From Isolation of Phosphate Solubilizing Microbes to Their Formulation and Use as Biofertilizers: Status and Needs
- 1AgroBioSciences Program, Mohammed VI Polytechnic University (UM6P), Benguerir, Morocco
- 2Université Cheikh Anta Diop, Laboratoire Commun de Microbiologie IRD/ISRA/UCAD, Centre de Recherche de Bel Air, Dakar, Senegal
- 3Faculté des Sciences Semlalia, Université Cadi Ayyad, Laboratoire of Microbial Biotechnologies, Agrosciences and Environment, Marrakesh, Morocco
The production of biofertilizers at industrial level is a bottleneck because bacterial strains are generally developed and managed by research laboratories and not by production units. A seamless transition from laboratory to field application is, therefore necessary. This review provides an overview of the constraints that limiting the application or the implementation of Actinobacteria based biofertilizers especially in agricultural field and suggests solutions to overcome some of these limits. General processes of making and controlling the quality of the inoculum are briefly described. In addition, the paper underlines the opportunity of biofertilizers alone or in combination with chemical fertilizers. This review also, highlights the latest studies (until June 2019) and focuses on P-solubilization microorganisms mainly Actinobacteria. The biotechnology of these bacteria is a glimmer of hope for rock phosphate (RP) bioformulation. Since direct application of RP fertilizer is not always agronomically effective due to its sparse solubility.
Introduction
The world population will reach at least 9.8 billion by 2050 according to the United Nation Food and Agriculture Organization (FAO) projections (Harold and Reetz, 2016). In order to ensure global food security, at least doubling our current agricultural production is required (FAO, 2017, 2018). To achieve this goal, it is necessary to have very fertile soils or to supplement nutrients in low fertility soils by applying a high amount of fertilizers (Keane, 2009). Until now, chemical fertilizers help in feeding the world by providing three major plant nutrients, nitrogen, phosphorus and potassium (NPK). Approximately 52.3 billion tons of P-based fertilizers are applied annually to maintain available P levels in soil–plant systems (FAO, 2017). Whereas, only, about 0.2%, i.e., <10 μM of this huge amount, is used by plants (Alori et al., 2017; Islam et al., 2019) and the rest is precipitated by metal cations in soil such as Fe, Al, Mg, Ca, etc. The extensive use of P may leads to inevitable depletion of world reserves of rock phosphate (Leghari et al., 2016). This depletion will be concomitant with the increase in the cost of these commercial fertilizer products. Recently, scientists from all over the world have focused their attention on sustainable agriculture by exploiting beneficial microbes in order to increase the contribution of biofertilizers to food and fiber production (Khan et al., 2007; Granada et al., 2018; Yadav and Sarkar, 2019). Biofertilizers are commonly defined as “Preparation containing live microbes which helps in enhancing the soil fertility either by fixing atmospheric nitrogen, solubilizing phosphorus or decomposing organic wastes or by augmenting plant growth by producing growth hormones with their biological activities” (Chaparro et al., 2014; Okur, 2018). Many strains, some of which have interesting biotechnological potential have been isolated from the extensive research programs on plant-beneficial microorganisms (Sivasakthivelan and Saranraj, 2013; Umesha et al., 2018; Nafis et al., 2019). Nitrogen fixers, potassium and phosphorus solubilizers, or their combinations with molds fungi are the organisms commonly used as components of biofertilizers (Mohammadi and Sohrabi, 2012; Pathak and Kumar, 2016). Most of these microorganisms used as biological fertilizers are isolated from the plant rhizosphere where they establish close contact with the roots (Xiang et al., 2012). Rhizobia, Arbuscular mycorrhizal fungi (AMF) and Frankia are symbiotic with roots whereas rhizobacteria live in the rhizosphere soil, and/or on the root surface or even in superficial intercellular spaces (Vasconcellos et al., 2010; Stamenković et al., 2018). Nowadays, few microorganisms have been marketed as biofertilizers, and most are in North America, Europe and Asia (particularly India) (Mishra et al., 2017). The low R&D funding in this area, poor quality of such products and low level of adaptability to the farmer's needs could explain their low usage despite their reputation of being easy to use, non-toxic, cheap, eco-friendly and sustainable (FAO, 1991; Date, 2001; Hungria et al., 2005; Shravani et al., 2019). This has resulted in a lack of credibility in these products and makes their sale difficult (Stamenković et al., 2018). Therefore, scientists and biofertilizers manufacturers need to collaborate in order to overcome the bottlenecks in the process of bacterial bioformulation. In this regard, Actinobacteria constitute promising source of novel biofertilizers in sustainable agriculture. Indeed, Actinobacteria have the advantage of surviving in very competitive environments and produce spores during unfavorable conditions (Nandimath et al., 2017). These filamentous organisms can bridge water free gaps between soil particles to move to a new nutritional site (Olanrewaju and Babalola, 2019). Their morphology also gives them a much higher surface to volume ratio and improves their ability to solubilize phosphorus. Therefore, there are immense opportunities for using phosphate and potassic rocks to produce biofertilizers based on Actinobacteria. The development of these biofertilizers could optimize the use of natural phosphate in the world. This comprehensive review aims to (i) make a current assessment of the works that have been done in microbial biotechnology research in particular the P-solubilizing Actinobacteria and (ii) to identify the major limitations and problems in their bioformulation before proposing future research pathways that can contribute to an effective industrialization.
Biofertilizers as Complement to Chemical Fertilizers
Microbes are very small but very powerful and useful. In fact, all the elements brought as chemical fertilizer can be provided by microorganisms, especially Nitrogen (N), Phosphorous (P), and Potassium (K) (Nath et al., 2018). Most of the time, these elements are sufficiently available in the soil (NPK) or in the atmosphere (N) but in an unassimilable form for plants and animals. Rhizobia, Frankia and free-living fixer convert dinitrogen gas (N2) into usable form (NH4+ mainly) for plants uptake, while Arbuscular Mycorrhizal fungi, potassium and phosphate solubilizing microbes dissolve mineral and/or organic sources to make P and K available to plants (Rashida et al., 2016; Bargaz et al., 2018). AMF can also supply other macro and micronutrients such as Fe, Mg, K, Ca, Cu Mn, Zn etc. (Chen et al., 2017). The other important benefits of microbes are their ubiquitous distribution and adaptation to environments (Qu et al., 2019). Some microbes are extremophilic and extremotolerant to various environmental factors such as pressure, drought, salinity, and pH (Rampelotto, 2010; Banga et al., 2018). In the current context where sustainable farming systems are much needed, the use of these plant-friendly microorganisms as biofertilizers is more and more urgent (Yadav and Sarkar, 2019). In fact, the high cost of chemical fertilizers formulation and the difficulties of meeting their demand have encouraged scientists to develop biofertilizers as a solution to replace or at least partially substitute chemo-synthetic and chemical fertilizers (Vaxevanidou et al., 2015). However, the task is not easy because chemical formulation in agro-products is standardized for long-term storage, resistance to abuse of application and ease of use by farmers (Bashan et al., 2014). Thus, biofertilizer need to meet these standards, and produce evident effects like those produced by chemical fertilizer, otherwise it will be difficult to convince farmers, with very low incomes to use them. This means that, in the absence of efficient biofertilizers with consistent quality, their contribution, compared to the chemical fertilizers will remain too low. However, biofertilizers have the ability to slowly release nutrients from a source according to the crop's need and complement other minerals and growth factors an advantage over chemical fertilizers (García-Fraile et al., 2015; Yadav and Sarkar, 2019). Some researchers have tried to test mixed fertilizers combining biofertilizers with organic and/or chemical fertilizers (Cisse et al., 2019). Chen (2006) showed that a cocktail of beneficial microorganisms in compost and urea could help to save the input of chemical or organic fertilizers and decrease P accumulation in the soil. Similar results were reported by Mondal et al. (2015), after combining chemical fertilizers with biofertilizers and vermicompost. They concluded that vermicompost can substitute at least 25% of NPK fertilizer amount and help to increase farmers' incomes. The authors Latha and Jeyaraman (2014) found that the use of chemical fertilizers alone in brinjal (Solanum melongena Linn.) crop had a higher cost-benefit ratio compared to the combination of biofertilizers and chemical fertilizers. Other positive effect of phosphorus biofertilizer in combination with chemical fertilizer on wheat (Farsani et al., 2013), rice (Naher et al., 2016), and soybean (Munda et al., 2015) have been published. Farmers' can therefore get more profit by combining both fertilizers. In view of these results, the urgency to formulate high efficient fertilizers which combine chemical, organic material, and microbe is considerably high and will become more efficient in the future.
Currently, the biofertilizers market is valued around $1.57 billion and is expected to reach $ 1.88 billion by 2020, while the global fertilizer market need will be around $245 billion. Biofertilizers based on P-solubilizing bacteria account for only 14% of this market (Market data forecast, 2018). The main P-solubilizing production units are currently in India and most of them are using strains belonging to Bacillus genus (Table 2). Limited information is available on the production capacity and most of these units are of local scale production. Therefore, P-biofertilizers still have a lot to gain in this market. However, there is an overdue need to understand their limiting factors and emphasize the great opportunities of their use.
Obstacles of Biofertilizers to Overcome
Relative to the Carrier
The weak point of commercialization is often the performance of the microbial inoculum (Mishra and Arora, 2016). The most common barriers of inoculants commercialization are the formulation inadequacies (Bashan et al., 2016a). Indeed, a micro-organism may be efficient in laboratory and/or greenhouse conditions but formulating that organism into an adequate carrier is a difficult step (Reddy and Saravanan, 2013). Hence, the choice of the carrier material is fundamental because carrier (inoculant) is the sole delivery vehicle of live microorganisms from the production unit to plants in the field. A good carrier should be: (i) nontoxic to organisms (ii) easily pulverized and sterilized (iii) able to carry exceptionally high microbial numbers (iv) easily available and cost effective, and (v) should have a good absorption capacity (Mishra and Arora, 2016). The carrier materials can be of various origins: inorganic, organic, or synthesized from specific molecules. However, whatever its origin, the carrier should keep microorganism's viability during storage in the farmer's warehouse and should have a long shelf life and stability (Bashan et al., 2016a). Research must therefore focus on this step-in order to find carriers that meet these needs. Many materials such as charcoal, peat, lignite, vermiculite, farm coal manure mix, charcoal farm yard manure (FYM) mixture, charcoal-soil mixture and kaolin have been identified as suitable carriers (Bashan, 1986; Berninger et al., 2018). However, these carriers are very prone to contamination after formulations that can reduce the shelf life of the inoculant after autoclaving (Brahmaprakash and Sahu, 2012). The innovations offered by materials science and engineering technologies help to avoid these limitations. For instance, formulations based on alginate beads encapsulation offer advantages of delivering microorganism at the right and precise concentration without losing efficacy (Bashan et al., 2014; Shang et al., 2019) and keeping the quality of inoculant products (Deaker et al., 2011). This technique which lately has become popular in the pharmaceutical, nanotechnology, medicine, aquaculture, and cosmetics industry, ensures the inclusion of liquids, gases or fine solid particles with natural or synthetic polymer (Bashan et al., 2014). The polymeric, deserves attention, because many studies have reported that alginate beads improve cell survival with remarkable flexibility, biodegradation and biocompatibility (Herrmann and Lesueur, 2013; Dragostin et al., 2017). Nevertheless, this approach need to be optimized especially the type of alginate, and the diameter of the beads to obtain the best function after bio-formulation by encapsulation (Bashan et al., 2016a).
Other research focuses on inorganic carrier such as water-in-oil emulsions (Molet-Rodríguez et al., 2018). The principle of this method is to mix water and oil, so, oil is dispersed as droplets in a continuous water phase. The microorganisms are trapped in water droplets and are protected by a physical barrier of emulsifiers. Sometimes, the process can be improved by adding a surfactant i.e., surface-active agent. This technology is widely used in pharmaceutical and cosmetic industries and cancan be adapted to microbial bioformulation (Malusá et al., 2012). However, a certain number of parameters such as oil type, oil density and, emulsion stability must be mastered (Molet-Rodríguez et al., 2018). To overcome these obstacles, collaboration between researchers in the field microbiology and materials science is necessary because bioformulation is at the crossroads of these two disciplines.
Relative to Quality Standards
Quality standards are the major concern of microbial biotechnology. Two important characteristics define the quality of a bio fertilizer: the presence of the recommended strain in active form and required number. If any of these characteristics is missing in the product, the biofertilizer could be termed as sub-standard (Ghosh et al., 2001). Currently, the quality control framework is not well-defined. Furthermore, the present quality standards as prescribed by regulatory agencies of a country, do not authenticate the strain used for commercial production (Balachandar, 2012). As a result, most of the inoculants produced in the world are of relatively poor in quality (Thuita et al., 2018). To solve this problem, international standards through the ISO Standards should be established and be adapted in the production and use of these biofertilizers. Although efforts to regulate the industries have been made by some countries such as India, Canada, Brazil, China, etc., there are still insufficient (Hungria et al., 2005). In China, the number of cell forming units (CFU) is considered as the main parameter in assessing the quality of the different kinds of biofertilizers (Malusá and Vassilev, 2014). In the European Union, quality parameters for biofertilizers are not yet standardized and they vary from one region to another (Malusá and Vassilev, 2014). Currently, India seem to have the most standardized and functional legal framework related to biofertilizers (Malusá and Vassilev, 2014; Manashi et al., 2017).
Quality Control Procedure
To ensure product safety, efficacy, and conformity to prescribed standards, quality control and regulation of bio-fertilizers are important steps (Arora et al., 2016). Inoculum quality control should be done at different stages: screening and efficiency at laboratory and the greenhouse scale by researchers, formulation including choice of support, packaging, and storing at industrial scale. Each step requires expertise, and everything must be governed by standards (under regulation) (Figure 1). Unfortunately, all biofertilizer production units do not follow this procedure due to lack of technical back up.
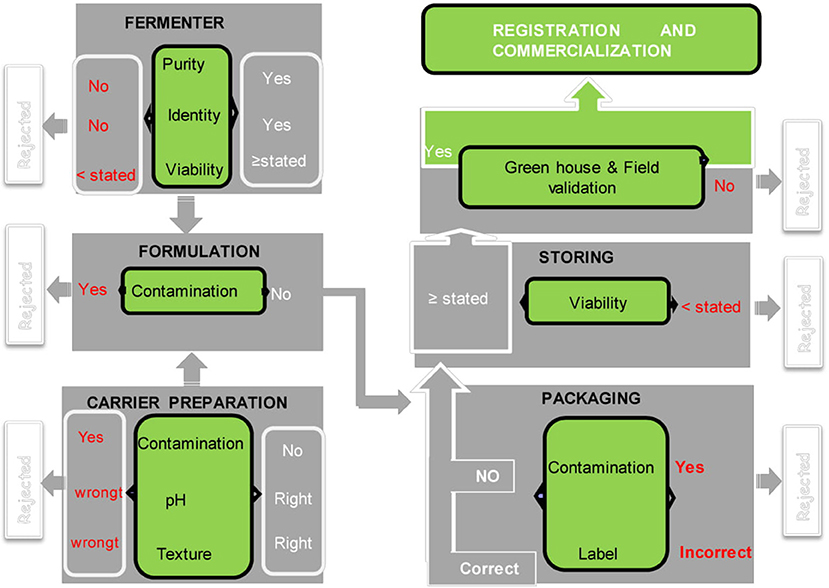
Figure 1. Quality control in the biofertilizer manufacturing process [modified from García-Fraile et al. (2015), Permission acquired from the author]. Writing in red means that the quality control is not compliant, and the project is rejected the writing in white corresponds to the compliant product. The diagram shows that bio-formulation has six main steps before commercialization and four each stage, a quality control is necessary.
Techniques' for Strain Identification
Until now, at the international level, there are still no quality control procedures and guidelines for monitoring microbial cells and activity during the production and formulation of inoculants. Many enumeration methods exist, each with its advantages and limitations (Table 1). Usually, the number of living cells is counted by spread plate or drop plate methods or Most Probable Number Method (MPN). However, all these enumeration methods are not specific to a particular strain and are not suitable enough to detect low population levels (Table 1). Additionally, contaminants have some effect on counting protocols and they do not allow identity of target strain. Molecular biology offers techniques for detecting the presence and abundance of specific microorganisms whether in the soil, rhizosphere or in a commercial inoculant, with high sensitivity. Sequence Characterized Amplified Region (SCAR) marker-based fingerprinting produces very good results as demonstrated by a recent research we carried out (Reddypriya et al., 2019) and those of Couillerot et al. (2010) and Reddypriya et al. (2016). Our results showed that, in addition to authentication, quantitative PCR and SCAR marker allow for assessing the cell loads per g/ml of inoculants (Figure 2). In this study, the presence and abundance of Azotobacter chroococcum (Ac1), Bacillus megaterium (Pb1), and Azospirillum brasilense (Sp7) in biofertilizer-inoculated maize rhizosphere soil was detected by small DNA fragments of 299, 375, and 584 bp, respectively. These results clearly showed that multiplex PCR and real-time PCR targeting SCAR markers could be applied in quality control of commercial biofertilizer production, to guarantee quality product for farmers satisfaction. This work can be extended to all potentially usable strains as biofertilizer.
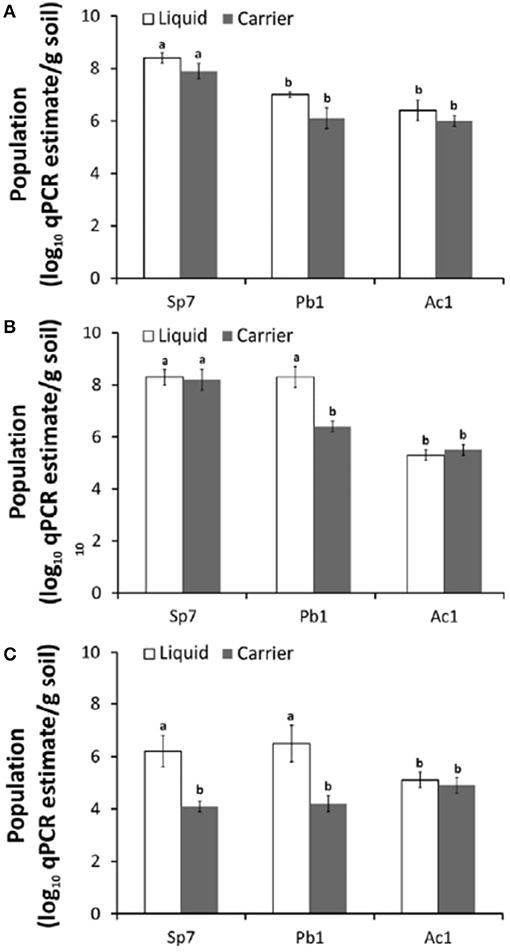
Figure 2. PCR targeting SCAR markers of biofertilizer strains for strain-level authentication and qualification in maize rhizosphere at different growth stages 15th (A), 30th (B), and 60th (C) day. Error bar indicates with letters “a” and “b” (ANOVA and DMRT test; p < 0.05) (Reddypriya et al., 2019) Reproduced with permission.
Previously, colony immunoblotting technique was evaluated for the specific detection and enumeration of Citrobacter freundii in sterilized or unsterilized carrier media in commercial products (Kecskés et al., 2009). Today, the sensitivity of microbial identification and enumeration in a sample is improved by next generation sequencing Technique (Abbasian et al., 2018). However, the analysis in Table 1 shows that it is currently difficult to find a technique that meets all the desired criteria, i.e., reliable, rapid, inexpensive, and relatively easy to use. Molecular techniques generally have good reliability but require expensive and sophisticated laboratory equipment and technical expertise (Table 1). From this comparative analysis, we conclude that SCAR marker studies have more advantages and deserve to be extended to all microorganisms used in bioformulation.
Potential Features of Phosphate Solubilizing Actinobacteria
Several strains cited in the scientific literature as potentially useful do not appear in the commercial market, perhaps because of inappropriate formulation (Bashan et al., 2014). Unfortunately, they are lost or forgotten. For example, in Africa, lots of efficient isolates belonging to Azospirillum, Rhizobia, Cyanobacteria, Azotobacter (for N2 fixation), Pseudomonas, Bacillus, Aspergillus, Penicillium (for P solubilization and PGPR), Bacillus (K solubilization), and arbuscular mycorrhiza (for P mobilization) are described in literature (Hamdali et al., 2012; Ndoye et al., 2013; Barka et al., 2016; Berruti et al., 2016; Hassimi et al., 2017; Soumare et al., 2017) but none of them (or only very few) has been formulated and marketed. This observation can be extended to many other countries and even all over the whole world. There are many reasons that might have led to this situation, but lack of funding is one of the most important part. Grant applications regarding bio-formulations are seldom successful. The lack of private sector involvement in the production and formulation of the inoculum is perhaps the missing link in the chain. The contribution of phosphate producing companies from rock P is a necessity for bio-formulation of phosphate solubilizing microbes (PSMs). This investment will be beneficial for these companies because it will allow them to diversify their offer to farmers. The phosphorus importance for plant growth and role of bacteria to make it available is presented in Box 1.
Box 1. Importance of P in live and role of bacteria to its availability
Phosphorus is considered as the second most vital plant nutrient as well as second most deficient after nitrogen (Leghari et al., 2016). It is highly abundant in nature as rock phosphate. The global resources of rock phosphate are of the order of 163,000 million tonnes. Africa contains about 41%, USA has 21%, USSR 13%, the Middle East 10%, Asia 8%, South America 3%, New-Zealand and Oceania together accounts for only 2% and entire Europe <1% (Kumari and Phogat, 2008). Without P, life would not exist because it is a vital component of cell walls, DNA, RNA, and ATP to transport energy to the brain. In crop growth, there is no substitute for phosphorus and phosphorus cannot be synthetically manufactured. It is available for plants in the form of orthophosphate ions H2 and (Leghari et al., 2016). Plants require this phosphorus for cell growth, the formation of fruits and seeds and ripening (Figure 4). Unfortunately, P is one of the least biologically available nutrients. The fact that certain soil microbes are capable of dissolving relatively insoluble phosphatic compounds has opened the possibility of inducing microbial solubilization of phosphates in the soil. The maximum level of phosphorus solubilization potential of Actinomyctes isolates selected from tropical soils was established around 1,727 μg/ml of Ca-P and 48.0 μg/ml of RP (Leghari et al., 2016).
The use of phosphate solubilizing microbes (PSMs) will efficiently increase fertilizers uptake by mobilizing insoluble phosphorus in the fertilizers and in soils to which they are applied (Suleman et al., 2018). In fact, chemical P fertilizer is subjected to chemical fixation (see Box 1) in soil with some metal cations (Viani et al., 2014) and losses by leaching (Fortune et al., 2005). Combined application of rock phosphate with slow-release PSMs would be a sustainable solution in the modern agriculture. Thus, natural RP is a valuable alternative and less expensive natural source for phosphate fertilizers (Biglari et al., 2016). Sane and Mehta (2015) showed that phosphate solubilizing bacteria and fungi in co-inoculation increase the mobilization of rock phosphate and plant growth. Authors Zaidi et al. (2017) and Ding et al. (2019) reported that PSMs isolated from the rice rhizosphere released 22–826 μg P mL−1 in in vitro test. Among the PSMs, Actinobacteria (Actinomyces) are of special interest since these filamentous sporulating bacteria (see details in Box 2) are able to develop in extremely different soils and offering a unique opportunity for biotechnological application (Mengual et al., 2016).
Box 2. Features and taxonomy of Actinobacteria
Actinobacteria are a distinct group of bacteria that are widely distributed in nature. They are gram-positive bacteria with high G+C content. Their taxonomy is extremely complex, they were first divided into two groups namely, Streptomyces and non-Streptomyces or also known as rare Actinobacteria. In volume 5 of the Bergey's, the phylum Actinobacteria is divided into 6 classes namely Actinobacteria, Acidimicrobiia, Coriobacteriia, Nitriliruptoria, Rubrobacteria and Thermoleophilia. According to the recent classification of Barka et al. (2016), the class Actinobacteria comprises five subclasses, 10 orders, 56 families, and 286 genera.
Actinobacteria are organisms with characteristics common to both bacteria and fungi but possessing distinctive features to delimit them into a distinct category. They are unicellular like bacteria but produce a mycelium which is non-septate. They resemble fungi because they are adapted to life on solid surfaces and they can produce mycelium and dry spores like most fungi (Kalakoutski and Agre, 1976). On culture media, they have different cultural characteristics (Figure 5) and occur in the soil in the spore stage as well as in the mycelial stage (Figure 5). Their mycelial growth form creates the potential for the formation of large networks (Krsek et al., 2000). They are typically present at densities in the order of 106-109 cells per gram of soil and they represent more than 30% of the total population of soil microbiomes (Polti et al., 2014). Actinobacteria play an important role in soil and in plant interaction because of their ability to produce a large number of secondary metabolites, many of which possess antibacterial activity (Lazzarini et al., 2000). Actinobacteria produce approximately two-thirds of the known antibiotics produced by all microorganisms (Dhanasekaran et al., 2012; Feina et al., 2019). This nutrient cycling capacity makes them an ideal candidate for natural fertilizers (Jog et al., 2016; Olanrewaju and Babalola, 2019). In addition, the ubiquitous presence and possible global distribution of many of these actinobacteria suggest a great environmental tolerance. Actinobacteria could be used for the formulation of novel biofertilizer and bio-control products constituted by spores and/or mycelium in association with pulverized rock phosphate (Reponen et al., 1998; Nandimath et al., 2017). Unfortunately, this great potential interest of Actinobacteria is almost unexplored in Africa and all over the world.
These bacteria are characterized with, thermo-tolerant, drought-tolerant, easier to cultivate and formulate, easier to store and transport, and extended shelf life. Actinobacteria spores can be stored for a long time because they are very resistant to several environmental factors (Sharma et al., 2014). Dry formulations, such as powders containing mostly spores or granules can be manufactured with simple and cost-effective time technology. On the other hand, while most P-solubilizing organisms act through a mechanism of acidification of the soil, Actinobacteria appears to be moreover disposed of by other, efficient mechanisms such as chelation (Delvasto et al., 2006; Sharma et al., 2013; Nandimath et al., 2017). The high buffering capacity of the soil, generally prevents acidification and causing a failure of some biofertilizers (Khan et al., 2007). Irrespective of the type of soil, it acid or alkaline, Actinobacteria are able to solubilize rock phosphate (RP) and potassium rock (Hamdali et al., 2008). Therefore, a biofertilizer based on Actinobacteria inoculated into these materials will provide continuous supply of P and K for sustainable plant growth. In addition to their fertilizing ability, Actinobacteria can be used as biopesticide and bio-immunizing agents against plant diseases (Majeed et al., 2015; Barka et al., 2016). In fact, some Actinobacteria limit Fe availability in the soil by producing a Fe-chelating agent, siderophore. This results in low availability of this element, which limits or suppresses the growth of pathogens and their ability to cause diseases (Solanki et al., 2012; Raimi et al., 2017). Otherwise, the sequestration of these cations by the excreted siderophores led to the solubilization of the insoluble phosphates. The figure below summarizes in a very simple way the different stage of the bioformulation of Actinobacteria as a fertilizer (Figure 3). Some Actinobacteria, in addition to their biofertilization effects (ability to solubilize RP) can act as growth-promoting rhizobacteria (PGPR) as like other PGPR through phytohormones production such as indole acetic acid (IAA), gibberellins and cytokinins (Gopalakrishnan et al., 2014; Sousa and Olivares, 2016). A large amount of IAA (222.75 ppm) is produced by Actinobacteria belonging to the genus of Nocardiopsis and isolated under the rhizosphere of mandarin (Shutsrirung et al., 2013). IAA is one of the main phytohormone enhancing plant growth by stimulating the elongation of stems and roots. Other Actinobacteria belonging to the genus of Streptomyces have also shown a positive effect on the growth and elongation of common bean roots (Igarashi et al., 2002). Similar results were reported by Rashad et al. (2015) who showed that marine Actinomyces produce lot of phytohormones, including gibberellic acid. These phytohormones have been tested on eggplant (Solanum melongena) and have improved the agronomic performance of the species. On the other hand, Endophytic Actinobacteria produces secondary metabolites that improve growth and resistance to various environmental stresses (Girão et al., 2019). For instance, kasugamycin and mildiomycin from Streptomyces kasugaensis and S. rimofaciens respectively protect against rice blast and powdery mildew diseases (Sathya et al., 2017). According to Srivastava et al. (2015), PGP-Streptomyces rochei SM3 increase chickpea biomass accumulation (20%) and induces stress tolerance against NaCl and Sclerotinia sclerotiorum. Previously (Hamdali et al., 2008) reported that Micromonospora aurantiaca- and Streptomyces griseus increase wheat shoots and roots weight, respectively 50–47% and 80–78. Using two P-solubilizing actinomycetes as biofertilizers, Mba (1994) showed that these strains led to a 43% increase in soybean yield and soil properties were also improved. Alam et al. (2012) achieved an increase in yield (11%) of geranium herbs inoculated with Streptomyces sp. The ability of some Streptomyces to fix nitrogen as free-Living is an additional advantage in offsetting nitrogen losses from soil (Dahal et al., 2017). It has been documented that Actinobacteria promotes legume symbioses through hyphal elongation for symbiotic fungi (Schrey and Tarkka, 2008) and /or by increasing nodulation for nitrogen fixing symbiosis (Sathya et al., 2017). The multifunctionality of Actinobacteria is a definite advantage in the prospect of using them as bioinoculants for sustainable agriculture. However, beneficial PGP traits of Actinobacteria require more extensive research and more field trials. Also, extensive studies on Actinobacteria mechanisms of PGP action are needed.
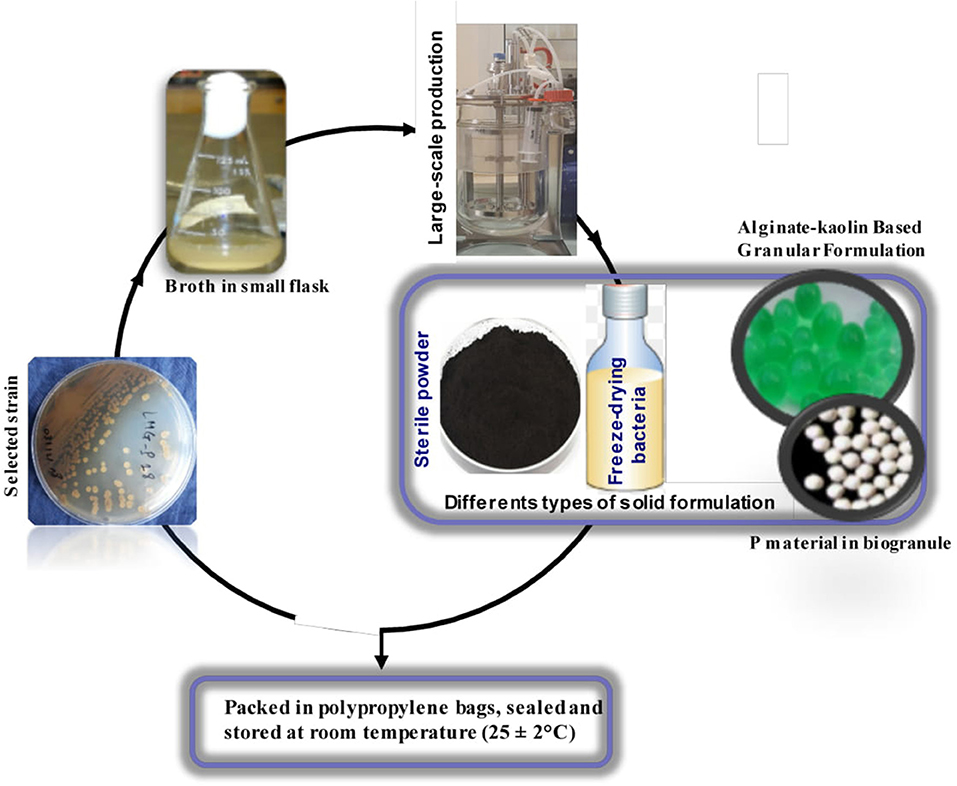
Figure 3. Simplified schematic flow chart for the production of Actinobacteria biofertilizer. Quality control is done at all stages of production a production as previously described (II.3).
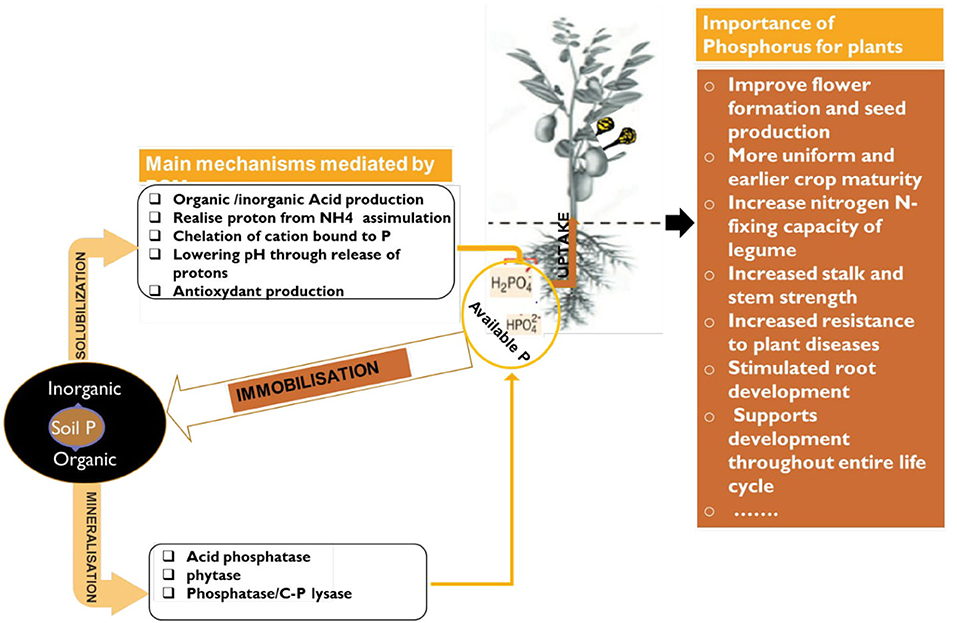
Figure 4. Schematic representation of P solubilization/mineralization/immobilization by PSM and its importance for plant growth.
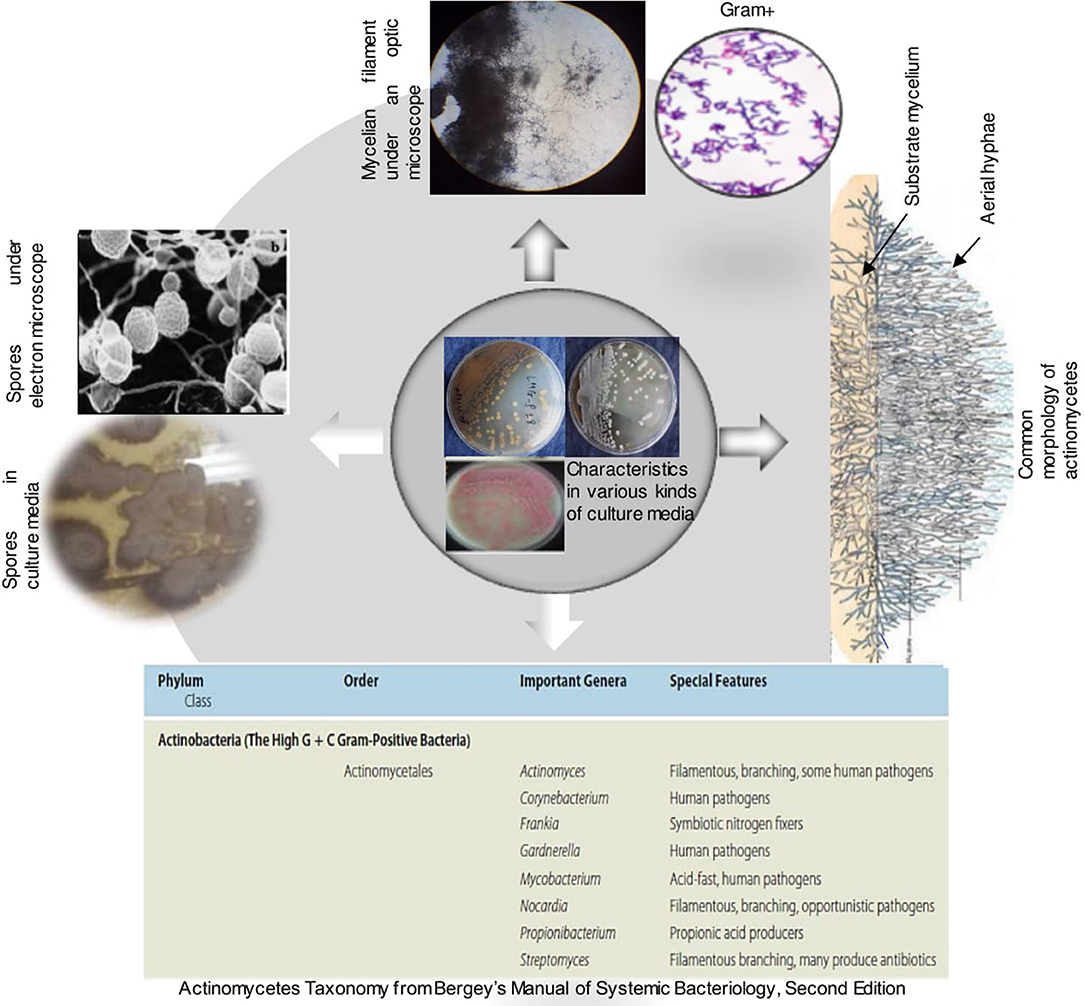
Figure 5. Some features of actinobacteria (Bergey's Manual of systematic Bacteriology, Barka et al., 2016).
Optimization of Actinobacteria For Mass Production
Bacterial production and formulation are close. Hence, it is important to find out how, when, where and under what conditions the selected bacteria for bioformulation work. For this, prior optimization of the bacteria by monitoring growth parameters such as pH, culturing conditions, temperature, incubation time etc. is required. Many Actinobacteria species are grown on the common bacteriological media under normal conditions i.e., pH 7, T°C 28–30°C (Pudi et al., 2016). However, some species belonging to Frankia genus require special incubation conditions and growth media. The maximum yield is usually obtained after 7–10 days of incubation. For sporulating Actinobacteria, modification of nutrient parameters can induce and increase spore yield. In order to reduce the use of chemicals for mass production, several cost-effective substrates have been tested successfully. For instance, Soares et al. (2007) and Diraviyam et al. (2011), have shown that sterile moistened rice, rice bran, wheat bran and sugarcane residues can be viable methods for inoculum mass production of Actinobacteria. Despite of this innovation, research must be continued to test natural, inexpensive and available products for microbial biomass production. After mastering mass production, validation of the inoculation methods is also an essential step for biofertilizer. Three main biofertilizers inoculation methods (seeds treatment, root dipping and soil application) are generally used. For Actinomyces, better plant growth and yield are found when inoculum has been applied to the soil or substrate before sowing seeds (Gopalakrishnan et al., 2012; Sousa and Olivares, 2016). According to these authors, this type of application leads to a better establishment of the inoculated strain and better colonization of soil and/or roots. However, more field research results are still needed to optimize inoculation techniques in actinobacterial biofertilizers.
Environmental Risk/Safety of Actinobacteria
There are few publications on the biosafety of biofertilizers, especially those based on Actinobacteria. Most of Actinobacteria fall in biosafety level 1, i.e., they are not known to be responsible for diseases (Shepherd et al., 2010). Only, few microorganisms used as bio fertilizers fall in biosafety level 2, i.e., opportunistic pathogens and presents health and/or environmental risk. This does not exclude the respect of good microbiological practices by following general laboratory safety rules. In this regard, research and industrial laboratories must verify the level of pathogenicity or any other risk related to microorganisms before embarking on a process of bioformulation or commercialization.
The environmental risks of inoculation are related to the fact that exotic microorganisms can disrupt the functioning of the soil microbiome because they can create invasive and competitive reactions (Glick, 2012; Sathya et al., 2017). Given these risks, it is more recommended to use native isolates because they have lower ecological risk to ecosystem. Otherwise, prior field studies must be carried out. On the other hand, Actinobacteria are major producers of antibiotics, so the horizontal transfer of genes between bacteria might be particularly risky in case of resistance (Egan et al., 2001). In a study, Martinez (2008) had already warned about the risk of disruption of rhizospheric population and resistance selection due to a large release of antimicrobial compounds. Additionally, about ten species belonging to genus of Streptomyces are known to be plant-pathogens and responsible of the common scab of potatoes (Labeda, 2011; Vurukonda et al., 2018). Such species should never be subject to bioformulation for biosecurity issues.
Conclusions and Perspectives
One of the main problems to be solved in the next decades is to minimize dependence on phosphate fertilizers. To meet this challenge, PSMs must play a bigger role. It is known and well-documented that PSMs are able to solubilize rock phosphate. Exploitation of this potential may be a promising technique for plant phosphate nutrition. Thus, several tests of selection, identification, performance, and bio-formulation have been carried out in recent past to meet this need. However, this review shown that most of the experiments are carried out at laboratory or greenhouse scales and few papers have presented results in the field. So, we suggest some future lines: (i) since we know that results in laboratory or greenhouse conditions do not always reflect those of the field, therefore, consistent field results are a prerequisite for a massive adoption of biofertilizers based PSMs; (ii) there is also need for further research to understand the complex associations between the microbes-rock phosphate, dynamics of microbial populations, mechanisms of PSMs, and suitable technique of inoculation according the target crop and/or stain.
These data will allow to model the process of solubilization and even bioformulation (Saeid, 2018); (iii) the market of biofertilizers based on PSMs is very low compared to the biofertilizer based on nitrogen-fixing bacteria, 14 vs. 79% according to Transparency Market Research (2014). Therefore, potential problems associated with quality and stability of PSMs inoculum should be investigated with new approaches stemming from the collaboration between microbiologists, materials scientists and agricultural scientists (Arora, 2015; Berninger et al., 2018). Additionally, inoculation techniques and methodology should be described in detail to allow repetition of the experiment (Bashan et al., 2016b); (iv) among PSMs, Actinobacteria are the least studied for their agronomic interest. These bacteria deserve special attention due to their ability to adapt to diverse ecosystems such as desert soil, marine soil, rock phosphate deposit etc. (Nafis et al., 2019; Qu et al., 2019). Therefore, future research should focused on this group which still has a hidden repertoire that can be explored to develop quality bio-fertilizers. By associating these bacteria with Arbuscular mycorrhiza fungi, they can solubilize and mobilize P stored within the soil resulting in efficient biofertilizer. It is also necessary to focus on multi-functionality of Actinobacteria including nitrogen fixers and solubilizing phosphorus since plant production is largely dependent on N and P availability. Actinobacteria biotechnology has the potential to induce profound modification of agricultural practices, if it is developed. Therefore, there is a need for disruptive innovation and scientific research to ensure optimal rock phosphate solubilizing and bioformulation for sustainable and ecofriendly agriculture. An optimization of the processes of selection, multiplication, storage of PSM and their interactions in the rhizosphere are steps to master in order to develop efficient microbial inoculants with high phosphorus solubilization capacity.
Author Contributions
All authors listed have made a substantial, direct and intellectual contribution to the work, and approved it for publication.
Conflict of Interest
The authors declare that the research was conducted in the absence of any commercial or financial relationships that could be construed as a potential conflict of interest.
References
Abbasian, F., Ghafar-Zadeh, E., and Magierowski, S. (2018). Microbiological sensing technologies: a review. Bioengineering 5:20. doi: 10.3390/bioengineering5010020
Alam, M., Dharni, S., Khaliq, A., Srivastava, S. K., Samad, A., and Kum, M. (2012). A promising Strain of Streptomyces sp. With agricultural traits for growth promotion and deseases management. Indian J. Exp. Biol. 50, 559–568.
Alori, E. T., Glick, B. R., and Babalola, O. (2017). Microbial phosphorus solubilization and its potential for use in sustainable agriculture. Front. Microbiol. 8:971. doi: 10.3389/fmicb.2017.00971
Arora, N. K., Verma, M., Prakash, J., and Mishra, J. (2016). Regulation of biopesticides: global concerns and policies. Bioformulations. 283–299. doi: 10.1007/978-81-322-2779-3_16
Balachandar, D. (2012). Biofertilizers–What Next? J. Biofertil. Biopestici. 3:1000e108. doi: 10.4172/2155-6202.1000e108
Banga, C., Dagana, T., Deines, P., Dubilierc, N., Duschld, W. J., Fraune, S., et al. (2018). Metaorganisms in extreme environments: do microbes play a role in organismal adaptation? Zoologie 127, 1–9. doi: 10.1016/j.zool.2018.02.004
Bargaz, A., Lyamlouli, K., Chtouki, M., Zeroual, Y., and Dhiba, D. (2018). Soil microbial resources for improving fertilizers efficiency in an integrated plant nutrient management system. Front. Microbiol. 9:1606. doi: 10.3389/fmicb.2018.01606
Barka, E. A., Vatsa, P., Sanchez, L., Gaveau-Vaillant, N., Jacquard, C., Klenk, H.-P., et al. (2016). Taxonomy, physiology, and natural products of actinobacteria. Microbiol. Mol. Biol. Rev. 80, 1–43. doi: 10.1128/MMBR.00019-15
Bashan, Y. (1986). Alginate beads as synthetic inoculant carriers for slow release of bacteria that affect plant growth. Appl. Environ. Microbiol. 51, 1089–1098.
Bashan, Y., de-Bashan, L., and Prahbu, S. R. (2016a). “Superior polymeric formulations and emerging innovative products of bacterial inoculants for sustainable agriculture and the environment,” in Agriculturally Important Microorganisms (Singapore), 15–46.
Bashan, Y., de-Bashan, L. E., Prabhu, S. R., and Hernandez, J. P. (2014). Advances in plant growth-promoting bacterial inoculant technology: formulations and practical perspectives (1998–2013). Plant Soil. 378, 1–33. doi: 10.1007/s11104-013-1956-x
Bashan, Y., Kloepper, J. W., de-Bashan, L. E., and Nannipieri, P. (2016b). A need for disclosure of the identity of microorganisms, constituents, and application methods when reporting tests with microbe- based or pesticide-based products. Biol. Fertil. Soils 52, 283–284. doi: 10.1007/s00374-016-1091-y
Berninger, T., López, G., Bejarano, A., Preininger, C., and Sessitsch, A. (2018). Maintenance and assessment of cell viability in formulation of non-sporulating bacterial inoculants. Microb. Biotechnol. 11, 277–301. doi: 10.1111/1751-7915.12880
Berruti, A., Lumini, E., Balestrini, R., and Bianciotto, V. (2016). Arbuscular mycorrhizal fungias natural biofertilizers: let's benefitfrom past successes. Front. Microbiol. 6:1559. doi: 10.3389/fmicb.2015.01559
Biglari, N., Mat-Hassan, H., and Amini, J. (2016). The Ability of Streptomyces spp. Isolated from Iranian Soil to Solubilize Rock Phosphate. Australian International Academic Centre, Australia.
Bonny, S. Q., Motalib, H. M. A., Kwai Lin, T., and Eaqub, A. (2018). Multiplex MPN-CR for the enumeration of three major Vibrios in raw fishes in Malaysia. Food Control. 90, 459–465. doi: 10.1016/j.foodcont.2018.02.034
Brahmaprakash, G. P., and Sahu, P. K. (2012). Biofertilizers for sustainability. J. Indian Inst. Sci. 92, 37–62.
Chaparro, J. M., Badri, D. V., and Vivanco, J. M. (2014). Rhizosphere microbiome assemblage is affected by plant development. ISME J. 8, 790–803. doi: 10.1038/ismej.2013.196
Chen, J. H. (2006). The Combined Use of Chemical and Organic Fertilizers and/or Bio-Fertilizers for Crop Growth and Soil Fertility. Available online at: http://www.agnet.org/htmlarea_file/library/20110808103954/tb174.pdf
Chen, S., Zhao, H., Zou, C., Li, Y., Chen, Y., Wang, Z., et al. (2017). Combined inoculation with multiple arbuscular mycorrhizal fungi improves growth, nutrient uptake and photosynthesis in cucumber seedlings. Front. Microbiol. 8:2516. doi: 10.3389/fmicb.2017.02516
Cisse, A., Arshad, A., Wang, X., Yattara, F., and Hu, Y. (2019). Contrasting impacts of long-term application of biofertilizers and organic manure on grain yield of winter wheat in North China Plain. Agronomy 9:312. doi: 10.3390/agronomy9060312
Clais, S., Boulet, G., Van kerckhoven, M., Lanckacker, E., Delputte, P., Maes, L., et al (2015) Com-parison of viable plate count, turbidity measurement real-time PCR for quantification of Por-phyromonas gingivalis. Lett. Appl. Microbiol. 60, 79–84. doi: 10.1111/lam.12341.
Couillerot, O., Bouffaud, M.-L., Baudoin, E., and Muller, D. (2010). Development of a real-time PCR method to quantify the PGPR strain Azospirillum lipoferum CRT1 on maize seedlings. Soil Biol. Biochem. 42, 2298–2305. doi: 10.1016/j.soilbio.2010.09.003
Dahal, B., NandaKafle, G., Perkins, L., and Brözel, V. S. (2017) Diversity of free-Living nitrogen fixing Streptomyces in soils of the badlands of South Dakota. Microbiol. Res. 195, 31–39. doi: 10.1016/j.micres.2016.11.004
Date, R. A. (2001). Advances in inoculant technology: a brief review. Aust. J. Exp. Agric. 41, 321–325. doi: 10.1071/EA00006
Deaker, R., Kecskés, M. L., Rose, M. T., Amprayn, K., Krishnen, G., Kim, T. C., et al. (2011). Practical Methods for the Quality Control of Inoculant Biofertilisers. ACIAR Monograph No.148. Australian Centre for International Agricultural Research: Canberra.
Delvasto, P., Valverde, A., Ballester, A., Igual, I. M., Muñoz, J. A., González, F., et al. (2006). Characterization of brushite as a re-crystallization product formed during bacterial solubilization of hydroxyapatite in batch cultures. Soil Biol. Biochem. 38, 2645–2654. doi: 10.1016/j.soilbio.2006.03.020
Dhanasekaran, D., Thajuddin, N., and Panneerselvam, A. (2012). “Applications of actinobacterial fungicides in agriculture and medicine,” in Fungicides for Plant and Animal Diseases (Rijeka: InTech), 29–54. doi: 10.5772/25549
Ding, L.-J., Cui, H.-L., Nie, S.-A., Long, X.-E., Duan, G.-L., and Zhu, Y.-G. (2019). Microbiomes inhabiting rice roots and rhizosphere. FEMS Microbiol. Ecol. 95:fiz040. doi: 10.1093/femsec/fiz040
Diraviyam, T., Radhakrishnan, M., and Balagurunathan, R. (2011) Antioxidant activity of mela-nin pigment from Streptomyces species D5 isolated from Desert soil. Drug Invent Today 3, 12–13.
Dragostin, O., Dragostin, O., Pelin, A. M., Grigore, C., and Zamfir, C. L. (2017). The importance of polymers for encapsulation process and for enhanced cellular functions. J. Macromol. Sci. Pure Appl. Chem. 54, 489–493. doi: 10.1080/10601325.2017.1320754
Egan, S., Wiener, P., Kallifidas, D., and Wellington, E. M. (2001) Phylogeny of Streptomyces species evidence for horizontal transfer of entire partial antibiotic gene clusters. Antoine Leeuwenhoek 79, 127–133. doi: 10.1023/A:1010296220929
Emerson, J. B., Adams, R. I., Román, C. M. B., Brooks, B., Coil, D. A., Dahlhausen, K., et al. (2017). Schrödinger's microbes: tools for distinguishing the living from the dead in microbial ecosystems. Microbiome 5:86. doi: 10.1186/s40168-017-0285-3
FAO. (1991). Expert Consultation on Legume Inoculant Production and Quality Control. Rome: FAO. Available online at: www.fao.org/publications).
FAO. (2017). The Future of Food and Agriculture – Trends and Challenges. Rome. Available online at: www.fao.org/publications.
FAO. (2018). The State of Agricultural Commodity Markets 2018. Agricultural trade, climate change and food security. Rome. Available online at: www.fao.org/publications.
Farsani, A. F., Chorom, M., and Enayatizamir, N. (2013). Effect of Biofertilizer and Chemical Fertilizer on wheat yield under two soil types in experimental greenhouse. J. Soil Water Conserv. 27, 441–451.
Feina, L., Shaowei, L., Qinpei, L., Hongyun, Z, Osterman, I. A., Lukyanov, D. A., et al. (2019). Studies on antibacterial activity and diversity of cultivable actinobacteria isolated from mangrove soil in futian and Maoweihai of China. Evid. Based Complement. Alternat. Med. 2019:3476567. doi: 10.1155/2019/3476567
Fortune, S., Lu, J., Addiscott, T. M., and Brookes, P. C. (2005). Assessment of phosphorus leaching losses from arable land. Plant Soil 269, 99–108. doi: 10.1007/s11104-004-1659-4
García-Fraile, P., Menéndez, E., and Rivas, R. (2015). Role of bacterial biofertilizers in agriculture and forestry. AIMS Bioeng. 2, 183–205. doi: 10.3934/bioeng.2015.3.183
Ghosh, T. K., Singh, R. P., Duhan, J. S., and Yada, D. S. (2001). A Review on Quality Control of Biofertilizer in India. The fertiliser association of India 10, Shaheed Jit Singh Marg New Delhi – 110067. Available online at: www.fertindia.com.
Girão, M., Ribeiro, I., Ribeiro, T., et al (2019) Actinobacteria Isolated From Laminaria ochroleuca: A Source of New Bioactive Compounds. Front. Microbiol.. 10, 683. doi: 10.3389/fmicb.2019.00683.
Glick, B. R. (2012) Plant growth-promoting bacteria: mechanisms and applications. Scientifica 2012:963401. doi: 10.6064/2012/963401.
Gopalakrishnan, S., Humayun, P., Vadlamudi, S., Vijayabharathi, R., Bhimineni, R. K., and Rupela, O. (2012). Plant growth-promoting traits of Streptomyceswith biocontrol potential isolated from herbal vermicompost. Biocontrol Sci. Technol. 22, 1199–1210. doi: 10.1080/09583157.2012.719151
Gopalakrishnan, S., Vadlamudi, S., Bandikinda, P., Satya, A., Vijayabharathi, R., Rupela, O., et al. (2014). Evaluation of Streptomyces strains isolated from herbal vermicompost for their plant growth-promotion traits in rice. Microbiol. Res. 169, 40–48. doi: 10.1016/j.micres.2013.09.008
Granada, C. E., Passaglia, L. M. P., de Souza, E. M., and Sperotto, R. A. (2018). Is phosphate solubilization the forgotten child of plant growth-promoting rhizobacteria? Front. Microbiol. 9:2054. doi: 10.3389/fmicb.2018.02054
Guo, R., McGoverin, C., Swift, S., and Vanholsbeeck, F. (2017). A rapid and low-cost estimation of bacteria counts in solution using fluorescenc spectroscopy. Anal. Bioanal. Chem. 409, 3959–3967. doi: 10.1007/s00216-017-0347-1
Hamdali, H., Hafidi, M., Virolle, M. J., and Ouhdouch, Y. (2008). Growth promotion and protection against damping-off of wheat by two rock phosphate solubilizing actinomycetes in a P-deficientsoilunder green house conditions. Agric. Ecosyst. Environ. Appl. Soil Ecol. 40, 510–517. doi: 10.1016/j.apsoil.2008.08.001
Hamdali, H., Moursalou, K., Tchangbedji, G., Ouhdouch, Y., and Mohamed, H. (2012). Isolation and characterization of rock phosphate solubilizing actinobacteria from a Togolese phosphate mine. Afr. J. Biotechnol. 11, 312–320. doi: 10.5897/AJB11.774
Harold, F., and Reetz, J. (2016). Fertilizers and Their Efficient Use. International Ferlizers Industry Association. Available online at: www.fertilizers.org.
Hassimi, M. S., Boukeskass, A., Adamou, I., Zoubeirou, A., Mayaki, O. Y., and Hafidi, M. (2017). Characterization of thermophilic phosphate solubilizing microorganisms during grassland anaerobic biotransformation. Int. J. Biol. Chem. Sci. 11, 2764–2777. doi: 10.4314/ijbcs.v11i6.17
Herrmann, L., and Lesueur, D. (2013). Challenges of formulation and quality of biofertilizers for successful inoculation. Appl. Microbiol. Biotechnol. 97, 8859–8873. doi: 10.1007/s00253-013-5228-8
Hungria, M., Loureiro, M. F., Mendes, I. C., Campo, R. J., and Graham, P. H. (2005). “Inoculant preparation, production and application,” in Nitrogen Fixation in Agriculture, Forestry, Ecology and the Environment. Series: Nitrogen Fixation: Origins, Applications, and Research Progress, eds D. Werner, W. E. Newton (Amsterdam: Springer), 223–254.
Igarashi, Y., Iida, T., Yoshida, R., and Furumai, T. (2002) Pteridic acids A B, novel plant growth promoters with auxin-like activity from Streptomyces hygroscopicus TP-A0451. J. Antibiot 55, 64–767. doi: 10.7164/antibiotics.55.764
Islam, M. K., Sano, A., Majumder, M. S. I., Hossain, M. A., and Sakagami, J. I. (2019). Isolation and molecular characterization of phosphate solubilizing filamentous fungi from subtropical soils in Okinawa. Appl. Ecol. Env. Res. 17, 9145–9157. doi: 10.15666/aeer/1704_91459157
Jog, R., Nareshkumar, G., and Rajkumar, S. (2016). “Enhancing soil health and plant growth promotion by actinomycetes,” in Plant Growth Promoting Actinobacteria, eds S. Gopalakrishnan, A. Sathya, R. Vijayabharathi (Singapore: Springer), 33–45. doi: 10.1007/978-981-10-0707-1_3
Kalakoutski, L. V., and Agre, N. S. (1976). Comparative aspects of development and differentiation in actinomycetes. Bacteriol. Rev. 40, 469–524.
Keane, E. (2009). Taking Stock of phosphorus and Biofuels, Seeking Alpha. Available online at: http://seekingalpha.com/article/182522-taking-stock-of-phosphorus-and-biofuels.
Kecskés, M. L., Choudhury, A. T. M. A., Casteriano, A. V., Deake, R., and Kennedy, I. R. (2009). Detection and enumeration of plant growth-promoting bacteria. Eng. Life Sci. 9, 431–436. doi: 10.1002/elsc.200800101
Khan, M. S., Zaidi, A., and Wani, P. A. (2007). Role of phosphate-solubilizing microorganisms in sustainable agriculture - A review. Agron. Sustain. Dev. 27, 29–43. doi: 10.1051/agro:2006011
Krsek, M., Morris, N., Egan, S., and Wellington, E. M. H. (2000). “Actinomycetes,” in Encyclopedia of Microbiology, 2nd Edn. ed J. Lederberg (New York, NY: Academic Press), 2841.
Kumari, K., and Phogat, V. K. (2008). Rock phosphate: its availability and solubilization in the soil – a review. Agric. Rev. 29, 108–116.
Labeda, D. P. (2011). Multilocus sequence analysis of phytopathogenic species of the genus Streptomyces. Int. J. Syst. Evol. Microbiol. 61, 2525–2531. doi: 10.1099/ijs.0.028514-0
Latha, P., Jeyaraman, and Prabakaran, R. (2014). Study effects of microbial and chemical fertilizer on yield components in Brinjal (Solanum melongena Linn.) C.Var CO-2. Int. J. Curr. Microbiol. App. Sci 3, 817–822.
Lazzarini, A., Cavaletti, L., Toppo, G., and Marinelli, F. (2000). Rare genera of actinomycetes as potential producers of new antibiotics. Antonie Van Leeuwenhoek 78, 399–405. doi: 10.1023/A:1010287600557
Leghari, S. J., Buriro, M., Jogi, Q., Kandhro, M. N., and Leghari, A. J. (2016). Depletion of phosphorus reserves, a big threat to agriculture: challenges and opportunities. Sci. Int. 28, 2697–2702.
Majeed, A., Abbasi, M. K., Hameed, S., Imran, A., and Rahim, N. (2015). Isolation and characterization of plant growth-promoting rhizobacteria from wheat rhizosphere and their effect on plant growth promotion. Front. Microbiol. 6:198. doi: 10.3389/fmicb.2015.00198
Malusá, E., Sas-Paszt, L., and Ciesielska, J. (2012). Technologies for beneficial microorganisms inocula used as biofertilizers. Sci. World J. (2012) 12:491206. doi: 10.1100/2012/491206
Malusá, E., and Vassilev, N. (2014). A contribution to set a legal framework for biofertilisers. Appl. Microbiol. Biotechnol. 98, 6599–6607. doi: 10.1007/s00253-014-5828-y
Manashi, B., Paul, S., Choudhury, A. G., Roy, P., and Sen, J. (2017). Biofertilizer as prospective input for sustainable agriculture in India. Int. J. Curr. Microbiol. App. Sci. 6, 1177–1186. doi: 10.20546/ijcmas.2017.611.141
Market data forecast. (2018). Available online at: https://www.marketdataforecast.com/market-reports/asia-pacific-biofertilizers-market (accessed August 2019).
Martinez, J. L. (2008) Antibiotics and antibiotic resistance genes in natural environments. Science 321, 365–367. doi: 10.1126/science.1159483.
Mba, C. C. (1994). Field studies on two rock phosphate solubilizing actinomycete isolates as biofertilizer sources. Environ. Manage. 18, 263–269. doi: 10.1007/BF02393767
Mengual, C., Schoebitz, M., Caravaca, F., and Roldán, A. (2016) Assessment of the potential role of Streptomyces strains in the revegetation of semiarid sites: the relative incidence of strain origin plantation site on plant performance soil quality indicators. Biol. Fertil. Soils 52, 53–64. doi: 10.1007/s00374-015-1052-x
Mishra, J., and Arora, N. K. (2016). “Bioformulations for plant growth promotion and combating phytopathogens: a sustainable approach,” in Bioformulations: for Sustainable Agriculture, eds N. K. Arora, S. Mehnaz, R. Balestrini (New Delhi: Springer), 3–33. doi: 10.1007/978-81-322-2779-3_1
Mishra, J., Prakash, J., and Arora, N. K. (2017). Role of beneficial soil microbes in sustainable agriculture and environmental management. Climate Change Environ. Sustain. 4, 137–149. doi: 10.5958/2320-642X.2016.00015.6
Mohammadi, K., and Sohrabi, Y. (2012). Bacterial biofertilizers for sustainable CROP production: a review. ARPN J. Agric. Biol. Sci. 7:5.
Molet-Rodríguez, A., Salvia-Trujillo, L., and Martín-Belloso, O. (2018). Beverage emulsions: key aspects of their formulation and physicochemical stability. Beverages 4:70. doi: 10.3390/beverages4030070
Mondal, T., Datta, J. K., and Mondal, N. K. (2015). Chemical fertilizer in conjunction with biofertilizer and vermicompost induced changes in morpho-physiological and bio-chemical traits of mustard crop. J. Saudi Soc. Agricult. Sci. 16, 135–144. doi: 10.1016/j.jssas.2015.05.001
Munda, S., Shivakuma, B. G., Gangaiah, B., Manjaiah, K. M., Rana, D. S., Layek, J., et al. (2015). Influence of direct and residual phosphorus fertilization on growth and yield of potato in a soybean-potato cropping system. AJCS 9, 191–202.
Nafis, A., Raklami, A., Bechtaoui, N., Khalloufi, F. E., Alaoui, A. E., Glick, B. R., et al. (2019). Actinobacteria from extreme niches in morocco and their plant growth-promoting potentials. Diversity 11:139. doi: 10.3390/d11080139
Naher, U. A., Panhwar, Q. A., Othman, R., Ismail, M. R., and Berahim, Z. (2016). Biofertilizer as a supplement of chemical fertilizer for yield maximization of rice. J. Agric. Food Dev. 2, 16–22. doi: 10.30635/2415-0142.2016.02.3
Nandimath, A. P., Karad, D. D., Gupta, S. G., and Kharat, A. S. (2017). Consortium inoculum of five thermo-tolerant phosphate solubilizing Actinomycetes for multipurpose biofertilizer preparation. Iran. J. Microbiol. 9, 295–304.
Nath, M., Bhatt, D., Bhatt, M. D., Prasad, R., and Tuteja, N. (2018). Microbe-mediated enhancement of nitrogen and phosphorus content for crop improvement. N. Fut. Dev. Microb. Biotechnol. Bioeng. 2018, 293–304. doi: 10.1016/B978-0-444-63987-5.00014-1
Ndoye, I., Soumaré, A., Agbangba, E. C., Thioulouse, J., Galiana, A., Prin, Y., et al. (2013). A new process to promote the use of controlled mycorrhization practice in forest nurseries. Afr. J. Agric. Res. 8, 308–313. doi: 10.5897/AJAR12.1642
Okur, N. (2018). A review: bio-fertilizers- power of beneficial microorganisms in soils. Biomed. J. Sci. Tech. Res. 4:BJSTR. MS.ID.001076. doi: 10.26717/BJSTR.2018.04.0001076
Olanrewaju, O. S., and Babalola, O. O. (2019). Streptomyces: implications and interactions in plant growth promotion. Appl. Microbiol. Biotechnol. 103, 1179–1188. doi: 10.1007/s00253-018-09577-y
Pathak, D., and Kumar, M. (2016). “Microbial inoculants as biofertilizers and biopesticides,” in Microbial Inoculants in Sustainable Agricultural Productivity, eds D. Pratap Singh, H. Bahadur Singh, and R. Prabha (Berlin: Springer), 197–209. doi: 10.1007/978-81-322-2647-5_11
Polti, M. A., Aparicio, J. D., Benimeli, C. S., and Amoroso, M. J. (2014). “Role of actinobacteria in bioremediation,” in Microbial Biodegradation and Bioremediation, ed S. Das, editor. (New York, NY: Elsevier), 269–286. doi: 10.1016/B978-0-12-800021-2.00011-X
Pudi, N., Varikuti, G. D., Badana, A. K., Gavara, M. M., Seema, K., and Malla, R. (2016) Studies on optimization of growth parameters for enhanced production of antibiotic alkaloids by isolated marine actinomycetes. J. App. Phar. Sci. 6, 181–188, doi: 10.7324/JAPS.2016.601025
Qu, K., Guo, F., Liu, X., Lin, Y., and Zou, Q. (2019). Application of machine learning in microbiology. Front. Microbiol. 10:827. doi: 10.3389/fmicb.2019.00827
Raimi, A., Adeleke, R., and Roopnarain, A. (2017). Soil fertility challenges and Biofertiliser as a viable alternative for increasing smallholder farmer crop productivity in sub-Saharan Africa. Cogent Food Agricult. 3:1400933. doi: 10.1080/23311932.2017.1400933
Rampelotto, P. H. (2010). Resistance of microorganisms to extreme environmental conditions and its contribution to astrobiology. Sustainability 2, 1602–1623. doi: 10.3390/su2061602
Rashad, F. M., Fathy, H. M., El-Zayat, A. S., and Elghonaimy, A. M. (2015) Isolation characterization of multifunctional Streptomyces species with antimicrobial, nematicidal phytohor-mone activities from marine environments in Egypt. Microbiol. Res. 175, 34–47 doi: 10.1016/j.micres.2015.03.002
Rashida, M. I., Mujawar, L. H., Shahzade, T., Almeelbi, T., Ismail, I. M. I., and Oves, M. (2016). Bacteria and fungi can contribute to nutrients bioavailability and aggregate formation in degraded soils. Microbiol. Res. 183, 26–41. doi: 10.1016/j.micres.2015.11.007
Reddy, C., and Saravanan, R. (2013). Polymicrobial multi-functional approach for enhancement of crop productivity. Adv. Appl. Microbiol. 82, 53–113. doi: 10.1016/B978-0-12-407679-2.00003-X
Reddypriya, P., Selastin, A. R., Gopalaswamy, G., and Balachandar, D. (2016). Development of sequence-characterized amplified region (SCAR) markers as a quality standard of inoculants based on Azospirillum. Arch. Microbiol. 198, 257–67. doi: 10.1007/s00203-016-1187-7
Reddypriya, P., Soumare, A., and Balachandar, D. (2019). Multiplex and quantitative PCR targeting SCAR markers for strain-level detection and quantification of biofertilizers. J. Basic Microbiol. 2019, 111–119. doi: 10.1002/jobm.201800318
Reponen, T. A., Gazenko, S. V., Grinshpun, S. A., Willeke, K., and Cole, E. C. (1998). Characteristics of airborne actinomycete spores. Appl. Environ. Microbiol. 64, 3807–3812
Saeid, A. (2018). “Phosphorus microbial solubilization as a key for phosphorus recycling in agriculture,” in Phosphorus Recovery and Recycling, ed Z. Tao (Chorwacja: InTechOpen). doi: 10.5772/intechopen.81487
Sane, S. A., and Mehta, S. K. (2015). Isolation and evaluation of rock phosphate solubilizing fungi as potential bio-fertilizer. J. Fertil. Pestic. 6:156. doi: 10.4172/2471-2728.1000156
Sathya, A., Vijayabharathi, R., and Gopalakrishnan, S. (2017) Plant growth-promoting actinobacteria: a new strategy for enhancing sustainable production protection of grain legumes. 3 Biotech. 7:102. doi: 10.1007/s13205-017-0736-3
Schrey, S. D., and Tarkka, M. T. (2008) Friends foes: streptomycetes as modulators of plant disease symbiosis. Antonie van Leeuw. 94, 11–19. doi: 10.1007/s10482-008-9241-3
Shang, Y., Hasan Md, K., Ahammed, G. J., Li, M., Yin, H., and Zhou, J. (2019). Applications of nanotechnology in plant growth and crop protection: a review. Molecules 24:2558. doi: 10.3390/molecules24142558
Sharma, M., Dangi, P., and Choudhary, M. (2014). Actinomycetes: source, identification, and their applications. Int. J. Curr. Microbiol. App. Sci. 3, 801–83.
Sharma, S. B., Sayyed, R. Z., Trivedi,1, M. H., and Gobi, T.A. (2013). Phosphate solubilizing microbes:sustainable approach for managing phosphorus deficiency in agricultural soils. Springerplus 2013:587. doi: 10.1186/2193-1801-2-587
Shepherd, M. D., Kharel, M. K., Bosserman, M. A., and Rohr, J. (2010). Laboratory maintenance of Streptomyces species. Curr Protoc Microbiol. 10:Unit−10E.1. doi: 10.1002/9780471729259.mc10e01s18
Shravani, K., Triveni, S., Latha, P. C., Ramulu, V., Tejashree, M., and Damodharachari, K. (2019). Influence of biofertilizers under drip fertigation system on NPK uptake and yield performance of greengram (Vigna radiata L.) Int. J. Curr. Microbiol. Appl. Sci. 8, 2409–2417. doi: 10.20546/ijcmas.2019.805.285
Shutsrirung, A., Chromkaew, Y., Pathom-Aree, W., Choonluchanon, S., and Boonkerd, N. (2013). Diversity of endophytic actinomycetes in mandarin grown in northern Thailand, their phytohormone production potential and plant growth promoting activity. Soil Sci. Plant Nutr. 59, 322–330. doi: 10.1080/00380768.2013.776935
Sivasakthivelan, P., and Saranraj, P. (2013). Azospirillum and its formulations: a review. Inter. J. Microbiol. Res. 4, 275–287. doi: 10.5829/idosi.ijmr.2013.4.3.825
Soares, A. C. F., Sousa, C. S., Garrido, M. D., and Perez, J. O. (2007). Production of streptomycete inoculum in sterilized rice. Sci. Agric. 64, 641–644. doi: 10.1590/S0103-90162007000600013
Solanki, M. K., Kumar, S., Pandey, A. K., Srivastava, S., Singh, R. K., Kashyap, P. L., et al. (2012). Diversity and antagonistic potential of Bacillus spp. associated to the rhizosphere of tomato for the management of Rhizoctonia solani. Biocontrol SC. Techn. 22, 203–217. doi: 10.1080/09583157.2011.649713
Soumare, A., Wade, M., Do Rego, F., Fall, S., and Wade, T. K. (2017). Preliminary screening of cowpea-Bradyrhizobium symbiotic traits subjected to salinity stress in Senegal. Afr. J. Agric. Res. 12, 3233–3243. doi: 10.5897/AJAR2017.12662
Sousa, J. A. J., and Olivares, F. L. (2016) Plant growth promotion by streptomycetes: ecophysiology, mechanisms applications. Chem. Biol. Technol. Agric. 3:24 doi: 10.1186/s40538-016-0073-5
Srivastava, S., Patel, J. S., Singh, H. B., Sinha, A., and Sarma, B. K. (2015) Streptomyces rochei SM3 induces stress tolerance in chickpea against Sclerotinia sclerotiorum and NaCl. J. Phytopathol. 163, 583–592. doi: 10.1111/jph.12358
Stamenković, S., Beškoski, V., Karabegović, I., Lazić, M., and Nikolić, N. (2018). Microbial fertilizers: a comprehensive review of current findings and future perspectives. Spanish J. Agric. Res. 16:e09R01. doi: 10.5424/sjar/2018161-12117
Suleman, M., Yasmin, S., Rasul, M., Yahya, M., Atta, B. M., and Mirza, M. S. (2018). Phosphate solubilizing bacteria with glucose dehydrogenase gene for phosphorus uptake and beneficial effects on wheat. PLoS ONE 13:e0204408. doi: 10.1371/journal.pone.0204408
Thuita, M., Vanlauwe, B., Mutegi, E., and Masso, C. (2018). Reducing spatial variability of soybean response to rhizobia inoculans in farms of variable soil fertility in Siaaya Country of west Kenya. Agr. Ecosyst. Environ. 261, 153–160. doi: 10.1016/j.agee.2018.01.007
Transparency Market Research (2014). Biofertilizers (Nitrogen Fixing, Phosphate Solubilizing and Others) Market for Seed Treatment and Soil Treatment Applications – Global Industry Analysis, Size, Share, Growth, Trends and Forecast, 2013-2019. Albany, NY: Transparency Market Research.
Umesha, S., Singh, P. K., and Singh, R. P. (2018). “Microbial biotechnology and sustainable agriculture,” in Biotechnology for Sustainable Agriculture, eds R. L. Singh and S. Monda (Sawston: Woodhead Publishing), 185–205. doi: 10.1016/B978-0-12-812160-3.00006-4
Vasconcellos, R. L. F., Silva, M. C. P., Ribeiro, C. M. R., and Cardoso, E. J. B. N. (2010). Isolation and screening for plant growth-promoting (PGP) actinobacteria from Araucaria angustifolia rhizosphere soil. Sci. Agric. 67, 743–746. doi: 10.1590/S0103-90162010000600019
Vaxevanidou, K., Christou, C., Kremmydas, G. F., Georgakopoulos, D. G., and Papassiopi, N. (2015). Role of indigenous arsenate and iron (III) Respiring microorganisms in controlling the mobilization of arsenic in a Contaminated Soil Sample. Bull. Environ. Cont. Toxicol. 94, 282, 282–288. doi: 10.1007/s00128-015-1458-z
Viani, R. A., Rodrigues, R. R., Dawson, T. E., Lambers, H., and Oliveira, R. S. (2014). Soil pH accounts for differences in species distribution and leaf nutrient concentrations of Brazilian woodland savannah and seasonally dry forest species. Perspect. Plant Ecol. Evol. Syst. 16, 64–74 doi: 10.1016/j.ppees.2014.02.001
Vurukonda, S. S. K. P., Giovanardi, D., and Stefani, E. (2018) Plant Growth Promoting Biocontrol Activity of Streptomyces spp. as Endophytes. Int. J. Mol. Sci. 19:952. doi: 10.3390/ijms19040952
Xiang, W., Zhao, L., Xu, X., Qin, Y., and Yu, G. (2012). Mutual information flow between beneficial microorganisms and the roots of host plants determined the bio-functions of biofertilizers. Am. J. Plant Sci. 3, 1115–1120. doi: 10.4236/ajps.2012.38134
Xie, W., Alison, J. N., and Zisserman, A. (2018). Microscopy cell counting and detection with fully convolutional regression networks. Comput. Methods Biomech. Biomed. Eng. 6, 283–292. doi: 10.1080/21681163.2016.1149104
Yadav, K. K., and Sarka, S. (2019). Biofertilizers, impact on soil fertility and crop productivity under sustainable agriculture. Environ. Ecol. 37, 89−93.
Keywords: Actinobacteria biotechnology, bioformulation, phosphorous, rock phosphate, quality standards
Citation: Soumare A, Boubekri K, Lyamlouli K, Hafidi M, Ouhdouch Y and Kouisni L (2020) From Isolation of Phosphate Solubilizing Microbes to Their Formulation and Use as Biofertilizers: Status and Needs. Front. Bioeng. Biotechnol. 7:425. doi: 10.3389/fbioe.2019.00425
Received: 20 July 2019; Accepted: 02 December 2019;
Published: 09 January 2020.
Edited by:
Lucia Gardossi, University of Trieste, ItalyReviewed by:
Vijay Singh Meena, ICAR-Vivekananda Parvatiya Krishi Anusandhan Sansthan, IndiaSumera Yasmin, National Institute for Biotechnology and Genetic Engineering, Pakistan
Copyright © 2020 Soumare, Boubekri, Lyamlouli, Hafidi, Ouhdouch and Kouisni. This is an open-access article distributed under the terms of the Creative Commons Attribution License (CC BY). The use, distribution or reproduction in other forums is permitted, provided the original author(s) and the copyright owner(s) are credited and that the original publication in this journal is cited, in accordance with accepted academic practice. No use, distribution or reproduction is permitted which does not comply with these terms.
*Correspondence: Abdoulaye Soumare, abdoulaye.soumare@um6p.ma