- 1School of Environmental and Life Sciences, The University of Newcastle, Callaghan, NSW, Australia
- 2College of Agronomy and Biotechnology, China Agricultural University, Beijing, China
- 3Beijing Key Laboratory of Growth and Developmental Regulation for Protected Vegetable Crops, College of Horticulture, China Agricultural University, Beijing, China
- 4Institute of Tropical Agriculture and Forestry, Hainan University, Haikou, China
- 5CSIRO Agriculture and Food, Canberra, ACT, Australia
- 6Leibniz Institute of Plant Genetics and Crop Plant Research, Gatersleben, Germany
Flowering plants depend on pollination and fertilization to activate the transition from ovule to seed and ovary to fruit, namely seed and fruit set, which are key for completing the plant life cycle and realizing crop yield potential. These processes are highly energy consuming and rely on the efficient use of sucrose as the major nutrient and energy source. However, it remains elusive as how sucrose imported into and utilizated within the female reproductive organ is regulated in response to pollination and fertilization. Here, we explored this issue in tomato by focusing on genes encoding cell wall invertase (CWIN) and sugar transporters, which are major players in sucrose phloem unloading, and sink development. The transcript level of a major CWIN gene, LIN5, and CWIN activity were significantly increased in style at 4 h after pollination (HAP) in comparison with that in the non-pollination control, and this was sustained at 2 days after pollination (DAP). In the ovaries, however, CWIN activity and LIN5 expression did not increase until 2 DAP when fertilization occurred. Interestingly, a CWIN inhibitor gene INVINH1 was repressed in the pollinated style at 2 DAP. In response to pollination, the style exhibited increased expressions of genes encoding hexose transporters, SlHT1, 2, SlSWEET5b, and sucrose transporters SlSUT1, 2, and 4 from 4 HAP to 2 DAP. Upon fertilization, SlSUT1 and SlHT1 and 2, but not SlSWEETs, were also stimulated in fruitlets at 2 DAP. Together, the findings reveal that styles respond promptly and more broadly to pollination for activation of CWIN and sugar transporters to fuel pollen tube elongation, whereas the ovaries do not exhibit activation for some of these genes until fertilization occurs.
HIGHLIGHTS:
Expression of genes encoding cell wall invertases and sugar transporters was stimulated in pollinated style and fertilized ovaries in tomato.
Introduction
In angiosperms, flowering signifies the onset of producing the next generation in the form of seeds embedded within fruit tissues. The stamen, the male organ of the flower, produces pollen grain that contains the male gamete-sperms. The pistil, the female organ of the flower, consists of stigma, style and ovary, which contains ovules carrying the female gamete-egg cells. At flowering stage, pollen grains from the stamen are transferred to the stigma aided by wind and animals (Regal, 1982) or insects such as bees, butterflies and moths (Regal, 1982; Free, 1993; Kremen et al., 2002; Lord and Russell, 2002), a process termed pollination. After landing on the stigma surface, pollen grains rehydrate to germinate as pollen tubes that carry the sperm cells to penetrate through the transmitting tissue of the style to the ovary. Upon reaching the ovules within the ovaries, the pollen tubes release the two sperm cells to complete the fertilization process (Heslop-Harrison, 1987; Mascarenhas, 1993; Franklin-Tong, 1999; Lord and Russell, 2002). The fertilized ovule and ovary then develop into seed and fruit, respectively. Thus, pollination and fertilization are the prerequisites for producing seeds. However, these processes are highly vulnerable to drought, heat and other abiotic stresses, which often leads to seed or fruit abortion, causing major crop yield loss (Boyer and Mclaughlin, 2007; Liu et al., 2016). Therefore, understanding the molecular players or pathways regulated by pollination and fertilization may help us identify valuable targets to improve reproductive success under stress.
After pollination, pollen grains germinate and elongate following a tip growth model at a rate up to 1 cm per hour, rendering it among the fastest growing cells (Lord and Russell, 2002). It takes hours to days for the pollen tube to reach the ovules for fertilization to occur (Taylor and Hepler, 1997). Structurally, the pollen tube is symplasmically isolated from the surrounding style tissue (Scott et al., 1991). Similarly, the filial tissues of angiosperm seeds, namely the embryo and endosperm, are isolated from the maternal tissue by an apoplasmic compartment (Stadler et al., 2005; Braun et al., 2014). Thus, all resources including sucrose, the predominant assimilate as carbon nutrient and energy source, must enter the elongating pollen tube and filial tissues apoplasmically (Reinders, 2016; Rottmann et al., 2016; Goetz et al., 2017).
In the apoplasmic unloading pathway, sucrose is unloaded into the cell wall matrix from the sieve element/companion cell (SE/CC) complex mediated by SWEET (sugars will eventually be exported transporter) proteins, which are typically localized on the plasma membrane to transport sucrose or hexoses down a concentration gradient in an energy independent manner (Chen et al., 2010; Eom et al., 2015). Within the cell wall, sucrose is often hydrolyzed by cell wall invertase (CWIN, with a pH optimum of 5–6) into glucose and fructose (Ruan et al., 2010). The hexoses are then taken up by H+-coupled hexose transporters (HXTs) (Ruan et al., 1995; Ruan et al., 2012; Ruan, 2014). It is common that CWIN and HXTs are co-expressed in the apoplasmic unloading region, indicating a synergistic functional relationship between CWIN and HXTs (Weber et al., 2005; Jin et al., 2009; McCurdy et al., 2010). It is less clear if the SWEETs for hexoses share a similar relationship with CWIN. Compelling evidence has demonstrated the importance of CWIN and sugar transporters in plant development. For instance, suppression of tomato hexose transporters, SlHT1, 2 or 3, causes a 55% decrease in fruit hexose accumulation (McCurdy et al., 2010). A deficit in SWEET transporters led to defective seed and impaired pollen grains in Arabidopsis (Guan et al., 2008; Chen et al., 2015) and rice (Sosso et al., 2015; Yang et al., 2018). Similarly, decreased CWIN activity is associate with grain growth repression and abortion in maize (Mclaughlin and Boyer, 2004; Shen et al., 2018), rice (Hirose et al., 2002; Cho et al., 2005; Wang et al., 2008), and wheat (Dorion et al., 1996). Conversely, increasing CWIN activity by suppressing its inhibitor gene improved tomato fruit and seed set under normal and heat stress conditions (Jin et al., 2009; Liu et al., 2016). These findings demonstrated the important roles of CWIN and sugar transporters in fruit and seed development.
Despite the progress outlined above, it remains largely unknown as to how pollination and fertilization may alter the expression of CWIN and sugar transporters to power pollen tube elongation and seed and fruit set. Given assimilate is unloaded apoplasmically to the elongating pollen tubes and developing seeds, we hypothesize CWIN- and transporter-related sugar import and utilization may be enhanced during pollination and fertilization to support the transition from ovule to seed and ovary to fruit. To this end, the expression of CWIN gene LIN5 was changed from a dispersed- to a phloem-specific pattern with CWIN activity dramatically increased in tomato ovaries from 2 days before anthesis to 2 days after anthesis (Palmer et al., 2015). These changes were proposed to be essential for the reproductive system to channel carbon nutrients more efficiently to the fertilized ovaries, named as a “Ready-Set-Grow” model (Palmer et al., 2015; Ru et al., 2017). It remains unknown, however, whether these changes were induced by the occurrences of pollination or fertilization. Historically, these two processes were suggested to affect several biological processes. For instance, the occurrence of pollination could induce petal and style withering (Van Doorn, 1997) or inhibit the elongation of maize silks as we observed in the field (Shen et al., 2018), while fertilization of the egg cell triggers endosperm proliferation in angiosperm embryogenesis (Nowack et al., 2006). Several recent studies have indicated the involvement of hexose transporters and CWIN in carbohydrate supply to the growing pollen tubes in Arabidopsis and tobacco (Reinders, 2016; Rottmann et al., 2016; Goetz et al., 2017). However, these studies did not differentiate the regulatory effect of pollination from fertilization on sugar transport and metabolism in the styles and fruitlets.
In this study, we aimed to dissect the potential effect of pollination and fertilization on the expression of genes encoding CWIN and sugar transporters in reproductive organs by using tomato (Solanum lycopersicum) as the experimental model. The choice of tomato was based on the following considerations: First, tomato is a major horticultural crop worldwide and a model system for studying fruit development. Thus, findings from tomato may be applicable to other fruit crops. Second, the tomato genome has been fully sequenced (Tomato Genome Consortium, 2012), with ample prior knowledge on genes controlling sugar transport, and metabolism (Godt and Roitsch, 1997; Fridman and Zamir, 2003; Proels et al., 2006). Third, it has been well established that, in tomato ovary, sucrose is unloaded apoplasmically (Jin et al., 2009; Palmer et al., 2015) where CWIN and sugar transporters play irreplaceable roles (Jin et al., 2009). We conducted emasculation and manual pollination treatments followed by sampling at specific time points to separately assess the effect of pollination and fertilization on the expression of genes for CWIN and sugar transporters.
Materials and Methods
Plant Growth Conditions
Tomato (S. lycopersicum) seeds were geminated on water-soaked filter paper at 25°C for 3 days. The seedlings were transferred to pots and grown in a greenhouse under a controlled day/night temperature regime of 25°C (6 am to 8 pm)/20°C with natural light illumination. Water and fertilizers were applied regularly as previously described (Liu et al., 2016; Ru et al., 2017).
Emasculation, Manual Pollination, and Sampling
For emasculation treatment, the anthers of tagged flowers were removed at 2 days before anthesis (DBA). Care was taken to avoid damage to other parts of the flowers. The emasculated flowers were bagged to prevent natural pollination. On the day of anthesis (∼2 days after emasculation), manual pollination was conducted by brushing pollen grains from newly opened flowers to the styles of emasculated flowers. The manually pollinated flowers were then re-bagged. The styles and ovaries were manually dissected at 4 h and 2 days after pollination (HAP and DAP, respectively) and dropped into liquid nitrogen and storage at -80°C for further laboratorial measurements.
Selection of Candidate CWIN, HXTs, SUTs, and SWEET Genes
There were 4 genes encoding CWIN in tomato (Godt and Roitsch, 1997). Among them, LIN5 was the predominant member expressed in developing fruit and seed, and LIN7 was restricted to the tapetum and pollen (Godt and Roitsch, 1997; Proels et al., 2006; Jin et al., 2009), whereas LIN6 was expressed in all organs except the ovary and pollen, and LIN8 was preferentially expressed in root (Fridman and Zamir, 2003). Since we focus on the style and ovary during pollination and fertilization processes, we selected LIN5 and 7 as the candidate genes for CWIN based on their tissue-specific expression pattern.
There were 3 hexose transporter genes (SlHT1, 2 and 3) identified from the tomato genome (Gear et al., 2000). SlHT1 and 3 were dominantly expressed in green fruit, SlHT2 was expressed at relatively high levels in source leaves and certain sink tissues (Gear et al., 2000; Dibley et al., 2005). Functionally, SlHT1 and 2 are energy-dependent glucose transporters based on sugar uptake assay in yeast. SlHT3 showed no sugar transport activity when expressed in yeast although its ortholog gene AtSTP3 was demonstrated to be a low affinity transporter of glucose and probably other hexoses (Büttner et al., 2000; Reuscher et al., 2014). Based on the tissue-specific analysis of the three SlHTs in floral tissues (Supplementary Figure S1), we selected SlHT1, 2, and 3 to examine their response to pollination and fertilization in this study.
Members of sucrose transporter (SUT) families were well established as sucrose/H+ symporters (Kühn and Grof, 2010). The tomato SUT family consists of SlSUT1, SlSUT2, and SlSUT4 (Reuscher et al., 2014); among them, SlSUT1 and 4 were expressed ubiquitously and thought to be the main importers of apoplasmic sucrose into phloem (Weise et al., 2000; Sauer, 2007). SlSUT2 was reported to be involved in pollen tube growth (Hackel et al., 2006; Reuscher et al., 2014). All three SlSUTs were investigated in this study.
A total of 29 putative SlSWEET transporter genes were identified from the tomato GDB database1 and the Solanaceae Genomics Network database2. Genes were assigned numbers SlSWEET1-17 based on their positions on tomato chromosomes 1–12 (Feng et al., 2015). We further selected the SlSWEET genes that are highly expressed in flower buds and fully opened flowers based on the RNA-seq data, available in the Tomato Functional Genomic Database3 and analyzed by Feng et al. (2015). We finally selected 5 candidate SlSWEET genes that are preferentially expressed in flower organs, which were SlSWEET5b, 10a, 11a, 12a, and 16. Among them, SlSWEET5b belongs to clade II for transport of hexose, whereas SlSWEET10a, 11a, and 12a belong to clade III for transport of sucrose, and SlSWEET16 belongs to clade IV transporters for hexose (Supplementary Figure S2). Phylogenetic analysis was conducted by comparing SWEET protein sequences in tomato and Arabidopsis by firstly conducting alignment of the SWEET amino acid sequences using ClustalW in Mega Alignment software followed by constructing an unrooted phylogenetic tree using Mega 7.0 with the neighbor-joining (NJ) method (Saitou and Nei, 1987). The settings of Poisson correction, pairwise deletion, and bootstrapping (1000 replicates; random seeds) served as default values. Based on the phylogenetic tree and prior knowledge from the literature, the locations and functions of the 5 candidate SlSWEET transporters were inferred.
RNA Extraction and qRT-PCR
RNA was extracted using the RNeasy Plant Mini Kit (Qiagen). Genomic DNA was removed by using DNase (Promega). The DNase-treated RNA was reverse-transcripted into cDNA using oligo(dT)20 primer and ProtoScript Reverse Transcriptase (New England Biolabs) according to the manufacturer’s instructions. The cDNA was diluted 1:20 for quantitative real-time PCR (qRT-PCR) analyses according to Ru et al. (2017). Relative gene expressions of target genes were calculated by comparing with the reference genes of SlTIP4 and SlExpress (Ru et al., 2017). Primers used for qRT-PCR are list in Supplementary Table S1.
Invertase Enzyme Activities Assay
Cell wall bound and soluble proteins were extracted and assayed according to Tomlinson et al. (2004) and Palmer et al. (2015).
Staining and Visualization of Pollen Tube Within the Pistils
Pollen tubes were visualized by staining with aniline blue, which gives a strong fluorescence under UV excitation after binding with callose in pollen and pollen tube (Martin, 1959). Following manual pollination on the day of anthesis, the pollinated pistils were sampled 24 and 48 HAP and fixed immediately in FAA solution (40% formaldehyde solution: 80% ethanol: acetic acid glacial = 1:8:1, v/v/v). The fixed pistils were then rinsed with distilled water for several times and transferred into 8 M NaOH solution for 24 h to soften the tissue (Martin, 1959). After neutralizing in acetic acid glacial for 30 s, the pistils were immersed in distilled water for 1 h to remove excess acetic acid. The pistils were stained with 0.1% aniline blue in 0.1 M K2HPO4-KOH (pH 11) for 4 h and then put on a glass slide. The pistils were evenly squashed by tapping the cover slip slightly with tweezers and then observed with a fluorescence microscope under UV.
Statistically Analysis and Illustration Drawing
All measurements described comprised at least four biological replicates. Each biological replication contained 2 ovaries or 4 styles. Statistical analyses were conducted by using Student’s t-test in the EXCEL software (Microsoft). Illustrations were drawn by Microsoft PowerPoint 2016 and Adobe Photoshop CS6.
Results
Validation of Emasculation and Pollination Treatments
Under natural condition, the styles elongated from 5 days before anthesis (DBA) and reached its maximum at 2 DBA (Figure 1A), implying that the pistil was developmentally ready to receive pollen grains at 2 DBA. Following anthesis, the petals changed their color from yellow to pink and purple and then became welted, whereas the ovaries did not enlarge and the style did not show any sign of senescence until the 4th day after anthesis (DAA, Figure 1A).
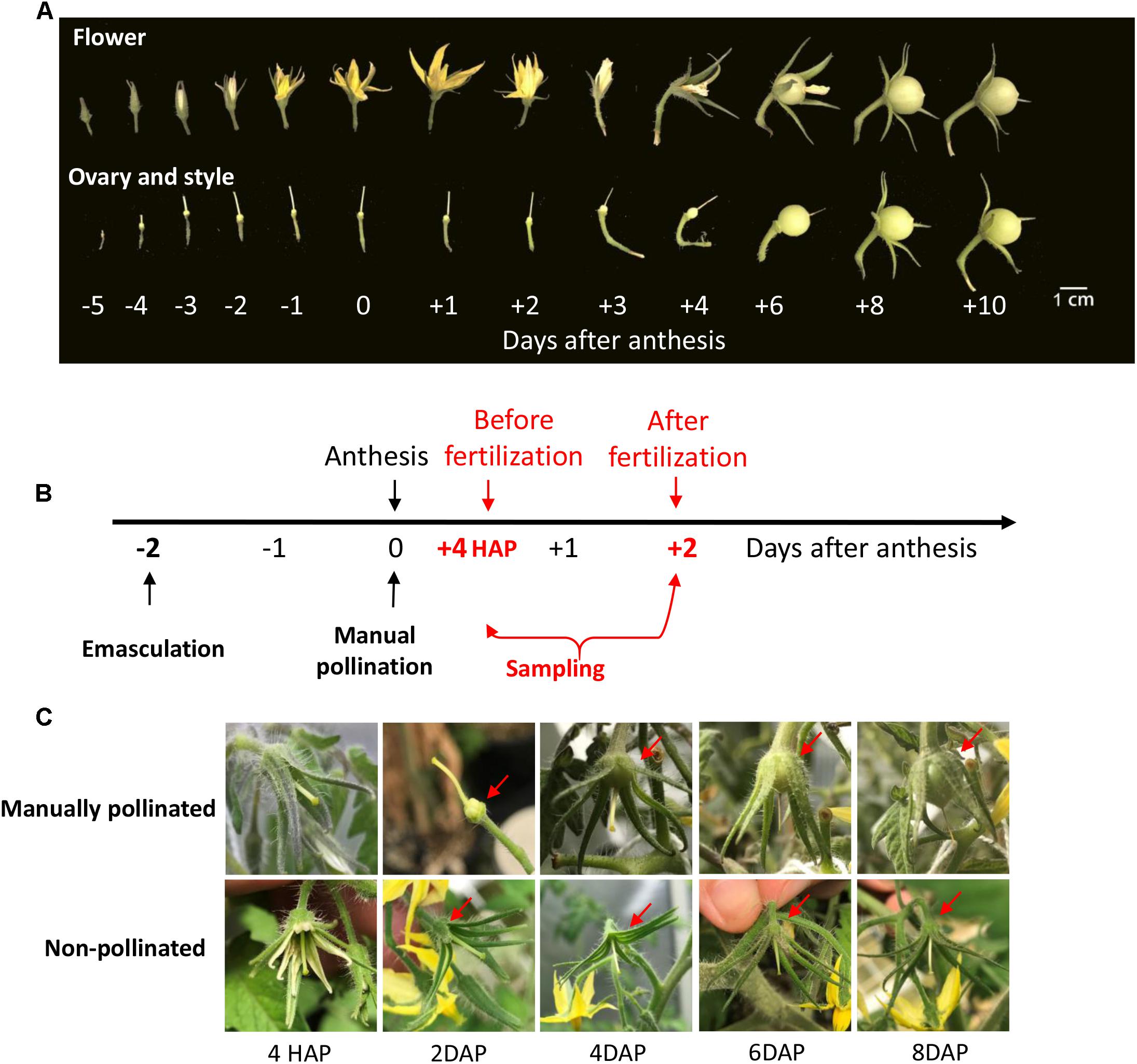
Figure 1. The phenotype of style and ovary during flowering stage in tomato and the illustration of time of manual pollination and sampling. (A) The phenotypes of tomato flower, ovary, and style from flower bud to fruitlets. The elongation of the style stopped at ∼2 days before anthesis. Fruitlet enlargement was not apparent until 3 DAP. (B) A schematic diagram about the timing of emasculation, pollination, and sampling. The ovary and style were harvested at 4 HAP and 2 DAP, representing the time points before and after fertilization, respectively. Emasculated flower with no pollination served as a control. (C) The phenotypes of manually pollinated ovary and style from 4 HAP to 8 DAP. The pollinated ovary increased its size at 2 DAP onward (red arrows). In contrast, the non-pollinated control ovaries were stunted and aborted at 6∼8 DAP. The findings validated the success of emasculation and pollination treatments. HAP, hours after pollination; DAP, days after pollination.
To examine the impact of pollination on CWIN and sugar transporter gene expression, we performed emasculation at 2 DBA and manually pollinated the emasculated stigma on the day of anthesis, i.e., 0 DAA (Figure 1B). In tomato, it takes ∼48 h for the elongating pollen tubes to reach the ovules to complete fertilization (see below). Thus, we sampled styles and ovaries at 4 h after pollination (HAP) and 2 days after pollination (DAP), which represented the stages of before- and after-fertilization, respectively (Figure 1B). The manually pollinated ovaries showed visible enlargement from 4 DAP, the same as that under natural pollination, whereas non-pollinated ovaries did not (Figures 1A,C), indicating the successes of manual pollination, and emasculation, respectively.
The Expression of CWIN Gene LIN5 and CWIN Activity Were Enhanced in the Style and Ovary During Pollination and Fertilization, Respectively
To determine when fertilization likely occurred, we investigated pollen tube elongation in vivo within the style. The analyses revealed that pollen tubes emitting green-blue fluorescence from aniline blue staining of callose entered the ovary and reached ovules at 24 and 48 HAP (Figures 2A,B). Intriguingly, the fluorescence was not only found at the top of the ovary, but also at the bottom (Figures 2A,B). To check if the fluorescence was derived from pollen tubes, two negative controls were included. No fluorescence was found at either the top or bottom of the ovary in negative control I (Figure 2C), in which the ovary was derived from pollinated flower, but not stained with aniline blue, indicating the fluorescence in Figures 2A,B is callose-specific. In negative control II (Figure 2D), the ovary was derived from non-pollinated flower, but stained with aniline blue. Here, some fluorescence was observed at the bottom of the ovary, but with no fluorescence detected in the region where ovules were located (Figure 2D). These results indicate that the fluorescence at the top of the ovaries in Figures 2A,B comes from the callose of pollen tubes, whereas the fluorescence at the bottom of the ovaries originates from pre-existing callose of other ovary tissues, likely the vascular bundle in the placenta region. The key point here is that, compared with the negative controls with no fluorescence in the ovules (Figures 2C,D), pollination treatment showed fluorescence of pollen tubes reached the ovules by 48 HAP (Figure 2B) as illustrated in the schematic diagrams (Figures 2E,F). Thus, it can be concluded that most pollen tubes have entered into ovaries at 24 HAP and reached ovules at 48 HAP (Figures 2A,B), indicating that fertilization did not happen at 24 HAP but took place at 48 HAP under our experimental conditions.
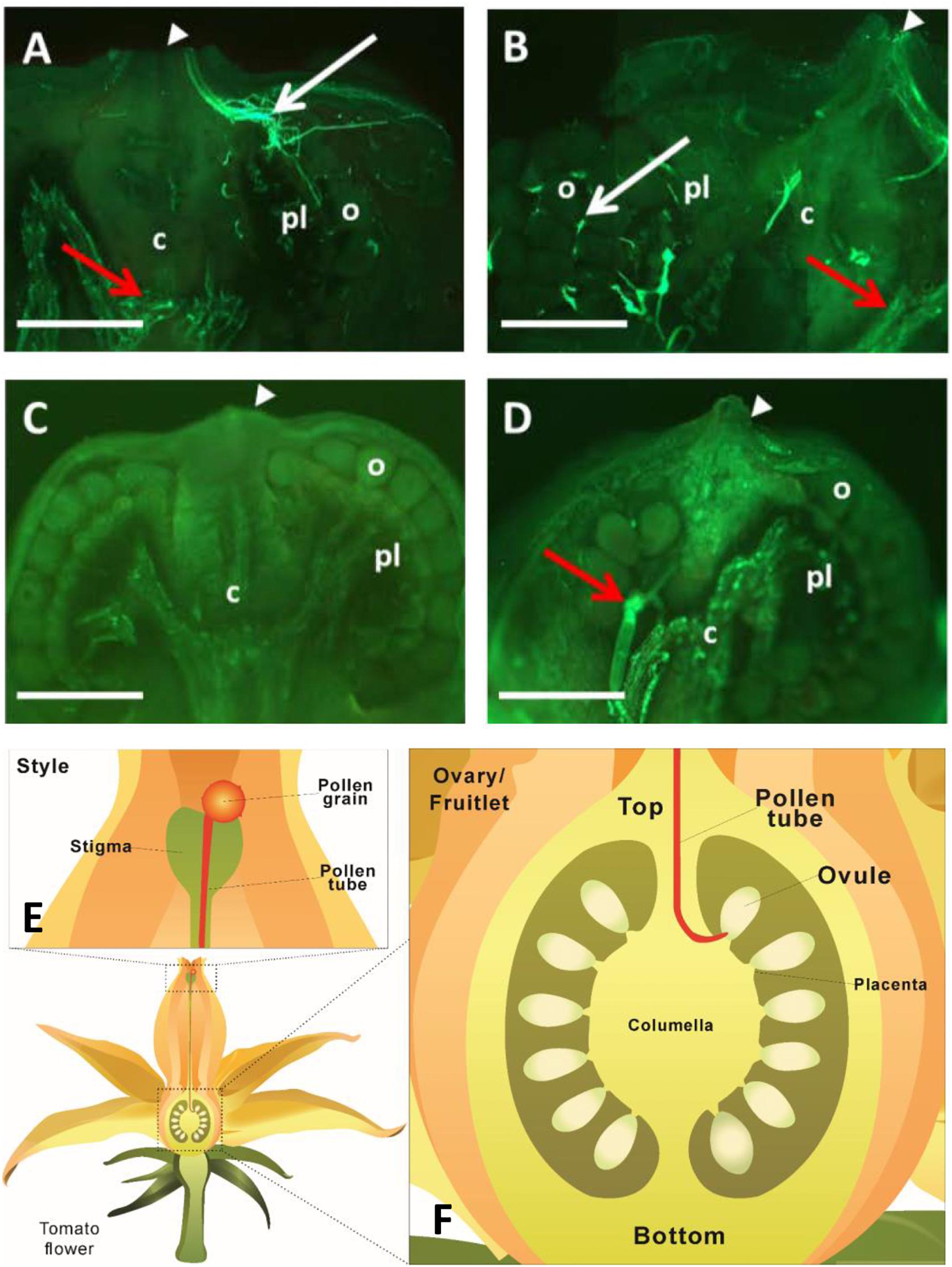
Figure 2. Visualization of pollen tube elongation within tomato pistils. (A,B) Longitudinal sections of ovary and fruitlet at 24 and 48 h after pollination (HAP), respectively. Pollen tubes, exhibiting fluorescence from the callose, entered the ovary through the junction of ovary and style (white arrowheads) at 24 HAP (A) and arrived at ovules at 48 HAP (B), as indicated by white arrows. (C) Negative control I, fruitlet derived from pollinated flower at 48 HAP without staining with aniline blue. No fluorescence was detected in the fruit. (D) Negative control II, fruitlet at 48 HAP, derived from non-pollinated flower, but stained with aniline blue. Fluorescence was only found at the bottom of fruit, but not in ovules. Red arrows at the bottom of ovaries in (A,B,D) indicate fluorescence from the pre-existing callose of ovary tissues, mainly the vascular bundles. White arrowheads indicate the connection between ovary and style in (A–D). Each image is one representative out of four biological replicates. c, columella; pl, placenta; o, ovule; Scale bars represent 0.25 mm in (A,B), 0.5 mm in (C,D). (E) An illustration showing that pollen tube elongates along the style. (F) A schematic diagram of a tomato ovary. The pollen tube enters through the top of the ovary and reaches ovules to finish the fertilization process.
Compared with non-pollinated treatment, the expression of LIN5 was induced by ∼2 times at 4 HAP and ∼3 times at 2 DAP in the manually pollinated style (Figure 3). Interestingly, LIN5 expression in the ovaries was not induced, but rather was slightly reduced by the pollination treatment at 4 HAP (Figure 3). Remarkably, the CWIN mRNA level was more than doubled in the ovaries by 2 DAP (Figure 3), when fertilization has occurred (Figure 2), turning the ovaries into fruitlets. By comparison, pollination treatment did not affect the expression of LIN7, which is mainly expressed in anthers and pollens (Godt and Roitsch, 1997), except a slight reduction in ovaries at 4HAP. The expression of INVINH1 encoding the inhibitory protein against CWIN activity (Jin et al., 2009) was significantly reduced at 2 DAA in pollinated style compared with non-pollinated ones but not affected in the ovaries by pollination treatment at 4 HAP or 2 DAP (Figure 3).
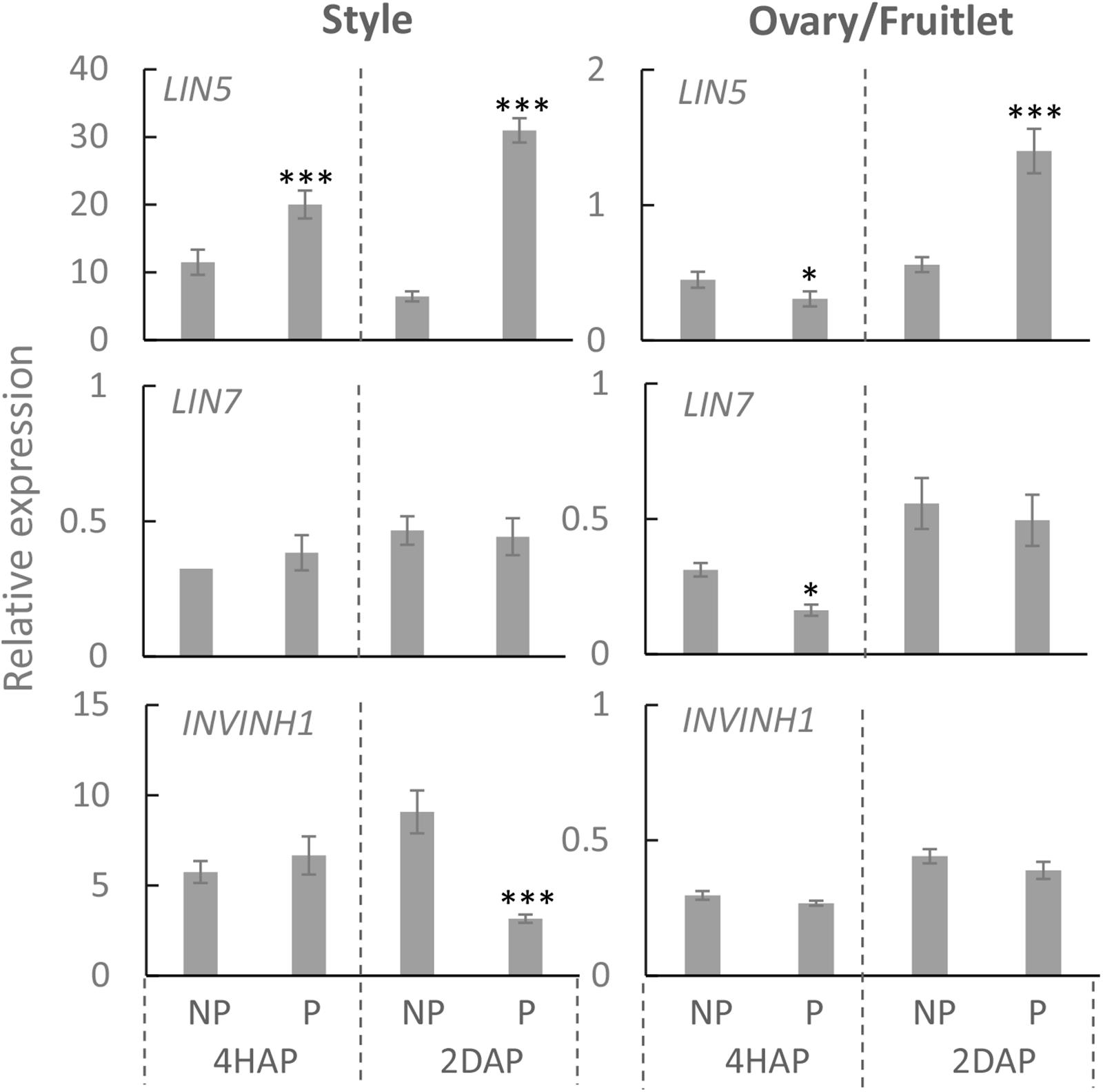
Figure 3. Relative expression of CWIN-related genes LIN5, LIN7, and INVINH1, in style and fruitlet in response to pollination at 4 HAP and fertilization at 2 DAP. Asterisks indicate significant differences (Student’s t-test, ∗p < 0.05; ∗∗p < 0.01; ∗∗∗p < 0.001, n = 4) between non-pollination and pollination treatments. DAP, days after pollination; HAP, hours after pollination.
Consistent with the gene expression data (Figure 3), an enzyme assay revealed that CWIN activity was significantly increased in the pollinated style from 4 HAP to 2 DAP and in the fertilized fruitlet at 2 DAP (Figure 4). In higher plants, there are other invertases hydrolyzing sucrose into hexoses, namely cytoplasmic invertase (CIN), and vacuolar invertase (VIN), classified based on their subcellular localizations (Ruan, 2014). Previous research has demonstrated that the VIN contributes to cell division and expansion, whereas the CIN may play a role in cytosolic hexose homeostasis (Ruan, 2014; Wan et al., 2018). In this experiment, the pollination-triggered increase in sucrose hydrolysis activity in the style appeared specific to CWIN since neither VIN nor CIN activity was induced during pollination and fertilization (Figure 4). Within the ovaries/fruitlets, VIN activity also showed a slight increase by the pollination treatment at 4 HAP and 2 DAP, with no changes in CIN activity (Figure 4).
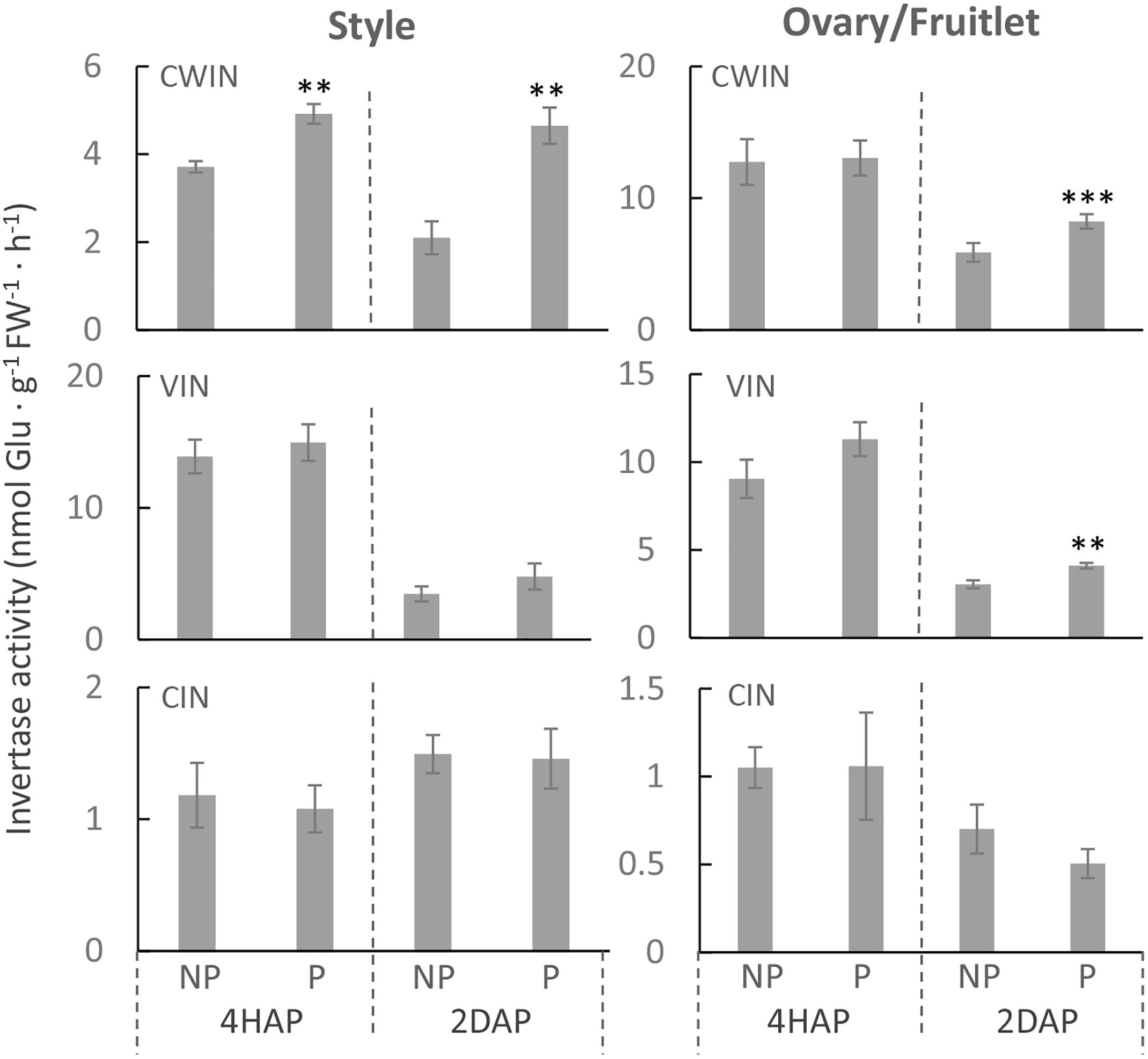
Figure 4. The activities of CWIN, VIN, and CIN in style and fruitlet in response to pollination at 4 HAP and fertilization at 2 DAP. Asterisks indicate significant differences (Student’s t-test, ∗p < 0.05; ∗∗p < 0.01; ∗∗∗p < 0.001, n = 4) between non-pollination and pollination treatments. DAP, days after pollination; HAP, hours after pollination.
Expression of Hexose Transporter Genes, SlHT1,2 and SlSWEET5a, Was Stimulated During Pollination in the Style With SlHT1, 2 Expression Enhanced Upon Fertilization in the Fruitlets
Three hexose transporter (HT) genes are expressed in developing tomato fruit, which are SlHT1, 2, and 3 (Gear et al., 2000). They belong to the STP sub-family of monosaccharide transporters that localize to the plasma membrane (McCurdy et al., 2010). Here, we found that in the pistil, only LeHT1 and 3 were highly expressed from 2DBA to 4DAA (Supplementary Figure S1), whereas, all three SlHT genes were expressed in anther and petal (Supplementary Figure S1). Interestingly, the SlHT1 transcript level was induced by ∼5 times in the pollinated style from 4 HAP to 2 DAP compared with the non-pollinated one (Figure 5). In the ovaries, the expression of SlHT1 showed a slight reduction at 4 HAP prior to fertilization but a significant increase at 2 DAP upon fertilization (Figure 5). Although SlHT2 was slightly expressed in pistil (Supplementary Figure S1), its expression was significantly increased in the pollinated style from 4 HAP to 2 DAP and in the fertilized ovary at 2DAP (Figure 5). SlHT3 showed no response to pollination, except a slight reduction in the fertilized fruitlet at 2 DAP (Figure 5).
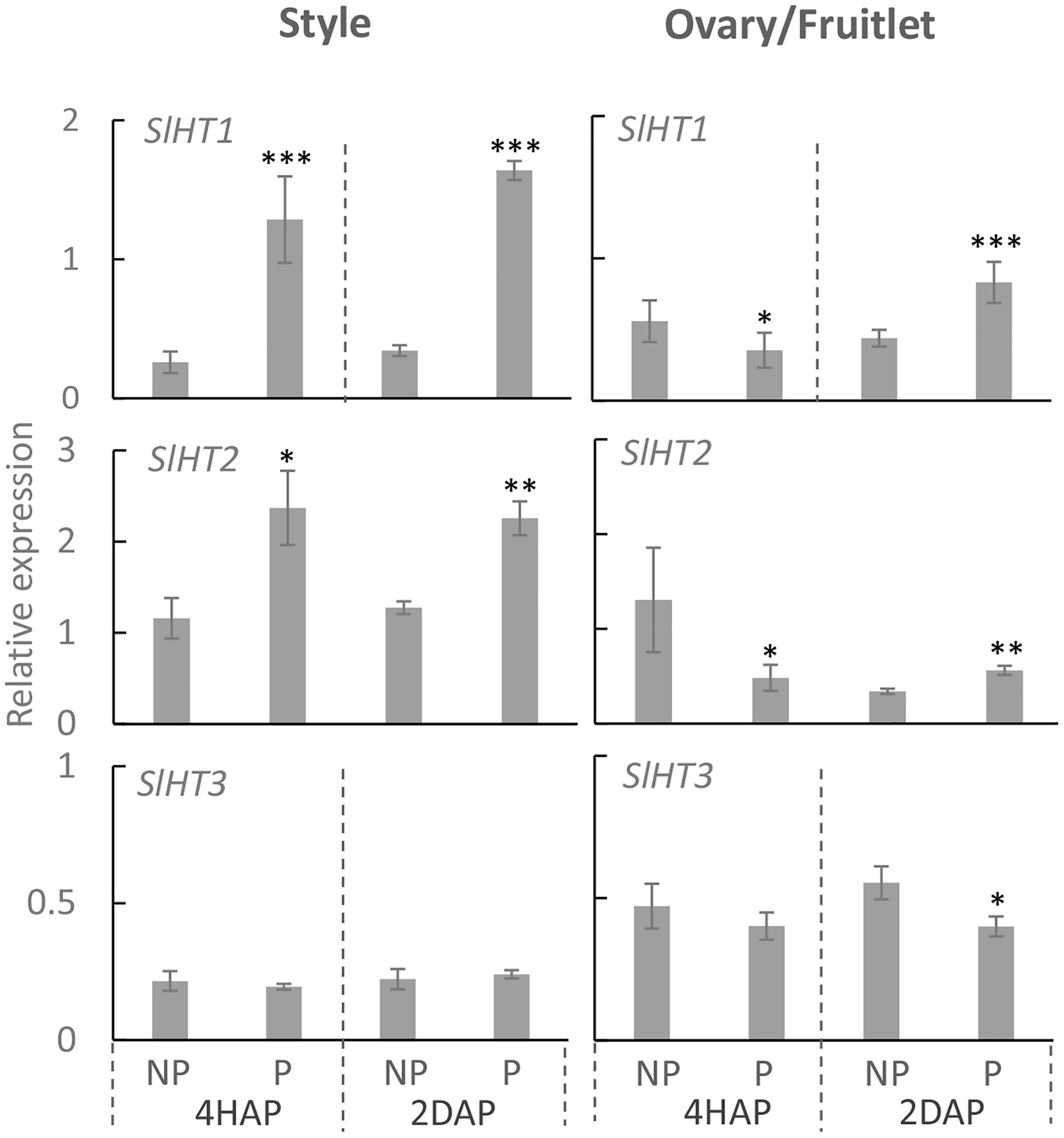
Figure 5. Relative gene expression of SlHT1, SlHT2, and SlHT3 in style and fruitlet in response to pollination at 4 HAP and fertilization at 2 DAP. Asterisks indicate significant differences (Student’s t-test, ∗p < 0.05; ∗∗p < 0.01; ∗∗∗p < 0.001, n = 4) between non-pollination and pollination treatments. DAP, days after pollination; HAP, hours after pollination.
We then examined the possible effect of pollination and fertilization on the expression of a class of recently identified genes encoding SWEETs. Among the 29 SWEETs in the tomato genome, only SlSWEET5b, 10b, 11a, 12a, and 16 were highly expressed in flower buds and fully opened flowers (Feng et al., 2015). Phylogenetic analysis of the tomato SWEETs with that in Arabidopsis (Supplementary Figure S2), coupled with current knowledge on the functionality of SWEETs (Chen et al., 2010; Chen et al., 2015; Eom et al., 2015), revealed that SWEET5b belonged to clade II for transport of hexoses, whereas SWEET10b, 11a, 12a were grouped with clade III for moving sucrose, and SWEET16 is a clade IV uniporter functioning on tonoplast for transport of hexoses.
Quantitative real-time PCR results showed that expressions of SlSWEET5b and 16 were increased in the style at 4 DAA compared with that at 2 DBA, whereas in the ovary or fruitlet SlSWEET10b and 12a were highly expressed at 2 DBA and 4 DAA (Supplementary Figure S3). SlSWEET11a exhibited little expression in the style and ovary (Supplementary Figure S3), and hence is not included in our further analyses. Notably, in the style SlSWEET5b gene expression was significantly stimulated from 4 HAP to 2 DAP by the pollination treatment (Figure 6). This gene was not expressed in the ovary or style at 2 DBA (Supplementary Figure S3) and not responsive to pollination in the fruitlet. SlSWEET10b and 12a were expressed in the ovaries and fruitlets (Supplementary Figure S3). However, neither of them responded to pollination or fertilization (Figure 6). SlSWEET16 also did not show any difference between pollinated and non-pollinated treatments (Figure 6).
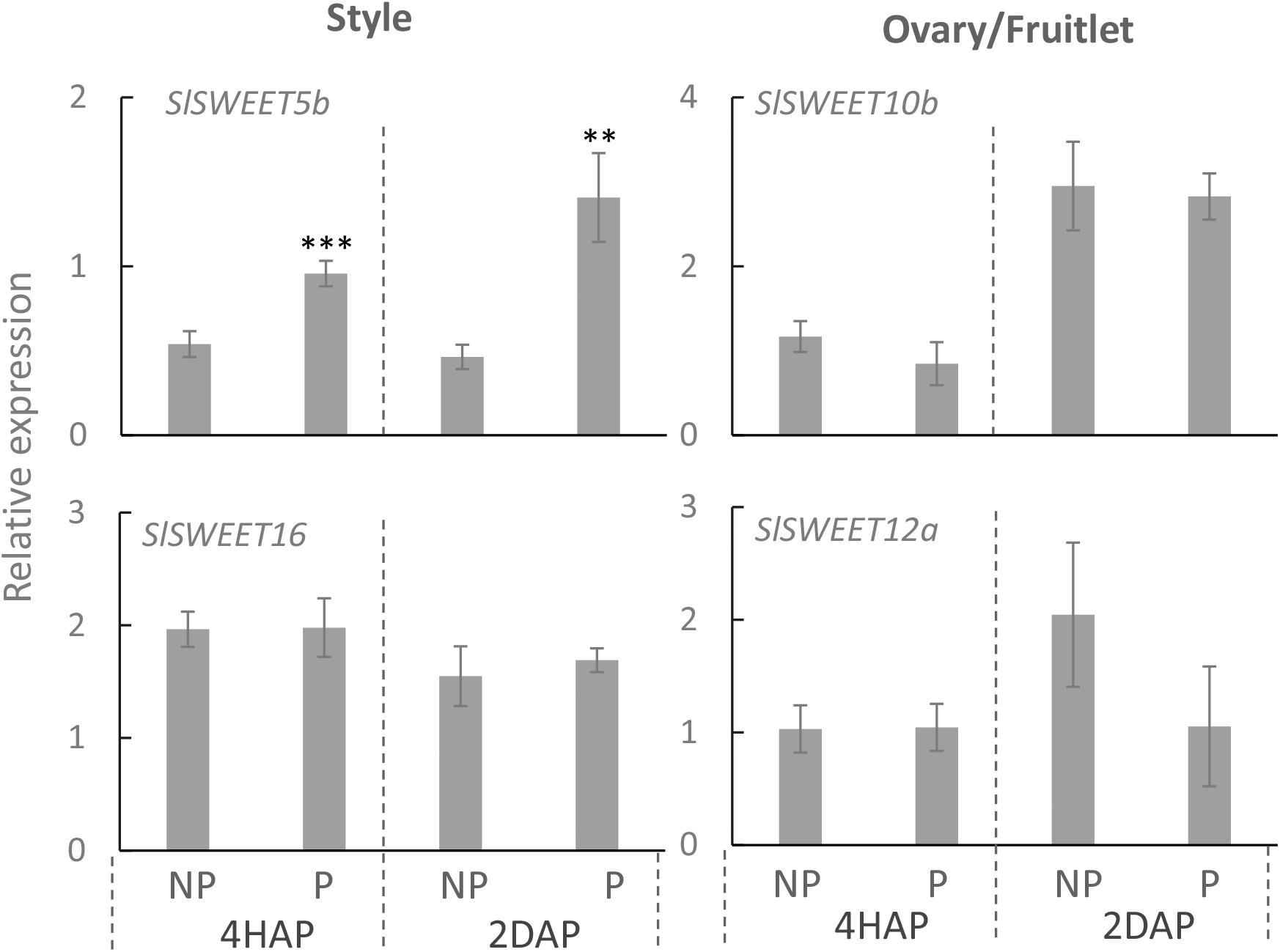
Figure 6. Relative expression of SlSWEET transporter genes in response to pollination at 4 HAP and fertilization at 2 DAP. Asterisks indicate significant differences (Student’s t-test, ∗p < 0.05; ∗∗p < 0.01; ∗∗∗p < 0.001, n = 4) between non-pollination and pollination treatments. DAP, days after pollination; HAP, hours after pollination.
Pollination Treatment Significantly Enhanced the Expression of SlSUT1, 2, and 4 in the Style but With Only SlSUT1 Increased in the Ovaries Upon Fertilization
Proton-coupled sucrose transporters (SUTs) are also involved in the delivery of sugars to support pollen tuber elongation in tomato (Hackel et al., 2006), tobacco (Goetz et al., 2017), Arabidopsis (Rottmann et al., 2018), and rice (Hirose et al., 2014). We therefore examined if expression of SlSUTs in the style and ovary were impacted upon pollination and fertilization. In tomato, there are three SIUT genes expressed in floral bud and flowers, namely SlSUT1, 2, and 4 (Hackel et al., 2006; Kühn and Grof, 2010). qRT-PCR analyses showed that SlSUIT 2 and 4 were upregulated in the pollinated style at 4 HAP, followed by a dramatic increase in the mRNA levels of SlSUT1 and 4 at 2 DAP (Figure 7). The ovaries did not respond to pollination at the transcript levels of the three SlSUTs at 4 HAP but exhibited an induction for SlSUT1 at 2 DAP when fertilization occurred (Figure 7).
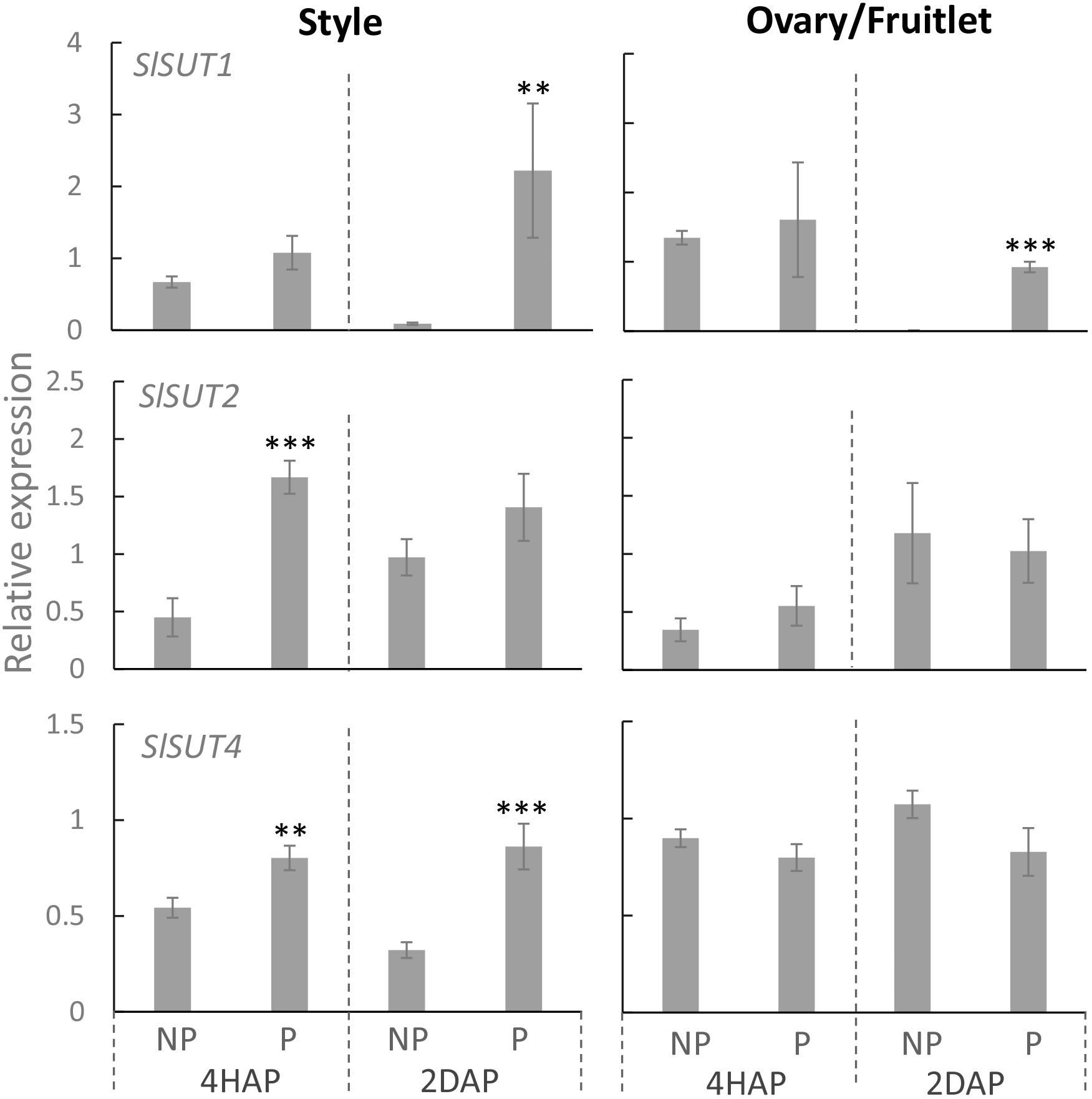
Figure 7. Relative expression of SlSUT transporter genes in response to pollination at 4 HAP and fertilization at 2 DAP. Asterisks indicate significant differences (Student’s t-test, ∗p < 0.05; ∗∗p < 0.01; ∗∗∗p < 0.001, n = 4) between non-pollination and pollination treatments. DAP, days after pollination; HAP, hours after pollination.
Discussion
Pollination and fertilization are prerequisite for flower plants to set seed and fruit. These processes are highly energy-consuming and rely on the efficient input of sugars to fuel the growth of pollen tubes and the young seeds and fruitlets (Ruan et al., 2012). CWIN and sugar transporters are known to play crucial roles in supplying nutritional and signaling sugars into elongating pollen tubes, embryos, and endosperms that were symplasmically isolated from the surrounding maternal tissues (Wang and Ruan, 2012; Goetz et al., 2017). To our knowledge, however, there have been no reports which dissected the specific effects of pollination and fertilization on the expression of these genes. Here, we present evidence that the expression of CWIN and some specific sugar transporters was differentially stimulated in style and fruitlet upon pollination and fertilization, respectively. These findings provide insights into the roles of pollination and fertilization in activating apoplasmic sucrose unloading and input in reproductive organs and identify new genetic targets for improving reproductive success.
CWIN Expression and Activity in the Style and Fruitlets Were Activated Upon Pollination and Fertilization, Respectively
It has been reported that by applying a chemical invertase inhibitor, miglitol, in vitro pollen germination and pollen tube growth were strongly inhibited, which could not be fully restored by adding sucrose into the medium (Goetz et al., 2017). This finding indicates the role of invertase in pollen germination and growth likely by facilitating apoplasmic unloading of sucrose to support heterotrophic pollen tube growth. Similarly, down-regulation of CWIN gene expression or activity correlates with pollen sterility and grain abortion in rice (Hirose et al., 2002; Oliver et al., 2005) and maize (Ruan et al., 2010; Shen et al., 2018). However, it remains largely unknown how CWIN and its functionally related genes respond to pollination and fertilization for seed and fruit set. To this end, the expression of LIN5 was changed from a dispersed pattern in unfertilized ovaries to a phloem-specific pattern in young fruitlet, indicating pollination or fertilization could elicit a signal or signals to transcriptionally or post-transcriptionally modulate CWIN gene expression (Palmer et al., 2015). However, whether the signals were derived from pollination or fertilization remains unknown. Here, we found that in the style LIN5 expression was stimulated by pollination treatment from 4 HAP to 2 DAP, whereas in the fertilized fruitlet LIN5 expression was enhanced at 2 DAP when fertilization was accomplished (Figures 2, 3). These findings strongly indicate that the CWIN gene was stimulated by signals emitted during pollination and fertilization in the style and fruitlet, respectively, although the identity of the signals is yet to be determined. Moreover, we noticed that the CWIN inhibitor gene INVINH1 was significantly suppressed in the style by pollination treatment at 2 DAP (Figure 3). Consequently, CWIN activity was significantly increased in the pollinated style and fertilized fruitlet (Figure 4). The elevated CWIN activity likely supports the rapid elongation of pollen tubes and the onset of seed and fruit development upon fertilization by (i) facilitating apoplasmic phloem unloading of sucrose in the style and in the fruitlets (Ruan et al., 1995; Palmer et al., 2015), and (ii) providing hexoses to fuel pollen tube elongation and to promote cell division in the newly formed fruitlets (Ruan et al., 2012).
Activated SlHT1 and 2 During Pollination and Fertilization Could Channel Hexoses to Pollen Tubes and Fertilized Fruitlets
After sucrose hydrolysis by CWIN, the resultant hexoses could be taken up into the cytoplasm of the recipient cells by H+-coupled HTs (Ruan and Patrick, 1995; Ruan, 2014). Among the three SlHTs, SlHT1, 2, and 3 that were expressed in flowers and young fruits (McCurdy et al., 2010), SlHT1 and 3 were highly expressed in ovary/fruitlet and the style whereas SlHT2 was preferentially expressed in the anther and petal (Supplementary Figure S1), indicating their different roles in the flowering and fruit set processes.
HTs have been shown to be responsive to pathogens (Fotopoulos et al., 2003; Lemonnier et al., 2014), abiotic stresses (Yamada et al., 2011), and its substrate sugars (Rottmann et al., 2016; Rottmann et al., 2018). In this study, we found that SlHT1 and 2, but not 3, were stimulated in the style after pollination and in the fruitlet upon fertilization (Figure 5). This finding complements the understandings on metabolic regulation on HT in reproductive organs. We hypothesize that the activated SlHT1 during pollination and fertilization is significant for the growth of pollen tubes and fertilized fruitlets for the following reasons: (i) SlHT1, a member of the STP families, was localized on the plasma membrane to transport hexoses (McCurdy et al., 2010), and it would provide hexoses into the growing pollen tube and rapidly proliferating fruitlet as an energy source and signaling molecules (Ruan et al., 2010; Ruan, 2014; Goetz et al., 2017; Rottmann et al., 2018); (ii) the activated HT may help in maintaining the cellular osmotic potential and turgor that are required for cell elongation and enlargement (Ruan et al., 2001; Zhang et al., 2017); and (iii) HT may play regulatory roles by adjusting the cellular hexose concentration and thus sugar signaling pathways (Rolland et al., 2006; McCurdy et al., 2010; Ruan, 2014; Wan et al., 2018).
SlSUTs in the Style Were Broadly Activated During Pollination but Less So in the Fruitlet Following Fertilization
In the processes of pollen germination and pollen tube elongation, SUT was demonstrated to operate in parallel with CWIN and HT (Lemoine et al., 1999; Goetz et al., 2017). In tomato, three SlSUTs have been identified, among them SlSUT1 and SlSUT2 were predominantly expressed in the pollen tubes as well as in the sieve elements of young fruitlets (Hackel et al., 2006), whereas SlSUT4 was expressed mainly in the styles and ovaries (Weise et al., 2000; Hackel et al., 2006). Immunolocalization analyses demonstrated that all three SlSUT proteins are localized at the plasma membrane of sieve elements in tomato (Reinders et al., 2002; Krügel et al., 2008). Previous studies have shown the critical roles of SUTs in the development of pollen grains (Lemoine et al., 1999; Hirose et al., 2010), pollen tube elongation (Hackel et al., 2006; Hirose et al., 2014), and seed development (Patrick and Offler, 2001). However, it remains unknown from these studies how the SUTs in styles and ovaries are potentially regulated during pollination or fertilization. Here, we found that expressions of SlSUT1, 2 and 4 in the style were all stimulated by pollination treatment, but only SlSUT1 was enhanced in the fruitlet when fertilization occurred (Figure 7). Combined with the tissue-specific gene expression and subcellular localization of SUTs as discussed above (Weise et al., 2000; Hackel et al., 2006), we propose that in the styles, SlSUT4 is rapidly activated, followed by SlSUT1 and 2 during pollination to uptake sucrose into the growing pollen tubes from the phloem unloading region in the style. In the fruitlet, on the other hand, SlSUT1 is stimulated upon fertilization to uptake more apoplasmic sucrose to support the rapid cell proliferation of the young seed and fruitlet. The analysis concurs with previous findings (Hackel et al., 2006) that SlSUT1 and 2 antisense plants exhibited smaller fruits and fewer seeds. Our findings demonstrated the significance of successful pollination and fertilization on stimulating SUTs to support the growth of pollen tubes and young seeds and fruits.
SlSWEET5b Expression Was Stimulated in the Style During Pollination but With No SlSWEETs Activated in the Fruitlet Upon Fertilization
In recent years, SWEETs were characterized and localized on the plasma membranes or tonoplasts to transport sucrose or hexoses, thereby regulating carbon allocation throughout the plant body (Chen et al., 2012; Eom et al., 2015). For instance, phloem loading was blocked and starch was accumulated in the Atsweet11;12 double mutant (Chen et al., 2012), whereas phloem unloading and post-phloem transport were impaired in the Arabidopsis sweet11;12;15 triple mutant, maize Zmsweet4c mutants and rice Ossweet4 mutant, all leading to defective seed filling (Chen et al., 2015; Sosso et al., 2015; Ma et al., 2017).
Findings from our study provide new information regarding the impacts of pollination and fertilization on SWEET expression in reproductive organs. We found that in the tomato ovary and fruitlet SlSWEET10b and 12a were the main ones expressed, whereas in style SlSWEET5b and 16 were dominant (Supplementary Figure S3). While SlSWEET10b and 12a did not respond to fertilization in the fruitlets, the expression of SlSWEET5b in the style was specifically stimulated during pollination at 4 HAP and was further enhanced at 2 DAP (Figure 6). Phylogenetic analysis revealed SlSWEET5b belongs to clade II of the SWEET subfamily that was predicted to localize on the plasma membrane to transport hexoses (Supplementary Figure S2). SlSWEET5b was not expressed in the styles at 2 DBA when there were no pollen grains or pollen tubes (Supplementary Figure S3). Thus, it is apparently induced by pollination and likely functions in the uptake of apoplasmic hexoses into pollen tubes or reversely exported intracellular hexoses out of the pollen tubes (Chen et al., 2012). This bidirectional transport of hexoses is probably required for keeping cytosolic sugar homeostasis (Wan et al., 2018). We hypothesize that the coexistence of SlHTs (energy-dependent) and SlSWEET5b (energy-independent) may be crucial for maintaining fundamental cellular metabolism and function (Ruan, 2014). To this end, co-expression of energy-dependent and -independent sucrose transporters was observed in rapidly elongating cotton fibers, single-celled seed trichomes, by Zhang et al. (2017). Furthermore, a recent study has shown that an excess of cytosolic glucose inhibited pollen tube elongation (Rottmann et al., 2018), which further supports the concept that a system for bidirectional transport of sugars into and out of cytoplasm is crucial for cytosolic sugar homeostasis and cell viability (Ruan, 2014; Wan et al., 2018). Our finding, together with that reported by Rottmann et al. (2018), thus strongly indicates that the operation of an efflux pathway for excessive glucose is necessary for pollen tube growth.
A Model on Rregulation of CWIN and Sugar Transporters During Pollination and Fertilization for Fruit and Seed Set
Based on the analysis above, we propose a model of how the expression of CWIN and sugar transporters are regulated during pollination and fertilization to enhance assimilate input and utilization to fuel pollen tube elongation and seed and fruit set (Figure 8). In this model, CWIN and sugar transporters, differentially regulated during pollination and fertilization in the styles and fruitlets, are spatiotemporally coordinating with each other and collectively contribute to the uptake of apoplasmic sugars into pollen tubes and fertilized ovules to fuel their growth, characterized by rapid cell elongation in the former and cell division in the latter. Experiments are underway to identify the exact signals emitted from elongating pollen tubes or that are released from sperms or newly formed embryo and endosperm that activate the expression of CWIN and sugar transporter genes in the pollinated styles and fertilized ovules, respectively.
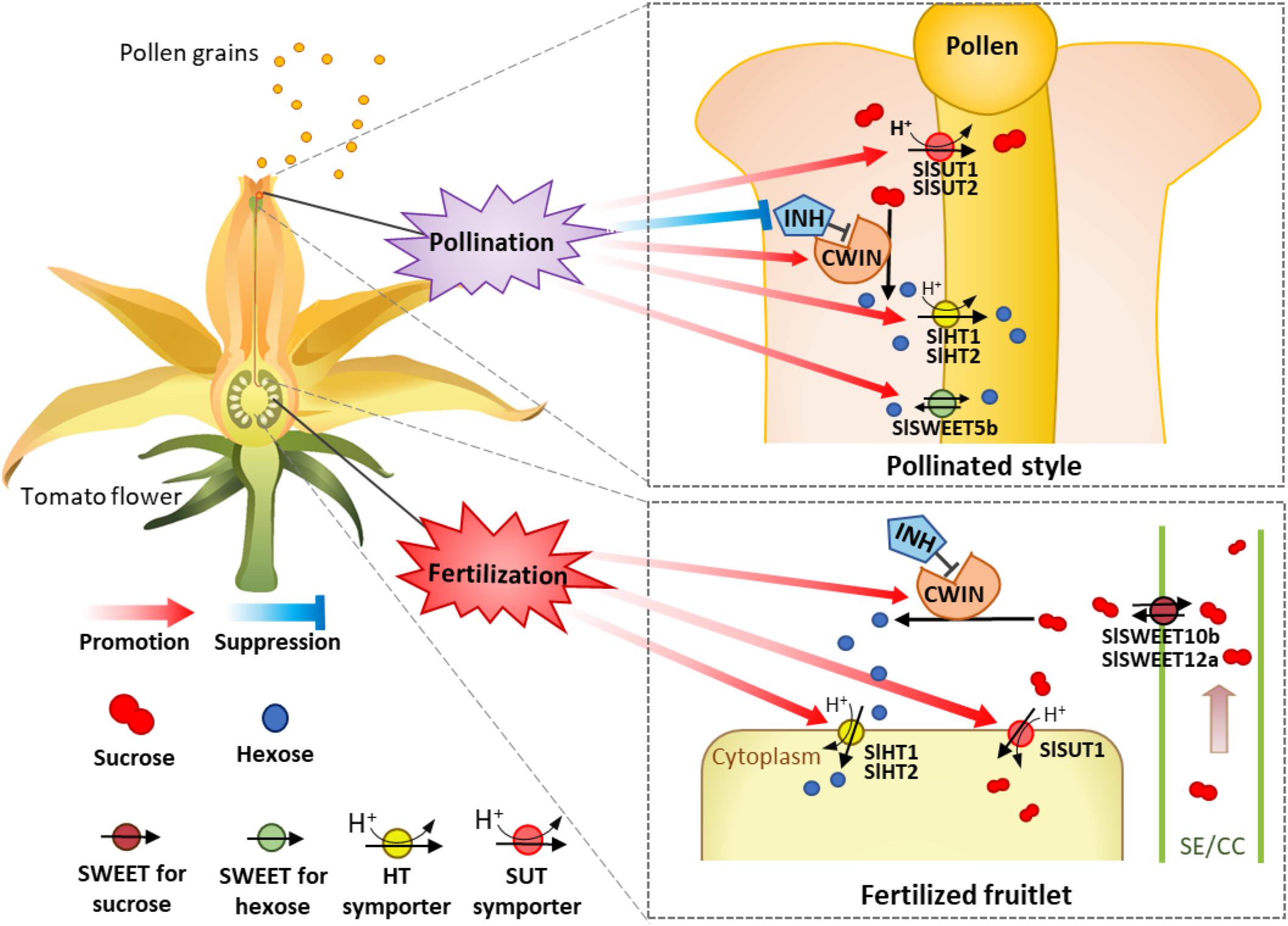
Figure 8. A model of how sugars transported into and metabolism within tomato style and ovary are regulated during pollination and fertilization. The occurrence of pollination stimulates and repressed cell wall invertase and its inhibitor gene (INVINH1), respectively, in the style, leading to high CWIN activity to hydrolyze sucrose into hexoses in the style apoplasm. Meanwhile, the expressions of sucrose transporter genes SlSUT1 and 2 as well as SlHT1 and 2 and SWEET5b for hexoses are also stimulated. The increased SlSWEET5b on the pollen tube might function in the uptake of apoplasmic hexose into pollen tubes or inversely exported intercellular hexose out of the pollen tube. The coexistences of SlSWEET and SlHT may contribute to the maintenance of cytosolic sugar homeostasis, which is crucial for metabolism and cellular function. These pollination-elicited changes enhance carbon input into and uses within pollen tubes to fuel their rapid elongation. For the ovaries or fruitlets, instead of pollination effect, the accomplishment of fertilization selectively stimulates the expression of genes for CWIN (LIN5), SlHT (SlHT1 and 2), and SlSUT (SlSUT1), but not those for SlSWEETs (SlSWEET10b and 12a), to support the growth of the newly formed fruitlets.
Author Contributions
Y-LR conceived the project. SS and Y-LR designed the experiments. SS, SM, YL, SL, JL, LW, H-PM, and DK conducted the experiments. SS, SM, and Y-LR analyzed the data and wrote the manuscript with inputs from LW and H-PM.
Funding
This work was funded by Australian Research Council (Grant No. DP180103834). SS gratefully acknowledges financial support from the China Scholarship Council for his living and study at the University of Newcastle.
Conflict of Interest Statement
The authors declare that the research was conducted in the absence of any commercial or financial relationships that could be construed as a potential conflict of interest.
Supplementary Material
The Supplementary Material for this article can be found online at: https://www.frontiersin.org/articles/10.3389/fpls.2019.00506/full#supplementary-material
FIGURE S1 | Relative expression of hexose transporter genes, SlHT1, SlHT2, and SlHT3, in different flower tissues of tomato at 2 days before (2 DBA) and 4 days after anthesis (4 DAA). Asterisks indicate significant differences (Student’s t-test, ∗p < 0.05; ∗∗p < 0.01; ∗∗∗p < 0.001, n = 4) between 2 DBA and 4 DAA.
FIGURE S2 | Phylogenetic analysis of SlSWEET families in tomato in relation to Arabidopsis. Based on the current knowledge, SWEETs in clade I and clade II transport hexoses; while those in clade III transport sucrose, all are predicted to be located on plasma membrane. SWEETs in clade IV are located on tonoplast to transport hexose.
FIGURE S3 | Relative expression of SlSWEET transporter genes, SlSWEET5b, 10b, 11a, 12a, and 16. Asterisks indicate significant differences (Student’s t-test, ∗p < 0.05; ∗∗p < 0.01; ∗∗∗p < 0.001, n = 4) between 2 DBA and 4 DAA.
TABLE S1 | The primers used in this study.
Footnotes
- ^ http://www.plantgdb.org/
- ^ https://solgenomics.net/
- ^ http://ted.bti.cornell.edu/cgi-bin/TFGD/digital/home.cgi
References
Boyer, J. S., and Mclaughlin, J. E. (2007). Functional reversion to identify controlling genes in multigenic responses: analysis of floral abortion. J. Exp. Bot. 58, 267–277. doi: 10.1093/jxb/erl177
Braun, D. M., Wang, L., and Ruan, Y. L. (2014). Understanding and manipulating sucrose phloem loading, unloading, metabolism, and signalling to enhance crop yield and food security. J. Exp. Bot. 65, 1713–1735. doi: 10.1093/jxb/ert416
Büttner, M., Truernit, E., Baier, K., Scholz-Starke, J., Sontheim, M., Lauterbach, C., et al. (2000). AtSTP3, a green leaf-specific, low affinity monosaccharide-H+ symporter of Arabidopsis thaliana. Plant Cell Environ. 23, 175–184. doi: 10.1046/j.1365-3040.2000.00538.x
Chen, L. Q., Hou, B. H., Lalonde, S., Takanaga, H., Hartung, M. L., Qu, X. Q., et al. (2010). Sugar transporters for intercellular exchange and nutrition of pathogens. Nature 468, 527–532. doi: 10.1038/nature09606
Chen, L. Q., Lin, I. W., Qu, X. Q., Sosso, D., Mcfarlane, H. E., Londono, A., et al. (2015). A cascade of sequentially expressed sucrose transporters in the seed coat and endosperm provides nutrition for the Arabidopsis embryo. Plant Cell 27, 607–619. doi: 10.1105/tpc.114.134585
Chen, L. Q., Qu, X. Q., Hou, B. H., Sosso, D., Osorio, S., Fernie, A. R., et al. (2012). Sucrose efflux mediated by SWEET proteins as a key step for phloem transport. Science 335, 207–211. doi: 10.1126/science.1213351
Cho, J. I., Lee, S. K., Ko, S., Kim, H. K., Jun, S. H., Lee, Y. H., et al. (2005). Molecular cloning and expression analysis of the cell-wall invertase gene family in rice (Oryza sativa L.). Plant Cell Rep. 24, 225–236. doi: 10.1007/s00299-004-0910-z
Dibley, S. J., Gear, M. L., Yang, X., Rosche, E. G., Offler, C. E., Mccurdy, D. W., et al. (2005). Temporal and spatial expression of hexose transporters in developing tomato (Lycopersicon esculentum) fruit. Funct. Plant Biol. 32, 777–785. doi: 10.1071/FP04224
Dorion, S., Lalonde, S., and Saini, H. S. (1996). Induction of male sterility in wheat by meiotic-stage water deficit is preceded by a decline in invertase activity and changes in carbohydrate metabolism in anthers. Plant Physiol. 111, 137–145. doi: 10.1104/pp.111.1.137
Eom, J. S., Chen, L. Q., Sosso, D., Julius, B. T., Lin, I. W., Qu, X. Q., et al. (2015). SWEETs, transporters for intracellular and intercellular sugar translocation. Curr. Opin. Plant Biol. 25, 53–62. doi: 10.1016/j.pbi.2015.04.005
Feng, C. Y., Han, J. X., Han, X. X., and Jiang, J. (2015). Genome-wide identification, phylogeny, and expression analysis of the SWEET gene family in tomato. Gene 573, 261–272. doi: 10.1016/j.gene.2015.07.055
Fotopoulos, V., Gilbert, M. J., Pittman, J., Marvier, A. C., Buchanan, A. J., Sauer, N., et al. (2003). The monosaccharide transporter gene, AtSTP4, and the cell-wall invertase, Atβfruct1, are induced in Arabidopsis during infection with the fungal biotroph Erysiphe cichoracearum. Plant Physiol. 132, 821–829. doi: 10.1104/pp.103.021428
Franklin-Tong, V. E. (1999). Signaling and the modulation of pollen tube growth. Plant Cell 11, 727–738. doi: 10.1105/tpc.11.4.727
Fridman, E., and Zamir, D. (2003). Functional divergence of a syntenic invertase gene family in tomato, potato, and Arabidopsis. Plant Physiol. 131, 603–609. doi: 10.1104/pp.014431
Gear, M. L., Mcphillips, M. L., Patrick, J. W., and Mccurdy, D. W. (2000). Hexose transporters of tomato: molecular cloning, expression analysis and functional characterization. Plant Mol. Biol. 44, 687–697. doi: 10.1023/A:1026578506625
Godt, D. E., and Roitsch, T. (1997). Regulation and tissue-specific distribution of mRNAs for three extracellular invertase isoenzymes of tomato suggests an important function in establishing and maintaining sink metabolism. Plant Physiol. 115, 273–282. doi: 10.1104/pp.115.1.273
Goetz, M., Guivarch, A., Hirsche, J., Bauerfeind, M. A., Gonzalez, M. C., Hyun, T. K., et al. (2017). Metabolic control of tobacco pollination by sugars and invertases. Plant Physiol. 173, 984–997. doi: 10.1104/pp.16.01601
Guan, Y. F., Huang, X. Y., Zhu, J., Gao, J. F., Zhang, H. X., and Yang, Z. N. (2008). RUPTURED POLLEN GRAIN1, a member of the MtN3/saliva gene family, is crucial for exine pattern formation and cell integrity of microspores in Arabidopsis. Plant Physiol. 147, 852–863. doi: 10.1104/pp.108.118026
Hackel, A., Schauer, N., Carrari, F., Fernie, A. R., Grimm, B., and Kühn, C. (2006). Sucrose transporter LeSUT1 and LeSUT2 inhibition affects tomato fruit development in different ways. Plant J. 45, 180–192. doi: 10.1111/j.1365-313X.2005.02572.x
Heslop-Harrison, J. (1987). Pollen germination and pollen-tube growth. Int. Rev. Cytol. 107, 1–78. doi: 10.1016/S0074-7696(08)61072-4
Hirose, T., Hashida, Y., Aoki, N., Okamura, M., Yonekura, M., Ohto, C., et al. (2014). Analysis of gene-disruption mutants of a sucrose phosphate synthase gene in rice, OsSPS1, shows the importance of sucrose synthesis in pollen germination. Plant Sci. 225, 102–106. doi: 10.1016/j.plantsci.2014.05.018
Hirose, T., Takano, M., and Terao, T. (2002). Cell wall invertase in developing rice caryopsis: molecular cloning of OsCIN1 and analysis of its expression in relation to its role in grain filling. Plant Cell Physiol. 43, 452–459. doi: 10.1093/pcp/pcf055
Hirose, T., Zhang, Z., Miyao, A., Hirochika, H., Ohsugi, R., and Terao, T. (2010). Disruption of a gene for rice sucrose transporter, OsSUT1, impairs pollen function but pollen maturation is unaffected. J. Exp. Bot. 61, 3639–3646. doi: 10.1093/jxb/erq175
Jin, Y., Ni, D. A., and Ruan, Y. L. (2009). Posttranslational elevation of cell wall invertase activity by silencing its inhibitor in tomato delays leaf senescence and increases seed weight and fruit hexose level. Plant Cell 21, 2072–2089. doi: 10.1105/tpc.108.063719
Kremen, C., Williams, N. M., and Thorp, R. W. (2002). Crop pollination from native bees at risk from agricultural intensification. Proc. Natl. Acad. Sci. U.S.A. 99, 16812–16816. doi: 10.1073/pnas.262413599
Krügel, U., Veenhoff, L. M., Langbein, J., Wiederhold, E., Liesche, J., Friedrich, T., et al. (2008). Transport and sorting of the Solanum tuberosum sucrose transporter SUT1 is affected by posttranslational modification. Plant Cell 20, 2497–2513. doi: 10.1105/tpc.108.058271
Kühn, C., and Grof, C. P. (2010). Sucrose transporters of higher plants. Curr. Opin. Plant Biol. 13, 287–297. doi: 10.1016/j.pbi.2010.02.001
Lemoine, R., Bürkle, L., Barker, L., Sakr, S., Kühn, C., Regnacq, M., et al. (1999). Identification of a pollen-specific sucrose transporter-like protein NtSUT3 from tobacco. FEBS Lett. 454, 325–330. doi: 10.1016/S0014-5793(99)00843-1
Lemonnier, P., Gaillard, C., Veillet, F., Verbeke, J., Lemoine, R., Coutos-Thévenot, P., et al. (2014). Expression of Arabidopsis sugar transport protein STP13 differentially affects glucose transport activity and basal resistance to Botrytis cinerea. Plant Mol. Biol. 85, 473–484. doi: 10.1007/s11103-014-0198-5
Liu, Y., Offler, C. E., and Ruan, Y. L. (2016). Cell wall invertase promotes fruit set under heat stress by suppressing ROS-independent plant cell death. Plant Physiol. 172, 163–180. doi: 10.1104/pp.16.00959
Lord, E. M., and Russell, S. D. (2002). The mechanisms of pollination and fertilization in plants. Annu. Rev. Cell Dev. Biol. 18, 81–105. doi: 10.1146/annurev.cellbio.18.012502.083438
Ma, L., Zhang, D., Miao, Q., Yang, J., Xuan, Y., and Hu, Y. (2017). Essential role of sugar transporter OsSWEET11 during the early stage of rice grain filling. Plant Cell Physiol. 58, 863–873. doi: 10.1093/pcp/pcx040
Martin, F. W. (1959). Staining and observing pollen tubes in the style by means of fluorescence. Stain Technol. 34, 125–128. doi: 10.3109/10520295909114663
Mascarenhas, J. P. (1993). Molecular mechanisms of pollen tube growth and differentiation. Plant Cell 5, 1303–1314. doi: 10.1105/tpc.5.10.1303
McCurdy, D. W., Dibley, S., Cahyanegara, R., Martin, A., and Patrick, J. W. (2010). Functional characterization and RNAi-mediated suppression reveals roles for hexose transporters in sugar accumulation by tomato fruit. Mol. Plant 3, 1049–1063. doi: 10.1093/mp/ssq050
Mclaughlin, J. E., and Boyer, J. S. (2004). Sugar-responsive gene expression, invertase activity, and senescence in aborting maize ovaries at low water potentials. Ann. Bot. 94, 675–689. doi: 10.1093/aob/mch193
Nowack, M. K., Grini, P. E., Jakoby, M. J., Lafos, M., Koncz, C., and Schnittger, A. (2006). A positive signal from the fertilization of the egg cell sets off endosperm proliferation in angiosperm embryogenesis. Nat. Genet. 38, 63–67. doi: 10.1038/ng1694
Oliver, S. N., Van Dongen, J. T., Alfred, S. C., Mamun, E. A., Zhao, X., Saini, H. S., et al. (2005). Cold-induced repression of the rice anther-specific cell wall invertase gene OSINV4 is correlated with sucrose accumulation and pollen sterility. Plant Cell Environ. 28, 1534–1551. doi: 10.1111/j.1365-3040.2005.01390.x
Palmer, W. M., Ru, L., Jin, Y., Patrick, J. W., and Ruan, Y. L. (2015). Tomato ovary-to-fruit transition is characterized by a spatial shift of mRNAs for cell wall invertase and its inhibitor with the encoded proteins localized to sieve elements. Mol. Plant 8, 315–328. doi: 10.1016/j.molp.2014.12.019
Patrick, J. W., and Offler, C. E. (2001). Compartmentation of transport and transfer events in developing seeds. J. Exp. Bot. 52, 551–564. doi: 10.1093/jexbot/52.356.551
Proels, R. K., González, M. C., and Roitsch, T. (2006). Gibberellin-dependent induction of tomato extracellular invertase Lin7 is required for pollen development. Funct. Plant Biol. 33, 547–554. doi: 10.1071/FP04146
Regal, P. J. (1982). Pollination by wind and animals: ecology of geographic patterns. Annu. Rev. Ecol. Syst. 13, 497–524. doi: 10.1146/annurev.es.13.110182.002433
Reinders, A. (2016). Fuel for the road-sugar transport and pollen tube growth. J. Exp. Bot. 67, 2121–2123. doi: 10.1093/jxb/erw113
Reinders, A., Schulze, W., Kühn, C., Barker, L., Schulz, A., Ward, J. M., et al. (2002). Protein–protein interactions between sucrose transporters of different affinities colocalized in the same enucleate sieve element. Plant Cell 14, 1567–1577. doi: 10.1105/tpc.002428
Reuscher, S., Akiyama, M., Yasuda, T., Makino, H., Aoki, K., Shibata, D., et al. (2014). The sugar transporter inventory of tomato: genome-wide identification and expression analysis. Plant Cell Physiol. 55, 1123–1141. doi: 10.1093/pcp/pcu052
Rolland, F., Baena-Gonzalez, E., and Sheen, J. (2006). Sugar sensing and signaling in plants: conserved and novel mechanisms. Ann. Rev. Plant Biol. 57, 675–709. doi: 10.1146/annurev.arplant.57.032905.105441
Rottmann, T., Zierer, W., Subert, C., Sauer, N., and Stadler, R. (2016). STP10 encodes a high-affinity monosaccharide transporter and is induced under low-glucose conditions in pollen tubes of Arabidopsis. J. Exp. Bot. 67, 2387–2399. doi: 10.1093/jxb/erw048
Rottmann, T. M., Fritz, C., Sauer, N., and Stadler, R. (2018). Glucose uptake via STP transporters inhibits in vitro pollen tube growth in a HEXOKINASE1- dependent manner in Arabidopsis thaliana. Plant Cell 30, 2057–2081. doi: 10.1105/tpc.18.00356
Ru, L., Osorio, S., Wang, L., Fernie, A. R., Patrick, J. W., and Ruan, Y. L. (2017). Transcriptomic and metabolomics responses to elevated cell wall invertase activity during tomato fruit set. J. Exp. Bot. 68, 4263–4279. doi: 10.1093/jxb/erx219
Ruan, Y. L. (2014). Sucrose metabolism: gateway to diverse carbon use and sugar signaling. Aust. J. Plant Physiol. 65, 33–67. doi: 10.1146/annurev-arplant-050213-040251
Ruan, Y. L., Jin, Y., Yang, Y. J., Li, G. J., and Boyer, J. S. (2010). Sugar input, metabolism, and signaling mediated by invertase: roles in development, yield potential, and response to drought and heat. Mol. Plant 3, 942–955. doi: 10.1093/mp/ssq044
Ruan, Y. L., Llewellyn, D. J., and Furbank, R. T. (2001). The control of single-celled cotton fiber elongation by developmentally reversible gating of plasmodesmata and coordinated expression of sucrose and K+ transporters and expansion. Plant Cell 13, 47–60.
Ruan, Y. L., Mate, C., Patrick, J. W., and Brady, C. J. (1995). Non-destructive collection of apoplast fluid from developing tomato fruit using a pressure dehydration procedure. Aust. J. Plant Physiol. 22, 761–769. doi: 10.1071/PP9950761
Ruan, Y. L., and Patrick, J. W. (1995). The cellular pathway of postphloem sugar transport in developing tomato fruit. Planta 196, 434–444. doi: 10.1007/BF00203641
Ruan, Y. L., Patrick, J. W., Bouzayen, M., Osorio, S., and Fernie, A. R. (2012). Molecular regulation of seed and fruit set. Trends Plant Sci. 17, 656–665. doi: 10.1016/j.tplants.2012.06.005
Saitou, N., and Nei, M. (1987). The neighbor-joining method: a new method for reconstructing phylogenetic trees. Mol. Biol. Evol. 4, 406–425.
Sauer, N. (2007). Molecular physiology of higher plant sucrose transporters. FEBS Lett. 581, 2309–2317. doi: 10.1016/j.febslet.2007.03.048
Scott, R., Hodge, R., Paul, W., and Draper, J. (1991). The molecular biology of anther differentiation. Plant Sci. 80, 167–191. doi: 10.1016/0168-9452(91)90281-C
Shen, S., Zhang, L., Liang, X. G., Zhao, X., Lin, S., Qu, L. H., et al. (2018). Delayed pollination and low availability of assimilates are major factors causing maize kernel abortion. J. Exp. Bot. 69, 1599–1613. doi: 10.1093/jxb/ery013
Sosso, D., Luo, D., Li, Q. B., Sasse, J., Yang, J., Gendrot, G., et al. (2015). Seed filling in domesticated maize and rice depends on SWEET-mediated hexose transport. Nat. Genet. 47, 1489–1493. doi: 10.1038/ng.3422
Stadler, R., Lauterbach, C., and Sauer, N. (2005). Cell-to-cell movement of green fluorescent protein reveals post-phloem transport in the outer integument and identifies symplastic domains in Arabidopsis seeds and embryos. Plant Physiol. 139, 701–712. doi: 10.1104/pp.105.065607
Taylor, L. P., and Hepler, P. K. (1997). Pollen germination and tube growth. Ann. Rev. Plant Biol. 48, 461–491. doi: 10.1146/annurev.arplant.48.1.461
Tomato Genome Consortium (2012). The tomato genome sequence provides insights into fleshy fruit evolution. Nature 485, 635–641. doi: 10.1038/nature11119
Tomlinson, K. L., McHugh, S., Labbe, H., Grainger, J. L., James, L. E., Pomeroy, K. M., et al. (2004). Evidence that the hexose-to-sucrose ratio does not control the switch to storage product accumulation in oilseeds: analysis of tobacco seed development and effects of overexpressing apoplastic invertase. J. Exp. Bot. 55, 2291–2303. doi: 10.1093/jxb/erh251
Van Doorn, W. G. (1997). Effects of pollination on floral attraction and longevity. J. Exp. Bot. 48, 1615–1622. doi: 10.1093/jxb/48.9.1615
Wan, H. J., Wu, L. M., Yang, Y. J., Zhou, G. Z., and Ruan, Y. L. (2018). Evolution of sucrose metabolism: the dichotomy of invertases. Trends Plant Sci. 23, 163–177. doi: 10.1016/j.tplants.2017.11.001
Wang, E., Wang, J., Zhu, X., Hao, W., Wang, L., Li, Q., et al. (2008). Control of rice grain-filling and yield by a gene with a potential signature of domestication. Nat. Genet. 40, 1370–1374. doi: 10.1038/ng.220
Wang, L., and Ruan, Y. L. (2012). New insights into roles of cell wall invertase in early seed development revealed by comprehensive spatial and temporal expression patterns of GhCWIN1 in cotton. Plant Physiol. 160, 777–787. doi: 10.1104/pp.112.203893
Weber, H., Borisjuk, L., and Wobus, U. (2005). Molecular physiology of legume seed development. Ann. Rev. Plant Biol. 56, 253–279. doi: 10.1146/annurev.arplant.56.032604.144201
Weise, A., Barker, L., Kühn, C., Lalonde, S., Buschmann, H., Frommer, W. B., et al. (2000). A new subfamily of sucrose transporters, SUT4, with low affinity/high capacity localized in enucleate sieve elements of plants. Plant Cell 12, 1345–1355. doi: 10.1105/tpc.12.8.1345
Yamada, K., Kanai, M., Osakabe, Y., Ohiraki, H., Shinozaki, K., and Yamaguchi-Shinozaki, K. (2011). Monosaccharide absorption activity of Arabidopsis roots depends on expression profiles of transporter genes under high salinity conditions. J. Biol. Chem. 286, 43577–43586. doi: 10.1074/jbc.M111.269712
Yang, J., Luo, D., Yang, B., Frommer, W. B., and Eom, J. S. (2018). SWEET11 and 15 as key players in seed filling in rice. New Phytol. 218, 604–615. doi: 10.1111/nph.15004
Keywords: cell wall invertase, fertilization, fruit set, pollination, pollen tube elongation, sugar transporter, sugar utilization, tomato
Citation: Shen S, Ma S, Liu Y, Liao S, Li J, Wu L, Kartika D, Mock H-P and Ruan Y-L (2019) Cell Wall Invertase and Sugar Transporters Are Differentially Activated in Tomato Styles and Ovaries During Pollination and Fertilization. Front. Plant Sci. 10:506. doi: 10.3389/fpls.2019.00506
Received: 16 November 2018; Accepted: 02 April 2019;
Published: 18 April 2019.
Edited by:
Miguel Angel Ballicora, Loyola University Chicago, United StatesReviewed by:
Marina Gavilanes-Ruiz, National Autonomous University of Mexico, MexicoChristina Kühn, Humboldt University of Berlin, Germany
Copyright © 2019 Shen, Ma, Liu, Liao, Li, Wu, Kartika, Mock and Ruan. This is an open-access article distributed under the terms of the Creative Commons Attribution License (CC BY). The use, distribution or reproduction in other forums is permitted, provided the original author(s) and the copyright owner(s) are credited and that the original publication in this journal is cited, in accordance with accepted academic practice. No use, distribution or reproduction is permitted which does not comply with these terms.
*Correspondence: Yong-Ling Ruan, yong-ling.ruan@newcastle.edu.au