled by 50 μL of filtered supernatant and plates incubated overnight at 37°C and finally, the inhibition zone around the wells was measured by digital calipers.
The proteinaceous nature of the inhibition was assessed. To this end, the active cell-free culture supernatants were obtained by centrifugation at 15000 RPM for 12 min at 4°C. They were subjected to various enzyme treatments, including catalase, trypsin, α-chymotrypsin, and proteinase K, at 1 mg/mL at 37°C for 2 h, after adjusting the pH at 6.2 with 1 M of NaOH. Then, the residual activity was assessed against pathogenic microorganisms. The protease sensitivity was determined by the absence of inhibition zones around the wells. To confirm the presence of hydrogen peroxide, the active supernatants were subjected to sterilized catalase (1 mg/mL) and incubated at 37°C for 2 h and finally their activities were assessed by the well diffusion method.
PCR Amplification of Known Enterocin Genes
All the structural genes concerned to the expression of well-known enterocins EntA, EntB, EntP, EntL50A, EntL50B, Ent31 (Toit et al., 2000), EntQ (Belgacem et al., 2010), and Ent1071 (Omar et al., 2004) were amplified with specific PCR primers (Table 2). PCR amplification was performed at a final volume of 50 μL that comprised of 1 Taq polymerase buffer, 200 μM of dNTP’s, 25 pM of each primer, 2 μL of template DNA (stock) and 1 U of Taq DNA polymerase (Thermo Fisher Scientific, United States). The PCR products were visualized by electrophoresis on 2% agarose gels.
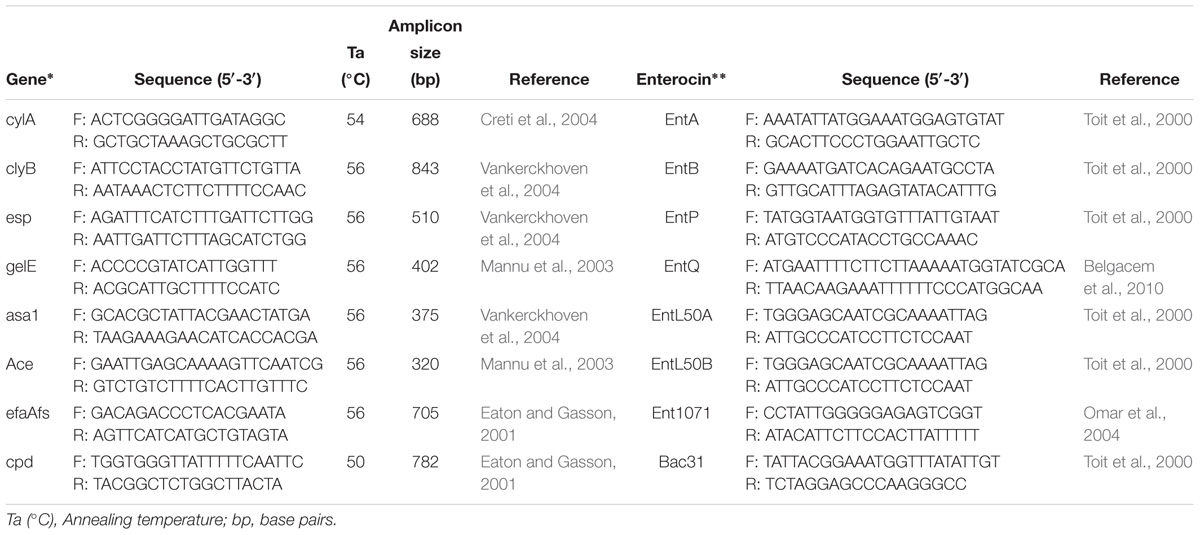
Table 2. Primers used for PCR amplification of virulence factors and enterocin detection genes in Enterococcus strains.
Exopolysaccharide (EPS) Production
The method used by Fguiri et al. (2016) was used for assessment of EPS production ability of isolates. Briefly, the cultures were streaked on m-MRS agar medium which was modified by replacing glucose with 100 g/L of sucrose and incubated at 37°C for 24 h aerobically. Metal loop was used to drag up formed colonies. If the length of slime was above 1.5 mm, the isolate was considered positive slimy producers.
Cell Surface Hydrophobicity
The adhesion ability of isolates to xylene was determined as previously described by Mishra and Prasad (2005).
Auto-Aggregation and Co-Aggregation
The ability of the isolates to auto-aggregate was performed according to the method described by Angmo et al. (2016). Auto-aggregation percentage was determined using the following equation:
Where A0 represents absorbance at t = 0 and at represents absorbance at time t.
Co-aggregation of Enterococcus isolates against the seven pathogens was performed at 37°C after 4 h of incubation based on method used by Zuo et al. (2016). Co-aggregation percentage was calculated based on equation:
Adhesion Ability to Human Intestinal Cells
Adhesion ability to human colon carcinoma cells (Caco-2) was evaluated as reported previously by Nami et al. (2014). Briefly, the Roswell Park Memorial Institute (RPMI-1640; Sigma) medium, supplemented with 10% heat-inactivated fetal bovine serum, was used to culture the human cells. The cells were cultured on 24-well tissue culture plates and incubated at 37°C in 5% CO2 in a relatively humid atmosphere until a confluent monolayer was achieved. The viable Caco-2 cells were counted in a Burker haematocytometer chamber. Then, the cell suspension including bacteria and Caco-2 cells was subjected to pure plate technique to determine C.F.U. bacteria adhesion was expressed as the total number of bacteria attached to viable Caco-2 cells.
Cholesterol Assimilation
Cholesterol removal percentage was determined by o-phthalaldehyde method described by Rudel and Morris (1973) with some alteration. A freshly prepared MRS broth was supplemented with 0.3% oxgall (Merk Germany) as bile salt and water-soluble cholesterol (150 μg/mL) was added as the cholesterol source (sterilized by 0.2 μL filter), the mixture inoculated with each isolate at 1% level and incubated anaerobically at 37°C for 20 h. The cells were removed by centrifugation (10000 rpm for 15 min) after the incubation period; subsequently, 1 mL of the cell-free broth was mixed with 1 mL KOH (33% W/V) and 2 mL ethanol 96%, vortexed for 2 min, followed by heating at 60°C for 15 min. Mixes cooled in room temperature, 2 mL distilled water and 3 mL hexane were added and vortexed for 1 min. One mL the hexane layer was transferred into a glass tube and evaporated in water bath at 80°C. The residue was immediately dissolved in 2 mL o-phthalaldehyde (Merck, Germany) reagent, Followed by 0.5 mL concentrated sulphuric acid and vortexed completely for 1 min. The samples were incubated at room temperature for 30 min and finally absorbance was read at 550 nm.
β-Galactosidase Activity
β-galactosidase activity of Enterococcus isolates was assessed according to Angmo et al. (2016). Bacterial cultures were streaked on MRS agar plates containing 60 μL X-gal (5-bromo-4-chloro-3-indolyl-β-D-galactopyranoside) and 10 μL of IPTG (iso-propyl-thio-β-D-galactopyranoside) solution as inducer. The presence of β-galactosidase activity in strains was determined after 42 h of incubation at 37°C.
Safety Assessment
Hemolytic Activity
Haemolytic activity of Enterococcus isolates was examined by culturing of fresh overnight cultures on Columbia agar plates (Oxoid) containing 7% (v/v) sheep blood (Oxoid) and incubated for 48 h at 37°C. Finally, hemolytic activities were detected by 3 categories: appearance of a halo around the colony: greenish zone considered as α-hemolysis, clear zone for β-hemolysis and no halo for γ-haemolysis (Abedi et al., 2018).
Bile Salts Hydrolysis
The bile salt hydrolysis was evaluated according to Argyri et al. (2013). The hydrolysis activity was indicated by 0.5% (w/v) taurodeoxycholic acid after 48 h of incubation at 37°C. The hydrolysis activity was evaluated by the formation of opaque agar halos around the colonies.
Detection of Virulence Factors
PCR amplification was performed to detect genes encoding potential virulence factors. Total bacterial DNA was isolated by the method described by Leenhouts et al. (1990). Virulence genes evaluated in this study were cylA, clyB, esp, gelE, asa1, Ace, efaAfs, and cpd. E. faecium ATCC 51299 was used as a control. PCR amplification was performed to detect genes encoding these factors using several primers (Table 2). The PCR amplification was carried out in 0.2 mL reaction tubes each with 25 μL of mixtures using 0.1 mM of deoxynucleoside triphosphates, 2.5 mM of MgCl2, 0.5 mM of each primer, PCR Buffer (1X), 2 U of Taq polymerase and 50 ng/μL of DNA template. PCR amplifications were performed with a cycle of initial denaturation (94°C for 5 min), followed by 32 cycles of denaturation (94°C for 60 s), annealing at an appropriate temperature (Table 2) for 60 s and elongation (72°C for 5 min). The PCR products were analyzed by gel electrophoresis in 1.5% agarose stained with ethidium bromide (0.5 g/mL).
Antibiotic Susceptibility
Antibiotic susceptibility test was carried out based on previously described method of Haghshenas et al. (2014). Briefly, disk diffusion assay was performed to determine antibiotic sensitivity of the isolates. MRS agar medium was used for this test. Antimicrobial disks (5 mm) were purchased from Padtan Teb Co, Iran. The tested antibiotics were vancomycin (30 μg), colistin (10 μg), streptomycin (10 μg), cefepime (30 μg), cefixime (15 μg), sulfamethoxazole (2 μg), kanamycin (30 μg), ciprofloxacin (5 μg), tetracycline (30 μg), erythromycin (15 μg), ampicillin (10 μg), gentamycin (10 μg), clindamycin (30 μg), ceftriaxon (30 μg), chloramphenicol (30 μg), and cefalexin (30 μg), using disk diffusion method. After overnight incubation at 37°C, the diameter of inhibition (mm) around each disk was measured.
16S-rDNA Gene Sequencing
Amplification of 16S-rDNA gene (1500 bp) of the isolate was performed by using a pair of lactic acid bacteria (LAB)-specific universal primers (Hal6F/Hal6R) (F: 5′-AGAGTTTGATCMTGGCTCAG-3′ and R: 5′-TACCTTGTTAGGACTTCACC-3′) previously described by Haghshenas et al. (2016). The PCR amplification was fulfilled for the total volume 50 μL under the following conditions: initial denaturation at 94°C for 5 min, followed by 35 cycles of denaturation at 94°C for 45 s, annealing at 59°C for 60 s, extension at 72°C for 90 s, and a final extension step at 72°C for 10 min. The PCR products were visualized through 1% (w/v) agarose gel (Sigma Chemical Co., Poole, United Kingdom) electrophoresis and stained via ethidium bromide. The PCR products were sent to the Macrogen DNA Sequencing Service (Korea) to be sequenced. Multiple sequence alignment of 16S rRNA genes was carried out using CLUSTAL W function (Thompson et al., 1994) with default parameters, and a phylogenetic tree of 16S rRNA genes was reconstructed using the neighbor-joining method (Saitou and Nei, 1987) implemented in the MEGA X software (Kumar et al., 2018) with p-distance parameter distance. Bootstrap values were calculated with 1,000 re-samples. The 16S rRNA gene sequence of Lactobacillus acidophilus (LC064893.1) was used as out-group for the analysis.
Statistical Analysis
Statistical analysis of data was carried out using SPSS (Ver. 19.0 SPSS, Chicago, IL, United States). The comparisons of differences between the means of the treatments were analyzed by one-way ANOVA at a significance level of P < 0.05. All the experiments were performed in triplicate and data expressed as means ± standard deviations.
Results
Characterization and Identification of Isolates
From 90 isolates from various dairy products of different regions of East Azerbaijan Province in Iran, 66 resulted isolates were gram-positive, catalase-negative and rod- or cocci-shaped bacteria. Among them, 32 isolates were cocci-shaped and exhibited optimum growth at 37°C but not at 30°C (data not shown). From 32 cocci-shaped isolates, 8 resulted isolates were from cheese, 8 from curd, and 16 from yogurt (Table 1). Based on morphological and biochemical assays, the authors assumed that these isolates are likely to be Enterococcus strains.
Acid and Bile Salt Tolerance
A stimulated in vitro gastric juice (pH 2.5) was used to assess the acid tolerance profile of Enterococcus isolates (Table 1). The survival rate of isolates displayed a significant variability, ranging from 10.3 to 81.6%. The highest survival rate was observed for isolate ES20 (81.6 ± 0.3%), followed by isolates ES32 (77.4 ± 0.3%) and ES11 (76.3 ± 0.2%), while the lowest survival rate was observed for isolate ES16 with 10.3 ± 0.1% survival rate.
The percentage of viability of Enterococcus isolates was assessed after 4 h of incubation in M-17 broth supplemented with 0.3% oxgall (Table 1). The viability rate of isolates exhibited a significant variability ranging from 09.1 ± 0.1 to 79.8 ± 0.3%. The highest viability rate belonged to isolate ES11 (79.8 ± 0.3%), followed by isolates ES20 (79.1 ± 0.2%) and ES32 (78.2 ± 0.3%), while the lowest viability rate belonged to isolate ES7 (09.1 ± 0.1%). Among the 32 isolates, only isolates 4, 9, 11, 20, 27, 28, and 32 exhibited more than 50% acid and bile tolerance. Hence, these seven isolates were selected for further investigation.
Antimicrobial Activity and Detection of Enterocin Genes
The inhibitory effect of isolated Enterococcus isolates against some important pathogenic microorganisms is shown in Table 3. The results showed that all the seven selected isolates were capable of inhibiting the growth of the majority of target pathogens. The target pathogens used in this study were Shigella flexneri, Staphylococcus aureus, Listeria monocytogenes, Yersinia enterocolitica, Klebsiella pneumoniae, Escherichia coli, and Bacillus subtilis (Table 4). Isolates ES20 and ES28 were able to inhibit the growth of all the tested pathogens. Moreover, isolates ES9, ES11, ES27, and ES32 were able to inhibit the growth of all the target pathogens except Shigella flexneri.
When pH adjusted to 6.2, the isolates ES4, ES9, ES11, and ES27 could not inhibit the growth of any pathogens. Also, the isolates ES20 and ES32 do not able to inhibit the growth of Shigella flexneri, Listeria monocytogenes, Klebsiella pneumoniae, and Bacillus subtilis. So, it resulted that the nature of inhibition of these isolates are because of acid production. Furthermore, after subjecting other isolates to catalase enzyme, none isolates were able to inhibit the growth of indicator pathogens, except isolates ES28 and ES32 against Staphylococcus aureus and Yersinia enterocolitica, respectively. To confirm the nature of inhibition of isolate ES28 against Staphylococcus aureus, and isolate ES32 against Yersinia enterocolitica, protease K enzyme was subjected. After applying this enzyme, the clear zone of inhibition was removed and it showed that the nature of inhibition is because of bacteriocin production.
Extracted DNA of Enterococcus isolates was subjected to PCR amplification to determine the existence of structural genes coding EntA, EntB, EntP, EntL50A, EntL50B, and Ent31 enterocins (Table 3). The PCR results indicated the presence of at least one enterocin structural genes in all the 7 isolates. The enterocins A and B structural genes were detected among all the isolates and the enterocins P, Q and L50A were found in 3 isolates. On the other hand, none of the evaluated isolates showed PCR amplification fragments for other tested enterocins (entL50B, ent1071, and bac31).
Cell Surface Hydrophobicity
The cell surface hydrophobicity rate is illustrated in Figure 1. It ranged from 23.3 ± 1.6 to 58.6 ± 2.3%. The highest cell hydrophobicity rate was observed for isolates ES20, followed by ES11 and ES32 with 58.6 ± 2.3%, 54.2 ± 1.9% and 51.8 ± 1.4, respectively. Furthermore, isolates ES4 and ES27 showed the lowest cell hydrophobicity rates with 23.3 ± 1.6 and 24.7 ± 1.3%, respectively.
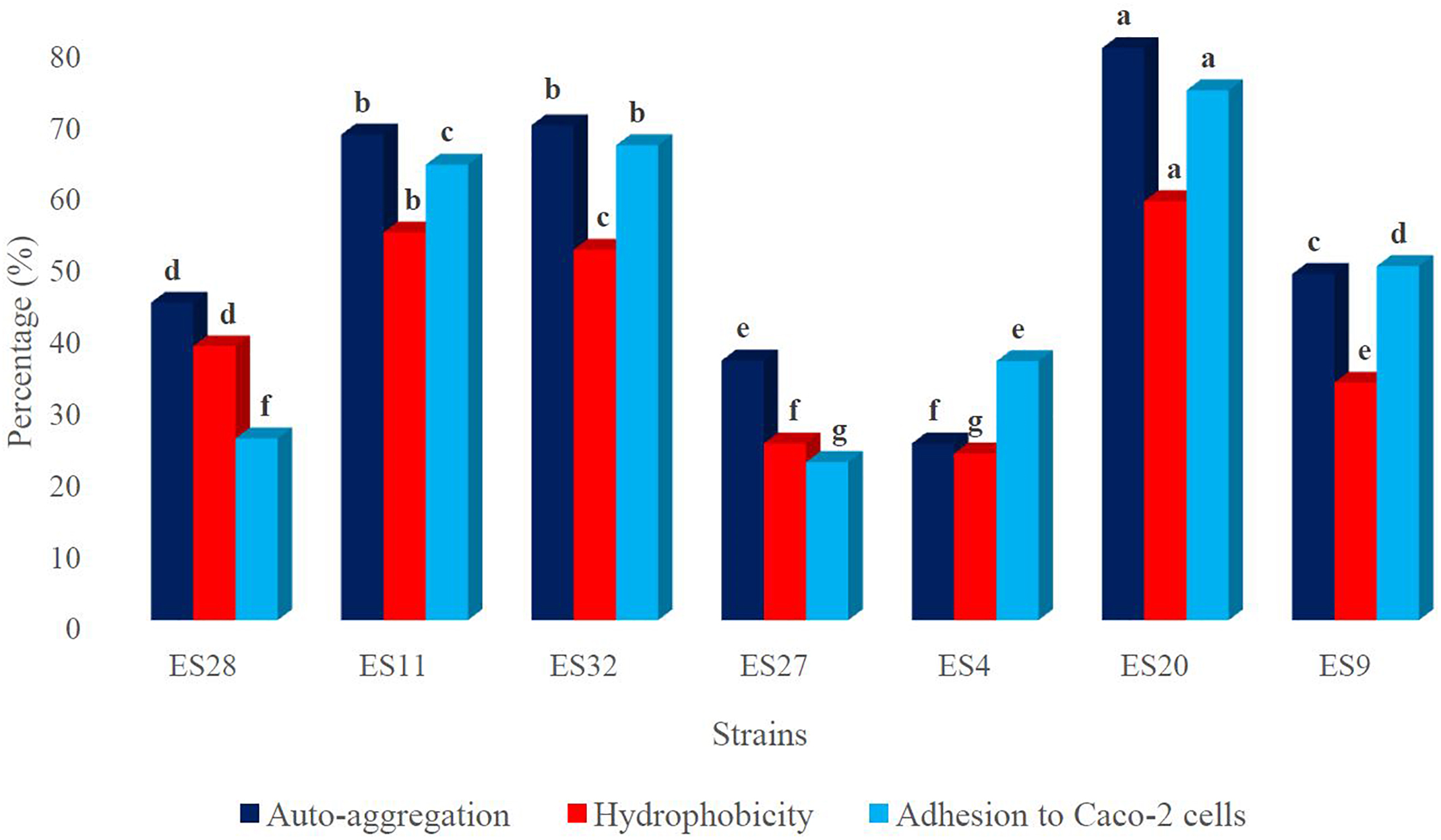
Figure 1. The hydrophobicity, auto-aggregation and adhesion ability of strains to human intestinal cells. a-gMeans in the same color with different lowercase letters differed significantly (p < 0.05).
Adhesion Capacity to Intestinal Cells
The adhesion capacity to human colon carcinoma cell line, Caco-2, was determined (Figure 1). Adhesion capacity to Caco-2 cells varied significantly among the tested bacteria, with adhesion ratio ranging from 22.1 ± 1.8 to 74.1 ± 1.9%. The highest adherence capacity belonged to isolates ES20, ES32, and ES11, with mean values of 74.1 ± 1.9, 66.4 ± 2.2, and 63.7 ± 1.8%, respectively.
EPS Production Ability
The ability of isolates to produce EPS is illustrated in Table 5. The results demonstrated that all the isolates exhibited EPS production ability.
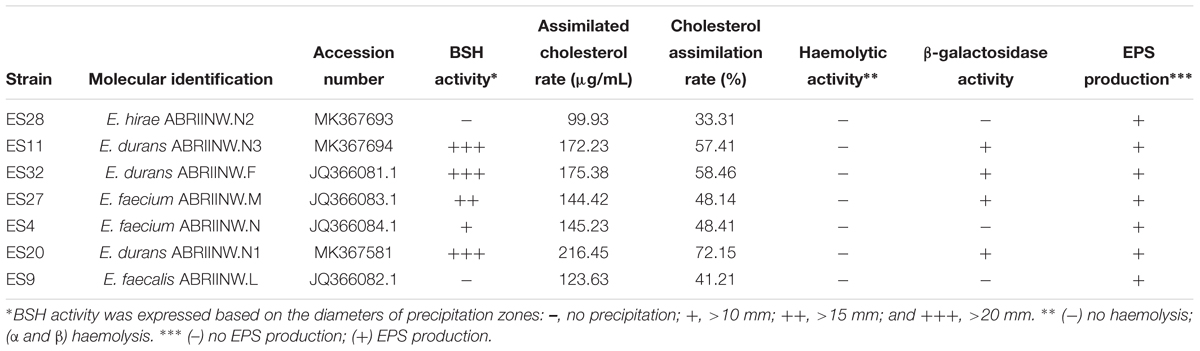
Table 5. Origin, Molecular identification, average cholesterol-removal ratio, BSH activity, EPS production, hemolytic activity and β-galactosidase activity of strains after 20 h of growth at 37°C.
Auto-aggregation and Co-aggregation
The results of cell auto-aggregation assay are shown in Figure 1. The cell auto-aggregation rates of the isolates ranged from 24.7 ± 2.3 to 81.2 ± 2.6%. The highest scores were obtained for isolates ES20, followed by ES32, and ES11 with 81.2 ± 2.6, 69.2 ± 2.1, and 67.9 ± 1.2%, respectively. Furthermore, isolate ES4 showed the lowest auto-aggregation rates with 24.7 ± 2.3%.
The results of co-aggregation of Enterococcus isolates in the presence of Staphylococcus aureus, Escherichia coli, Listeria monocytogenes, Shigella flexneri, Klebsiella pneumoniae, Yersinia enterocolitica, and Bacillus subtilis separately at 37°C at 2 and 4 h of incubation are shown in Table 6. The results showed that isolates 11, 20, and 32 exhibited higher co-aggregation ability compared to other isolates. The co-aggregation percentages increased (P < 0.05) during incubation. Co-aggregations of Enterococcus isolates with all the pathogens at 4 h of incubation were higher (P < 0.05) compared to 2 h of incubation. Isolates demonstrated lower co-aggregation (P < 0.05) toward gram-positive pathogens (S. aureus, L. monocytogenes, and B. subtilis) compared to gram-negative ones (Y. enterocolitica, Sh. Flexneri, K. pneumoniae, and Escherichia coli).
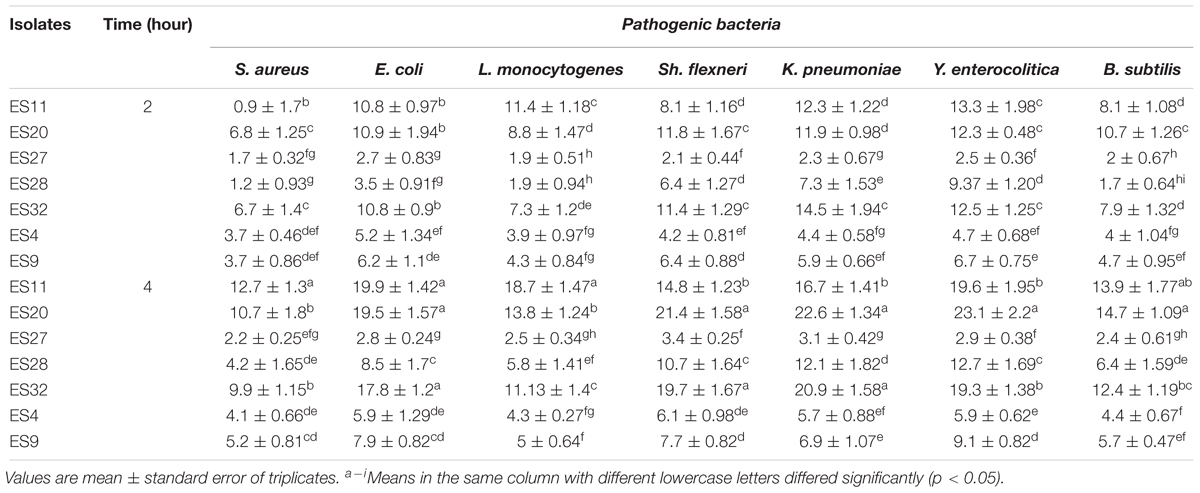
Table 6. Co-aggregation (%) of Enterococcus isolates with 7 pathogens during 4 h incubation at 37°C.
Cholesterol Assimilation and Bile Salt Hydrolysis
Table 5 presents the levels of cholesterol assimilation by isolates in the presence of 0.3% bile oxgall at 37°C for 20 h. The content of cholesterol removed varied (P < 0.05) and ranged from 99.93 to 216.45 μg/mL. The highest content of cholesterol assimilation was observed in isolates ES32, ES20, and ES11, which belonged to Enterococcus durans species with 216.45, 175.38, and 172.23 μg/mL. In contrast, the lowest content belonged to isolate ES28.
The bile salt hydrolysis of the Enterococcus isolates is shown in Table 5. The results indicated that isolates ES11, ES20, and ES32 showed the highest BSH activity (+++), whereas isolate ES27 exhibited moderate BSH activity (++). Furthermore, isolate ES4 showed less BSH activity (+), while isolates ES9 and ES28 showed no activity (-).
Hemolytic Activity
Hemolytic activity of isolates is represented in Table 5. All the isolates showed no β-hemolytic activity.
β-Galactosidase Activity
Isolates ES11, ES20, ES27, and ES32 indicated the presence of β-galactosidase activity, while isolates ES4, ES7, and ES28 did not show the presence of this enzyme.
Detection of Virulence Factors
The presence of genes encoding eight known virulence factors in the Enterococcus isolates was assessed. The results of PCR amplification revealed that none of the isolates harbored any virulence factors except E. faecalis (ES9), which showed the presence of esp gene.
Antibiotic Susceptibility
Table 7 illustrates the antibiotic resistance of Enterococcus isolates against 16 tested antibiotics. Overall, all the isolates showed the ability to resist the impact of tetracycline and colistin, whilst all the isolates were susceptible to gentamycin, vancomycin and chloramphenicol. The impact of other antibiotics against isolates varied from susceptible to resistant. Among the tested Enterococcus isolates, isolate ES11 was susceptible to all the antibiotics, except for tetracycline and colistin.
16S-rDNA Sequencing
16S-rDNA sequencing was performed as molecular phylogeny analysis to identify selected Enterococcus isolates at the species level. Phylogenetic tree (Figure 2) was constructed based on the 16S-rDNA sequences from evolutionary distances by the neighbor-joining method. Analysis of the sequences depicted that isolate ES9 clustered with sequences of Enterococcus faecalis, isolates ES4 and ES27 clustered with sequences of Enterococcus faecium, isolate ES28 clustered with Enterococcus hirae and three isolates ES11, ES20 and ES32 clustered with Enterococcus durans.
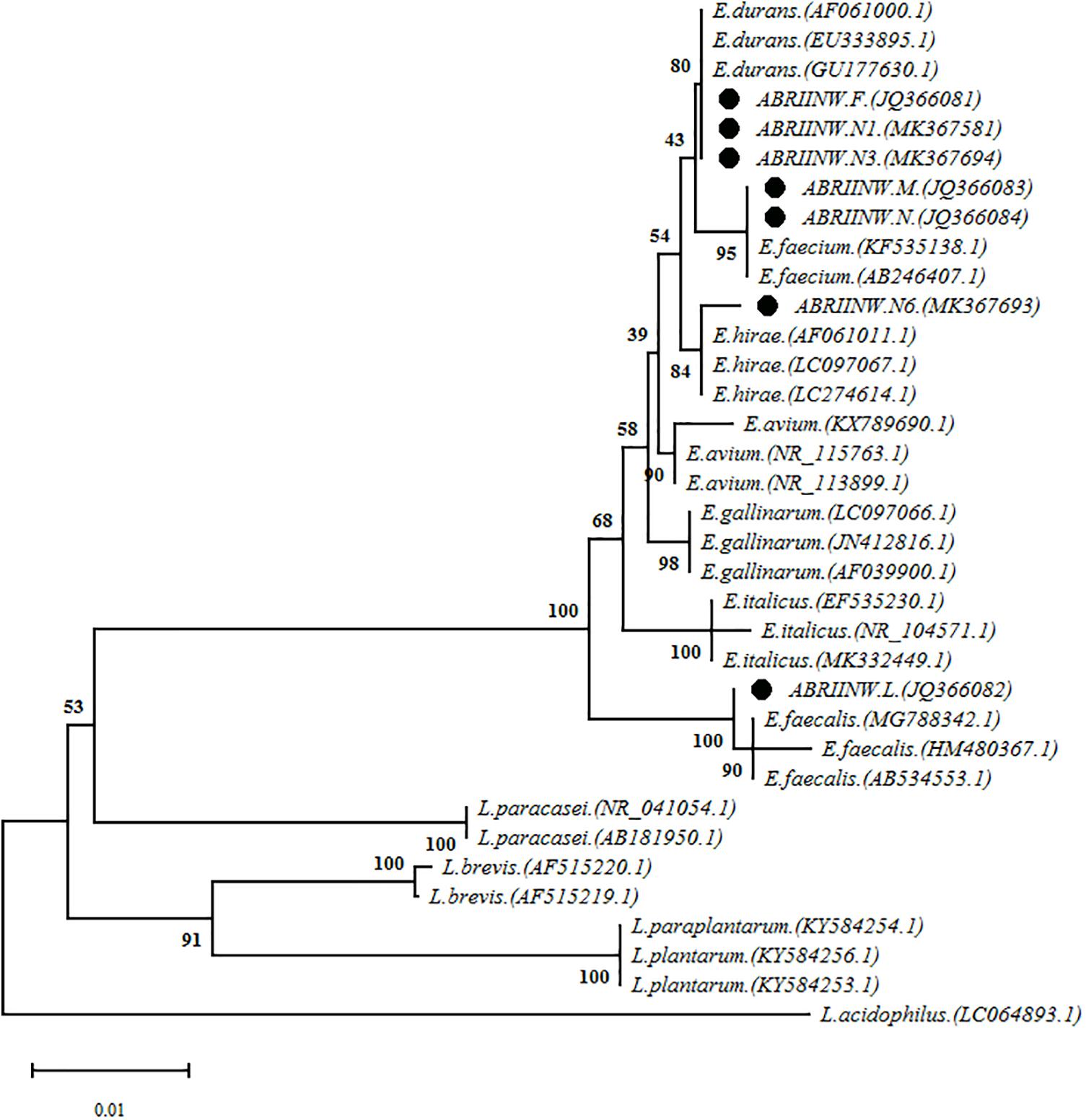
Figure 2. The analysis of the phylogeny of the isolated Enterococcus strains alignment of the sequences was performed with the sequences of different Enterococcus and Lactobacillus species which were submitted in NCBI database as complete sequence.
Discussion
The ability of isolates to survive under high acidic conditions and to show acceptable tolerance against bile salts in the human intestine are two key properties for a candidate to be considered a probiotic (Kandylis et al., 2016; Ayyash et al., 2018). In this study, the survival rate of isolates in acidic conditions and bile salts displayed a significant variability, which might be due to the fact that mechanisms of acid and bile tolerance are species and strain-dependent. Isolates ES4, ES9, ES11, ES20, ES27, ES28, and ES32 showed favorable acid and bile tolerance compared to the other isolates. Therefore, only these seven isolates were subjected to further tests. The acid and bile tolerances of the seven isolates are consistent with the results reported by Nami et al. (2014); El-Jeni et al. (2015), Haghshenas et al. (2016), and Ayyash et al. (2018).
The nature of inhibitory effect of isolates was assessed by adjusting pH to 6.2 and also using catalase and protease enzymes. After treating with protease enzymes, the clear zones around the halos were disappeared. It could be because of proteinaceous nature of secreted metabolites by isolates. It has been shown by some studies (Balla et al., 2000; Cintas et al., 2000) that bacteriocins secreted by Enterococcus isolates are strong inhibitors of food-borne pathogens such as S. aureus, L. monocytogenes and Clostridium tyrobutyricum. In our study, the inhibitory profile of the Enterococcus isolates under assessment tended to be active against a wide range of gram-positive and gram-negative bacteria and food-borne pathogens, including Staphylococcus, Listeria, Yersinia, Bacillus, Shigella, Escherichia coli and Klebsiella. These recorded activities are in contrast with Stevens et al. (1991) and Zommiti et al. (2018), who theorized that bacteriocins of LAB are ineffective against gram-negative bacteria because the outer membrane blocks the bacteriocin target. Moreover, PCR amplification of genes coding for enterocins (EntA, EntB, EntQ, EntP, EntL50A, EntL50B, Ent1071, and Bac31) was investigated. All the isolates contained at least one enterocin gene and the enterocins A and B were detected in all the strains. This is consistent with the results reported by Cintas et al. (2000) and Belgacem et al. (2010), who detected these putative enterocin factors in Enterococcus isolates. Isolates ES11, ES20, and ES32, which showed the ability to compete against all the seven tested pathogens, contained a combination of four enterocins such as entA, entB, entQ and entL50A. This is consistent with Sánchez et al. (2007), who proposed the co-production of two or more enterocins by a strain generating supernatants with a higher antagonistic activity.
Probiotic capacity to remain alive in the gastrointestinal tract is one of the most desirable features of probiotics. To be colonized in the intestine, probiotics have to adhere to the intestinal mucosa to avoid being removed from the colon by peristalsis. In this study, strains ES20, ES32, and ES11 exhibited favorable adherence capacity (Duary et al., 2011; Kumar et al., 2015). Similar to these results, high capability to adhere to Caco-2 cells was reported for Enterococcus isolates (Cebrián et al., 2012; Pimentel et al., 2012).
The ability of isolates to produce EPS was determined by the presence of ropy white mucus on skimmed milk plates containing ruthenium red. It has been shown that LAB is able to produce EPS, which improves the viscosity and texture of dairy products. Hence, EPS-producing LAB is widely used in the dairy industry. The presence of (glyco-) proteinaceous on the cell surface results in higher hydrophobicity, while the presence of polysaccharides leads to hydrophilic surfaces (Osmanagaoglu et al., 2010).
Hydrophobicity is one of the indicative parameters for cell surface properties of probiotics, which correlates with the adhesion ability of probiotics to epithelial cells (Duary et al., 2011; Zuo et al., 2016; Ayyash et al., 2018). Thus, the higher hydrophobicity resulted in higher ability of probiotics to attach to epithelial cells and promote health benefits. In the current study, isolates ES20, ES11, and ES32 exhibited better hydrophobicity percentages compared with the results reported by Ayyash et al. (2018). Moreover, Das et al. (2016) also reported that hydrophobicity of three LAB ranged from 22.2 to 25.0%, which is lower as compared with our findings.
The auto-aggregation and co-aggregation ability are two important properties of probiotics, which are defined as the bacterial accumulation of the same species and of different species, respectively (Campana et al., 2017). The auto-aggregation and co-aggregation are fundamental for probiotics because it seems that auto-aggregation is correlated with adherence to epithelial cells (Collado et al., 2008), while co-aggregation represents a defensive barrier for the colonization of pathogenic microorganisms (Kos et al., 2003; Abushelaibi et al., 2017). In addition, the bacterial equilibrium in the gastrointestinal tract is increased by aggregation of probiotics in the human gut (Tulumoglu et al., 2013) and the probiotic properties of the LAB are improved by their co-aggregation ability in the presence of gut pathogens. The formation of a defensive barrier because of co-aggregation of LAB in the presence of pathogens will not allow pathogens to colonize in the human gut (Vidhyasagar and Jeevaratnam, 2013). Tareb et al. (2013) reported that the ability of LAB isolates to co-aggregate with pathogens could be attributed to proteinaceous components present on the cell surface and interactions between carbohydrate and lectin. Nevertheless, Collado et al. (2008) revealed that the co-aggregation ability of LAB is time- and strain- dependent. Our results correspond with those of Angmo et al. (2016); Taheur et al. (2016), and Abushelaibi et al. (2017). Our study showed that the co-aggregation ability is significantly affected by incubation time and strain.
In vitro studies on cholesterol reduction by Enterococcus species have been considered as an important parameter for the selection of probiotic strains with diverse health-promoting benefits. The hypocholesterolemic effect on host is another important but not essential property of probiotics. Several mechanisms have been postulated for lowering cholesterol by probiotic bacteria (Miremadi et al., 2014), including conversion of cholesterol to coprostanol by reductase, cholesterol incorporation in the cell wall and disruption of cholesterol micelle in the intestine by deconjugated bile salts. Cholesterol removal results in the current study are in agreement with the results of studies by Ayyash et al. (2018). In the current study, the cholesterol removal could be attributed mainly to cholesterol micelle in the intestine by deconjugated bile salts.
Presently the role of BSH is controversial because it might act either positively in lowering of serum cholesterol or negatively in increasing the level of undesirable deconjugated bile salts (Xie et al., 2015; Fadda et al., 2017). On the other hand, BSH activity by probiotic bacteria might be desirable because it increases the intestinal survival and persistence of producing strains, which in turn increases the overall beneficial effects associated with the strain (Begley et al., 2006; Park et al., 2007).
The safety assessment is obligatory before a strain is qualified as beneficial for the host health for use in food industry. The absence of hemolytic activity and antibiotic resistance are the basic requirements for the selection of safe probiotic strains (Oh and Jung, 2015). No β-hemolytic activity was detected in the 7 tested strains.
The presence of β-galactosidase, which is a useful enzyme that hydrolyses lactose into glucose and galactose was performed. Lactose intolerance is due to the lack or shortage of this enzyme; hence lactose mal-digestion symptoms could be improved by consumption of probiotics that release β-galactosidase (Hussain et al., 2008). On the other hand, the products fermented with β-galactosidase producers play an essential role in the treatment of lactose intolerance (Vasiljevic and Jelen, 2001). Our findings proved that the tested enterococci isolates showed good β-galactosidase activity. Isolates ES32, ES20 and ES11 showed the highest β-galactosidase activity. Hence, these isolates may have an effective application in the dairy industry and also for the treatment of lactose intolerance.
A desirable characteristic for enterococcal bacteria used in food industry is the absence of cytolysin-encoding genes. Cytolysin is a bacterial toxin expressed by some E. faecalis isolates. In our study, only three isolates showed the presence of cytolysin-encoding genes, which belonged to E. faecalis isolates. None of the isolates belonging to E. durans, E. hirae and E. faecium showed the presence of these genes. In addition, only five isolates belonging to E. faecalis showed the presence of esp gene. The absence of esp in E. faecium was reported (Eaton and Gasson, 2001), which revealed the frequent presence of the esp gene in medical E. faecium isolates. Our results are in accordance with the results of Eaton and Gasson (2001) and Liu et al. (2015). Overall, the presence of virulence genes is higher in E. faecalis species than in E. faecium species, which is consistent with our results.
The resistance of Enterococcus species to various antibiotics has been reported by some studies (Vidhyasagar and Jeevaratnam, 2013). Because of transferring the resistance factors from probiotics to pathogenic microorganisms via the interchange of genetic materials, the intake of antibiotic-resistant strains disrupts the original flora in the intestine (Mathur and Singh, 2005; Hummel et al., 2007; Taheur et al., 2016). In the current study, all the isolates were resistant to colistin and tetracycline. This can be attributed to the overuse of these antibiotics in rural areas.
Conclusion
The results of this study indicated that E. durans ES11, E. durans ES20 and E. durans ES32 are safe probiotic strains with the potential to assimilate total cholesterol. These strains fulfilled several criteria to be used as probiotic microorganisms, including auto- and co-aggregation ability, resistance to low pH and high bile salts, adherence to hydrocarbons, susceptibility to some antibiotics as well as EPS production. As these isolates are from the food sources which display a wide spectrum of capability against certain intestinal and food-borne pathogens, it could be used in functional foods since these probiotic strains adapt to the conditions and could provide protection against pathogens. Further studies will be required to determine the mechanisms underlying the cholesterol-lowering effect and to evaluate the long-term probiotic potential of these strains.
Author Contributions
All authors listed have made a substantial, direct and intellectual contribution to the work, and approved it for publication.
Funding
This work was supported by the Agricultural Biotechnology Research Institute of Iran (ABRII) (Grant No. 3-05-0551-88020).
Conflict of Interest Statement
The authors declare that the research was conducted in the absence of any commercial or financial relationships that could be construed as a potential conflict of interest.
Acknowledgments
The financial support of the Agricultural Biotechnology Research Institute of Iran (ABRII) is gratefully acknowledged.
References
Abedi, J., Saatloo, M. V., Nejati, V., Hobbenaghi, R., Tukmechi, A., Nami, Y., et al. (2018). Selenium-enriched Saccharomyces cerevisiae reduces the progression of colorectal cancer. Biol. Trace Elem. Res. 185, 424–432. doi: 10.1007/s12011-018-1270-9
Abushelaibi, A., Al-Mahadin, S., El-Tarabily, K., Shah, N. P., and Ayyash, M. (2017). Characterization of potential probiotic lactic acid bacteria isolated from camel milk. LWT Food Sci. Technol. 79, 316–325. doi: 10.1155/2018/7970463
Ahl, D., Liu, H., Schreiber, O., Roos, S., Phillipson, M., and Holm, L. (2016). Lactobacillus reuteri increases mucus thickness and ameliorates dextran sulphate sodium-induced colitis in mice. Acta Physiol. 217, 300–310. doi: 10.1111/apha.12695
Angmo, K., Kumari, A., and Bhalla, T. C. (2016). Probiotic characterization of lactic acid bacteria isolated from fermented foods and beverage of Ladakh. LWT Food Sci. Technol. 66, 428–435. doi: 10.1016/j.lwt.2015.10.057
Argyri, A. A., Zoumpopoulou, G., Karatzas, K.-A. G., Tsakalidou, E., Nychas, G.-J. E., Panagou, E. Z., et al. (2013). Selection of potential probiotic lactic acid bacteria from fermented olives by in vitro tests. Food Microbiol. 33, 282–291. doi: 10.1016/j.fm.2012.10.005
Arias, C. A., and Murray, B. E. (2012). The rise of the Enterococcus: beyond vancomycin resistance. Nat. Rev. Microbiol. 10, 266–278. doi: 10.1038/nrmicro2761
Ayyash, M., Abushelaibi, A., Al-Mahadin, S., Enan, M., El-Tarabily, K., and Shah, N. (2018). In-vitro investigation into probiotic characterisation of Streptococcus and Enterococcus isolated from camel milk. LWT Food Sci. Technol. 87, 478–487. doi: 10.1016/j.lwt.2017.09.019
Balla, E., Dicks, L., Du Toit, M., Van Der Merwe, M., and Holzapfel, W. (2000). Characterization and cloning of the genes encoding enterocin 1071A and enterocin 1071B, two antimicrobial peptides produced by Enterococcus faecalis BFE 1071. Appl. Environ. Microbiol. 66, 1298–1304. doi: 10.1128/AEM.66.4.1298-1304.2000
Begley, M., Hill, C., and Gahan, C. G. (2006). Bile salt hydrolase activity in probiotics. Appl. Environ. Microbiol. 72, 1729–1738. doi: 10.1128/AEM.72.3.1729-1738.2006
Belgacem, Z. B., Abriouel, H., Omar, N. B., Lucas, R., Martínez-Canamero, M., Gálvez, A., et al. (2010). Antimicrobial activity, safety aspects, and some technological properties of bacteriocinogenic Enterococcus faecium from artisanal Tunisian fermented meat. Food Control 21, 462–470. doi: 10.1016/j.foodcont.2009.07.007
Brandão, A., Almeida, T., Muñoz-Atienza, E., Torres, C., Igrejas, G., Hernández, P., et al. (2010). Antimicrobial activity and occurrence of bacteriocin structural genes in Enterococcus spp. of human and animal origin isolated in Portugal. Arch. Microbiol. 192, 927–936. doi: 10.1007/s00203-010-0619-z
Campana, R., Van Hemert, S., and Baffone, W. (2017). Strain-specific probiotic properties of lactic acid bacteria and their interference with human intestinal pathogens invasion. Gut Pathog. 9:12. doi: 10.1186/s13099-017-0162-4
Carlos, A., Semedo-Lemsaddek, T., Barreto-Crespo, M., and Tenreiro, R. (2010). Transcriptional analysis of virulence-related genes in enterococci from distinct origins. J. Appl. Microbiol. 108, 1563–1575. doi: 10.1111/j.1365-2672.2009.04551.x
Cebrián, R., Baños, A., Valdivia, E., Pérez-Pulido, R., Martínez-Bueno, M., and Maqueda, M. (2012). Characterization of functional, safety, and probiotic properties of Enterococcus faecalis UGRA10, a new AS-48-producer strain. Food Microbiol. 30, 59–67. doi: 10.1016/j.fm.2011.12.002
Cintas, L. M., Casaus, P., Herranz, C., Håvarstein, L. S., Holo, H., Hernández, P. E., et al. (2000). Biochemical and genetic evidence that Enterococcus faecium L50 produces enterocins L50A and L50B, the sec-dependent enterocin P, and a novel bacteriocin secreted without an N-terminal extension termed enterocin Q. J. Bacteriol. 182, 6806–6814. doi: 10.1128/JB.182.23.6806-6814.2000
Collado, M. C., Meriluoto, J., and Salminen, S. (2008). Adhesion and aggregation properties of probiotic and pathogen strains. Eur. Food Res. Technol. 226, 1065–1073. doi: 10.1007/s00217-007-0632-x
Creti, R., Imperi, M., Bertuccini, L., Fabretti, F., Orefici, G., Di Rosa, R., and Baldassarri, L. (2004). Survey for virulence determinants among Enterococcus faecalis isolated from different sources. J. Med. Microbiol. 53, 13–20. doi: 10.1099/jmm.0.05353-0
Das, P., Khowala, S., and Biswas, S. (2016). In vitro probiotic characterization of Lactobacillus casei isolated from marine samples. LWT Food Sci. Technol. 73, 383–390. doi: 10.1016/j.lwt.2016.06.029
Duary, R. K., Rajput, Y. S., Batish, V. K., and Grover, S. (2011). Assessing the adhesion of putative indigenous probiotic lactobacilli to human colonic epithelial cells. Indian J. Med. Res. 134, 664–671. doi: 10.4103/0971-5916.90992
Eaton, T. J., and Gasson, M. J. (2001). Molecular screening of Enterococcus virulence determinants and potential for genetic exchange between food and medical isolates. Appl. Environ. Microbiol. 67, 1628–1635. doi: 10.1128/AEM.67.4.1628-1635.2001
El Soda, M., Ahmed, N., Omran, N., Osman, G., and Morsi, A. (2003). Isolation, identification and selection of lactic acid bacteria cultures for cheesemaking. Emir. J. Food Agric. Sci. 15, 51–71. doi: 10.9755/ejfa.v15i2.5006
El-Jeni, R., El Bour, M., Calo-Mata, P., Böhme, K., Fernández-No, I. C., Barros-Velázquez, J., et al. (2015). In vitro probiotic profiling of novel Enterococcus faecium and Leuconostoc mesenteroides from Tunisian freshwater fishes. Can. J. Microbiol. 62, 60–71. doi: 10.1139/cjm-2015-0481
Fadda, M. E., Mossa, V., Deplano, M., Pisano, M. B., and Cosentino, S. (2017). In vitro screening of Kluyveromyces strains isolated from Fiore Sardo cheese for potential use as probiotics. LWT Food Sci. Technol. 75, 100–106. doi: 10.1016/j.lwt.2016.08.020
Fguiri, I., Ziadi, M., Atigui, M., Ayeb, N., Arroum, S., Assadi, M., et al. (2016). Isolation and characterisation of lactic acid bacteria strains from raw camel milk for potential use in the production of fermented Tunisian dairy products. Int. J. Dairy Technol. 69, 103–113. doi: 10.1111/1471-0307.12226
Haghshenas, B., Haghshenas, M., Nami, Y., Khosroushahi, A. Y., Abdullah, N., Barzegari, A., et al. (2016). Probiotic assessment of Lactobacillus plantarum 15HN and Enterococcus mundtii 50H isolated from traditional dairies microbiota. Adv. Pharm. Bull. 6, 37–47. doi: 10.15171/apb.2016.007
Haghshenas, B., Nami, Y., Abdullah, N., Radiah, D., Rosli, R., Barzegari, A., et al. (2015). Potentially probiotic acetic acid bacteria isolation and identification from traditional dairies microbiota. Int. J. Food Sci. Technol. 50, 1056–1064. doi: 10.1111/ijfs.12718
Haghshenas, B., Nami, Y., Abdullah, N., Radiah, D., Rosli, R., and Khosroushahi, A. Y. (2014). Anti-proliferative effects of Enterococcus strains isolated from fermented dairy products on different cancer cell lines. J. Funct. Foods 11, 363–374. doi: 10.1016/j.jff.2014.10.002
Haghshenas, B., Nami, Y., Almasi, A., Abdullah, N., Radiah, D., Rosli, R., et al. (2017). Isolation and characterization of probiotics from dairies. Iran. J. Microbiol. 9, 234–243.
Hummel, A. S., Hertel, C., Holzapfel, W. H., and Franz, C. M. (2007). Antibiotic resistances of starter and probiotic strains of lactic acid bacteria. Appl. Environ. Microbiol. 73, 730–739. doi: 10.1128/AEM.02105-06
Hussain, M., Khan, M. T., Wajid, A., and Rasool, S. A. (2008). Technological characterization of indigenous enterococcal population for probiotic potential. Pak. J. Bot. 40, 867–875.
Kandylis, P., Pissaridi, K., Bekatorou, A., Kanellaki, M., and Koutinas, A. A. (2016). Dairy and non-dairy probiotic beverages. Curr. Opin. Food Sci. 7, 58–63. doi: 10.1016/j.cofs.2015.11.012
Kobyliak, N., Conte, C., Cammarota, G., Haley, A. P., Styriak, I., Gaspar, L., et al. (2016). Probiotics in prevention and treatment of obesity: a critical view. Nutr. Metab. 13:14. doi: 10.1186/s12986-016-0067-0
Kos, B., Šušković, J., Vuković, S., Šimpraga, M., Frece, J., and Matošić, S. (2003). Adhesion and aggregation ability of probiotic strain Lactobacillus acidophilus M92. J. Appl. Microbiol. 94, 981–987. doi: 10.1046/j.1365-2672.2003.01915.x
Kumar, B. V., Vijayendra, S. V. N., and Reddy, O. V. S. (2015). Trends in dairy and non-dairy probiotic products-a review. J. Food Sci. Technol. 52, 6112–6124. doi: 10.1007/s13197-015-1795-2
Kumar, S., Stecher, G., Li, M., Knyaz, C., and Tamura, K. (2018). MEGA X: molecular evolutionary genetics analysis across computing platforms. Mol. Biol. Evol. 35, 1547–1549. doi: 10.1093/molbev/msy096
Lau, C. S., and Chamberlain, R. S. (2016). Probiotics are effective at preventing Clostridium difficile-associated diarrhea: a systematic review and meta-analysis. Int. J. Gen. Med. 9, 27–37. doi: 10.1093/molbev/msy096
Leenhouts, K. J., Kok, J., and Venema, G. (1990). Stability of integrated plasmids in the chromosome of Lactococcus lactis. Appl. Environ. Microbiol. 56, 2726–2735.
Li, B., Zhan, M., Evivie, S. E., Jin, D., Zhao, L., Chowdhury, S., et al. (2018). Evaluating the safety of potential probiotic Enterococcus durans KLDS6. 0930 using whole genome sequencing and oral toxicity study. Front. Microbiol. 9:1943. doi: 10.3389/fmicb.2018.01943
Liu, W., Zhang, L., Shi, J., Yi, H., Zhang, Y., Zhang, S., et al. (2015). Assessment of the safety and applications of bacteriocinogenic Enterococcus faecium Y31 as an adjunct culture in North-eastern Chinese traditional fermentation paocai. Food Control 50, 637–644. doi: 10.1016/j.foodcont.2014.10.004
Mannu, L., Paba, A., Daga, E., Comunian, R., Zanetti, S., Dupré, I., et al. (2003). Comparison of the incidence of virulence determinants and antibiotic resistance between Enterococcus faecium strains of dairy, animal and clinical origin. Int. J. FoodMicrobiol. 88, 291–304. doi: 10.1016/S0168-1605(03)00191-0
Mathur, S., and Singh, R. (2005). Antibiotic resistance in food lactic acid bacteria—a review. Int. J. Food Microbiol. 105, 281–295. doi: 10.1016/j.ijfoodmicro.2005.03.008
Miremadi, F., Ayyash, M., Sherkat, F., and Stojanovska, L. (2014). Cholesterol reduction mechanisms and fatty acid composition of cellular membranes of probiotic Lactobacilli and Bifidobacteria. J. Funct. Foods 9, 295–305. doi: 10.1016/j.jff.2014.05.002
Mishra, V., and Prasad, D. (2005). Application of in vitro methods for selection of Lactobacillus casei strains as potential probiotics. Int. J. Food Microbiol. 103, 109–115. doi: 10.1016/j.ijfoodmicro.2004.10.047
Moreno, M. F., Sarantinopoulos, P., Tsakalidou, E., and De Vuyst, L. (2006). The role and application of enterococci in food and health. Int. J. Food Microbiol. 106, 1–24. doi: 10.1016/j.ijfoodmicro.2005.06.026
Nami, Y., Abdullah, N., Haghshenas, B., Radiah, D., Rosli, R., and Khosroushahi, A. Y. (2014). Probiotic assessment of Enterococcus durans 6HL and Lactococcus lactis 2HL isolated from vaginal microflora. J. Med. Microbiol. 63, 1044–1051. doi: 10.1099/jmm.0.074161-0
Nami, Y., Haghshenas, B., Haghshenas, M., Abdullah, N., and Yari Khosroushahi, A. (2015a). The prophylactic effect of probiotic Enterococcus lactis IW5 against different human cancer cells. Front. Microbiol. 6:1317. doi: 10.3389/fmicb.2015.01317
Nami, Y., Haghshenas, B., Haghshenas, M., and Yari Khosroushahi, A. (2015b). Antimicrobial activity and the presence of virulence factors and bacteriocin structural genes in Enterococcus faecium CM33 isolated from ewe colostrum. Front. Microbiol. 6:782. doi: 10.3389/fmicb.2015.00782
Oh, B., Kim, B. S., Kim, J. W., Kim, J. S., Koh, S. J., Kim, B. G., et al. (2016). The effect of probiotics on gut microbiota during the Helicobacter pylori eradication: randomized controlled trial. Helicobacter 21, 165–174. doi: 10.1111/hel.12270
Oh, Y. J., and Jung, D. S. (2015). Evaluation of probiotic properties of Lactobacillus and Pediococcus strains isolated from Omegisool, a traditionally fermented millet alcoholic beverage in Korea. LWT Food Sci. Technol. 63, 437–444. doi: 10.1016/j.lwt.2015.03.005
Omar, N. B., Castro, A., Lucas, R., Abriouel, H., Yousif, N. M., Franz, C. M., et al. (2004). Functional and safety aspects of enterococci isolated from different Spanish foods. Syst. Appl. Microbiol. 27, 118–130. doi: 10.1078/0723-2020-00248
Osmanagaoglu, O., Kiran, F., and Ataoglu, H. (2010). Evaluation of in vitro probiotic potential of Pediococcus pentosaceus OZF isolated from human breast milk. Probiotics Antimicrob. Proteins 2, 162–174. doi: 10.1007/s12602-010-9050-7
Park, Y. H., Kim, J. G., Shin, Y. W., Kim, S. H., and Whang, K. Y. (2007). Effect of dietary inclusion of Lactobacillus acidophilus ATCC 43121 on cholesterol metabolism in rats. J. Microbiol. Biotechnol. 17, 655–662.
Pimentel, L. L., Mättö, J., Malcata, F. X., Pintado, M. E., and Saarela, M. (2012). Survival of potentially probiotic enterococci in dairy matrices and in the human gastrointestinal tract. Int. Dairy J. 27, 53–57. doi: 10.1016/j.idairyj.2012.07.008
Rudel, L. L., and Morris, M. D. (1973). Determination of cholesterol using o-phthalaldehyde. J. Lipid. Res. 14, 364–366.
Saitou, N., and Nei, M. (1987). The neighbor-joining method: a new method for reconstructing phylogenetic trees. Mol. Biol. Evol. 4, 406–425.
Sánchez, J., Basanta, A., Gómez-Sala, B., Herranz, C., Cintas, L., and Hernández, P. (2007). Antimicrobial and safety aspects, and biotechnological potential of bacteriocinogenic enterococci isolated from mallard ducks (Anas platyrhynchos). Int. J. Food Microbiol. 117, 295–305. doi: 10.1016/j.ijfoodmicro.2007.04.012
Sheikhi, A., Shakerian, M., Giti, H., Baghaeifar, M., Jafarzadeh, A., Ghaed, V., et al. (2016). Probiotic yogurt culture Bifidobacterium animalis subsp. lactis BB-12 and Lactobacillus acidophilus LA-5 modulate the cytokine secretion by peripheral blood mononuclear cells from patients with ulcerative colitis. Drug Res. 66, 300–305. doi: 10.1055/s-0035-1569414
Stevens, K., Sheldon, B., Klapes, N. A., and Klaenhammer, T. (1991). Nisin treatment for inactivation of Salmonella species and other gram-negative bacteria. Appl. Environ. Microbiol. 57, 3613–3615.
Taheur, F. B., Kouidhi, B., Fdhila, K., Elabed, H., Slama, R. B., Mahdouani, K., et al. (2016). Anti-bacterial and anti-biofilm activity of probiotic bacteria against oral pathogens. Microb. Pathog. 97, 213–220. doi: 10.1016/j.micpath.2016.06.018
Tareb, R., Bernardeau, M., Gueguen, M., and Vernoux, J.-P. (2013). In vitro characterization of aggregation and adhesion properties of viable and heat-killed forms of two probiotic Lactobacillus strains and interaction with foodborne zoonotic bacteria, especially Campylobacter jejuni. J. Med. Microbiol. 62, 637–649. doi: 10.1099/jmm.0.049965-0
Thompson, J. D., Higgins, D. G., and Gibson, T. J. (1994). Clustal-W: improving the sensitivity of progressive multiple sequence alignment through sequence weighting, position-specific gap penalties and weight matrix choice. Nucleic Acids Res. 22, 4673–4680. doi: 10.1093/nar/22.22.4673
Toit, M. D., Franz, C., Dicks, L., and Holzapfel, W. (2000). Preliminary characterization of bacteriocins produced by Enterococcus faecium and Enterococcus faecalis isolated from pig faeces. J. Appl. Microbiol. 88, 482–494. doi: 10.1046/j.1365-2672.2000.00986.x
Tulumoglu, S., Yuksekdag, Z. N., Beyatli, Y., Simsek, O., Cinar, B., and Yaşar, E. (2013). Probiotic properties of lactobacilli species isolated from children’s feces. Anaerobe 24, 36–42. doi: 10.1016/j.anaerobe.2013.09.006
Vankerckhoven, V., Van Autgaerden, T., Vael, C., Lammens, C., Chapelle, S., Rossi, R., et al. (2004). Development of a multiplex PCR for the detection of asa1, gelE, cylA, esp, and hyl genes in enterococci and survey for virulence determinants among European hospital isolates of Enterococcus faecium. J. Clin. Microbiol. 42, 4473–4479. doi: 10.1128/JCM.42.10.4473-4479.2004
Vasiljevic, T., and Jelen, P. (2001). Production of β-galactosidase for lactose hydrolysis in milk and dairy products using thermophilic lactic acid bacteria. Innov. Food Sci. Emerg. Technol. 2, 75–85. doi: 10.1016/S1466-8564(01)00027-3
Vidhyasagar, V., and Jeevaratnam, K. (2013). Evaluation of Pediococcus pentosaceus strains isolated from Idly batter for probiotic properties in vitro. J. Funct. Foods 5, 235–243. doi: 10.1016/j.jff.2012.10.012
Xie, Y., Zhang, H., Liu, H., Xiong, L., Gao, X., Jia, H., et al. (2015). Hypocholesterolemic effects of Kluyveromyces marxianus M3 isolated from Tibetan mushrooms on diet-induced hypercholesterolemia in rat. Braz. J. Microbiol. 46, 389–395. doi: 10.1590/S1517-838246220131278
Zommiti, M., Cambronel, M., Maillot, O., Barreau, M., Sebei, K., Feuilloley, M., et al. (2018). Evaluation of probiotic properties and safety of Enterococcus faecium isolated from artisanal Tunisian meat “Dried Ossban”. Front. Microbiol. 9:1685. doi: 10.3389/fmicb.2018.01685
Zorriehzahra, M. J., Delshad, S. T., Adel, M., Tiwari, R., Karthik, K., Dhama, K., et al. (2016). Probiotics as beneficial microbes in aquaculture: an update on their multiple modes of action: a review. Vet. Q. 36, 228–241. doi: 10.1080/01652176.2016.1172132
Keywords: Enterococcus, probiotic properties, dairy products, low cholesterol, antimicrobial activity, safety evaluation, Enterococcus as probiotics, virulence factors
Citation: Nami Y, Vaseghi Bakhshayesh R, Mohammadzadeh Jalaly H, Lotfi H, Eslami S and Hejazi MA (2019) Probiotic Properties of Enterococcus Isolated From Artisanal Dairy Products. Front. Microbiol. 10:300. doi: 10.3389/fmicb.2019.00300
Received: 26 September 2018; Accepted: 04 February 2019;
Published: 26 February 2019.
Edited by:
Learn-Han Lee, Monash University Malaysia, MalaysiaReviewed by:
Sergio Enrique Pasteris, Universidad Nacional de Tucumán, ArgentinaKonstantinos Papadimitriou, Agricultural University of Athens, Greece
Copyright © 2019 Nami, Vaseghi Bakhshayesh, Mohammadzadeh Jalaly, Lotfi, Eslami and Hejazi. This is an open-access article distributed under the terms of the Creative Commons Attribution License (CC BY). The use, distribution or reproduction in other forums is permitted, provided the original author(s) and the copyright owner(s) are credited and that the original publication in this journal is cited, in accordance with accepted academic practice. No use, distribution or reproduction is permitted which does not comply with these terms.
*Correspondence: Mohammad Amin Hejazi, aminhejazi@abrii.ac.ir; aminhejazi@yahoo.com