- 1Department of Hematology, Second Affiliated Hospital of Nanchang University, Nanchang, China
- 2Department of Medicine, Nanchang University, Nanchang, China
- 3Department of Neurology, Second Affiliated Hospital of Nanchang University, Nanchang, China
Over recent years, C-X-C motif ligand 7 (CXCL7) has received widespread attention as a chemokine involved in inflammatory responses. Abnormal production of the chemokine CXCL7 has been identified in different inflammatory diseases; nevertheless, the exact role of CXCL7 in the pathogenesis of inflammatory diseases is not fully understood. Persistent infection or chronic inflammation can induce tumorigenesis and progression. Previous studies have shown that the pro-inflammatory chemokine CXCL7 is also expressed by malignant tumor cells and that binding of CXCL7 to its cognate receptors C-X-C chemokine receptor 1 (CXCR1) and C-X-C chemokine receptor 2 (CXCR2) can influence tumor biological behavior (proliferation, invasion, metastasis, and tumor angiogenesis) in an autocrine and paracrine manner. CXCL7 and its receptor CXCR1/CXCR2, which are aberrantly expressed in tumors, may represent new targets for clinical tumor immunotherapy.
1 Introduction
In recent years, the role of chemokines in inflammatory diseases and tumors has received increasing attention (Van Damme et al., 2004). Chemokines are a family of small molecular weight proteins known for their ability to act as leukocyte chemotactic agents and are key regulators of the inflammatory response (Turner et al., 2007; White et al., 2013). For example, CXCL7 is a chemokine released by platelet activation, a potent chemotactic agent and neutrophil activator, with pro-inflammatory and pro-angiogenic effects (Walz et al., 1989). More recently, it has been found that CXCL7 is expressed not only by platelets, but also by neutrophils, megakaryocytes, natural killer cells, and lymphocytes, where it regulates their activation process and function, suggesting its important role in inflammation (Fu et al., 2004; Gleissner et al., 2008; Almeida et al., 2016). Furthermore, abnormal production of the CXCL7 chemokine has been identified in many inflammatory diseases, such as acute lung injury/acute respiratory distress syndrome, autoimmune diseases, graft-versus-host disease, and viral infections. However, the exact role of CXCL7 in the pathogenesis of inflammatory diseases is complex (Hughes-Austin et al., 2013; Bdeir et al., 2017; Yu et al., 2021).
Inflammation is an important component of the tumor microenvironment and a hallmark of cancer. Chemokines are key mediators in cancer-associated inflammation that can be present at sites of chronic inflammation, where they induce tumorigenesis or are secreted by tumor cells to form an inflammatory environment conducive to tumor development (Grivennikov et al., 2010; Greten and Grivennikov, 2019).
There is growing evidence indicating that CXCL7 is expressed by a variety of tumors, including renal cell carcinoma, breast cancer, and colorectal cancer, and that its aberrant expression can serve as a valid marker for multiple systemic malignancies, thus helping clinicians in early identification of malignancies and monitoring of treatment efficacy (Wang et al., 2013; Dufies et al., 2017; Li et al., 2021). By binding to CXCR1 and CXCR2, CXCL7 can affect tumor proliferation, invasion, migration, and tumor angiogenesis in an autocrine and paracrine manner through multiple signaling pathways (Wang et al., 2013; Mollica Poeta et al., 2019).
In this review, we summarize the role of CXCL7 in inflammatory diseases and tumors, describe the value of CXCL7 as a diagnostic and prognostic biomarker in inflammatory diseases and cancer, and discuss the importance of CXCL7 and its receptors as therapeutic targets.
2 The Biological Characteristics of CXCL7
CXCL7 is an ELR + CXC chemokine, a platelet-derived growth factor known as neutrophil-activating peptide 2 (NAP2). The basic proplatelet protein (PPBP) is the precursor protein of CXCL7, which is cleaved into the connective tissue activating peptide III (CTAP-III) and β-thromboglobulin antigen (βTG-Ag) (Walz et al., 1989; Malkowski et al., 1997; Schenk et al., 2002; Gleissner et al., 2008; Pilatova et al., 2013). CXCL7 exists as monomers, dimers and tetramers, which can be interconverted in different states (Brown et al., 2017a). Monomers predominate at lower concentrations while tetramers at higher concentrations. Dimers, which are the most preferred form in the glycosaminoglycan (GAG) bound state (Brown et al., 2017b). Recent studies have shown that CXCL7 also forms heterodimers with other platelet-derived chemokines and that GAG has a regulatory function in CXCL7 heterodimer and homodimer formation (Brown et al., 2017c).
The activity of CXCL7 is mediated by CXCR1 and CXCR2, members of the G protein-coupled receptor family with seven structural transmembrane domains (Gear and Camerini, 2003; Vandercappellen et al., 2008; Cheng et al., 2019). A main distinction between CXCR1 and CXCR2 is its selectivity for chemokines. CXCR1 binds to CXCL6 and CXCL8 with high affinity, but there is increasing evidence that CXCL7 is also the ligand for CXCR1 (Gear and Camerini, 2003). CXCR2 binds to CXCL1,CXCL2,CXCL3,CXCL5,CXCL6, CXCL7 and CXCL8 (Rajarathnam and Desai, 2020). CXCL7 has a stronger affinity for CXCR2 than CXCR1 (Ludwig et al., 1997). Tyrosine sulfation, a post-translational modification of chemokine receptors, regulates the receptor affinity for chemokine ligands (Moussouras et al., 2017). Different sites of tyrosine sulfation of CXCR1 and CXCR2 can result in changes in their respective affinities for CXCL7 (Moussouras et al., 2017). CXCR1 and CXCR2 are expressed in a variety of immune and non-immune cells, including tumor cells (Russo et al., 2014).
CXCL7 mainly has the following functions: i) promotes angiogenesis and acts as a potent chemotactic agent and activator of neutrophils; ii) regulates the expression of vascular endothelial growth factor (VEGF) C and D, two major growth factors of lymphatic vascular endothelial cells, and is involved in the lymphatic network. CXCL7 is also involved in cellular processes, such as DNA synthesis, glycolysis, mitosis, intracellular cAMP accumulation, prostaglandin E2 secretion, and hyaluronan and fibrinogen activator (Castor et al., 1983; Tai et al., 1992; Kalwitz et al., 2011).
3 The Relationship Between CXCL7 and Inflammatory Diseases
CXCL7 released by activated platelets induces the aggregation and activation of neutrophils and other immune cells, which play an important role in inflammatory diseases (Zarbock et al., 2007). In recent years, numerous studies have shown that CXCL7 is aberrantly expressed in various inflammatory diseases and has a strong correlation with pathogenesis (Hughes-Austin et al., 2013; Bdeir et al., 2017).
3.1 Acute Lung Injury/Acute Respiratory Distress Syndrome
Acute lung injury/acute respiratory distress syndrome (ALI/ARDS) is a severe unbalanced inflammatory response caused by multiple etiologies and respiratory disease with a high mortality rate (He et al., 2021). Its pathology is characterized by loss of alveolar epithelial and capillary barrier function, leading to alveolar infiltration of proteins and neutrophils, and a clinical presentation of intractable hypoxic respiratory failure (Amatullah et al., 2021; Quijada et al., 2021).
Previous studies have shown that CXCL7 is closely associated with the development and severity of ALI/ARDS disease. In a mouse model of ALI, deletion of CXCL7/PPBP strengthens the barrier function of the alveolar epithelium and attenuates neutrophil migration and activation, thus protecting mice from lung injury (Bdeir et al., 2017). In the bronchoalveolar lavage fluid (BALF) from patients with ALI, an increase in CXCL7/PPBP transcripts and proteins was accompanied by an increase in total protein and neutrophils, leading to a more severe state of ALI (Bein et al., 2021).
In recent years, a new coronavirus pneumonia (COVID-19) caused by SARS-CoV-2 has ravaged the world (Nazy et al., 2021). However, the clinical symptoms of SARS-CoV-2 infected patients are extremely variable, ranging from asymptomatic infection to acute pneumonia and even ARDS requiring mechanical ventilation support. The “cytokine storm” is characterized by an overproduction of cytokines and chemokines leading to an uncontrolled inflammatory response as a leading cause of ARDS. Chemokines CXCL10, IL6, CCL2, CXCL1, and CXCL5 have been shown to be up-regulated in patients with COVID-19, similar to that observed in the SARS and MERS epidemics (Coperchini et al., 2020). Patients who required admission to the intensive care unit had significantly higher circulating concentrations of CXCL10 and CCL2 than those with less severe clinical symptoms (Huang G. et al., 2020). Recently, studies have shown an increase in the expression of genes related to platelet activation in critically ill patients with COVID-19. The expression of CXCL7/PPBP was even more up-regulated in a disease severity-dependent manner (Yatim et al., 2021). The ROC curve analysis of CXCL7/PPBP showed that it was significant in predicting intubation ventilation treatment (area under the curve [AUC] = 0.8, p = 0.046), the optimal cut-off values for CXCL7 were 100% NPV and 45% PPV (Yatim et al., 2021). This suggests that CXCL7 may play a contributing role in the more critical forms of COVID-19.
Bronchopulmonary dysplasia (BPD) is a lung injury disorder that occurs after ventilator and oxygen therapy in preterm infants. Previous studies demonstrated the critical role of neutrophil- and pro-inflammatory cytokine-mediated inflammatory responses in the development of BPD (Choo-Wing et al., 2007; Fahy, 2009). Intratracheal transplantation of human umbilical cord blood (UCB)-derived mesenchymal stem cells (MSC) can attenuate hyperoxia-induced lung injury (Chang et al., 2011). Available studies have shown that hyperoxia significantly increases the expression of the inflammatory marker CXCL7 in lung tissue from neonatal rats. Early administration of MSCs for transplantation treatment significantly reduced CXCL7 expression (Chang et al., 2013). These findings suggest that CXCL7 may play a key role in the anti-inflammatory effects of MSC transplantation, and the main reason may be that CXCL7 mediates neutrophil aggregation in hyperoxic lung injury.
The presence of ALI/ARDS is a critical state of systemic inflammatory response syndrome (SIRS). The role of neutrophils in acute lung injury is well recognized, including cellular radicalization and release of reactive oxygen species (ROS) and protein hydrolysis granules (Moraes et al., 2006). NADPH oxidase 2 (NOX2) has an anti-inflammatory protective role in the pathogenesis of ARDS (Whitmore et al., 2013). In experimental models of SIRS in animals, only NOX2-deficient mice develop acute and severe lung lesions, including inflammation, diffuse thrombosis, and hemorrhage. Further investigation of the mechanism by which NOX2 deficiency induces ALI/ARDS showed that NOX2 deficiency can activate platelets to increase their release of CXCL7, induce neutrophil migration to the alveolar lumen, and the formation of extracellular neutrophil traps (NET), leading to ALI/ARDS (Hook et al., 2019). Consistent with these results, platelet activation-induced NET have been shown to cause acute lung injury associated with transfusions and influenza (Narasaraju et al., 2011; Caudrillier et al., 2012).
In the treatment of ARDS, the open lung approach (OLA), including the application of positive end-expiratory pressure (PEEP) and the lung recruitment maneuver (RM), has been shown to be beneficial in ARDS (Suzumura et al., 2014). OLA significantly improved pulmonary ventilation, arterial oxygenation, and gas exchange. Investigation of the mechanism of OLA revealed that OLA significantly reduced the level of platelet-derived chemokine CXCL7 in BALF, suggesting that OLA protects against lung injury by inhibiting platelet activation (Tojo et al., 2018). This may be related to platelet activation that improves pulmonary vascular thrombosis and CXCL7 release that leads to neutrophil infiltration into the alveolar lumen. This suggests that CXCL7 is a potential therapeutic target for lung injury.
3.2 Chronic Obstructive Pulmonary Disease
Chronic obstructive lung disease (COPD) is a chronic inflammatory disease of the airways and lung parenchyma associated with massive immune cell infiltration. Patients with severe COPD have increased expression of CXCL7 in the bronchial mucosa, as well as increased neutrophils and CXCR2 (Di Stefano et al., 2009). These neutrophils highly express CD44 and CD11b and can bind to intercellular adhesion molecule 1 (ICAM-1) and endothelial leukocyte adhesion molecule 1 (ELAM-1), which are highly expressed in the epithelium of the bronchial mucosa (Di Stefano et al., 1994). Another study showed an enhanced chemotactic response of monocytes to CXCL7 in COPD patients, mediated primarily by CXCR2, which can contribute to increased macrophage recruitment and activation in the lung (Traves et al., 2004). It is suggested that the CXCL7/CXCR2 axis induces the migration and adhesion of immune cells to the bronchial mucosa, leading to increased airway inflammation in COPD. Airway smooth muscle cells are a potent source of many cytokines and chemokine inflammatory mediators associated with local amplification of the airway inflammatory response. The inhibitor of I-kappa B kinase (IKK) in primary human airway smooth muscle (HASM) cells interfered with the NF-κB signaling pathway and thus significantly reduced CXCL7 transcription (Catley et al., 2006). Based on the important role of CXCL7 and HASM in the pathogenesis of COPD, IKK inhibitors and other NF-κB inhibitors can provide effective anti-inflammatory effects in patients with COPD.
3.3 Vascular Diseases
Alterations in chemokines can be detected during myocardial and cerebral ischemic infarction. On the one hand, chemokines recruit circulating leukocytes into the lesion and cause further damage, and on the other hand, they recruit stem cells to repair the damage (Gleissner et al., 2008).
CXCL7 induces neutrophil adhesion to blood vessels and transendothelial migration (Schenk et al., 2002). Leukocyte infiltration into the vessel wall is a key step in the development of hypertension. Alterations in CXCL7 can lead to a change in the inflammatory state in stress hypertension (Wu et al., 2020). Neutrophils play an important role in the development of delayed cerebral vasospasm (DCV) after aneurysmal subarachnoid hemorrhage. Neutrophils in cerebrospinal fluid (CSF) can act as predictors of DCV (Provencio et al., 2011). Anti-CXCR2 treatment significantly reduces infiltration of neutrophils into the infarcted area and decreases the infarct size (Oral et al., 2013).
Stem cell homing to the site of injury may play a reparative role. CXCL7 induces the homing of mesenchymal stem cells (MSCs) at the site of injury (Almeida et al., 2016). In chronic heart failure, reduced CXCL7 secretion from injured heart tissue impaired migration, survival and proliferation of cardiac stem cells (Boucek et al., 2015). This may be related to the regulation of angiogenesis by CXCL7 after binding to CXCR2 and stimulating the PI3K/AKT/mTOR pathway (Boucek et al., 2015). Circulating endothelial progenitor cells can be induced by the CXCL7/CXCR2 axis to the site of arterial injury and eventually differentiate into mature endothelial cells (Hristov et al., 2007a). Smooth muscle cells in the vasculature also promote endothelial regeneration after arterial injury through protein kinase C-delta (PKCδ)/signal transducer and activator of transcription 3 (STAT3)/CXCL7 paracrine mechanism (Ren et al., 2019). In stent interventions, these process may help promote stent endothelialization and prevent stenosis (Hristov et al., 2007b).
3.4 Inflammatory Bowel Disease
Inflammatory bowel disease (IBD), which includes Crohn’s disease (CD) and ulcerative colitis (UC), is associated with chronic inflammation of the gastrointestinal tract. In a rat model of UC, CXCL7 levels were significantly increased in intestinal tissues (Boshagh et al., 2019). Similarly in UC patients, CXCL7 levels were increased 13-fold (Kruidenier et al., 2006). CXCL7 is induced by matrix metalloproteinase 3 (MMP-3) and leads to subsequent neutrophil infiltration that promotes intestinal inflammation (Kruidenier et al., 2006). However, CXCL7 expression levels are low in CD, so monitoring intestinal tissue levels of CXCL7 may help distinguish between UC and CD (Kruidenier et al., 2006).
3.5 Rheumatoid Arthritis
Rheumatoid arthritis (RA) is a chronic autoimmune disease characterized by symmetric inflammatory polyarthritis (England et al., 2018). Several studies have shown that autoantibodies and other biomarkers, including chemokines and proteases are elevated in patients even years before the onset of RA (Hughes-Austin et al., 2013). CXCL7 was found to be significantly elevated in synovial fluid and serum of patients with RA 12 weeks before the onset of RA (Smolen et al., 2007). CXCL7, in combination with MMP3, can be used as a biomarker for RA (Guerrero et al., 2021). CXCL7 and CXCL4 are elevated in the synovium of early RA, which can help distinguish early RA from remitting arthritis (Yeo et al., 2016). The stimulator-of-interferon-gene (STING) signaling pathway limits Chikungunya virus (CHIKV) infection and postinfection arthritis, while STING deficiency leads to abnormal chemokine responses, including up-regulation of CXCL10 and down-regulation of CXCL5, CXCL7, and CXCR2, which promote the development of CHIKV arthritis (Geng et al., 2021).
Previous studies have shown that platelet-derived extracellular vesicles (PEVs) promote the migration and invasion of fibroblast-like synoviocytes (RA-FLS) in rheumatoid arthritis (Wang et al., 2021). CXCL7 is an important component of PEVs, and the use of the CXCR2 antagonist SB225002 partially prevents PEV-induced migration and invasion of RA-FLS. It can also reduce the phosphorylation of I-κB and NF-κB in RA-FLS (Wang et al., 2021), which in turn suggests that PEVs may activate the I-κB and NF-κB signaling pathway by binding CXCL7 to CXCR2, thus promoting migration and invasion of RA-FLS (Zhang et al., 2018; Wang et al., 2021).
3.6 Graft-Versus-Host Disease
Graft-versus-host disease (GVHD), both acute and chronic, remains a serious adverse immune reaction after allogeneic hematopoietic stem cell transplantation (HSCT), which poses a great life threat to transplantation (Zeiser and Blazar, 2017; Srinagesh and Ferrara, 2019; Hill et al., 2021).
CXCL7 has been shown to be involved in the immunomodulatory function of CD4+ T cells in acute GVHD (aGVHD) (Yu et al., 2021). The lower level of CXCL7 expression in CD4+ T cells of allogeneic HSCT mice compared to autologous HSCT was demonstrated by in vitro and in vivo experiments, whose results were consistent with those of RNA sequencing. Pathway enrichment analysis of CD4+ T cell differential genes in autologous and allogeneic HSCT revealed a non-negligible role of a chemokine signaling pathway in the signaling pathways related to immunomodulation related to aGVHD (Yu et al., 2021).
In a mouse model with chronic GVHD (cGVHD), four upregulated proteins were identified, including RAS and JUN kinase activators, CRKL, CXCL7, CCL8, and CCL9 chemokines (Du et al., 2018a). Although the application of low concentrations of CXCL7 antibodies for cGVHD showed a trend toward disease reduction, increasing the dose of CXCL7 antibodies did not further improve symptoms in cGVHD mice (Du et al., 2018a).
3.7 Inflammatory Demyelinating Diseases
Optic neuromyelitis optica (NMO) and multiple sclerosis (MS) are chronic and inflammatory idiopathic demyelinating diseases (IDDs). Until pathogenic anti-NMO antibodies were identified, NMO was believed to be a specific variant of MS (Lennon et al., 2004). Available studies have shown that CXCL1, CXCL5, and CXCL7 levels are significantly elevated in the CSF of NMO patients compared to MS patients and can be used as good bioindicators to differentiate NMO from MS, especially CXCL7 (Liu et al., 2020). Unlike MS, elevated neutrophil chemokines (CXCL7) and Th2 cell chemokines (CCL1, CCL22, and CCL17) were also found in the CSF of acute disseminated encephalomyelitis (ADEM), another inflammatory demyelinating disease, helping to differentiate ADEM from MS (Franciotta et al., 2006).
3.8 Other Inflammatory Diseases
CXCL7 levels, which are expressed at elevated levels in the CSF of patients with neurosyphilis, may participate in the pathogenesis of neurosyphilis by altering the permeability of the blood-brain barrier and promoting the spread of T pallidum (Li et al., 2020). However, CXCL7 has only moderate diagnostic efficiency for neurosyphilis (Li et al., 2020). CXCL7 concentration was also correlated with total protein levels in neurosyphilis CSF (Li et al., 2020). Urokinase plasminogen activator (uPA) concentrations were significantly higher in neurosyphilis CSF than in non-neurosyphilis. Furthermore, uPA levels were found to be correlated with total protein levels (Lu et al., 2016). CXCL7 is a strong neutrophil activator, and activated neutrophils release uPA (Abraham et al., 2003), suggesting that CXCL7 may participate in neurosyphilis by inducing and activating neutrophils to release uPA, thus increasing the protein content in the CSF of neurosyphilis patients.
In allergic pulmonary inflammation, leukotrienes D4 (LTD4) inhibit platelet activation and CXCL7 release by blocking the leukotrienes C4 (LTC4) activated cysteinyl leukotriene receptor 2 (CysLT2R) signaling pathway, thus limiting the severity of allergic pulmonary inflammation (Liu et al., 2021). Further studies have shown that LTD4 also blocks LTC4-induced phosphorylation of three kinase families, extracellular signal-regulated kinase (ERK), c-Jun N-terminal kinase (JNK), and mitogen-activated protein kinase (p38 MAPK), of the mitogen-activated protein kinase (MAPK) signaling pathway in platelets (Liu et al., 2021). Furthermore, a selective inhibitor of p38 (SB203580) blocks LTC4-induced CXCL7 release, suggesting that it may be regulated by the p38 MAPK signaling pathway.
In patients with autoimmune thyroid disease, monocytes are in an abnormal pro-inflammatory state, as evidenced by the overexpression of Ly6Clow clone monocytes (Nikolic et al., 2005). Previous studies have shown that significant upregulation of CXCL7/PPBP expression was associated with a pro-inflammatory state of monocytes (van der Heul-Nieuwenhuijsen et al., 2010). Antiphospholipid syndrome (APS) is an autoimmune thrombotic disorder, has been associated with a significantly higher plasma levels of platelet activation-related chemokines CXCL4, CXCL7, and CCL5, suggesting a role for CXCL7 in APS thrombotic events (Patsouras et al., 2015). Higher plasma levels of CXCL7 have also been found in other autoimmune diseases, such as Sjogren syndrome, Vogt-Koyanagi-Harada syndrome (VKH), and vitiligo (Egerer et al., 2006; Liang et al., 2019; Egbeto et al., 2020), further indicating that CXCL7 could play an important role in the pathogenesis of autoimmune diseases that should be further studied in more detail.
4 The Relationship Between CXCL7 and Neoplastic Diseases
Inflammation is an important component of the tumor microenvironment; The pro-inflammatory activity of CXCL7 is also manifested in a variety of tumorigenic diseases (Chatterjee et al., 2015). Moreover, CXCL7 can regulate tumor cell proliferation, invasion, and migration through multiple pathways (Wang et al., 2013; Mollica Poeta et al., 2019). The proangiogenic function of CXCL7 also provides favorable conditions for tumor cell survival. The expression, specific functions, and therapeutic targeting of CXCL7 in different types of cancers are discussed in detail in the following sections (Table 1).
4.1 Abnormal Expression of CXCL7 in Tumors
CXCL7 is aberrantly expressed in a variety of tumors of the digestive system. Plasma levels of CXCL7 are significantly reduced in patients with pancreatic cancer, an alteration that occurs at any stage of pancreatic cancer, including the early stages (stages I and II) (Matsubara et al., 2011). CXCL7 is a new biomarker independent of CA19-9 (Matsubara et al., 2011). In contrast, in patients with CRC, the plasma levels of CXCL7 was found to be significantly higher compared to healthy controls (Li et al., 2019). The ROC curve was used to evaluate the clinical diagnostic efficacy of CXCL7 and other CRC tumor markers CEA, CA19-9, and CA125, and the results showed that CXCL7 had the highest diagnostic power, with an AUC of 0.862 (95% CI: 0.831–0.890) (Li et al., 2019), thus indicating that abnormal levels of CXCL7 may contribute to early detection and diagnosis of intestinal system tumors.
CXCL7 may also play an important role in the early diagnosis of gynecological tumors. CTAPIII/CXCL7 is expressed in normal cervical epithelial cells; however, its expression gradually disappears with the appearance and progression of cervical cancer (Grisaru et al., 2000). Another study reported that in early ovarian cancer, the expression of CTAPIII/CXCL7 decreased (Clarke et al., 2011). CXCL7 not only significantly improves the diagnostic sensitivity of CA125 to early ovarian cancer, but also helps with the diagnosis of ovarian cancer that does not express CA125 (Clarke et al., 2011).
For hematological diseases, a decrease in plasma CXCL7 levels can be used as a diagnostic marker for advanced disease of myelodysplastic syndrome refractory anemia with excess blasts (RAEB) and RAEB in transformation (RAEB-t) (FAB classification) or RAEB-1 and RAEB-2 (WHO classification) (Aivado et al., 2007). Decreased CXCL7 levels can also be used to differentiate pediatric acute lymphoblastic leukemia (ALL) patients from healthy controls and pediatric acute myeloid leukemia (AML) patients (Shi et al., 2009). Bone marrow stromal cells can induce monocytes to secrete CXCL7, which in turn regulates CXCL7 expression in megakaryocytes (Pillai et al., 2006). CXCL4 and CXCL7 promote the survival of hematopoietic progenitor cells and reduce their chemosensitivity to cytotoxic drugs (Han et al., 1997). Excess CXCL4 and CXCL7 in the bone marrow microenvironment contribute to the resistance towards decitabine in chronic monocytic leukemia (Meldi et al., 2015).
In the respiratory system, CXCL7 has been found to be significantly elevated 29 months prior to lung cancer development, making it the first blood biomarker capable of diagnosing stage 0 cancer (Yee et al., 2009; Lee et al., 2011; Ulivi et al., 2013). The concentration of CXCL7 is significantly higher in the blood draining tumors from patients with lung cancer compared to peripheral blood, and the CXCL7 gradient was correlated with the absolute number of helper T cells (r = 0.49, p = 0.03) (Yee et al., 2009; Spaks, 2017). CTAPIII/CXCL7 levels decreased after radical surgery, which in turn suggested that CXCL7 is useful for postoperative monitoring of small residual lesions and the prediction of disease recurrence (Yee et al., 2009). When comparing bronchial airway epithelial cell samples from mice with early stage lung squamous cell carcinoma (LSCC) with those of normal mice, CXCL7 gene is overexpressed 115-fold, indicating that CXCL7 is not only a blood biomarker for the diagnosis of non-small cell lung cancer (NSCLC), but also a biomarker for bronchial brush samples (Xiong et al., 2017). Furthermore, Du et al. (2018b) found that CXCL7 had a higher diagnostic efficacy for NSCLC (AUC of ROC: 0.806, 95% CI: 0.748–0.863) compared to traditional diagnostic biomarkers for lung cancer (CEA, SCCAg, and Cyfra211) (Du et al., 2018b). These studies have consistently suggested that CXCL7 can be used as a diagnostic marker for early-stage lung cancer (especially stage 0 lung cancer).
In the urinary system, Kinouchi et al. (2017) demonstrated that the expression of the CXCL7 gene in the peripheral blood from patients with renal cell carcinoma (RCC) was significantly higher than that of healthy controls and could be used as an independent diagnostic marker for RCC. In particular, CXCL7 was elevated from stage pT1a in patients with RCC and gradually increased with the stage TNM of RCC.
4.2 Effects and Molecular Mechanisms of CXCL7 on Tumor Biological Behavior
4.2.1 Renal Cancer
In clear cell renal cell carcinoma (ccRCC), mutations in the von Hippel-Lindau gene lead to overexpression of VEGF, making ccRCC a highly vascularized tumor (Hutson et al., 2010; Wallace et al., 2016). The pro-inflammatory pro-angiotropic factor CXCL7 was shown to promote ccRCC cell proliferation in vitro and tumor growth in vivo (in nude mice) (Grépin et al., 2014). In contrast, an inhibitor of the CXCL7 receptors CXCR1 and CXCR2 (SB225002) inhibited the proliferation of ccRCC cells and endothelial cells, thereby strongly suppressing tumor growth and aberrant tumor angiogenesis (Grépin et al., 2014). The central pro-inflammatory cytokine interleukin 1β (IL-1β) induces increased CXCL7 secretion by ccRCC cells, which can directly act on the surface receptor CXCR1/2 of ccRCC cells (Grépin et al., 2014); CXCL7 also acts paracrinely by promoting the proliferation of RCC cells, which in turn promotes further secretion of CXCL7 by peripheral blood monocytes in ccRCC (Kinouchi et al., 2017).
Mechanistically, CXCL7 enhances the activity of the PI3K/AKT/mTOR and ERK cell proliferation signaling pathways by binding to the CXCR1 and CXCR2 ccRCC cell membrane receptors and exerting a proliferative effect on tumor cells (Figure 1) (Grépin et al., 2014). CXCL7 also acts through the Ras/Ras/MAPK signaling pathway associated with tumor angiogenesis (Figure 1) (Strieter et al., 2006). Treatment of nude mice bearing tumors using anti-CXCL7 antibodies may inhibit tumor growth in three ways (Grépin et al., 2014): i) it decreases the activity of ERK and AKT signaling pathways; ii) it decreases the number of Ki-67-labeled tumor cells and increases the number of necrotic regions; iii) it decreases carbonic anhydrase 9 (CA Ⅸ) expression and the hypoxic regions associated with the induction of invasive cell production, which results in the inhibition of tumor growth of ccRCC.
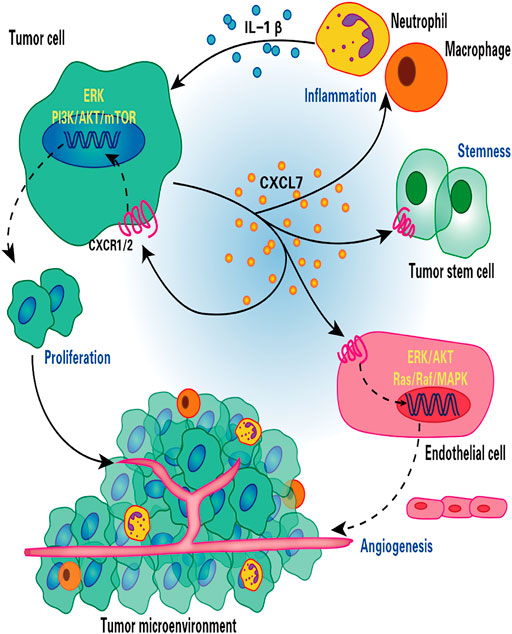
FIGURE 1. Role of CXCL7 in renal cell carcinoma. CXCL7 secreted by renal cancer cells binds to CXCR1/2 on the surface of tumor cells and endothelial cells, stimulating tumor cell and endothelial cell proliferation and tumor angiogenesis through ERK/AKT and Ras/Raf/MAPK signaling cascades, respectively. CXCL7 also maintains tumor stem cell properties and recruits neutrophils and macrophages to infiltrate, while inflammatory cells secrete IL-1β in turn promotes CXCL7 secretion by renal cancer cells, forming a positive feedback loop. Thus, the CXCL7/CXCR1/CXCR2 signaling axis is critical for tumor growth and angiogenesis and for maintaining the tumor inflammatory environment.
According to previous studies, tumor stem cells have an extreme capacity for self-renewal and tumorsphere formation, promoting tumor formation and metastasis, and participating in the development of tumor drug resistance (Lytle et al., 2018; Huang T. et al., 2020). Galectin-3 (Gals-3) maintains the properties of RCC tumor stem cells. Studies on the mechanism of action of Gals-3 revealed that down-regulation of Gals-3 inhibits the expression of CXCL7 and CXCR2, thus suppressing tumor formation and colony formation capacity of renal cancer cells. Conversely, up-regulation of CXCR2 expression restores the tumorigenic properties of Gals-3 knockdown cells (Huang et al., 2018). These results suggest that the CXCL7/CXCR1/CXCR2 axis may represent an effective target for the treatment of RCC. In addition, Dufies et al. (2017) have identified CXCL7 as a valid predictor of the efficacy of sunitinib in clear cell RCC.
The CXCL7/CXCR2 axis promotes early metanephric development. Thus, active CXCL7/CXCR2 signaling maintains nephroblastoma survival (Levashova et al., 2007). CXCL7 induces tumor angiogenesis and invasiveness through upregulation of matrix metalloproteinase 9 (MMP9) and endothelial and thylakoid markers (Levashova et al., 2007). Nephroblastoma is sensitive to CXCR2 antagonist-induced apoptosis (Levashova et al., 2007). The CXCL7/CXCR2 axis could be a new target for nephroblastoma therapy.
4.2.2 Cholangiocarcinoma
The expression of CXCL7 is higher in tissues with cholangiocarcinoma (CC) than in adjacent nontumor tissues, and the high expression of CXCL7 is significantly associated with poor differentiation, lymph node metastases, vascular invasion, and advanced clinical stages of CC. Furthermore, the overall survival (OS) of patients with CC with high expression of CXCL7 was significantly shorter (Guo et al., 2017). In vitro studies showed that CXCL7 and CXCR2 were highly expressed in CC cell lines HuCCT1, HuH28, QBC939, EGI-1, OZ and WITT, and overexpression or knockdown of CXCL7 promoted or inhibited proliferation and invasion of CC cells, respectively, suggesting that CXCL7 can act in an autocrine mode in tumors (Guo et al., 2017). Furthermore, the addition of exogenous CXCL7 medium or conditioned medium overexpressing CXCL7 human liver stellate cells also promoted invasiveness of QBC939 cells, indicating that CXCL7 could also act paracrinely in CC (Guo et al., 2017). Sueoka et al. (Sueoka et al., 2014) also demonstrated that in the intrahepatic cholangiocarcinoma (ICC) cell lines RBE and SSP25, both CXCR2 siRNA and SB225002 significantly inhibited cell proliferation, migration, and invasion, while SB225002 also inhibited the growth of subcutaneous transplanted tumors in nude mice.
The AKT signaling pathway has been shown to be involved in human tumorigenesis and development, while the overexpression of CXCL7 in cholangiocarcinoma increased the activity of the AKT signaling pathway, suggesting that the AKT signaling pathway is involved in the malignant phenotype mediated by the CXCL7/CXCR2 axis of cholangiocarcinoma cells (Guo et al., 2017; Cheng et al., 2019). At the site of metastasis, a microenvironment conducive to disease progression is formed by the interaction of tumor cells and host cells, called a metastatic niche. Leukocytes have been shown to be recruited to the metastatic niche and support metastasis (Qian et al., 2011; Spicer et al., 2012). Tumor cells can adhere to and activate platelets and recruit neutrophils through the CXCL5/CXCL7/CXCR2 axis to promote the formation of early metastatic niches (Labelle et al., 2014).
4.2.3 Breast Cancer
In primary breast cancer, CXCL7 concentration was found to be significantly correlated with Ki67 expression, indicating that CXCL7 was associated with the active proliferation of tumor cells (Wang et al., 2013). There is growing evidence that MSCs and cytokines in the tumor microenvironment (TME) can influence tumorigenesis and progression (Whiteside, 2018; Yin et al., 2020; Choi et al., 2021). Previous studies using in vitro and mouse xenograft models revealed that bone marrow MSCs can accelerate tumor growth by increasing the self-renewal capacity (number) of breast cancer stem cells (CSCs), and this effect depends on the regulation of the intercellular IL-6/CXCL7 cytokine network (Liu et al., 2011). Among them, IL six produced by breast cancer cells acts on IL-6R/GP130 receptors in bone marrow MSCs to stimulate the production of CXCL7 by bone marrow MSCs. In turn, CXCL7 induces breast cancer cells and MSCs to secrete a large number of cytokines, including IL-6, IL-8, CXCL6, and CXCL5, which then induce an increase in the population of breast CSCs. In addition, increased IL-6 interacts with MSCs to form a positive feedback loop (Liu et al., 2011). These results suggest that the IL-6/CXCL7 network in the TME plays an important role in the growth of breast cancer (Figure 2).
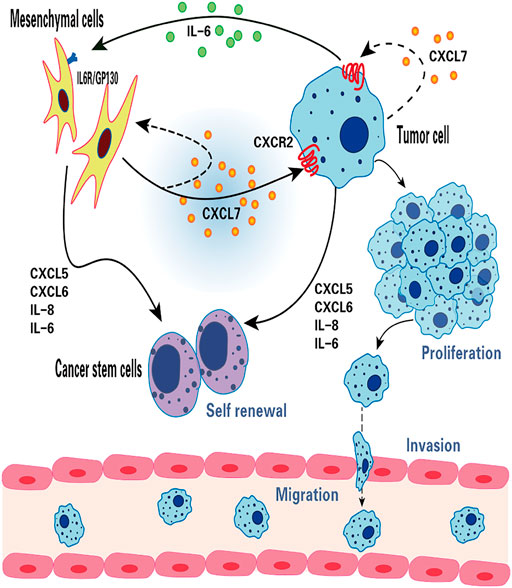
FIGURE 2. The function of CXCL7 in breast cancer. CXCL7 mediates the interaction between MSCs, breast cancer cells and breast cancer stem cells through IL-6 and IL-8, CXCL6 and CXCL5 cytokine networks. CXCL7 promotes tumor cell proliferation, migration and invasion by autocrine or paracrine means, attracts MSCs into the tumor niche and maintains the self-renewal capacity of tumor stem cells.
CXCL7 promotes the growth, invasion, and metastasis of breast cancer. Malignant breast cancer cells (MCF10CA1a.cl1) expression of both CXCL7 and CXCR2 is higher than that of precancerous cells (MCF10AT), where MCF10AT transfected with CXCL7 were significantly more invasive through the basement membrane of the stroma, an effect that was markedly inhibited by the use of the CXCL7 antibody (Tang et al., 2008). CXCL7 is confirmed to play a role in breast cancer invasion (Figure 2).
Zcharia et al. (2001) found that heparanase activity was closely related to the invasive and metastatic ability of breast cancer cells. Heparanase activity was significantly higher in malignant breast cancer cells than in MCF10AT cells and increased in MCF10AT cells after transfection with CXCL7 (Tang et al., 2008). In addition, Yu et al. (2010) found that CXCL7 siRNA inhibited the heparanase activity and invasive ability of CXCL7-transfected MCF10AT cells. In breast cancer, CXCL7 also induced the expression of lymphangiogenic factors VEGF-C and VEGF-D and promoted the lymphatic spread of tumor cells. The ability to secrete VEGF-C and VEGF-D and the invasive capacity of MCF10AT transfected with CXCL7 were also decreased by acting with the selective CXCR2 inhibitor SB225002 (Yu et al., 2007; Yu et al., 2010). These results suggest that CXCL7 facilitates breast cancer invasion and metastasis by increasing heparanase activity and promoting the secretion of VEGF-C and VEGF-D in breast cancer cells.
Low concentrations of piperine fractional piper nigrum extract (PFPE) have also been shown to exert anticancer immune effects in breast cancer rats. PEPE promotes Th1 expression, inhibits Th2, Treg, and CXCL7 expression, and reduces neutrophil infiltration, thus significantly reducing breast cancer tumor volume without side effects on blood biochemical parameters in rats (Saetang et al., 2021). Interestingly, the components of PFPE, 3-carene and pellitorine cause tumor regression by inhibiting CXCL7 production mediated by the activator IL-6 (Basholli-Salihu et al., 2017). It is shown to mediate antitumor effects through the regulation of CXCL7.
4.2.4 Colorectal Cancer
In CRC, high expression of CXCL7 is closely associated with tumor vascular production. Previous studies have shown that CXCL7 is positively correlated with VEGF and that the coexpression of CXCL7 and VEGF is a risk factor for a poor prognosis in CRC patients (Li et al., 2021).
Patients with obstructive CRC (OCRC) have a higher risk of surgery requirement and a worse prognosis than patients with non-obstructive CRC (non-OCRC). According to previous studies, serum CXCL7 concentrations are not only higher in patients with OCRC compared to healthy controls, but also in patients without OCRC (Li et al., 2019). CXCL7 was reported to have the highest diagnostic efficiency (AUC of 0.918, sensitivity of 86.54% and specificity of 81.87%) compared to existing diagnostic indicators for OCRC (CEA, CA199, CA125) (Li et al., 2019). Serum CXCL7 levels in OCRC patients have also been associated with stage N lymph node metastasis (N0-N2) and TNM stage (I-II to IV), and analysis of the multifactorial Cox proportional risk regression model analysis showed that high serum CXCL7 levels were an independent factor for poorer OS in OCRC patients (HR = 2.216, p = 0.032) (Li et al., 2019). These results suggest that serum CXCL7 is a potential biomarker for the diagnosis and prognosis of patients with OCRC, which can help improve the early diagnosis of OCRC, reduce the risk of acute surgery, and improve patient survival.
The expression of CXCL7 and CXCR2 was found to be negatively correlated with disease-free survival (DFS) and OS in patients with liver metastatic CRC, suggesting that the CXCL7/CXCR2 signaling pathway may be involved in tumor recurrence and progression (Desurmont et al., 2015). 5-fluorouracil-based neoadjuvant chemotherapy regimens increased CXCL7 and CXCR2 gene expression in patients with CRC developing liver metastases, thus suggesting that neoadjuvant chemotherapy may have a role in promoting the spread of CRC cells in vivo through the CXCL7/CXCR2 axis (Desurmont et al., 2015).
4.2.5 Gliomas
Increased infiltration of myeloid cells, particularly myeloid-derived suppressor cells (MDSCs) and tumor-associated macrophages (TAMs), in the TME is associated with glioma progression and resistance to anti-angiogenic therapies (AATs) (Hussain et al., 2006; Sica et al., 2006; Lu-Emerson et al., 2013). Investigations have shown that CSF1-CSF1R signaling is associated with monocyte proliferation and differentiation and plays an important role in tumor angiogenesis and progression (Priceman et al., 2010; Hume and MacDonald, 2012; Zhu et al., 2014). The use of CSF1R inhibitors (GW2580) reduces myeloid cells in the TME of gliomas and significantly decreases the expression of chemokine CXCL7, thus inhibiting tumor growth (Achyut et al., 2015). The ERK-MAPK pathway plays a regulatory role in the expression of CXCL7 by myeloid cells and is correlated with the Ki67 proliferation data (Achyut et al., 2015).
In gliomas, Fibrinogen-like protein 2 (FGL2) secreted by glioma cells recruits macrophages to the TME and induces CXCL7 (Yan et al., 2021). In vitro, CXCL7 improves tumor stem cell properties, including tumorsphere formation capacity and migration ability; in vivo, CXCL7 increases the incidence and shortens the survival time of gliomas in mice (Yan et al., 2021).
Additional studies have shown that FGL2 secreted by glioma cells activates the downstream signaling pathway Syk/PI3K/AKT/HIF1 by binding to CD16a receptors on the surface of macrophages, thus mediating the release of CXCL7 from macrophages and further promoting the development and progression of glioma of glioma by CXCL7 (Sironi et al., 2006; Bournazos et al., 2016; Yan et al., 2021). Glucocorticoids and macrophage-inactivating factors IL-4 and IL-10 were also found to significantly downregulate CXCL7 mRNA and protein levels in mononuclear phagocytes (El-Gedaily et al., 2004).
4.2.6 Other Neoplastic Diseases
Infiltration of various immune cells has been shown to be strongly associated with the biological behavior of tumors and the clinical outcome of patients with cancer (Sarode et al., 2020; Sui et al., 2020). Furthermore, the recruitment of immune cells is largely dependent on the expression of chemokines (Li et al., 2018; Strazza and Mor, 2020). The infiltration of CD8+ T lymphocytes has been shown to be an independent and good prognostic factor in epithelial ovarian cancer (Sato et al., 2005). The transcription factor myeloid ecotropic viral integration site 1 (MEIS1) binds to a specific region on the promoter of the CXCL7 gene and induces the synthesis and secretion of CXCL7 in cells of ovarian cancer, thus triggering the recruitment of CD8+ T lymphocytes in ovarian cancer (Karapetsas et al., 2018). Yamamoto et al. (2019) found that CXCL7 was expressed in 36.4% of 590 patients with gastric cancer and was correlated with clinicopathological characteristics such as diffuse gastric cancer, lymph node metastasis, lymphovascular, and vascular invasion. The high expression of CXCR1 and the low expression of CXCR2 in gastric cancer were shown to be associated with higher levels of AFP, greater tumor size, and a higher stage of TNM tumor stage (Chen et al., 2020). After surgery in patients with gastric cancer, CXCL7 levels in peripheral blood and tumor drainage blood were higher in the recurrence group than in the group without recurrence, and the concentration gradient of CXCL7 was correlated with the absolute number of helper T cells in TMN (Chen et al., 2020). Inhibition of CXCL7 expression in gastric cancer cells by siRNA significantly reduced their migration ability (Chen et al., 2020). Significantly elevated levels of CXCL7 were obtained in tissues of bladder cancer (BLCA) by bioinformatics analysis (Sun et al., 2021). CXCL7 may regulate protein kinase N1 and G protein-coupled receptor kinase 2, and the mRNA encoding CXCL7 is targeted and regulated by miR-154, miR-487, miR-525, and miR-524 (Sun et al., 2021). According to previous studies, the expression of CXCL7 was associated with infiltration of dendritic cells, neutrophils and CD8 + T cells in BLCA (Sun et al., 2021). CXCL1, CXCL5, CXCL7, and CXCL8 expression in NSCLC have been associated with low patient survival, and CXCL7 is positively correlated with macrophage infiltration in TME, while its receptor CXCR2 is positively correlated with both neutrophil and macrophage infiltration (Unver, 2021). It is well known that M2-type TAMs are often associated with tumor progression. M2-type TAMs increase the proliferation and migratory capacity of Lewis lung cancer (LLC) cells (Lamagna et al., 2006; Zhang et al., 2012). In lung adenocarcinoma, M2-type macrophages are also associated with accelerated lymphangiogenesis (Zhang et al., 2012). In the LLC model, overexpression of CXCL7 chemokine induced increased M2-polarized macrophage infiltration and accelerated early LLC tumor development (Unver et al., 2015). This suggests that CXCL7 chemokines can influence immune cell infiltration and inflammatory responses in the TME, which in turn affects tumor progression as well as clinical treatment and outcomes of tumor patients.
Tumor cells are often present in the hypoxic TME, and hypoxia can induce changes in chemokines in the TME, which affects the biological behavior of the tumor (Korbecki et al., 2021). Chronic hypoxia increases the expression of CXCL7 in cervical cancer cells and astrocytes (Xie et al., 2015; Samy et al., 2018). However, chronic hypoxia does not influence CXCL7 expression in lung adenocarcinoma cells and HepG2 hepatocellular carcinoma cells (Huang et al., 2016; Ye et al., 2016). CXCR1 and CXCR2 act as receptors for CXCL7, and hypoxia can affect the function of CXCL7 by affecting the expression of the CXCL7 receptor. Chronic hypoxia increases the expression of CXCR1 and CXCR2 in prostate cancer cells and cervical cancer cells, and this effect is based on hypoxia-inducible factors 1 (HIF-1) and NF-κB activation (Maxwell et al., 2007; Liu et al., 2014; Taniguchi and Karin, 2018). Chronic hypoxia also increased CXCR2 expression in aortic endothelial cells, suggesting that hypoxia influences tumor angiogenesis by increasing the sensitivity of endothelial cells to CXCR2 ligands (Moldobaeva and Wagner, 2005). However, this effect varies depending on the type of tumor, e.g., chronic hypoxia decreased CXCR2 expression in gastric cancer cells (Kinoshita et al., 2015). Hypoxia and hypoxia-activated signaling pathways are effective targets in cancer therapy, where the former can cause alterations in the expression of CXCL7 and its receptor CXCR1/2 in tumors. Consequently, targeting CXCL7 and its receptor has the potential to be used as a new oncology therapy (Jing et al., 2019; Ivan et al., 2021).
5 Intracellular Signaling Pathways of CXCL7
5.1 The PI3K/AKT/mTOR Signaling Pathway
PI3K, the major downstream intracellular signal of CXCL7, induces phosphorylation of its substrate Akt. mTOR is a component of the AKT signaling pathway, which is a central regulator of cell metabolism, proliferation, growth, and survival (Alzahrani, 2019). mTOR is activated in various pathological cellular processes, such as tumor formation, angiogenesis, insulin resistance, and T lymphocyte activation (Wysocki, 2009). CXCL7 activates the PI3K/AKT/mTOR signaling pathway and promotes proliferation and migration of tumor cells (Grépin et al., 2014; Guo et al., 2017). CXCL7 induces tumor angiogenesis also through activation of the PI3K/AKT/mTOR pathway in endothelial cells (Figure 3) (Grépin et al., 2014).
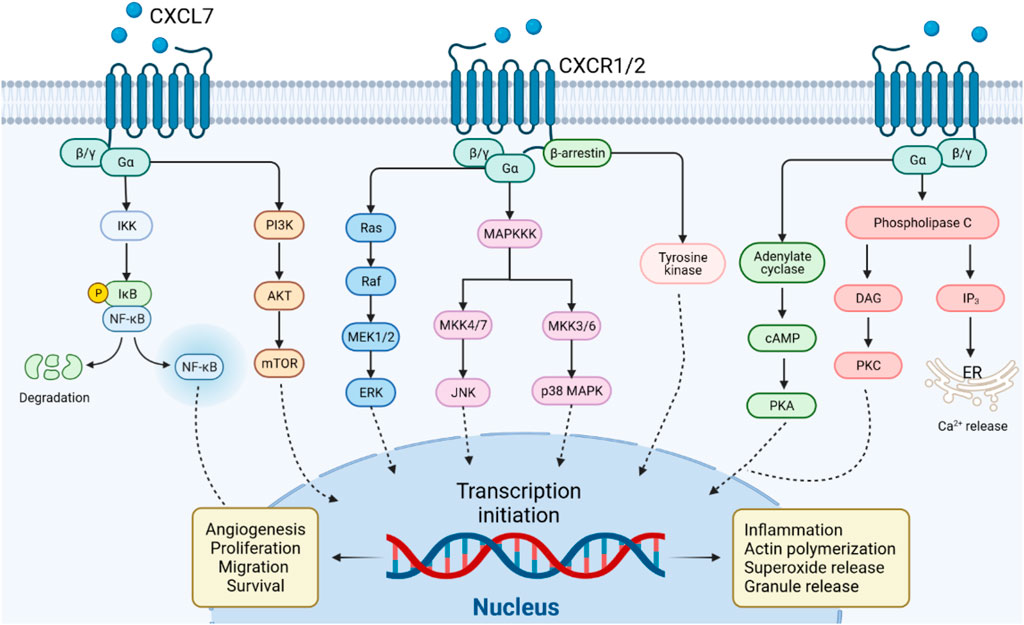
FIGURE 3. CXCL7/CXCR1/2 signal transduction pathway. CXCL7 binds to CXCR1/2 receptors via G protein or β-arrestin coupling to activate PI3K/AKT/mTOR, NF-κB, PKA, PKC or MAPK tertiary activation signaling pathways, mediating the role of CXCL7 in inflammation and tumor.
5.2 The MAPK Signaling Pathway
The MAPK signaling cascade consists of multiple serine and threonine kinases. MAPK can be divided into four subfamilies, including ERK, p38 MAPK, JNK, and ERK5 (Fang and Richardson, 2005). The MAPK subfamily signaling pathways perform different duties: ERK regulates cell growth and differentiation, and the JNK and p38 MAPK signaling pathways exert important roles in stress responses such as apoptosis and inflammation (Santarpia et al., 2012; Arthur and Ley, 2013). CXCL7 activates the classical MAPK signaling pathway Ras/Raf/ERK, which promotes cell proliferation and angiogenesis in tumors (Grépin et al., 2014). CXCL7 also plays a role in inflammatory diseases through the JNK and p38 MAPK signaling pathways (Figure 3).
5.3 The NF-κB Signaling Pathway
The NF-κB signaling pathway may be stimulated by a variety of cytokines, growth factors, and tyrosine kinases (Hoesel and Schmid, 2013). Activated NF-κB signaling pathway regulates CXCL7 transcription and, in turn, CXCL7 influences inflammation and tumorigenesis via NF-κB (Figure 3) (Wang et al., 2021). In arthritis, CXCL7 induces NF-κB activation to promote the migration of fibroblastic synovial cells (Wang et al., 2021). This may be related to the NF-κB-induced expression of cell adhesion molecules (e.g. ICAM-1) and invasion-related proteins (Lawrence, 2009). Furthermore, activation of signaling pathways such as PI3K/Akt and Ras/MAPK can also regulate the function of the NF-κB pathway (Tang et al., 2022). The crosstalk of CXCL7 on the above signaling pathways needs to be further investigated.
5.4 The G Protein or β-arrestin Signaling Pathway
CXCL7 is a strong chemotactic agent of neutrophils, which activates G-protein or β-arrestin signaling pathways upon binding to CXCR1 or CXCR2, coordinating the recruitment of neutrophils to infected or injured tissues (Ludwig et al., 1997). Activation of G proteins induces the production of cyclic adenosine monophosphate (cAMP), inositol triphosphate (IP3) and diacylglycerol (DAG). β-arrestin induces MAPK and tyrosine kinase activation (Rajarathnam et al., 2019). The G protein and β-arrestin signaling cascades contribute to actin remodeling and integrin activation, mediating neutrophil adhesion to blood vessels and subsequent transendothelial migration (Detmers et al., 1991; Schenk et al., 2002). These pathway also induces the release of cytotoxic enzymes and ROS from neutrophil granules, which play an important role in the inflammatory response (Walz and Baggiolini, 1989). In general, G protein-mediated signaling starts rapidly and diminishes within minutes. In contrast, β-arrestin-mediated events are characterized by a slower onset and a longer duration (Luttrell and Gesty-Palmer, 2010). Due to selection bias, whether CXCL7 exerts its chemotactic effect on neutrophils through G-protein or β-arrestin cascade signaling after binding to CXCR1/CXCR2 needs to be further investigated (Figure 3).
6 Clinical Trials for CXCL7/CXCR1/2
Considering the role played by CXCL7/CXCR1/2 in inflammatory and neoplastic diseases, the development of drugs targeting the CXCL7/CXCR1/2 axis may benefit disease treatment. The corresponding drugs are now being tested in clinical trials with encouraging results (Table 2).
Reparixin is a noncompetitive metastable inhibitor of CXCR1 and CXCR2 with a 400-fold greater potency to inhibit CXCR1 activity than CXCR2 (Bertini et al., 2004). Clinical studies on reparixin have been completed evaluating its use for the treatment of ischemia-reperfusion injury, organ transplant-associated disease. The results showed that reparixin can effectively regulate neutrophil recruitment and attenuate inflammatory injury in ischemia-reperfusion (Opfermann et al., 2015). In the treatment of breast cancer, Reparixin has been shown to be safe and well-tolerated, and has shown benefit in reducing breast cancer tumor stem cells (Goldstein et al., 2020). Given the potent anti-inflammatory effects of reparixin, a number of clinical trials on the safety and efficacy of reparixin in the treatment of COVID-19 pneumonia are ongoing, as reparixin will hopefully be used as an adjuvant treatment for COVID-19 pneumonia.
AZD5069 is a selective CXCR2 antagonist. The clinical safety, tolerability, and efficacy of AZD5069 have been studied in acute and chronic inflammatory lung diseases and in advanced metastatic cancers (prostate and head and neck cancer). In inflammatory lung disease, AZD5069 treatment has been shown to be well tolerated in clinical trials with a reduction in neutrophil infiltration in blood and sputum. However, it was not associated with the improved clinical outcomes explored in the trial, so additional clinical trials are needed to explore its effectiveness (O'Byrne et al., 2016; De Soyza et al., 2015). AZD5069 has been shown to improve TGF-β signaling pathway-mediated adriamycin resistance through inhibition of CXCR2 in triple-negative breast cancer cells and to enhance atezolizumab immunotherapy efficacy (Ghallab et al., 2022). Therefore, the results of the ongoing clinical trials of AZD5069 antitumor therapy are worthy of our expectation.
Sch527123, also known as Navarixin, is a CXCR1 and CXCR2 antagonist with a high affinity for CXCR2. Current clinical studies on SCH527123 have focused on inflammatory diseases such as COPD, asthma and solid tumors (non-small cell lung cancer, prostate cancer and colon cancer). These studies evaluated the safety, efficacy and dose range of SCH527123 in the treatment of respective diseases. In a randomized, double-blind, placebo-controlled study, SCH527123 resulted in a significant reduction in ozone-induced airway neutrophils in healthy subjects, which was safe and well tolerated (Holz et al., 2010). However, further evaluation in a large trial in patients with pulmonary dysfunction is warranted.
GSK1325756 is a potent competitive CXCR2 receptor antagonist. Clinical trials evaluated the safety, tolerability, pharmacokinetics, and bioavailability studies of GSK1325756 in COPD and respiratory viral infectious diseases (Lazaar et al., 2018).
SB-656933 is a selective CXCR2 antagonist. Clinical studies have been conducted in airway inflammatory and intestinal inflammatory diseases. SB-656933 was shown to have a dose-dependent effect on neutrophil activation and recruitment in the tolerated dose range, making this drug potentially effective in neutrophil-dominant diseases (Lazaar et al., 2011; Moss et al., 2013).
SX-682 is a small molecule inhibitor of CXCR1 and CXCR2. Clinical trials of SX-682 are currently underway for the treatment of solid tumors (e.g., pancreatic and colon cancer) and non-solid tumors (e.g., myelodysplastic syndromes). SX-682 has been shown to inhibit MDSCs transport and enhance the immune efficacy of NK cells in head and neck tumor models (Greene et al., 2020).
7 Conclusion
Aberrant expression of CXCL7 in inflammatory diseases and tumors may serve as a biomarker for disease diagnosis and prognosis. CXCL7 acts as an inflammatory cytokine that induces various immune cells to participate in the pathogenesis of inflammatory diseases. In various tumors, CXCL7 has been preliminarily found to affect tumor growth, invasion, metastasis and tumor angiogenesis to some extent. CXCL7 binding to receptors CXCR1 or CXCR2 mediates its role in inflammatory diseases and tumors through signaling pathways such as PI3K/AKT/mTOR, NF-κB or MAPK. Currently, clinical trials have demonstrated the efficacy and safety of targeted CXCL7/CXCR1/CXCR2 therapy, which is encouraging for future clinical applications.
Author Contributions
QW wrote the review and designed the figures. QW, HT, and JL corrected the manuscript.
Funding
This review was supported by Natural Science Foundation of Jiangxi Province (Grant No. 20171BAB205045) and National Natural Science Foundation of China (Grant No. 8176010176).
Conflict of Interest
The authors declare that the research was conducted in the absence of any commercial or financial relationships that could be construed as a potential conflict of interest.
Publisher’s Note
All claims expressed in this article are solely those of the authors and do not necessarily represent those of their affiliated organizations, or those of the publisher, the editors and the reviewers. Any product that may be evaluated in this article, or claim that may be made by its manufacturer, is not guaranteed or endorsed by the publisher.
References
Abraham, E., Gyetko, M. R., Kuhn, K., Arcaroli, J., Strassheim, D., Park, J. S., et al. (2003). Urokinase-type Plasminogen Activator Potentiates Lipopolysaccharide-Induced Neutrophil Activation. J. Immunol. 170 (11), 5644–5651. doi:10.4049/jimmunol.170.11.5644
Achyut, B. R., Shankar, A., Iskander, A. S., Ara, R., Angara, K., Zeng, P., et al. (2015). Bone Marrow Derived Myeloid Cells Orchestrate Antiangiogenic Resistance in Glioblastoma through Coordinated Molecular Networks. Cancer Lett. 369 (2), 416–426. doi:10.1016/j.canlet.2015.09.004
Aivado, M., Spentzos, D., Germing, U., Alterovitz, G., Meng, X. Y., Grall, F., et al. (2007). Serum Proteome Profiling Detects Myelodysplastic Syndromes and Identifies CXC Chemokine Ligands 4 and 7 as Markers for Advanced Disease. Proc. Natl. Acad. Sci. U. S. A. 104 (4), 1307–1312. doi:10.1073/pnas.0610330104
Almeida, C. R., Caires, H. R., Vasconcelos, D. P., and Barbosa, M. A. (2016). NAP-2 Secreted by Human NK Cells Can Stimulate Mesenchymal Stem/Stromal Cell Recruitment. Stem Cell. Rep. 6 (4), 466–473. doi:10.1016/j.stemcr.2016.02.012
Alzahrani, A. S. (2019). PI3K/Akt/mTOR Inhibitors in Cancer: At the Bench and Bedside. Semin. Cancer Biol. 59, 125–132. doi:10.1016/j.semcancer.2019.07.009
Amatullah, H., Maron-Gutierrez, T., Shan, Y., Gupta, S., Tsoporis, J. N., Varkouhi, A. K., et al. (2021). Protective Function of DJ-1/PARK7 in Lipopolysaccharide and Ventilator-Induced Acute Lung Injury. Redox Biol. 38, 101796. doi:10.1016/j.redox.2020.101796
Arthur, J. S., and Ley, S. C. (2013). Mitogen-activated Protein Kinases in Innate Immunity. Nat. Rev. Immunol. 13 (9), 679–692. doi:10.1038/nri3495
Basholli-Salihu, M., Schuster, R., Hajdari, A., Mulla, D., Viernstein, H., Mustafa, B., et al. (2017). Phytochemical Composition, Anti-inflammatory Activity and Cytotoxic Effects of Essential Oils from Three Pinus Spp. Pharm. Biol. 55 (1), 1553–1560. doi:10.1080/13880209.2017.1309555
Bdeir, K., Gollomp, K., Stasiak, M., Mei, J., Papiewska-Pajak, I., Zhao, G., et al. (2017). Platelet-Specific Chemokines Contribute to the Pathogenesis of Acute Lung Injury. Am. J. Respir. Cell. Mol. Biol. 56 (2), 261–270. doi:10.1165/rcmb.2015-0245OC
Bein, K., Birru, R. L., Wells, H., Larkin, T. P., Ge, T., and Leikauf, G. D. (2021). Sex-dependent Acrolein Sensitivity in Mice Is Associated with Differential Lung Cell, Protein, and Transcript Changes. Physiol. Rep. 9 (19), e14997. doi:10.14814/phy2.14997
Bertini, R., Allegretti, M., Bizzarri, C., Moriconi, A., Locati, M., Zampella, G., et al. (2004). Noncompetitive Allosteric Inhibitors of the Inflammatory Chemokine Receptors CXCR1 and CXCR2: Prevention of Reperfusion Injury. Proc. Natl. Acad. Sci. U. S. A. 101 (32), 11791–11796. doi:10.1073/pnas.0402090101
Boshagh, M. A., Foroutan, P., Moloudi, M. R., Fakhari, S., Malakouti, P., Nikkhoo, B., et al. (2019). ELR Positive CXCL Chemokines Are Highly Expressed in an Animal Model of Ulcerative Colitis. J. Inflamm. Res. 12, 167–174. doi:10.2147/JIR.S203714
Boucek, R. J., Steele, J., Jacobs, J. P., Steele, P., Asante-Korang, A., Quintessenza, J., et al. (2015). Ex Vivo paracrine Properties of Cardiac Tissue: Effects of Chronic Heart Failure. J. Heart Lung Transpl. 34 (6), 839–848. doi:10.1016/j.healun.2014.07.010
Bournazos, S., Wang, T. T., and Ravetch, J. V. (2016). The Role and Function of Fcγ Receptors on Myeloid Cells. Microbiol. Spectr. 4 (6), 10.1128/microbiolspec.MCHD-0045-2016. doi:10.1128/microbiolspec.MCHD-0045-2016
Brown, A. J., Joseph, P. R., Sawant, K. V., and Rajarathnam, K. (2017c). Chemokine CXCL7 Heterodimers: Structural Insights, CXCR2 Receptor Function, and Glycosaminoglycan Interactions. Int. J. Mol. Sci. 18 (4), 748. doi:10.3390/ijms18040748
Brown, A. J., Sepuru, K. M., and Rajarathnam, K. (2017a). Structural Basis of Native CXCL7 Monomer Binding to CXCR2 Receptor N-Domain and Glycosaminoglycan Heparin. Int. J. Mol. Sci. 18 (3), 508. doi:10.3390/ijms18030508
Brown, A. J., Sepuru, K. M., Sawant, K. V., and Rajarathnam, K. (2017b). Platelet-Derived Chemokine CXCL7 Dimer Preferentially Exists in the Glycosaminoglycan-Bound Form: Implications for Neutrophil-Platelet Crosstalk. Front. Immunol. 8, 1248. doi:10.3389/fimmu.2017.01248
Castor, C. W., Miller, J. W., and Walz, D. A. (1983). Structural and Biological Characteristics of Connective Tissue Activating Peptide (CTAP-III), a Major Human Platelet-Derived Growth Factor. Proc. Natl. Acad. Sci. U. S. A. 80 (3), 765–769. doi:10.1073/pnas.80.3.765
Catley, M. C., Sukkar, M. B., Chung, K. F., Jaffee, B., Liao, S. M., Coyle, A. J., et al. (2006). Validation of the Anti-inflammatory Properties of Small-Molecule IkappaB Kinase (IKK)-2 Inhibitors by Comparison with Adenoviral-Mediated Delivery of Dominant-Negative IKK1 and IKK2 in Human Airways Smooth Muscle. Mol. Pharmacol. 70 (2), 697–705. doi:10.1124/mol.106.023150
Caudrillier, A., Kessenbrock, K., Gilliss, B. M., Nguyen, J. X., Marques, M. B., Monestier, M., et al. (2012). Platelets Induce Neutrophil Extracellular Traps in Transfusion-Related Acute Lung Injury. J. Clin. Invest. 122 (7), 2661–2671. doi:10.1172/JCI61303
Chang, Y. S., Choi, S. J., Ahn, S. Y., Sung, D. K., Sung, S. I., Yoo, H. S., et al. (2013). Timing of Umbilical Cord Blood Derived Mesenchymal Stem Cells Transplantation Determines Therapeutic Efficacy in the Neonatal Hyperoxic Lung Injury. PLoS One 8 (1), e52419. doi:10.1371/journal.pone.0052419
Chang, Y. S., Choi, S. J., Sung, D. K., Kim, S. Y., Oh, W., Yang, Y. S., et al. (2011). Intratracheal Transplantation of Human Umbilical Cord Blood-Derived Mesenchymal Stem Cells Dose-Dependently Attenuates Hyperoxia-Induced Lung Injury in Neonatal Rats. Cell. Transpl. 20 (11-12), 1843–1854. doi:10.3727/096368911X565038
Chatterjee, A., Gogolak, P., Blottière, H. M., and Rajnavölgyi, É. (2015). The Impact of ATRA on Shaping Human Myeloid Cell Responses to Epithelial Cell-Derived Stimuli and on T-Lymphocyte Polarization. Mediat. Inflamm. 2015, 579830. doi:10.1155/2015/579830
Chen, X., Chen, R., Jin, R., and Huang, Z. (2020). The Role of CXCL Chemokine Family in the Development and Progression of Gastric Cancer. Int. J. Clin. Exp. Pathol. 13 (3), 484–492.
Cheng, Y., Ma, X. L., Wei, Y. Q., and Wei, X. W. (2019). Potential Roles and Targeted Therapy of the CXCLs/CXCR2 axis in Cancer and Inflammatory Diseases. Biochim. Biophys. Acta Rev. Cancer 1871 (2), 289–312. doi:10.1016/j.bbcan.2019.01.005
Choi, S., Yu, J., Kim, W., and Park, K. S. (2021). N-cadherin Mediates the Migration of Bone Marrow-Derived Mesenchymal Stem Cells toward Breast Tumor Cells. Theranostics 11 (14), 6786–6799. doi:10.7150/thno.59703
Choo-Wing, R., Nedrelow, J. H., Homer, R. J., Elias, J. A., and Bhandari, V. (2007). Developmental Differences in the Responses of IL-6 and IL-13 Transgenic Mice Exposed to Hyperoxia. Am. J. Physiol. Lung Cell. Mol. Physiol. 293 (1), L142–L150. doi:10.1152/ajplung.00434.2006
Clarke, C. H., Yip, C., Badgwell, D., Fung, E. T., Coombes, K. R., Zhang, Z., et al. (2011). Proteomic Biomarkers Apolipoprotein A1, Truncated Transthyretin and Connective Tissue Activating Protein III Enhance the Sensitivity of CA125 for Detecting Early Stage Epithelial Ovarian Cancer. Gynecol. Oncol. 122 (3), 548–553. doi:10.1016/j.ygyno.2011.06.002
Coperchini, F., Chiovato, L., Croce, L., Magri, F., and Rotondi, M. (2020). The Cytokine Storm in COVID-19: An Overview of the Involvement of the Chemokine/chemokine-Receptor System. Cytokine Growth Factor Rev. 53, 25–32. doi:10.1016/j.cytogfr.2020.05.003
De Soyza, A., Pavord, I., Elborn, J. S., Smith, D., Wray, H., Puu, M., et al. (2015). A Randomised, Placebo-Controlled Study of the CXCR2 Antagonist AZD5069 in Bronchiectasis. Eur. Respir. J. 46 (4), 1021–1032. doi:10.1183/13993003.00148-2015
Desurmont, T., Skrypek, N., Duhamel, A., Jonckheere, N., Millet, G., Leteurtre, E., et al. (2015). Overexpression of Chemokine Receptor CXCR2 and Ligand CXCL7 in Liver Metastases from Colon Cancer Is Correlated to Shorter Disease-free and Overall Survival. Cancer Sci. 106 (3), 262–269. doi:10.1111/cas.12603
Detmers, P. A., Powell, D. E., Walz, A., Clark-Lewis, I., Baggiolini, M., and Cohn, Z. A. (1991). Differential Effects of Neutrophil-Activating Peptide 1/IL-8 and its Homologues on Leukocyte Adhesion and Phagocytosis. J. Immunol. 147 (12), 4211–4217.
Di Stefano, A., Caramori, G., Gnemmi, I., Contoli, M., Bristot, L., Capelli, A., et al. (2009). Association of Increased CCL5 and CXCL7 Chemokine Expression with Neutrophil Activation in Severe Stable COPD. Thorax 64 (11), 968–975. doi:10.1136/thx.2009.113647
Di Stefano, A., Maestrelli, P., Roggeri, A., Turato, G., Calabro, S., Potena, A., et al. (1994). Upregulation of Adhesion Molecules in the Bronchial Mucosa of Subjects with Chronic Obstructive Bronchitis. Am. J. Respir. Crit. Care Med. 149 (3 Pt 1), 803–810. doi:10.1164/ajrccm.149.3.7509705
Du, J., Flynn, R., Paz, K., Ren, H. G., Ogata, Y., Zhang, Q., et al. (2018). Murine Chronic Graft-Versus-Host Disease Proteome Profiling Discovers CCL15 as a Novel Biomarker in Patients. Blood 131 (15), 1743–1754. doi:10.1182/blood-2017-08-800623
Du, Q., Li, E., Liu, Y., Xie, W., Huang, C., Song, J., et al. (2018). CTAPIII/CXCL7: a Novel Biomarker for Early Diagnosis of Lung Cancer. Cancer Med. 7 (2), 325–335. doi:10.1002/cam4.1292
Dufies, M., Giuliano, S., Viotti, J., Borchiellini, D., Cooley, L. S., Ambrosetti, D., et al. (2017). CXCL7 Is a Predictive Marker of Sunitinib Efficacy in Clear Cell Renal Cell Carcinomas. Br. J. Cancer 117 (7), 947–953. doi:10.1038/bjc.2017.276
Egbeto, I. A., Garelli, C. J., Piedra-Mora, C., Wong, N. B., David, C. N., Robinson, N. A., et al. (2020). Case Series: Gene Expression Analysis in Canine Vogt-Koyanagi-Harada/Uveodermatologic Syndrome and Vitiligo Reveals Conserved Immunopathogenesis Pathways between Dog and Human Autoimmune Pigmentary Disorders. Front. Immunol. 11, 590558. doi:10.3389/fimmu.2020.590558
Egerer, T., Martinez-Gamboa, L., Dankof, A., Stuhlmüller, B., Dörner, T., Krenn, V., et al. (2006). Tissue-specific Up-Regulation of the Proteasome Subunit Beta5i (LMP7) in Sjögren's Syndrome. Arthritis Rheum. 54 (5), 1501–1508. doi:10.1002/art.21782
El-Gedaily, A., Schoedon, G., Schneemann, M., and Schaffner, A. (2004). Constitutive and Regulated Expression of Platelet Basic Protein in Human Monocytes. J. Leukoc. Biol. 75 (3), 495–503. doi:10.1189/jlb.0603288
England, B. R., Thiele, G. M., Anderson, D. R., and Mikuls, T. R. (2018). Increased Cardiovascular Risk in Rheumatoid Arthritis: Mechanisms and Implications. BMJ 361, k1036. doi:10.1136/bmj.k1036
Fahy, J. V. (2009). Eosinophilic and Neutrophilic Inflammation in Asthma: Insights from Clinical Studies. Proc. Am. Thorac. Soc. 6 (3), 256–259. doi:10.1513/pats.200808-087RM
Fang, J. Y., and Richardson, B. C. (2005). The MAPK Signalling Pathways and Colorectal Cancer. Lancet Oncol. 6 (5), 322–327. doi:10.1016/S1470-2045(05)70168-6
Franciotta, D., Zardini, E., Ravaglia, S., Piccolo, G., Andreoni, L., Bergamaschi, R., et al. (2006). Cytokines and Chemokines in Cerebrospinal Fluid and Serum of Adult Patients with Acute Disseminated Encephalomyelitis. J. Neurol. Sci. 247 (2), 202–207. doi:10.1016/j.jns.2006.05.049
Fu, W. X., Gong, S. Y., Qian, X. P., Li, Y., Zhu, M. L., Dong, X. Y., et al. (2004). Differential Chemotactic Potential of Mouse Platelet Basic Protein for Thymocyte Subsets. Cell. Mol. Life Sci. 61 (15), 1935–1945. doi:10.1007/s00018-004-4137-5
Gear, A. R., and Camerini, D. (2003). Platelet Chemokines and Chemokine Receptors: Linking Hemostasis, Inflammation, and Host Defense. Microcirculation 10 (3-4), 335–350. doi:10.1038/sj.mn.7800198
Geng, T., Lin, T., Yang, D., Harrison, A. G., Vella, A. T., Fikrig, E., et al. (2021). A Critical Role for STING Signaling in Limiting Pathogenesis of Chikungunya Virus. J. Infect. Dis. 223 (12), 2186–2196. doi:10.1093/infdis/jiaa694
Ghallab, A. M., Eissa, R. A., and El Tayebi, H. M. (2022). CXCR2 Small-Molecule Antagonist Combats Chemoresistance and Enhances Immunotherapy in Triple-Negative Breast Cancer. Front. Pharmacol. 13, 862125. doi:10.3389/fphar.2022.862125
Gleissner, C. A., von Hundelshausen, P., and Ley, K. (2008). Platelet Chemokines in Vascular Disease. Arterioscler. Thromb. Vasc. Biol. 28 (11), 1920–1927. doi:10.1161/ATVBAHA.108.169417
Goldstein, L. J., Perez, R. P., Yardley, D., Han, L. K., Reuben, J. M., Gao, H., et al. (2020). A Window-Of-Opportunity Trial of the CXCR1/2 Inhibitor Reparixin in Operable HER-2-Negative Breast Cancer. Breast Cancer Res. 22 (1), 4. doi:10.1186/s13058-019-1243-8
Greene, S., Robbins, Y., Mydlarz, W. K., Huynh, A. P., Schmitt, N. C., Friedman, J., et al. (2020). Inhibition of MDSC Trafficking with SX-682, a CXCR1/2 Inhibitor, Enhances NK-Cell Immunotherapy in Head and Neck Cancer Models. Clin. Cancer Res. 26 (6), 1420–1431. doi:10.1158/1078-0432.CCR-19-2625
Grépin, R., Guyot, M., Giuliano, S., Boncompagni, M., Ambrosetti, D., Chamorey, E., et al. (2014). The CXCL7/CXCR1/2 axis Is a Key Driver in the Growth of Clear Cell Renal Cell Carcinoma. Cancer Res. 74 (3), 873–883. doi:10.1158/0008-5472.CAN-13-1267
Greten, F. R., and Grivennikov, S. I. (2019). Inflammation and Cancer: Triggers, Mechanisms, and Consequences. Immunity 51 (1), 27–41. doi:10.1016/j.immuni.2019.06.025
Grisaru, D., Vlodavsky, I., Prus, D., Levavi, H., Lessing, J. B., Eldor, A., et al. (2000). Connective Tissue Activating Peptide III Expression Disappears Progressively with Increased Dysplasia in Human Cervical Epithelium. Gynecol. Oncol. 79 (1), 23–27. doi:10.1006/gyno.2000.5915
Grivennikov, S. I., Greten, F. R., and Karin, M. (2010). Immunity, Inflammation, and Cancer. Cell. 140 (6), 883–899. doi:10.1016/j.cell.2010.01.025
Guerrero, S., Sánchez-Tirado, E., Agüí, L., González-Cortés, A., Yáñez-Sedeño, P., and Pingarrón, J. M. (2021). Simultaneous Determination of CXCL7 Chemokine and MMP3 Metalloproteinase as Biomarkers for Rheumatoid Arthritis. Talanta 234, 122705. doi:10.1016/j.talanta.2021.122705
Guo, Q., Jian, Z., Jia, B., and Chang, L. (2017). CXCL7 Promotes Proliferation and Invasion of Cholangiocarcinoma Cells. Oncol. Rep. 37 (2), 1114–1122. doi:10.3892/or.2016.5312
Han, Z. C., Lu, M., Li, J., Defard, M., Boval, B., Schlegel, N., et al. (1997). Platelet Factor 4 and Other CXC Chemokines Support the Survival of Normal Hematopoietic Cells and Reduce the Chemosensitivity of Cells to Cytotoxic Agents. Blood 89 (7), 2328–2335. doi:10.1182/blood.v89.7.2328
He, Y. Q., Zhou, C. C., Yu, L. Y., Wang, L., Deng, J. L., Tao, Y. L., et al. (2021). Natural Product Derived Phytochemicals in Managing Acute Lung Injury by Multiple Mechanisms. Pharmacol. Res. 163, 105224. doi:10.1016/j.phrs.2020.105224
Hill, G. R., Betts, B. C., Tkachev, V., Kean, L. S., and Blazar, B. R. (2021). Current Concepts and Advances in Graft-Versus-Host Disease Immunology. Annu. Rev. Immunol. 39, 19–49. doi:10.1146/annurev-immunol-102119-073227
Hoesel, B., and Schmid, J. A. (2013). The Complexity of NF-Κb Signaling in Inflammation and Cancer. Mol. Cancer 12, 86. doi:10.1186/1476-4598-12-86
Holz, O., Khalilieh, S., Ludwig-Sengpiel, A., Watz, H., Stryszak, P., Soni, P., et al. (2010). SCH527123, a Novel CXCR2 Antagonist, Inhibits Ozone-Induced Neutrophilia in Healthy Subjects. Eur. Respir. J. 35 (3), 564–570. doi:10.1183/09031936.00048509
Hook, J. S., Cao, M., Potera, R. M., Alsmadi, N. Z., Schmidtke, D. W., and Moreland, J. G. (2019). Nox2 Regulates Platelet Activation and NET Formation in the Lung. Front. Immunol. 10, 1472. doi:10.3389/fimmu.2019.01472
Hristov, M., Zernecke, A., Bidzhekov, K., Liehn, E. A., Shagdarsuren, E., Ludwig, A., et al. (2007a). Importance of CXC Chemokine Receptor 2 in the Homing of Human Peripheral Blood Endothelial Progenitor Cells to Sites of Arterial Injury. Circ. Res. 100 (4), 590–597. doi:10.1161/01.RES.0000259043.42571.68
Hristov, M., Zernecke, A., Liehn, E. A., and Weber, C. (2007b). Regulation of Endothelial Progenitor Cell Homing after Arterial Injury. Thromb. Haemost. 98 (2), 274–277. doi:10.1160/th07-03-0181
Huang, C., Wang, Y., Li, X., Ren, L., Zhao, J., Hu, Y., et al. (2020). Clinical Features of Patients Infected with 2019 Novel Coronavirus in Wuhan, China. Lancet 395 (10223), 497–506. doi:10.1016/S0140-6736(20)30183-5
Huang, C. S., Tang, S. J., Lee, M. H., Chang Wang, C. C., Sun, G. H., and Sun, K. H. (2018). Galectin-3 Promotes CXCR2 to Augment the Stem-like Property of Renal Cell Carcinoma. J. Cell. Mol. Med. 22 (12), 5909–5918. doi:10.1111/jcmm.13860
Huang, G., Tao, L., Shen, S., and Chen, L. (2016). Hypoxia Induced CCL28 Promotes Angiogenesis in Lung Adenocarcinoma by Targeting CCR3 on Endothelial Cells. Sci. Rep. 6, 27152. doi:10.1038/srep27152
Huang, T., Song, X., Xu, D., Tiek, D., Goenka, A., Wu, B., et al. (2020). Stem Cell Programs in Cancer Initiation, Progression, and Therapy Resistance. Theranostics 10 (19), 8721–8743. doi:10.7150/thno.41648
Hughes-Austin, J. M., Deane, K. D., Derber, L. A., Kolfenbach, J. R., Zerbe, G. O., Sokolove, J., et al. (2013). Multiple Cytokines and Chemokines Are Associated with Rheumatoid Arthritis-Related Autoimmunity in First-Degree Relatives without Rheumatoid Arthritis: Studies of the Aetiology of Rheumatoid Arthritis (SERA). Ann. Rheum. Dis. 72 (6), 901–907. doi:10.1136/annrheumdis-2012-201505
Hume, D. A., and MacDonald, K. P. (2012). Therapeutic Applications of Macrophage Colony-Stimulating Factor-1 (CSF-1) and Antagonists of CSF-1 Receptor (CSF-1R) Signaling. Blood 119 (8), 1810–1820. doi:10.1182/blood-2011-09-379214
Hussain, S. F., Yang, D., Suki, D., Aldape, K., Grimm, E., and Heimberger, A. B. (2006). The Role of Human Glioma-Infiltrating Microglia/macrophages in Mediating Antitumor Immune Responses. Neuro Oncol. 8 (3), 261–279. doi:10.1215/15228517-2006-008
Hutson, T. E., Davis, I. D., Machiels, J. P., De Souza, P. L., Rottey, S., Hong, B. F., et al. (2010). Efficacy and Safety of Pazopanib in Patients with Metastatic Renal Cell Carcinoma. J. Clin. Oncol. 28 (3), 475–480. doi:10.1200/JCO.2008.21.6994
Ivan, M., Fishel, M. L., Tudoran, O. M., Pollok, K. E., Wu, X., and Smith, P. J. (2021). Hypoxia Signaling: Challenges and Opportunities for Cancer Therapy. Semin. Cancer Biol. S1044-579X (21), 00252–2. doi:10.1016/j.semcancer.2021.10.002
Jing, X., Yang, F., Shao, C., Wei, K., Xie, M., Shen, H., et al. (2019). Role of Hypoxia in Cancer Therapy by Regulating the Tumor Microenvironment. Mol. Cancer 18 (1), 157. doi:10.1186/s12943-019-1089-9
Kalwitz, G., Neumann, K., Ringe, J., Sezer, O., Sittinger, M., Endres, M., et al. (2011). Chondrogenic Differentiation of Human Mesenchymal Stem Cells in Micro-masses Is Impaired by High Doses of the Chemokine CXCL7. J. Tissue Eng. Regen. Med. 5 (1), 50–59. doi:10.1002/term.288
Karapetsas, A., Tokamani, M., Evangelou, C., and Sandaltzopoulos, R. (2018). The Homeodomain Transcription Factor MEIS1 Triggers Chemokine Expression and Is Involved in CD8+ T-Lymphocyte Infiltration in Early Stage Ovarian Cancer. Mol. Carcinog. 57 (9), 1251–1263. doi:10.1002/mc.22840
Kinoshita, H., Yashiro, M., Fukuoka, T., Hasegawa, T., Morisaki, T., Kasashima, H., et al. (2015). Diffuse-type Gastric Cancer Cells Switch Their Driver Pathways from FGFR2 Signaling to SDF1/CXCR4 axis in Hypoxic Tumor Microenvironments. Carcinogenesis 36 (12), 1511–1520. doi:10.1093/carcin/bgv134
Kinouchi, T., Uemura, M., Wang, C., Ishizuya, Y., Yamamoto, Y., Hayashi, T., et al. (2017). Expression Level of CXCL7 in Peripheral Blood Cells Is a Potential Biomarker for the Diagnosis of Renal Cell Carcinoma. Cancer Sci. 108 (12), 2495–2502. doi:10.1111/cas.13414
Korbecki, J., Kojder, K., Kapczuk, P., Kupnicka, P., Gawrońska-Szklarz, B., Gutowska, I., et al. (2021). The Effect of Hypoxia on the Expression of CXC Chemokines and CXC Chemokine Receptors-A Review of Literature. Int. J. Mol. Sci. 22 (2), 843. doi:10.3390/ijms22020843
Kruidenier, L., MacDonald, T. T., Collins, J. E., Pender, S. L., and Sanderson, I. R. (2006). Myofibroblast Matrix Metalloproteinases Activate the Neutrophil Chemoattractant CXCL7 from Intestinal Epithelial Cells. Gastroenterology 130 (1), 127–136. doi:10.1053/j.gastro.2005.09.032
Labelle, M., Begum, S., and Hynes, R. O. (2014). Platelets Guide the Formation of Early Metastatic Niches. Proc. Natl. Acad. Sci. U. S. A. 111 (30), E3053–E3061. doi:10.1073/pnas.1411082111
Lamagna, C., Aurrand-Lions, M., and Imhof, B. A. (2006). Dual Role of Macrophages in Tumor Growth and Angiogenesis. J. Leukoc. Biol. 80 (4), 705–713. doi:10.1189/jlb.1105656
Lawrence, T. (2009). The Nuclear Factor NF-kappaB Pathway in Inflammation. Cold Spring Harb. Perspect. Biol. 1 (6), a001651. doi:10.1101/cshperspect.a001651
Lazaar, A. L., Miller, B. E., Tabberer, M., Yonchuk, J., Leidy, N., Ambery, C., et al. (2018). Effect of the CXCR2 Antagonist Danirixin on Symptoms and Health Status in COPD. Eur. Respir. J. 52 (4), 1801020. doi:10.1183/13993003.01020-2018
Lazaar, A. L., Sweeney, L. E., MacDonald, A. J., Alexis, N. E., Chen, C., and Tal-Singer, R. (2011). SB-656933, a Novel CXCR2 Selective Antagonist, Inhibits Ex Vivo Neutrophil Activation and Ozone-Induced Airway Inflammation in Humans. Br. J. Clin. Pharmacol. 72 (2), 282–293. doi:10.1111/j.1365-2125.2011.03968.x
Lee, G., Gardner, B. K., Elashoff, D. A., Purcell, C. M., Sandha, H. S., Mao, J. T., et al. (2011). Elevated Levels of CXC Chemokine Connective Tissue Activating Peptide (CTAP)-III in Lung Cancer Patients. Am. J. Transl. Res. 3 (3), 226–233. doi:10.1007/978-1-4419-6612-4_66
Lennon, V. A., Wingerchuk, D. M., Kryzer, T. J., Pittock, S. J., Lucchinetti, C. F., Fujihara, K., et al. (2004). A Serum Autoantibody Marker of Neuromyelitis Optica: Distinction from Multiple Sclerosis. Lancet 364 (9451), 2106–2112. doi:10.1016/S0140-6736(04)17551-X
Levashova, Z. B., Sharma, N., Timofeeva, O. A., Dome, J. S., and Perantoni, A. O. (2007). ELR+-CXC Chemokines and Their Receptors in Early Metanephric Development. J. Am. Soc. Nephrol. 18 (8), 2359–2370. doi:10.1681/ASN.2006040380
Li, J., Byrne, K. T., Yan, F., Yamazoe, T., Chen, Z., Baslan, T., et al. (2018). Tumor Cell-Intrinsic Factors Underlie Heterogeneity of Immune Cell Infiltration and Response to Immunotherapy. Immunity 49 (1), 178–193.e7. doi:10.1016/j.immuni.2018.06.006
Li, L., Zhang, L., Tian, Y., Zhang, T., Duan, G., Liu, Y., et al. (2019). Serum Chemokine CXCL7 as a Diagnostic Biomarker for Colorectal Cancer. Front. Oncol. 9, 921. doi:10.3389/fonc.2019.00921
Li, L., Jiang, K., Li, D., Li, D., Fan, Z., Dai, G., et al. (2021). The Chemokine CXCL7 Is Related to Angiogenesis and Associated with Poor Prognosis in Colorectal Cancer Patients. Front. Oncol., 11. doi:10.3389/fonc.2021.754221
Li, X. X., Zhang, J., Wang, Z. Y., Chen, S. Q., Zhou, W. F., Wang, T. T., et al. (2020). Increased CCL24 and CXCL7 Levels in the Cerebrospinal Fluid of Patients with Neurosyphilis. J. Clin. Lab. Anal. 34 (9), e23366. doi:10.1002/jcla.23366
Liang, L., Li, Y., Tian, X., Zhou, J., and Zhong, L. (2019). Comprehensive Lipidomic, Metabolomic and Proteomic Profiling Reveals the Role of Immune System in Vitiligo. Clin. Exp. Dermatol 44 (7), e216–e223. doi:10.1111/ced.13961
Liu, L. B., Xie, F., Chang, K. K., Li, M. Q., Meng, Y. H., Wang, X. H., et al. (2014). Hypoxia Promotes the Proliferation of Cervical Carcinoma Cells through Stimulating the Secretion of IL-8. Int. J. Clin. Exp. Pathol. 7 (2), 575–583. doi:10.4103/0377-4929.130943
Liu, S., Ginestier, C., Ou, S. J., Clouthier, S. G., Patel, S. H., Monville, F., et al. (2011). Breast Cancer Stem Cells Are Regulated by Mesenchymal Stem Cells through Cytokine Networks. Cancer Res. 71 (2), 614–624. doi:10.1158/0008-5472.CAN-10-0538
Liu, T., Barrett, N. A., Nagai, J., Lai, J., Feng, C., and Boyce, J. A. (2021). Leukotriene D4 Paradoxically Limits LTC4-Driven Platelet Activation and Lung Immunopathology. J. Allergy Clin. Immunol. 148 (1), 195–e5. e5. doi:10.1016/j.jaci.2020.10.041
Liu, Z., Chen, J., Wang, Z., Wang, Y., Zheng, D., Wang, H., et al. (2020). The CSF Levels of Neutrophil-Related Chemokines in Patients with Neuromyelitis Optica. Ann. Clin. Transl. Neurol. 7 (7), 1245–1251. doi:10.1002/acn3.51094
Lu, P., Zheng, D. C., Fang, C., Huang, J. M., Ke, W. J., Wang, L. Y., et al. (2016). Cytokines in Cerebrospinal Fluid of Neurosyphilis Patients: Identification of Urokinase Plasminogen Activator Using Antibody Microarrays. J. Neuroimmunol. 293, 39–44. doi:10.1016/j.jneuroim.2015.12.010
Lu-Emerson, C., Snuderl, M., Kirkpatrick, N. D., Goveia, J., Davidson, C., Huang, Y., et al. (2013). Increase in Tumor-Associated Macrophages after Antiangiogenic Therapy Is Associated with Poor Survival Among Patients with Recurrent Glioblastoma. Neuro Oncol. 15 (8), 1079–1087. doi:10.1093/neuonc/not082
Ludwig, A., Petersen, F., Zahn, S., Götze, O., Schröder, J. M., Flad, H. D., et al. (1997). The CXC-Chemokine Neutrophil-Activating Peptide-2 Induces Two Distinct Optima of Neutrophil Chemotaxis by Differential Interaction with Interleukin-8 Receptors CXCR-1 and CXCR-2. Blood 90 (11), 4588–4597. doi:10.1182/blood.v90.11.4588.4588_4588_4597
Luttrell, L. M., and Gesty-Palmer, D. (2010). Beyond Desensitization: Physiological Relevance of Arrestin-dependent Signaling. Pharmacol. Rev. 62 (2), 305–330. doi:10.1124/pr.109.002436
Lytle, N. K., Barber, A. G., and Reya, T. (2018). Stem Cell Fate in Cancer Growth, Progression and Therapy Resistance. Nat. Rev. Cancer 18 (11), 669–680. doi:10.1038/s41568-018-0056-x
Malkowski, M. G., Lazar, J. B., Johnson, P. H., and Edwards, B. F. (1997). The amino-terminal residues in the crystal structure of connective tissue activating peptide-III (des10) block the ELR chemotactic sequence. J. Mol. Biol. 266 (2), 367–380. doi:10.1006/jmbi.1996.0796
Matsubara, J., Honda, K., Ono, M., Tanaka, Y., Kobayashi, M., Jung, G., et al. (2011). Reduced Plasma Level of CXC Chemokine Ligand 7 in Patients with Pancreatic Cancer. Cancer Epidemiol. Biomarkers Prev. 20 (1), 160–171. doi:10.1158/1055-9965.EPI-10-0397
Maxwell, P. J., Gallagher, R., Seaton, A., Wilson, C., Scullin, P., Pettigrew, J., et al. (2007). HIF-1 and NF-kappaB-Mediated Upregulation of CXCR1 and CXCR2 Expression Promotes Cell Survival in Hypoxic Prostate Cancer Cells. Oncogene 26 (52), 7333–7345. doi:10.1038/sj.onc.1210536
Meldi, K., Qin, T., Buchi, F., Droin, N., Sotzen, J., Micol, J. B., et al. (2015). Specific Molecular Signatures Predict Decitabine Response in Chronic Myelomonocytic Leukemia. J. Clin. Invest. 125 (5), 1857–1872. doi:10.1172/JCI78752
Moldobaeva, A., and Wagner, E. M. (2005). Difference in Proangiogenic Potential of Systemic and Pulmonary Endothelium: Role of CXCR2. Am. J. Physiol. Lung Cell. Mol. Physiol. 288 (6), L1117–L1123. doi:10.1152/ajplung.00370.2004
Mollica Poeta, V., Massara, M., Capucetti, A., and Bonecchi, R. (2019). Chemokines and Chemokine Receptors: New Targets for Cancer Immunotherapy. Front. Immunol. 10, 379. doi:10.3389/fimmu.2019.00379
Moraes, T. J., Zurawska, J. H., and Downey, G. P. (2006). Neutrophil Granule Contents in the Pathogenesis of Lung Injury. Curr. Opin. Hematol. 13 (1), 21–27. doi:10.1097/01.moh.0000190113.31027.d5
Moss, R. B., Mistry, S. J., Konstan, M. W., Pilewski, J. M., Kerem, E., Tal-Singer, R., et al. (2013). Safety and Early Treatment Effects of the CXCR2 Antagonist SB-656933 in Patients with Cystic Fibrosis. J. Cyst. Fibros. 12 (3), 241–248. doi:10.1016/j.jcf.2012.08.016
Moussouras, N. A., Getschman, A. E., Lackner, E. R., Veldkamp, C. T., Dwinell, M. B., and Volkman, B. F. (2017). Differences in Sulfotyrosine Binding Amongst CXCR1 and CXCR2 Chemokine Ligands. Int. J. Mol. Sci. 18 (9), 1894. doi:10.3390/ijms18091894
Narasaraju, T., Yang, E., Samy, R. P., Ng, H. H., Poh, W. P., Liew, A. A., et al. (2011). Excessive Neutrophils and Neutrophil Extracellular Traps Contribute to Acute Lung Injury of Influenza Pneumonitis. Am. J. Pathol. 179 (1), 199–210. doi:10.1016/j.ajpath.2011.03.013
Nazy, I., Jevtic, S. D., Moore, J. C., Huynh, A., Smith, J. W., Kelton, J. G., et al. (2021). Platelet‐activating Immune Complexes Identified in Critically Ill COVID‐19 Patients Suspected of Heparin‐induced Thrombocytopenia. J. Thromb. Haemost. 19 (5), 1342–1347. doi:10.1111/jth.15283
Nikolic, T., Bouma, G., Drexhage, H. A., and Leenen, P. J. (2005). Diabetes-prone NOD Mice Show an Expanded Subpopulation of Mature Circulating Monocytes, Which Preferentially Develop into Macrophage-like Cells In Vitro. J. Leukoc. Biol. 78 (1), 70–79. doi:10.1189/jlb.1104662
O'Byrne, P. M., Metev, H., Puu, M., Richter, K., Keen, C., Uddin, M., et al. (2016). Efficacy and Safety of a CXCR2 Antagonist, AZD5069, in Patients with Uncontrolled Persistent Asthma: a Randomised, Double-Blind, Placebo-Controlled Trial. Lancet Respir. Med. 4 (10), 797–806. doi:10.1016/S2213-2600(16)30227-2
Opfermann, P., Derhaschnig, U., Felli, A., Wenisch, J., Santer, D., Zuckermann, A., et al. (2015). A Pilot Study on Reparixin, a CXCR1/2 Antagonist, to Assess Safety and Efficacy in Attenuating Ischaemia-Reperfusion Injury and Inflammation after On-Pump Coronary Artery Bypass Graft Surgery. Clin. Exp. Immunol. 180 (1), 131–142. doi:10.1111/cei.12488
Oral, H., Kanzler, I., Tuchscheerer, N., Curaj, A., Simsekyilmaz, S., Sönmez, T. T., et al. (2013). CXC Chemokine KC Fails to Induce Neutrophil Infiltration and Neoangiogenesis in a Mouse Model of Myocardial Infarction. J. Mol. Cell. Cardiol. 60, 1–7. doi:10.1016/j.yjmcc.2013.04.006
Patsouras, M. D., Sikara, M. P., Grika, E. P., Moutsopoulos, H. M., Tzioufas, A. G., and Vlachoyiannopoulos, P. G. (2015). Elevated Expression of Platelet-Derived Chemokines in Patients with Antiphospholipid Syndrome. J. Autoimmun. 65, 30–37. doi:10.1016/j.jaut.2015.08.001
Pilatova, K., Greplova, K., Demlova, R., Bencsikova, B., Klement, G. L., and Zdrazilova-Dubska, L. (2013). Role of Platelet Chemokines, PF-4 and CTAP-III, in Cancer Biology. J. Hematol. Oncol. 6, 42. doi:10.1186/1756-8722-6-42
Pillai, M. M., Iwata, M., Awaya, N., Graf, L., and Torok-Storb, B. (2006). Monocyte-derived CXCL7 Peptides in the Marrow Microenvironment. Blood 107 (9), 3520–3526. doi:10.1182/blood-2005-10-4285
Priceman, S. J., Sung, J. L., Shaposhnik, Z., Burton, J. B., Torres-Collado, A. X., Moughon, D. L., et al. (2010). Targeting Distinct Tumor-Infiltrating Myeloid Cells by Inhibiting CSF-1 Receptor: Combating Tumor Evasion of Antiangiogenic Therapy. Blood 115 (7), 1461–1471. doi:10.1182/blood-2009-08-237412
Provencio, J. J., Altay, T., Smithason, S., Moore, S. K., and Ransohoff, R. M. (2011). Depletion of Ly6G/C(+) Cells Ameliorates Delayed Cerebral Vasospasm in Subarachnoid Hemorrhage. J. Neuroimmunol. 232 (1-2), 94–100. doi:10.1016/j.jneuroim.2010.10.016
Qian, B. Z., Li, J., Zhang, H., Kitamura, T., Zhang, J., Campion, L. R., et al. (2011). CCL2 Recruits Inflammatory Monocytes to Facilitate Breast-Tumour Metastasis. Nature 475 (7355), 222–225. doi:10.1038/nature10138
Quijada, H., Bermudez, T., Kempf, C. L., Valera, D. G., Garcia, A. N., Camp, S. M., et al. (2021). Endothelial eNAMPT Amplifies Pre-clinical Acute Lung Injury: Efficacy of an eNAMPT-Neutralising Monoclonal Antibody. Eur. Respir. J. 57 (5), 2002536. doi:10.1183/13993003.02536-2020
Rajarathnam, K., and Desai, U. R. (2020). Structural Insights into How Proteoglycans Determine Chemokine-Cxcr1/cxcr2 Interactions: Progress and Challenges. Front. Immunol. 11, 660. doi:10.3389/fimmu.2020.00660
Rajarathnam, K., Schnoor, M., Richardson, R. M., and Rajagopal, S. (2019). How Do Chemokines Navigate Neutrophils to the Target Site: Dissecting the Structural Mechanisms and Signaling Pathways. Cell. Signal 54, 69–80. doi:10.1016/j.cellsig.2018.11.004
Ren, J., Zhou, T., Pilli, V. S. S., Phan, N., Wang, Q., Gupta, K., et al. (2019). Novel Paracrine Functions of Smooth Muscle Cells in Supporting Endothelial Regeneration Following Arterial Injury. Circ. Res. 124 (8), 1253–1265. doi:10.1161/CIRCRESAHA.118.314567
Russo, R. C., Garcia, C. C., Teixeira, M. M., and Amaral, F. A. (2014). The CXCL8/IL-8 Chemokine Family and its Receptors in Inflammatory Diseases. Expert Rev. Clin. Immunol. 10 (5), 593–619. doi:10.1586/1744666X.2014.894886
Saetang, J., Tedasen, A., Sangkhathat, S., Sangkaew, N., Dokduang, S., Prompat, N., et al. (2021). Low Piperine Fractional Piper Nigrum Extract Enhanced the Antitumor Immunity via Regulating the Th1/Th2/Treg Cell Subsets on NMU-Induced Tumorigenesis Rats. Planta Med. 88 (7), 527–537. doi:10.1055/a-1458-5646
Samy, Z. A., Al-Abdullah, L., Turcani, M., Craik, J., and Redzic, Z. (2018). Rat Astrocytes during Anoxia: Secretome Profile of Cytokines and Chemokines. Brain Behav. 8 (7), e01013. doi:10.1002/brb3.1013
Santarpia, L., Lippman, S. M., and El-Naggar, A. K. (2012). Targeting the MAPK-RAS-RAF Signaling Pathway in Cancer Therapy. Expert Opin. Ther. Targets 16 (1), 103–119. doi:10.1517/14728222.2011.645805
Sarode, P., Schaefer, M. B., Grimminger, F., Seeger, W., and Savai, R. (2020). Macrophage and Tumor Cell Cross-Talk Is Fundamental for Lung Tumor Progression: We Need to Talk. Front. Oncol. 10, 324. doi:10.3389/fonc.2020.00324
Sato, E., Olson, S. H., Ahn, J., Bundy, B., Nishikawa, H., Qian, F., et al. (2005). Intraepithelial CD8+ Tumor-Infiltrating Lymphocytes and a High CD8+/regulatory T Cell Ratio Are Associated with Favorable Prognosis in Ovarian Cancer. Proc. Natl. Acad. Sci. U. S. A. 102 (51), 18538–18543. doi:10.1073/pnas.0509182102
Schenk, B. I., Petersen, F., Flad, H. D., and Brandt, E. (2002). Platelet-derived Chemokines CXC Chemokine Ligand (CXCL)7, Connective Tissue-Activating Peptide III, and CXCL4 Differentially Affect and Cross-Regulate Neutrophil Adhesion and Transendothelial Migration. J. Immunol. 169 (5), 2602–2610. doi:10.4049/jimmunol.169.5.2602
Shi, L., Zhang, J., Wu, P., Feng, K., Li, J., Xie, Z., et al. (2009). Discovery and Identification of Potential Biomarkers of Pediatric Acute Lymphoblastic Leukemia. Proteome Sci. 7, 7. doi:10.1186/1477-5956-7-7
Sica, A., Schioppa, T., Mantovani, A., and Allavena, P. (2006). Tumour-associated Macrophages Are a Distinct M2 Polarised Population Promoting Tumour Progression: Potential Targets of Anti-cancer Therapy. Eur. J. Cancer 42 (6), 717–727. doi:10.1016/j.ejca.2006.01.003
Sironi, M., Martinez, F. O., D'Ambrosio, D., Gattorno, M., Polentarutti, N., Locati, M., et al. (2006). Differential Regulation of Chemokine Production by Fcgamma Receptor Engagement in Human Monocytes: Association of CCL1 with a Distinct Form of M2 Monocyte Activation (M2b, Type 2). J. Leukoc. Biol. 80 (2), 342–349. doi:10.1189/jlb.1005586
Smolen, J. S., Aletaha, D., Koeller, M., Weisman, M. H., and Emery, P. (2007). New Therapies for Treatment of Rheumatoid Arthritis. Lancet 370 (9602), 1861–1874. doi:10.1016/S0140-6736(07)60784-3
Spaks, A. (2017). Role of CXC Group Chemokines in Lung Cancer Development and Progression. J. Thorac. Dis. 9 (Suppl. 3), S164–S171. doi:10.21037/jtd.2017.03.61
Spicer, J. D., McDonald, B., Cools-Lartigue, J. J., Chow, S. C., Giannias, B., Kubes, P., et al. (2012). Neutrophils Promote Liver Metastasis via Mac-1-Mediated Interactions with Circulating Tumor Cells. Cancer Res. 72 (16), 3919–3927. doi:10.1158/0008-5472.CAN-11-2393
Srinagesh, H. K., and Ferrara, J. L. M. (2019). MAGIC Biomarkers of Acute Graft-Versus-Host Disease: Biology and Clinical Application. Best. Pract. Res. Clin. Haematol. 32 (4), 101111. doi:10.1016/j.beha.2019.101111
Strazza, M., and Mor, A. (2020). The Complexity of Targeting Chemokines to Promote a Tumor Immune Response. Inflammation 43 (4), 1201–1208. doi:10.1007/s10753-020-01235-8
Strieter, R. M., Burdick, M. D., Mestas, J., Gomperts, B., Keane, M. P., and Belperio, J. A. (2006). Cancer CXC Chemokine Networks and Tumour Angiogenesis. Eur. J. Cancer 42 (6), 768–778. doi:10.1016/j.ejca.2006.01.006
Sueoka, H., Hirano, T., Uda, Y., Iimuro, Y., Yamanaka, J., and Fujimoto, J. (2014). Blockage of CXCR2 Suppresses Tumor Growth of Intrahepatic Cholangiocellular Carcinoma. Surgery 155 (4), 640–649. doi:10.1016/j.surg.2013.12.037
Sui, S., An, X., Xu, C., Li, Z., Hua, Y., Huang, G., et al. (2020). An Immune Cell Infiltration-Based Immune Score Model Predicts Prognosis and Chemotherapy Effects in Breast Cancer. Theranostics 10 (26), 11938–11949. doi:10.7150/thno.49451
Sun, X., Chen, Q., Zhang, L., Chen, J., and Zhang, X. (2021). Exploration of Prognostic Biomarkers and Therapeutic Targets in the Microenvironment of Bladder Cancer Based on CXC Chemokines. Math. Biosci. Eng. 18 (5), 6262–6287. doi:10.3934/mbe.2021313
Suzumura, E. A., Figueiró, M., Normilio-Silva, K., Laranjeira, L., Oliveira, C., Buehler, A. M., et al. (2014). Effects of Alveolar Recruitment Maneuvers on Clinical Outcomes in Patients with Acute Respiratory Distress Syndrome: a Systematic Review and Meta-Analysis. Intensive Care Med. 40 (9), 1227–1240. doi:10.1007/s00134-014-3413-6
Tai, P. K., Liao, J. F., Hossler, P. A., Castor, C. W., and Carter-Su, C. (1992). Regulation of Glucose Transporters by Connective Tissue Activating Peptide-III Isoforms. J. Biol. Chem. 267 (27), 19579–19586. doi:10.1016/s0021-9258(18)41814-5
Tang, K. H., Li, S., Khodadadi-Jamayran, A., Jen, J., Han, H., Guidry, K., et al. (2022). Combined Inhibition of SHP2 and CXCR1/2 Promotes Antitumor T-Cell Response in NSCLC. Cancer Discov. 12 (1), 47–61. doi:10.1158/2159-8290.CD-21-0369
Tang, Z., Yu, M., Miller, F., Berk, R. S., Tromp, G., and Kosir, M. A. (2008). Increased Invasion through Basement Membrane by CXCL7-Transfected Breast Cells. Am. J. Surg. 196 (5), 690–696. doi:10.1016/j.amjsurg.2008.08.001
Taniguchi, K., and Karin, M. (2018). NF-κB, Inflammation, Immunity and Cancer: Coming of Age. Nat. Rev. Immunol. 18 (5), 309–324. doi:10.1038/nri.2017.142
Tojo, K., Yoshida, T., Yazawa, T., and Goto, T. (2018). Driving-pressure-independent Protective Effects of Open Lung Approach against Experimental Acute Respiratory Distress Syndrome. Crit. Care 22 (1), 228. doi:10.1186/s13054-018-2154-2
Traves, S. L., Smith, S. J., Barnes, P. J., and Donnelly, L. E. (2004). Specific CXC but Not CC Chemokines Cause Elevated Monocyte Migration in COPD: a Role for CXCR2. J. Leukoc. Biol. 76 (2), 441–450. doi:10.1189/jlb.1003495
Turner, J. E., Steinmetz, O. M., Stahl, R. A., and Panzer, U. (2007). Targeting of Th1-Associated Chemokine Receptors CXCR3 and CCR5 as Therapeutic Strategy for Inflammatory Diseases. Mini Rev. Med. Chem. 7 (11), 1089–1096. doi:10.2174/138955707782331768
Ulivi, P., Mercatali, L., Casoni, G. L., Scarpi, E., Bucchi, L., Silvestrini, R., et al. (2013). Multiple Marker Detection in Peripheral Blood for NSCLC Diagnosis. PLoS One 8 (2), e57401. doi:10.1371/journal.pone.0057401
Unver, N., Esendagli, G., Yilmaz, G., and Guc, D. (2015). CXCL7-induced Macrophage Infiltration in Lung Tumor Is Independent of CXCR2 Expression: CXCL7-Induced Macrophage Chemotaxis in LLC Tumors. Cytokine 75 (2), 330–337. doi:10.1016/j.cyto.2015.07.018
Unver, N. (2021). Identification of the Dominant Angiogenic CXCL Class Chemokines Associated with Non-small Cell Lung Cancer via Bioinformatics Tools. Med. Oncol. 38 (6), 68. doi:10.1007/s12032-021-01517-7
Van Damme, J., Struyf, S., and Opdenakker, G. (2004). Chemokine-protease Interactions in Cancer. Semin. Cancer Biol. 14 (3), 201–208. doi:10.1016/j.semcancer.2003.10.007
van der Heul-Nieuwenhuijsen, L., Padmos, R. C., Drexhage, R. C., de Wit, H., Berghout, A., and Drexhage, H. A. (2010). An Inflammatory Gene-Expression Fingerprint in Monocytes of Autoimmune Thyroid Disease Patients. J. Clin. Endocrinol. Metab. 95 (4), 1962–1971. doi:10.1210/jc.2009-1455
Vandercappellen, J., Van Damme, J., and Struyf, S. (2008). The Role of CXC Chemokines and Their Receptors in Cancer. Cancer Lett. 267 (2), 226–244. doi:10.1016/j.canlet.2008.04.050
Wallace, E. M., Rizzi, J. P., Han, G., Wehn, P. M., Cao, Z., Du, X., et al. (2016). A Small-Molecule Antagonist of HIF2α Is Efficacious in Preclinical Models of Renal Cell Carcinoma. Cancer Res. 76 (18), 5491–5500. doi:10.1158/0008-5472.CAN-16-0473
Walz, A., and Baggiolini, M. (1989). A Novel Cleavage Product of Beta-Thromboglobulin Formed in Cultures of Stimulated Mononuclear Cells Activates Human Neutrophils. Biochem. Biophys. Res. Commun. 159 (3), 969–975. doi:10.1016/0006-291x(89)92203-1
Walz, A., Dewald, B., von Tscharner, V., and Baggiolini, M. (1989). Effects of the Neutrophil-Activating Peptide NAP-2, Platelet Basic Protein, Connective Tissue-Activating Peptide III and Platelet Factor 4 on Human Neutrophils. J. Exp. Med. 170 (5), 1745–1750. doi:10.1084/jem.170.5.1745
Wang, J., He, Q., Shao, Y. G., and Ji, M. (2013). Chemokines Fluctuate in the Progression of Primary Breast Cancer. Eur. Rev. Med. Pharmacol. Sci. 17 (5), 596–608. doi:10.1007/s13318-012-0097-6
Wang, W., Deng, Z., Liu, G., Yang, J., Zhou, W., Zhang, C., et al. (2021). Platelet-derived Extracellular Vesicles Promote the Migration and Invasion of Rheumatoid Arthritis Fibroblast-like Synoviocytes via CXCR2 Signaling. Exp. Ther. Med. 22 (4), 1120. doi:10.3892/etm.2021.10554
White, G. E., Iqbal, A. J., and Greaves, D. R. (2013). CC Chemokine Receptors and Chronic Inflammation-Ttherapeutic Opportunities and Pharmacological Challenges. Pharmacol. Rev. 65 (1), 47–89. doi:10.1124/pr.111.005074
Whiteside, T. L. (2018). Exosome and Mesenchymal Stem Cell Cross-Talk in the Tumor Microenvironment. Semin. Immunol. 35, 69–79. doi:10.1016/j.smim.2017.12.003
Whitmore, L. C., Hilkin, B. M., Goss, K. L., Wahle, E. M., Colaizy, T. T., Boggiatto, P. M., et al. (2013). NOX2 Protects against Prolonged Inflammation, Lung Injury, and Mortality Following Systemic Insults. J. Innate Immun. 5 (6), 565–580. doi:10.1159/000347212
Wu, Q., Chen, Y., Zhang, W., Song, S., Xu, Z., Zhang, H., et al. (2020). Upregulation of Chemokines in the Paraventricular Nucleus of the Hypothalamus in Rats with Stress-Induced Hypertension. Med. Sci. Monit. 26, e926807. doi:10.12659/MSM.926807
Wysocki, P. J. (2009). mTOR in Renal Cell Cancer: Modulator of Tumor Biology and Therapeutic Target. Expert Rev. Mol. Diagn 9 (3), 231–241. doi:10.1586/erm.09.8
Xie, F., Liu, L. B., Shang, W. Q., Chang, K. K., Meng, Y. H., Mei, J., et al. (2015). The Infiltration and Functional Regulation of Eosinophils Induced by TSLP Promote the Proliferation of Cervical Cancer Cell. Cancer Lett. 364 (2), 106–117. doi:10.1016/j.canlet.2015.04.029
Xiong, D., Pan, J., Zhang, Q., Szabo, E., Miller, M. S., Lubet, R. A., et al. (2017). Bronchial Airway Gene Expression Signatures in Mouse Lung Squamous Cell Carcinoma and Their Modulation by Cancer Chemopreventive Agents. Oncotarget 8 (12), 18885–18900. doi:10.18632/oncotarget.13806
Yamamoto, Y., Kuroda, K., Sera, T., Sugimoto, A., Kushiyama, S., Nishimura, S., et al. (2019). The Clinicopathological Significance of the CXCR2 Ligands, CXCL1, CXCL2, CXCL3, CXCL5, CXCL6, CXCL7, and CXCL8 in Gastric Cancer. Anticancer Res. 39 (12), 6645–6652. doi:10.21873/anticanres.13879
Yan, J., Zhao, Q., Wang, J., Tian, X., Wang, J., Xia, X., et al. (2021). FGL2-wired Macrophages Secrete CXCL7 to Regulate the Stem-like Functionality of Glioma Cells. Cancer Lett. 506, 83–94. doi:10.1016/j.canlet.2021.02.021
Yatim, N., Boussier, J., Chocron, R., Hadjadj, J., Philippe, A., Gendron, N., et al. (2021). Platelet Activation in Critically Ill COVID-19 Patients. Ann. Intensive Care 11 (1), 113. doi:10.1186/s13613-021-00899-1
Ye, L. Y., Chen, W., Bai, X. L., Xu, X. Y., Zhang, Q., Xia, X. F., et al. (2016). Hypoxia-Induced Epithelial-To-Mesenchymal Transition in Hepatocellular Carcinoma Induces an Immunosuppressive Tumor Microenvironment to Promote Metastasis. Cancer Res. 76 (4), 818–830. doi:10.1158/0008-5472.CAN-15-0977
Yee, J., Sadar, M. D., Sin, D. D., Kuzyk, M., Xing, L., Kondra, J., et al. (2009). Connective Tissue-Activating Peptide III: a Novel Blood Biomarker for Early Lung Cancer Detection. J. Clin. Oncol. 27 (17), 2787–2792. doi:10.1200/JCO.2008.19.4233
Yeo, L., Adlard, N., Biehl, M., Juarez, M., Smallie, T., Snow, M., et al. (2016). Expression of Chemokines CXCL4 and CXCL7 by Synovial Macrophages Defines an Early Stage of Rheumatoid Arthritis. Ann. Rheum. Dis. 75 (4), 763–771. doi:10.1136/annrheumdis-2014-206921
Yin, P., Gui, L., Wang, C., Yan, J., Liu, M., Ji, L., et al. (2020). Targeted Delivery of CXCL9 and OX40L by Mesenchymal Stem Cells Elicits Potent Antitumor Immunity. Mol. Ther. 28 (12), 2553–2563. doi:10.1016/j.ymthe.2020.08.005
Yu, M., Berk, R., and Kosir, M. A. (2010). CXCL7-Mediated Stimulation of Lymphangiogenic Factors VEGF-C, VEGF-D in Human Breast Cancer Cells. J. Oncol. 2010, 939407. doi:10.1155/2010/939407
Yu, M., Tang, Z., Alousi, S., Berk, R. S., Miller, F., and Kosir, M. A. (2007). Expression Patterns of Lymphangiogenic and Angiogenic Factors in a Model of Breast Ductal Carcinoma In Situ. Am. J. Surg. 194 (5), 594–599. doi:10.1016/j.amjsurg.2007.08.007
Yu, Z., Qin, C., Cao, M., He, X., Ren, H., and Liu, H. (2021). The Potential Genes Mediate the Pathogenicity of Allogeneic CD4(+)T Cell in aGVHD Mouse Model. Biomed. Res. Int. 2021, 9958745. doi:10.1155/2021/9958745
Zarbock, A., Polanowska-Grabowska, R. K., and Ley, K. (2007). Platelet-neutrophil-interactions: Linking Hemostasis and Inflammation. Blood Rev. 21 (2), 99–111. doi:10.1016/j.blre.2006.06.001
Zcharia, E., Metzger, S., Chajek-Shaul, T., Friedmann, Y., Pappo, O., Aviv, A., et al. (2001). Molecular Properties and Involvement of Heparanase in Cancer Progression and Mammary Gland Morphogenesis. J. Mammary Gland. Biol. Neoplasia 6 (3), 311–322. doi:10.1023/a:1011375624902
Zeiser, R., and Blazar, B. R. (2017). Pathophysiology of Chronic Graft-Versus-Host Disease and Therapeutic Targets. N. Engl. J. Med. 377 (26), 2565–2579. doi:10.1056/NEJMra1703472
Zhang, B., Zhang, Y., Yao, G., Gao, J., Yang, B., Zhao, Y., et al. (2012). M2-polarized Macrophages Promote Metastatic Behavior of Lewis Lung Carcinoma Cells by Inducing Vascular Endothelial Growth Factor-C Expression. Clin. (Sao Paulo) 67 (8), 901–906. doi:10.6061/clinics/2012(08)08
Zhang, Z., Tan, X., Luo, J., Cui, B., Lei, S., Si, Z., et al. (2018). GNA13 Promotes Tumor Growth and Angiogenesis by Upregulating CXC Chemokines via the NF-Κb Signaling Pathway in Colorectal Cancer Cells. Cancer Med. 7 (11), 5611–5620. doi:10.1002/cam4.1783
Zhu, Y., Knolhoff, B. L., Meyer, M. A., Nywening, T. M., West, B. L., Luo, J., et al. (2014). CSF1/CSF1R Blockade Reprograms Tumor-Infiltrating Macrophages and Improves Response to T-Cell Checkpoint Immunotherapy in Pancreatic Cancer Models. Cancer Res. 74 (18), 5057–5069. doi:10.1158/0008-5472.CAN-13-3723
Glossary
CXCL7 C-X-C motif ligand 7
CXCR1 C-X-C chemokine receptor 1
CXCR2 C-X-C chemokine receptor 2
NAP2 neutrophil-activating peptide 2
PPBP pro-platelet basic protein
CTAP-III connective tissue-activating peptide III
βTG-Ag β-thromboglobulin antigen
GAG glycosaminoglycan
VEGF vascular endothelial growth factor
ALI/ARDS acute lung injury/acute respiratory distress syndrome
BALF bronchoalveolar lavage fluid
COVID-19 new coronavirus pneumonia
AUC area under the curve
BPD Bronchopulmonary dysplasia
UCB umbilical cord blood
SIRS systemic inflammatory response syndrome
ROS reactive oxygen species
NOX2 NADPH oxidase 2
NET extracellular neutrophil traps
OLA open lung approach
PEEP positive end-expiratory pressure
RM recruitment maneuver
COPD Chronic obstructive lung disease
ICAM-1 intercellular adhesion molecule 1
ELAM-1 endothelial leukocyte adhesion molecule 1
IKK I-kappa B kinase
HASM human airway smooth muscle
DCV delayed cerebral vasospasm
CSF cerebrospinal fluid
MSCs mesenchymal stem cell
PKCδ protein kinase C-delta
STAT3 signal transducer and activator of transcription 3
IBD inflammatory bowel disease
CD Crohn’s disease
UC ulcerative colitis
MMP3 matrix metalloproteinase 3
RA rheumatoid arthritis
STING stimulator-of-interferon-gene
CHIKV chikungunya virus
PEVs platelet-derived extracellular vesicles
RA-FLS rheumatoid arthritis fibroblast-like synoviocytes
GVHD graft-versus-host disease
HSCT hematopoietic stem cell transplantation
NMO optic neuromyelitis optica
MS multiple sclerosis
IIDDs idiopathic inflammatory demyelinating diseases
ADEM acute disseminated encephalomyelitis
uPA urokinase plasminogen activator
LTD4 leukotrienes D4
LTC4 leukotrienes C4
CysLT2R cysteinyl leukotriene receptor 2
ERK extracellular signal-regulated kinase
JNK c-Jun terminal kinase
p38 MAPK p38 mitogen-activated protein kinase
MAPK mitogen-activated protein kinase
APS antiphospholipid syndrome
VKH Vogt-Koyanagi-Harada syndrome
RAEB refractory anemia with excess blasts
RAEB-t refractory anemia with excess blasts in transformation
ALL acute lymphoblastic leukemia
AML acute myeloid leukemia
LSCC lung squamous cell carcinoma
NSCL non-small cell lung cancer
ccRCC clear cell renal cell carcinoma
RCC renal cell carcinoma
IL-1β interleukin 1β
CA Ⅸ carbonic anhydrase 9
Gals-3 galectin-3
MMP9 matrix metalloproteinase 9
CC cholangiocarcinoma
OS overall survival
ICC intrahepatic cholangiocarcinoma
TME tumor microenvironment
CSCs cancer stem cells
PFPE piperine fractional piper nigrum extract
CRC colorectal cancer
OCRC obstructive colorectal cancer
DFS disease-free survival
MDSCs myeloid-derived suppressor cells
TAMs tumor-associated macrophages
AATs anti-angiogenic therapies
FGL2 fibrinogen-like protein 2
MEIS1 myeloid ecotropic viral integration site 1
BLCA bladder cancer
LLC Lewis lung cancer
HIF-1 hypoxia-inducible factors 1
cAMP cyclic adenosine monophosphate
IP3 inositol triphosphate
DAG diacylglycerol
Keywords: CXCL7, CXCR1, CXCR2, inflammatory diseases, tumor
Citation: Wu Q, Tu H and Li J (2022) Multifaceted Roles of Chemokine C-X-C Motif Ligand 7 in Inflammatory Diseases and Cancer. Front. Pharmacol. 13:914730. doi: 10.3389/fphar.2022.914730
Received: 07 April 2022; Accepted: 07 June 2022;
Published: 28 June 2022.
Edited by:
Chiara Bolego, University of Padua, ItalyReviewed by:
Massimiliano Cadamuro, University of Padua, ItalyKrishna Mohan Poluri, Indian Institute of Technology Roorkee, India
Copyright © 2022 Wu, Tu and Li. This is an open-access article distributed under the terms of the Creative Commons Attribution License (CC BY). The use, distribution or reproduction in other forums is permitted, provided the original author(s) and the copyright owner(s) are credited and that the original publication in this journal is cited, in accordance with accepted academic practice. No use, distribution or reproduction is permitted which does not comply with these terms.
*Correspondence: Jian Li, ndefy03048@ncu.edu.cn