- 1College of Basic Medical Sciences, Army Medical University, Chongqing, China
- 2Queen Mary College, Nanchang University, Nanchang, China
- 3The First Affiliated Hospital of Nanchang University, Nanchang, China
The accessory gene regulator (agr) quorum-sensing system is an important global regulatory system of Staphylococcus aureus and contributes to its pathogenicity. The S. aureus agr system is divided into four agr groups based on the amino acid polymorphisms of AgrB, AgrD, and AgrC. The agr activation is group-specific, resulting in variations in agr activity and pathogenicity among the four agr groups. Strains with divergent agr system always have different phenotypes. In the present report, we, respectively, exchanged the agr system of a certain S. aureus with other three agr alleles and assessed the corresponding phenotypes of these congenic strains. Replacement of the agr system led to significant variations in hemolytic activity, protein expression, and virulence gene expression comparing with that of the parental strain. Interestingly, we found that the biological characteristics of these agr congenic strains in the same strain background were highly similar to each other, and the allele-dependent differences of the agr systems were weakened. These findings indicate that the allele-dependent agr predilections of S. aureus are determined by some factors in addition to the polymorphisms of AgrB, AgrD, and AgrC. Future studies may reveal the novel mechanism to improve our understanding of the agr network.
Introduction
The accessory gene regulator (agr) quorum-sensing system, a globe transcriptional regulator of Staphylococcus aureus, plays a key role in its pathogenesis and resistance (Bernabè et al., 2021) and has been intensively studied to aid drug and vaccine development (Tan et al., 2018). The agr locus comprises two adjacent transcripts, RNAII and RNAIII, which are controlled by P2 and P3 promoters, respectively (Ji et al., 1995). RNAII is composed of four genes, agrB, agrD, agrC, and agrA (Novick et al., 1995). agrD encodes the propeptide for an autoinducing peptide (AIP). agrB encodes an endopeptidase that is the processor of AIP. AgrC and AgrA, encoded by agrC and agrA, function as a two-component regulatory system. When agr system is activated, AIP propeptide is processed to an octapeptide by AgrB and secreted to extracellular space (Queck et al., 2008). As AIP reaches a threshold, the membrane-bound histidine kinase AgrC autophosphorylates and becomes activated, which leads to the phosphorylation of its cognate response regulator, AgrA (Queck et al., 2008). Phosphorylated AgrA activates the transcription of its own RNAII transcript to produce more AIP and also activates promoter P3 to increase the expression of RNAIII (Queck et al., 2008; Wang and Muir, 2016). The two main intracellular effectors, AgrA and RNAIII, regulate expression of virulence factors and contribute to the pathogenicity of S. aureus (Bronesky et al., 2016). AgrA can activate the synthesis of phenol soluble modulin (PSM) peptides, which are the only known toxins regulated by AgrA (Queck et al., 2008). RNAIII was shown to regulate primarily the expression of many important virulence factors and several transcriptional regulators (Wang and Muir, 2016).
The amino acid sequences of AgrB, AgrD, and AgrC are variable (Supplementary Figure 1), whereas AgrA, RNAIII, and their promoter regions are highly conserved (Supplementary Figure 2). According to the polymorphisms of AgrB, AgrD, and AgrC, the agr system in S. aureus is divided into four types named agrI, agrII, agrIII, and agrIV. Each agr variant produces its own specific AIP, which triggers autoinduction. The heterologous pairing of AIP inhibits the response of other agr types and leads to heterologous mutual inhibition (Ji et al., 1997). The variations of agr specificity may form specific functional units that drive evolutionary diversification in Staphylococcus and also have significant implications for host disease (Wright et al., 2005). Cues between special agr groups and some disease predilections have been reported by previous studies. For example, most of agrII clinical S. aureus strains are isolated from acute infection, and half of clinical methicillin-resistant S. aureus (MRSA) bloodstream isolates are in the agrII group. Menstrual toxic shock syndrome is usually caused by agrIII and agrIV S. aureus (Jarraud et al., 2002), and agrIV strains are associated with exfoliative syndromes and bullous impetigo (Gomes et al., 2005). The agrII and agrIV strains exhibit higher biofilm formation capacity (Khoramrooz et al., 2016). The distributions of certain toxin genes and mobile genetic elements also show agr group specificity (Wright et al., 2005). It has been reported that the specific lineage and geographical distribution of S. aureus may correlate with agr types (Holtfreter et al., 2007). In summary, clinical symptoms caused by some staphylococcal strains are closely associated with their agr subgroups (Traber et al., 2008). However, it is not known whether there are any other factors involved in the agr predilection and agr-specific virulence genotypes of S. aureus in addition to the specific agr type, or the polymorphous AgrB, AgrD, and AgrC.
In this study, we replaced the agr system of S. aureus Newman strain (agrI type) or N315 (agrII type) with different agr alleles. The resulting four congenic strains were assessed for their agrA activity, hemolytic activity, pigmentation, exoprotein expression, and virulence factor expressions.
Materials and Methods
Bacterial Strains, Plasmids, Primers, and Growth Conditions
S. aureus strain Newman, an agr group I prototype used as the backbone strain in this study, was originally isolated from a secondarily infected tubercular osteomyelitis lesion (Duthie and Lorenz, 1952). N315, an agrII prototype MRSA with defective agr system, was also used as control background strain (Tsompanidou et al., 2011). MW2 is a prototypical agr group III strain originally isolated from a child with fatal septicemia and septic arthritis (Mei et al., 2011). XQ is a clinical community-associated MRSA belongs to group IV prototype strain isolated from an adolescent patient with staphylococcal scalded skin syndrome (Rao et al., 2015). Escherichia coli strain DH5α was used for plasmid construction and genetic manipulation. All strains were stored in 10% glycerol at −80°C (Supplementary Table 1). E. coli strains were grown in Luria–Bertani broth, whereas S. aureus strains were cultivated in trypticase soy broth (TSB; Sigma) or brain–heart infusion medium (Sigma). When necessary, ampicillin (100 μg/mL) and chloramphenicol (20 μg/mL) were added to the medium. The temperature-sensitive S. aureus plasmid pBT2 and E. coli–S. aureus shuttle plasmid pLI50 kindly provided by Prof. Baolin Sun (University of Science and Technology of China, China) were used for gene mutation and complementation assay, respectively. Transformations of S. aureus strains were performed by electroporation (Bio-Rad Gene Pulser). The primers used in this study were presented in Supplementary Table 2.
Construction of Accessory Gene Regulator Gene Markerless Deletion Mutant
The NewmanΔagrBDC and N315ΔagrBDCA markerless deletion mutants were constructed with pBT2 plasmid using homologous recombinant strategy as described previously (Yuan et al., 2013). Take NewmanΔagrBDC construction as an example, a 803-bp DNA fragment upstream of agrBDC locus was amplified from Newman genome DNA using primer pairs, ΔagrBDC up-for and ΔagrBDC up-rev (Supplementary Table 2). The polymerase chain reaction (PCR) product was digested with HindIII and SalI and subcloned into the same site of pBT2 to obtain plasmid pagrBDCF. Then, a 957-bp DNA fragment downstream of agrBDC locus was amplified and subcloned into the BamHI and EcoRI site of pagrBDCF, yielding the ΔagrBDC knockout plasmid (pagrBDC). The pagrBDC plasmid was identified by restriction enzyme digestion and DNA sequencing and then electrotransformed into S. aureus RN4220 and Newman to construct NewmanΔagrBDC markerless deletion mutant by homologous recombination. The mutants were screened and confirmed by PCR amplification and DNA sequencing. The N315ΔagrBDCA markerless deletion mutant was constructed with similar strategy.
Construction of Accessory Gene Regulator Gene Allele Replacement Strains
The agrBDC fragments of agrII, agrIII, and agrIV were separately obtained from N315, MW2, and XQ by PCR amplification and were, respectively, inserted into the SalI and BamHI sites of pBT2 plasmid to yield pagrBDC-II, pagrBDC-III, and pagrBDC-IV knock-in vectors. Then, these pagrBDC vectors were sequentially introduced into S. aureus RN4220 and NewmanΔagrBDC deletion mutant strain to construct allelic replacement strains via homologous recombination. The substitution of agrBDC fragments of allelic congenic strains was also verified by PCR amplification and DNA sequencing.
Construction of agrBDCA Plasmid Complemented Strains
Full gene DNA of types I through IV agr clusters and their promoters were separately amplified from Newman, N315, MW2, and XQ using the primers described in Supplementary Table 2 and subcloned into the pLI50 plasmid. All positive recombinant plasmids were confirmed by DNA sequencing. Then, these plasmids were, respectively, transformed into E. coli DH5α, S. aureus RN4220, and finally into NewmanΔagrBDC or N315ΔagrBDCA mutant to construct types I–IV agrBDCA genes complemented strains.
Biological Characteristic Analysis
The hemolytic activity, pigment formation, exoprotein production, and gene transcription of agr allelic congenic strains were analyzed to assess the influence of agr system replacement on S. aureus biological characteristics.
For hemolytic activity assessment, overnight culture of each single S. aureus colony was diluted into the same colony-forming units (CFUs) and plated on rabbit’s blood agar plates, followed by overnight growth at 37°C for hemolysis analysis. Furthermore, the hemolytic activity was also evaluated according to the hemolysis of rabbit erythrocytes. As the method described previously (Pader et al., 2014), 100 μL overnight culture supernatant was mixed with 6% rabbit blood in phosphate-buffered saline and then incubated at 37°C for 20 min. The unlysed blood cells were removed by centrifugation, and the erythrocyte lysis was determined with the OD543 values of supernatant.
To evaluate staphyloxanthin production, the diluted overnight inoculation of S. aureus strains was also cultured on TSB plate to assess the effect of agr replacement on pigment formation. Moreover, staphyloxanthin productions were also quantitatively analyzed and adapted from a previously published method (Liu et al., 2018). Cells in 1-mL overnight culture were collected and washed thrice with sterilized water and then resuspended with 200 μL methanol and heated at 55°C for 3 min. After the cells were centrifuged at 10,000 × g for 1 min, the OD462 value of supernatant was detected.
The overnight culture supernatants of S. aureus were collected by centrifugation. Proteins in supernatants were precipitated with trichloroacetic acid and analyzed by sodium dodecyl sulfate–polyacrylamide gel electrophoresis (SDS-PAGE) in a 12% polyacrylamide gel.
The change in gene transcription was appraised with quantitative real-time PCR (qRT-PCR). Cells were harvested at 6 h after inoculation, which represent the mid-log phase of the S. aureus growth. The total bacterial RNA was isolated using SV Total RNA Isolation System kit (Z3100; Promega, United States). The contaminated genomic DNA in RNA was degraded with DNaseI. The cDNA was prepared using PrimeScript RT Reagent Kit (RR047A; Takara, Japan) and used for qRT-PCR using GoTaq ® qPCR Master Mix (A6001; Promega, United States) on an ABI SimpliAmp PCR detection system (United States). Specific primers for qRT-PCR (Supplementary Table 3) were designed according to the target gene sequences. All PCR reactions were performed in triplicate, with 16S rDNA as internal control. The relative expression of gene products was normalized to the housekeeping gene 16S and calculated using the 2–ΔΔCT method.
Results
Construction of Congenic Strains Containing Accessory Gene Regulator Alleles
The agr systems were divided into four agr groups named agrI, agrII, agrIII, and agrIV in S. aureus (Wang and Muir, 2016). AgrA is highly conserved, whereas AgrB, AgrD, and AgrC are variable among the four agr groups (Supplementary Figure 1). In this study, S. aureus Newman and N315 were selected for agr congenic strains construction to assess the effects of divergent agr alleles. The whole RNAII transcripts (involved agrB, agrD, agrC, and agrA genes) were allelic substituted to construct congenic strains in N315, whereas only agrB, agrD, and agrC genes were replaced in Newman congenic strains. The congenic strains were constructed via homologous recombination and confirmed by PCR amplification (Figure 1 and Supplementary Table 2) and DNA sequencing.
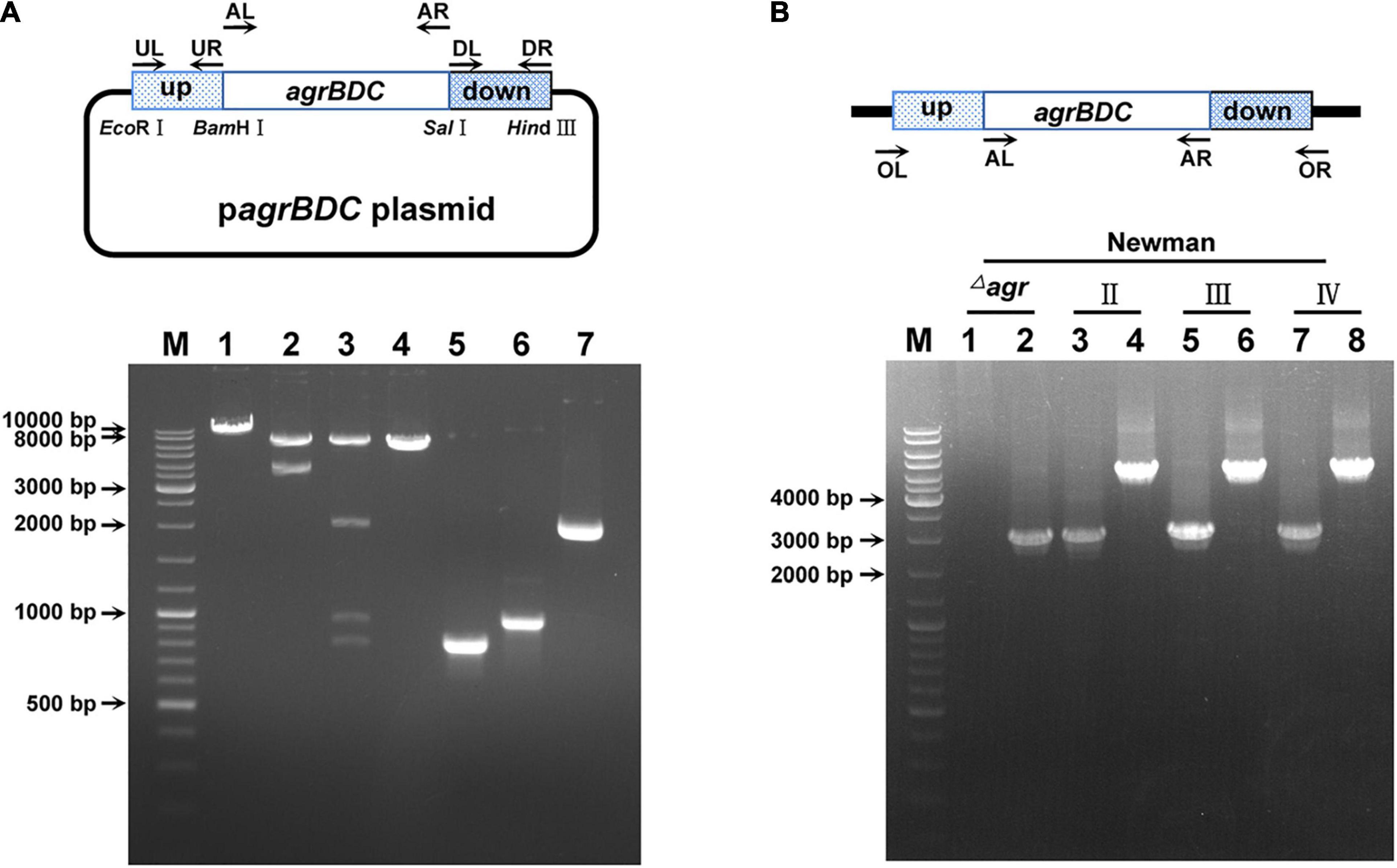
Figure 1. The identification of agr allelic mutant in S. aureus Newman. (A) The confirmation of agr allele replacement plasmid by restriction enzyme digestion (take type II agrBDC replacement as an example). Bands 1, 2, and 3 are the single (BamHI), double (EcoRI + HindIII), and four (BamHI, EcoRI, HindIII, and SalI) enzymes digestion patterns of agrBDC-II allelic replacement plasmid, respectively. Band 4 is the pattern of empty pBT2 plasmid linearized by BamHI. Bands 5, 6, and 7 are patterns of the PCR amplification products of agrBDC-up (Band5, primers UL + UR), agrBDC-down (Band6, primers DL + DR), and agrBDC-II from N315 (Band7, primers AL + AR) fragments, respectively. (B) The confirmation of agr allelic replacement strains in Newman background by PCR amplification with primers AL + AR (Bands 1, 3, 5, 7) and primers OL + OR (Bands 2, 4, 6, 8). Bands 1–2 refer to the PCR products of NewmanΔagrBDC mutants, Bands 3–4 are the PCR products of the congenic strain harbor agrBDC-II sequence, bands 5–6 refer to PCR products of the congenic strain with agrBDC-III replacement, and bands 7–8 for agrBDC-IV replacement. All primers are shown in Supplementary Table 2.
Effects of Accessory Gene Regulator Alleles on Hemolytic Activity
S. aureus is able to secrete a variety of toxins, such as α-hemolysin, bicomponent leukocidins, γ-hemolysin, Panton–Valentine leukocidin (PVL), β-hemolysin, δ-hemolysin, PSMs, and so on (Cheung and Otto, 2012). These exotoxins have leukotoxic and hemolytic activities and are widely associated with the pathogenicity of S. aureus (Powers et al., 2015; Seilie and Bubeck Wardenburg, 2017). α-Hemolysin, γ-hemolysin, PVL, δ-hemolysin, and PSM belong to pore-forming toxins capable of forming transmembrane aqueous channel and leading to host cell lysis (Reyes-Robles and Torres, 2017). β-Hemolysin is a neutral sphingomyelinase capable of digesting sphingomyelin into ceramide and phosphorylcholine (Vandenesch et al., 2012). The production of hemolysins in S. aureus is tightly regulated, and the agr system plays an important role in this process (Johansson et al., 2019). It was reported that the agr system controls the expression of α-, β-, and γ-hemolysin and PVL by RNAIII and regulates the transcription of δ-hemolysin and PSMs through AgrA (Arya and Princy, 2013; Singh and Ray, 2014). The hemolytic capabilities of S. aureus vary with their agr types. As shown in Figure 2A, the Newman (agrI) and XQ (agrIV) strains have strong hemolytic activities, the MW2 (agrIII) strain showed weak hemolysis, and the N315 (agrII) strain presented the lowest hemolytic toxicity (Figure 2A).
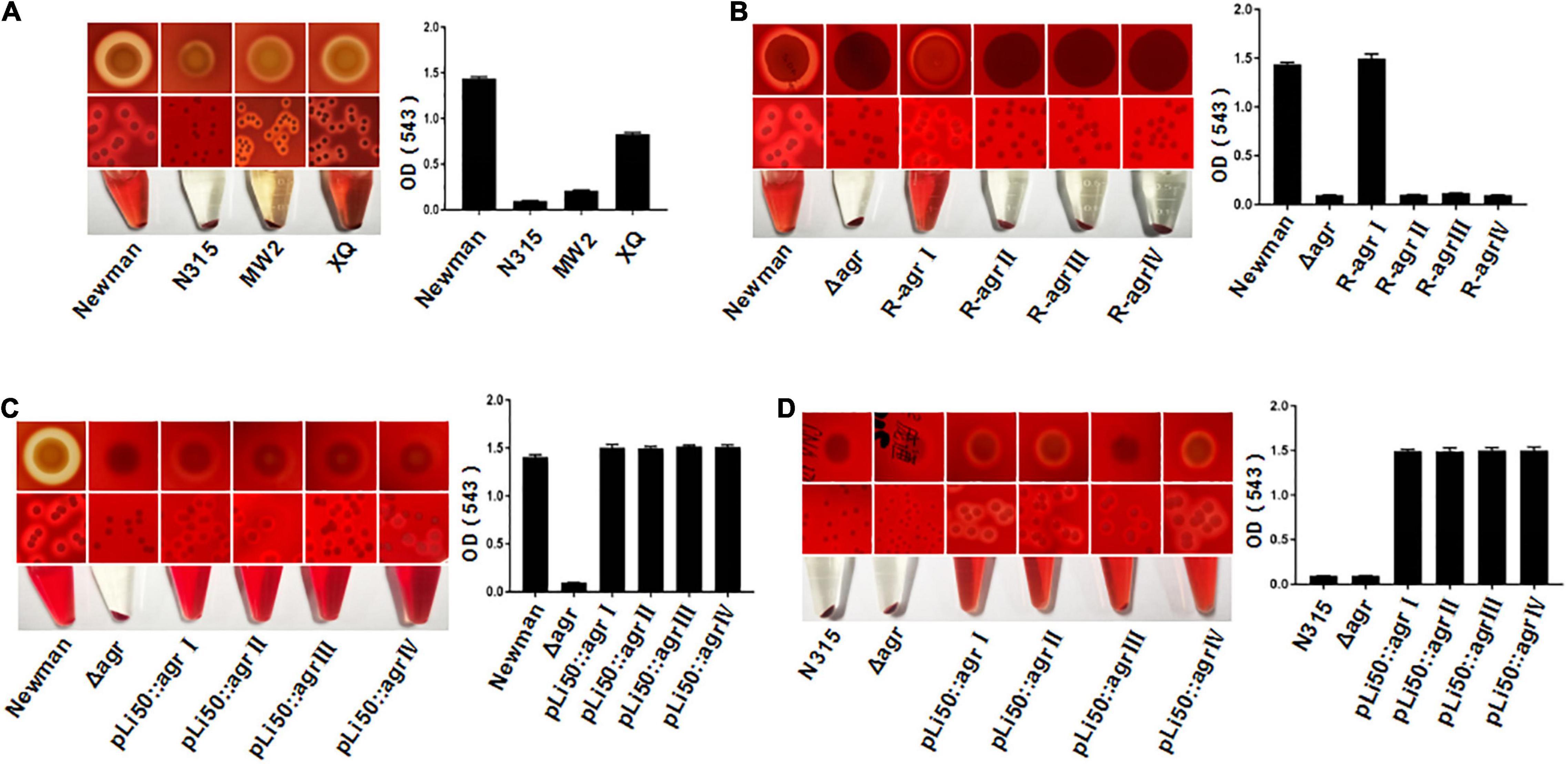
Figure 2. Effects of agr alleles on the hemolytic activities of S. aureus. The overnight cultures of S. aureus strains with the same CFUs were plated on Columbia blood agar plates and grown for 24 h at 37°C. (A) Hemolytic activities of the four wild agr allelic strains: agrI (Newman), agrII (N315), agrIII (MW2), and agrIV (XQ). (B) Hemolytic activities of the wild Newman strain, NewmanΔ agrBDC, and agr genomic in situ replacement mutants of Newman (agrI to agr IV). Hemolytic activities of the wild type, ΔagrBDC mutants and pLI50-agr plasmid complement mutants of Newman (C) and N315 (D). The hemolytic activities of the relative strains were determined by measuring the optical density (OD543).
To assess the effects of different agr alleles on S. aureus hemolytic activity, the agrBDC genes in Newman strain (agrBDC-I) were deleted and in situ substituted with other three agrBDC alleles (agrBDC-II, agrBDC-III, and agrBDC-IV). As expected, the hemolytic activity of the NewmanΔagrBDC strain was significantly lower than that of the wild strain (Figure 2B). The knock-in of type I agrBDC genes back to the genome of NewmanΔagrBDC mutant recovered the hemolytic activity of revertant strain (Figure 2B). Contrary to our expectations, no visible hemolysis and very low hemolytic activity were discovered on three congenic replacement strains harboring heterologous agr systems (Figure 2B). The hemolytic activity controlled by agr system seemed to be severely suppressed when the three heterologous agr alleles were in situ recombined into the genome of NewmanΔagrBDC mutant.
To investigate this further, four recombinant pLI50 plasmids containing types I to IV agrBDCA genes and their promoter sequences were, respectively, transformed into the ΔagrBDC mutant strains of Newman or N315. As shown in Figures 2C,D, the four agr complemented strains showed similar hemolytic activities to each other. The differences in hemolysis across agr groups were significantly weakened in the same Newman or N315 background when compared with the standard agr allelic strains.
Effects of Accessory Gene Regulator Alleles on Pigment Formation
Staphyloxanthin, a carotenoid pigment produced by S. aureus, protects bacteria from neutrophil oxidants (Xue et al., 2019). When the agrBDC sequence was deleted, NewmanΔagrBDC mutant strain lost staphyloxanthin production and formed white colonies (Figures 3B,C). All congenic strains of Newman constructed by genomic replacement or plasmid complemented did not bring back the yellow color of wild Newman strain and showed white colonies (Figures 3B,C). The deletion of genes agrBDCA of N315 did not lead to visible color change (Figure 3D). However, four agr alleles complemented mutants in N315 presented white colonies in contrast to the golden colonies from the wild N315 and N315ΔagrBDCA mutant strains (Figure 3D).
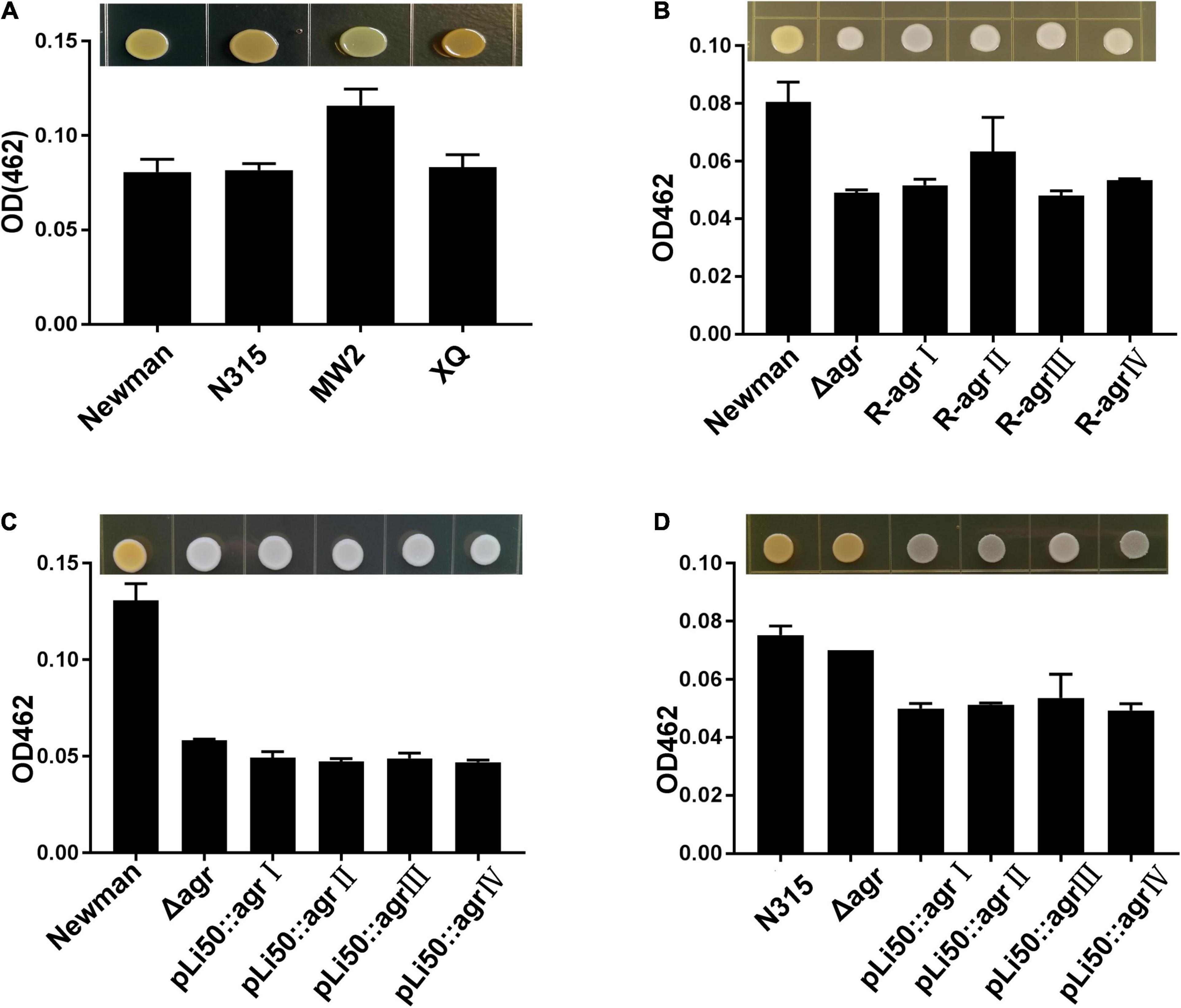
Figure 3. Effects of agr alleles on the pigment formations of S. aureus. The overnight cultures of S. aureus strains with the same CFUs were plated on TSB agar plates and grown for 24 h at 37°C. (A) Pigment productions of the four wild agr allelic strains: agrI (Newman), agrII (N315), agrIII (MW2), and agrIV (XQ). (B) Pigment productions of the wild Newman strain, NewmanΔagrBDC, and agr genomic in situ replacement mutants (R-agrIto agrIV) of Newman. Pigment productions of the wild type, ΔagrBDC mutants, and pLI50-agr plasmid complement mutants of Newman (C) and N315 (D). Pigment productions of the relative strains were determined by measuring the optical density (OD462).
Five genes, crtOPQMN, organized in an operon are responsible for the biosynthesis of staphyloxanthin (Xue et al., 2019). Two colorless farnesyl diphosphate successively catalyzed by five enzymes (CrtM, CrtN, CrtP, CrtQ, CrtO) to yield orange staphyloxanthin. Dehydrosqualene desaturase, CrtN, catalyzes the formation of the first deep yellow-colored carotenoid intermediate product, 4,4′-diaponeurosporene (Wieland et al., 1994). The sigma factor B (SigB) plays an essential role in regulating staphyloxanthin biosynthesis by binding to the promoter that laid in upstream of crtO (Kullik et al., 1998; Pelz et al., 2005). In the present study, the transcription levels of genes crtN and sigB were analyzed and found to be down-regulated in congenic strains when compared with the wild strains, Newman or N315 (Figure 4). It can be inferred that the down-regulated transcriptions of crt operon, especially that of crtN, are responsible for the weaken pigmentations of congenic strains, and the decreased SigB expression may play an important role in these processes.
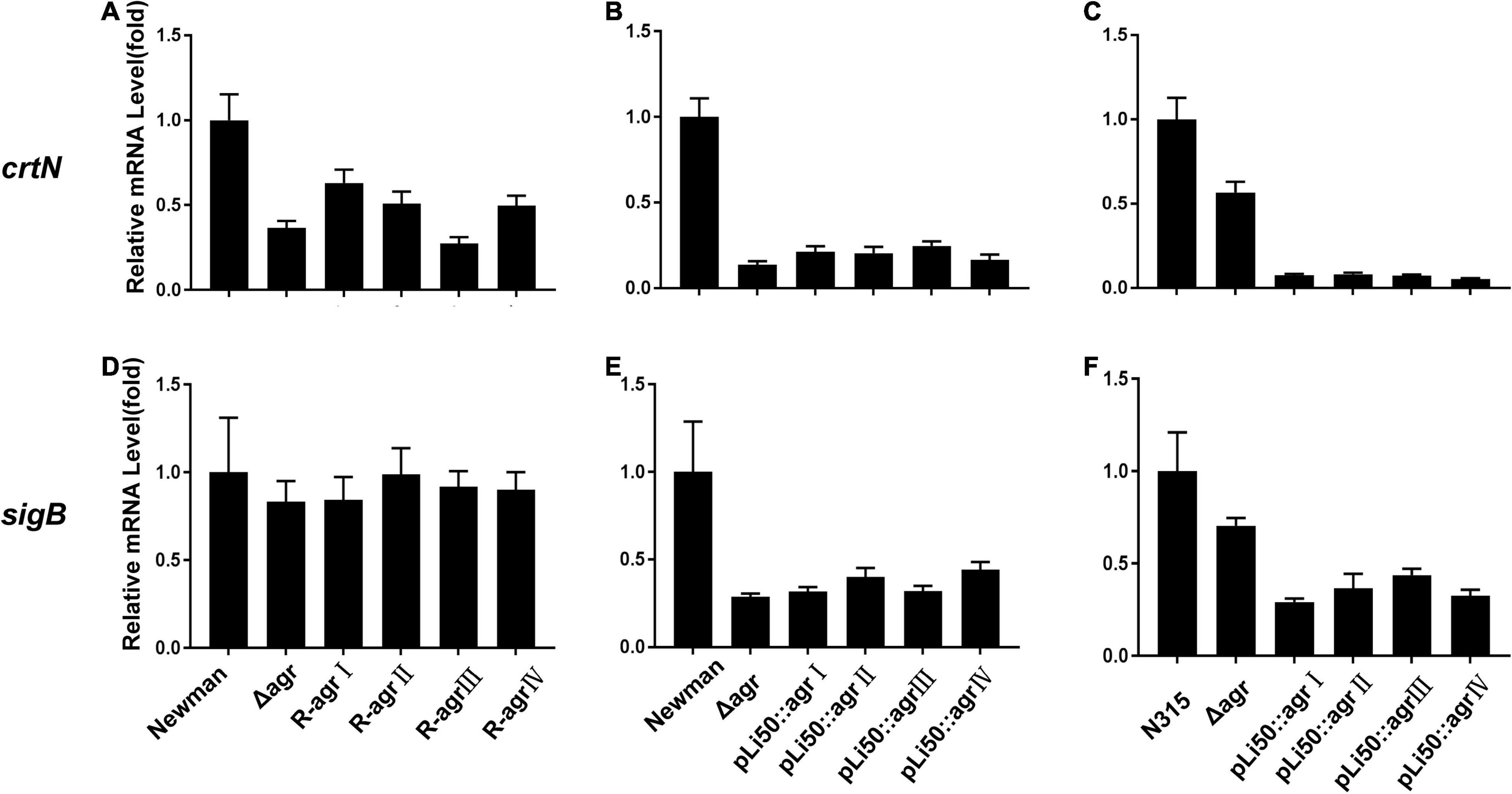
Figure 4. Effects of agr alleles on the transcriptions of staphyloxanthin biosynthesis–related genes. The relative transcription levels of crtN (A–C) and sigB (D–F) were determined. (A,D) The relative transcription levels of wild Newman strain, NewmanΔagrBDC mutant, and agr genomic in situ replacement mutants (R-agrIto agrIV) of Newman. (B,E) The relative transcription levels of wild Newman strain, NewmanΔagrBDC mutant, and four agr plasmid complemented strains in Newman background. (C,F) The relative transcription levels of wild N315 strain, N315ΔagrBDCA mutant, and four agr plasmid complemented strains in N315 background.
Effects of Accessory Gene Regulator Alleles on Exoprotein Productions
To explore the influences of agr alleles on S. aureus exoprotein expressions, overnight culture supernatants were collected and analyzed by SDS-PAGE. As shown in Figure 5A, each agr type strain has its distinctive exoprotein profiles. Deletion of agrBDC genes led to significant changes in exoprotein expressions of Newman (Figure 5A). The knock-in of agrII, agrIII, or agrIV agrBDC genes did not change the exoprotein expressions of NewmanΔagrBDC; the three allelic strains exhibited similar exoprotein profiles as that of NewmanΔagrBDC mutant (Figure 5A). However, when the four allelic agrBDCA genes were introduced by the pLI50 plasmid, the exoprotein patterns of four agr allelic complemented strains changed, which were highly similar to each other (Figure 5B) but different from those of wild agr allelic strains and the genomic replacement strains (Figures 5A,B). The agr allele–dependent difference in exoprotein expression was diminished when in the same Newman background. The similar results were also observed in N315 (Figure 5C). These complement strains exhibited significantly higher exoprotein expression than wild strains (Figures 5B,C). The two most abundant bands at approximately 25 and 35 kDa were identified as bicomponent hemolysin, α-hemolysin, or serine protease with mass spectrometry (Supplementary Tables 4, 5), which have been demonstrated to be regulated by agr system.
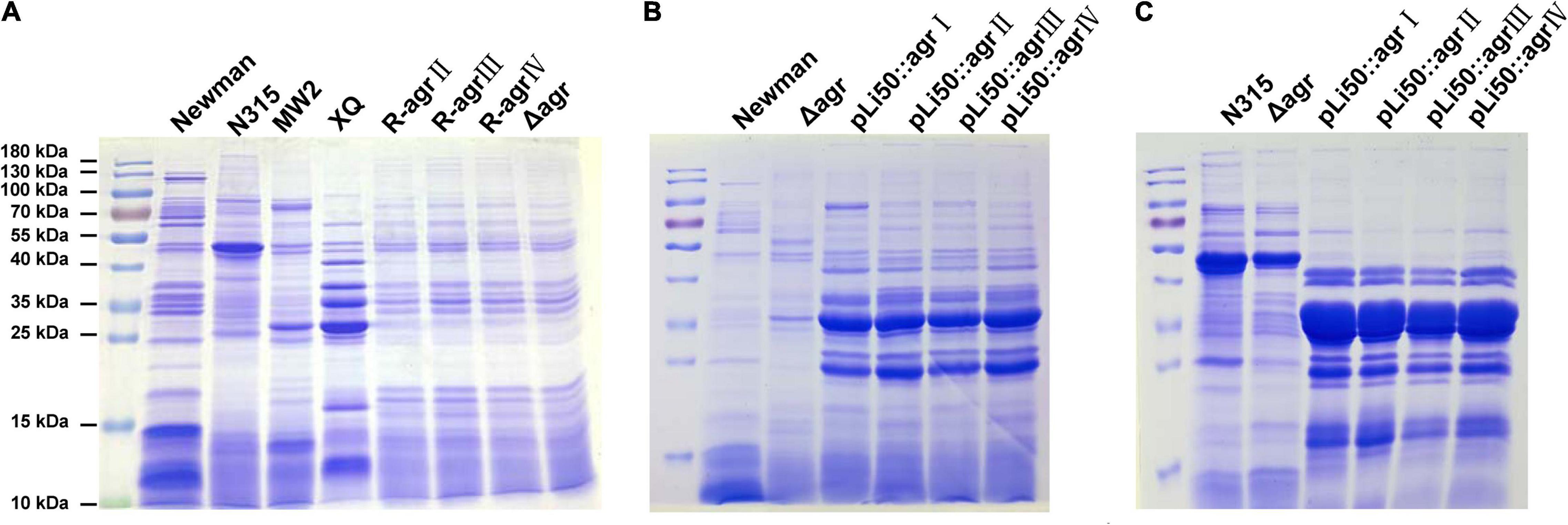
Figure 5. Effects of agr alleles on the exoprotein productions of S. aureus. Overnight culture supernatants of S. aureus strains were collected and analyzed with SDS-PAGE. (A) Exoprotein profiles of the four wild agr allelic strains (agrI/Newman, agrII/N315, agrIII/MW2, and agrIV/XQ), NewmanΔagrBDC mutant and three agr genomic in situ replacement strains in Newman background. (B) Exoprotein profiles of wild Newman strain, NewmanΔagrBDC mutant, and four agr plasmid complemented strains in Newman background. (C) Exoprotein profiles of wild N315 strain, ΔagrBDCA-N315 mutant, and four agr plasmid complemented strains in N315 background.
Effects of Accessory Gene Regulator Alleles on Virulence Gene Regulations
The ability of S. aureus to invade host tissues and cause infections depends on the production of a number of virulence factors. Many of them are regulated by the agr system such as α-toxin, β-toxin, δ-toxin, serine protease, fibrinolysin, PSM, and enterotoxin B (Seilie and Bubeck Wardenburg, 2017). In this study, we sought to examine the effects of different agr alleles on the transcription of virulence factors as hla, hlb, hld, hlg, psmα, psmβ, pvl, and aur (Figure 6 and Supplementary Figure 4).
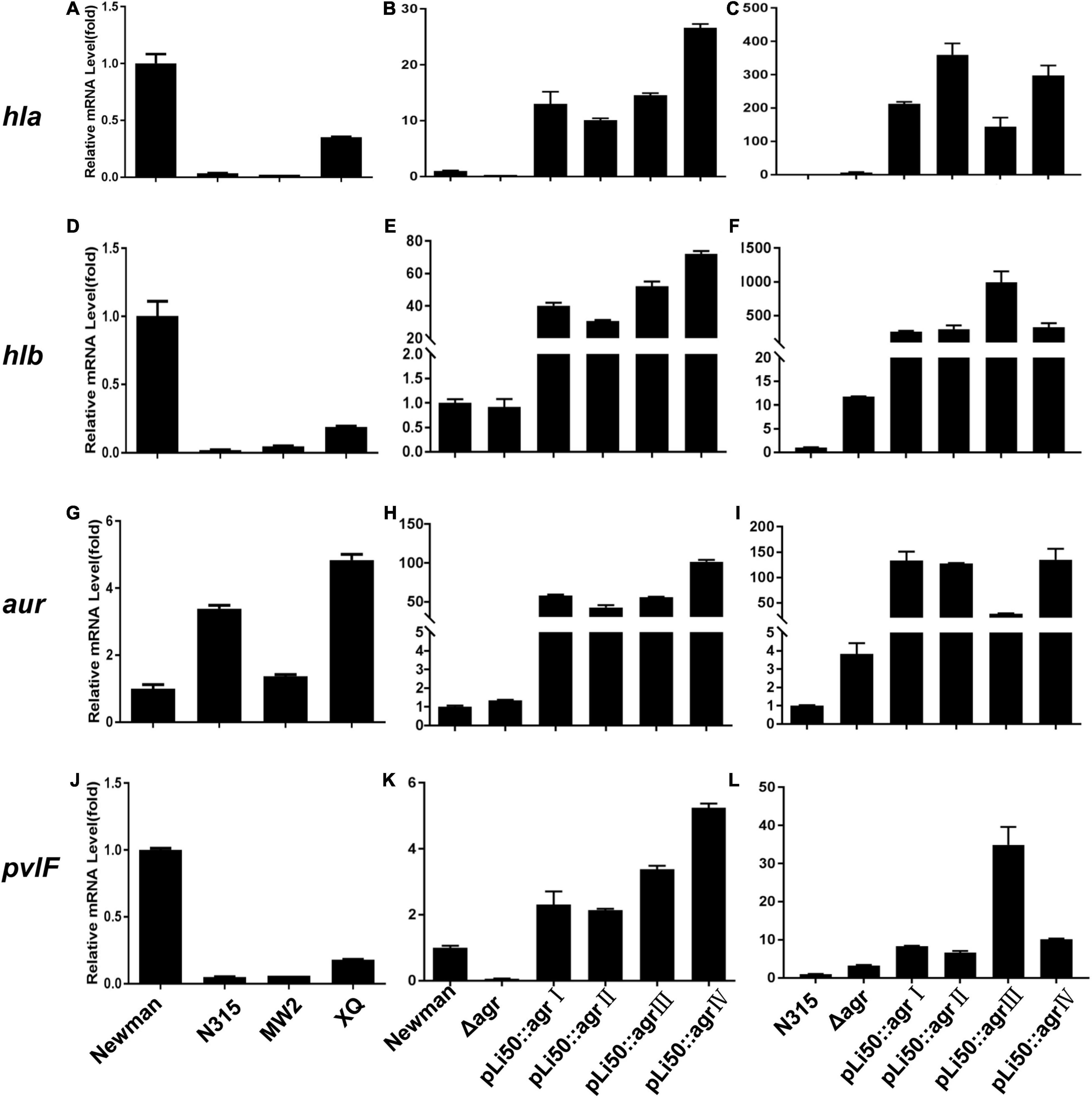
Figure 6. Effects of agr alleles on virulence gene transcriptions of S. aureus. The relative transcription levels of hla (A–C), hlb (D–F), aur (G–I), and pvlF (J–L) among the four wild agr allelic strains (agrI/Newman, agrII/N315, agrIII/MW2, and agrIV/XQ) (left); agr plasmid complemented congenic strains in Newman (middle); or N315 (right) background were analyzed. Error bars represent the average standard deviation (SD) of three separate experiments.
We found that the transcription of most toxins that are known to be activated by agr system (Bronesky et al., 2016) was now down-regulated in the NewmanΔagrBDC mutant strain; the transcriptional factors and some surface proteins (Foster et al., 2014) that are usually inhibited by agr were now up-regulated. When the agrBDC genes of Newman strain (agrI) were genomically replaced by agrBDC genes from the other three agr alleles, the resulting mutants showed very low agr expression (data not shown). This is consistent with the finding of hemolytic assay, staphyloxanthin formation test, and exoprotein profile analysis. As the agrBDC deletion was complemented by plasmid pLI50, the transcription of agrA and RNAIII of NewmanΔagrBDC returned to the original level of wild Newman (Supplementary Figures 3B,E). While the relative mRNA level of agrA increased 30–50 times and the RNAIII increased about thousand folds compared with that of wild N315 (Supplementary Figures 3C,F), the virulence factor transcription of these allelic complemented strains constructed in the same background varied in the same trend, showing an elimination of agr-dependent difference in identical background (Figure 6 and Supplementary Figure 4). For example, the transcription levels of hla gene of four agr congenic Newman strains increased 15–30 times compared with the wild Newman (Figure 6B), whereas they significantly increased 150–300 times when the four agr alleles were, respectively, complemented in agr defective N315 by plasmid pLI50 (Figure 6C). The hlb mRNA level showed 260- to 990-fold increase in N315 background, whereas only 30- to 72-fold in Newman background (Figures 6E,F). Similar changes were also observed in transcriptions of proteases such as aureolysin-encoding gene (aur, Figures 6H,I) and PVL-encoding gene (pvl, Figures 6K,L). Interestingly, the levels of psmα and psmβ in all the four complemented strains were significantly decreased in the Newman background (Supplementary Figures 4H,K) compared with the wild Newman strain, but increased hundreds or thousands of folds in the N315 background (Supplementary Figures 4I,L).
Discussion
The agr quorum-sensing system is a global regulator in S. aureus and controls the expression of numerous surface molecules, secreted enzymes, and cytotoxins (Ford et al., 2020). The amino acid polymorphisms in AgrB, AgrD, and AgrC separate the agr system into four groups. Strains with divergent agr groups also show different biological phenotypes and virulence factor production. However, the influence factors of the S. aureus agr-specific genotypes and virulence remain poorly understood. To dissect the contributions of four divergent agr alleles on S. aureus biological properties, Geisinger et al. (2012) constructed agr congenic strains by individually inserting different agr allele at the staphylococcal pathogenicity island (SaPI)-1 attC locus site of the same background strain. They found that divergent agr alleles showed different agr activation dynamic and virulence factor production, and the allele-dependent differences are mediated by the polymorphisms in agrBDCA genes (Geisinger et al., 2012). In our study, the agrBDC genes of Newman (agrI) and the agrBDCA genes of N315 (agrII) were, respectively, replaced with those of other three agr groups by in situ genomic substitution or plasmid complementation. As expected, the knockout of agrBDC genes led to significant variations in hemolysis activity, pigment formation, exoprotein expression, and virulence factor expressions of NewmanΔagrBDC mutant. No discernible difference was observed between wild N315 and N315ΔagrBDCA mutant because of the low activity of the N315 agr system under normal condition (Figures 2–6). However, when the three heterologous agr alleles (agrII, agrIII, and agrIV) were individually introduced into the native agr site of NewmanΔagrBDC genome, the hemolytic activity, pigment formation, and exoprotein expression of three congenic strains were almost identical to those of NewmanΔagrBDC mutant. In addition, the agrBDCA and promoter regions of four agr alleles were also introduced into S. aureus ΔagrBDC strain (Newman or N315) by plasmid complementation. The hemolysis, exoprotein expression, pigment formation and virulence gene transcription of these plasmid complemented congenic strains were different from the wild strains harboring divergent agr allele (Newman for agrI, N315 for agrII, MW2 for agrIII, and XQ for agrIV) and background strains (Newman or N315), but exactly similar to each other. According to the findings of our study, the congenic strains present similar biological properties when different agr alleles are individually introduced in the same background strain whether by in situ genomic replacement or plasmid complement. It appears that the agr allele–dependent differences were weakened when in an identical background, which is distinct to the study by Geisinger et al. (2012) Perhaps, there are some other factors involved in the presentation of agr-controlled biological phenotype in addition to the polymorphous in agrBDCA genes.
The agr system is a critical regulatory system in S. aureus. Four allelic variants were reported, and each agr variant mediates the autoinduction of its own AIP (Jarraud et al., 2000). The agr cross-inhibition may drive evolutionary diversification in S. aureus. Our results indicate that cross-inhibition driven by agr polymorphisms may be affected by additional unknown factors in addition to the polymorphous of AgrBDCA proteins. It is well known that the S. aureus agr system can regulate the transcriptions of many genes and also be directly and indirectly controlled by other regulators (Queck et al., 2008). For example, SarA (Arya and Princy, 2013), SarU (Manna and Cheung, 2003), and MgrA (Manna and Cheung, 2006) are reported to up-regulate the expression of the agr system, whereas SigB (Bischoff et al., 2001), SarX (Manna and Cheung, 2006), and CodY (Majerczyk et al., 2010) may lead to the down-regulation of the agr system. The transcription levels of sarA and sigB were analyzed in this study (data not shown). Unfortunately, no direct correlation was observed between the expressions of these regulators and the similar phenotypes of agr allelic congenic strains. More in-depth studies are needed to reveal the putative factors and regulation mechanism, which may improve our understanding of the S. aureus agr system.
Taken together, our results further indicate that diversities of AgrB, AgrD, and AgrC contribute to the allele-dependent differences in agr-regulated activities in S. aureus. Additional unknown factors may also interfere with the agr-regulated phenotypes in S. aureus.
Data Availability Statement
The raw data supporting the conclusions of this article will be made available by the authors, without undue reservation.
Author Contributions
SL, XH, and XR conceived and designed this study. LT, SL, and YH carried out the experiments and analyzed the results. YW, ZH, and YR gave important suggestions to the data interpretation. WS, YY, and HP provided guidance in performing the experiments. XR, QH, ML, and KC provided guidance on the ideas for the study. All authors discussed the results and commented on the manuscript.
Funding
This work was supported by the National Natural Science Foundation of China (grant nos. 31470241, 31570127, and 31900118).
Conflict of Interest
The authors declare that the research was conducted in the absence of any commercial or financial relationships that could be construed as a potential conflict of interest.
Publisher’s Note
All claims expressed in this article are solely those of the authors and do not necessarily represent those of their affiliated organizations, or those of the publisher, the editors and the reviewers. Any product that may be evaluated in this article, or claim that may be made by its manufacturer, is not guaranteed or endorsed by the publisher.
Supplementary Material
The Supplementary Material for this article can be found online at: https://www.frontiersin.org/articles/10.3389/fmicb.2022.700894/full#supplementary-material
Supplementary Figure 1 | The amino acids comparisons of AgrB (A), AgrD (B), and AgrC (C) among the four S. aureus agr allelic strains: agrI (Newman), agrII (N315), agrIII (MW2), and agrIV (XQ). The sequences were obtained from NCBI (National Center for Biotechnology Information) database and aligned with the Clustal X and DNAMAN software. The blast results show that the amino acid sequences of AgrB, AgrD, and AgrC are variable.
Supplementary Figure 2 | The comparisons of AgrA (A), RNAIII (B), and promoter sequences (C) among the four S. aureus agr allelic strains: agrI (Newman), agrII (N315), agrIII (MW2), and agrIV (XQ). The sequences were obtained from NCBI (National Center for Biotechnology Information) database (http://www.ncbi.nlm.nih.gov) and aligned with the Clustal X and DNAMAN software. The blast results show that the AgrA, RNAIII, and promoter region are highly conserved among the four S. aureus agr allelic strains.
Supplementary Figure 3 | Effects of agr alleles on agr transcriptions. The total bacterial RNA was isolated in mid-log phase, and gene transcription was appraised with quantitative RT-PCR. The transcription of agrA (A–C) and RNAIII (D–F) among the four wild agr allele strains (agrI/Newman, agrII/N315, agrIII/MW2, and agrIV/XQ) (left) and agr plasmid complemented congenic strains in Newman (middle) or N315 (right) background were analyzed. Error bars represent the average standard deviation (SD) of three separate experiments.
Supplementary Figure 4 | Effects of agr alleles on virulence gene transcriptions of S. aureus. The total bacterial RNA was isolated in mid-log phase, and gene transcription was appraised with quantitative RT-PCR. The transcription of hlgB (A–C), hld (D–F), psmα (G–I), and psmβ (J–L) among the four wild agr allelic strains (agrI/Newman, agrII/N315, agrIII/MW2, and agrIV/XQ) (left); agr plasmid complemented congenic strains in Newman (middle); or N315 (right) background were analyzed. Error bars represent the average standard deviation (SD) of three separate experiments.
References
Arya, R., and Princy, S. (2013). An insight into pleiotropic regulators Agr and Sar: molecular probes paving the new way for antivirulent therapy. Future Microbiol. 8, 1339–1353. doi: 10.2217/fmb.13.92
Bernabè, G., Dal Pra, M., Ronca, V., Pauletto, A., Marzaro, G., Saluzzo, F., et al. (2021). A novel Aza-derivative inhibits quorum sensing signaling and synergizes Methicillin-Resistant Staphylococcus aureus to clindamycin. Front. Microbiol. 12:610859. doi: 10.3389/fmicb.2021.610859
Bischoff, M., Entenza, J. M., and Giachino, P. (2001). Influence of a functional sigB operon on the global regulators sar and agr in Staphylococcus aureus. J. Bacteriol. 183, 5171–5179. doi: 10.1128/JB.183.17.5171-5179.2001
Bronesky, D., Wu, Z., Marzi, S., Walter, P., Geissmann, T., Moreau, K., et al. (2016). Staphylococcus aureus RNAIII and its regulon link quorum sensing, stress responses, metabolic adaptation, and regulation of virulence gene expression. Ann. Rev. Microbiol. 70, 299–316. doi: 10.1146/annurev-micro-102215-095708
Cheung, G., and Otto, M. (2012). The potential use of toxin antibodies as a strategy for controlling acute Staphylococcus aureus infections. Expert Opin. Ther. Targets 16, 601–612. doi: 10.1517/14728222.2012.682573
Duthie, E., and Lorenz, L. (1952). Staphylococcal coagulase; mode of action and antigenicity. J. Gen. Microbiol. 6, 95–107. doi: 10.1099/00221287-6-1-2-95
Ford, C., Hurford, I., and Cassat, J. (2020). Staphylococcus aureus antivirulence strategies for the treatment of infections: a mini review. Front. Microbiol. 11:632706. doi: 10.3389/fmicb.2020.632706
Foster, T., Geoghegan, J., Ganesh, V., and Höök, M. (2014). Adhesion, invasion and evasion: the many functions of the surface proteins of Staphylococcus aureus. Nat. Rev. Microbiol. 12, 49–62. doi: 10.1038/nrmicro3161
Geisinger, E., Chen, J., and Novick, R. (2012). Allele-dependent differences in quorum-sensing dynamics result in variant expression of virulence genes in Staphylococcus aureus. J. Bacteriol. 194, 2854–2864. doi: 10.1128/jb.06685-11
Gomes, A., Vinga, S., Zavolan, M., and de Lencastre, H. (2005). Analysis of the genetic variability of virulence-related loci in epidemic clones of methicillin-resistant Staphylococcus aureus. Antimicrob. Agents Chemother. 49, 366–379. doi: 10.1128/aac.49.1.366-379.2005
Holtfreter, S., Grumann, D., Schmudde, M., Nguyen, H., Eichler, P., Strommenger, B., et al. (2007). Clonal distribution of superantigen genes in clinical Staphylococcus aureus isolates. J. Clin. Microbiol. 45, 2669–2680. doi: 10.1128/jcm.00204-07
Jarraud, S., Lyon, G., Figueiredo, A., Lina, G., Gérard, L., Vandenesch, F., et al. (2000). Exfoliatin-producing strains define a fourth agr specificity group in Staphylococcus aureus. J. Bacteriol. 182, 6517–6522. doi: 10.1128/jb.182.22.6517-6522.2000
Jarraud, S., Mougel, C., Thioulouse, J., Lina, G., Meugnier, H., Forey, F., et al. (2002). Relationships between Staphylococcus aureus genetic background, virulence factors, agr groups (alleles), and human disease. Infect. Immun. 70, 631–641. doi: 10.1128/iai.70.2.631-641.2002
Ji, G., Beavis, R., and Novick, R. (1995). Cell density control of staphylococcal virulence mediated by an octapeptide pheromone. Proc. Natl. Acad. Sci. U.S.A. 92, 12055–12059. doi: 10.1073/pnas.92.26.12055
Ji, G., Beavis, R., and Novick, R. (1997). Bacterial interference caused by autoinducing peptide variants. Science 276, 2027–2030. doi: 10.1126/science.276.5321.2027
Johansson, C., Rautelin, H., and Kaden, R. (2019). Staphylococcus argenteus and are cytotoxic to human cells due to high expression of alpha-hemolysin Hla. Virulence 10, 502–510. doi: 10.1080/21505594.2019.1620062
Khoramrooz, S., Mansouri, F., Marashifard, M., Malek Hosseini, S., Akbarian Chenarestane-Olia, F., Ganavehei, B., et al. (2016). Detection of biofilm related genes, classical enterotoxin genes and agr typing among Staphylococcus aureus isolated from bovine with subclinical mastitis in southwest of Iran. Microbial. Pathog. 97, 45–51. doi: 10.1016/j.micpath.2016.05.022
Kullik, I., Giachino, P., and Fuchs, T. (1998). Deletion of the alternative sigma factor sigma B in Staphylococcus aureus reveals its function as a global regulator of virulence genes. J. Bacteriol. 180, 4814–4820. doi: 10.1128/JB.00536-07
Liu, H., Shang, W., Hu, Z., Zheng, Y., Yuan, J., Hu, Q., et al. (2018). A novel SigB(Q225P) mutation in Staphylococcus aureus retains virulence but promotes biofilm formation. Emerg. Microbes Infect. 7:72. doi: 10.1038/s41426-018-0078-1
Majerczyk, C., Dunman, P., Luong, T., Lee, C., Sadykov, M., Somerville, G., et al. (2010). Direct targets of CodY in Staphylococcus aureus. J. Bacteriol. 192, 2861–2877. doi: 10.1128/jb.00220-10
Manna, A. C., and Cheung, A. L. (2003). sarU, a sarA homolog, is repressed by SarT and regulates virulence genes in Staphylococcus aureus. Infect. Immun. 71, 343–353. doi: 10.1128/IAI.71.1.343-353.2003
Manna, A. C., and Cheung, A. L. (2006). Expression of SarX, a Negative regulator of agr and exoprotein synthesis, Is activated by MgrA in Staphylococcus aureus. J. Bacteriol. 188, 4288–4299. doi: 10.1128/JB.00297-06
Mei, G. L., Cue, D., Roux, C. M., Dunman, P. M., and Lee, C. Y. (2011). Rsp inhibits attachment and biofilm formation by repressing fnbA in Staphylococcus aureus MW2. J. Bacteriol. 193, 5231–5241. doi: 10.1128/JB.05454-11
Novick, R., Projan, S., Kornblum, J., Ross, H., Ji, G., Kreiswirth, B., et al. (1995). The agr P2 operon: an autocatalytic sensory transduction system in Staphylococcus aureus. Mol. Gen. Genet. 248, 446–458. doi: 10.1007/bf02191645
Pader, V., James, E., Painter, K., Wigneshweraraj, S., and Edwards, A. (2014). The Agr quorum-sensing system regulates fibronectin binding but not hemolysis in the absence of a functional electron transport chain. Infect. Immun. 82, 4337–4347. doi: 10.1128/IAI.02254-14
Pelz, A., Wieland, K., Putzbach, K., Hentschel, P., Albert, K., and Götz, F. (2005). Structure and biosynthesis of staphyloxanthin from Staphylococcus aureus. J. Biol. Chem. 280, 32493–32498. doi: 10.1074/jbc.M505070200
Powers, M., Becker, R., Sailer, A., Turner, J., and Bubeck Wardenburg, J. (2015). Synergistic action of Staphylococcus aureus α-Toxin on platelets and myeloid lineage cells contributes to lethal sepsis. Cell Host Microbe 17, 775–787. doi: 10.1016/j.chom.2015.05.011
Queck, S., Jameson-Lee, M., Villaruz, A., Bach, T., Khan, B., Sturdevant, D., et al. (2008). RNAIII-independent target gene control by the agr quorum-sensing system: insight into the evolution of virulence regulation in Staphylococcus aureus. Mol. Cell 32, 150–158. doi: 10.1016/j.molcel.2008.08.005
Rao, Q., Zhou, K., Zhang, X., Hu, Q., Zhu, J., Chen, Z., et al. (2015). Fatal multiple organ failure in an adolescent due to community-acquired methicillin-susceptible Staphylococcus aureus ST121/agrIV lineage: a case report. Rev. Med. Microbiol. 26:1. doi: 10.1097/MRM.0000000000000050
Reyes-Robles, T., and Torres, V. (2017). Staphylococcus aureus pore-forming toxins. Curr. Topics Microbiol. Immunol. 409, 121–144. doi: 10.1007/82_2016_16
Seilie, E., and Bubeck Wardenburg, J. (2017). Staphylococcus aureus pore-forming toxins: the interface of pathogen and host complexity. Semin. Cell Dev. Biol. 72, 101–116. doi: 10.1016/j.semcdb.2017.04.003
Singh, R., and Ray, P. (2014). Quorum sensing-mediated regulation of staphylococcal virulence and antibiotic resistance. Future Microbiol. 9, 669–681. doi: 10.2217/fmb.14.31
Tan, L., Li, S., Jiang, B., Hu, X., and Li, S. (2018). Staphylococcus aureusTherapeutic Targeting of the Accessory Gene Regulator (agr) System. Front. Microbiol. 9:55. doi: 10.3389/fmicb.2018.00055
Traber, K., Lee, E., Benson, S., Corrigan, R., and Novick, R. (2008). Agr function in clinical Staphylococcus aureus isolates. Microbiology 154, 2265–2274. doi: 10.1099/mic.0.2007/011874-0
Tsompanidou, E., Sibbald, M., Chlebowicz, M., Dreisbach, A., Back, J., van Dijl, J., et al. (2011). Requirement of the agr locus for colony spreading of Staphylococcus aureus. J. Bacteriol. 193, 1267–1272. doi: 10.1128/JB.01276-10
Vandenesch, F., Lina, G., and Henry, T. (2012). Staphylococcus aureus hemolysins, bi-component leukocidins, and cytolytic peptides: a redundant arsenal of membrane-damaging virulence factors? Front. Cell. Infect. Microbiol. 2:12. doi: 10.3389/fcimb.2012.00012
Wang, B., and Muir, T. (2016). Regulation of virulence in Staphylococcus aureus: molecular mechanisms and remaining puzzles. Cell Chem. Biol. 23, 214–224. doi: 10.1016/j.chembiol.2016.01.004
Wieland, B., Feil, C., Gloria-Maercker, E., Thumm, G., Lechner, M., Bravo, J., et al. (1994). Genetic and biochemical analyses of the biosynthesis of the yellow carotenoid 4,4’-diaponeurosporene of Staphylococcus aureus. J. Bacteriol. 176, 7719–7726. doi: 10.1128/jb.176.24.7719-7726.1994
Wright, J., Traber, K., Corrigan, R., Benson, S., Musser, J., and Novick, R. (2005). The agr radiation: an early event in the evolution of staphylococci. J. Bacteriol. 187, 5585–5594. doi: 10.1128/jb.187.16.5585-5594.2005
Xue, L., Chen, Y., Yan, Z., Lu, W., and Zhu, H. (2019). Staphyloxanthin: a potential target for antivirulence therapy. Infect. Drug Resist. 12, 2151–2160. doi: 10.2147/IDR.S193649
Keywords: Staphylococcus aureus, quorum sensing system, accessory gene regulator (agr) alleles, agr polymorphisms, virulence factors
Citation: Tan L, Huang Y, Shang W, Yang Y, Peng H, Hu Z, Wang Y, Rao Y, Hu Q, Rao X, Hu X, Li M, Chen K and Li S (2022) Accessory Gene Regulator (agr) Allelic Variants in Cognate Staphylococcus aureus Strain Display Similar Phenotypes. Front. Microbiol. 13:700894. doi: 10.3389/fmicb.2022.700894
Received: 27 April 2021; Accepted: 14 January 2022;
Published: 25 February 2022.
Edited by:
Alina Maria Holban, University of Bucharest, RomaniaReviewed by:
James Q. Boedicker, University of Southern California, United StatesTao Xu, Fudan University, China
Copyright © 2022 Tan, Huang, Shang, Yang, Peng, Hu, Wang, Rao, Hu, Rao, Hu, Li, Chen and Li. This is an open-access article distributed under the terms of the Creative Commons Attribution License (CC BY). The use, distribution or reproduction in other forums is permitted, provided the original author(s) and the copyright owner(s) are credited and that the original publication in this journal is cited, in accordance with accepted academic practice. No use, distribution or reproduction is permitted which does not comply with these terms.
*Correspondence: Kaisen Chen, chenks100@126.com; Shu Li, 1416234121@qq.com
†These authors have contributed equally to this work