Tectono-Thermal Evolution of the Red Sea Rift
- 1School of Geography, Earth and Atmospheric Sciences, The University of Melbourne, Melbourne, VIC, Australia
- 2Istituto di Geoscienze e Georisorse, Consiglio Nazionale delle Ricerche, UOS Firenze, Firenze, Italy
The Oligocene-Recent Red Sea rift is one of the preeminent examples of lithospheric rupture in the recent geological past, forming the basis for many models of how continental breakup occurs and progresses to the formation of new oceanic crust. Utilisation of low-temperature thermochronology in the Red Sea Rift since the 1980s has been key to constraining its spatio-temporal evolution, providing constraints for the propagation of strain and geomorphological development of its margins where datable syn-tectonic strata and/or markers are absent. We review the wealth of published apatite fission track and (U-Th-Sm)/He data from along the Red Sea, affording insights into the Oligocene-Recent thermo-tectonic evolution of the Nubian and Arabian margins. A regional interpolation protocol was employed to synthesise time-temperature reconstructions generated from the mined thermochronology data and burial histories produced from vitrinite reflectance and well data. These cooling-heating maps record a series of pronounced episodes of upper crustal thermal flux related to the development of the Oligocene-Recent Red Sea Rift. Assimilation of these regional thermal history maps with paleogeographic reconstructions and regional magmatic and strain histories provide regional perspectives on the roles of tectonism and geodynamic activity in Red Sea formation and their effects on rift margin development.
Introduction
The development of the Red Sea Rift since the Oligocene has facilitated the separation of Western Arabia from Africa. The rift system formed in the presence of the Afar mantle plume and far-field tectonic stresses related to subduction of the Neotethys Ocean beneath Eurasia far to the north (Bosworth et al., 2005; Almalki et al., 2015), eventually leading to formation of new oceanic crust as early as 13 Ma (Mohriak, 2019; Augustin et al., 2021). Consequently, it has formed the basis for a variety of rifting models, including for different rifting modes, active versus passive rifting, the role of mantle plumes in continental breakup, plume-induced topography development, the onset of sea floor spreading, rift shoulder uplift and extensional faulting (e.g. Milanovsky, 1972; Burke and Dewey, 1973; Sengör and Burke, 1978; Bonatti, 1985; van der Beek et al., 1995; Khalil and McClay, 2002; Burov and Gerya, 2014).
Key to resolving the spatiotemporal evolution of the Red Sea has been the application of low-temperature thermochronology techniques, such as apatite fission track (AFT) and (U-Th-Sm)/He (AHe), to constrain the tectono-thermal history of its rifted margins. These temperature-sensitive radiometric dating techniques provide information on when a rock cooled through the respective method-specific temperature sensitivity range; ∼60 to 110–120°C for AFT (Gleadow and Duddy, 1981) and ∼40–80°C for AHe (Wolf et al., 1998). For AFT, additional quantification of the distribution of fission track lengths and their mean (MTL, mean track length) allow the rate and character of a cooling path to be characterised (Laslett et al., 1987). By jointly numerically modelling these data, the thermal history of a sample can then be reconstructed (e.g. Gallagher, 1995; Ketcham, 2005). As such, thermochronology data provide unique insights into the upper crustal thermal history of rifts in relation to tectonic and erosional exhumation, syn-rift deposition and burial, and passive margin evolution, particularly important where datable syn-tectonic strata and/or markers are absent (Braun and van der Beek, 2004; Ehlers, 2005; Stockli, 2005; Wildman et al., 2019).
Here, we summarise the Oligocene-recent tectono-thermal evolution of the Red Sea rift and adjacent hinterlands in the context of the wealth of published low-temperature thermochronology data (Figure 1), time-temperature reconstructions and burial history models from across the region (Figure 2). Through a synthesis of the upper crustal thermal evolution of the Red Sea margins, rift nucleation, inception and propagation mechanisms are explored.
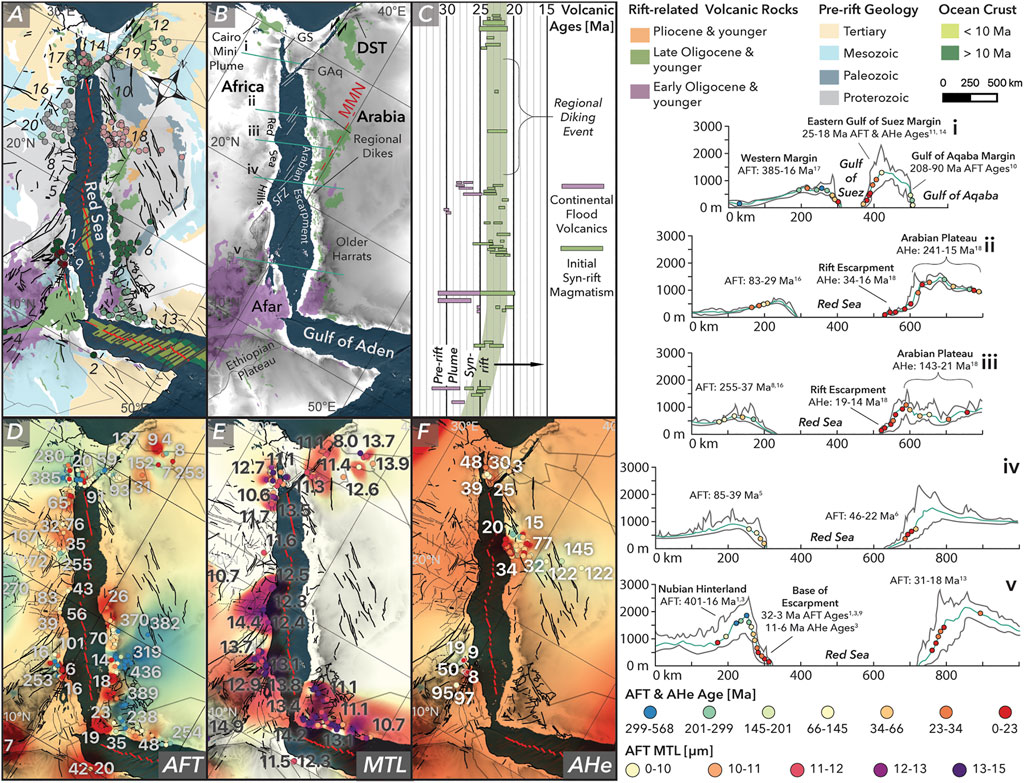
FIGURE 1. Synthesis of the surface geology, plume and syn-rift volcanism, topography and low-temperature thermochronology of the Red Sea and adjacent hinterlands. (A) Simplified geological map (after Bosworth, 2015; Thiéblemont et al., 2016; Bosworth and Stockli, 2016) showing the locations of published AFT (green circles: 1,2Abbate et al., 2002, 2001; 3Balestrieri et al., 2005; 4Abebe et al., 2010; 5Balestrieri et al., 2009; 6Bohannon et al., 1989; 7Bojar et al., 2002; 8Feinstein et al., 2013; 9Ghebreab et al., 2002; 10Kohn and Eyal, 1981; 11Kohn et al., 1997; 12Kohn et al., 2019; 13Menzies et al., 1992, 1997; 14Morag et al., 2019; 15Naylor et al., 2013; 16,17Omar et al., 1987, 1989) and AHe data (red circles: 3Balestrieri et al., 2005; 12Kohn et al., 2019; 14Morag et al., 2019; 18Szymanski et al., 2016; 19Vermeesch et al., 2009). Note, detailed AFT data from Omar and Steckler (1995) was not published (location 20). (B) Digital elevation model of the Red Sea region illustrating the distribution of pre-rift plume-related continental flood volcanics and initial syn-rift volcanism, with the locations of swath profiles (righthand) shown with representative AFT and AHe ages projected onto them (corresponding reference numbers refer to study locations). Pre-existing shear zones, such as the Shagara Fracture Zone (SFZ) shown in white, after Crane and Bonatti (1987). The corresponding radiometric determined emplacement ages are illustrated in a chronogram (C) after Bosworth and Stockli (2016). (D–F) Inverse distance weighted interpolations (distance coefficient P of 5) of AFT ages, AFT mean track lengths (MTL) and AHe median single grain ages. While thermochronology data are sporadically distributed along the Red Sea margins, AFT and AHe ages proximal to the coast appear to generally increase and corresponding MTL decrease northwards. In all panels, dark solid lines are major faults, light solid lines are minor faults, hashed lines are inferred faults and red lines are spreading ridges (from Thiéblemont et al., 2016). DST, Dead Sea Transform; GS, Gulf of Suez; GAq, Gulf of Aqaba; MMN, Makkah-Madinah-Nafud volcanic line.
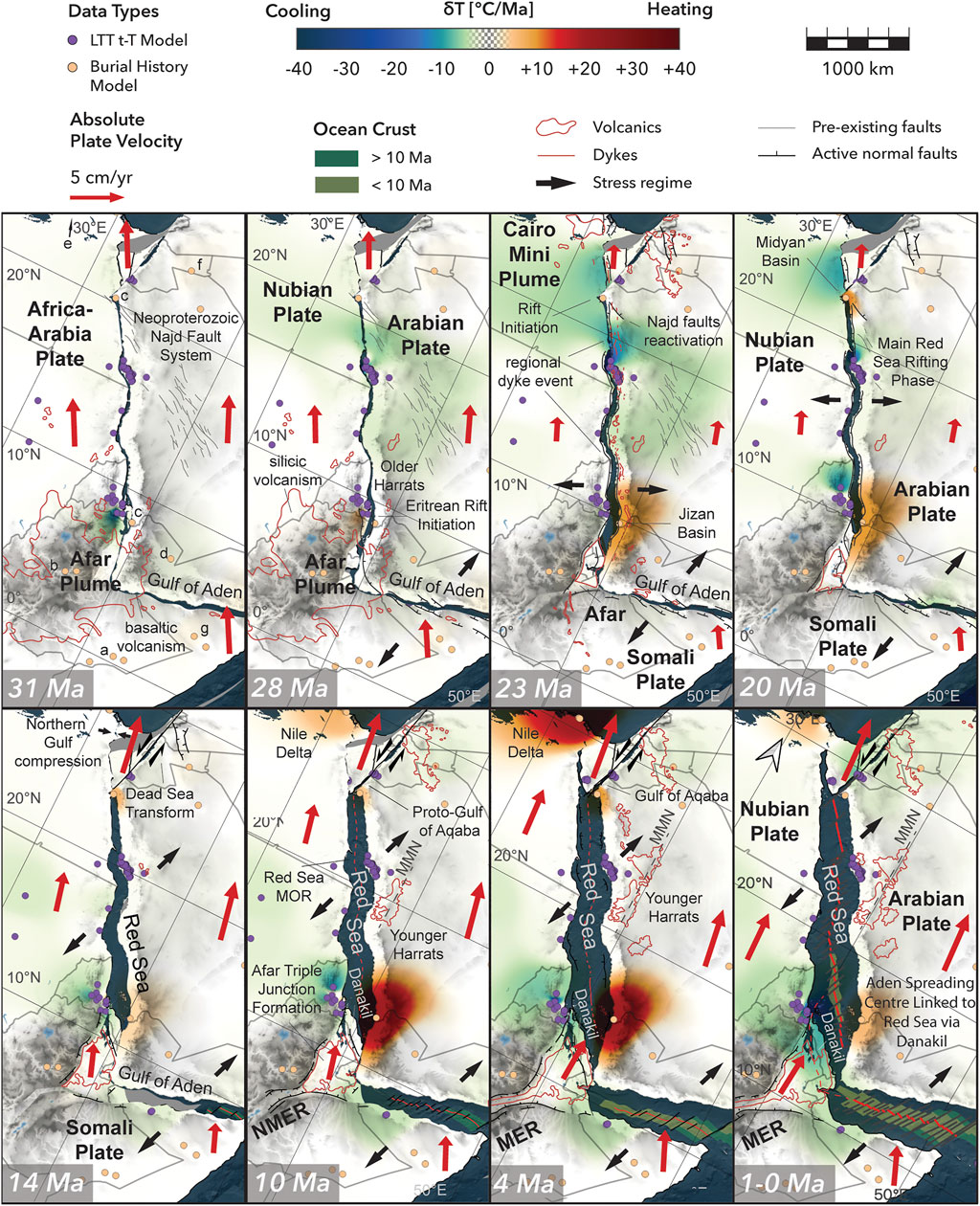
FIGURE 2. Paleogeographic reconstructions of the opening of the Red Sea and absolute plate velocities since 31 Ma (rectangular projection centered on the Arabian Plate after Müller et al., 2016) overlain by cooling-heating maps. Cooling-heating maps were generated by performing inverse distance weighted interpolations (distance coefficient P of 5) of δT°C as recorded by published best-fit time-temperature models generated from low-temperature thermochronology (LTT) data (purple circles – refer to study locations 1, 2, 3, 5, 8, 9, 12, 14, 18, 19 in Figure 1) and well data (orange circles: aTsegaye et al., 2018; bWolela, 2007; cCole et al., 1995; dAl-Areeq et al., 2018; eKeshta et al., 2012; fNaylor et al., 2013; gAli and Lee, 2019 - locations in 31 Ma panel). Simplified structures after Thiéblemont et al. (2016) and Bosworth et al. (2005). Age of Red Sea mid oceanic ridge (MOR) after Augustin et al. (2021). Syn-rift igneous rock locations after Bosworth and Stockli (2016). (N)MER, (North) Main Ethiopian Ridge; MMN, Makkah-Madinah-Nafud volcanic line.
Geomorphology and Low-temperature Thermochronology of the Red Sea Rifted Margins
The Red Sea displays longitudinal and along-strike variations in rift flank morphology and exhumation histories, as recorded by low-temperature thermochronology data, that reflect its asymmetric Oligocene-recent rift evolution. The Nubian margin is characterised by the narrow, 1,500 km long “Red Sea Hills,” which have a mean elevation of 500 m and reach heights >2,000 m along the Ethiopian plateau (Figure 1). The conjugate Arabian Escarpment has experienced even greater surface uplift, with broad highlands averaging 1,000–1,500 m in elevation and attaining heights of up to 2,750–3,200 m in Yemen (Figure 1). This east-to-west discrepancy in rift flank uplift is reflected in the generally younger thermochronology ages along the Arabian margin, indicative of cooling from higher temperature and interpreted as recording exhumation from greater depths (Figure 1i, ii, iii, iv). However, this pattern may be exaggerated by the dearth of lower temperature-sensitive AHe data along the Nubian margin. Explanations for longitudinal asymmetry in Red Sea physiography include northeast tilting of Arabia due to its collision with Eurasia since the Paleogene (Bohannon et al., 1989), potential underplating due to Cenozoic volcanism being largely restricted to the Arabian plate (Dixon et al., 1989), the presence of a broad low velocity anomaly beneath Arabia (Ritsema et al., 1999; Debayle et al., 2001; Chang and Van der Lee, 2011), associated dynamic topography (Daradich et al., 2003), and strong lateral thermal gradients beneath Arabia towards significantly hotter temperatures along the Red Sea margin (Benoit et al., 2003).
Both Red Sea margins are characterised by a marked northward decrease in rift flank topography, north of ∼21°N (Figure 1). This transition to more subdued topography coincides with the intersection of the Makkah-Madinah-Nafud volcanic line (Camp and Roobol, 1989) and the offshore Shagara Fracture Zone (Crane and Bonatti, 1987), leading Szymanski et al. (2016) to suggest first-order magmatic and structural controls on rift margin morphology. South of this point, initial extension occurred in a narrow deformation zone, limited to a 60–120 km wide domain of the Arabian margin, with no evidence for significant normal faulting and syn-rift dike emplacement inland of the coastal escarpment (Bohannon et al., 1989; Davison et al., 1994). Along the conjugate Eritrean rifted margin (Figure 1v), AFT and AHe data suggest that the escarpment evolved by plateau degradation, whereby initial localized rift scarps gradually retreated to form the high escarpment that now flanks the Nubian margin (Abbate et al., 2002; Balestrieri et al., 2005). The Central and Northern Red Sea rift, by contrast, experienced diffuse stretching, dissecting >150 km of the Arabian margin alone with active normal faults and dikes (Szymanski et al., 2016). Consequently, the rift escarpment north of ∼21°N is more attenuated, mirroring the wide distribution of strain, and reflected by a general increase in thermochronology ages and reduction in MTL (Figure 1).
Tectono-thermal Evolution of the Red Sea Rift
Onset of Afro-Arabian Breakup and Afar Plume Impingement
The Red Sea Rift developed in the Neoproterozoic Arabian-Nubian Shield (ANS) of northeast Africa and Arabia, which comprises a collage of low-metamorphic grade island-arc terranes that collided during the Pan-African Orogeny. During this series of collisional events between ∼870 and 542 Ma that amalgamated East and West Gondwana (e.g. Kröner and Stern 2004), regional-scale, deep-seated shear zones developed which acted as important stress guides during later tectonism.
Afro-Arabian breakup first began sometime during the late Eocene with initiation of rifting in the eastern proto-Gulf of Aden in a ∼NNE-SSW oriented far-field stress regime resulting from slab-pull in the subduction zone along the northern Neotethys (Bosworth et al., 2005). A subsequent period of subtle early Oligocene cooling recorded along the Egyptian margin by AFT data was initially heralded as recording the onset of rifting in the Northern Red Sea (Omar and Steckler, 1995). However, this cooling phase, also documented by thermal history modelling of AFT vertical profiles and burial history models of exploration wells in Jordan, was later shown to significantly predate the age of the nearby Gulf of Suez and Dead Sea rifts (Feinstein et al., 2013; Naylor et al., 2013). (U-Th-Sm)/He data from the central ANS, by contrast, record thermal stability or possible slight reheating until the late Oligocene (Figure 2; Szymanski et al., 2016), indicating early Oligocene cooling was restricted to Northern Egypt and the Levant. This erosional phase has, therefore, been interpreted as representing a period of regional denudation restricted to the Northern Arabian margin, related to Oligocene peneplanation in Southern Israel, Transjordan and Sinai (e.g. Avni et al., 2012). Bosworth and McClay (2001) suggested that this exhumation phase was likely related to well-documented late Eocene Syrian Arc compression (Guiraud and Bosworth, 1999).
Soon after, between 31 and 29 Ma, >600,000 km2 of plume-related flood basalts were emplaced over Ethiopia, Northeast Sudan and Southwest Yemen, centered around the Afar (Figure 1; Bosworth and Stockli, 2016). Afar plume magmatism is considered pre-rift, due to a dearth of significant faulting in Arabia, Yemen and the Ethiopia plateau during flood volcanism and the notable lack of early Oligocene syn-rift strata in Red Sea exploration wells (Hughes et al., 1991; Davison et al., 1994; Menzies et al., 1997; Corti, 2009). Shortly after early Oligocene Afar magmatic activity, an extensive series of volcanic centres began erupting along the Western Arabian margin at 30 Ma, collectively referred to as the Older Harrats (Bosworth and Stockli, 2016).
Birth of the Southern Red Sea Rift
Significant extensional strain in the proto-southern Red Sea Rift first nucleated in a narrow zone of thermo-mechanically weakened crust between 29 and 26 Ma (Wolfenden et al., 2005), accompanied by volumetrically insignificant volcanism (Bosworth and Stockli, 2016). Well data from offshore Eritrea revealed a late Oligocene basal syn-rift section between 28 and 23 Ma (Hughes et al., 1991). The onset of rift-related uplift and denudation via normal faulting along the conjugate Eritrean and Yemeni Southern Red Sea margins soon followed at sometime between ∼26 and 20 Ma (Figure 2; Menzies et al., 1997; Balestrieri et al., 2009; Ghebreab et al., 2002). A coeval late Oligocene onset of rapid syn-rift deposition in the Jizan Basin along the Southern Arabian margin is recorded by burial history modelling (Figure 2; Cole et al., 1995). Major extension in the Afar began soon after ∼25 Ma (Zanettin et al., 1978).
Synchronous Initiation of the Central and Northern Red Sea Rift
Between 24 and 21 Ma, the Red Sea Rift initiated concomitantly north of the Afar (Bosworth et al., 2005; Bosworth and Stockli, 2016). While earlier proposed models (e.g. Girdler, 1984; Voggenreiter et al., 1988) advocated a gradual south-to-north propagation of rifting, a wealth of geochemical, geodetic, well and structural data collected since record a synchronous onset of strain, syn-rift deposition and shoulder uplift along the length of the Central and Northern Red Sea (e.g. ArRajehi et al., 2010; Almalki et al., 2015; Bosworth, 2015; Szymanski et al., 2016).
The latest Oligocene-early Miocene was marked by extensive dyking, granitic intrusions and silicic volcanism along the 1,700 km length of the Western Arabian margin 24–23 Ma, magmatically linking the Afar with the Cairo mini-plume (Bosworth and Stockli, 2016). This was accompanied by coeval initiation of orthogonal extension along the length of the Red Sea north of Afar at 24 ± 2.2 Ma, as indicated by geodetic data (ArRajehi et al., 2010) and the relationship between brittle structures and syn-rift depositional ages (Bojar et al., 2002). A series of pre-existing, oblique shear zones, such as the Shagara Shear Zone (Figure 1), played a critical role in the early structural evolution of the rift, offsetting the axial trough (Crane and Bonatti, 1987). Further northward propagation of the Gulf of Suez may have been halted by the sharp increase in lithospheric strength of the Mediterranean continental margin (Steckler and ten Brink, 1986).
Earliest syn-tectonic sedimentation was marked by widespread deposition of the uppermost Oligocene-lower Miocene Al Wajh Formation and its lateral equivalents throughout the Red Sea coastal basins (Hughes and Johnson, 2005; Tubbs et al., 2014). Basal syn-rift strata comprise often poorly-sorted arkosic and granitic clasts, indicating direct derivation from the unconformably underlying and adjacent Proterozoic basement (Hughes and Johnson, 2005) and record early localized footwall uplift (Bosworth et al., 2005).
The nearly simultaneous latest Oligocene-early Miocene initiation of rift shoulder uplift and erosion along the length of the Central and Northern Red Sea is recorded by AFT and AHe data and thermal history modelling (Figure 2; Kohn and Eyal, 1981; Omar et al., 1987; Omar et al., 1989; Szymanski et al., 2016; Morag et al., 2019). However, in certain areas, such as along the Sudanese margin, rift shoulder uplift was insufficient to exhume rocks with reset Neogene AFT ages (Figure 1; Balestrieri et al., 2009). Older AFT ages preserved in three deep boreholes from Miocene syn-rift sediments in the Eastern Suez Rift indicate that the rift-related thermal regime was moderate (Kohn et al., 1997), despite the coincident onset of regional alkali-basalt magmatism and basin formation in the Northern Red Sea.
Main Phase of Red Sea Rifting
The principal phase of Red Sea rifting followed, as recorded by a shift to rapid rift shoulder exhumation (Figure 2) and pronounced extension-driven subsidence, bio-stratigraphically dated to ∼20.4 Ma (Bosworth, 2015). Well data from the Midyan Basin records a concurrent phase of rapid early Miocene sedimentation at the junction of the Red Sea and Gulf of Aqaba (Figure 2; Cole et al., 1995). In Northern Egypt, this was recorded by rapid early Miocene cooling of the Western Suez margin (Omar et al., 1989). Similarly, rapid early Miocene exhumational cooling along the Southern Red Sea margins related to isostatic footwall uplift is recorded by thermochronology data in Sudan, Eritrea, Yemen (Davison et al., 1994; Abbate et al., 2002; Ghebreab et al., 2002; Balestrieri et al., 2009) and possibly Southern Saudi Arabia (Bohannon et al., 1989).
This main phase of Miocene rifting in the Red Sea and Gulf of Suez did not correspond to volumetrically significant rift-related volcanism, suggesting that plate-boundary forces were the primary mechanism facilitating the breakup of Western Arabia from Africa (Bosworth and Stockli, 2016). Nevertheless, the complex interplay between thermo-mechanical weakening of the lithosphere via plume impingement and the presence of pre-existing lithospheric heterogeneities is thought to have played a fundamental role in along-strike changes in Red Sea Rift propagation and style (e.g. Cochran and Karner, 2007; Khalil et al., 2020). The most important of these inherited crustal discontinuities were the WNW-ESE striking, left-lateral Neoproterozoic Najd shear zone which played a significant role in governing Miocene strain (Younes and McClay, 2002; Khalil and McClay, 2009). The relationship between magmatically attenuated lithosphere and pre-existing fabrics is thought to have controlled the length and orientation of border faults (Hubert-Ferrari et al., 2003; Bellahsen et al., 2013) and distribution of accommodation zones (Younes and McClay, 2002).
Formation of the Dead Sea Transform
The Dead Sea Transform (DST) extends over 1,000 km from the Gulf of Aqaba to the Southern Turkey convergent zone and accommodates relative motion between Sinai (Africa) and Arabia. The DST was thought to have formed sometime between ∼20 and 14 Ma, based on stratigraphic, magmatic and structural evidence (Garfunkel, 2014). However, recent direct dating of syn-faulting calcite has further constrained the formation of the DST plate boundary to 20.8–18.5 Ma in Southern Israel and propagating >500 km northwards by 17.1 Ma (Nuriel et al., 2017). DST formation was, therefore, broadly concurrent with the initiation of sea floor spreading in the Eastern Gulf of Aden (∼19–18 Ma; Leroy et al., 2004). Early DST brittle deformation and related low-temperature hydrothermal fluid flow is also recorded by thermally-reset 17 Ma ZHe and AFT ages (Kohn et al., 2019); the latter representing intra-strata recrystallisation of authigenic carbonate-fluorapatite from Southern Israel.
A dramatic reduction in uplift, exhumation and cooling along the Eastern Suez Rift occurred after 18 Ma (Figure 2), as strain was preferentially accommodated by the newly formed DST (Morag et al., 2019). As a result of the strike-slip dominated strain regime of the DST, a lesser degree of margin uplift along the Eastern Sinai accompanied Gulf of Aqaba formation. Consequently, older AFT ages are preserved along its shores compared to the Suez rift, where more rift-normal extension dominates (Figure 1; Kohn and Eyal, 1981). Since its formation, the DST has accommodated ∼105 km of left-lateral displacement, as recorded by offset of Precambrian to Palaeogene stratigraphic markers, depositional patterns and tectonic elements (Garfunkel, 2014).
A second important stage of minor displacement along the DST occurred later in the Pliocene due to changes in regional plate kinematics, resulting in some southern areas developing a more rift-like morphology, in part manifested as pull-apart basins such as the Gulf of Aqaba and Dead Sea (Garfunkel, 2014). Thereupon, ∼1 km of Tertiary to Senonian section was removed from the DST rift shoulders and deposited in adjacent basins. A resulting ∼35–40°C of Plio-Pleistocene denudational cooling is recorded by AHe ages of authigenic apatite in Southern Israel (Kohn et al., 2019) and independently corroborated by Ar-Ar data (Gur et al., 1995).
Onset of Sea Floor Spreading in the Red Sea
Early estimates for a 5 Ma onset of sea floor spreading in the Red Sea were based on magnetic anomalies preserved in exposed oceanic crust, and were therefore limited to areas of the Southern Red Sea unobstructed by overlying thick successions of sediment and salt deposits (Courtillot, 1982; Cochran, 1983; Almalki et al., 2016). However, a recent study (Augustin et al., 2021) utilising earthquake and vertical gravity gradient data, able to observe the extent of oceanic crust beneath the salt and sediment cover, indicates that sea floor spreading began much earlier along the full length of the Red Sea by between 13 and 12 Ma. The middle Miocene transition to mid-ocean spreading corresponded to a rotation of Red Sea extension from rift orthogonal (N°60°E) to highly oblique and parallel to the DST (N°15°E; Bosworth et al., 2005) and was accompanied by a 70% increase in Arabia-Nubia relative motion since 13 Ma (ArRajehi et al., 2010). A concurrent period of rift flank uplift and cooling is recorded by middle Miocene AHe ages from several fault blocks in Western Arabian (Figure 1; Szymanski et al., 2016) and thermochronology data from along the Southern Arabian and conjugate Eritrean margins (Figure 2; Bohannon et al., 1989; Balestrieri et al., 2005).
This abrupt change in plate kinematics was marked by the coeval propagation of seafloor spreading into the Central Gulf of Aden, termination of onshore syn-rift deposition along the Aden margins (Bosworth, 2015) and the main phase of the Zagros orogeny ∼14 Ma (Sengör and Yilmaz, 1981; Burke, 1996). Renewed volcanism in the Arabian Shield followed with the eruption of the so-called Younger Harrats in the middle Miocene (∼13 Ma; Figure 2), thought to be associated with movement along the DST (Bosworth and Stockli, 2016).
Afar Triple Junction Formation and Danakil Block Rifting
Circa 11 Ma, extensional deformation in the Afar propagated southward with the development of the northern Main Ethiopian Rift (NMER) (Wolfenden et al., 2004), resulting in the configuration of the Afar triple junction (Corti, 2009). This was marked by development and pronounced erosion of the Western Afar scarp (Pik et al., 2003). Soon after (∼10 Ma) sea floor spreading in the Gulf of Aden rapidly propagated more than 400 km westward towards the Afar (Bosworth et al., 2005). By this time, the Danakil block, a narrow, NW-trending Precambrian basement block along the Northern Afar margin, began to slowly rift away independently from Nubia, Somalia and Arabia (Figure 2; Eagles et al., 2002). Sometime between 8 and 5 Ma, the central Main Ethiopian Rift formed, as recorded by AFT data (beyond the extent of Figure 2; Abebe et al., 2010) and structural observations (Bonini et al., 2005), linking the Red Sea-Gulf of Aden rift systems to the greater East African Rift System. Over the past 1 Myr, the Gulf of Aden spreading centre has continued to propagate westwards into the Afar and now links to the Red Sea plate boundary via the west side of the Danakil microplate. Consequently, Arabia and Africa are now kinematically decoupled.
Author Contributions
SB and M-LB compiled the data, SB performed data interpolation and produced figures, and SB, M-LB, and BK wrote the manuscript.
Funding
Infrastructure support for the University of Melbourne Thermochronology Laboratory comes from the AuScope program of the National Collaborative Research Infrastructure Strategy (NCRIS).
Conflict of Interest
The authors declare that the research was conducted in the absence of any commercial or financial relationships that could be construed as a potential conflict of interest.
Acknowledgments
We thank the editor, Daniele Trippanera, and two anonymous reviewers for their constructive comments and suggestions. Infrastructure support for the University of Melbourne Thermochronology Laboratory comes from the AuScope program of the National Collaborative Research Infrastructure Strategy (NCRIS).
References
Abbate, E., Balestrieri, M. L., and Bigazzi, G. (2002). Morphostructural Development of the Eritrean Rift Flank (Southern Red Sea) Inferred from Apatite Fission Track Analysis. J. Geophys. Res. 107 (B11), 1–12. doi:10.1029/2001JB001009
Abbate, E., Balestrieri, M. L., and Bigazzi, G. (2001). Uplifted Rift-Shoulder of the Gulf of Aden in Northwestern Somalia: Palinspastic Reconstructions Supported by Apatite Fission-Track Data. Mém. Mus. natl. hist. nat. 186, 629–640
Abebe, T., Balestrieri, M. L., and Bigazzi, G. (2010). The Central Main Ethiopian Rift Is Younger Than 8 Ma: Confirmation Through Apatite Fission-Track Thermochronology. Terra Nova 22 (6), 470–476. doi:10.1111/j.1365-3121.2010.00968.x
Al-Areeq, N. M., Al-Badani, M. A., Salman, A. H., and Albaroot, M. A. (2018). Petroleum Source Rocks Characterization and Hydrocarbon Generation of the Upper Jurassic Succession in Jabal Ayban Field, Sabatayn Basin, Yemen. Egypt. J. Pet. 27 (4), 835–851. doi:10.1016/j.ejpe.2017.12.005
Ali, M. Y., and Lee, J. H. (2019). Petroleum Geology of the Nogal Basin and Surrounding Area, Northern Somalia, Part 2: Hydrocarbon Potential. J. Pet. Geology. 42 (3), 233–260. doi:10.1111/jpg.12732
Almalki, K. A., Betts, P. G., and Ailleres, L. (2016). Incipient Seafloor Spreading Segments: Insights from the Red Sea. Geophys. Res. Lett. 43 (6), 2709–2715. doi:10.1002/2016GL068069
Almalki, K. A., Betts, P. G., and Ailleres, L. (2015). The Red Sea - 50 Years of Geological and Geophysical Research. Earth-Science Rev. 147, 109–140. doi:10.1016/j.earscirev.2015.05.002
ArRajehi, A., McClusky, S., Reilinger, R., Daoud, M., Alchalbi, A., Ergintav, S., et al. (2010). Geodetic Constraints on Present‐day Motion of the Arabian Plate: Implications for Red Sea and Gulf of Aden Rifting. Tectonics 29 (3), 1–10. doi:10.1029/2009TC002482
Augustin, N., van der Zwan, F. M., Devey, C. W., and Brandsdóttir, B. (2021). 13 Million Years of Seafloor Spreading Throughout the Red Sea Basin. Nat. Commun. 12 (1), 1–10. doi:10.1038/s41467-021-22586-2
Avni, Y., Segev, A., and Ginat, H. (2012). Oligocene Regional Denudation of the Northern Afar Dome: Pre- and Syn-Breakup Stages of the Afro-Arabian Plate. Geol. Soc. America Bull. 124 (11–12), 1871–1897. doi:10.1130/B30634.1
Balestrieri, M. L., Abbate, E., Bigazzi, G., and El Bedri Ali, O. (2009). Thermochronological Data from Sudan in the Frame of the Denudational History of the Nubian Red Sea Margin. Earth Surf. Process. Landforms 34, 1279–1290. doi:10.1002/esp10.1002/esp.1820
Balestrieri, M. L., Stuart, F. M., Persano, C., Abbate, E., and Bigazzi, G. (2005). Geomorphic Development of the Escarpment of the Eritrean Margin, Southern Red Sea from Combined Apatite Fission-Track and (U-Th)/He Thermochronometry. Earth Planet. Sci. Lett. 231, 97–110. doi:10.1029/2001JB00100910.1016/j.epsl.2004.12.011
Bellahsen, N., Husson, L., Autin, J., Leroy, S., and d'Acremont, E. (2013). The Effect of Thermal Weakening and Buoyancy Forces on Rift Localization: Field Evidences from the Gulf of Aden Oblique Rifting. Tectonophysics 607, 80–97. doi:10.1016/j.tecto.2013.05.042
Benoit, M. H., Nyblade, A. A., VanDecar, J. C., and Gurrola, H. (2003). Upper Mantle P Wave Velocity Structure and Transition Zone Thickness Beneath the Arabian Shield. Geophys. Res. Lett. 30 (10), 1–4. doi:10.1029/2002gl016436
Bohannon, R. G., Naeser, C. W., Schmidt, D. L., and Zimmermann, R. A. (1989). The Timing of Uplift, Volcanism, and Rifting Peripheral to the Red Sea: A Case for Passive Rifting? J. Geophys. Res. 94 (B2), 1683–1701. doi:10.1029/JB094iB02p01683
Bojar, A.-V., Fritz, H., Kargl, S., and Unzog, W. (2002). Phanerozoic Tectonothermal History of the Arabian-Nubian Shield in the Eastern Desert of Egypt: Evidence from Fission Track and Paleostress Data. J. Afr. Earth Sci. 34 (3–4), 191–202. doi:10.1016/S0899-5362(02)00018-0
Bonatti, E. (1985). Punctiform Initiation of Seafloor Spreading in the Red Sea during Transition from a continental to an Oceanic Rift. Nature 316, 1–5. doi:10.1038/316033a0
Bonini, M., Corti, G., Innocenti, F., Manetti, P., Mazzarini, F., Abebe, T., et al. (2005). Evolution of the Main Ethiopian Rift in the Frame of Afar and Kenya Rifts Propagation. Tectonics 24 (1), 1–12. doi:10.1029/2004TC001680
Bosworth, W. (2015). “Geological Evolution of the Red Sea: Historical Background, Review, and Synthesis,” in The Red Sea (Trenton, NJ: Springer, Berlin, Heidelberg), 45–78.
Bosworth, W., Huchon, P., and McClay, K. (2005). The Red Sea and Gulf of Aden Basins. J. Afr. Earth Sci. 43 (1–3), 334–378. doi:10.1016/j.jafrearsci.2005.07.020
Bosworth, W., and McClay, K. (2001). Structural and Stratigraphic Evolution of the Gulf of Suez Rift, Egypt: A Synthesis. Mém. Mus. natl. hist. nat. 186, 567–606
Bosworth, W., and Stockli, D. F. (2016). Early Magmatism in the Greater Red Sea Rift: Timing and Significance. Can. J. Earth Sci. 53, 1158–1176. doi:10.1139/cjes-2016-0019Available at: http://www.ling.upenn.edu/∼kurisuto/germanic/oe_bosworthtoller_about.html
Braun, J., and van der Beek, P. (2004). Evolution of Passive Margin Escarpments: What Can We Learn from Low-Temperature Thermochronology? J. Geophys. Res. 109 (F4), 1–14. doi:10.1029/2004jf000147
Burke, K., and Dewey, J. F. (1973). Plume-Generated Triple Junctions: Key Indicators in Applying Plate Tectonics to Old Rocks. J. Geology. 81, 406–433. doi:10.1086/627882
Burov, E., and Gerya, T. (2014). Asymmetric Three-Dimensional Topography over Mantle Plumes. Nature 513 (7516), 85–89. doi:10.1038/nature13703
Camp, V. E., and Roobol, M. J. (1989). The Arabian Continental Alkali Basalt Province: Part I. Evolution of Harrat Rahat, Kingdom of Saudi Arabia. Geol. Soc. America Bull. 101, 71–95. doi:10.1130/0016-7606(1989)101<0071:tacabp>2.3.co;2
Chang, S. J., and Van der Lee, S. (2011). Mantle Plumes and Associated Flow beneath Arabia and East Africa. Earth Planet. Sci. Lett. 302, 448–454. doi:10.1016/j.epsl.2010.12.050
Cochran, J. R. (1983). A Model for Development of Red Sea. Am. Assoc. Pet. Geologists Bull. 67 (1), 41–69. doi:10.1306/03b5acbe-16d1-11d7-8645000102c1865d
Cochran, J. R., and Karner, G. D. (2007). Constraints on the Deformation and Rupturing of continental Lithosphere of the Red Sea: The Transition from Rifting to Drifting. Geol. Soc. Lond. Spec. Publications 282, 265–289. doi:10.1144/SP282.13
Cole, G. A., Abu-Ali, M. A., Coiling, E. L., Halpern, H. I., Carrigan, W. J., Savage, G. R., et al. (1995). Petroleum Geochemistry of the Midyan and Jaizan Basins of the Red Sea, Saudi Arabia. Mar. Pet. Geology. 12 (6), 597–614. doi:10.1016/0264-8172(95)98087-L
Corti, G. (2009). Continental Rift Evolution: From Rift Initiation to Incipient Break-Up in the Main Ethiopian Rift, East Africa. Earth-Science Rev. 96 (1–2), 1–53. doi:10.1016/j.earscirev.2009.06.005
Courtillot, V. (1982). Propagating Rifts and continental Breakup. Tectonics 1 (3), 239–250. doi:10.1029/tc001i003p00239
Crane, K., and Bonatti, E. (1987). The Role of Fracture Zones during Early Red Sea Rifting: Structural Analysis Using Space Shuttle Radar and LANDSAT Imagery, J. Geol. Soc. 144, 407–420. doi:10.1144/gsjgs.144.3.0407
Daradich, A., Mitrovica, J. X., Pysklywec, R. N., Willett, S. D., and Forte, A. M. (2003). Mantle Flow, Dynamic Topography, and Rift-Flank Uplift of Arabia. Geol. 31 (10), 901–904. doi:10.1130/G19661.1
Davison, I., Al-Kadasi, M., Al-Khirbash, S., Al-Subbary, A. K., Baker, J., Blakey, S., et al. (1994). Geological Evolution of the Southeastern Red Sea Rift Margin, Republic of Yemen. Geol. Soc. America Bull. 106, 1474–1493. doi:10.1130/0016-7606(1994)106<1474:geotsr>2.3.co;2
Debayle, E., Lévêque, J.-J., and Cara, M. (2001). Seismic Evidence for a Deeply Rooted Low-Velocity Anomaly in the Upper Mantle Beneath the Northeastern Afro/Arabian Continent. Earth Planet. Sci. Lett. 193 (3–4), 423–436. doi:10.1016/S0012-821X(01)00509-X
Dixon, T. H., Ivins, E. R., and Franklin, B. J. (1989). Topographic and Volcanic Asymmetry Around the Red Sea: Constraints on Rift Models. Tectonics 8 (6), 1193–1216. doi:10.1029/tc008i006p01193
Eagles, G., Gloaguen, R., and Ebinger, C. (2002). Kinematics of the Danakil Microplate. Earth Planet. Sci. Lett. 203 (2), 607–620. doi:10.1016/S0012-821X(02)00916-0
Ehlers, T. A. (2005). Crustal Thermal Processes and the Interpretation of Thermochronometer Data. Rev. Mineralogy Geochem. 58, 315–350. doi:10.2138/rmg.2005.58.12
Feinstein, S., Eyal, M., Kohn, B. P., Steckler, M. S., Ibrahim, K. M., Moh'd, B. K., et al. (2013). Uplift and Denudation History of the Eastern Dead Sea Rift Flank, SW Jordan: Evidence from Apatite Fission Track Thermochronometry. Tectonics 32, 1513–1528. doi:10.1002/tect.20082
Gallagher, K. (1995). Evolving Temperature Histories from Apatite Fission-Track Data. Earth Planet. Sci. Lett. 136 (3–4), 421–435. doi:10.1016/0012-821x(95)00197-k
Garfunkel, Z. (2014). “Lateral Motion and Deformation along the Dead Sea Transform,” in Dead Sea Transform Fault System: Reviews. Editors Z. Garfunkel, Z. Ben-Avraham, and E. Kagan (Dordrecht: Springer), 109–150. doi:10.1007/978-94-017-8872-4_5
Ghebreab, W., Carter, A., Hurford, A. J., and Talbot, C. J. (2002). Constraints for Timing of Extensional Tectonics in the Western Margin of the Red Sea in Eritrea. Earth Planet. Sci. Lett. 200 (1–2), 107–119. doi:10.1016/S0012-821X(02)00621-0
Girdler, R. W. (1984). The Evolution of the Gulf of Aden and Red Sea in Space and Time. Deep Sea Res. A. Oceanographic Res. Pap. 31 (6–8), 747–762. doi:10.1016/0198-0149(84)90039-6 Available at: http://mobile.kwansei.ac.jp/s_hws/attached/0000052629.pdf%5Cnhttp://scholar.google.com/scholar?hl=en&btnG=Search&q=intitle:No+Title#0
Gleadow, A. J. W., and Duddy, I. R. (1981). A Natural Long-Term Track Annealing Experiment for Apatite. Nucl. Tracks 5 (1–2), 169–174. doi:10.1016/0191-278x(81)90039-1
Guiraud, R., and Bosworth, W. (1999). Phanerozoic Geodynamic Evolution of Northeastern Africa and the Northwestern Arabian Platform. Tectonophysics 315 (1–4), 73–104. doi:10.1016/S0040-1951(99)00293-0
Gur, D., Steinitz, G., Kolodny, Y., Starinsky, A., and McWilliams, M. (1995). 40Ar39Ar Dating of Combustion Metamorphism ("Mottled Zone", Israel). Chem. Geology. 122 (1–4), 171–184. doi:10.1016/0009-2541(95)00034-J
Hubert-Ferrari, A., King, G., Manighetti, I., Armijo, R., Meyer, B., and Tapponnier, P. (2003). Long-term Elasticity in the Continental Lithosphere; Modelling the Aden Ridge Propagation and the Anatolian Extrusion Process. Geophys. J. Int. 153 (1), 111–132. doi:10.1046/j.1365-246X.2003.01872.x
Hughes, G. W. G., and Johnson, R. S. (2005). Lithostratigraphy of the Red Sea Region. GeoArabia 10 (3), 49–126.
Hughes, G. W., Varol, O., and Beydoun, Z. R. (1991). Evidence for Middle Oligocene Rifting of the Gulf of Aden and for Late Oligocene Rifting of the Southern Red Sea. Mar. Pet. Geology. 8, 354–358. doi:10.1016/0264-8172(91)90088-i
Keshta, S., Metwalli, F. J., and Al Arabi, H. S. (2012). Analysis of Petroleum System for Exploration and Risk Reduction in Abu Madi/Elqar’a Gas Field, Nile Delta, Egypt. Int. J. Geophys. 2012, 1–10. doi:10.1155/2012/187938
Ketcham, R. A. (2005). Forward and Inverse Modeling of Low-Temperature Thermochronometry Data. Rev. Mineralogy Geochem. 58, 275–314. doi:10.2138/rmg.2005.58.11
Khalil, H. M., Capitanio, F. A., Betts, P. G., and Cruden, A. R. (2020). 3‐D Analog Modeling Constraints on Rifting in the Afar Region. Tectonics 39 (10), 1–23. doi:10.1029/2020TC006339
Khalil, S. M., and McClay, K. R. (2002). Extensional Fault-Related Folding, Northwestern Red Sea, Egypt. J. Struct. Geology. 24 (4), 743–762. doi:10.1016/S0191-8141(01)00118-3
Khalil, S. M., and McClay, K. R. (2009). Structural Control on Syn-Rift Sedimentation, Northwestern Red Sea Margin, Egypt. Mar. Pet. Geology. 26 (6), 1018–1034. doi:10.1016/j.marpetgeo.2008.09.001
Kohn, B. P., and Eyal, M. (1981). History of Uplift of the Crystalline Basement of Sinai and its Relation to Opening of the Red Sea as Revealed by Fission Track Dating of Apatites. Earth Planet. Sci. Lett. 52, 129–141. doi:10.1016/0012-821x(81)90215-6
Kohn, B. P., Feinstein, S., Foster, D. A., Steckler, M. S., and Eyal, M. (1997). Thermal History of the Eastern Gulf of Suez, II. Reconstruction from Apatite Fission Track and K-Feldspar Measurements. Tectonophysics 283 (1–4), 219–239. doi:10.1016/S0040-1951(97)00069-3
Kohn, B., Weissbrod, T., Chung, L., Farley, K., and Bodorkos, S. (2019). Low‐temperature Thermochronology of Francolite: Insights into Timing of Dead Sea Transform Motion. Terra Nova 31 (3), 205–219. doi:10.1111/ter.12387
Kröner, A., and Stern, R. J. (2004). Pan-African Orogeny. Encycl. Geology. 1, 1–12. doi:10.1016/S1342-937X(05)70162-3
Laslett, G. M., Green, P. F., Duddy, I. R., and Gleadow, A. J. W. (1987). Thermal Annealing of Fission Tracks in Apatite 2. A Quantitative Analysis. Chem. Geology. Isotope Geosci. section 65, 1–13. doi:10.1016/0168-9622(87)90057-1
Leroy, S., Gente, P., Fournier, M., d’Acremont, E., Patriat, P., Beslier, M.-O., et al. (2004). From Rifting to Spreading in the Eastern Gulf of Aden: A Geophysical Survey of a Young Oceanic basin from Margin to Margin. Terra Nova 16, 185–192. doi:10.1111/j.1365-3121.2004.00550.x
Menzies, M. A., Baker, J., Bosence, D., Dart, C., Davison, I., Hurford, A., et al. (1992). The Timing of Magmatism, Uplift and Crustal Extension: Preliminary Observations from Yemen. Geol. Soc. Lond. Spec. Publications, 68, 293–304. doi:10.1144/GSL.SP.1992.068.01.18
Menzies, M., Gallagher, K., Yelland, A., and Hurford, A. J. (1997). Volcanic and Nonvolcanic Rifted Margins of the Red Sea and Gulf of Aden: Crustal Cooling and Margin Evolution in Yemen. Geochim. Cosmochim. Acta 61 (12), 2511–2527. doi:10.1016/s0016-7037(97)00108-7
Milanovsky, E. E. (1972). Continental Rift Zones: Their Arrangement and Development. Tectonophysics 15, 65–70. doi:10.1016/B978-0-444-41087-0.50013-010.1016/0040-1951(72)90052-2
Mohriak, W. (2019). “Rifting and Salt Deposition on Continental Margins: Differences and Similarities Between the Red Sea and the South Atlantic Sedimentary Basins,” in Geological Setting, Palaeoenvironment and Archaeology of the Red Sea. Editors N. M. A. Rasul, and I. C. F. Stewart (New York: Springer International Publishing), 159–202.
Morag, N., Haviv, I., Eyal, M., Kohn, B. P., and Feinstein, S. (2019). Early Flank Uplift along the Suez Rift: Implications for the Role of Mantle Plumes and the Onset of the Dead Sea Transform. Earth Planet. Sci. Lett. 516, 56–65. doi:10.1016/j.epsl.2019.03.002
Müller, R. D., Seton, M., Zahirovic, S., Williams, S. E., Matthews, K. J., Wright, N. M., et al. (2016). Ocean Basin Evolution and Global-Scale Plate Reorganization Events since Pangea Breakup. Annu. Rev. Earth Planet. Sci. 44, 107–138. doi:10.1146/annurev-earth-060115-012211
Naylor, D., Al-Rawi, M., Clayton, G., Fitzpatrick, M. J., and Green, P. F. (2013). Hydrocarbon Potential in Jordan. J. Pet. Geology. 36 (3), 205–236. doi:10.1111/jpg.12553
Nuriel, P., Weinberger, R., Kylander-Clark, A. R. C., Hacker, B. R., and Craddock, J. P. (2017). The Onset of the Dead Sea Transform Based on Calcite Age-Strain Analyses. Geology 45 (7), 587–590. doi:10.1130/G38903.1
Omar, G. I., Kohn, B. P., Lutz, T. M., and Faul, H. (1987). The Cooling History of Silurian to Cretaceous Alkaline Ring Complexes, South Eastern Desert, Egypt, as Revealed by Fission-Track Analysis. Earth Planet. Sci. Lett. 83 (1–4), 94–108. doi:10.1016/0012-821X(87)90054-9
Omar, G. I., Steckler, M. S., Buck, W. R., and Kohn, B. P. (1989). Fission-track Analysis of Basement Apatites at the Western Margin of the Gulf of Suez Rift, Egypt: Evidence for Synchroneity of Uplift and Subsidence. Earth Planet. Sci. Lett. 94 (3–4), 316–328. doi:10.1016/0012-821X(89)90149-0
Omar, G. I., and Steckler, M. S. (1995). Fission Track Evidence on the Initial Rifting of the Red Sea: Two Pulses, No Propagation. Science 270 (5240), 1341–1344. doi:10.1126/science.270.5240.1341
Pik, R., Marty, B., Carignan, J., and Lavé, J. (2003). Stability of the Upper Nile Drainage Network (Ethiopia) Deduced from (U-Th)/He Thermochronometry: Implications for Uplift and Erosion of the Afar Plume Dome. Earth Planet. Sci. Lett. 215, 73–88. doi:10.1016/S0012-821X(03)00457-6
Ritsema, J., van Heijst, H.-J., and Woddhouse, J. H. (1999). Complex Shear Wave Velocity Structure Imaged Beneath Africa and Iceland. Science 286 (5446), 1925–1928. doi:10.1126/science.286.5446.1925
Sengör, A. M. C., and Burke, K. (1978). Relative Timing of Rifting and Volcanism on Earth and its Tectonic Implications. Geophys. Res. Lett. 5 (6), 419–421. doi:10.1029/gl005i006p00419
Sengör, A. M. C., and Yilmaz, Y. (1981). Tethyan Evolution of Turkey: A Plate Tectonic Approach. Tectonophysics 75 (3–4), 181–241. doi:10.1016/0040-1951(81)90275-4
Steckler, M. S., and ten Brink, U. S. (1986). Lithospheric Strength Variations as a Control on New Plate Boundaries: Examples from the Northern Red Sea Region. Earth Planet. Sci. Lett. 79 (1–2), 120–132. doi:10.1016/0012-821X(86)90045-2
Stockli, D. F. (2005). Application of Low-Temperature Thermochronometry to Extensional Tectonic Settings. Rev. Mineralogy Geochem. 58, 411–448. doi:10.2138/rmg.2005.58.16
Szymanski, E., Stockli, D. F., Johnson, P. R., and Hager, C. (2016). Thermochronometric Evidence for Diffuse Extension and Two-phase Rifting Within the Central Arabian Margin of the Red Sea Rift. Tectonics 35 (12), 2863–2895. doi:10.1002/2016TC004336
Thiéblemont, D., Liégeois, J. P., Fernandez-Alonso, M., Ouabadi, A., Le Gall, B., Maury, R., et al. (2016). Geological Map of Africa at 1:10M Scale, CGMW-BRGM 2016.
Tsegaye, S. G., Nton, M. E., Boboye, O. A., and Ahmed, W. (2018). Geochemical Characteristics and Hydrocarbon Generation Modelling of Early Triassic to Late Cretaceous Formations within Ogaden Basin, Ethiopia. J. Pet. Sci. Tech. 8 (4), 58–75. doi:10.22078/jpst.2018.3163.1508
Tubbs, R. E., Fouda, H. G. A., Afifi, A. M., Raterman, N. S., Hughes, G. W., and Fadolalkarem, Y. K. (2014). Midyan Peninsula, Northern Red Sea, Saudi Arabia: Seismic Imaging and Regional Interpretation. GeoArabia 19 (3), 165–184
van der Beek, P., Andriessen, P., and Cloetingh, S. (1995). Morphotectonic Evolution of Rifted continental Margins: Inferences from a Coupled Tectonic-Surface Processes Model and Fission Track Thermochronology. Tectonics 14 (2), 406–421. doi:10.1029/94tc02445
Vermeesch, P., Avigad, D., and McWilliams, M. O. (2009). 500 m.Y. Of thermal History Elucidated by Multi-Method Detrital Thermochronology of North Gondwana Cambrian sandstone (Eilat Area, Israel). Bull. Geol. Soc. America 121 (7–8), 1204–1216. doi:10.1130/B26473.1
Voggenreiter, W., Hötzl, H., and Jado, A. R. (1988). Red Sea Related History of Extension and Magmatism in the Jizan Area (Southwest Saudi Arabia): Indication for Simple-Shear During Early Red Sea Rifting. Geol. Rundsch 77 (1), 257–274. doi:10.1007/BF01848688
Wildman, M., Cogné, N., and Beucher, R. (2019). “Fission-Track Thermochronology Applied to the Evolution of Passive Continental Margins,” in Fission-Track Thermochronology and its Application to Geology. Editors M. G. Malusà, and P. G. Fitzgerald (New York: Springer, Berlin, Heidelberg), 351–371.
Wolela, A. (2007). Source Rock Potential of the Blue NIle (Abay) Basin, Ethiopia. J. Pet. Geol. 30, 389–402. doi:10.1111/j.1747-5457.2007.00389.x
Wolf, R. A., Farley, K. A., and Kass, D. M. (1998). Modeling of the Temperature Sensitivity of the Apatite (U-Th)/He Thermochronometer. Chem. Geology. 148, 105–114. doi:10.1016/s0009-2541(98)00024-2
Wolfenden, E., Ebinger, C., Yirgu, G., Deino, A., and Ayalew, D. (2004). Evolution of the Northern Main Ethiopian Rift: Birth of A Triple Junction. Earth Planet. Sci. Lett. 224 (1–2), 213–228. doi:10.1016/j.epsl.2004.04.022
Wolfenden, E., Ebinger, C., Yirgu, G., Renne, P. R., and Kelley, S. P. (2005). Evolution of a Volcanic Rifted Margin: Southern Red Sea, Ethiopia. Geol. Soc. America Bull. 117 (7–8), 846–864. doi:10.1130/B25516.1
Younes, A. I., and Mcclay, K. (2002). Development of Accommodation Zones in the Gulf of Suez-Red Sea Rift, Egypt. AAPG Bull. 86 (6), 1003–1026
Keywords: fission track, (U-Th-Sm)/He, tectonics, geodynamics, rifting, geomorphology, thermochronology, continental breakup
Citation: Boone SC, Balestrieri M-L and Kohn B (2021) Tectono-Thermal Evolution of the Red Sea Rift. Front. Earth Sci. 9:713448. doi: 10.3389/feart.2021.713448
Received: 23 May 2021; Accepted: 30 June 2021;
Published: 12 July 2021.
Edited by:
Daniele Trippanera, King Abdullah University of Science and Technology, Saudi ArabiaReviewed by:
Stuart Hardy, Catalan Institution for Research and Advanced Studies (ICREA), SpainJing Ye, King Abdullah University of Science and Technology, Saudi Arabia
Copyright © 2021 Boone, Balestrieri and Kohn. This is an open-access article distributed under the terms of the Creative Commons Attribution License (CC BY). The use, distribution or reproduction in other forums is permitted, provided the original author(s) and the copyright owner(s) are credited and that the original publication in this journal is cited, in accordance with accepted academic practice. No use, distribution or reproduction is permitted which does not comply with these terms.
*Correspondence: Samuel C. Boone, samuel.boone@unimelb.edu.au