- 1Faculty of Life Science and Technology, Kunming University of Science and Technology, Kunming, China
- 2Faculty of Architecture and City Planning, Kunming University of Science and Technology, Kunming, China
- 3UWA School of Agriculture and Environment, Faculty of Science, University of Western Australia, Perth, WA, Australia
Aluminum (Al) toxicity is a key factor limiting plant growth and crop production on acid soils. Increasing the plant Al-detoxification capacity and/or breeding Al-resistant cultivars are a cost-effective strategy to support crop growth on acidic soils. The plasma membrane H+-ATPase plays a central role in all plant physiological processes. Changes in the activity of the plasma membrane H+-ATPase through regulating the expression and phosphorylation of this enzyme are also involved in many plant responses to Al toxicity. The plasma membrane H+-ATPase mediated H+ influx may be associated with the maintenance of cytosolic pH and the plasma membrane gradients as well as Al-induced citrate efflux mediated by a H+-ATPase-coupled MATE co-transport system. In particular, modulating the activity of plasma membrane H+-ATPase through application of its activators (e.g., magnesium or IAA) or using transgenics has effectively enhanced plant resistance to Al stress in several species. In this review, we critically assess the available knowledge on the role of the plasma membrane H+-ATPase in plant responses to Al stress, incorporating physiological and molecular aspects.
Introduction
Acid soils (pHwater < 5.5) are found worldwide, occupying up to 50% of arable land (Kochian et al., 2015), mostly in developing countries in Africa, Asia, and South America. Aluminum (Al) toxicity is a major factor limiting plant growth and crop yield in acidic soils. Al toxicity has been shown to affect the plasma membrane structure, induce root cell death and inhibit nutrient uptake, thereby leading to significant reductions in plant growth and development (Chen et al., 2011). Liming can increase soil pH, but this is frequently neither an economic option for farmers nor an effective strategy for alleviating subsoil acidity (Whitten et al., 2000). In contrast, increasing capacity of plants to cope with Al toxicity by enhancing Al detoxification and/or breeding Al-resistant cultivars is a more cost-effective way of mitigating the problem.
The plasma membrane H+-ATPase, the most abundant membrane protein as a single polypeptide of about 100 kDa, belongs to a large superfamily of pumps termed P-type ATPases (Palmgren, 2001; Rengel et al., 2015). Using the chemical energy of ATP, the plasma membrane H+-ATPases extrude protons from cells to generate an electrochemical proton gradient. The generation of this gradient has a major role in providing the energy for secondary ion transport across the plasma membrane, enabling physiological functions such as nutrient uptake, intracellular pH regulation, stomatal opening, and cell growth (Falhof et al., 2016). Additionally, the plasma membrane H+-ATPase is involved in plant adaptation to environmental stresses, such as salinity, P deficiency and Al toxicity (Janicka-Russak and Kabala, 2015; Yu et al., 2015).
Aluminum toxicity affects the expression and post-translation of the plasma membrane H+-ATPase in some plant species (Shen et al., 2005; Chen et al., 2013, 2015; Guo et al., 2013). In particular, modulation of the activity of this enzyme through application of Mg, IAA or using transgenic methods can effectively enhance Al-resistance in faba bean (Chen et al., 2015), soybean (Wang et al., 2016), Arabidopsis (Wu et al., 2008), and tobacco (Guo, 2013). The plant Al-resistance mechanisms, especially those based on Al-induced organic acid anion exudation, have been reviewed many times (Liu et al., 2014; Kochian et al., 2015; Chen and Liao, 2016); however, a role of the plasma membrane H+-ATPase in plant responses to Al stress has not been well assessed. In this review, we appraise the literature on this nascent field, paying particular attention to physiological and molecular aspects of the plasma membrane H+-ATPase involvement in plant responses to Al stress.
Aluminum Detoxification Strategies
Plants have evolved two main Al resistance strategies, namely avoidance and tolerance. Tolerant plants, such as buckwheat (Ma et al., 1998), hydrangea (Ma et al., 1997), melastoma (Watanabe et al., 1998), and tea (Morita et al., 2008) allow Al accumulation in plant tissues, using Al sequestration in the vacuole and/or Al detoxification via Al binding to organic acid anions or proteins as the tolerance mechanisms. In contrast, plants with the avoidance mechanisms decrease Al accumulation in roots via cell wall polysaccharide modifications (Schmohl et al., 2000; Yang et al., 2008, 2011a, 2016; Li et al., 2016) or exudation of organic acid anions from root tips (Ma, 2000; Chen and Liao, 2016).
Many publications progressed the knowledge of plant resistance to Al stress (Kochian et al., 2015; Chen and Liao, 2016). For example, Al resistance is associated with upregulation of the ABC transporters (ALS3 and ALS1) in Arabidopsis (Larsen et al., 2005, 2007; Huang et al., 2009) and the bacterial-type ABC transporters (STAR1 and STAR2) in rice (Huang et al., 2009). The Al-induced expression of the ALMT (Al-activated malate transporter) and MATE (multidrug and toxic compound extrusion) transporter genes (Sasaki et al., 2004; Hoekenga et al., 2006; Magalhaes et al., 2007) enhances root exudation of malate and citrate, respectively. The zinc-finger transcription factors, such as STOP1 (sensitive to proton rhizotoxicity) in Arabidopsis (Iuchi et al., 2007; Sawaki et al., 2009) and ART1 (Al resistance transcription factor) in rice (Yamaji et al., 2009), play central roles in regulating expression of many genes associated with Al resistance. Additionally, activation of the plasma membrane H+-ATPase through regulating the expression and/or phosphorylation of this enzyme coincides with citrate exudation and Al resistance in many plant species (Yu et al., 2015).
Functions and Regulation of the Plasma Membrane H+-ATPase
Critical Functions
The plasma membrane H+-ATPases (proton pumps) are the primary transporters that translocate positive charges (protons) out of the cytosol using ATP as an energy source, thereby forming a membrane potential difference across the plasma membrane (negative on the inside). Using the proton electrical gradients created by the plasma membrane H+-ATPase, cations (e.g., K+, Na+, NH4+, Mg2+, Ca2+), anions (e.g., NO3-, SO42-, Cl-), and neutral compounds (e.g., glucose) can be taken up across the plasma membrane via secondary carrier proteins (Figure 1).
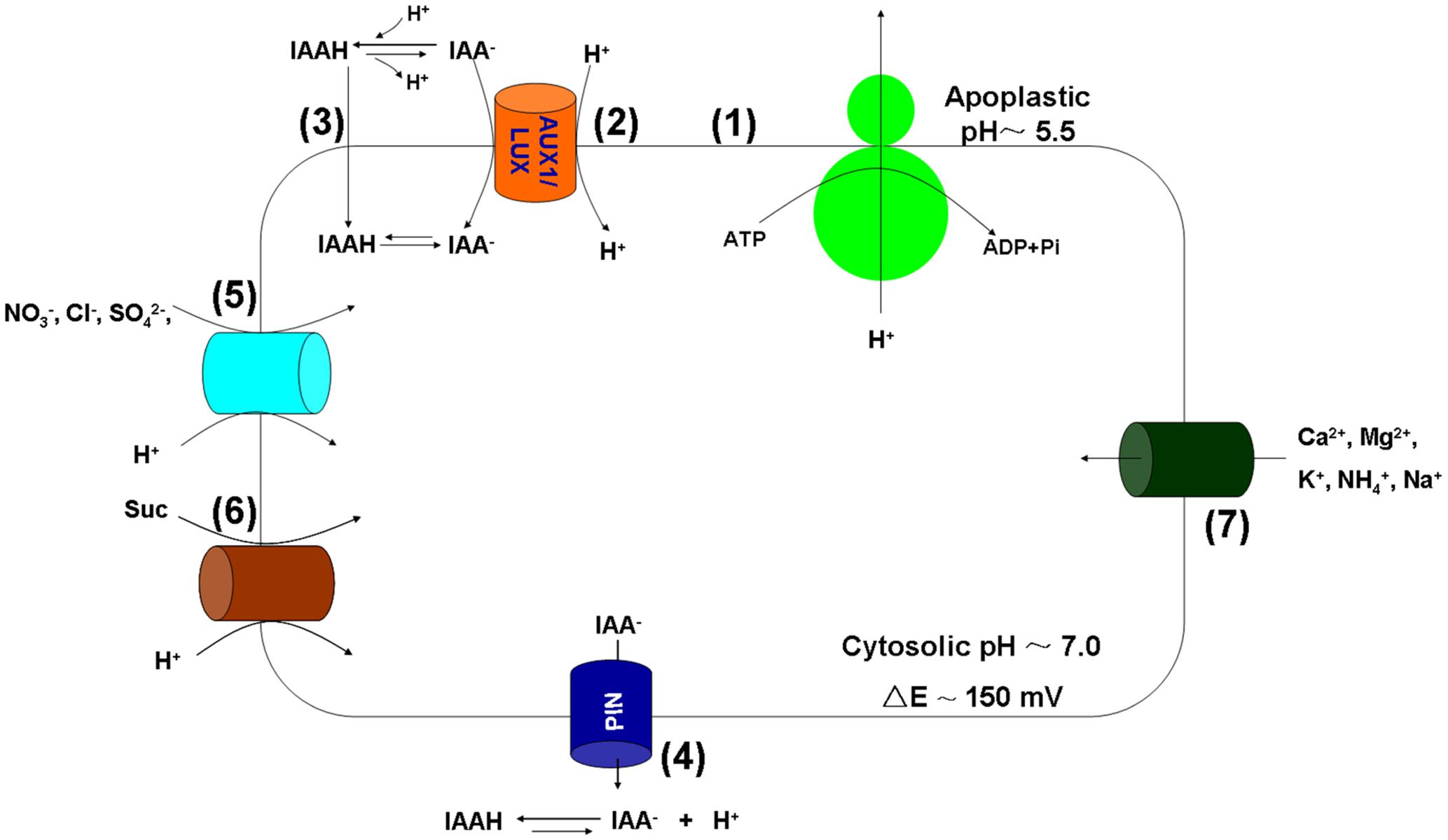
FIGURE 1. The functions of the plasma membrane H+-ATPase in IAA polar transport and nutrient uptake. (1) The proton pump; (2) AUX1/LAX-mediated IAA-/H+ symport; (3) Lipophilic diffusion of protonated IAAH; (4) PIN proteins-mediated IAA- efflux; (5) Anion (NO3-, Cl-, SO42-, etc.) uptake via symport with H+; (6) Proton-coupled sucrose transporters-mediated sucrose (Suc) phloem loading; (7) cation (Mg2+, Ca2+, K+, etc.) uptake through various transporters.
One of the most basic functions of the plasma membrane H+-ATPase in plants is the involvement in auxin polar transport and signaling. Auxin is synthesized in the plant apical growing regions and transported to roots through the vascular and bundle sheath tissues (Titapiwatanakun and Murphy, 2009). As protons accumulate in the apoplast (pH∼5.5) (lower pH than in the cytosol, Figure 1), 10–25% of IAA (pKa 4.85) is protonated to form electrically neutral IAAH (Yang et al., 2006). IAA can thus enter the cell by either lipophilic diffusion of protonated IAAH along the concentration gradient or via the AUX1/LAX influx carrier-mediated IAA-/H+ symport (Figure 1). Inside the cell, all IAA molecules are deprotonated because the cytosolic pH is relatively high (pH∼7.0). The efflux of IAA- is accomplished by PIN proteins, resulting in activation of the plasma membrane H+-ATPase (Figure 1) and acidification of the cell wall (apoplast). For instance, activation of the plasma membrane H+-ATPase is important in auxin-mediated cell elongation during wheat embryo development (Rober-Kleber et al., 2003). In Arabidopsis, auxin induces hypocotyl elongation through phosphorylation and activation of the plasma membrane H+-ATPase (Takahashi et al., 2012).
Regulatory Mechanisms
The plasma membrane H+-ATPase is encoded by a multigene family and contains several isoforms. For example, 11 isoforms of plasma membrane H+-ATPase in Arabidopsis thaliana (AHA1–AHA11) (Palmgren, 2001), 10 genes in Oryza sativa (OsA1–OsA10) (Arango et al., 2003), 9 genes in Nicotiana plumbaginifolia (PMA1–PMA9) (Oufattole et al., 2000), and 5 genes in Vicia faba (VHA1–VHA5) (Neuhaus et al., 2013) have been identified. Various genes are expressed in the same organ or plant tissues. For example, AHA1 and AHA2 are expressed in virtually all tissues and organs, AHA10 has been identified during seed development (Harper et al., 1994), and isoform AHA4 is expressed in the root endodermis, flowers and during silique maturation (Vitart et al., 2001). In faba bean, VHA1 and VHA2 are expressed mainly in guard cells, but to some extent throughout the plant as well (Hentzen et al., 1996). The expression of genes encoding the plasma membrane H+-ATPase is regulated by various environmental factors, including iron deficiency (Santi et al., 2005), Al toxicity (Yu et al., 2015), and heavy metals stress (Jemo et al., 2007).
The activity of H+-ATPase is tightly regulated by the phosphorylation and dephosphorylation processes, although neither the protein kinase nor the protein phosphatase directly regulating phosphorylation of this enzyme in plant cells has been identified. The best characterized mechanism involves the phosphorylation of the penultimate Thr residue in the autoinhibitory domain at the C-terminus, and the subsequent binding of 14-3-3 regulatory proteins (Falhof et al., 2016). The binding of 14-3-3 protein displaces the C-autoinhibitory domain, resulting in the formation of a dodecameric complex composed of six H+-ATPases and six 14-3-3 regulatory proteins and resulting in the activated plasma membrane H+-ATPase (Haruta et al., 2015). Many signals, including auxin, blue light and the fungal toxin fusicoccin (FC), regulate the phosphorylation level of the penultimate Thr at the C terminus of H+-ATPase and its interaction with the 14-3-3 proteins, affecting cell growth and stomatal movements (Emi et al., 2001; Hohm et al., 2014; Falhof et al., 2016; Wang et al., 2016).
The Plasma Membrane H+-ATPase and Aluminum Toxicity
Changes in the Plasma Membrane H+-ATPase Activity in Plant Responses to Aluminum Toxicity
Under Al stress, the most important functional change occurring in the plasma membrane is the alteration of membrane potential (Ahn and Matsumoto, 2006), which is dependent on the plasma membrane H+-ATPase. The changes in the membrane potential and the plasma membrane H+-ATPase activity are related to the root part, the degree of Al stress and Al sensitivity of plant species and genotypes. For example, the Al stress induced depolarization of the plasma membrane in tobacco suspension-cultured cells (Sivaguru et al., 2005), squash (Ahn et al., 2001), and barley (Matsumoto, 1988), suggesting decreased H+-ATPase activity, but either depolarization or hyperpolarization of the plasma membrane was recorded in Arabidopsis (Bose et al., 2010a,b) and soybean (Wang et al., 2016). In wheat root tips, Al exposure caused hyperpolarization in the Al-resistant and depolarization in the Al-sensitive genotypes (Ahn et al., 2004). Al-induced proton release is associated with activation of the plasma membrane H+-ATPase in tea plants (Qing et al., 2017). In soybean and faba bean roots, Al significantly increased the activity of the plasma membrane H+-ATPase in the Al-resistant but not the Al-sensitive cultivars (Shen et al., 2005; Kim et al., 2010; Chen et al., 2013).
The activity of plasma membrane H+-ATPase is affected by Al at the transcription, translation, and post-translation levels. In soybean and faba bean, higher plasma membrane H+-ATPase concentration and activity coincided with enhanced expression of the gene and greater abundance of the plasma membrane H+-ATPase protein in the Al-resistant cultivars (Shen et al., 2005; Guo et al., 2013). Furthermore, Al increased the phosphorylation levels of the plasma membrane and its interaction with the 14-3-3 proteins in a time-dependent manner in the Al-resistant cultivars of faba bean and soybean (Shen et al., 2005; Chen Q. et al., 2012; Chen et al., 2013). It is likely that Al activates an unknown kinase, resulting in phosphorylation of the plasma membrane H+-ATPase, maintenance of strong interaction with the 14-3-3 proteins and thus increased H+-ATPase activity. However, identification and characterization of a kinase that regulates phosphorylation of the plasma membrane H+-ATPase remains to be reported.
Involvement of the Plasma Membrane H+-ATPase in MATE-Mediated Citrate Exudation
Citrate and malate are intermediates in the tricarboxylic acid (TCA) cycle. The Al-induced organic acid anion exudation is controlled by specific transporters, such as ALMT1 for malate and MATE for citrate, along with enzymes of organic acid metabolism, such as citrate synthase, malate dehydrogenase, and phosphoenolpyruvate carboxylase (Chen and Liao, 2017). Beside the expression of citrate transporter, the exudation of citrate also requires activation of the plasma membrane H+-ATPase as shown in many plant species. Under low-P conditions, increased citrate exudation was related to activation of the plasma membrane H+-ATPase in white lupin (Lupinus albus) (Yan et al., 2002) and blue lupin (Lupinus pilosus) (Ligaba et al., 2004). A mutant carrot (Daucus carota) cell line (can grow on water-insoluble phosphate) exhibited an enhancement in citrate exudation and plasma membrane H+-ATPase activity when grown in an Al-phosphate medium (Ohno et al., 2003). In Al-resistant soybean roots under Al stress, fusicoccin significantly enhanced (by 85%) and vanadium ions significantly inhibited (by 53%) activity of the plasma membrane H+-ATPase, which was associated with Al-induced citrate exudation increasing by 58% and decreasing by 52% in the fusicoccin and vanadium treatments, respectively (Shen et al., 2005). Chen et al. (2013) in faba bean and Guo et al. (2013) in soybean reported similar results.
In soybean roots, the Al-induced enhancement of the plasma membrane H+-ATPase and the related citrate exudation coincided with the increased gene expression and protein abundance as well as enhanced phosphorylation of this enzyme (Shen et al., 2005; Guo et al., 2013). In both Al-resistant and Al-sensitive cultivars of faba bean, the interaction between the phosphorylated plasma membrane H+-ATPase and the 14-3-3 protein was stimulated by FC but inhibited by 5′-AMP (adenosine 5′-monophosphate) in the presence of Al; in addition, the activity of the plasma membrane H+-ATPase and the related citrate exudation were increased, respectively, about 1.7- and 2.7-fold by FC and decreased, respectively, about 60 and 70% by 5′-AMP (Chen et al., 2013), indicating that post-translational regulation of the plasma membrane H+-ATPase plays an important role in Al-induced citrate exudation.
It is interesting to note that, unlike the secretion of malate and oxalate, only the exudation of citrate is dependent on the plasma membrane H+-ATPase. For example, neither P-deficiency-induced malate exudation by Lupinus pilosus (Ligaba et al., 2004) nor Al-induced oxalate exudation by tomato (Yang et al., 2011b) was related to the plasma membrane H+-ATPase activity. Wu et al. (2014) found that transgenic Arabidopsis lines containing Brassica oleracea MATE gene had stronger citrate exudation coupled with a higher H+ efflux activity than wild-type plants.
Electrophysiological analysis using Xenopus oocytes showed that the MATE family transporters from sorghum (SbMATE) (Magalhaes et al., 2007), maize (ZmMATE1) (Maron et al., 2010), and rice bean (VuMATE1) (Yang et al., 2011c) mediated significant 14C-labeled-citrate efflux as well as proton influx across the plasma membrane, suggesting that the MATEs might be citrate/H+ antiport transporters (Kochian et al., 2015). This suggestion, however, needs to be interpreted with respect to the ionic as well as charge gradients across the plasma membrane. Citric acid is a triprotic weak acid having pKa values for the three stepwise carboxylic group dissociations of 3.1, 4.8, and 6.4. Hence, at a relatively high (∼7.0) cytosolic pH, citric acid is dissociated to citrate anion (Cit3-) and H+ (Figure 2). The MATE-mediated citrate anion efflux is likely to be coupled with the H+-ATPase-driven H+ efflux (to balance charges), meaning that Al-induced citrate exudation is mediated by a plasma membrane co-transport (symport) system in which H+-ATPase and MATE are coupled. The H+ influx (purportedly via MATE transporters) as observed in Xenopus oocytes (Magalhaes et al., 2007; Maron et al., 2010; Yang et al., 2011c) may be secondary in nature, and its role remains to be properly explained. It may be tempting to speculate that such influx of H+ may be associated with the general maintenance of cytosolic pH and the plasma membrane gradients as well as balancing of secondary ion transports rather than primarily as an antiport action directly coupled with citrate exudation via MATEs. This explanation is well aligned with the primary roles of MATEs as organic cation (citrate is never a cation) antiporters (coupled with H+ or Na+ influx, Jin et al., 2014) in waste disposal (e.g., in kidney and liver cells, Nies et al., 2016) as well as in bacterial or cancer cell resistance to drugs (e.g., Tanaka et al., 2013). Hence, there is no doubt that MATEs can allow H+ influx (for the mechanism, see Nishima et al., 2016), but we would suggest it is premature to assign the label of citrate/H+ antiporters to the MATE transporters functioning in citrate (anion) efflux. Alternatively, it may also be tempting to suggest that in plants MATEs transport Al::citrate complexes from roots to shoots, thus playing a role in Al detoxification, rather than effluxing citrate from roots to mediate Al exclusion at the root surface; however, there is no experimental evidence to support this suggestion, except that MATE transporter OsFRDL1 was found to transport Fe::citrate complexes from rice roots to shoots (Yokosho et al., 2009), and the same route of Al::malate transport in Arabidopsis was claimed for the nodulin 26-like intrinsic protein (NIP1;2) from the aquaporin family (Wang J. et al., 2017; Wang Y. et al., 2017). The MATE transport system in plants is indeed complex, and a substantial future work is needed to shed more light on that complexity.
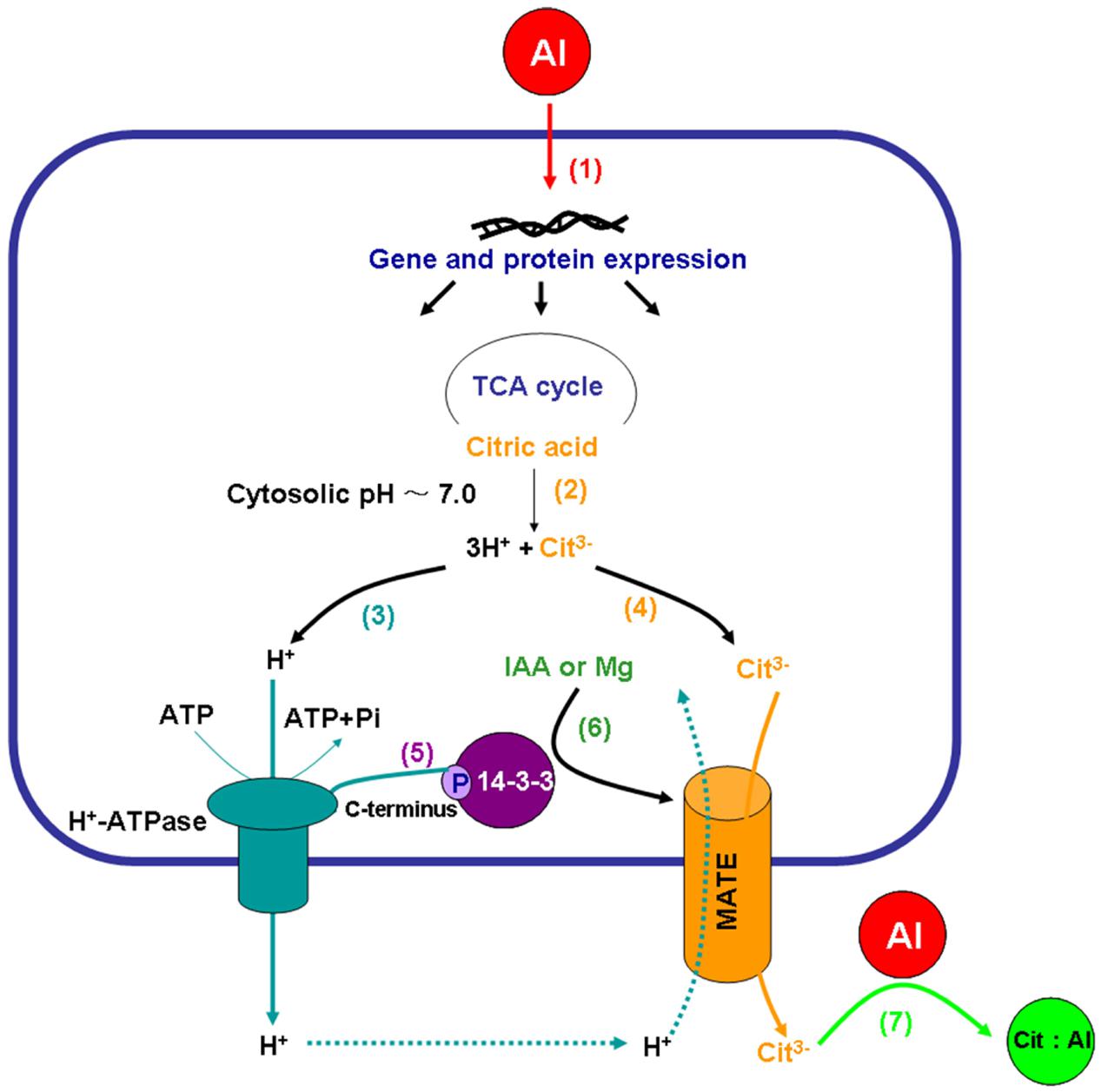
FIGURE 2. The proposed regulatory roles of plasma membrane H+-ATPase in Al-induced citrate exudation from plant roots. Al toxicity is perceived by root cells, inducing expression of numerous genes and proteins (1), including tricarboxylic acid (TCA) cycle enzymes, the plasma membrane H+-ATPase, 14-3-3 proteins and MATE transporters. Citric acid is dissociated to citrate anion (Cit3-) and H+ at a relative high cytosolic pH∼7.0 (2), followed by the plasma membrane H+-ATPase-mediated H+ efflux (3) coupled with MATE-mediated citrate (Cit3-) exudation (4) possibly associated with proton influx. The phosphorylation of the plasma membrane H+-ATPase and interaction with the 14-3-3 proteins (5) were enhanced by the application of Mg and/or IAA (6) under Al stress, resulting in activation of both the plasma membrane H+-ATPase and the MATE citrate transporter. Citrate exuded from plant roots forms a stable non-toxic complex with Al in the rhizosphere (7).
Activation of the Plasma Membrane H+-ATPase Improves Plant Resistance to Aluminum Stress
Magnesium Availability
Magnesium (Mg) is an essential nutrient for plant growth and has a number of key functions in plants (Cakmak and Kirkby, 2008). It is an activator of a large number of enzymes, such as phosphatases, protein kinases, RNA polymerases, and carboxylases (Bose et al., 2011). Magnesium is also pivotal to the function of most ATPase proteins and essential for maintaining the proton pump activity at the plasma membrane (Brooker and Slayman, 1983; Gibrat et al., 1989). Under constant Al3+ ionic activity, the Al-resistant genotypes had higher Mg concentration than the Al-sensitive genotypes of wheat (Silva et al., 2010), maize (Mariano and Keltjens, 2005; Giannakoula et al., 2008), sorghum (Baligar et al., 1993), Arabidopsis (Bose et al., 2013), and rice (Sivaguru and Paliwal, 1993). Additionally, it has been shown that Mg can alleviate Al toxicity to plants (Rengel et al., 2015). Relatively high concentrations (millimolar) of Mg were found to alleviate Al toxicity in Poaceae species, such as wheat and rice (Ryan et al., 1997; Watanabe and Okada, 2005; Chen Z.C. et al., 2012). This effect may be attributed to Mg reducing Al saturation at the apoplasmic binding sites and decreasing Al activity at the root-cell plasma-membrane surface. On the other hand, in legumes, relatively low (micromolar) concentrations of Mg significantly alleviated Al-induced root growth inhibition in soybean (Silva et al., 2001), rice bean (Yang et al., 2007), and faba bean (Chen et al., 2015) through enhancement of Al-induced citrate exudation. It is important to point out that the Mg-related enhancement of Al-induced citrate exudation was dependent on the activation of the plasma membrane H+-ATPase in rice bean (Yang et al., 2007) and faba bean (Chen et al., 2015).
Chen et al. (2015) determined the expression of the genes encoding MATE as well as the plasma membrane H+-ATPase in the presence or absence of Mg under Al stress in faba bean roots. The expression of both a putative MATE-like gene and vha2 (Vicia faba plasma membrane H+-ATPase 2) was induced by Al but not Mg. Furthermore, immunoprecipitation, pull-down and Western blot analyses showed that phosphorylation of the penultimate Thr-residue in VHA2, as well as its in vivo and in vitro association with the vf14-3-3b protein, both increased in the presence of Mg under Al stress, which corresponded well with the Mg-mediated enhancement of (i) the plasma membrane H+-ATPase activity and (ii) citrate exudation under Al stress (Chen, 2012; Chen et al., 2015). The Mg-mediated increase in phosphorylation of the Thr-residue in the plasma membrane H+-ATPase may enhance binding of the 14-3-3 protein and thus activation of the enzyme.
Auxin Transport
The auxin signaling has been shown to be involved in abiotic stresses such as salt (Albacete et al., 2008) and low P (Shen et al., 2006). In soybean roots, low-P induced endogenous IAA accumulation that (similarly to an exogenous application of 10 μM IAA) increased the activity of plasma membrane H+-ATPase and enhanced P uptake (Shen et al., 2006). In Arabidopsis, the pin2 and aux1 mutants exhibited the much decreased activity of the plasma membrane H+-ATPase and depressed root elongation under alkaline (Xu et al., 2012) and acidic stress (Inoue et al., 2016).
The Al-induced auxin accumulation in Arabidopsis root-apex transition zone was crucial for Al-related root growth inhibition (Yang et al., 2014). Primary root elongation was less inhibited in the Arabidopsis auxin-polar-transport mutants AUX1 (AUXIN RESISTANT 1) and PIN2 (PIN-FORMED) than wild-type plants under Al stress (Sun et al., 2010). Consistently, auxin signaling was involved in the Al-induced primary root growth inhibition, but promoted lateral root formation and maturation (Ruiz-Herrera and Lopez-Bucio, 2013). In wheat roots, the Al-induced endogenous IAA accumulation correlated significantly with malate exudation; similarly, the exogenous treatment with 10 μM IAA enhanced, whereas that with 30 μM 1-naphthylphthalamic acid (NPA, an auxin efflux transport inhibitor) decreased, malate efflux and Al concentration (Yang et al., 2011d). In soybean roots, Wang (2014) found that the mRNA abundance of GmPIN2 and IAA concentration in soybean roots were increased after treatment with 25 or 50 μM Al, but not 200 μM Al. Furthermore, the Al-induced citrate exudation as well as the expression of GmMATE and the phosphorylation of the plasma membrane H+-ATPase in soybean roots were significantly increased by IAA, but decreased by IAA transport inhibitor TIBA (2,3,5-triiodobenzoic acid) (Wang et al., 2016). In accordance with these pharmacological results obtained in soybean, the genetic data using pin2 and aux1-7 Arabidopsis mutants showed a decrease not just in the activity of the plasma membrane H+-ATPase, but also in citrate exudation and expression of AtMATE (Yu, 2017). Hence, it is suggested that activation of the plasma membrane H+-ATPase and upregulation of the MATE expression are involved in the auxin enhancement of Al-induced citrate exudation.
Transgenic Methods to Increase Activity of the Plasma Membrane H+-ATPase
Overexpressing either the plasma membrane H+-ATPase directly or its regulatory components has been shown to be effective in enhancing the activity of this enzyme. Ectopic expression of PeHA1 from Populus euphratica significantly increased the proton pumping activity of the plasma membrane H+-ATPase and salt tolerance in transgenic Arabidopsis (Wang et al., 2013). In tobacco plants, expressing a plasma membrane H+-ATPase isoform lacking the autoinhibitory domain (ΔPMA4) constitutively activated the plasma membrane H+-ATPase and resulted in abnormal leaf inclination and twisted stems, suggesting alterations in cell expansion (Gevaudant et al., 2007). Under salt stress conditions, the ΔPMA4 plants displayed increased salt tolerance during germination and seedling growth (Gevaudant et al., 2007).
Under Al stress, overexpression of AHA1 in Arabidopsis significantly increased organic acid anion exudation and Al resistance in comparison with the wild-type plants (Wu et al., 2008). To investigate the interaction between 14-3-3 proteins and plasma membrane H+-ATPase of soybean in the regulation of citrate exudation and Al resistance, Guo (2013) obtained transgenic tobacco overexpressing soybean SGF14a that encodes a 14-3-3 protein. The expression of SGF14a in tobacco significantly increased the activity and phosphorylation of the plasma membrane H+-ATPase and interaction with the 14-3-3 proteins, resulting in activation of the Al-induced citrate exudation and Al resistance. Overexpression of soybean ΔGHA2 lacking the autoinhibitory domain of the plasma membrane H+-ATPase isoform had no effect on phosphorylation of the plasma membrane H+-ATPase and the interaction with 14-3-3 proteins, but it significantly improved plasma membrane H+-ATPase activity, citrate exudation, Al resistance, and the growth of transgenic tobacco in acidic soil (Guo, 2013).
Conclusion
The plant plasma membrane H+-ATPase plays the important roles in plant growth under optimal and Al stress conditions. In several plant species, the Al-resistant genotypes show higher activity of the plasma membrane H+-ATPase than the Al-sensitive ones, which is associated with higher gene expression and protein abundance as well as the penultimate threonine-residue phosphorylation of the enzyme and its interaction with 14-3-3 proteins. Providing the motive force for MATE-mediated transport of citrate anion out of root epidermal cells and maintaining the physiological H+ and charge gradients across the plasma membrane might be the two main functions of the plasma membrane H+-ATPase under Al stress. Further work is needed to improve plant Al resistance by modulating activity of the plasma membrane H+-ATPase, especially in plant species relying on facilitated citrate exudation as the main Al-resistance mechanism. However, some fundamental questions remain unclear. For example, activation of the plasma membrane H+-ATPase occurs also under P deficiency and salt and heavy metal stresses. Possibly, Al toxicity shares a similar signaling pathway with these abiotic stresses to regulate the plasma membrane H+-ATPase activity. Moreover, the research on the interaction between Al toxicity and plasma membrane H+-ATPase has focused so far on just several plant species, such as soybean, faba bean, wheat, tea, and Arabidopsis; therefore, the function of plasma membrane H+-ATPase in response to Al toxicity in other plant species, especially in Gramineae such as barley, maize, and sorghum where MATE-mediated citrate exudation occurs under Al stress, needs to be elucidated.
Author Contributions
ZR and QC conceived this work, wrote and revised the manuscript. JZ, JW, and DL co-wrote the manuscript. XK, LC, YY, and XC provided suggestions and revised the manuscript. All of the authors reviewed the final version of the manuscript and approved the manuscript for publication.
Conflict of Interest Statement
The authors declare that the research was conducted in the absence of any commercial or financial relationships that could be construed as a potential conflict of interest.
Acknowledgments
This work was supported by the National Natural Science Foundation of China (Nos. 31360340 and 31660595). ZR was supported by Australian Research Council (DP160104434).
References
Ahn, S. J., and Matsumoto, H. (2006). The role of the plasma membrane in the response of plant roots to aluminum toxicity. Plant Signal. Behav. 1, 37–45. doi: 10.4161/psb.1.2.2588
Ahn, S. J., Rengel, Z., and Matsumoto, H. (2004). Aluminum-induced plasma membrane surface potential and H+-ATPase activity in near-isogenic wheat lines differing in tolerance to aluminum. New Phytol. 162, 71–79. doi: 10.1111/j.1469-8137.2004.01009.x
Ahn, S. J., Sivaguru, M., Osawa, H., Chung, G. C., and Matsumoto, H. (2001). Aluminum inhibits the H(+)-ATPase activity by permanently altering the plasma membrane surface potentials in squash roots. Plant Physiol. 126, k1381–1390. doi: 10.1104/pp.126.4.1381
Albacete, A., Ghanem, M. E., Martinez-Andujar, C., Acosta, M., Sanchez-Bravo, J., Martinez, V., et al. (2008). Hormonal changes in relation to biomass partitioning and shoot growth impairment in salinized tomato (Solanum lycopersicum L.) plants. J. Exp. Bot. 59, 4119–4131. doi: 10.1093/jxb/ern251
Arango, M., Gevaudant, F., Oufattole, M., and Boutry, M. (2003). The plasma membrane proton pump ATPase: the significance of gene subfamilies. Planta 216, 355–365.
Baligar, V. C., Schaffert, R. E., Dossantos, H. L., Pitta, G. V. E., and Filho, D. C. (1993). Growth and nutrient-uptake parameters in sorghum as influenced by aluminum. Agron. J. 85, 1068–1074. doi: 10.2134/agronj1993.00021962008500050021x
Bose, J., Babourina, O., and Rengel, Z. (2011). Role of magnesium in alleviation of aluminium toxicity in plants. J. Exp. Bot. 62, 2251–2264. doi: 10.1093/jxb/erq456
Bose, J., Babourina, O., Shabala, S., and Rengel, Z. (2010a). Aluminium-induced ion transport in Arabidopsis: the relationship between Al tolerance and root ion flux. J. Exp. Bot. 61, 3163–3175. doi: 10.1093/jxb/erq143
Bose, J., Babourina, O., Shabala, S., and Rengel, Z. (2010b). Aluminum-dependent dynamics of ion transport in Arabidopsis: specificity of low pH and aluminum responses. Physiol. Plant. 139, 401–412. doi: 10.1111/j.1399-3054.2010.01377.x
Bose, J., Babourina, O., Shabala, S., and Rengel, Z. (2013). Low-pH and aluminum resistance in Arabidopsis correlates with high cytosolic magnesium content and increased magnesium uptake by plant roots. Plant Cell Physiol. 54, 1093–1104. doi: 10.1093/pcp/pct064
Brooker, R. J., and Slayman, C. W. (1983). Effects of Mg2+ Ions on the Plasma membrane H+-ATPase of Neurospora Crassa. II. Kinetic studies. J. Biol. Chem. 258, 8833–8838.
Cakmak, I., and Kirkby, E. A. (2008). Role of magnesium in carbon partitioning and alleviating photooxidative damage. Physiol. Plant 133, 692–704. doi: 10.1111/j.1399-3054.2007.01042.x
Chen, L., and Liao, H. (2017). Engineering crop nutrient efficiency for sustainable agriculture. J. Integr. Plant Biol. doi: 10.1111/jipb.12559 [Epub ahead of print].
Chen, Q. (2012). The Physiological Basis and Molecular Mechanisms of Alfalfa (Medicago sativa) and Broad Bean (Vicia faba) in Response to Al Stress. Ph.D. thesis, Kunming University of Science and Technology, Kunming.
Chen, Q., Guo, C. L., Wang, P., Chen, X. Q., Wu, K. H., Li, K. Z., et al. (2013). Up-regulation and interaction of the plasma membrane H+-ATPase and the 14-3-3 protein are involved in the regulation of citrate exudation from the broad bean (Vicia faba L.) under Al stress. Plant Physiol. Biochem. 70, 504–511. doi: 10.1016/j.plaphy.2013.06.015
Chen, Q., Kan, Q., Wang, P., Yu, W., Yu, Y., Zhao, Y., et al. (2015). Phosphorylation and interaction with the 14-3-3 protein of the plasma membrane H+-ATPase are involved in the regulation of magnesium-mediated increases in aluminum-induced citrate exudation in broad bean (Vicia faba. L). Plant Cell Physiol. 56, 1144–1153. doi: 10.1093/pcp/pcv038
Chen, Q., Wu, K., Zhang, Y., Xuan-Huyen, P., Li, K., Yu, Y., et al. (2012). Physiological and molecular responses of broad bean (Vicia faba L.) to aluminum stress. Acta Physiol. Plant. 34, 2251–2263. doi: 10.1007/s11738-012-1026-7
Chen, Z. C., Yamaji, N., Motoyama, R., Nagamura, Y., and Ma, J. F. (2012). Up-regulation of a magnesium transporter gene OsMGT1 is required for conferring aluminum tolerance in rice. Plant Physiol. 159, 1624–1633. doi: 10.1104/pp.112.199778
Chen, Q., Zhang, X., Wang, S., Wang, Q., Wang, G., Nian, H., et al. (2011). Transcriptional and physiological changes of alfalfa in response to aluminium stress. J. Agric. Sci. 149, 737–751. doi: 10.1017/S0021859611000256
Chen, Z. C., and Liao, H. (2016). Organic acid anions: an effective defensive weapon for plants against aluminum toxicity and phosphorus deficiency in acidic soils. J. Genet. Genomics 43, 631–638. doi: 10.1016/j.jgg.2016.11.003
Emi, T., Kinoshita, T., and Shimazaki, K. (2001). Specific binding of vf14-3-3a isoform to the plasma membrane H+-ATPase in response to blue light and fusicoccin in guard cells of broad bean. Plant Physiol. 125, 1115–1125. doi: 10.1104/pp.125.2.1115
Falhof, J., Pedersen, J. T., Fuglsang, A. T., and Palmgren, M. (2016). Plasma membrane H(+)-ATPase regulation in the center of plant physiology. Mol. Plant 9, 323–337. doi: 10.1016/j.molp.2015.11.002
Gevaudant, F., Duby, G., Von Stedingk, E., Zhao, R., Morsomme, P., and Boutry, M. (2007). Expression of a constitutively activated plasma membrane H+-ATPase alters plant development and increases salt tolerance. Plant Physiol. 144, 1763–1776. doi: 10.1104/pp.107.103762
Giannakoula, A., Moustakas, M., Mylona, P., Papadakis, I., and Yupsanis, T. (2008). Aluminum tolerance in maize is correlated with increased levels of mineral nutrients, carbohydrates and proline, and decreased levels of lipid peroxidation and Al accumulation. J. Plant Physiol. 165, 385–396. doi: 10.1016/j.jplph.2007.01.014
Gibrat, R., Grouzis, J. P., Rigaud, J., Galtier, N., and Grignon, C. (1989). Electrostatic analysis of effects of Ions on the inhibition of corn root plasma membrane Mg2+-ATPase by the bivalent ortho-vanadate. Biochim. Biophys. Acta 979, 46–52. doi: 10.1016/0005-2736(89)90521-X
Guo, C. (2013). 14-3-3 Proteins and Plasma Membrane H+-ATPase of Black Soybean in Response Mechanism to Aluminum Stress. Master’s thesis, Kunming University of Science and Technology, Kunming.
Guo, C. L., Chen, Q., Zhao, X. L., Chen, X. Q., Zhao, Y., Wang, L., et al. (2013). Al-enhanced expression and interaction of 14-3-3 protein and plasma membrane H+-ATPase is related to Al-induced citrate secretion in an Al-resistant black soybean. Plant Mol. Biol. Rep. 31, 1012–1024. doi: 10.1007/s11105-013-0569-0
Harper, J. F., Manney, L., and Sussman, M. R. (1994). The plasma membrane H(+)-ATPase gene family in Arabidopsis: genomic sequence of AHA10 which is expressed primarily in developing seeds. Mol. Gen. Genet. 244, 572–587. doi: 10.1007/BF00282747
Haruta, M., Gray, W. M., and Sussman, M. R. (2015). Regulation of the plasma membrane proton pump (H+-ATPase) by phosphorylation. Curr. Opin. Plant Biol. 28, 68–75. doi: 10.1016/j.pbi.2015.09.005
Hentzen, A. E., Smart, L. B., Whimmers, L. E., Fang, H. H., Schroeder, J. I., and Bennett, A. B. (1996). Two plasma membrane H+-ATPase genes expressed in guard cells of Vicia faba are also expressed throughout the plant. Plant Cell Physiol. 37, 650–659. doi: 10.1093/oxfordjournals.pcp.a028994
Hoekenga, O. A., Maron, L. G., Pineros, M. A., Cancado, G. M. A., Shaff, J., Kobayashi, Y., et al. (2006). AtALMT1, which encodes a malate transporter, is identified as one of several genes critical for aluminum tolerance in Arabidopsis. Proc. Natl. Acad. Sci. U.S.A. 103, 9738–9743. doi: 10.1073/pnas.0602868103
Hohm, T., Demarsy, E., Quan, C., Allenbach Petrolati, L., Preuten, T., Vernoux, T., et al. (2014). Plasma membrane H+-ATPase regulation is required for auxin gradient formation preceding phototropic growth. Mol. Syst. Biol. 10, 751. doi: 10.15252/msb.20145247
Huang, C. F., Yamaji, N., Mitani, N., Yano, M., Nagamura, Y., and Ma, J. F. (2009). A bacterial-type ABC transporter is involved in aluminum tolerance in rice. Plant Cell 21, 655–667. doi: 10.1105/tpc.108.064543
Inoue, S., Takahashi, K., Okumura-Noda, H., and Kinoshita, T. (2016). Auxin influx carrier AUX1 confers acid resistance for Arabidopsis root elongation through the regulation of plasma membrane H+-ATPase. Plant Cell Physiol. 57, 2194–2201. doi: 10.1093/pcp/pcw136
Iuchi, S., Koyama, H., Iuchi, A., Kobayashi, Y., Kitabayashi, S., Kobayashi, Y., et al. (2007). Zinc finger protein STOP1 is critical for proton tolerance in Arabidopsis and coregulates a key gene in aluminum tolerance. Proc. Natl. Acad. Sci. U.S.A. 104, 9900–9905. doi: 10.1073/pnas.0700117104
Janicka-Russak, M., and Kabala, K. (2015). “The role of plasma membrane H+-ATPase in salinity stress of plants,” in Progress in Botany 76, eds U. Lüttge and W. Beyschlag (Cham: Springer International Publishing), 77–92.
Jemo, M., Abaidoo, C., Nolte, C., and Horst, W. J. (2007). Aluminum resistance of cowpea as affected by phosphorus-deficiency stress. J. Plant Physiol. 164, 442–451. doi: 10.1016/j.jplph.2005.12.010
Jin, Y., Nair, A., and van Veen, H. W. (2014). Multidrug transport protein NorM from Vibrio cholerae simultaneously couples to sodium- and proton-motive force. J. Biol. Chem. 289, 14624–14632. doi: 10.1074/jbc.M113.546770
Kim, Y. S., Park, W., Nian, H., Sasaki, T., Ezaki, B., Jang, Y. S., et al. (2010). Aluminum tolerance associated with enhancement of plasma membrane H+-ATPase in the root apex of soybean. Soil Sci. Plant Nutr. 56, 140–149. doi: 10.1111/j.1747-0765.2009.00437.x
Kochian, L. V., Pineros, M. A., Liu, J. P., and Magalhaes, J. V. (2015). Plant adaptation to acid soils: the molecular basis for crop aluminum resistance. Annu. Rev. Plant Biol. 66, 571–598. doi: 10.1146/annurev-arplant-043014-114822
Larsen, P. B., Cancel, J., Rounds, M., and Ochoa, V. (2007). Arabidopsis ALS1 encodes a root tip and stele localized half type ABC transporter required for root growth in an aluminum toxic environment. Planta 225, 1447–1458. doi: 10.1007/s00425-006-0452-4
Larsen, P. B., Geisler, M. J. B., Jones, C. A., Williams, K. M., and Cancel, J. D. (2005). ALS3 encodes a phloem-localized ABC transporter-like protein that is required for aluminum tolerance in Arabidopsis. Plant J. 41, 353–363. doi: 10.1111/j.1365-313X.2004.02306.x
Li, X. W., Li, Y. L., Qu, M., Xiao, H. D., Feng, Y. M., Liu, J. Y., et al. (2016). Cell wall pectin and its methyl-esterification in transition zone determine Al resistance in cultivars of pea (Pisum sativum). Front. Plant Sci. 7:39. doi: 10.3389/fpls.2016.00039
Ligaba, A., Yamaguchi, M., Shen, H., Sasaki, T., Yamamoto, Y., and Matsumoto, H. (2004). Phosphorus deficiency enhances plasma membrane H+-ATPase activity and citrate exudation in greater purple lupin (Lupinus pilosus). Funct. Plant Biol. 31, 1075–1083. doi: 10.1071/FP04091
Liu, J. P., Pineros, M. A., and Kochian, L. V. (2014). The role of aluminum sensing and signaling in plant aluminum resistance. J. Integr. Plant Biol. 56, 221–230. doi: 10.1111/jipb.12162
Ma, J. F. (2000). Role of organic acids in detoxification of aluminum in higher plants. Plant Cell Physiol. 41, 383–390. doi: 10.1093/pcp/41.4.383
Ma, J. F., Hiradate, S., and Matsumoto, H. (1998). High aluminum resistance in buckwheat. II. Oxalic acid detoxifies aluminum internally. Plant Physiol. 117, 753–759. doi: 10.1104/pp.117.3.753
Ma, J. F., Zheng, S. J., Matsumoto, H., and Syuntaro, H. (1997). Detoxifying aluminium with buckwheat. Nature 390, 569–570. doi: 10.1038/37518
Magalhaes, J. V., Liu, J., Guimaraes, C. T., Lana, U. G., Alves, V. M., Wang, Y. H., et al. (2007). A gene in the multidrug and toxic compound extrusion (MATE) family confers aluminum tolerance in sorghum. Nature Genet. 39, 1156–1161. doi: 10.1038/ng2074
Mariano, E. D., and Keltjens, W. G. (2005). Long-term effects of aluminum exposure on nutrient uptake by maize genotypes differing in aluminum resistance. J. Plant Nutr. 28, 323–333. doi: 10.1081/PLN-200047625
Maron, L. G., Pineros, M. A., Guimaraes, C. T., Magalhaes, J. V., Pleiman, J. K., Mao, C., et al. (2010). Two functionally distinct members of the MATE (multi-drug and toxic compound extrusion) family of transporters potentially underlie two major aluminum tolerance QTLs in maize. Plant J. 61, 728–740. doi: 10.1111/j.1365-313X.2009.04103.x
Matsumoto, H. (1988). Inhibition of proton transport activity of microsomal membrane vesicles of barley roots by aluminium. Soil Sci. Plant Nutr. 34, 499–506. doi: 10.1080/00380768.1988.10416466
Morita, A., Yanagisawa, O., Takatsu, S., Maeda, S., and Hiradate, S. (2008). Mechanism for the detoxification of aluminum in roots of tea plant (Camellia sinensis (L.) Kuntze). Phytochemistry 69, 147–153. doi: 10.1016/j.phytochem.2007.06.007
Neuhaus, C., Geilfus, C. M., Zörb, C., and Mühling, K. H. (2013). Transcript expression of Mg-chelatase and H+-ATPase isogenes in Vicia faba leaves as influenced by root and foliar magnesium supply. Plant Soil 368, 41–50. doi: 10.1007/s11104-013-1711-3
Nies, A. T., Damme, K., Kruck, S., Schaeffeler, E., and Schwab, M. (2016). Structure and function of multidrug and toxin extrusion proteins (MATEs) and their relevance to drug therapy and personalized medicine. Arch. Toxicol. 90, k1555–1584. doi: 10.1007/s00204-016-1728-5
Nishima, W., Mizukami, W., Tanaka, Y., Ishitani, R., Nureki, O., and Sugita, Y. (2016). Mechanisms for two-step proton transfer reactions in the outward-facing form of MATE transporter. Biophys. J. 110, 1346–1354. doi: 10.1016/j.bpj.2016.01.027
Ohno, T., Koyama, H., and Hara, T. (2003). Characterization of citrate transport through the plasma membrane in a carrot mutant cell line with enhanced citrate excretion. Plant Cell Physiol. 44, 156–162. doi: 10.1093/pcp/pcg025
Oufattole, M., Arango, M., and Boutry, M. (2000). Identification and expression of three new Nicotiana plumbaginifolia genes which encode isoforms of a plasma-membrane H+-ATPase, and one of which is induced by mechanical stress. Planta 210, 715–722. doi: 10.1007/s004250050672
Palmgren, M. G. (2001). Plant plasma membrane H+-ATPases: powerhouses for nutrient uptake. Annu. Rev. Plant Physiol. Plant Mol. Biol. 52, 817–845. doi: 10.1146/annurev.arplant.52.1.817
Qing, W., Mi, Y. H., Li, X. H., and Xu, R. K. (2017). Aluminium-enhanced proton release associated with plasma membrane H+-ATPase activity and excess cation uptake in Camellia sinensis roots. Pedosphere (in press). doi: 10.1016/S1002-0160(17)60460-0
Rengel, Z., Bose, J., Chen, Q., and Tripathi, B. N. (2015). Magnesium alleviates plant toxicity of aluminium and heavy metals. Crop Pasture Sci. 66, 1298–1307. doi: 10.1016/j.nut.2016.10.012
Rober-Kleber, N., Albrechtova, J. T., Fleig, S., Huck, N., Michalke, W., Wagner, E., et al. (2003). Plasma membrane H+-ATPase is involved in auxin-mediated cell elongation during wheat embryo development. Plant Physiol. 131, 1302–1312. doi: 10.1104/pp.013466
Ruiz-Herrera, L. F., and Lopez-Bucio, J. (2013). Aluminum induces low phosphate adaptive responses and modulates primary and lateral root growth by differentially affecting auxin signaling in Arabidopsis seedlings. Plant Soil 371, 593–609. doi: 10.1007/s11104-013-1722-0
Ryan, P. R., Reid, R. J., and Smith, F. A. (1997). Direct evaluation of the Ca2+-displacement hypothesis for Al toxicity. Plant Physiol. 113, 1351–1357. doi: 10.1104/pp.113.4.1351
Santi, S., Cesco, S., Varanini, Z., and Pinton, R. (2005). Two plasma membrane H+-ATPase genes are differentially expressed in iron-deficient cucumber plants. Plant Physiol. Biochem. 43, 287–292. doi: 10.1016/j.plaphy.2005.02.007
Sasaki, T., Yamamoto, Y., Ezaki, B., Katsuhara, M., Ahn, S. J., Ryan, P. R., et al. (2004). A wheat gene encoding an aluminum-activated malate transporter. Plant J. 37, 645–653. doi: 10.1111/j.1365-313X.2003.01991.x
Sawaki, Y., Iuchi, S., Kobayashi, Y., Kobayashi, Y., Ikka, T., Sakurai, N., et al. (2009). STOP1 regulates multiple genes that protect arabidopsis from proton and aluminum toxicities. Plant Physiol. 150, 281–294. doi: 10.1104/pp.108.134700
Schmohl, N., Pilling, J., Fisahn, J., and Horst, W. J. (2000). Pectin methylesterase modulates aluminium sensitivity in Zea mays and Solanum tuberosum. Physiol. Plant. 109, 419–427. doi: 10.1034/j.1399-3054.2000.100408.x
Shen, H., Chen, J., Wang, Z., Yang, C., Sasaki, T., Yamamoto, Y., et al. (2006). Root plasma membrane H+-ATPase is involved in the adaptation of soybean to phosphorus starvation. J. Exp. Bot. 57, 1353–1362. doi: 10.1093/jxb/erj111
Shen, H., He, L. F., Sasaki, T., Yamamoto, Y., Zheng, S. J., Ligaba, A., et al. (2005). Citrate secretion coupled with the modulation of soybean root tip under aluminum stress. Up-regulation of transcription, translation, and threonine-oriented phosphorylation of plasma membrane H+-ATPase. Plant Physiol. 188, 287–296. doi: 10.1104/pp.104.058065
Silva, I. R., Smyth, T. J., Israel, D. W., Raper, C. D., and Rufty, T. W. (2001). Magnesium is more efficient than calcium in alleviating aluminum rhizotoxicity in soybean and its ameliorative effect is not explained by the Gouy-Chapman-Stern model. Plant Cell Physiol. 42, 538–545. doi: 10.1093/pcp/pce066
Silva, S., Pinto-Carnide, O., Martins-Lopes, P., Matos, M., Guedes-Pinto, H., and Santos, C. (2010). Differential aluminium changes on nutrient accumulation and root differentiation in an Al sensitive vs. tolerant wheat. Environ. Exp. Bot. 68, 91–98. doi: 10.1016/j.envexpbot.2009.10.005
Sivaguru, M., and Paliwal, K. (1993). Differential aluminum tolerance in some tropical rice cultivars. 2. Mechanism of aluminum tolerance. J. Plant Nutr. 16, 1717–1732. doi: 10.1080/01904169309364645
Sivaguru, M., Yamamoto, Y., Rengel, Z., Ahn, S., and Matsumoto, H. (2005). Early events responsible for aluminum toxicity symptoms in suspension-cultured tobacco cells. New Phytol. 165, 99–109. doi: 10.1111/j.1469-8137.2004.01219.x
Sun, P., Tian, Q. Y., Chen, J., and Zhang, W. H. (2010). Aluminium-induced inhibition of root elongation in Arabidopsis is mediated by ethylene and auxin. J. Exp. Bot. 61, 347–356. doi: 10.1093/jxb/erp306
Takahashi, K., Hayashi, K., and Kinoshita, T. (2012). Auxin activates the plasma membrane H+-ATPase by phosphorylation during hypocotyl elongation in Arabidopsis. Plant Physiol. 159, 632–641. doi: 10.1104/pp.112.196428
Tanaka, Y., Hipolito, C. J., Maturana, A. D., Ito, K., Kuroda, T., Higuchi, T., et al. (2013). Structural basis for the drug extrusion mechanism by a MATE multidrug transporter. Nature 496, 247–251. doi: 10.1038/nature12014
Titapiwatanakun, B., and Murphy, A. S. (2009). Post-transcriptional regulation of auxin transport proteins: cellular trafficking, protein phosphorylation, protein maturation, ubiquitination, and membrane composition. J. Exp. Bot. 60, k1093–1107. doi: 10.1093/jxb/ern240
Vitart, V., Baxter, I., Doerner, P., and Harper, J. F. (2001). Evidence for a role in growth and salt resistance of a plasma membrane H+-ATPase in the root endodermis. Plant J. 27, 191–201. doi: 10.1046/j.1365-313x.2001.01081.x
Wang, J., Hou, Q., Li, P., Yang, L., Sun, X., Benedito, V. A., et al. (2017). Diverse functions of multidrug and toxin extrusion (MATE) transporters in citric acid efflux and metal homeostasis in Medicago truncatula. Plant J. 90, 79–95. doi: 10.1111/tpj.13471
Wang, M. J., Wang, Y., Sun, J., Ding, M. Q., Deng, S. R., Hou, P. C., et al. (2013). Overexpression of PeHA1 enhances hydrogen peroxide signaling in salt-stressed Arabidopsis. Plant Physiol. Biochem. 71, 37–48. doi: 10.1016/j.plaphy.2013.06.020
Wang, P. (2014). Physiological and Molecular Mechanisms of Exogenous Application of IAA Increasing Aluminum Resistance in Black Soybean (Glycine max). Master’s thesis, Kunming University of Science and Technology, Kunming.
Wang, P., Yu, W., Zhang, J., Rengel, Z., Xu, J., Han, Q., et al. (2016). Auxin enhances aluminum-induced citrate exudation through upregulation of GmMATE and activation of the plasma membrane H+-ATPase in soybean roots. Ann. Bot. 118, 933–940. doi: 10.1093/aob/mcw133
Wang, Y., Li, R., Li, D., Jia, X., Zhou, D., Li, J., et al. (2017). NIP1; 2 is a plasma membrane-localized transporter mediating aluminum uptake, translocation, and tolerance in Arabidopsis. Proc. Natl. Acad. Sci. U.S.A. 114, 5047–5052. doi: 10.1073/pnas.1618557114
Watanabe, T., and Okada, K. (2005). Interactive effects of Al, Ca and other cations on root elongation of rice cultivars under low pH. Ann. Bot. 95, 379–385. doi: 10.1093/aob/mci032
Watanabe, T., Osaki, M., Yoshihara, T., and Tadano, T. (1998). Distribution and chemical speciation of aluminum in the Al accumulator plant, Melastoma malabathricum L. Plant Soil 201, 165–173. doi: 10.1023/A:1004341415878
Whitten, M. G., Wong, M. T. F., and Rate, A. W. (2000). Amelioration of subsurface acidity in the south-west of Western Australia: downward movement and mass balance of surface-incorporated lime after 2-15 years. Aust. J. Soil Res. 38, 711–728. doi: 10.1071/SR99054
Wu, X., Li, R., Shi, J., Wang, J., Sun, Q., Zhang, H., et al. (2014). Brassica oleracea MATE encodes a citrate transporter and enhances aluminum tolerance in Arabidopsis thaliana. Plant Cell Physiol. 55, 1426–1436. doi: 10.1093/pcp/pcu067
Wu, Y., Shen, H., and Chen, J. H. (2008). Aluminum-resistance of AHA1 transgenic Arabidopsis thaliana: physiological analysis. J. Appl. Ecol. 19, 1125–1130.
Xu, W., Jia, L., Baluška, F., Ding, G., Shi, W., Ye, N., et al. (2012). PIN2 is required for the adaptation of Arabidopsis roots to alkaline stress by modulating proton secretion. J. Exp. Bot. 63, 6105–6114. doi: 10.1093/jxb/ers259
Yamaji, N., Huang, C. F., Nagao, S., Yano, M., Sato, Y., Nagamura, Y., et al. (2009). A zinc finger transcription factor ART1 regulates multiple genes implicated in aluminum tolerance in rice. Plant Cell 21, 3339–3349. doi: 10.1105/tpc.109.070771
Yan, F., Zhu, Y., Müller, C., Zörb, C., and Schubert, S. (2002). Adaptation of H+-pumping and plasma membrane H+-ATPase activity in proteoid roots of white lupin under phosphate deficiency. Plant Physiol. 129, 50–68. doi: 10.1104/pp.010869
Yang, J., Qu, M., Fang, J., Shen, R. F., Fong, Y. M., Liu, J. Y., et al. (2016). Alkali-soluble pectin is the primary target of aluminum immobilization in root border cells of pea (Pisum sativum). Front. Plant Sci. 7:1297. doi: 10.3389/fpls.2016.01297
Yang, J. L., Li, Y. Y., Zhang, Y. J., Zhang, S. S., Wu, Y. R., Wu, P., et al. (2008). Cell wall polysaccharides are specifically involved in the exclusion of aluminum from the rice root apex. Plant Physiol. 146, 602–611. doi: 10.1104/pp.107.111989
Yang, J. L., You, J. F., Li, Y. Y., Wu, P., and Zheng, S. J. (2007). Magnesium enhances aluminum-induced citrate secretion in rice bean roots (Vigna umbellata) by restoring plasma membrane H+-ATPase activity. Plant Cell Physiol. 48, 66–73. doi: 10.1093/pcp/pcl038
Yang, J. L., Zhu, X. F., Peng, Y. X., Zheng, C., Li, G. X., Liu, Y., et al. (2011a). Cell wall hemicellulose contributes significantly to aluminum adsorption and root growth in Arabidopsis. Plant Physiol. 155, 1885–1892. doi: 10.1104/pp.111.172221
Yang, J. L., Zhu, X. F., Peng, Y. X., Zheng, C., Ming, F., and Zheng, S. J. (2011b). Aluminum regulates oxalate secretion and plasma membrane H+-ATPase activity independently in tomato roots. Planta 234, 281–291. doi: 10.1007/s00425-011-1402-3
Yang, X. Y., Yang, J. L., Zhou, Y., Pineros, M. A., Kochian, L. V., Li, G. X., et al. (2011c). A de novo synthesis citrate transporter, Vigna umbellata multidrug and toxic compound extrusion, implicates in Al-activated citrate efflux in rice bean (Vigna umbellata) root apex. Plant Cell Environ. 34, 2138–2148. doi: 10.1111/j.1365-3040.2011.02410.x
Yang, Y., Wang, Q. L., Geng, M. J., Guo, Z. H., and Zhao, Z. N. (2011d). Effect of indole-3-acetic acid on aluminum-induced efflux of malic acid from wheat (Triticum aestivum L). Plant Soil 346, 215–230. doi: 10.1007/s11104-011-0811-1
Yang, Y. D., Hammes, U. Z., Taylor, C. G., Schachtman, D. P., and Nielsen, E. (2006). High-affinity auxin transport by the AUX1 influx carrier protein. Curr. Biol. 16, 1123–1127. doi: 10.1016/j.cub.2006.04.029
Yang, Z. B., Geng, X., He, C., Zhang, F., Wang, R., Horst, W. J., et al. (2014). TAA1-regulated local auxin biosynthesis in the root-apex transition zone mediates the aluminum-induced inhibition of root growth in Arabidopsis. Plant Cell 26, 2889–2904. doi: 10.1105/tpc.114.127993
Yokosho, K., Yamaji, N., Ueno, D., Mitani, N., and Ma, J. F. (2009). OsFRDL1 is a citrate transporter required for efficient translocation of iron in rice. Plant Physiol. 149, 297–305. doi: 10.1104/pp.108.128132
Yu, W. (2017). The Regulatory Mechanisms of AtPIN2 and AtAUX1 in Arabidopsis thaliana Response to Aluminum Stress. Master’s thesis, Kunming University of Science and Technology, Kunming.
Keywords: Al toxicity, H+-ATPase, Mg, IAA, transgenic, citrate exudation
Citation: Zhang J, Wei J, Li D, Kong X, Rengel Z, Chen L, Yang Y, Cui X and Chen Q (2017) The Role of the Plasma Membrane H+-ATPase in Plant Responses to Aluminum Toxicity. Front. Plant Sci. 8:1757. doi: 10.3389/fpls.2017.01757
Received: 19 June 2017; Accepted: 25 September 2017;
Published: 17 October 2017.
Edited by:
Sergey Shabala, University of Tasmania, AustraliaCopyright © 2017 Zhang, Wei, Li, Kong, Rengel, Chen, Yang, Cui and Chen. This is an open-access article distributed under the terms of the Creative Commons Attribution License (CC BY). The use, distribution or reproduction in other forums is permitted, provided the original author(s) or licensor are credited and that the original publication in this journal is cited, in accordance with accepted academic practice. No use, distribution or reproduction is permitted which does not comply with these terms.
*Correspondence: Qi Chen, chenq0321@163.com
†These authors have contributed equally to this work.