- 1Department of Radiology, Shanghai Jiao Tong University Affiliated Sixth People’s Hospital, Shanghai, China
- 2Department of Neurosurgery, Shanghai Jiao Tong University Affiliated Sixth People’s Hospital, Shanghai, China
- 3Division of Cardiology, Pauley Heart Center, Virginia Commonwealth University, Richmond, VA, United States
- 4Institute for Global Health Sciences, University of California, San Francisco, San Francisco, CA, United States
Objective: To investigate the association of MRI-visible perivascular spaces (PVS) with cognitive impairment in military veterans with traumatic brain injury (TBI), and whether cerebrospinal fluid (CSF) p-tau and Aβ mediate this effect.
Materials and Methods: We included 55 Vietnam War veterans with a history of TBI and 52 non-TBI Vietnam War veterans from the Department of Defense Alzheimer’s Disease Neuroimaging Initiative (ADNI) database. All the subjects had brain MRI, CSF p-tau, Aβ, and neuropsychological examinations. MRI-visible PVS number and grade were rated on MRI in the centrum semiovale (CSO-PVS) and basal ganglia (BG-PVS). Multiple linear regression was performed to assess the association between MRI-visible PVS and cognitive impairment and the interaction effect of TBI. Additionally, mediation effect of CSF biomarkers on the relationship between MRI-visible PVS and cognitive impairment was explored in TBI group.
Results: Compared with military control, TBI group had higher CSO-PVS number (p = 0.001), CSF p-tau (p = 0.022) and poorer performance in verbal memory (p = 0.022). High CSO-PVS number was associated with poor verbal memory in TBI group (β = -0.039, 95% CI −0.062, −0.016), but not in military control group (β = 0.019, 95% CI −0.004, 0.043) (p-interaction = 0.003). Further mediation analysis revealed that CSF p-tau had a significant indirect effect (β = −0.009, 95% CI: −0.022 −0.001, p = 0.001) and mediated 18.75% effect for the relationship between CSO-PVS and verbal memory in TBI group.
Conclusion: MRI-visible CSO-PVS was more common in Vietnam War veterans with a history of TBI and was associated with poor verbal memory, mediated partially by CSF p-tau.
Introduction
Traumatic brain injury (TBI) is a major cause of morbidity and mortality worldwide, especially in military veterans (1–3). Previous studies have revealed that military veterans with TBI would have a poor performance in cognitive and psychological functioning (4, 5). Epidemiologic Studies even demonstrated an increased incidence of dementia including Alzheimer’s disease (AD) in military veterans with TBI (6, 7). Amyloid beta (Aβ) and/or tau accumulation may play an important role. Recent studies have detected tau and Aβ deposition in the brain of military veterans with TBI by PET examinations (8, 9). However, the upstream mechanisms underlying cognitive impairment remain poorly understood.
MRI-visible perivascular spaces (PVS) are fluid-filled spaces surrounding small penetrating blood vessels (10, 11). Traditionally, MRI-visible PVS was regarded as an imaging marker of small vessel disease and correlated with aging (12). However, more and more studies suggested MRI-visible PVS may be an important imaging marker of dysfunction of brain glymphatic system, through which brain waste like tau and Aβ could be cleared away (13–15). PVS has been proved to be associated with tau pathology (16) and cognitive impairment in Parkinson’s disease (17). In an animal study of TBI, Iliff et al. (18) found chronic impairment of glymphatic system after TBI which may further promote tau aggregation. At present, only a few studies found an increased number of MRI-visible PVS in TBI (19–21). However, the previous studies of TBI did not discriminate CSO-PVS from BG-PVS. It remains unclear about the distribution characteristics of MRI-visible PVS in military veterans with TBI and its association with cognitive impairment and tau and Aβ pathology.
In this study, we hypothesize that (1) military veterans with TBI will have higher MRI-visible PVS number and cerebrospinal fluid (CSF) p-tau and Aβ42/40; (2) MRI-visible PVS would be associated with cognitive impairment, and CSF biomarkers will mediate this effect in military veterans with TBI. Our study would provide an innovative imaging biomarker and a new potential therapeutic target for cognitive impairment in military veterans with TBI.
Materials and Methods
Study Subjects
The study data in this study were collected from the DOD-ADNI as part of Alzheimer’s Disease Neuroimaging Initiative (ADNI) database,1 which was launched in 2003, led by Principal Investigator Michael W. Weiner, M (22). The primary goal of ADNI project was to test whether serial MRI, PET, other biological markers, and clinical and neuropsychological assessments can be combined to measure the mild cognitive impairment progression and early AD. DOD-ADNI aimed to investigate whether TBI and post-traumatic stress disorder (PTSD) increase the risk for AD in Veteran subjects. Written informed consent was obtained from all the study subjects, and the study protocol was proved by local participating ADNI institutions.
The inclusion and exclusion procedures were shown in Figure 1. Our study included male Vietnam War veterans with or without a history of non-penetrating TBI, who had complete brain MRI, CSF p-tau, Aβ, and neuropsychological tests results information. Patients with dementia, history of psychosis or bipolar affective disorder, history of schizophrenia (DSM-IV criteria), history of alcohol or substance abuse/dependence within the past 5 years, MRI related exclusions (metal in the body, pacemakers), contraindications for lumbar puncture were excluded in this project. Demographics including age, education, and APOE ε4 status were collected. Medical history of hypertension, diabetes, and hyperlipidemia was also collected from the medical history dataset.
Traumatic Brain Injury Classification
The documented history of TBI was identified from the Department of Defense or Veterans Administration records, and TBI severity was classified. Mild TBI was defined as loss of consciousness lasting for less than 30 min, and/or a period of post-traumatic amnesia lasting for less than 24 h; moderate-severe TBI was defined as loss of consciousness lasting for at least 30 min, and/or a period of post-traumatic amnesia lasting for at least 24 h (23).
CSF Biomarker Measurement
CSF samples in this project were analyzed using the ADNI methods to measure the levels of Aβ42, Aβ40, and phosphorylated tau at threonine 181 (p-tau) on a fully automated cobas e601 analyzer (Roche Diagnostics). We especially focused on CSF Aβ42/40 ratio and CSF p-tau, as they were generally thought to reflect Aβ pathology (24) and tau pathology (25).
Psychiatric and Cognitive Assessment
Lifetime PTSD was recorded using the clinician-administered PTSD scale (CAPS) within DSM-IV (CAPS score > 40). Geriatric depression scale (GDS) test was used to measure depression in older adults. Mini-Mental Status Examination (MMSE) test was used to reflect general cognitive performance. Cognitive tests recommended to assess TBI-related cognitive impairment (26) were selected including Trail Making Test Part A (processing speed), Trail Making Test Part B (executive functioning), Rey Auditory Verbal Learning Test (RAVLT) total score of trials 1–5 (learning), and RAVLT total delayed recall and recognition total (verbal memory). Raw scores of all these cognitive tests were standardized to z-scores for each participant. The average z-scores of RAVLT total delayed recall score and recognition total score were calculated to reflect verbal memory. Higher z-scores indicated poorer performance on the processing speed and executive functioning measures, and lower z-scores indicated poorer performance on verbal learning and verbal memory.
MRI Data Acquisition and Analysis
All MR examinations were performed according to the ADNI MRI scanning method (GE or Siemens), which included axial T1-weighted, T2-weighted, fluid-attenuated inversion recovery (FLAIR), and T2-star gradient-echo weighted sequences (Supplementary Table 1). Two trained neuroradiologists (M-LW and W-BL with 10 and 24 years of experience, respectively) who were blinded to the clinical and CSF data, assessed PVS, white matter hyperintensities (WMH), and cerebral microbleeds (CMBs) according to the Standards for Reporting Vascular Changes on Neuroimaging (27).
Perivascular spaces was assessed by comprehensively reading the T1-weighted, T2-weighted, and fluid-attenuated inversion recovery (FLAIR) sequence and counted specially on T2-weighted sequence. PVS were characterized by locating along the penetrating arteries and shown as low signals on T1-weighted and FLAIR images and high signal on T2-weighted images (Figure 2). PVS was rated in the basal ganglia (BG) and the centrum semiovale (CSO). CSO was defined as white matter superior to the lateral ventricles in each of cerebral hemispheres. The largest number of PVS was documented on one slice of one side of the brain. A validated visual rating scale was also used to assess MRI-visible PVS (0 = no PVS, 1 = 1–10 PVS, 2 = 11–20 PVS, 3 = 21–40 PVS, and 4 = 41 or more PVS) (12, 28). The PVS number and grade were both assessed for analyses.
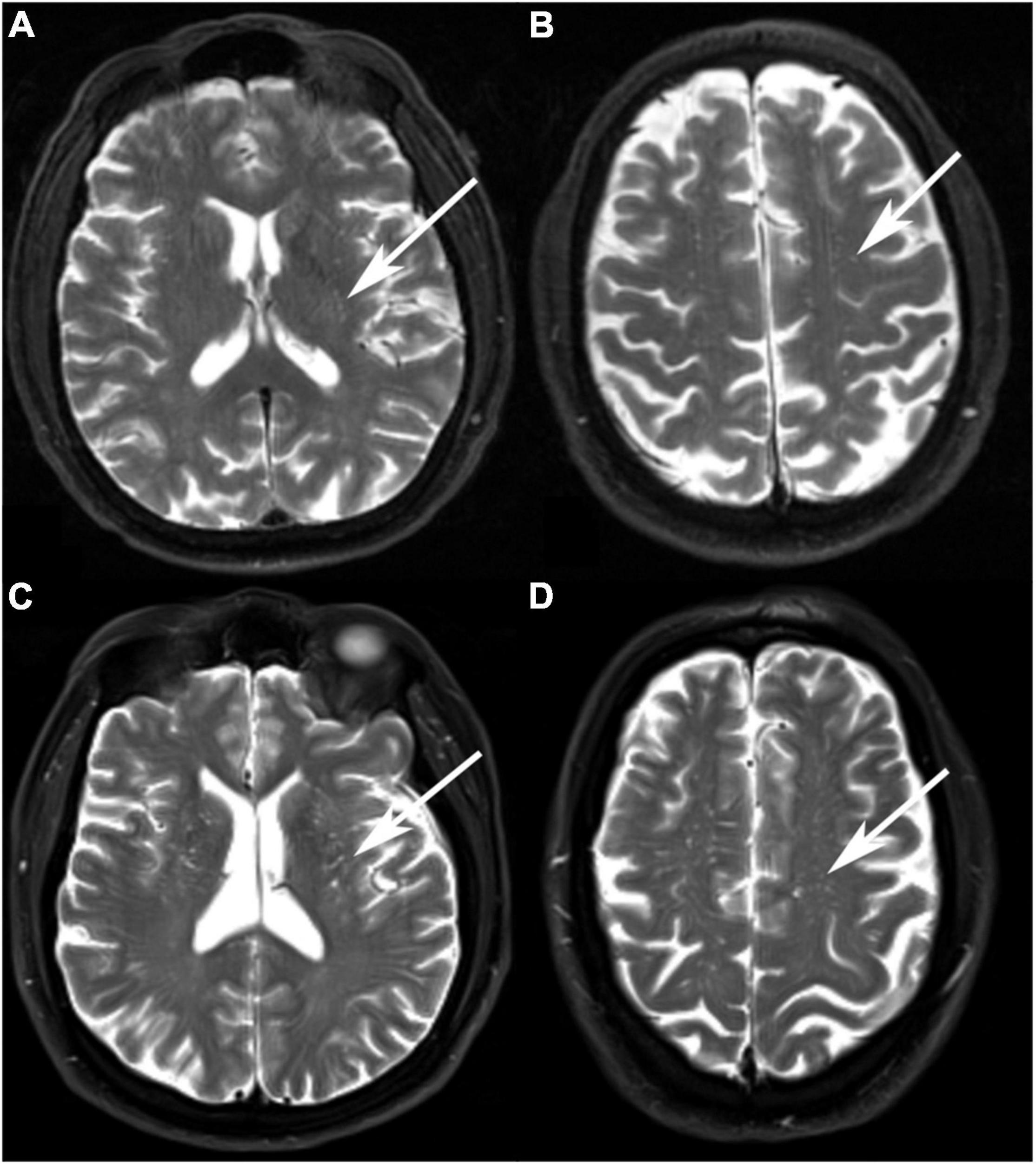
Figure 2. Examples of MRI-visible perivascular spaces. (A,B) A 68-year-old Vietnam war veteran without a history of traumatic brain injury (TBI) had a low degree of BG-PVS and CSO-PVS. (C,D) A 67-year-old Vietnam War veteran with a history of TBI had a high degree of BG-PVS and CSO-PVS.
White matter hyperintensities was evaluated in the periventricular region (PWMH) and deep white matter region (DWMH) according to Fazekas rating scale (29). Severe WMH was defined as having a score > 1 of the Fazekas scale. The total WMH volume was also extracted from the database, and a ln-transform was applied over the data to generate normal distribution data. CMBs, characterized as low signal on the T2 star sequence (30), were visually counted and rated as 0 = microbleeds absent and 1 = microbleeds present.
Statistical Analyses
Categorical variables were expressed as frequency (percentage) and continuous variables were expressed as mean (standard deviation). The demographics were compared using the chi-square test for qualitative variables and the t-test for quantitative variables, as appropriate between TBI and military control group. Analyses of covariance (ANCOVA) was done adjusting for (1) age and APOE ε4 status to examine group differences in CSF biomarkers; (2) age, education, and APOE ε4 status to examine group differences in cognitive performance; (3) age, education, APOE ε4 status, MR scanners (General Electric or Simens), CMBs and lnWMH to examine group differences in MRI-visible PVS number. MRI-visible PVS grade was also analyzed using chi-square test.
Multiple linear regression analyses were applied to estimate the association between MRI-visible PVS and cognitive impairment. The cognitive tests scores were taken as outcome variables with MRI-visible PVS as the predictor. We constructed five models to adjust potential confounding factors: Model 1 was unadjusted; model 2 was adjusted for age, education and APOE ε4 status; model 3 was adjusted for CMBs and lnWMH plus the variables in model 2; model 4 was adjusted for CSF biomarkers plus the variables in model 3. All the analyses were done in TBI group subjects and military control subjects respectively, and the interaction effect of TBI status was explored. Furthermore, as increased PVS may indicate the glymphatic system impairment, which would slow the clearance of brain waste like tau and Aβ and finally cause the occurrence of cognitive impairment, mediation analyses were done to investigate whether CSF biomarkers mediated the association between PVS and cognitive impairment using the SPSS PROCESS module (31, 32). As there were four cognitive scales analyzed in this study, Bonferroni corrected p-value was set for 0.05/4 = 0.0125 for the analyses of cognitive scale. P < 0.05 was considered as significant for other statistical analyses. All statistical tests were performed by IBM SPSS Statistics for Windows, version 20.0.
Results
Demographics and Neuropsychological Function of the Study Population
A total of 55 Vietnam War veterans with a history of TBI (age 68.8 ± 3.8 years, all male) and 52 non-TBI Vietnam War veterans (age 68.3 ± 4.4 years, all male) were included in this study. Compared with military control subjects, TBI group had poorer performance in RAVLT verbal memory composite score (p = 0.022) after adjusting for age, education and APOE ε4 carrier status (Table 1). There were no statistical differences in demographics and other neuropsychological tests between military control group and TBI group (p > 0.05). Supplementary Table 2 shows the injury characteristics of TBI subjects.
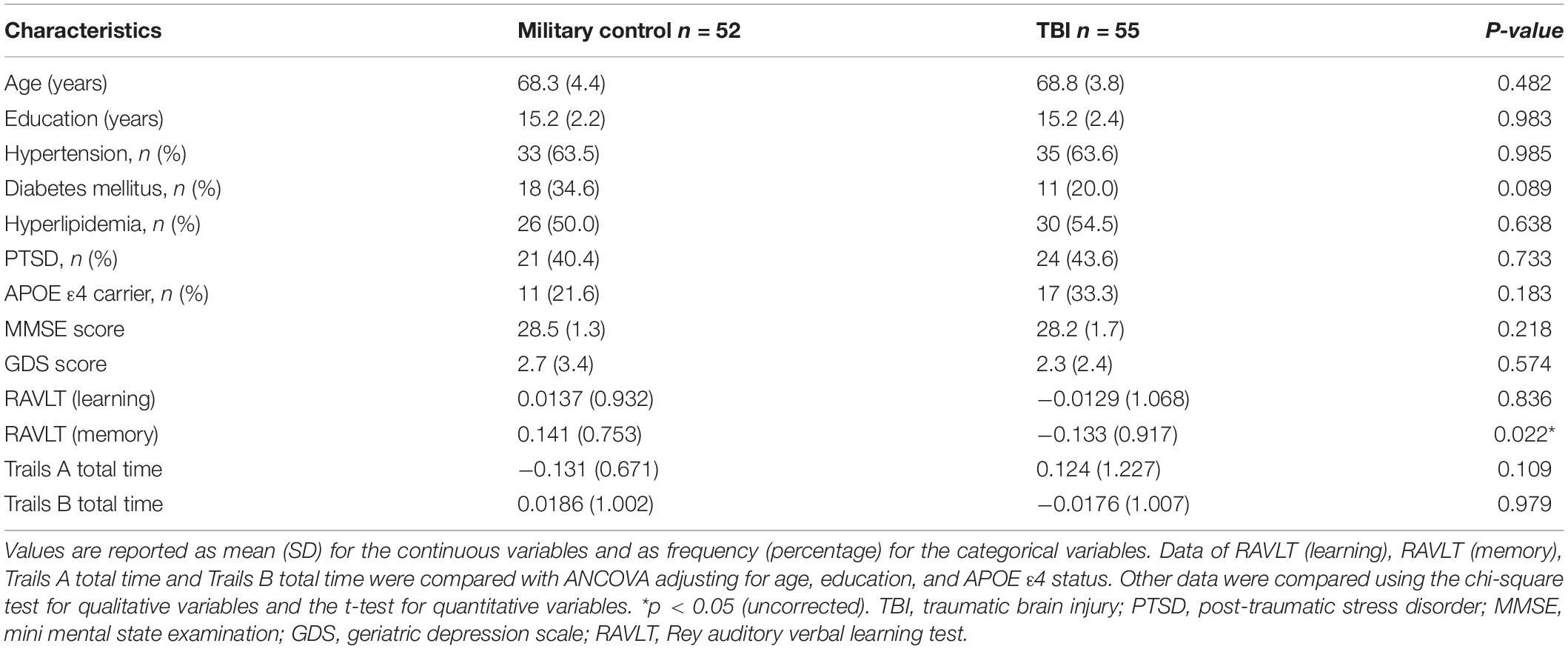
Table 1. Demographics and neuropsychological tests in Vietnam War veterans with and without a history of traumatic brain injury (TBI).
Comparisons of MRI Findings and CSF Biomarkers Between Military Control and Traumatic Brain Injury Group
There were 15 Siemens data (four in military control group and 11 in TBI group) and 92 GE data (48 in military control group and 44 in TBI group) used in our study. The inter-rater reliability was excellent for the CSO-PVS number [intraclass correlation coefficient (ICC) = 0.89, 95% CI: 0.84–0.92], BG-PVS number (ICC = 0.86, 95% CI: 0.81–0.90), CMBs (κ = 0.93, 95% CI: 0.88–0.97), PWMH (κ = 0.86, 95% CI: 0.84–0.90), and DWMH (κ = 0.88,95% CI: 0.83–0.92). The intrarater reliability was determined from a random sample of 50 participants with 1 month interval between the first and second image assessments evaluated by one neuroradiologist. The intra-rater reliability was also excellent for the CSO-PVS number (ICC = 0.92, 95% CI: 0.86–0.95), BG-PVS number (ICC = 0.88, 95% CI: 0.80–0.93), CMBs (κ = 0.96, 95%CI: 0.91–1.00), PWMH (κ = 0.92, 95% CI: 0.87–0.97), and DWMH (κ = 0.91,95% CI: 0.86–0.96). The PVS rating scores of the senior radiologist was used for the final analysis.
Table 2 shows the comparison of MRI findings and CSF biomarkers between military control group and TBI group. Compared with military control group, TBI group had more CSO-PVS both in number (p = 0.001) after adjusting age, education, APOE ε4 status, CMBs and WMH and grade (p = 0.001). The TBI group also had higher levels of CSF p-tau after adjusting for age and APOE ε4 status (p = 0.022). There was no statistical difference in BG-PVS, WMH, and CSF Aβ42/40 (p > 0.05).
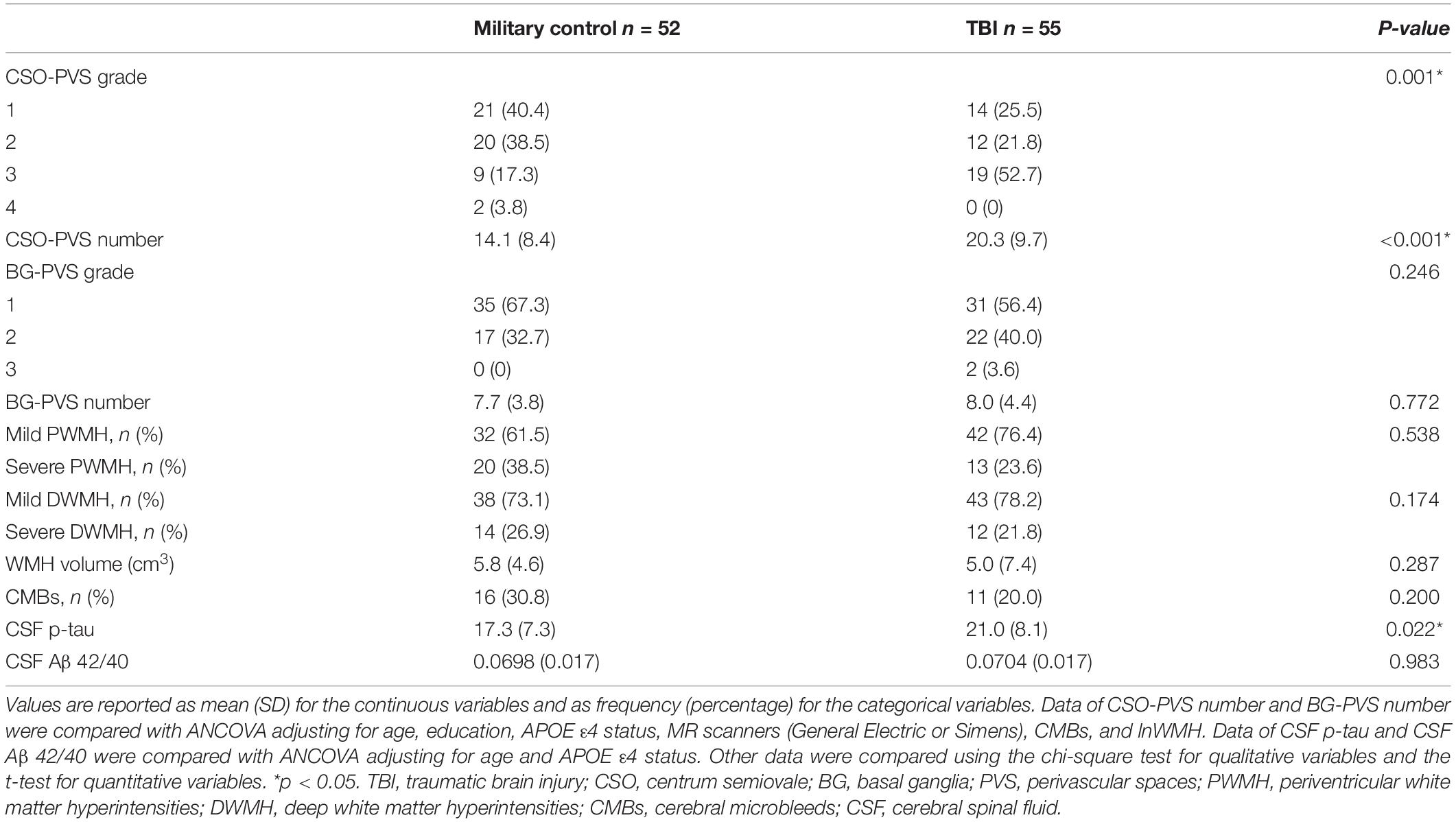
Table 2. MRI findings and CSF markers in Vietnam War veterans with and without a history of traumatic brain injury (TBI).
Multiple Regression Analysis Between MRI-Visible PVS and CSF Biomarkers and Cognitive Impairment
In multiple regression analysis, high CSO-PVS number was associated with poor RAVLT memory even after adjusting for age, education, apo ε4, CMBs, lnWMH and CSF biomarkers in TBI group (β = −0.039, 95% CI −0.062, −0.016), but not in military control group (β = 0.019, 95% CI −0.004, 0.043) (p-interaction = 0.003). There was tendency of association between high CSO-PVS number and poor RAVLT verbal learning in TBI group (β = −0.037, 95% CI −0.066, −0.007), but not in military control group (β = 0.015, 95% CI −0.020, 0.050) (Table 3). No significant associations were found between CSO-PVS with TMA and TMB test results (p > 0.05).
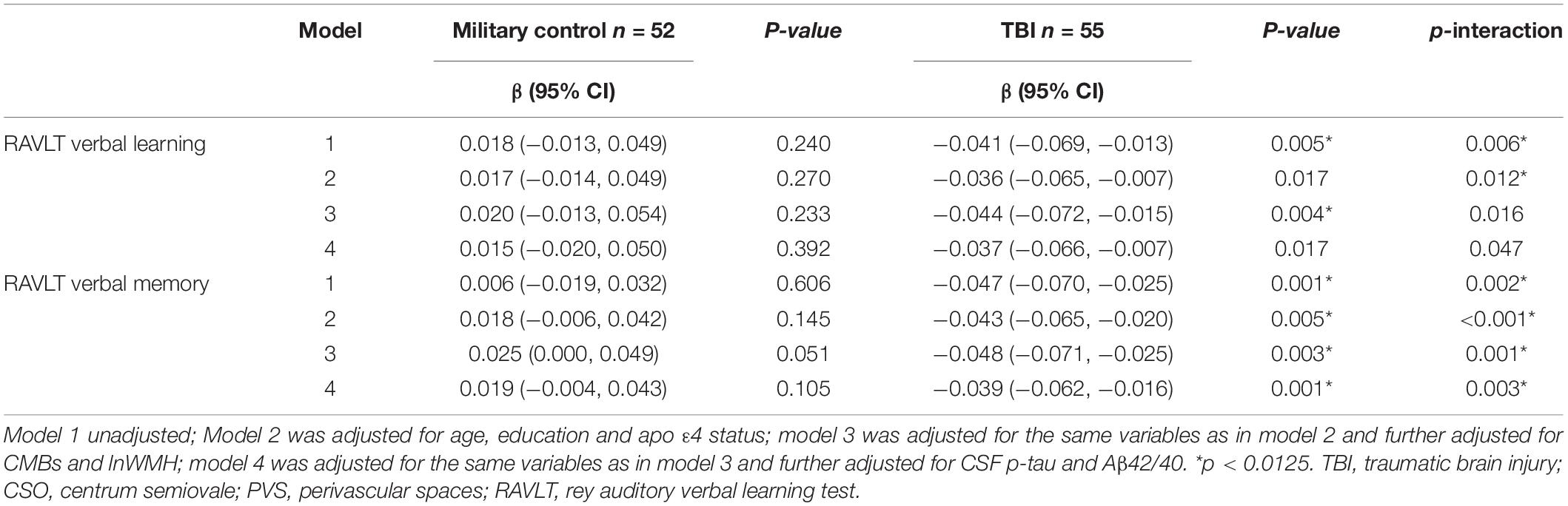
Table 3. Multiple linear regression of CSO-PVS and cognitive test results: overall and stratified to traumatic brain injury (TBI) history.
Further mediation analysis revealed that CSF p-tau had a significant indirect effect (β = −0.009, 95% CI: −0.022 −0.001, p = 0.001) and mediated 18.75% effect on the relationship between CSO-PVS and verbal memory in TBI group after adjusting for age, education, apo ε4 status, CMBs, and lnWMH (Figure 3).
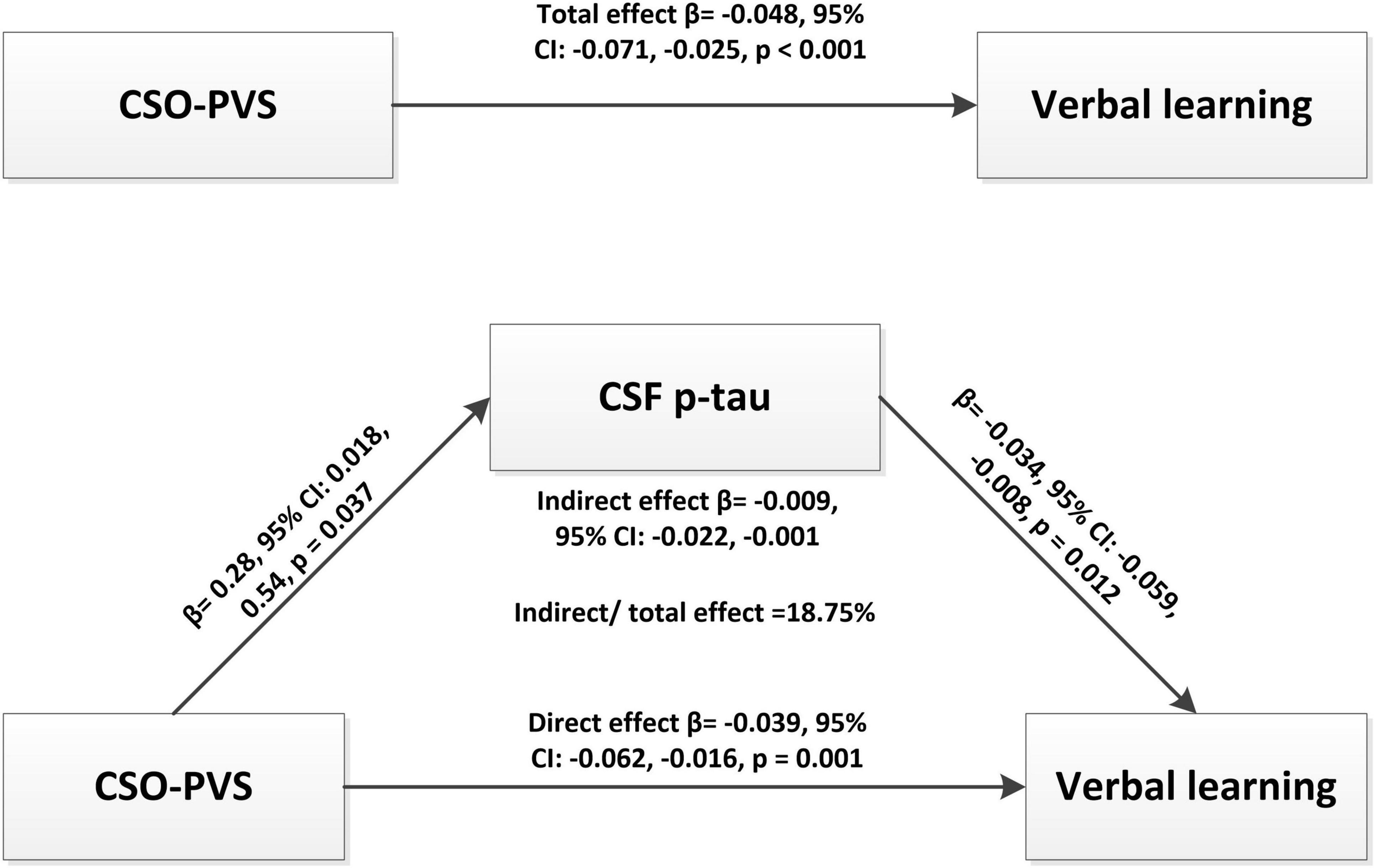
Figure 3. Mediation analysis revealed that CSF p-tau had a significant indirect effect (β = -0.009, 95% CI: -0.022 -0.001, p = 0.001) and mediated 18.75% effect for the relationship between CSO-PVS and verbal memory in traumatic brain injury (TBI) group after adjusting for age, education, apo e4, CMBs, and lnWMH.
Discussion
In our study, we aimed to investigate the distribution characteristics of MRI-visible PVS and its association with cognitive impairment in military veterans with TBI, and whether CSF biomarkers mediate this effect. Our study revealed that CSO-PVS was more common in Vietnam War veterans with a history of TBI and was associated with poor verbal memory, mediated partially by CSF p-tau. Our study suggested that MRI-visible CSO-PVS could be a promising indicator for cognitive impairment and the CSF p-tau may be the missing link between MRI-visible CSO-PVS and cognitive impairment in military veterans with TBI.
With the wide use of MRI, PVS is frequently detected in clinical practice. MRI-visible PVS has been reported to occur in 100% normal elderly (33–36) and several studies found an increased number of MRI-visible PVS in TBI (19–21). A previous small study revealed that TBI subjects had an asymmetric distribution of MRI-visible PVS, but no higher MRI-visible PVS number compared with healthy controls (37). Compared with military control subjects, Vietnam War veterans with a history of TBI had increased PVS only in CSO region but not in the BG region in our study. Our study suggested that CSO-PVS may be more easily affected by TBI than BG-PVS. The underlying pathophysiological mechanism of MRI-visible PVS in TBI was poorly investigated. One possible explanation was that the cortical surface, and cerebral white matter were commonly affected areas by the TBI-related shear-strain injury, which could stretch perivascular space and finally lead to MRI-visible PVS.
Interestingly, high MRI-visible CSO-PVS number was associated with poor verbal memory in TBI group but not military control group after adjusting for clinical characteristics and other neuroimaging markers. Previous studies have revealed associations of MRI-visible CSO-PVS with Alzheimer’s disease (38) and longitudinal cognitive impairment (39). Our study suggested that there may be an independent mechanism for the association between MRI-visible CSO-PVS and verbal memory in veterans with TBI, possibly by impaired glymphatic system. A recent study revealed associations of PVS in the posterior cingulate, fusiform, and postcentral with somatization symptoms in mTBI (40). Furthermore, TBI and poor sleep had an enhanced interaction effect on PVS indicating a possible role of the glymphatic system (41, 42). However, these mechanisms may be weak in subjects without TBI. A recent study also found no association between MRI-visible CSO-PVS and cognitive tests in cognitively unimpaired (43). Our study demonstrated that MRI-visible CSO-PVS could be a promising imaging biomarker for verbal memory impairment in veterans with TBI.
In our study, higher CSF p-tau was found in military veterans with TBI and CSF p-tau mediated partially the relationship between MRI-visible CSO-PVS and verbal memory. A recent study using the same database also revealed higher CSF p-tau in veterans with TBI than military control (44). The higher CSF p-tau might partially reflect the insufficient function of brain glymphatic system. A previous animal study suggested chronic impairment of brain glymphatic system and tau aggregation after TBI (18). The excessive tau protein might stack in the PVS causing the enlargement of PVS which would be visible on MRI, and the malfunction of PVS would slow the clearance of brain waste, suggesting a feed-forward mechanism. The heavy tau burden would further cause damage to the brain neurons and finally lead to cognitive impairment. A recent study also revealed blood p-tau was associated with cognitive impairment in veterans with a history of TBI (45). Therefore, CSF p-tau may be the missing link between MRI-visible CSO-PVS and cognitive impairment. However, a recent study about mild cognitive impairment found no association between PVS volume fraction and CSF p-tau (46). We speculated that tau pathology may be more prominent in cognitive impairment with TBI. Glymphatic failure has been suggested as a final common pathway to dementia and may constitute a therapeutically targetable pathway (47). Our findings add to the evidence linking MRI-visible PVS to cognitive impairment through possible glymphatic system in veterans with TBI.
Our study had several limitations. First, the number of subjects with a history of TBI was small (n = 55). Studies using large sample are needed to replicate our results. Second, due to the cross-sectional nature of this study, the exact order of causal effects between MRI-visible CSO-PVS, CSF p-tau and cognitive impairment cannot be determined. Further longitudinal studies are required to better assess the causal effects. Third, the MRI scanners used in this study were from two different vendors (GE, or Siemens). However, the MRI scanning protocol and parameters were almost identical. To reduce the influence of scanners in the detectability of PVS, we also took MRI scanners as a covariate into the ANCOVA analysis of PVS. Fourth, the detectability of PVS on MRI does not necessarily mean that they are the enlargement of perivascular spaces. Future correlational study with the histopathological changes in autopsy study or animal study is needed to figure out the comprehensive mechanism of MRI-visible CSO-PVS in TBI.
Furthermore, we only used visual rating scales to evaluate PVS, which was observer-dependent. There have been many semi-automatic or automatic methods to quantify PVS (48–55). However, these methods also had limitations and restrictions like 3D MRI sequence in 7T MRI (51, 53, 54). Imperfection of the brain parcellation could affect automated quantification (48). Closely aligned multimodal data sets is needed and there is uncertainty in volume estimates resulting from partial volume effects present in radiologic methods (49). Although called “automatic,” these methods still require some manual intervention (50), and visual checking and editing is likely to be needed in complex cases (52). As these methods were still not applied in clinical practice, we only used visual rating measurement. The inter-rater and intra-rater reliability for the rating of PVS was excellent in our study and could be applied easily in practical clinical practice.
In conclusion, our study found that MRI-visible CSO-PVS was more common in Vietnam War veterans with a history of TBI and was associated with poor verbal memory, mediated partially by CSF p-tau. Our study suggested that MRI-visible CSO-PVS could be a promising indicator for cognitive decline and the CSF p-tau may be the missing link between MRI-visible CSO-PVS and cognitive impairment in military veterans with TBI.
Data Availability Statement
The datasets presented in this study can be found in online repositories. The names of the repository/repositories and accession number(s) can be found below: http://adni.loni.usc.edu/.
Ethics Statement
The studies involving human participants were reviewed and approved by the Institutional Review Boards of all participating ADNI sites. The patients/participants provided their written informed consent to participate in this study.
Author Contributions
M-LW and Y-HL: study concept and design. M-LW, D-XY, and ZS: analysis and interpretation of data and drafting of the manuscript. M-LW, D-XY, Q-QZ, P-YL, XW, and W-BL: statistical analysis and critical revision of the manuscript. Y-HL: administrative, technical and material support, and study supervision. All authors gave final approval of the version published.
Funding
This study was supported by National Natural Science Foundation of China (81901727), Shanghai Sixth People’s Hospital (ynts202109), New developing and Frontier Technologies of Shanghai Shen Kang Hospital Development Center (SHDC12018117), Shanghai Municipal Education Commission-Gaofeng Clinical Medicine Grant Support (2016427), Excellent discipline leader of Shanghai Municipal Planning Commission (2017BR041), and Shanghai key discipline of medical imaging (2017ZZ02005).
Conflict of Interest
The authors declare that the research was conducted in the absence of any commercial or financial relationships that could be construed as a potential conflict of interest.
Publisher’s Note
All claims expressed in this article are solely those of the authors and do not necessarily represent those of their affiliated organizations, or those of the publisher, the editors and the reviewers. Any product that may be evaluated in this article, or claim that may be made by its manufacturer, is not guaranteed or endorsed by the publisher.
Acknowledgments
Data collection and sharing for this project was funded by the Alzheimer’s Disease Neuroimaging Initiative (ADNI) (National Institutes of Health Grant U01 AG024904) and DOD ADNI (Department of Defense award number W81XWH-12-2-0012). ADNI is funded by the National Institute on Aging, the National Institute of Biomedical Imaging and Bioengineering, and through generous contributions from the following: AbbVie, Alzheimer’s Association; Alzheimer’s Drug Discovery Foundation; Araclon Biotech; BioClinica, Inc.; Biogen; Bristol-Myers Squibb Company; CereSpir, Inc.; Cogstate; Eisai Inc.; Elan Pharmaceuticals, Inc.; Eli Lilly and Company; EuroImmun; F. Hoffmann-La Roche Ltd., and its affiliated company Genentech, Inc.; Fujirebio; GE Healthcare; IXICO Ltd.; Janssen Alzheimer Immunotherapy Research & Development, LLC.; Johnson & Johnson Pharmaceutical Research & Development LLC.; Lumosity; Lundbeck; Merck & Co., Inc.; Meso Scale Diagnostics, LLC.; NeuroRx Research; Neurotrack Technologies; Novartis Pharmaceuticals Corporation; Pfizer Inc.; Piramal Imaging; Servier; Takeda Pharmaceutical Company; and Transition Therapeutics. The Canadian Institutes of Health Research is providing funds to support ADNI clinical sites in Canada. Private sector contributions are facilitated by the Foundation for the National Institutes of Health (www.fnih.org). The grantee organization is the Northern California Institute for Research and Education, and the study is coordinated by the Alzheimer’s Therapeutic Research Institute at the University of Southern California. ADNI data are disseminated by the Laboratory for Neuro Imaging at the University of Southern California.
Supplementary Material
The Supplementary Material for this article can be found online at: https://www.frontiersin.org/articles/10.3389/fpsyt.2022.921203/full#supplementary-material
Footnotes
References
1. Dewan MC, Rattani A, Gupta S, Baticulon RE, Hung Y-C, Punchak M, et al. Estimating the global incidence of traumatic brain injury. J Neurosurg. (2018) 130:1080–97.
2. Langlois JA, Rutland-Brown W, Wald MM. The epidemiology and impact of traumatic brain injury: a brief overview. J Head Trauma Rehabil. (2006) 21:375–8.
4. Kaup AR, Peltz C, Kenney K, Kramer JH, Diaz-Arrastia R, Yaffe K. Neuropsychological profile of lifetime traumatic brain injury in older veterans. J Int Neuropsychol Soc. (2017) 23:56–64. doi: 10.1017/S1355617716000849
5. Lippa SM, French LM, Bell RS, Brickell TA, Lange RT. United States military service members demonstrate substantial and heterogeneous long-term neuropsychological dysfunction after moderate, severe, and penetrating traumatic brain injury. J Neurotrauma. (2020) 37:608–17. doi: 10.1089/neu.2019.6696
6. Barnes DE, Byers AL, Gardner RC, Seal KH, Boscardin WJ, Yaffe K. Association of mild traumatic brain injury with and without loss of consciousness with dementia in US military veterans. JAMA Neurol. (2018) 75:1055–61. doi: 10.1001/jamaneurol.2018.0815
7. McKee AC, Robinson ME. Military-related traumatic brain injury and neurodegeneration. Alzheimers Dementia. (2014) 10(3 Suppl.):S242–53.
8. Mohamed AZ, Cumming P, Srour H, Gunasena T, Uchida A, Haller CN, et al. Amyloid pathology fingerprint differentiates post-traumatic stress disorder and traumatic brain injury. Neuroimage Clin. (2018) 19:716–26.
9. Mohamed AZ, Cumming P, Götz J, Nasrallah F. Tauopathy in veterans with long-term posttraumatic stress disorder and traumatic brain injury. Eur J Nucl Med Mol Imaging. (2019) 46:1139–51.
10. Wardlaw JM, Benveniste H, Nedergaard M, Zlokovic BV, Mestre H, Lee H, et al. Perivascular spaces in the brain: anatomy, physiology and pathology. Nat Rev Neurol. (2020) 16:137–53.
12. Doubal FN, MacLullich AMJ, Ferguson KJ, Dennis MS, Wardlaw JM. Enlarged perivascular spaces on MRI are a feature of cerebral small vessel disease. Stroke. (2010) 41:450–4.
13. Iliff JJ, Wang M, Liao Y, Plogg BA, Peng W, Gundersen GA, et al. A paravascular pathway facilitates CSF flow through the brain parenchyma and the clearance of interstitial solutes, including amyloid β. Sci Transl Med. (2012) 4:147ra11. doi: 10.1126/scitranslmed.3003748
14. Rasmussen MK, Mestre H, Nedergaard M. The glymphatic pathway in neurological disorders. Lancet Neurol. (2018) 17:1016–24.
15. Venkat P, Chopp M, Zacharek A, Cui C, Zhang L, Li Q, et al. White matter damage and glymphatic dysfunction in a model of vascular dementia in rats with no prior vascular pathologies. Neurobiol Aging. (2017) 50:96–106. doi: 10.1016/j.neurobiolaging.2016.11.002
16. Vilor-Tejedor N, Ciampa I, Operto G, Falcón C, Suárez-Calvet M, Crous-Bou M, et al. Perivascular spaces are associated with tau pathophysiology and synaptic dysfunction in early Alzheimer’s continuum. Alzheimers Res Ther. (2021) 13:135. doi: 10.1186/s13195-021-00878-5
17. Park YW, Shin N-Y, Chung SJ, Kim J, Lim SM, Lee PH, et al. Magnetic resonance imaging-visible perivascular spaces in basal ganglia predict cognitive decline in Parkinson’s disease. Mov Disord. (2019) 34:1672–9. doi: 10.1002/mds.27798
18. Iliff JJ, Chen MJ, Plog BA, Zeppenfeld DM, Soltero M, Yang L, et al. Impairment of glymphatic pathway function promotes tau pathology after traumatic brain injury. J Neurosci. (2014) 34:16180–93. doi: 10.1523/JNEUROSCI.3020-14.2014
19. Inglese M, Bomsztyk E, Gonen O, Mannon LJ, Grossman RI, Rusinek H. Dilated perivascular spaces: hallmarks of mild traumatic brain injury. AJNR Am J Neuroradiol. (2005) 26:719–24.
20. Inglese M, Grossman RI, Diller L, Babb JS, Gonen O, Silver JMA, et al. Clinical significance of dilated Virchow-Robin spaces in mild traumatic brain injury. Brain Inj. (2006) 20:15–21. doi: 10.1080/02699050500309593
21. Orrison WW, Hanson EH, Alamo T, Watson D, Sharma M, Perkins TG, et al. Traumatic brain injury: a review and high-field MRI findings in 100 unarmed combatants using a literature-based checklist approach. J Neurotrauma. (2009) 26:689–701. doi: 10.1089/neu.2008.0636
22. Mueller SG, Weiner MW, Thal LJ, Petersen RC, Jack CR, Jagust W, et al. Ways toward an early diagnosis in Alzheimer’s disease: the Alzheimer’s disease neuroimaging initiative (ADNI). Alzheimers Dementia. (2005) 1:55–66. doi: 10.1016/j.jalz.2005.06.003
23. Malec JF, Brown AW, Leibson CL, Flaada JT, Mandrekar JN, Diehl NN, et al. The mayo classification system for traumatic brain injury severity. J Neurotrauma. (2007) 24:1417–24.
24. Hansson O, Lehmann S, Otto M, Zetterberg H, Lewczuk P. Advantages and disadvantages of the use of the CSF Amyloid β (Aβ) 42/40 ratio in the diagnosis of Alzheimer’s disease. Alzheimers Res Ther. (2019) 11:34. doi: 10.1186/s13195-019-0485-0
25. Jack CR, Bennett DA, Blennow K, Carrillo MC, Dunn B, Haeberlein SB, et al. NIA-AA research framework: toward a biological definition of Alzheimer’s disease. Alzheimers Dement. (2018) 14:535–62. doi: 10.1016/j.jalz.2018.02.018
26. Hicks R, Giacino J, Harrison-Felix C, Manley G, Valadka A, Wilde EA. Progress in developing common data elements for traumatic brain injury research: version two–the end of the beginning. J Neurotrauma. (2013) 30:1852–61. doi: 10.1089/neu.2013.2938
27. Wardlaw JM, Smith EE, Biessels GJ, Cordonnier C, Fazekas F, Frayne R, et al. Neuroimaging standards for research into small vessel disease and its contribution to ageing and neurodegeneration. Lancet Neurol. (2013) 12:822–38.
28. Maclullich AMJ, Wardlaw JM, Ferguson KJ, Starr JM, Seckl JR, Deary IJ. Enlarged perivascular spaces are associated with cognitive function in healthy elderly men. J Neurol Neurosurg Psychiatry. (2004) 75:1519–23.
29. Fazekas F, Chawluk JB, Alavi A, Hurtig HI, Zimmerman RA. MR signal abnormalities at 1.5 T in Alzheimer’s dementia and normal aging. AJR Am J Roentgenol. (1987) 149:351–6. doi: 10.2214/ajr.149.2.351
30. Cordonnier C, Potter GM, Jackson CA, Doubal F, Keir S, Sudlow CLM, et al. improving interrater agreement about brain microbleeds: development of the brain observer microbleed scale (BOMBS). Stroke. (2009) 40:94–9. doi: 10.1161/STROKEAHA.108.526996
31. Hayes AF. Introduction to Mediation, Moderation, and Conditional Process Analysis: A Regression-Based Approach. New York, NY: Guilford Press (2013).
32. Gong L, Xu R, Liu D, Lan L, Zhang B, Zhang C. The specific impact of apolipoprotein E epsilon 2 on cognition and brain function in cognitively normal elders and mild cognitive impairment patients. Front Aging Neurosci. (2020) 11:374. doi: 10.3389/fnagi.2019.00374
33. Zhu YC, Dufouil C, Mazoyer B, Soumaré A, Ricolfi F, Tzourio C, et al. Frequency and location of dilated Virchow-Robin spaces in elderly people: a population-based 3D MR imaging study. AJNR Am J Neuroradiol. (2011) 32:709–13. doi: 10.3174/ajnr.A2366
34. Yakushiji Y, Charidimou A, Hara M, Noguchi T, Nishihara M, Eriguchi M, et al. Topography and associations of perivascular spaces in healthy adults: the Kashima scan study. Neurology. (2014) 83:2116–23. doi: 10.1212/WNL.0000000000001054
35. Piantino J, Boespflug EL, Schwartz DL, Luther M, Morales AM, Lin A, et al. Characterization of MR imaging-visible perivascular spaces in the white matter of healthy adolescents at 3T. AJNR Am J Neuroradiol. (2020) 41:2139–45. doi: 10.3174/ajnr.A6789
36. Barisano G, Sheikh-Bahaei N, Law M, Toga AW, Sepehrband F. Body mass index, time of day and genetics affect perivascular spaces in the white matter. J Cereb Blood Flow Metab. (2021) 41:1563–78. doi: 10.1177/0271678X20972856
37. Duncan D, Barisano G, Cabeen R, Sepehrband F, Garner R, Braimah A, et al. Analytic tools for post-traumatic epileptogenesis biomarker search in multimodal dataset of an animal model and human patients. Front Neuroinform. (2018) 12:86. doi: 10.3389/fninf.2018.00086
38. Banerjee G, Kim HJ, Fox Z, Jäger HR, Wilson D, Charidimou A, et al. MRI-visible perivascular space location is associated with Alzheimer’s disease independently of amyloid burden. Brain. (2017) 140:1107–16. doi: 10.1093/brain/awx003
39. Paradise M, Crawford JD, Lam BCP, Wen W, Kochan NA, Makkar S, et al. Association of dilated perivascular spaces with cognitive decline and incident dementia. Neurology. (2021) 96:e1501–11.
40. Sibilia F, Custer RM, Irimia A, Sepehrband F, Toga AW, Cabeen RP. Life after mild traumatic brain injury: widespread structural brain changes associated with psychological distress revealed with multimodal magnetic resonance imaging. Biol Psychiatry Glob Open Sci. (2022). doi: 10.1016/j.bpsgos.2022.03.004
41. Opel RA, Christy A, Boespflug EL, Weymann KB, Case B, Pollock JM, et al. Effects of traumatic brain injury on sleep and enlarged perivascular spaces. J Cereb Blood Flow Metab. (2019) 39:2258–67. doi: 10.1177/0271678X18791632
42. Piantino J, Schwartz DL, Luther M, Newgard C, Silbert L, Raskind M, et al. Link between mild traumatic brain injury, poor sleep, and magnetic resonance imaging: visible perivascular spaces in veterans. J Neurotrauma. (2021) 38:2391–9. doi: 10.1089/neu.2020.7447
43. Gertje EC, van Westen D, Panizo C, Mattsson-Carlgren N, Hansson O. Association of enlarged perivascular spaces and measures of small vessel and Alzheimer disease. Neurology. (2021) 96:e193–202.
44. Clark AL, Weigand AJ, Bangen KJ, Thomas KR, Eglit GML, Bondi MW, et al. Higher cerebrospinal fluid tau is associated with history of traumatic brain injury and reduced processing speed in Vietnam-era veterans: a department of defense Alzheimer’s disease neuroimaging initiative (DOD-ADNI) study. Alzheimers Dement (Amst). (2021) 13:e12239. doi: 10.1002/dad2.12239
45. Peltz CB, Kenney K, Gill J, Diaz-Arrastia R, Gardner RC, Yaffe K. Blood biomarkers of traumatic brain injury and cognitive impairment in older veterans. Neurology. (2020) 95:e1126–33.
46. Sepehrband F, Barisano G, Sheikh-Bahaei N, Choupan J, Cabeen RP, Lynch KM, et al. Volumetric distribution of perivascular space in relation to mild cognitive impairment. Neurobiol Aging. (2021) 99:28–43. doi: 10.1016/j.neurobiolaging.2020.12.010
47. Nedergaard M, Goldman SA. Glymphatic failure as a final common pathway to dementia. Science. (2020) 370:50–6. doi: 10.1126/science.abb8739
48. Sepehrband F, Barisano G, Sheikh-Bahaei N, Cabeen RP, Choupan J, Law M, et al. Image processing approaches to enhance perivascular space visibility and quantification using MRI. Sci Rep. (2019) 9:12351. doi: 10.1038/s41598-019-48910-x
49. Boespflug EL, Schwartz DL, Lahna D, Pollock J, Iliff JJ, Kaye JA, et al. MR imaging-based multimodal autoidentification of perivascular spaces (mMAPS): automated morphologic segmentation of enlarged perivascular spaces at clinical field strength. Radiology. (2018) 286:632–42. doi: 10.1148/radiol.2017170205
50. Wang X, Valdés Hernández MDC, Doubal F, Chappell FM, Piper RJ, Deary IJ, et al. Development and initial evaluation of a semi-automatic approach to assess perivascular spaces on conventional magnetic resonance images. J Neurosci Methods. (2016) 257:34–44. doi: 10.1016/j.jneumeth.2015.09.010
51. Hou Y, Park SH, Wang Q, Zhang J, Zong X, Lin W, et al. Enhancement of perivascular spaces in 7 T MR image using Haar transform of non-local cubes and block-matching filtering. Sci Rep. (2017) 7:8569. doi: 10.1038/s41598-017-09336-5
52. Ballerini L, Lovreglio R, Valdés Hernández MDC, Ramirez J, MacIntosh BJ, Black SE, et al. Perivascular spaces segmentation in brain MRI using optimal 3D filtering. Sci Rep. (2018) 8:2132. doi: 10.1038/s41598-018-19781-5
53. Zong X, Park SH, Shen D, Lin W. Visualization of perivascular spaces in the human brain at 7T: sequence optimization and morphology characterization. Neuroimage. (2016) 125:895–902. doi: 10.1016/j.neuroimage.2015.10.078
54. Park SH, Zong X, Gao Y, Lin W, Shen D. Segmentation of perivascular spaces in 7T MR image using auto-context model with orientation-normalized features. Neuroimage. (2016) 134:223–35. doi: 10.1016/j.neuroimage.2016.03.076
Keywords: traumatic brain injury, MRI, CSF, tau, Aβ, MRI-visible perivascular spaces
Citation: Wang M-L, Yang D-X, Sun Z, Li W-B, Zou Q-Q, Li P-Y, Wu X and Li Y-H (2022) MRI-Visible Perivascular Spaces Associated With Cognitive Impairment in Military Veterans With Traumatic Brain Injury Mediated by CSF P-Tau. Front. Psychiatry 13:921203. doi: 10.3389/fpsyt.2022.921203
Received: 15 April 2022; Accepted: 14 June 2022;
Published: 06 July 2022.
Edited by:
Thomas Nickl-Jockschat, The University of Iowa, United StatesReviewed by:
Liang Gong, Chengdu Second People’s Hospital, ChinaJeiran Choupan, University of Southern California, United States
Giuseppe Barisano, University of Southern California, United States
Copyright © 2022 Wang, Yang, Sun, Li, Zou, Li, Wu and Li. This is an open-access article distributed under the terms of the Creative Commons Attribution License (CC BY). The use, distribution or reproduction in other forums is permitted, provided the original author(s) and the copyright owner(s) are credited and that the original publication in this journal is cited, in accordance with accepted academic practice. No use, distribution or reproduction is permitted which does not comply with these terms.
*Correspondence: Yue-Hua Li, liyuehua312@163.com
†These authors have contributed equally to this work and share first authorship