Theranostics Based on Magnetic Nanoparticles and Polymers: Intelligent Design for Efficient Diagnostics and Therapy
- 1Department of Chemistry, Indiana University, Bloomington, IN, United States
- 2Department of Physics, Faculty of Science, King Abdulaziz University, Jeddah, Saudi Arabia
- 3A.N. Nesmeyanov Institute of Organoelement Compounds, Russian Academy of Sciences, Moscow, Russia
Theranostics is a fast-growing field due to demands for new, efficient therapeutics which could be precisely delivered to the target site using multimodal imaging with enhancing auxiliary actions. In this review article we discuss theranostic nanoplatforms containing polymers and magnetic nanoparticles along with other components. Magnetic nanoparticles allow for both diagnostic and therapeutic (hyperthermia) capabilities, while polymers can be reservoirs for drugs and are easily functionalized for cell targeting. We focus on the most important design strategies to achieve optimal theranostic effects as well as the roles of different components included in theranostics, reviewing the literature from the last 5 years.
Introduction
Over the years drug delivery systems have become so sophisticated that they are capable of combining therapeutic action from a drug or an external field with imaging capabilities such as magnetic resonance imaging (MRI), magnetic particle imaging (MPI), near infrared (NIR), and photoacoustic imaging (PAI), etc. The combination of imaging with therapy, called theranostics, allows for more precise delivery of a treatment, thus, increasing its efficacy. To fabricate theranostic drug delivery vehicles, one needs to combine in a single design the capacity to adsorb and release a drug, imaging properties, as well as some enhancing actions. We believe for the development of such efficient nanoplatforms, a combination of a polymer and magnetic nanoparticles (NPs), is favorable as the polymer can provide a reservoir for drugs and a platform for additional functionalization (for cell targeting or imaging), while magnetic NPs allow for MRI, MPI and hyperthermia—a cancer treatment which can be combined with a drug action. For the last 5 years, several review articles have been published discussing applications of hybrid nanomaterials based on bifunctional proteins, functional, and conducting polymers, dendrimers, biopolymers, etc. for diagnostics and therapies of serious diseases including cancer (Bonilla and Gonzalez, 2017; Deyev and Lebedenko, 2017; Kudr et al., 2017; Niu et al., 2017; Liu et al., 2018; Ray et al., 2018; Srinivasan et al., 2018; Aisida et al., 2019). Polymer-assisted magnetic NP assemblies showed a significant promise due to their controllable magnetic properties and collective functions (Li et al., 2020). Despite these excellent reviews, we believe there is a gap in understanding of key design elements needed to fulfill the desired theranostic functions for real life applications. In this review, we discuss the design of the most promising theranostics containing polymer and magnetic nanoparticles, allowing for the best combination of properties and possibilities of practical applications, analyzing literature from the last 5 years.
Design Strategies
The major design strategies are 3-fold, focusing on (i) magnetic NPs with an enhanced magnetic response, (ii) a combination of magnetic and Au NPs, and (iii) sophisticated engineering solutions for fabrication of shells/containers.
Magnetic NPs With Improved Magnetic Response
A typical way for increasing a magnetic response is iron oxide NP clustering which is discussed in section Nanoprecipitation of a Polymer and NPs. The other methods focus on varying a magnetic phase composition. A significantly improved magnetic response in terms of MRI and hyperthermia was achieved by combining soft magnetic phase (MnFe2O4 NPs) and hard magnetic phase (CoFe2O4 NPs) in bi-magnetic NP clusters (NPCs) stabilized by a biocompatible sodium dodecyl sulfate polymer (Vamvakidis et al., 2018). Paramagnetic ultrasmall NPs of Prussian blue [Fe3+(Fe2+(CN)6)] types containing Gd3+ ions in their exterior showed exceptional longitudinal relaxivities > 40 mM−1 s−1 per Gd3+ independently of the polymer shell (Fetiveau et al., 2019). In addition, a great photothermal effect and PAI of tumors in vivo were achieved.
A Combination of Magnetic and Au NPs
Janus particles composed of trisoctahedral magnetite NPs and Au NPs coated with poly-L-lysine yielded a single nanoprobe with increased stability in vivo and efficient photothermal tumor ablation (due to gold) as well as enhanced MRI contrast properties due to the polymer layer (Abedin et al., 2018). Each constituent of these multicomponent particles plays an important role in theranostic applications. Other examples of a combination of gold and magnetic NPs were reported by Cherkasov et al. (2020), Wang et al. (2018), and (Sun et al., 2016).
Engineering Design
Zhang et al. developed sophisticated yolk-shell particles (YSPs) from simple components such as poly(ε-caprolactone) (PCL), silicone oil, and magnetite NPs (MNP) using tri-needle coaxial electrospray engineering to create a Fe3O4 NP-PCL shell surrounding an interfacing silicone oil layer, located around a PCL core (Figure 1; Zhang C. et al., 2017). The authors illustrated a possible simultaneous encapsulation and delivery of different drugs using the separate compartments of the YSPs. This situation was simulated using Nile blue (NB) and acridine yellow (AY)—model hydrophilic compounds—and Sudan red G (SRG) as a model for a hydrophobic drug. Dual-mode resonance (ultrasonic and magnetic) as well as multiple mechanisms of drug release (inversion, applied magnetic field, acoustic waves) and the possibility of using different polymers and NPs make these theranostics especially promising as universal systems for therapy and diagnostic.
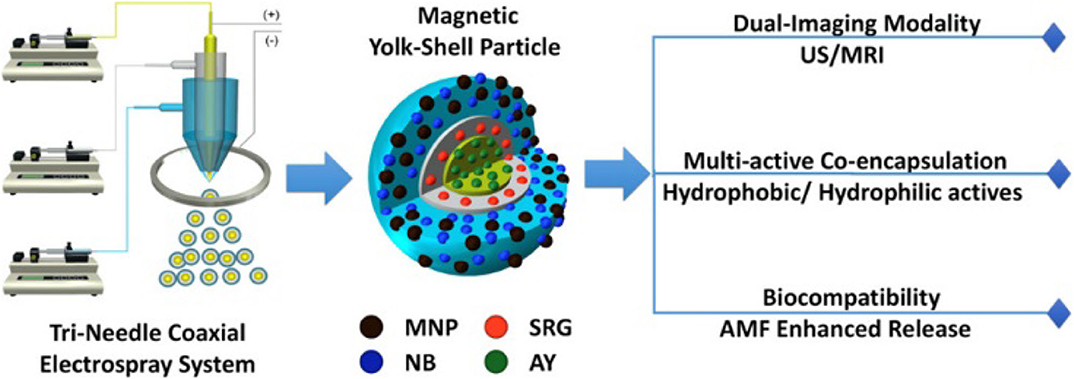
Figure 1. Schematic representation of tri-needle coaxial electrospray system for developing YSPs (Zhang C. et al., 2017). Reproduced with the permission of the copyright holder (American Chemical Society).
High-power ultrasound was utilized to develop theranostics for gene silencing capabilities via a one-pot method (Israel et al., 2016). The NPs were functionalized with a polyethylene imine (b-PEI25) and a G2 PAMAM dendrimer biopolymer for attachment of small interfering RNA (siRNA) and decreasing cytotoxicity. This resulted in effective gene silencing (90–95%) combined with T2 MRI capabilities.
Polymeric Component
Coating of Magnetic NPs With a Polymer
An in-situ surface polymerization was developed to coat magnetite NPs with polypyrrole as well as poly(3,4-ethylenedioxythiophene):poly(4-styrenesulfonate; PEDOT:PSS; Yan et al., 2017). A conjugated polymer here allows for NIR absorbance. Powerful multimodal imaging capabilities of the NPs were utilized through PAI and MRI, while efficient hyperthermia resulted in effective tumor-ablation in mice.
Polyarabic acid, which is a major component of acacia gum, is biocompatible and facilitates penetration of cell membranes. Coating of magnetite NPs with polyarabic acid followed by further functionalization and DOX loading allowed fabrication of theranostic nanosystems with excellent cell penetration, DOX uptake, and pH sensitive DOX release in breast cancer tumor cells (Patitsa et al., 2017). Additionally, the NPs demonstrated great biocompatibility, minimal cytotoxicity, and contrasting properties comparable to current commercial agents. Coating with poly(ethylene glycol) (PEG), dextran, and chitosan allowed for better control over the polymer coat properties, enhancing both MRI and hyperthermia applications (Zahraei et al., 2016).
A novel nanotheranostic platform was developed from magnetite NPs conjugated to cyclodextrin polymer nanosponges, further functionalized with folic acid (FA) (Gholibegloo et al., 2019). The drug curcumin was then loaded into the lipophilic central cavity of cyclodextrins and a magnetic field was applied to guide the system toward the tumor whose acidic pH causes release of the drug.
A core-shell magneto-fluorescent nanogel was developed with iron oxide NP cores and a photoluminescent shell, where comacromers [PEG-maleic acid-glycine] were linked together by a crosslinker (Vijayan et al., 2019). The nanogel was found to possess high cytocompatibility, good cellular uptake, as well as fluorescent imaging and hyperthermia treatment capabilities. Crosslinking was utilized in another nanotheranostic platform to combine α-lactalbumin to itself via PEG and glutaraldehyde (Delavari et al., 2019). The resulting polymer was then attached to magnetite NPs using PEI. The redox sensitive protein complex holding DOX allowed for targeted release of the chemotherapeutic agent once in the acidic environment of a tumor.
In another example, a thermo-responsive fluorescent polymer (TFP) was conjugated to the surface of iron oxide magnetic NPs for use as a degradable and non-toxic theranostic agent (Pandey et al., 2020). For this, poly(N-isopropylacrylamide) (PNIPAM), allylamine and a fluorescent polymer were copolymerized and conjugated to NPs via free radical polymerization to form TFP-NPs. In vitro studies demonstrated low cytotoxicity and high biocompatibility of DOX loaded polymer-NP conjugates.
Yan et al. showed that the thickness of the PNIPAM coat around iron oxide NPs plays an important role in the drug uptake and MRI properties (Yan et al., 2017). It was established that thicker layers of PNIPAM translated to a higher uptake of DOX but subsequently led to a slower release and longer times to reach the target area during magnetic drug delivery.
Nanoprecipitation of a Polymer and NPs
A pH switch nanoprecipitation method has been utilized for encapsulation of iron oxide NPs by a copolymer-drug conjugate including a tumor homing peptide (iRGD) with tumor specificity (Herranz-Blanco et al., 2016). The nanosystem showed increased lysosomal escape due to presence of poly(histidine) via the proton sponge effect and subsequent release of the polymer into the cytoplasm. The magnetic response was strong due to clustering, allowing for magnetic guided therapy. Additionally, intracellular cleavage of the DOX-polymer linkage was observed, allowing for efficient DOX delivery and accumulation in the nuclei of tumor cells.
Micelles prepared by nanoprecipitation of poly-(N-ε-carbobenzyloxy-L-lysine) grafted hyaluronan copolymer in the presence of iron oxide NPs and DOX were designed for targeted tumor diagnosis and treatment (Yang et al., 2020). The copolymer used in coating consisted of polysaccharides and polypeptides linked by disulfide bonds which, once reduced by the high concentration of glutathione (GSH) in the tumor tissue, released DOX. In vitro studies using HepG2 cells showed accelerated drug release over a 24 h period from these redox-sensitive theranostic NPs and increased intracellular uptake under a high GSH concentration, which mimics tumor cell conditions.
Self-Assembly in the Formation of Smart Materials
A NP beacon based on gold and ferrihydrite NPs coated with a flexible polymer (custom designed amino-group terminated oligonucleotides with terminal biotin) was fabricated by conjugation using streptavidin and DNA to form a highly sensitive input-switchable structure (Cherkasov et al., 2020). This structure controls accessibility of the terminal receptor for binding to the target (Figure 2). Here, DNA serves as a molecular cue to cause the smart material to undergo a serious of transformations allowing them to bind to cancer cells and initiate drug release. Biotin affinity to the gold surface shielded it from interaction until the specific input DNA turned the complex on so that biotin could interact with the target (streptavidin). These amazing constructs demonstrate an unusual signal transmittance between a biochemical cue (input) and a nanoprobe (targeting receptor) via surface polymer interactions and hold promise of remarkable future applications.
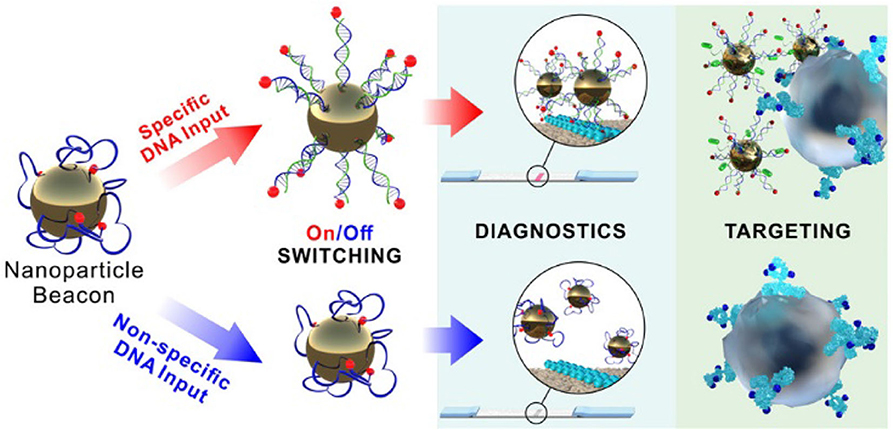
Figure 2. Schematic representation of the NP beacon action (Cherkasov et al., 2020). Reproduced with the permission of the copyright holder (American Chemical Society).
Surface Modifications
Fighting Phagocytosis With Self-Peptide
The mononuclear phagocyte system (MPS) poses as a barrier to NP delivery systems in vivo, and most delivery vehicles use a “passive stealth” approach through the presence of PEG or zwitterionic polymers, which delay the coating of nanovehicles with serum proteins and thus phagocytosis by the MPS. However, in the body, cell surface protein CD47 displayed on host cells interacts with phagocyte surface receptors, thus promoting anti-phagocytosis and acting as a “self-peptide” for the MPS to distinguish host from foreign particles. Zhang et al. explored an assembly of biodegradable poly(lactide-glycolide)-PEG (PLGA-PEG) with synthetic CD47 self-peptide for interactions with the SIRPα receptor expressed on phagocytes, i.e., an “active stealth” approach within the body (Zhang K.-L. et al., 2017). Also, iron oxide NPs absorbed anticancer drug through self-assembly into micelles, allowing for increased blood circulation, imaging, and drug delivery in vivo.
Modification for Tumor Targeting
Functionalization of core-shell iron oxide NP-polymer constructs with FA allows for enhanced targeting and uptake in tumor cells via FA receptor mediated endocytosis (Huang et al., 2017; Roy et al., 2017; Gholibegloo et al., 2019). Functionalization with lysine and cysteine derivatives allowed for enhanced tumor cell ligand targeting and uptake (Wei et al., 2017). A tissue-type plasminogen activator peptide was employed for modification of a magnetic construct and targeting pancreatic cancer cells (Dobiasch et al., 2016).
Use of Surfactant for Crossing Blood Brain Barrier
The blood brain barrier (BBB) often prevents crossing of theranostic agents into the brain, and thus poses a significant hurdle for any potential anticancer treatment targeting brain tumors. Iron oxide NPs modified with PEG, PEI, and Tween 80 (non-ionic surfactant) along with an applied magnetic field allowed for active penetration of the BBB in vivo, suggesting future potential for theranostic delivery through the BBB via Tween-NP conjugates (Huang et al., 2016). Other polymer based surfactants such as Brij-35, Pluronic F68, and Vitamin E-TPGS improved passage through BBB allowing for access to brain tumors such as glioblastoma (Luque-Michel et al., 2019).
It is noteworthy that lyophilization was efficiently used for preservation of theranostics with zero effect on physical, chemical, and magnetic properties after prolonged storage (Yang et al., 2017; Luque-Michel et al., 2019).
Diagnostics
MRI and MPI
Theranostic nanoplatforms with iron oxide NPs are normally utilized as T2 (negative) MRI contrast agents. Considering that MRI measures a proton response, NPs with higher magnetization and higher loading of water protons in a polymeric shell make a better MRI contrast agent (Liao et al., 2017; Yang et al., 2020). Polymer-NP constructs containing Gd3+ complexes (Roy et al., 2016; Fetiveau et al., 2019) or Fe3+- terpyridine complexes (Patra et al., 2018) can provide highly efficient T1 (positive) MRI contrast.
MPI—a comparatively recent imaging method—is based on a direct quantification of spatial distribution of iron oxide NPs in a tissue as a magnetization response to a magnetic field. It provides a higher contrast than that of MRI. A polymer-NP system showed promising MPI contrast properties (Rost et al., 2020).
Fluorescence Imaging
Normally, fluorescence imaging is performed along with MRI by using a fluorescently labeled polymer (Pandey et al., 2020). For example, functionalization of iron oxide NPs with the two- photons fluorescent dye labeled polymer yielded a nanoprobe capable of quantifying pancreatic Beta cell mass (BCM), an effective indicator of onset of type 2 diabetes (Xin et al., 2020). Dye release was achieved via the acidic environment of the Beta pancreatic cells, thus allowing for fluorescence detection of Beta cells via confocal one-photon and two-photon microscopy. Furthermore, the nanoprobe displayed powerful inhibition of the toxic aggregation of human islet amyloid polypeptide, the suspected cause of Beta cell degeneration observed with type 2 diabetes. Magneto-fluorescent nanogels discussed in Section Nanoprecipitation of a Polymer and NPs displayed dual emissions (red and green) in Hela cells under different excitation wavelengths showing that this nanogel has fluorescence imaging applications toward cancer cells (Vijayan et al., 2019).
Photoacoustic Imaging
PAI is a comparatively novel imaging modality, based on optical (laser) excitation and ultrasound imaging to detect sound waves (Attia et al., 2019). It is often combined with other imaging methods to impart multimodality and higher accuracy of imaging. Fetiveau et al. observed a strong photo-acoustic signal intratumorally after irradiation at ~808 nm of Gd-containing Fe3+[Fe2+(CN)6] NPs (Fetiveau et al., 2019). Lu et al. combined PAI, MRI and computer tomography utilizing dendrimer-stabilized nanoflowers containing Au and ultrasmall iron oxide NPs (Lu et al., 2018). Multimodality imaging including, PAI, MRI and fluorescence was demonstrated for fluorescent Janus nanostructures (Song et al., 2019).
Therapeutic Properties
Magnetic Delivery of Anti-cancer Agents
Multiblock polyurethanes (MPU) containing soft and hard polymer linkages formed iron oxide NPCs as a nanovehicle capable of magnetically targeted delivery in vivo (Wei et al., 2017). PEG connected by benzoic-imine linkage allowed for pH sensitive release of an anticancer drug in extracellular acidic environments. Overall, the MPU nanovehicle demonstrated powerful anticancer properties in vivo and MRI contrast properties.
Kumar et al. studied magnetic navigation steering capabilities of the nanostructures formed by maghemite NPs and thermoresponsive poly(2-ethyl-2oxazoline) in a synthetic blood vessel model (Kumar et al., 2018). High efficiency of magnetic steering navigation was achieved for all directions of blood flow studied, including angular, parallel, and antiparallel flow to the target destination combined with efficient release of anticancer drug (paclitaxel) at 41°C. Delavari et al. and Herranz-Blanco et al. also reported targeted delivery to tumor sites via magnetic guidance which allowed for increased DOX uptake in cancer cells (Herranz-Blanco et al., 2016; Delavari et al., 2019). This significantly improved cytotoxicity and apoptosis within the tumor while reducing those effects on healthy tissue.
Reduction of Hypoxia
Hypoxia is a key aspect of tumor cell environments, contributing increased resistance to anticancer therapies. Loading of porous hollow Fe3O4 NPs with a FDA approved oxygen carrier, perfluorocarbon, was performed to study potential for decreasing hypoxia induced resistance to these treatments (Zhou et al., 2019). Modification of these NPs with lactobionic acid-containing block copolymer allowed for enhanced targeting of tumor cells and uptake via cleavage of the hydrophilic PEG segment by intratumoral GSH. Effective release of oxygen was achieved, leading to a decrease in hypoxia induced tumor resistance.
Hyperthermia
Upon irradiation with an infrared laser or under the influence of an alternating magnetic field, many magnetic NPs undergo hyperthermia. It can be utilized in two major ways: enhanced drug release through the heat-expansion of a polymer coat (Zhang C. et al., 2017; Vijayan et al., 2019) and achieving temperatures above the minimum for inducing apoptosis (42°C) of surrounding cells (Thirunavukkarasu et al., 2018; Yar et al., 2018; Fetiveau et al., 2019) or by a combination of both methods (Vijayan et al., 2019). In all cases, once the nanomaterials had accumulated at the tumor site, they were triggered to reach required temperatures, resulting in a highly controlled and localized method for killing cancer cells.
Gene Silencing
An enhanced interest has been shown for utilizing gene silencing and RNA interference techniques for cancer treatment. However, RNA interference is often limited due to fast degradation of siRNA by endonucleases prior to cell uptake. Functionalization of iron oxide NPs with chitosan and siRNA was performed for MRI contrast and RNA interference capabilities (Bruniaux et al., 2017). The addition of PEG and poly-L-arginine coating significantly increased the biocompatibility and siRNA transfection of the nanoplatform. Additionally, chitosan allowed for endosomal escape and thus pH sensitive release of siRNA into the cytoplasm. The use of polymer coated magnetic NPs as carriers for siRNA for gene silencing therapy was also reported by Israel et al. (2016).
Summary and Outlook
What does the future hold in the field of magnetic theranostics? The literature shows that successful theranostics are based on three major principles: (i) multimodality of imaging allowing for high fidelity, (ii) efficacy of a therapeutic action often requiring additional functionalization for targeted delivery and an external field for an enhancement of the drug action, and (iii) potentials for robust scale up of the theranostic system production. The combination of all these factors can be realized in multicomponent systems, whose fabrication can be performed in a few steps. These requirements are met using tri-needle coaxial electrospray engineering resulting in YSPs. Moreover, this approach allows a wide variation of polymers, NPs, drugs, modifiers, etc., thus presenting the opportunity for a wide array of imaging modalities and therapeutic actions. Another promising fabrication strategy is self-assembly of multiple components into micelles (smart materials), where there is a molecular cue (for example, DNA), allowing one to trigger an ON-OFF mechanism of a therapeutic action. It is noteworthy that self-assembly is easy to scale up and to modify with various components.
At the same time, there is a number of shortcomings in this field, the major of which is a slow transition from laboratory studies to real world applications. This is often determined by high costs, fabrication issues, and the length of both preclinical and clinical studies. Thus, a concerted effort from scientists, engineers and clinicians is required to move the field forward.
Author Contributions
All authors listed have made a substantial, direct and intellectual contribution to the work, and approved it for publication.
Conflict of Interest
The authors declare that the research was conducted in the absence of any commercial or financial relationships that could be construed as a potential conflict of interest.
Acknowledgments
We thank the Department of Chemistry, Indiana University, for support of this work.
References
Abedin, M. R., Umapathi, S., Mahendrakar, H., Laemthong, T., Coleman, H., Muchangi, D., et al. (2018). Polymer coated gold-ferric oxide superparamagnetic nanoparticles for theranostic applications. J. Nanobiotechnol. 16:80. doi: 10.1186/s12951-018-0405-7
Aisida, S. O., Akpa, P. A., Ahmad, I., Zhao, T.-K., Maaza, M., and Ezema, F. I. (2019). Bio-inspired encapsulation and functionalization of iron oxide nanoparticles for biomedical applications. Eur. Polym. J. (Ahead of Print). doi: 10.1016/j.eurpolymj.2019.109371
Attia, A., Balasundaram, G., Moothanchery, M., Dinish, U. S., Bi, R., Olivo, M., et al. (2019). A review of clinical photoacoustic imaging: current and future trends. Photoacoustics 16:100144. doi: 10.1016/j.pacs.2019.100144
Bonilla, A. M., and Gonzalez, P. H. (2017). Hybrid polymeric-magnetic nanoparticles in cancer treatments. Curr. Pharm. Des. 23, 5392–5402. doi: 10.2174/1381612823666170519115601
Bruniaux, J., Ben Djemaa, S., Herve-Aubert, K., Marchais, H., Chourpa, I., and David, S. (2017). Stealth magnetic nanocarriers of siRNA as platform for breast cancer theranostics. Int. J. Pharm. (Amsterdam, Neth.) 532, 660–668. doi: 10.1016/j.ijpharm.2017.05.022
Cherkasov, V. R., Mochalova, E. N., Babenyshev, A. V., Vasilyeva, A. V., Nikitin, P. I., and Nikitin, M. P. (2020). Nanoparticle beacons: supersensitive smart materials with on/off-switchable affinity to biomedical targets. ACS Nano 14, 1792–1803. doi: 10.1021/acsnano.9b07569
Delavari, B., Bigdeli, B., Mamashli, F., Gholami, M., Bazri, B., Khoobi, M., et al. (2019). Theranostic α-lactalbumin-polymer-based nanocomposite as a drug delivery carrier for cancer therapy. ACS Biomater. Sci. Eng. 5, 5189–5208. doi: 10.1021/acsbiomaterials.9b01236
Deyev, S. M., and Lebedenko, E. N. (2017). Targeted bifunctional proteins and hybrid nanoconstructs for cancer diagnostics and therapies. Mol. Biol. (Moscow, Russ. Fed., Engl. Ed.) 51, 788–803. doi: 10.1134/S002689331706005X
Dobiasch, S., Szanyi, S., Kjaev, A., Werner, J., Strauss, A., Weis, C., et al. (2016). Synthesis and functionalization of protease-activated nanoparticles with tissue plasminogen activator peptides as targeting moiety and diagnostic tool for pancreatic cancer. J. Nanobiotechnol. 14, 81/81–81/18. doi: 10.1186/s12951-016-0236-3
Fetiveau, L., Paul, G., Nicolas-Boluda, A., Volatron, J., George, R., Laurent, S., et al. (2019). Tailored ultra-small Prussian blue-based nanoparticles for MRI imaging and combined photothermal/photoacoustic theranostics. Chem. Commun. (Cambridge, U. K.) 55, 14844–14847. doi: 10.1039/C9CC07116D
Gholibegloo, E., Mortezazadeh, T., Salehian, F., Forootanfar, H., Firoozpour, L., Foroumadi, A., et al. (2019). Folic acid decorated magnetic nanosponge: an efficient nanosystem for targeted curcumin delivery and magnetic resonance imaging. J. Colloid Interface Sci. 556, 128–139. doi: 10.1016/j.jcis.2019.08.046
Herranz-Blanco, B., Shahbazi, M.-A., Correia, A. R., Balasubramanian, V., Kohout, T., Hirvonen, J., et al. (2016). pH-Switch nanoprecipitation of polymeric nanoparticles for multimodal cancer targeting and intracellular triggered delivery of doxorubicin. Adv. Healthcare Mater. 5, 1904–1916. doi: 10.1002/adhm.201600160
Huang, Y., Mao, K., Zhang, B., and Zhao, Y. (2017). Superparamagnetic iron oxide nanoparticles conjugated with folic acid for dual target-specific drug delivery and MRI in cancer theranostics. Mater. Sci. Eng. C 70, 763–771. doi: 10.1016/j.msec.2016.09.052
Huang, Y., Zhang, B., Xie, S., Yang, B., Xu, Q., and Tan, J. (2016). Superparamagnetic iron oxide nanoparticles modified with tween 80 pass through the intact blood-brain barrier in rats under magnetic field. ACS Appl. Mater. Interfaces 8, 11336–11341. doi: 10.1021/acsami.6b02838
Israel, L. L., Lellouche, E., Greneche, J.-M., Bechor, M., Michaeli, S., and Lellouche, J.-P. (2016). Ultrasound-mediated surface engineering of theranostic magnetic nanoparticles: an effective one-pot functionalization process using mixed polymers for siRNA delivery. J. Nanomed. Nanotechnol. 7, 385/381–385/314. doi: 10.4172/2157-7439.1000385
Kudr, J., Haddad, Y., Richtera, L., Heger, Z., Adam, V., Zitka, O., et al. (2017). Magnetic nanoparticles: from design and synthesis to real world applications. Nanomaterials (Basel) 7:243. doi: 10.3390/nano7090243
Kumar, N., Tyeb, S., Manzar, N., Behera, L., Ateeq, B., and Verma, V. (2018). Entropically driven controlled release of paclitaxel from poly(2-ethyl-2-oxazoline) coated maghemite nanostructures for magnetically guided cancer therapy. Soft Matter 14, 6537–6553. doi: 10.1039/C8SM01220B
Li, Y., Wang, N., Huang, X., Li, F., Davis, T. P., Qiao, R., et al. (2020). Polymer-assisted magnetic nanoparticle assemblies for biomedical applications. ACS Appl. Bio Mater. 3, 121–142. doi: 10.1021/acsabm.9b00896
Liao, J., Wei, X., Ran, B., Peng, J., Qu, Y., and Qian, Z. (2017). Polymer hybrid magnetic nanocapsules encapsulating IR820 and PTX for external magnetic field-guided tumor targeting and multifunctional theranostics. Nanoscale 9, 2479–2491. doi: 10.1039/C7NR00033B
Liu, L., Li, X., Chen, L., and Zhang, X. (2018). Nanoscale functional biomaterials for cancer theranostics. Curr. Med. Chem. 25, 2987–3000. doi: 10.2174/0929867324666170406111036
Lu, S., Li, X., Zhang, J., Peng, C., Shen, M., and Shi, X. (2018). Dendrimer-stabilized gold nanoflowers embedded with ultrasmall iron oxide nanoparticles for multimode imaging-guided combination therapy of tumors. Adv. Sci. 5:1801612. doi: 10.1002/advs.201801612
Luque-Michel, E., Sebastian, V., Larrea, A., Marquina, C., and Blanco-Prieto, M. J. (2019). Co-encapsulation of superparamagnetic nanoparticles and doxorubicin in PLGA nanocarriers: Development, characterization and in vitro antitumor efficacy in glioma cells. Eur. J. Pharm. Biopharm. 145, 65–75. doi: 10.1016/j.ejpb.2019.10.004
Niu, D., Li, Y., and Shi, J. (2017). Silica/organosilica cross-linked block copolymer micelles: a versatile theranostic platform. Chem. Soc. Rev. 46, 569–585. doi: 10.1039/C6CS00495D
Pandey, N., Menon, J. U., Nguyen, K. T., Wadajkar, A. S., Pandey, N., Menon, J. U., et al. (2020). Thermo-responsive fluorescent nanoparticles for multimodal imaging and treatment of cancers. Nanotheranostics 4, 1–13. doi: 10.7150/ntno.39810
Patitsa, M., Karathanou, K., Kanaki, Z., Tzioga, L., Pippa, N., Demetzos, C., et al. (2017). Magnetic nanoparticles coated with polyarabic acid demonstrate enhanced drug delivery and imaging properties for cancer theranostic applications. Sci. Rep. 7, 1–8. doi: 10.1038/s41598-017-00836-y
Patra, D., Mukherjee, S., Chakraborty, I., Dash, T. K., Senapati, S., Bhattacharyya, R., et al. (2018). Iron(III) coordinated polymeric nanomaterial: a next-generation theranostic agent for high-resolution T1-weighted magnetic resonance imaging and anticancer drug delivery. ACS Biomater. Sci. Eng. 4, 1738–1749. doi: 10.1021/acsbiomaterials.8b00294
Ray, S., Li, Z., Hsu, C.-H., Hwang, L.-P., Lin, Y.-C., Chou, P.-T., et al. (2018). Dendrimer- and copolymer-based nanoparticles for magnetic resonance cancer theranostics. Theranostics 8, 6322–6349. doi: 10.7150/thno.27828
Rost, N. C. V., Sen, K., Savliwala, S., Singh, I., Liu, S., Unni, M., et al. (2020). Magnetic particle imaging performance of liposomes encapsulating iron oxide nanoparticles. J. Magn. Magn. Mater. 504:166675. doi: 10.1016/j.jmmm.2020.166675
Roy, E., Patra, S., Madhuri, R., and Sharma, P. K. (2016). Stimuli-responsive poly(N-isopropyl acrylamide)-co-tyrosine@gadolinium: Iron oxide nanoparticle-based nanotheranostic for cancer diagnosis and treatment. Colloids Surf. B 142, 248–258. doi: 10.1016/j.colsurfb.2016.02.053
Roy, E., Patra, S., Madhuri, R., and Sharma, P. K. (2017). Anisotropic gold nanoparticle decorated magnetopolymersome: an advanced nanocarrier for targeted photothermal therapy and dual-mode responsive T1 MRI imaging. ACS Biomater. Sci. Eng. 3, 816–831. doi: 10.1021/acsbiomaterials.7b00089
Song, G., Zheng, X., Wang, Y., Xia, X., Chu, S., and Rao, J. (2019). A magneto-optical nanoplatform for multimodality imaging of tumors in mice. ACS Nano 13, 7750–7758. doi: 10.1021/acsnano.9b01436
Srinivasan, S. Y., Paknikar, K. M., Gajbhiye, V., and Bodas, D. (2018). Magneto-conducting core/shell nanoparticles for biomedical applications. Chem. Nano. Mat. 4, 151–164. doi: 10.1002/cnma.201700278
Sun, L., Joh, D. Y., Al-Zaki, A., Stangl, M., Murty, S., Davis, J. J., et al. (2016). Theranostic application of mixed gold and superparamagnetic iron oxide nanoparticle micelles in glioblastoma multiforme. J. Biomed. Nanotechnol. 12, 347–356. doi: 10.1166/jbn.2016.2173
Thirunavukkarasu, G. K., Cherukula, K., Lee, H., Jeong, Y. Y., Park, I.-K., and Lee, J. Y. (2018). Magnetic field-inducible drug-eluting nanoparticles for image-guided thermo-chemotherapy. Biomaterials 180, 240–252. doi: 10.1016/j.biomaterials.2018.07.028
Vamvakidis, K., Mourdikoudis, S., Makridis, A., Paulidou, E., Angelakeris, M., and Dendrinou-Samara, C. (2018). Magnetic hyperthermia efficiency and MRI contrast sensitivity of colloidal soft/hard ferrite nanoclusters. J. Colloid Interface Sci. 511, 101–109. doi: 10.1016/j.jcis.2017.10.001
Vijayan, V. M., Beeran, A. E., Shenoy, S. J., Muthu, J., and Thomas, V. (2019). New magneto-fluorescent hybrid polymer nanogel for theranostic applications. ACS Appl. Bio Mater. 2, 757–768. doi: 10.1021/acsabm.8b00616
Wang, G., Qian, K., and Mei, X. (2018). A theranostic nanoplatform: magneto-gold@fluorescence polymer nanoparticles for tumor targeting T1&T2-MRI/CT/NIR fluorescence imaging and induction of genuine autophagy mediated chemotherapy. Nanoscale 10, 10467–10478. doi: 10.1039/C8NR02429D
Wei, J., Shuai, X., Wang, R., He, X., Li, Y., Ding, M., et al. (2017). Clickable and imageable multiblock polymer micelles with magnetically guided and PEG-switched targeting and release property for precise tumor theranosis. Biomaterials 145, 138–153. doi: 10.1016/j.biomaterials.2017.08.005
Xin, F., Li, Y., Fu, C., Javed, I., Huang, X., Schaschkow, A., et al. (2020). Multimodal nanoprobe for pancreatic beta cell detection and amyloidosis mitigation. Chem. Mater. 32, 1080–1088. doi: 10.1021/acs.chemmater.9b03841
Yan, H., Zhao, L., Shang, W., Liu, Z., Xie, W., Qiang, C., et al. (2017). General synthesis of high-performing magneto-conjugated polymer core-shell nanoparticles for multifunctional theranostics. Nano Res. 10, 704–717. doi: 10.1007/s12274-016-1330-4
Yang, H., Miao, Y., Chen, L., Li, Z., Yang, R., Xu, X., et al. (2020). Redox-responsive nanoparticles from disulfide bond-linked poly-(N-ε-carbobenzyloxy-L-lysine)-grafted hyaluronan copolymers as theranostic nanoparticles for tumor-targeted MRI and chemotherapy. Int. J. Biol. Macromol. 148, 483–492. doi: 10.1016/j.ijbiomac.2020.01.071
Yang, H.-K., Bao, J.-F., Mo, L., Yang, R.-M., Xu, X.-D., Tang, W.-J., et al. (2017). Bioreducible amphiphilic block copolymers based on PCL and glycopolypeptide as multifunctional theranostic nanocarriers for drug delivery and MR imaging. RSC Adv. 7, 21093–21106. doi: 10.1039/C7RA01440F
Yar, Y., Khodadust, R., Akkoc, Y., Utkur, M., Saritas, E. U., Gozuacik, D., et al. (2018). Development of tailored SPION-PNIPAM nanoparticles by ATRP for dually responsive doxorubicin delivery and MR imaging. J. Mater. Chem. B 6, 289–300. doi: 10.1039/C7TB00646B
Zahraei, M., Marciello, M., Lazaro-Carrillo, A., Villanueva, A., Herranz, F., Talelli, M., et al. (2016). Versatile theranostics agents designed by coating ferrite nanoparticles with biocompatible polymers. Nanotechnology 27, 255702/255701–255702/255712. doi: 10.1088/0957-4484/27/25/255702
Zhang, C., Yao, Z.-C., Ding, Q., Choi, J. J., Ahmad, Z., Chang, M.-W., et al. (2017). Tri-needle coaxial electrospray engineering of magnetic polymer yolk-shell particles possessing dual-imaging modality, multiagent compartments, and trigger release potential. ACS Appl. Mater. Interfaces 9, 21485–21495. doi: 10.1021/acsami.7b05580
Zhang, K.-L., Zhou, J., Zhou, H., Wu, Y., Liu, R., Wang, L.-L., et al. (2017). Bioinspired “active” stealth magneto-nanomicelles for theranostics combining efficient MRI and enhanced drug delivery. ACS Appl. Mater. Interfaces 9, 30502–30509. doi: 10.1021/acsami.7b10086
Keywords: theranostics, magnetic nanoparticles, polymer, engineering design, functionalization for cell targeting
Citation: Gauger AJ, Hershberger KK and Bronstein LM (2020) Theranostics Based on Magnetic Nanoparticles and Polymers: Intelligent Design for Efficient Diagnostics and Therapy. Front. Chem. 8:561. doi: 10.3389/fchem.2020.00561
Received: 14 April 2020; Accepted: 02 June 2020;
Published: 07 July 2020.
Edited by:
Raghvendra Singh Yadav, Tomas Bata University in Zlín, CzechiaReviewed by:
Xiangyang Shi, Donghua University, ChinaMarie-France Penet, Johns Hopkins University, United States
Kai Yang, Soochow University, China
Copyright © 2020 Gauger, Hershberger and Bronstein. This is an open-access article distributed under the terms of the Creative Commons Attribution License (CC BY). The use, distribution or reproduction in other forums is permitted, provided the original author(s) and the copyright owner(s) are credited and that the original publication in this journal is cited, in accordance with accepted academic practice. No use, distribution or reproduction is permitted which does not comply with these terms.
*Correspondence: Lyudmila M. Bronstein, lybronst@indiana.edu
†These authors have contributed equally to this work