- 1School of Basic Medical Science, Guangzhou University of Chinese Medicine, Guangzhou, China
- 2Laboratory of Orthopaedics and Traumatology, Lingnan Medical Research Center, Guangzhou University of Chinese Medicine, Guangzhou, China
- 3Key Laboratory of Orthopaedics and Traumatology, The First Affiliated Hospital of Guangzhou University of Chinese Medicine, Guangzhou University of Chinese Medicine, Guangzhou, China
- 4Department of Traumatology, the Third Affiliated Hospital of Guangzhou University of Chinese Medicine, Guangzhou, China
MicroRNAs (MiRNAs) are small endogenous non-coding RNAs that bind to the 3′-untranslated region of target genes and promote their degradation or inhibit translation, thereby regulating gene expression. MiRNAs are ubiquitous in biology and are involved in many biological processes, playing an important role in a variety of physiological and pathological processes. MiRNA-21 (miR-21) is one of them. In recent years, miR-21 has received a lot of attention from researchers as an emerging player in orthopedic diseases. MiR-21 is closely associated with the occurrence, development, treatment, and prevention of orthopedic diseases through a variety of mechanisms. This review summarizes its effects on osteoblasts, osteoclasts and their relationship with osteoporosis, fracture, osteoarthritis (OA), osteonecrosis, providing a new way of thinking for the diagnosis, treatment and prevention of these bone diseases.
Introduction
MiRNAs, first discovered by Lee et al. in elegans, are a class of endogenous non-coding small RNAs that play an important regulatory role in gene expression at the post-transcriptional level (Lee et al., 1993; Zhang et al., 2012). The biogenesis of mature miRNA undergoes the following stages. Firstly, primary miRNA (pri-miRNA) is produced in the nucleus by RNA polymerase II/III, then cleaved into precursor miRNA (Pre-miRNA) by the DROSHA-DGCR8 complex, which is exported via the nuclear membrane protein Exportin5 to the cytoplasm. In the cytoplasm, Pre-miRNA is further processed by the RNase III enzyme Dicer and the double-stranded RNA-binding domain proteins TRBP into miRNA duplex, one strand of which is referred to as the miR-5p strand and the other as the miR-3p strand. The duplex is incorporated into the RNA-induced silencing complex (RISC), in which Argonaute (AGO) directs the mature strand (guide strand) to bind to the complementary site in the 3′ untranslated region of the target mRNA, leading to degradation of the target mRNA or inhibition of translation of the target mRNA, thereby regulating gene expression (Sayed and Abdellatif, 2011; Takada and Asahara, 2012; Lo et al., 2013; Báez-Vega et al., 2016; Michlewski and Caceres, 2019; Gareev et al., 2020). The other strand (passenger strand), on the other hand, is degraded due to its low steady-state level. However, there is growing evidence that passenger strands can be accumulated to substantial levels and can also play biological roles as microRNAs (Guo and Lu, 2010; Lo et al., 2013; Doberstein et al., 2014) (Figure 1).
MiRNAs are ubiquitous in all organisms, and it is estimated that miRNAs regulate approximately more than 60% of protein-coding genes (Smirnova et al., 2014), indicating that miRNAs play a vital role in a variety of physiological and pathological processes. Currently, there is increasing evidence that miRNAs are involved in skeletal development and play an important role in regulating bone homeostasis (Gamez et al., 2014; Zhao et al., 2014). Also miRNAs are closely related to a variety of orthopedic diseases, which includes participation in the occurrence and development of bone diseases, and have an impact on the diagnosis, treatment and prognosis of bone diseases (Gordon et al., 2014; Gennari et al., 2017; Yang and Fang, 2017; Feichtinger et al., 2018; van Meurs et al., 2019). MiR-21 is one of the miRNAs that has received a lot of attention as an emerging player in bone diseases. Therefore, this article will review the relationship between miR-21 and osteoblasts, osteoclasts, osteoporosis, fractures, OA and osteonecrosis.
MiR-21 Regulates Osteogenic Differentiation
MiR-21 has a regulatory role in osteogenic differentiation. As we all know, mesenchymal stem cells (MSCs) are a kind of multipotent stem cell with the potential of multi-directional differentiation (Lin et al., 2019). It is the precursor cell of osteoblasts and osteocytes in the process of bone formation (Hou et al., 2021). A variety of mechanisms are involved in regulating the differentiation of MSCs into osteoblasts and osteocytes by miR-21. MiR-21 has been reported to activate the ERK-MAPK signaling pathway, and activation of the ERK-MAPK signaling pathway can promote osteogenesis in MSCs. For example, Yang et al. found that miR-21 promoted osteogenic differentiation of MSCs by suppressing the expression of its target gene SPRY1, a process that indirectly activates the ERK-MAPK signaling pathway (Yang et al., 2013). MiR-21 maintains the activation of ERK-MAPK signaling by decreasing the level of SPRY2, thereby increasing the expression of osteogenic differentiation-related transcription factors (Mei et al., 2013). Valproic acid, a flavonoid, activates the ERK/MAPK signaling pathway by upregulating the expression of mir-21 in mouse MSCs, thereby promoting the differentiation of mouse MSCs into osteoblasts (Akshaya et al., 2021). Furthermore, in fetal amniotic fluid-derived MSCs, induction of miR-21 expression accelerates bone formation, a process associated with miR-21 suppressing the expression of the transcription factor SOX2 and regulating the differentiation properties of amniotic fluid-derived MSCs (Trohatou et al., 2014). In human umbilical cord mesenchymal stem cells (HUMSCs), miR-21 promotes osteogenic differentiation by inhibiting PTEN and activating the PI3K-AKT-GSK3β pathway (Meng et al., 2015). MiR-21 can also promote osteogenic differentiation of bone marrow mesenchymal stem cells (BMMSCs) by targeting the SMAD7-SMAD1/5/8-RUNX2 pathway (Li et al., 2017).
In addition, miR-21 positively regulates the osteogenic differentiation of MC3T3-E1 cells, murine multilineage cells (MMCs) and periodontal ligament cells (PDLCs). In MC3T3-E1 cells, icariin attenuated the inhibitory effect of titanium particles on osteoblast differentiation and matrix mineralization by upregulating miR-21-5p (A mature sequence from the 5′ end of the miR-21 stem-loop precursor for the miR-21 guide strand) expression, revealing the promotional role of miR-21-5p in osteoblast differentiation and mineralization (Lian et al., 2018). Oka et al. further demonstrated that miR-21 positively regulated osteogenic differentiation and mineralization by promoting the expression of key osteogenic factors ALP, RUNX2, OPN and OSX in MC3T3-E1 cells, and this result was verified in miR-21 knockout mice (Oka et al., 2021). Similarly, in MC3T3-E1 cells, miR-21 promotes osteogenic differentiation by inhibiting the translation of SMAD7 protein (Li et al., 2015). And in MMCs cells, SONG et al. further showed that by upregulating miR-21 expression, the level of SMAD7 could be reduced to maintain the activation of BMP9/SMAD signaling, thus promoting osteogenic differentiation (Song et al., 2015). In 2012, Li et al. initially found that miR-21 regulates the expression of PLAP-1, and they are inversely correlated, while PLAP-1 plays a negative role in osteogenic differentiation in maintaining the homeostasis of the periodontium. This finding suggests that miR-21 may be involved in the osteogenic differentiation of PDLCs (Li et al., 2012). Later, miR-21 was further demonstrated to promote stretch-induced osteogenic differentiation in human periodontal ligament stem cells (HPDLSCs), and this effect may be achieved by miR-21 inhibiting the expression of its target gene ACVR2B (Wei et al., 2015). Also in HPDLSCs, tumor necrosis factor-α (TNF-α) inhibition of miR-21 expression impaired osteogenic differentiation. The elevated SPRY1 level caused by inhibition of miR-21 may be one of the reasons for the impaired osteogenic differentiation in HPDLSCs (Yang et al., 2017), which is consistent with the previous finding of Yang et al. in MSCs (Yang et al., 2013).
However, some of the studies were not consistent with these results. Sheng et al. detected that downregulation of miR-21 in a rabbit tibial fracture model promoted osteoblast proliferation by positively regulating the expression of growth factors downstream of the ERK signaling pathway (Sheng et al., 2019). MiR-21-5p was raised in glucocorticoid-induced rat BMMSCs, and the overexpression of miR-21-5p significantly suppressed osteogenic differentiation and proliferation of BMMSCs and promoted apoptosis (Hao et al., 2021) (Table 1; Figure 2A).
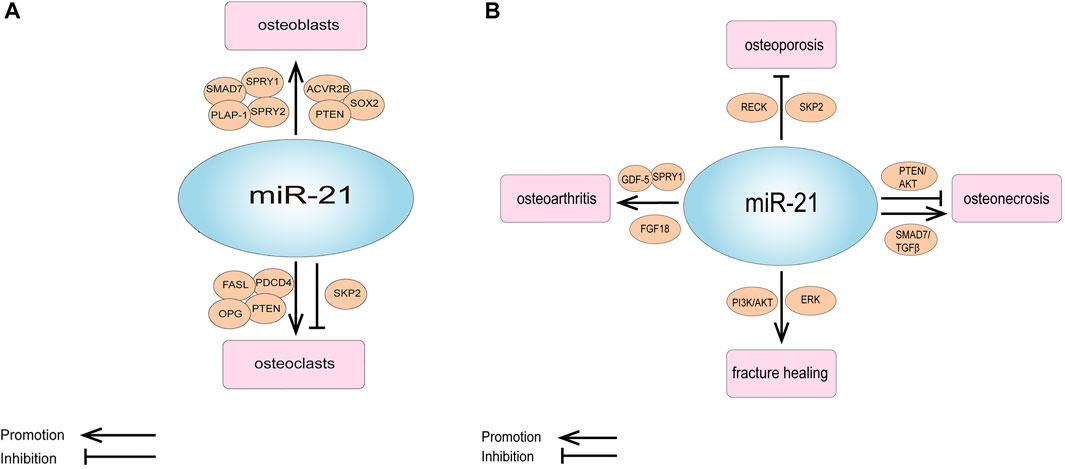
FIGURE 2. (A) Schematic representation of the role of miR-21 and its targets in osteogenesis and osteoclastic. (B) Schematic representation of the role of miR-21 and its targets in orthopedic diseases.
MiR-21 Regulates Osteoclastic Differentiation
The regulatory effects of miR-21 on osteoclasts are complex and involve multiple mechanisms. First, the study by Sugatani et al. showed that miR-21 expression was upregulated during RANKL-induced osteoclastogenesis, while they proposed a new molecular mechanism regarding osteoclastogenesis, namely the C-Fos/miR-21/PDCD4 positive feedback loop. C-Fos upregulated miR-21 expression and downregulated PDCD4 protein expression, and attenuated PDCD4 eliminated the inhibitory effect of C-Fos, which in turn promoted osteoclastogenesis (Sugatani et al., 2011). This positive feedback loop was also validated in the mouse model of particle-induced osteolysis (Zhou et al., 2012). Later, using miR-21 knockout mice models, miR-21 was shown to promote bone resorption in vivo by directly regulating osteoclast function through targeting PDCD4 (Hu et al., 2017). Lung adenocarcinoma cell-derived exosomal miR-21 also promotes osteoclast formation by targeting PDCD4 (Xu et al., 2018). In periodontal accelerated osteogenesis orthodontics (PAOO) facilitated orthodontic tooth movement (TM), the positive feedback loop of C-Fos/miR-21/PDCD4 leads to an increase in osteoclast production, thus increasing movement of orthodontic teeth (Zhang Y. et al., 2020). Second, Wang et al. confirmed by bioinformatics and dual-luciferase reporter assays that PTEN is a target gene of miR-21, which promotes osteoclast formation and bone resorption by targeting Pten to activate the PI3K/AKT signaling pathway (Wang et al., 2020). Circulating miR-21 of lung cancer cell origin also targets PTEN and promotes osteoclastogenesis (Zhao et al., 2020). However, an inhibitory effect of miR-21 on osteoclast differentiation has also been reported, for instance, Huang et al. investigated that miR-21-5p was significantly decreased during osteoclast differentiation and that miR-21-5p inhibited osteoclast differentiation by acting on its target gene SKP2 (Huang et al., 2021). In juvenile idiopathic arthritis, miR-21 could inhibit the production of osteoclasts induced from rheumatoid arthritis fibroblast-like synovial cells by M-CSF (Li and Zeng, 2020).
MiR-21 also affects osteoclasts by influencing the RANKL/OPG ratio. It was shown that OPG is a potential target of miR-21. MiR-21 expression was significantly enhanced in bone marrow stromal cells (BMSCs) adherent to multiple myeloma cells, while OPG expression was significantly reduced. Inhibition of miR-21 expression restored the RANKL/OPG balance and significantly impaired the resorptive activity of mature osteoclasts (Pitari et al., 2015). In the miR-21 knockout mice orthodontic TM model, miR-21 was revealed to enhance osteoclast differentiation by inducing RANKL secretion from activated T cells, which in turn regulated the RANKL/OPG balance and partially relieved the decreased orthodontic TM distance in miR-21 knockout mice (Wu et al., 2020). A study by Li et al. yielded different findings. They demonstrated that in the presence of mechanically induced maxillary expansion, by comparing miR-21 knockout and wild-type mice, it was found that miR-21 deficiency induced more osteoclasts by downregulating OPG expression and upregulating RANKL expression. And after intraperitoneal injection of agomir-21 to miR-21 knockout mice, the OPG/RANKL ratio was rescued (Li et al., 2020). Clinical studies have discovered that serum miR-21 is positively correlated with RANKL levels and negatively correlated with OPG levels in postmenopausal hypoestrogenic women with osteoporosis (PMOP). Moreover, MiR-21 overexpression leads to an increase in RANKL/OPG ratio, and a higher RANKL/OPG ratio increases the number of osteoclasts and bone resorption activity (Suarjana et al., 2019).
MiR-21 is also involved in osteoclast apoptosis. It has been reported that estrogen causes osteoclast apoptosis by down-regulating miR-21 biogenesis and increasing the post-transcriptional levels of the FasL protein, the target of miR-21 (Sugatani and Hruska, 2013). MiR-21 was also found to interact directly with lncRNA GAS5 to promote apoptosis in osteoclasts (Cong et al., 2020) (Table 2; Figure 2A).
Potential Role of miR-21 in Diagnosis and Treatment of Osteoporosis
Osteoporosis is a bone disease with a high prevalence, which is common in the elderly, especially in postmenopausal women. Osteoporosis is characterized by decreased bone mass and destruction of bone tissue microstructure, resulting in increased bone brittleness and fracture risk. It has brought a serious economic burden to human society (Wu et al., 2021). The clinical diagnosis of osteoporosis is mainly based on bone mineral density (BMD). Low BMD often increases the risk of osteoporosis. The imbalance between osteoblast-induced bone formation and osteoclast-induced bone resorption plays an important role in the pathogenesis of osteoporosis (Khosla et al., 2011; Lu et al., 2021). MiR-21 has effects on both osteoblasts and osteoclasts, so miR-21 may also play an important role in osteoporosis.
In laboratory studies, Zhao et al. showed that miR-21 regulates osteoporosis by targeting RECK. They established a cell model of osteoporosis by adding TNF-α to the medium of MSCs and found that miR-21 mimics as well as RECK siRNA attenuated the effects of TNF-α on apoptosis, proliferation and differentiation of MSCs, and increased the expression of MT1-MMP. A luciferase reporter gene assay showed that RECK was a direct target of miR-21. They further used ovariectomy (OVX) mouse model of osteoporosis, and discovered that the expression of miR-21 decreased while RECK increased in the OVX mice; when treatment with lentiviral RECK shRNA, the osteoporosis of OVX mice could be inhibited (Zhao et al., 2015). In addition, miR-21 can also regulate osteoporosis by affecting osteoclastogenesis, as mentioned earlier in the study by Huang et al. who noticed that miR-21-5p targeting SKP2 inhibited osteoclast differentiation. They also revealed that miR-21-5p treatment inhibited bone resorption and maintained bone cortex and trabecular structures (Huang et al., 2021). All of these results suggest that miR-21 is a new target for the treatment of osteoporosis (Figure 2B; Table 3).
In clinical studies, miR-21 is differentially expressed in patients with osteoporosis and may serve not only as a new biomarker for the diagnosis of osteoporosis, but also as a potential target for therapeutic inhibition. Li et al. measured the level of miR-21 in plasma of 120 Chinese postmenopausal women and divided them into normal group, osteopenia group and osteoporosis group. MiR-21 expression was downregulated in plasma of patients with osteoporosis and osteopenia compared with the normal group, and plasma miR-21 levels were positively correlated with BMD (Li et al., 2014). Yavropoulou et al. examined the serum miR-21 level in 100 postmenopausal women and found that the expression of miR-21-5p in serum of postmenopausal women with low bone mass, at least one case of moderate vertebral fracture and low bone mass without fracture was significantly lower than that of the control group with normal BMD and no history of fracture (Yavropoulou et al., 2017). Zhao et al. collected bone tissue and serum from 48 osteoporotic patients and 48 normal subjects, concluded that miR-21 was expressed at low levels in bone tissue and serum in osteoporotic patients (Zhao et al., 2019).
However, the results of some clinical studies are not consistent with the above results. Seeliger et al. and Kelch et al. showed that miR-21-5p was significantly up-regulated in serum and bone tissue of osteoporotic patients, and Kelch et al. also studied miR-21-5p in bone tissue was negatively correlated with BMD (Seeliger et al., 2014; Kelch et al., 2017). Perksanusak et al. analyzed the expression of miR-21 in the plasma of postmenopausal women in Thailand and found that miR-21 expression was significantly higher in the low BMD group (osteopenia and osteoporosis) compared to the normal BMD group, and miR-21-5p was mildly negatively correlated with BMD (Perksanusak et al., 2018). By comparing 60 postmenopausal women with hypoestrogenism with osteoporosis and 60 postmenopausal women with hypoestrogenism without osteoporosis, Suarjana et al. revealed that the expression of serum miR-21 was higher in postmenopausal hypoestrogenism with osteoporosis than in non-osteoporotic patients and was negatively correlated with spinal BMD (Suarjana et al., 2019) (Table 4).
The above findings reveal that the role of miR-21 in regulating osteogenic differentiation and osteoclast formation remains controversial, due to the different regulatory mechanisms. Apart from that, it may also be related to the number of patients involved in each study, different research groups, etc. First of all, the number of patients involved in the above study is relatively small, so it is necessary to further clarify the diagnostic value of miR-21 in osteoporosis in larger samples. Secondly, the subjects selected in the above studies are not the same. For example, fracture patients were included in the studies of Seeliger et al. and Kelch et al. (Seeliger et al., 2014; Kelch et al., 2017), but not in the studies of Li et al. and Zhao et al. (Li et al., 2014; Zhao et al., 2019). Fracture is a complex biological process that leads to an imbalance in the endoskeletal dynamics of the body and also leads to significant changes in the signaling pathways of the molecules involved in the bone reconstruction process, thus having a significant impact on the expression level of miR-21. Also, different inclusion and exclusion criteria for study subjects in each study as well as different methods of measuring outcomes during the study can have an impact on miR-21 expression. Suarjana et al. detected the level of estrogen in the subjects. However, estrogen inhibits miR-21 expression and induces apoptosis in osteoclasts (Sugatani and Hruska, 2013). In summary, due to the inconsistent results of miR-21, more studies are needed to confirm the clinical application of miR-21 to determine whether it can be used as an indicator to assess the occurrence of osteoporosis and as a potential target for the treatment of osteoporosis.
The Regulatory Role of miR-21 in Fracture
Fracture is a common clinical disease, which refers to the loss of bone integrity, pain, swelling and dysfunction at the fracture site. Although bone has the ability to reconstruct and repair itself, there are still about 5–10% of fractures result in delayed unions or non-unions. The disorder of fracture healing will cause not only personal losses, but also economic losses (Jayankura et al., 2021). Therefore, it is necessary to deeply study the mechanism of promoting fracture healing in order to lighten the burden for human society.
As we all know, Fracture healing is a complex biological process, which includes the inflammation stage, callus stage and bone remodeling stage (Hadjiargyrou and O'Keefe, 2014; Liao et al., 2017). Studies have shown that miR-21 plays an important role in the above stages. Sun et al. discovered that in a rat osteoporotic fracture model, local injection of miR-21 nanocapsules promoted early bone repair in osteoporotic fractures, thereby accelerating bone healing into the molding phase earlier (Sun et al., 2020). Overexpression of miR-21 in a rat fracture model was found to accelerate endochondral ossification, increase the volume of callus and restore biomechanical strength of femur fractures (Sun et al., 2015). Liu et al. obtained the same results and found that miR-21 promotes fracture healing in rats by activating PI3K/AKT signaling pathway (Liu et al., 2019). However, in a rabbit tibial fracture model, miR-21 downregulation activated the ERK signaling pathway, which promoted the proliferation of osteoblasts and provided collagen and fibrous connective tissue required for fracture healing, thus shortening the formation time of bone callus and accelerating fracture healing (Sheng et al., 2019). The above different results show that miR-21 has a complex regulation mechanism in the process of fracture healing. Therefore, we need to analyze its role in fracture healing more deeply and comprehensively, to lay a good foundation for clinical research (Figure 2B; Table 3).
It is well known that osteoporosis increases the risk of fracture. Clinical studies have reported that miR-21 is also differentially expressed in patients with osteoporotic fracture and is related to bone turnover markers. This represents a possible association of miR-21 with an increased risk of osteoporotic fractures, while providing a new target for the prevention and treatment of osteoporotic fractures. The study of Seeliger et al. and Kelch et al. found that miR-21 was significantly up-regulated in patients with osteoporotic hip fracture (Seeliger et al., 2014; Kelch et al., 2017), and Panach et al. got the same results, in further, they showed that miR-21-5p was positively correlated with serum C-telopeptide (CTx) levels (Panach et al., 2015). Zarecki et al. detected significant upregulation of miR-21 in patients with vertebral fractures and low BMD, but they did not find any correlation between miR-21-5p and CTx (Zarecki et al., 2020). The findings of Yavropoulou et al. were contrary to the three studies mentioned above, and serum miR-21-5p levels were lower in patients who had suffered at least one vertebral fracture compared to those who had no fracture (Yavropoulou et al., 2017) (Table 4).
These opposite results may be related to the different control groups selected in the study population. Seeliger et al. and Kelch et al. chose a control group that included patients with fractures, while Panach et al. chose a control group of women with severe hip osteoarthritis requiring hip prosthesis implantation. Zarecki et al. and Yavropoulou et al. used postmenopausal women with normal BMD values and no fractures as a control group. Both fracture and OA affect miR-21 expression in vivo. In addition, Panach et al. found that there was a positive correlation between miR-21-5p and CTx levels, which also reflected that miR-21 was closely related to osteoclast production, but Zarecki et al. did not find any correlation between miR-21-5p and CTx, which indicated that the correlation between bone transition markers and miR-21 was not consistent, so more large-scale studies may be needed to further confirm the correlation between them.
The Regulatory Role of miR-21 in OA
OA is a chronic debilitating disease that affects millions of people around the world (Ghouri and Conaghan, 2020). Joint pain, stiffness, swelling and dysfunction caused by it have brought great physical and mental damage to patients. Progressive destruction of articular cartilage is a major feature of OA (Sun et al., 2021).
MiR-21 is reported to be differentially expressed in OA cartilage and regulates cartilage degeneration. Clinical studies have shown that miR-21 is expressed at lower levels in cartilage from OA patients compared to normal cartilage biopsy, and that inhibition of miR-21 led to apoptosis of chondrocytes and degeneration of cartilage (Song et al., 2014). In contrast, Wang et al. identified significant upregulation of miR-21-5p expression in OA cartilage tissue compared to trauma patients without a history of OA (Wang et al., 2019). Zhang et al. reported that the expression level of miR-21 in the cartilage of patients with OA is higher than that of traumatic amputees. Meanwhile, they investigated the effect of miR-21 on chondrogenesis in a chondrocyte cell line and found that miR-21 promotes OA pathogenesis by targeting GDF-5. MiR-21 inhibits the expression of GDF-5 and its overexpression attenuates the progression of OA (Zhang et al., 2014). This finding was validated in the mouse temporomandibular joint osteoarthritis (TMJOA) model, where Zhang et al. claimed that knockdown of miR-21-5p reduced cartilage matrix degradation in TMJOA by targeting GDF-5 (Zhang A. et al., 2020). Also in the mouse TMJOA model, researchers discovered that miR-21-5p knockout mice had less temporomandibular joint cartilage destruction than wild-type mice, and in vitro experiments showed that miR-21-5p promotes extracellular matrix degradation and angiogenesis in TMJOA by suppressing the expression of target gene SPRY1, which in turn promotes the development of TMJOA (Ma et al., 2020). Furthermore, miR-21-5p upregulation also initiates and promotes OA by targeting FGF18, and intra-articular injection of antagomiR-21 attenuates cartilage degeneration in OA model mice, suggesting that targeting miR-21-5p is a promising option for the treatment of OA (Wang et al., 2019). However, the laboratory findings of Zhu et al. were not consistent with the above, as they discovered that miR-21-5p was significantly downregulated in OA chondrocytes and, more importantly, that miR-21-5p expression levels were negatively correlated with cartilage degeneration. Upregulation of miR-21-5p in OA chondrocytes improved changes in cartilage extracellular matrix-associated factors. These results suggest that miR-21-5p can act as a disease modifier in OA and play an important role in the pathological development of OA (Zhu et al., 2019).
Pain is the most prominent symptom in patients with OA (Abramoff and Caldera, 2020). MiR-21 was also associated with chronic pain in OA. MiR-21 was highly expressed in the synovial tissue and synovial fluid of OA model rats, and extracellular miR-21 released from synovial tissue caused knee joint pain in OA model rats through activation of TLR7. Notably, intra-articular injection of miR-21 inhibitors or TLR7-9 antagonists alone provided the long-term relief of pain in OA model rats. Therefore, extracellular miR-21 may be a possible target for OA pain treatment (Hoshikawa et al., 2020) (Figure 2B; Tables 3, 4).
MiR-21 Involvement in the Diagnosis and Treatment of Osteonecrosis
Osteonecrosis is a common and refractory disease in orthopedics, which is caused by temporary or permanent interruption of blood supply in the affected bone area, resulting in bone structure collapse, joint pain and loss of related function (Lespasio et al., 2019).
Abnormal expression of miR-21 in osteonecrosis may be relevant to the diagnosis and treatment of osteonecrosis. MiR-21-3p (Another mature sequence, from the 3′ end of the miR-21 stem-loop precursor, is the miR-21 passenger strand) was discovered to be upregulated in BMMSCs in mice with steroid-induced osteonecrosis of the femoral head (SIONFH) (Wang et al., 2015). In the rat SIONFH model, miR-21-5p expression was also significantly upregulated, and it was further found circular RNA PVT1 attenuated SIONFH through regulation of the miR-21-5p-mediated Smad7/TGFβ signaling pathway (Hao et al., 2021). Yang et al. established rat bisphosphonate-related osteonecrosis of the jaw (BRONJ) model and found that miR-21 expression was upregulated in the serum of BRONJ rats, and miR-21 together with miR-23 and miR-145 could be used as a combined indicator for diagnosing or predicting the initiation and development of BRONJ. They obtained the same result in the serum of BRONJ patients (Yang et al., 2018). Another clinical study detected the total RNAs of circulating lymphocytes in healthy people and in multiple myeloma patients with BRONJ, they found that the expression profile of miRNA changed, and the expression level of 14 miRNAs increased in multiple myeloma patients with BRONJ. Targeting these miRNAs can provide a new opportunity for the prevention or treatment of BRONJ, and miR-21 is one of them (Musolino et al., 2018). In addition, miR-21 is also involved in osteocyte apoptosis in osteonecrosis. Kuang et al. showed that human Wharton’s jelly of umbilical cord mesenchymal stem cells (hWJ-MSCs) derived exosomes inhibit osteocyte apoptosis in glucocorticoid-induced osteonecrosis of the femoral head (GIONFH) in rats, and this effect was achieved through the miR-21-PTEN-AKT signaling pathway, which provides a new idea for the treatment of GIONFH (Kuang et al., 2019) (Figure 2B; Tables 3, 4).
Conclusions and Perspectives
To sum up, miR-21 is a multi-target miRNA that plays an important role in bone metabolism, affecting the differentiation of osteoblasts and osteoclasts, and is closely related to osteopathic diseases such as osteoporosis, fracture, osteoarthritis and osteonecrosis. Firstly, there is differential expression of miR-21 in patients with osteoporosis, which can not only be used as a new biomarker for the diagnosis of osteoporosis, but also provide a new potential target for the treatment of osteoporosis. Secondly, miR-21 is also differentially expressed in patients with osteoporotic fractures, which may be related to the increased risk of osteoporotic fracture. MiR-21 can also promote fracture healing through a variety of mechanisms. Thirdly, in OA, miR-21 is differentially expressed in articular cartilage and thus regulates cartilage degeneration, providing a new target for the treatment of OA, and miR-21 is also associated with chronic pain caused by OA. Finally, miR-21 also showed changes in expression levels in osteonecrosis, which suggests that miR-21 may be relevant to the diagnosis and treatment of osteonecrosis. The function of miR-21 is complex and controversial, and the development, progression, and treatment of these orthopedic diseases is also a complex biological process that involves multiple cell types, multiple signaling pathways, and changes in the expression of related factors. Furthermore, clinical studies are inconsistent due to individual differences, many variables and difficulty to control, etc. Therefore, to further clarify the effect of miR-21 on osteoblasts and osteoclasts and its role in the above orthopedic diseases needs to be studied in depth.
Author Contributions
CC and L-LX designed and conceptualized the review. CC and B-LF collected and organized the relevant literature, and then CC and Y-ML finished writing the manuscript. L-LX and BW guided the writing, created resources, reviewed, revised and finally approved the manuscript.
Funding
This study was supported by the National Natural Science Foundation of China (Grant No. 81873326).
Conflict of Interest
The authors declare that the research was conducted in the absence of any commercial or financial relationships that could be construed as a potential conflict of interest.
Publisher’s Note
All claims expressed in this article are solely those of the authors and do not necessarily represent those of their affiliated organizations, or those of the publisher, the editors and the reviewers. Any product that may be evaluated in this article, or claim that may be made by its manufacturer, is not guaranteed or endorsed by the publisher.
References
Abramoff, B., and Caldera, F. E. (2020). Osteoarthritis: Pathology, Diagnosis, and Treatment Options. Med. Clin. North. Am. 104, 293–311. doi:10.1016/j.mcna.2019.10.007
Akshaya, N., Prasith, P., Abinaya, B., Ashwin, B., Chandran, S. V., and Selvamurugan, N. (2021). Valproic Acid, A Potential Inducer of Osteogenesis in Mouse Mesenchymal Stem Cells. Curr. Mol. Pharmacol. 14, 27–35. doi:10.2174/1874467213666200713102410
Báez-Vega, P. M., Echevarría Vargas, I. M., Valiyeva, F., Encarnación-Rosado, J., Roman, A., Flores, J., et al. (2016). Targeting miR-21-3p Inhibits Proliferation and Invasion of Ovarian Cancer Cells. Oncotarget 7, 36321–36337. doi:10.18632/oncotarget.9216
Cong, C., Tian, J., Gao, T., Zhou, C., Wang, Y., Cui, X., et al. (2020). lncRNA GAS5 Is Upregulated in Osteoporosis and Downregulates miR-21 to Promote Apoptosis of Osteoclasts. Clin. Interv. Aging 15, 1163–1169. doi:10.2147/CIA.S235197
Doberstein, K., Bretz, N. P., Schirmer, U., Fiegl, H., Blaheta, R., Breunig, C., et al. (2014). miR-21-3p Is a Positive Regulator of L1CAM in Several Human Carcinomas. Cancer Lett. 354, 455–466. doi:10.1016/j.canlet.2014.08.020
Feichtinger, X., Muschitz, C., Heimel, P., Baierl, A., Fahrleitner-Pammer, A., Redl, H., et al. (2018). Bone-related Circulating MicroRNAs miR-29b-3p, miR-550a-3p, and miR-324-3p and Their Association to Bone Microstructure and Histomorphometry. Sci. Rep. 8, 4867. doi:10.1038/s41598-018-22844-2
Gámez, B., Rodriguez-Carballo, E., and Ventura, F. (2014). MicroRNAs and post-transcriptional Regulation of Skeletal Development. J. Mol. Endocrinol. 52, R179–R197. doi:10.1530/JME-13-0294
Gareev, I., Beylerli, O., Yang, G., Sun, J., Pavlov, V., Izmailov, A., et al. (2020). The Current State of MiRNAs as Biomarkers and Therapeutic Tools. Clin. Exp. Med. 20, 349–359. doi:10.1007/s10238-020-00627-2
Gennari, L., Bianciardi, S., and Merlotti, D. (2017). MicroRNAs in Bone Diseases. Osteoporos. Int. 28, 1191–1213. doi:10.1007/s00198-016-3847-5
Ghouri, A., and Conaghan, P. G. (2020). Prospects for Therapies in Osteoarthritis. Calcif Tissue Int. doi:10.1007/s00223-020-00672-9
Gordon, J. A., Montecino, M. A., Aqeilan, R. I., Stein, J. L., Stein, G. S., and Lian, J. B. (2014). Epigenetic Pathways Regulating Bone Homeostasis: Potential Targeting for Intervention of Skeletal Disorders. Curr. Osteoporos. Rep. 12, 496–506. doi:10.1007/s11914-014-0240-1
Guo, L., and Lu, Z. (2010). The Fate of miRNA* Strand through Evolutionary Analysis: Implication for Degradation as Merely Carrier Strand or Potential Regulatory Molecule? PLoS One 5, e11387. doi:10.1371/journal.pone.0011387
Hadjiargyrou, M., and O'Keefe, R. J. (2014). The Convergence of Fracture Repair and Stem Cells: Interplay of Genes, Aging, Environmental Factors and Disease. J. Bone Miner Res. 29, 2307–2322. doi:10.1002/jbmr.2373
Hao, Y., Lu, C., Zhang, B., Xu, Z., Guo, H., and Zhang, G. (2021). CircPVT1 Up-Regulation Attenuates Steroid-Induced Osteonecrosis of the Femoral Head through Regulating miR-21-5p-Mediated Smad7/TGFβ Signalling Pathway. J. Cel Mol Med. 25, 4608–4622. doi:10.1111/jcmm.16294
Hoshikawa, N., Sakai, A., Takai, S., and Suzuki, H. (2020). Targeting Extracellular miR-21-TLR7 Signaling Provides Long-Lasting Analgesia in Osteoarthritis. Mol. Ther. Nucleic Acids 19, 199–207. doi:10.1016/j.omtn.2019.11.011
Hou, Y., Lin, W., Li, Y., Sun, Y., Liu, Y., Chen, C., et al. (2021). De-osteogenic-differentiated Mesenchymal Stem Cells Accelerate Fracture Healing by Mir-92b. J. Orthopaedic Translation 27, 25–32. doi:10.1016/j.jot.2020.10.009
Hu, C. H., Sui, B. D., Du, F. Y., Shuai, Y., Zheng, C. X., Zhao, P., et al. (2017). miR-21 Deficiency Inhibits Osteoclast Function and Prevents Bone Loss in Mice. Sci. Rep. 7, 43191. doi:10.1038/srep43191
Huang, Y., Yang, Y., Wang, J., Yao, S., Yao, T., Xu, Y., et al. (2021). miR-21-5p Targets SKP2 to Reduce Osteoclastogenesis in a Mouse Model of Osteoporosis. J. Biol. Chem. 296, 100617. doi:10.1016/j.jbc.2021.100617
Jayankura, M., Schulz, A. P., Delahaut, O., Witvrouw, R., Seefried, L., Berg, B. V., et al. (2021). Percutaneous Administration of Allogeneic Bone-Forming Cells for the Treatment of Delayed Unions of Fractures: a Pilot Study. Stem Cel Res Ther. 12, 363. doi:10.1186/s13287-021-02432-4
Kelch, S., Balmayor, E. R., Seeliger, C., Vester, H., Kirschke, J. S., and van Griensven, M. (2017). miRNAs in Bone Tissue Correlate to Bone mineral Density and Circulating miRNAs Are Gender Independent in Osteoporotic Patients. Sci. Rep. 7, 15861. doi:10.1038/s41598-017-16113-x
Khosla, S., Melton, L. J., and Riggs, B. L. (2011). The Unitary Model for Estrogen Deficiency and the Pathogenesis of Osteoporosis: Is a Revision Needed? J. Bone Miner Res. 26, 441–451. doi:10.1002/jbmr.262
Kuang, M. J., Huang, Y., Zhao, X. G., Zhang, R., Ma, J. X., Wang, D. C., et al. (2019). Exosomes Derived from Wharton's Jelly of Human Umbilical Cord Mesenchymal Stem Cells Reduce Osteocyte Apoptosis in Glucocorticoid-Induced Osteonecrosis of the Femoral Head in Rats via the miR-21-PTEN-AKT Signalling Pathway. Int. J. Biol. Sci. 15, 1861–1871. doi:10.7150/ijbs.32262
Lee, R. C., Feinbaum, R. L., and Ambros, V. (1993). The C. elegans Heterochronic Gene Lin-4 Encodes Small RNAs with Antisense Complementarity to Lin-14. Cell 75, 843–854. doi:10.1016/0092-8674(93)90529-y
Lespasio, M. J., Sodhi, N., and Mont, M. A. (2019). Osteonecrosis of the Hip: A Primer. Perm J. 23, 18–100. doi:10.7812/TPP/18-100
Li, C., Li, C., Yue, J., Huang, X., Chen, M., Gao, J., et al. (2012). miR-21 and miR-101 Regulate PLAP-1 Expression in Periodontal Ligament Cells. Mol. Med. Rep. 5, 1340–1346. doi:10.3892/mmr.2012.797
Li, H., Wang, Z., Fu, Q., and Zhang, J. (2014). Plasma miRNA Levels Correlate with Sensitivity to Bone mineral Density in Postmenopausal Osteoporosis Patients. Biomarkers 19, 553–556. doi:10.3109/1354750X.2014.935957
Li, H., Yang, F., Wang, Z., Fu, Q., and Liang, A. (2015). MicroRNA-21 Promotes Osteogenic Differentiation by Targeting Small Mothers against Decapentaplegic 7. Mol. Med. Rep. 12, 1561–1567. doi:10.3892/mmr.2015.3497
Li, H. W., and Zeng, H. S. (2020). Regulation of JAK/STAT Signal Pathway by miR-21 in the Pathogenesis of Juvenile Idiopathic Arthritis. World J. Pediatr. 16, 502–513. doi:10.1007/s12519-019-00268-w
Li, M., Zhang, Z., Gu, X., Jin, Y., Feng, C., Yang, S., et al. (2020). MicroRNA-21 Affects Mechanical Force-Induced Midpalatal Suture Remodelling. Cell Prolif 53, e12697. doi:10.1111/cpr.12697
Li, X., Guo, L., Liu, Y., Su, Y., Xie, Y., Du, J., et al. (2017). MicroRNA-21 Promotes Osteogenesis of Bone Marrow Mesenchymal Stem Cells via the Smad7-Smad1/5/8-Runx2 Pathway. Biochem. Biophys. Res. Commun. 493, 928–933. doi:10.1016/j.bbrc.2017.09.119
Lian, F., Zhao, C., Qu, J., Lian, Y., Cui, Y., Shan, L., et al. (2018). Icariin Attenuates Titanium Particle-Induced Inhibition of Osteogenic Differentiation and Matrix Mineralization via miR-21-5p. Cell Biol Int 42, 931–939. doi:10.1002/cbin.10957
Liao, M. H., Lin, P. I., Ho, W. P., Chan, W. P., Chen, T. L., and Chen, R. M. (2017). Participation of GATA-3 in Regulation of Bone Healing through Transcriptional Upregulation of Bcl-xL Expression. Exp. Mol. Med. 49, e398. doi:10.1038/emm.2017.182
Lin, W., Xu, L., Lin, S., Shi, L., Wang, B., Pan, Q., et al. (2019). Characterisation of Multipotent Stem Cells from Human Peripheral Blood Using an Improved Protocol. J. Orthop. Translat 19, 18–28. doi:10.1016/j.jot.2019.02.003
Liu, Y., Liu, J., Xia, T., Mi, B. B., Xiong, Y., Hu, L. C., et al. (2019). MiR-21 Promotes Fracture Healing by Activating the PI3K/Akt Signaling Pathway. Eur. Rev. Med. Pharmacol. Sci. 23, 2727–2733. doi:10.26355/eurrev_201904_17544
Lo, T. F., Tsai, W. C., and Chen, S. T. (2013). MicroRNA-21-3p, a Berberine-Induced miRNA, Directly Down-Regulates Human Methionine Adenosyltransferases 2A and 2B and Inhibits Hepatoma Cell Growth. PLoS One 8, e75628. doi:10.1371/journal.pone.0075628
Lu, J., Zhang, Y., Liang, J., Diao, J., Liu, P., and Zhao, H. (2021). Role of Exosomal MicroRNAs and Their Crosstalk with Oxidative Stress in the Pathogenesis of Osteoporosis. Oxid Med. Cel Longev. 2021, 6301433. doi:10.1155/2021/6301433
Ma, S., Zhang, A., Li, X., Zhang, S., Liu, S., Zhao, H., et al. (2020). MiR-21-5p Regulates Extracellular Matrix Degradation and Angiogenesis in TMJOA by Targeting Spry1. Arthritis Res. Ther. 22, 99. doi:10.1186/s13075-020-2145-y
Mei, Y., Bian, C., Li, J., Du, Z., Zhou, H., Yang, Z., et al. (2013). miR-21 Modulates the ERK-MAPK Signaling Pathway by Regulating SPRY2 Expression during Human Mesenchymal Stem Cell Differentiation. J. Cel Biochem. 114, 1374–1384. doi:10.1002/jcb.24479
Meng, Y. B., Li, X., Li, Z. Y., Zhao, J., Yuan, X. B., Ren, Y., et al. (2015). microRNA-21 Promotes Osteogenic Differentiation of Mesenchymal Stem Cells by the PI3K/β-Catenin Pathway. J. Orthop. Res. 33, 957–964. doi:10.1002/jor.22884
Michlewski, G., and Cáceres, J. F. (2019). Post-transcriptional Control of miRNA Biogenesis. RNA 25, 1–16. doi:10.1261/rna.068692.118
Musolino, C., Oteri, G., Allegra, A., Mania, M., D'Ascola, A., Avenoso, A., et al. (2018). Altered microRNA Expression Profile in the Peripheral Lymphoid Compartment of Multiple Myeloma Patients with Bisphosphonate-Induced Osteonecrosis of the Jaw. Ann. Hematol. 97, 1259–1269. doi:10.1007/s00277-018-3296-7
Oka, S., Li, X., Zhang, F., Tewari, N., Ma, R., Zhong, L., et al. (2021). MicroRNA-21 Facilitates Osteoblast Activity. Biochem. Biophys. Rep. 25, 100894. doi:10.1016/j.bbrep.2020.100894
Panach, L., Mifsut, D., Tarín, J. J., Cano, A., and García-Pérez, M. Á. (2015). Serum Circulating MicroRNAs as Biomarkers of Osteoporotic Fracture. Calcif Tissue Int. 97, 495–505. doi:10.1007/s00223-015-0036-z
Perksanusak, T., Panyakhamlerd, K., Hirankarn, N., Suwan, A., Vasuratna, A., and Taechakraichana, N. (2018). Correlation of Plasma microRNA-21 Expression and Bone Turnover Markers in Postmenopausal Women. Climacteric 21, 581–585. doi:10.1080/13697137.2018.1507020
Pitari, M. R., Rossi, M., Amodio, N., Botta, C., Morelli, E., Federico, C., et al. (2015). Inhibition of miR-21 Restores RANKL/OPG Ratio in Multiple Myeloma-Derived Bone Marrow Stromal Cells and Impairs the Resorbing Activity of Mature Osteoclasts. Oncotarget 6, 27343–27358. doi:10.18632/oncotarget.4398
Sayed, D., and Abdellatif, M. (2011). MicroRNAs in Development and Disease. Physiol. Rev. 91, 827–887. doi:10.1152/physrev.00006.2010
Seeliger, C., Karpinski, K., Haug, A. T., Vester, H., Schmitt, A., Bauer, J. S., et al. (2014). Five Freely Circulating miRNAs and Bone Tissue miRNAs Are Associated with Osteoporotic Fractures. J. Bone Miner Res. 29, 1718–1728. doi:10.1002/jbmr.2175
Sheng, J., Liang, W. D., Xun, C. H., Xu, T., Zhang, J., and Sheng, W. B. (2019). Downregulation of miR-21 Promotes Tibial Fracture Healing in Rabbits through Activating ERK Pathway. Eur. Rev. Med. Pharmacol. Sci. 23, 10204–10210. doi:10.26355/eurrev_201912_19655
Smirnova, L., Block, K., Sittka, A., Oelgeschläger, M., Seiler, A. E., and Luch, A. (2014). MicroRNA Profiling as Tool for In Vitro Developmental Neurotoxicity Testing: the Case of Sodium Valproate. PLoS One 9, e98892. doi:10.1371/journal.pone.0098892
Song, J., Ahn, C., Chun, C. H., and Jin, E. J. (2014). A Long Non-coding RNA, GAS5, Plays a Critical Role in the Regulation of miR-21 during Osteoarthritis. J. Orthop. Res. 32, 1628–1635. doi:10.1002/jor.22718
Song, Q., Zhong, L., Chen, C., Tang, Z., Liu, H., Zhou, Y., et al. (2015). miR-21 Synergizes with BMP9 in Osteogenic Differentiation by Activating the BMP9/Smad Signaling Pathway in Murine Multilineage Cells. Int. J. Mol. Med. 36, 1497–1506. doi:10.3892/ijmm.2015.2363
Suarjana, I. N., Isbagio, H., Soewondo, P., Rachman, I. A., Sadikin, M., Prihartono, J., et al. (2019). The Role of Serum Expression Levels of Microrna-21 on Bone Mineral Density in Hypostrogenic Postmenopausal Women with Osteoporosis: Study on Level of RANKL, OPG, TGFβ-1, Sclerostin, RANKL/OPG Ratio, and Physical Activity. Acta Med. Indones 51, 245–252.
Sugatani, T., and Hruska, K. A. (2013). Down-regulation of miR-21 Biogenesis by Estrogen Action Contributes to Osteoclastic Apoptosis. J. Cel Biochem 114, 1217–1222. doi:10.1002/jcb.24471
Sugatani, T., Vacher, J., and Hruska, K. A. (2011). A microRNA Expression Signature of Osteoclastogenesis. Blood 117, 3648–3657. doi:10.1182/blood-2010-10-311415
Sun, K., Guo, J., Yao, X., Guo, Z., and Guo, F. (2021). Growth Differentiation Factor 5 in Cartilage and Osteoarthritis: A Possible Therapeutic Candidate. Cel Prolif 54, e12998. doi:10.1111/cpr.12998
Sun, X., Li, X., Qi, H., Hou, X., Zhao, J., Yuan, X., et al. (2020). MiR-21 Nanocapsules Promote Early Bone Repair of Osteoporotic Fractures by Stimulating the Osteogenic Differentiation of Bone Marrow Mesenchymal Stem Cells. J. Orthop. Translat 24, 76–87. doi:10.1016/j.jot.2020.04.007
Sun, Y., Xu, L., Huang, S., Hou, Y., Liu, Y., Chan, K. M., et al. (2015). mir-21 Overexpressing Mesenchymal Stem Cells Accelerate Fracture Healing in a Rat Closed Femur Fracture Model. Biomed. Res. Int. 2015, 412327. doi:10.1155/2015/412327
Takada, S., and Asahara, H. (2012). Current Strategies for microRNA Research. Mod. Rheumatol. 22, 645–653. doi:10.1007/s10165-011-0583-8
Trohatou, O., Zagoura, D., Bitsika, V., Pappa, K. I., Antsaklis, A., Anagnou, N. P., et al. (2014). Sox2 Suppression by miR-21 Governs Human Mesenchymal Stem Cell Properties. Stem Cell Transl Med 3, 54–68. doi:10.5966/sctm.2013-0081
van Meurs, J. B., Boer, C. G., Lopez-Delgado, L., and Riancho, J. A. (2019). Role of Epigenomics in Bone and Cartilage Disease. J. Bone Miner Res. 34, 215–230. doi:10.1002/jbmr.3662
Wang, B., Yu, P., Li, T., Bian, Y., and Weng, X. (2015). MicroRNA Expression in Bone Marrow Mesenchymal Stem Cells from Mice with Steroid-Induced Osteonecrosis of the Femoral Head. Mol. Med. Rep. 12, 7447–7454. doi:10.3892/mmr.2015.4386
Wang, S., Liu, Z., Wang, J., Ji, X., Yao, Z., and Wang, X. (2020). miR-21 P-romotes O-steoclastogenesis through A-ctivation of PI3K/Akt S-ignaling by T-argeting Pten in RAW264.7 C-ells. Mol. Med. Rep. 21, 1125–1132. doi:10.3892/mmr.2020.10938
Wang, X. B., Zhao, F. C., Yi, L. H., Tang, J. L., Zhu, Z. Y., Pang, Y., et al. (2019). MicroRNA-21-5p as a Novel Therapeutic Target for Osteoarthritis. Rheumatology (Oxford), kez102. doi:10.1093/rheumatology/kez102
Wei, F., Liu, D., Feng, C., Zhang, F., Yang, S., Hu, Y., et al. (2015). microRNA-21 Mediates Stretch-Induced Osteogenic Differentiation in Human Periodontal Ligament Stem Cells. Stem Cell Dev 24, 312–319. doi:10.1089/scd.2014.0191
Wu, D., Cline-Smith, A., Shashkova, E., Perla, A., Katyal, A., and Aurora, R. (2021). T-cell Mediated Inflammation in Postmenopausal Osteoporosis. Front. Immunol. 12, 687551. doi:10.3389/fimmu.2021.687551
Wu, L., Su, Y., Lin, F., Zhu, S., Wang, J., Hou, Y., et al. (2020). MicroRNA-21 Promotes Orthodontic Tooth Movement by Modulating the RANKL/OPG Balance in T Cells. Oral Dis. 26, 370–380. doi:10.1111/odi.13239
Xu, Z., Liu, X., Wang, H., Li, J., Dai, L., Li, J., et al. (2018). Lung Adenocarcinoma Cell-Derived Exosomal miR-21 Facilitates Osteoclastogenesis. Gene 666, 116–122. doi:10.1016/j.gene.2018.05.008
Yang, N., Li, Y., Wang, G., Ding, Y., Jin, Y., and Xu, Y. (2017). Tumor Necrosis Factor-α Suppresses Adipogenic and Osteogenic Differentiation of Human Periodontal Ligament Stem Cell by Inhibiting miR-21/Spry1 Functional axis. Differentiation 97, 33–43. doi:10.1016/j.diff.2017.08.004
Yang, N., Wang, G., Hu, C., Shi, Y., Liao, L., Shi, S., et al. (2013). Tumor Necrosis Factor α Suppresses the Mesenchymal Stem Cell Osteogenesis Promoter miR-21 in Estrogen Deficiency-Induced Osteoporosis. J. Bone Miner Res. 28, 559–573. doi:10.1002/jbmr.1798
Yang, R., Tao, Y., Wang, C., Shuai, Y., and Jin, L. (2018). Circulating microRNA Panel as a Novel Biomarker to Diagnose Bisphosphonate-Related Osteonecrosis of the Jaw. Int. J. Med. Sci. 15, 1694–1701. doi:10.7150/ijms.27593
Yang, Y., and Fang, S. (2017). Small Non-coding RNAs-Based Bone Regulation and Targeting Therapeutic Strategies. Mol. Cel Endocrinol. 456, 16–35. doi:10.1016/j.mce.2016.11.018
Yavropoulou, M. P., Anastasilakis, A. D., Makras, P., Tsalikakis, D. G., Grammatiki, M., and Yovos, J. G. (2017). Expression of microRNAs that Regulate Bone Turnover in the Serum of Postmenopausal Women with Low Bone Mass and Vertebral Fractures. Eur. J. Endocrinol. 176, 169–176. doi:10.1530/EJE-16-0583
Zarecki, P., Hackl, M., Grillari, J., Debono, M., and Eastell, R. (2020). Serum microRNAs as Novel Biomarkers for Osteoporotic Vertebral Fractures. Bone 130, 115105. doi:10.1016/j.bone.2019.115105
Zhang, A., Ma, S., Yuan, L., Wu, S., Liu, S., Wei, X., et al. (2020a). Knockout of miR-21-5p Alleviates Cartilage Matrix Degradation by Targeting Gdf5 in Temporomandibular Joint Osteoarthritis. Bone Jt. Res 9, 689–700. doi:10.1302/2046-3758.910.BJR-2020-0140.R1
Zhang, Y., Jia, J., Yang, S., Liu, X., Ye, S., and Tian, H. (2014). MicroRNA-21 Controls the Development of Osteoarthritis by Targeting GDF-5 in Chondrocytes. Exp. Mol. Med. 46, e79. doi:10.1038/emm.2013.152
Zhang, Y., Tian, Y., Yang, X., Zhao, Z., Feng, C., and Zhang, Y. (2020b). MicroRNA-21 S-erves an I-mportant R-ole during PAOO-facilitated O-rthodontic T-ooth M-ovement. Mol. Med. Rep. 22, 474–482. doi:10.3892/mmr.2020.11107
Zhang, Z., Qin, Y. W., Brewer, G., and Jing, Q. (2012). MicroRNA Degradation and Turnover: Regulating the Regulators. Wiley Interdiscip. Rev. RNA 3, 593–600. doi:10.1002/wrna.1114
Zhao, Q., Liu, C., Xie, Y., Tang, M., Luo, G., Chen, X., et al. (2020). Lung Cancer Cells Derived Circulating miR-21 Promotes Differentiation of Monocytes into Osteoclasts. Onco Targets Ther. 13, 2643–2656. doi:10.2147/OTT.S232876
Zhao, W., Dong, Y., Wu, C., Ma, Y., Jin, Y., and Ji, Y. (2015). MiR-21 Overexpression Improves Osteoporosis by Targeting RECK. Mol. Cel Biochem 405, 125–133. doi:10.1007/s11010-015-2404-4
Zhao, X., Xu, D., Li, Y., Zhang, J., Liu, T., Ji, Y., et al. (2014). MicroRNAs Regulate Bone Metabolism. J. Bone Miner Metab. 32, 221–231. doi:10.1007/s00774-013-0537-7
Zhao, Z., Li, X., Zou, D., Lian, Y., Tian, S., and Dou, Z. (2019). Expression of microRNA-21 in Osteoporotic Patients and its Involvement in the Regulation of Osteogenic Differentiation. Exp. Ther. Med. 17, 709–714. doi:10.3892/etm.2018.6998
Zhou, Y., Liu, Y., and Cheng, L. (2012). miR-21 Expression Is Related to Particle-Induced Osteolysis Pathogenesis. J. Orthop. Res. 30, 1837–1842. doi:10.1002/jor.22128
Zhu, H., Yan, X., Zhang, M., Ji, F., and Wang, S. (2019). miR-21-5p Protects IL-1β-induced Human Chondrocytes from Degradation. J. Orthop. Surg. Res. 14, 118. doi:10.1186/s13018-019-1160-7
Glossary
ACVR2B activin receptor type IIB
AGO Argonaute
ALP alkaline phosphatase
BMP9 Bone morphogenetic protein 9
BMMSCs bone marrow mesenchymal stem cells
BMD bone mineral density
BMSCs bone marrow stromal cells
BRONJ bisphosphonate-related osteonecrosis of the jaw
CTx C-telopeptide
DGCR8 DiGeorge critical region 8
ERK extracellular signal-regulated kinase
ERK-MAPK extracellular signal–regulated kinase-mitogen-activated protein kinase
FasL fas ligand
FGF18 fibroblast growth factor 18
GDF-5 growth differentiation factor 5
GIONFH glucocorticoid-induced osteonecrosis of the femoral head
GSK3β Glycogen synthase kinase 3β
HPDLSCs human periodontal ligament stem cells
HUMSCs human umbilical cord mesenchymal stem cells
M-CSF macrophage colony-stimulating factor
miR-21 miRNA-21
MiRNAs MicroRNAs
MMCs murine multilineage cells
MSCs mesenchymal stem cells
MT1-MMP Membrane type 1 metalloprotease
OPG osteoprotegerin
OPN osteocalcin
OSX Osterix
OVX ovariectomy
PAOO periodontal accelerate osteogenesis orthodontics
PDCD4 programmed cell death 4
PDLCs periodontal ligament cells
PI3K phosphatidylinositol 3-kinase
PLAP-1 periodontal ligament-associated protein-1
PMOP postmenopausal hypoestrogenic women with osteoporosis
pri-miRNA primary miRNA
Pre-miRNA precursor miRNA
PTEN phosphatase and tensin homologue deleted on chromosome 10
RANKL receptor activator of nuclear factor κB ligand
RECK reversion-inducing cysteine-rich protein with Kazal motifs
RISC RNA-induced silencing complex
RUNX2 runt-related gene-2
SIONFH steroid-induced osteonecrosis of the femoral head
SMAD7 small mothers against decapentaplegic 7
SMAD1/5/8 small mothers against decapentaplegic 1/5/8
SOX2 SRY sex determination SRY region Y-box2
SPRY1 Sprouty1
SPRY2 Sprouty2
SKP2 s-phase kinase associated protein 2
TLR7 Toll-like receptor
TM tooth movement
TMJOA temporomandibular joint osteoarthritis
TNF-α tumor necrosis factor-α
Keywords: miRNAs, miR-21, osteoblasts, osteoclasts, bone diseases
Citation: Chen C, Liu Y-M, Fu B-L, Xu L-L and Wang B (2021) MicroRNA-21: An Emerging Player in Bone Diseases. Front. Pharmacol. 12:722804. doi: 10.3389/fphar.2021.722804
Received: 09 June 2021; Accepted: 24 August 2021;
Published: 07 September 2021.
Edited by:
Michael Holinstat, University of Michigan, United StatesReviewed by:
Giovanni Lombardi, Galeazzi Orthopedic Institute (IRCCS), ItalySeyed Mohammad Amin Haramshahi, Iran University of Medical Sciences, Iran
Copyright © 2021 Chen, Liu, Fu, Xu and Wang. This is an open-access article distributed under the terms of the Creative Commons Attribution License (CC BY). The use, distribution or reproduction in other forums is permitted, provided the original author(s) and the copyright owner(s) are credited and that the original publication in this journal is cited, in accordance with accepted academic practice. No use, distribution or reproduction is permitted which does not comply with these terms.
*Correspondence: Bin Wang, wangbin1973@163.com; Liang-Liang Xu, xull-2016@gzucm.edu.cn
†These authors have contributed equally to this work