- 1Pharmaceutical College, Changsha Health Vocational College, Changsha, China
- 2Department of Pathology, Changsha Health Vocational College, Changsha, China
- 3National Engineering Laboratory for Rice and By-Product Deep Processing, College of Food Science and Engineering, Central South University of Forestry and Technology, Changsha, China
- 4Department of Spleen, Stomach and Liver Diseases, Affiliated Hospital of Hunan Academy of Traditional Chinese Medicine, Changsha, China
Introduction: Chronic and acute chlorogenic acid (CGA) can improve glucose tolerance (GT) and insulin sensitivity (IS). However, whether acute administration of CGA has beneficial effects on hepatic lipid metabolism and cecal microbiota composition remains unclear.
Methods: In the current study, diabetic db/db mice were administered CGA or metformin, and db/m mice were used as controls to explore the effects of CGA on hepatic lipid metabolism, including fatty acid oxidation and transportation and triglyceride (TG) lipolysis and synthesis. Moreover, alterations in the inflammatory response and oxidative stress in the liver and gut microbe composition were evaluated.
Results: The results showed that CGA decreased body weight and improved glucose tolerance and insulin resistance, and these effects were similar to those of metformin. CGA decreased hepatic lipid content by increasing the expression of CPT1a (carnitine palmitoyltransferase 1a), ACOX1 (Acyl-CoA oxidase 1), ATGL (adipose triglyceride lipase), and HSL (hormone-sensitive lipase) and decreasing that of MGAT1 (monoacylglycerol O-acyltransferase 1), DGAT1 (diacylglycerol O-acyltransferase), DGAT2, CD36, and FATP4 (fatty acid transport protein 4). Additionally, CGA restored the expression of inflammatory genes, including TNF-α (tumor necrosis factor-alpha), IL-1β (interleukin-1beta), IL-6, and IL-10, and genes encoding antioxidant enzymes, including SOD1 (superoxide dismutases 1), SOD2 (superoxide dismutases 2), and GPX1 (glutathione peroxidase 1). Furthermore, CGA improved the bacterial alpha and beta diversity in the cecum. Moreover, CGA recovered the abundance of the phylum Bacteroidetes and the genera Lactobacillus, Blautia, and Enterococcus.
Discussion: CGA can improve the antidiabetic effects, and microbes may critically mediate these beneficial effects.
Introduction
Chlorogenic acid (CGA), a member of the hydroxycinnamic acid family, is abundant in many plants, including coffee beans, apples, tea, and tobacco leaves (1). CGA has been proven to be one of the most promising phenolic acids, serving as either a food additive or nutraceutical due to its efficacy in alleviating oxidative stress, the inflammatory response, and glucose and lipid metabolism disorders (1–3). Based on these effects, the use of CGA for treating metabolic syndromes, such as type 2 diabetes mellitus and cardiovascular diseases, has been widely studied (4). Human and animal studies have confirmed that CGA can dramatically lower total cholesterol and total triglyceride levels (5, 6). In overweight patients, taking CGA twice a day can improve blood glucose, insulin sensitivity and other metabolic parameters (7). In high-fat diet (HFD)-fed mice, CGA exerts its protective effect on obesity and related metabolic syndrome by regulating gut microbiota structure, diversity, and changes in relative abundance at the phylum to genus levels (8). AMPK (AMP-activated protein kinase) signaling and gut microbes are involved in the anti-diabetic and anti-lipidemic effects of CGA (9). However, the detailed mechanisms require further investigation.
Gut microbes are considered to be important health regulators, and dietary components are one of the major factors determining the composition of the gut microbiota (10, 11). Approximately 30% of dietary CGA is absorbed in the small intestine (12, 13), indicating that CGA can reach the large intestine and exert direct effects on microbes. Numerous studies have demonstrated the modulatory effects of CGA on the diversity and composition of the microbiota. CGA can decrease the abundance of Lachnospiraceae, Ruminococcaceae, Desulfovibrionaceae, and Erysipelotrichaceae and increase that of Lactobacillaceae and Bacteroidaceae (14). Significantly, CGA improved the abundance of beneficial bacteria, including Ruminococcaceae UCG-005, Akkermansia, Christensenellaceae R-7_group, and Rikenellaceae RC9_gut_group (15) and short-chain fatty acid producers such as Faecalibaculum, Romboutsia, Mucispirillum, and Dubosiella (16). Meanwhile, CGA reduced the abundance of bacteria involved in inflammation, such as Sutterella species and those that cause intestinal dysfunction, such as Escherichia coli and other LPS-producers (17, 18). These changes in microbiota composition, induced by CGA, mediate the positive effects of CGA on metabolic disorders. However, whether CGA administration can recover the microbiota composition of diabetic mice has not been fully determined.
A previous study reported the acute effects of CGA on glucose tolerance (GT), insulin sensitivity (IS), gluconeogenesis, and fatty acid synthesis in Leprdb diabetic (db/db) mice (11). However, whether acute treatment with CGA affects fatty acid oxidation, inflammatory responses, and microbiota composition remains to be explored. Consequently, we investigated the acute effects of CGA on lipid metabolism and the inflammatory and antioxidative status in db/db diabetic mice. Furthermore, the involvement of intestinal microbes in the beneficial effects of CGA has been elucidated
Materials and methods
Animal experiments
Eighteen male diabetic db/db mice and six male db/m mice were provided by Gene&Peace Biotech Co., Ltd (Guangzhou, China). The db/db mice were assigned to the following treatment groups: (1) DB group, animals were orally gavaged with 0.1 mL PBS once a day; (2) CA group, animals were gavaged with 0.25 g CGA/kg body weight (BW) once a day; (3) MET, animals were gavaged with 0.25 g metformin/kg BW once a day. The concentration of CGA used was based on our preliminary experiment. The db/m mice were assigned as the control (CON). CGA and metformin were purchased from Sigma (Shanghai, China). The experiment lasted 18 days. All mice had free access to water and feed. Feed intake and BW were recorded during the experiment. Animals were treated humanely, using approved procedures in accordance with the guidelines of Changsha Health Vocational College. The study was approved by the Institutional Animal Care and Use Committee of Changsha Health Vocational College (2021053).
Oral GT and IS tests
Fasting blood glucose was tested every 7 days, and it was significantly decreased on Day 14 after treatment with CGA or metformin. Then, oral GT and IS tests were performed. After fasting for 6 h, mice were either orally gavaged with a dose of 2.0 g glucose or intraperitoneally injected with a dose of 0.65 U insulin/kg BW. Blood was obtained from the tail vein, and the glucose concentration was analyzed at 0, 30, 60 and 120 min.
Sample collection
First, blood was obtained from the retro-orbital sinus and serum samples were separated. Then, after cervical dislocation, liver, inguinal and epididymal adipose tissue were separated and weighed. Furthermore, liver samples were either fixed in formaldehyde solution or stored at −80°C for analysis of gene expression. Additionally, cecum content was obtained for the determination of gut microbiota.
Serum biochemistry assay
The contents of glucose, high-density lipoprotein cholesterol (HDL-C), low-density lipoprotein cholesterol (LDL-C), total cholesterol (TC) and triglycerides (TGs), and the activities of alanine aminotransferase (ALT) and aspartate aminotransferase (AST) were determined by using commercial kits (Meimian, Nantong, China) as previously described (19).
Hepatic histological and lipid content analyses
Fixed liver samples were paraffin embedded and sectioned into 8-μm thick sections; then, the samples were either subjected to H&E (hematoxylin–eosin) staining for histological observation or Oil Red O staining for the determination of lipid content as previously described (20).
RT‐qPCR
Total RNA was extracted using Trizol reagent (Invitrogen, Shanghai, China) and DNA was removed after treatment with DNase I (Takara, Beijing, China). Then, cDNA was synthesized using a RevertAid Reverse Transcription Kit (Thermo Scientific) as previously described (21). RT‐qPCR was carried out by using SYBR Green mix (Applied Biosystems). The primers used for qPCR are shown in Supplementary Table 1.
Gut microbiota profiling
DNA was extracted with cecum contents, and bacterial 16S rRNA gene sequences (V3–V4 regions) were amplified. PCRs were carried out with Phusion High-Fidelity PCR Master Mix (Thermo Fisher). Then, MiSeq Illumina sequencing was performed with products with a length of 400-450 bp. The Greengenes database with the RDP algorithm was used to analyze representative OTUs.
Statistical analysis
Statistical analysis was performed using one-way ANOVA with Tukey’s post hoc test using SPSS 18.0. All data were expressed as the means ± SEM, and significant differences were confirmed when P < 0.05.
Results
Effects of chlorogenic acid on body weight, feed intake and adipose tissue and liver weight in db/db mice
As shown in Table 1, the BW of db/db diabetic mice was significantly higher than that of db/m mice at the beginning of the experiments. The BW of mice in the CA and MET groups was significantly lower than that in the DB group, whereas it remained significantly higher than that in the CON group on day 7, 14 and 18. Mice in the CA and MET group had lower daily feed intake than those in the DB group, whereas they had higher daily feed intake than those in the CON group. As shown in Table 2, the weights of inguinal fat and liver in mice in the CA and MET groups were significantly lower than those in the DB group, whereas they were significantly higher than those in the CON group. Epididymal fat weight was significantly lower in mice in the CON group than in the other three groups.
Effects of chlorogenic acid on GT and IS in db/db mice
According to Figure 1, either chlorogenic acid or metformin improved GT and IS in db/db mice, whereas all the db/db mice still showed lower GT and IS than the db/m mice.
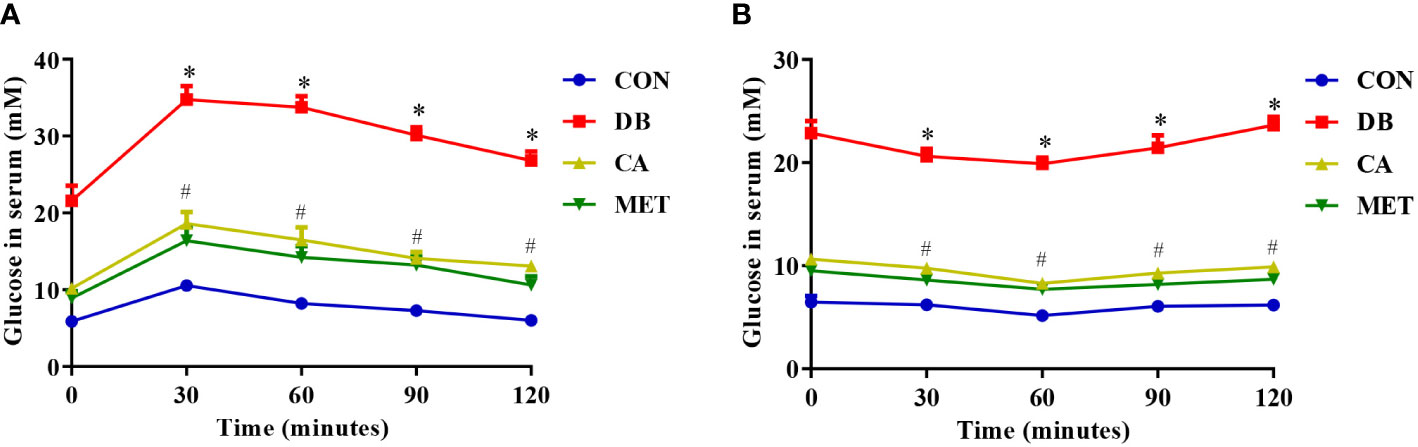
Figure 1 Effects of chlorogenic acid on glucose tolerance and insulin resistance in diabetic db/db mice. (A) Glucose tolerance; (B) Insulin resistance. Data are expressed as the means ± SEMs, n=6. *P < 0.05 (significant difference between mice in the DB group and mice in the other groups); #P < 0.05 (significant difference between mice in the CON group and mice in the CA and MET groups).
Effects of chlorogenic acid on serum biochemical indicators in db/db mice
As shown in Table 3, glucose and TG contents were significantly lower in mice in the CA and MET groups than in the DB group, whereas they were significantly higher than those in the CON group. Compared with those in the DB group, mice in the other three groups had significantly lower TC and LDL-C contents, and higher HDL-C contents. The activities of AST and ALT were significantly lower in mice in the CA and MET groups than in the DB group, whereas they were significantly higher in mice in the CA group than in the CON group.
Effects of chlorogenic acid on hepatic morphology and lipid content in db/db mice
According to Figure 2, H&E staining showed that liver morphology was significantly damaged (microvesicular fatty change) in db/db diabetic mice, whereas the administration of chlorogenic acid and metformin both alleviated the impairment, although the morphology was still not returned to normal. Oil red O staining showed that db/db diabetic mice had higher lipid accumulation in the liver, whereas the administration of chlorogenic acid and metformin both decreased hepatic lipid accumulation.
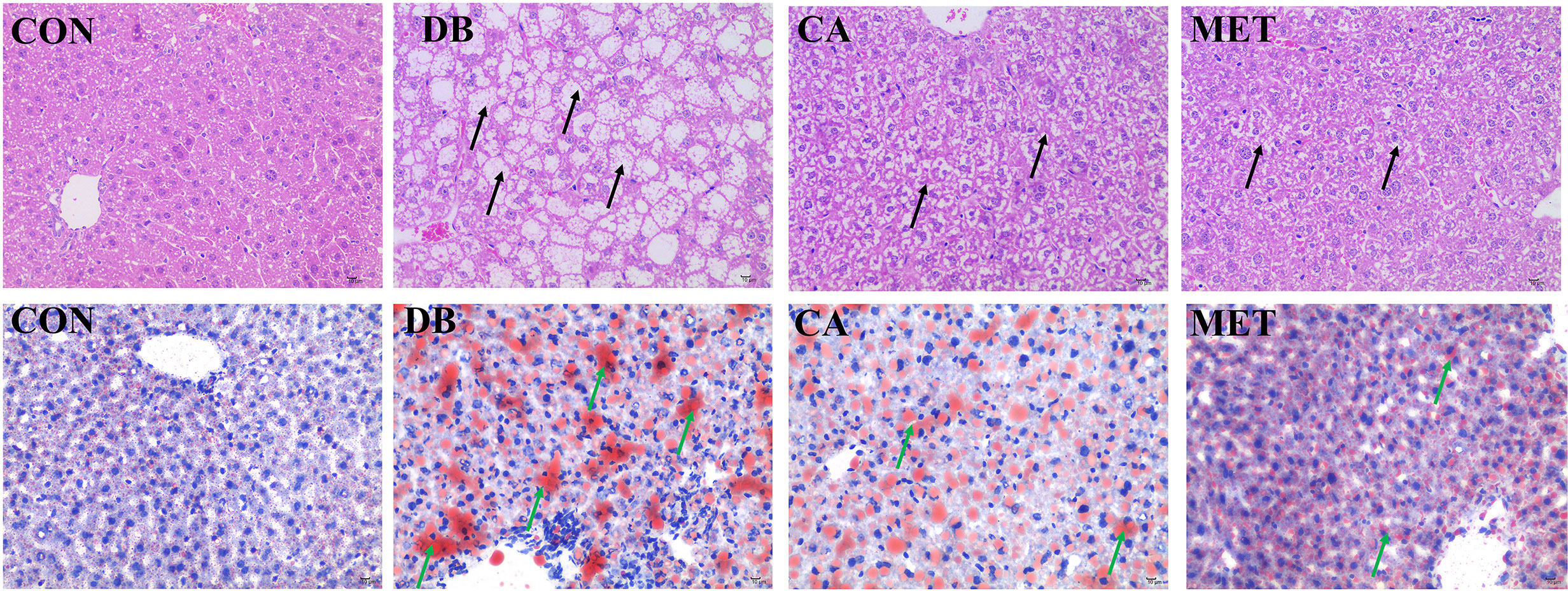
Figure 2 Effects of chlorogenic acid on liver morphology and lipid accumulation in diabetic db/db mice. Upper panel, representative results of H&E staining (400×); Lower panel, representative results of Oil red O staining (400×). Black arrows, damaged hepatocytes; green arrows, lipid droplets.
Effects of chlorogenic acid on expression of lipid metabolism-related genes in live db/db mice
The expression of genes including CPT1a, ACOX1 (Figure 3A), ATGL and HSL (Figure 3B) was significantly lower in mice in the CON and CA groups than in the MET group, whereas they were significantly higher than those in the DB group. Compared with mice in the DB group, mice in the other three groups had significantly lower expression of genes involved in TG synthesis (MGAT1, DGAT1 and DGAT2) (Figure 3C) and fatty acid transport (CD36 and FATP4) (Figure 3D).
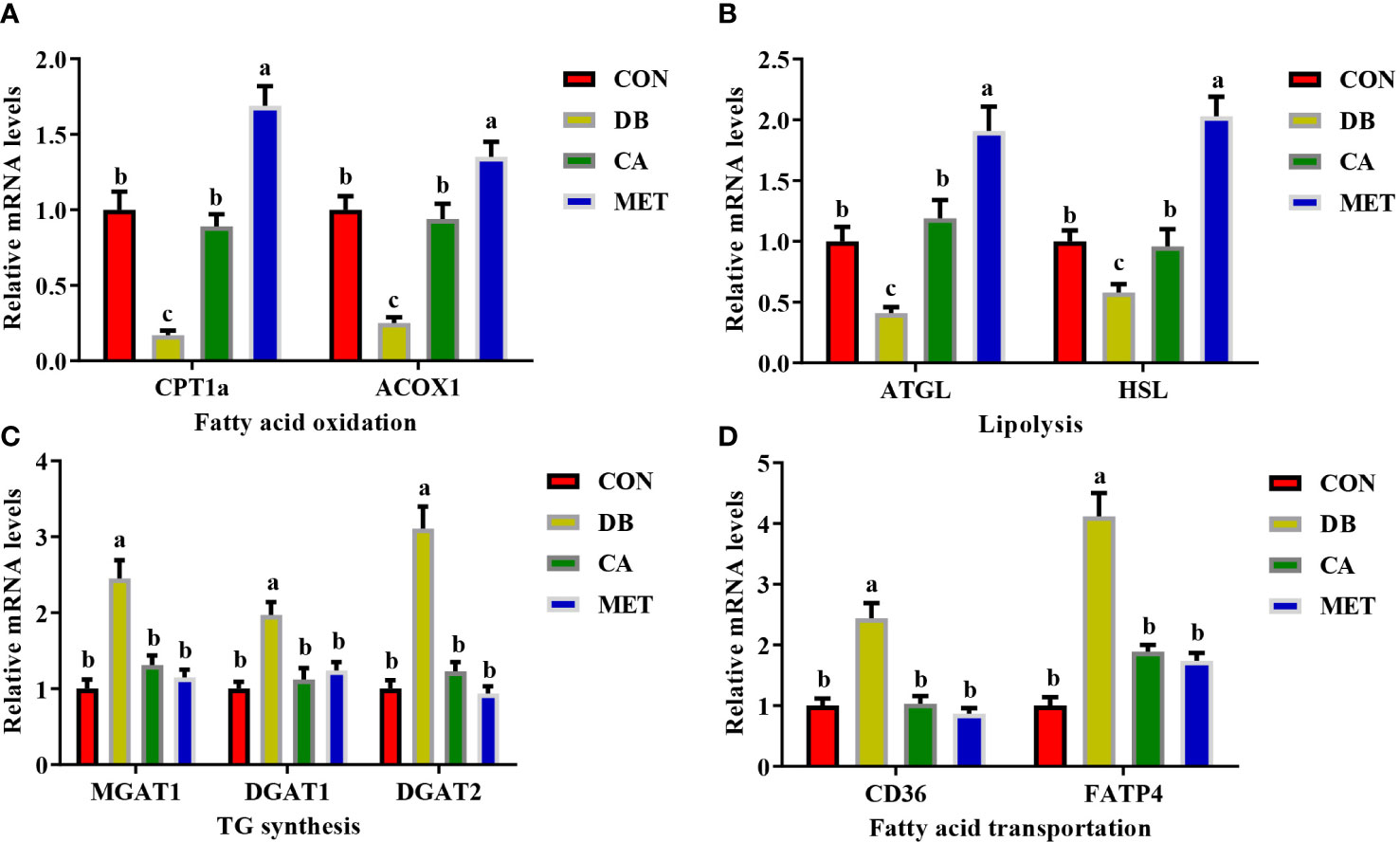
Figure 3 Effects of chlorogenic acid on the expression of genes involved in lipid metabolism in the livers of diabetic db/db mice. (A) Expression of genes involved in fatty acid oxidation; (B) Expression of genes involved in lipolysis; (C) Expression of genes involved in TG synthesis; (D) Expression of genes involved in fatty acid transportation. Data are expressed as the means ± SEMs, n=6. a,b,c Groups that share the same superscript letters are not significantly different from each other (P < 0.05).
Effects of chlorogenic acid on the expression of inflammation- and antioxidant ability-related genes in live db/db mice
Compared with mice in the DB group, mice in the other three groups had significantly lower expression of genes (IL-1β, TNF-α and IL-6) encoding proinflammatory cytokines and higher expression of the IL10 gene encoding an anti-inflammatory cytokine (Figure 4A). Compared with mice in the DB group, mice in the other three groups had significantly higher expression of genes including SOD1, SOD2 and GPX1 (Figure 4B).
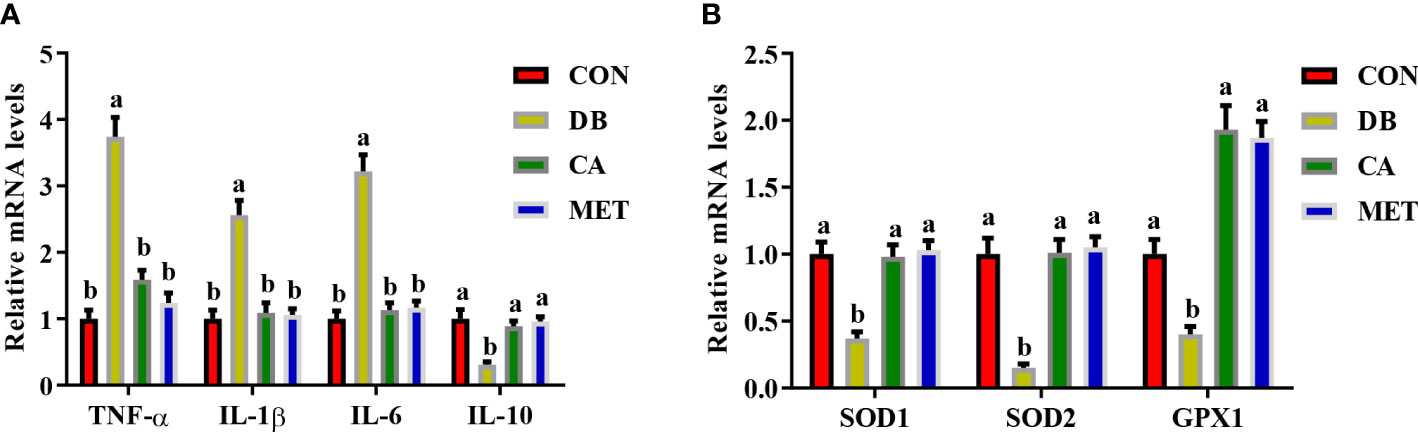
Figure 4 Effects of chlorogenic acid on the expression of genes encoding inflammatory cytokines and antioxidant enzymes in the livers of diabetic db/db mice. (A) Expression of genes encoding inflammatory cytokines; (B) Expression of genes encoding antioxidant enzymes. Data are expressed as the means ± SEMs, n=6. a,b Groups that share the same superscript letters are not significantly different from each other (P < 0.05).
Effects of chlorogenic acid on cecal microbiota composition in db/db mice
The alpha diversity of the microbial community, as indicated by the Shannon and Simpson index (Figures 5A, B), was significantly higher in mice in the CA and MET groups than in the DB group, whereas there was no significant difference among mice in the CA, MET and CON groups. Principal coordinates analysis (PCoA) showed that mice in the DB group were clearly separated from those in the other treatment groups (Figure 5C). Firmicutes, Proteobacteria and Bacteroidetes were the most abundant microbes at the phylum- level (Figure 5D). Compared with mice in the other groups, Bacteroidetes abundance was lower while Proteobacteria abundance was higher in mice of the DB group. Compared with mice in the other groups, Lactobacillus abundance at a genus-level taxonomy was lower while Blautia, Robinsoniella and Enterococcus abundance were higher in mice of the DB group (Figure 5E).
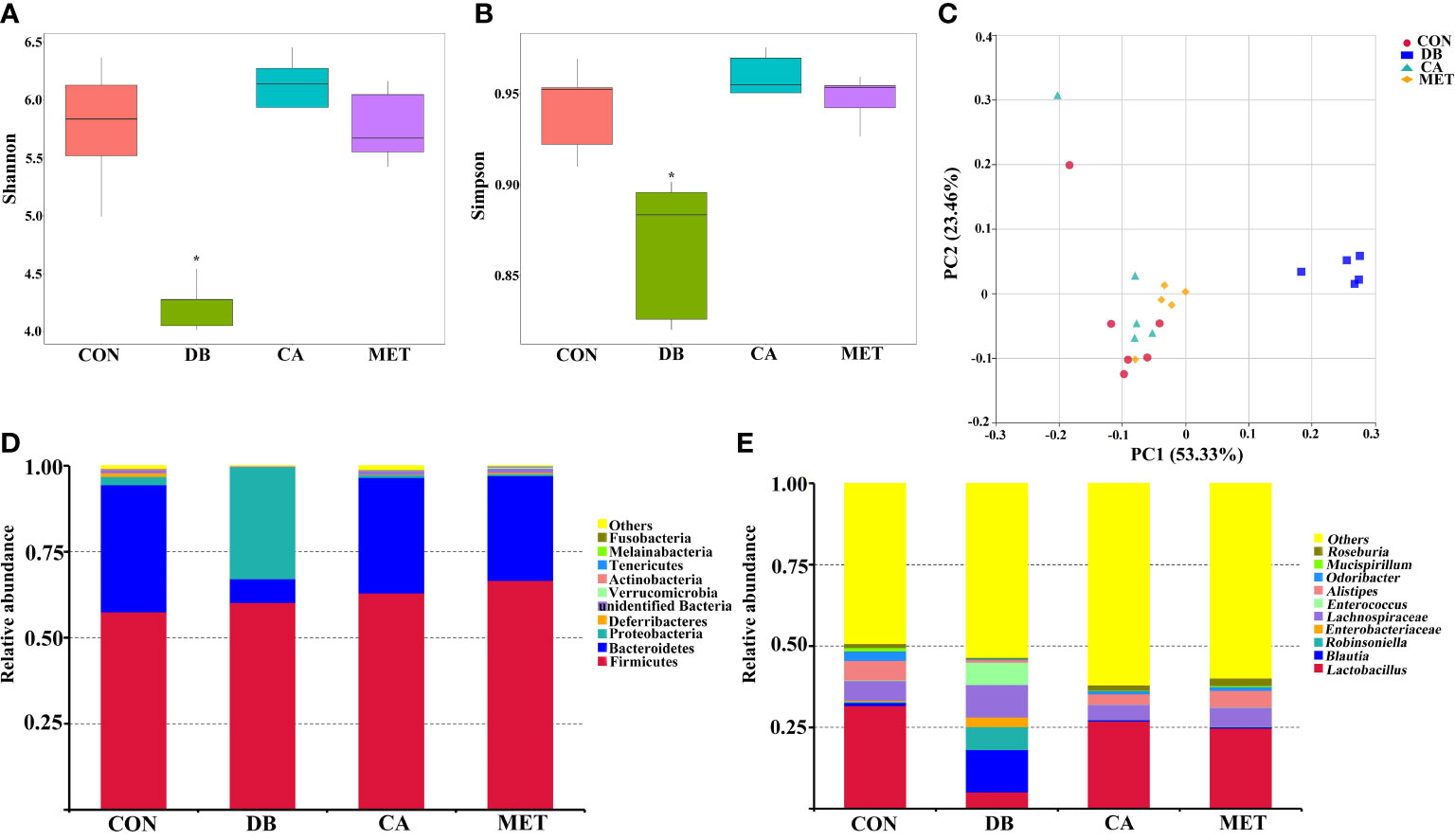
Figure 5 Effects of chlorogenic acid on cecal microbe composition in diabetic db/db mice. (A) Shannon index; (B) Simpson index; (C) PCoA plot of the microbiota based on an unweighted UniFrac metric; (D) Relative abundance of predominant bacteria at the phylum level; (E) Relative abundance of predominant bacteria at the genus level. *P < 0.05 (significant difference between mice in the DB group and mice in the other groups).
Discussion
Db/db mice are commonly used models for studying diabetes. Chronic administration of a relatively low CGA concentration or acute administration of a high concentration in db/db mice improved GT and IS (22, 23). In the present study, CGA alleviated hyperglycemia, and hyperlipidemia and decreased hepatic lipid overaccumulation in db/db mice. Moreover, our results suggest that CGA alleviates the inflammatory response and enhances antioxidant activity. Furthermore, CGA improved microbiota diversity and recovered their composition in the cecum of db/db mice.
CGA can decrease lipid accumulation in obese individuals. In the present study, short-term administration of CGA reduced body weight, adipose tissue, and liver weight in db/db mice, although their weight was not comparable to that of control db/m mice. Previous studies have focused on the effects of CGA on gluconeogenesis and fatty acid synthesis (24–26), while our results suggest that CGA enhances hepatic lipolysis and reduces TG synthesis and fatty acid transportation in the liver of db/db mice. In addition, CGA improved the expression of genes involved in hepatic lipid metabolism in high-fat diet (HFD)-fed mice (27). These results suggest that CGA improves hepatic lipid metabolism in animal models of lipid metabolic disorders. Hepatic lipid overaccumulation is usually associated with activated inflammatory responses (28–30). Our results suggest that CGA alleviates inflammation, similar to a previous study in mice with HFD-induced obesity (31). HFD resulted in an imbalance in the oxidative status, which always accompanied by inflammation, and CGA administration recovered the antioxidant status in rats. The results confirmed the antioxidative ability of CGA, as indicated by the increased expression of genes encoding enzymes such as SOD and GPX (32, 33). Although our results showed the anti-inflammatory and anti-lipidemic effects of CGA in db/db mice, the specific targets of CGA and the possible signaling pathways that regulate the alteration in inflammation and lipid metabolism remain to be explored.
The gut microbiota can influence the differentiation and apoptosis of intestinal epithelial cells and positive regulation of the gut microbiota is conducive to alleviating metabolic syndromes (34–36). Some short-chain fatty acids among the metabolites of gut microbiota are capable of improving diet-induced obesity and insulin resistance (37). Several studies have demonstrated that the beneficial role of CGA is positively related to gut microbiota regulation (18, 38). For instance, CGA could increase the contents of Bifidobacterium and reduce Escherichia coli in NAFLD mice (17). CGA also exerts its antiobesity effects by ameliorating HFD-induced gut microbiota dysbiosis by inhibiting the growth of Desulfovibrionaceae, Ruminococcaceae, Lachnospiraceae, and Erysipelotrichaceae, and raising the growth of Bacteroidaceae, Lactobacillaceae (14, 39). However, studies have also demonstrated that CGA- treated mice showed microbial structures similar to those of the control group (40–43). According to these sudies and our results, we speculated that CGA could regulate gut microbiota structure under different pathological conditions in different ways. In our study, we found that CGA or metformin increased the α-diversity of the gut microbiota in db/db mice. Additionally, the β-diversity, as indicated by the PCoA, showed that mice administered CGA or metformin were similar to control mice but different from untreated db/db mice. These effects of CGA on the improvement of gut microbiota diversity were consistent with previous results using different models (44, 45). HFD-induced obesity and glucose and lipid metabolism disorders are often accompanied by increased abundance of Firmicutes and decreased abundance of Bacteroidetes and Proteobacteria (16). However, our results only showed similar results that Bacteroidetes abundance was decreased, whereas Firmicutes abundance was not changed and Proteobacteria abundance was increased in db/db mice. Another study showed that db/db mice did not show the alterations mentioned above when compared to wild-type m/m mice (46). These results suggest that different models of diabetic mice exhibit inconsistent changes in microbiota composition. Nevertheless, CGA restored the structure of the gut microbiota, and these effects were similar to those of metformin.
The most significant change at the genus level after CGA administration was the recovery of Lactobacillus abundance. This result suggested that CGA exerted beneficial effects, as most Lactobacillus species are considered to be beneficial microbes (47, 48). Surprisingly, Blautia abundance significantly increased in db/db mice, whereas CGA administration reduced this abundance. Blautia is a functional genus with probiotic properties, including improved metabolic function and host health (49). We speculated that the recovery of Blautia abundance by CGA could be an indirect effect and that Blautia may not be the critical genus mediating the beneficial effects of CGA. However, the underlying mechanisms need to be elucidated further. Additionally, the abundance of Robinsoniella, a spore-forming genus, was significantly increased in db/db mice, whereas CGA administration restored it. Alterations in the abundance of Robinsoniella are responsible for causing diseases such as nonalcoholic fatty liver and colitis (50, 51), indicating its role in lipid metabolism and inflammation. CGA administration notably decreased the abundance of Enterococcus, an important pathogen, when it reached a high density (24). These results indicate the modulatory effects of CGA on dysbiosis of the intestinal microbiota in diabetic db/db mice. However, the changes in microbes that contribute to the beneficial effects of CGA, especially the lipid-lowering effects, need to be further elucidated.
In conclusion, our results suggest that the acute administration of CGA improves GT and IS in diabetic db/db mice. CGA improved lipid metabolism by enhancing fatty acid oxidation and TG lipolysis and reducing TG synthesis and fatty acid transportation in the liver. Moreover, CGA alleviated the hepatic inflammatory response and oxidative stress. Significantly, CGA improved the diversity of the microbiota and promoted the recovery of microbiota composition. These data suggest that acute CGA treatment aids the anti-diabetic effect, and microbes may mediate these effects. Our results indicate that gut microbial alterations should be carefully monitored during the use of CGA in metabolic syndromes.
Data availability statement
The data presented in the study are deposited in the NCBI repository, accession number PRJNA891362.
Ethics statement
The animal study was reviewed and approved by Institutional Animal Care and Use Committee of Changsha Health Vocational College.
Author contributions
YY and XZ designed the experiments. YY, QL, LS and KG performed the experiments, analyzed the data, and drafted the manuscript. XZ revised the manuscript. All authors contributed to the article and approved the submitted version.
Funding
This work was supported by the Changsha Municipal Natural Science Foundation (grant number kq2014029) and Natural Science Foundation of Hunan Province (2022JJ60111).
Conflict of interest
The authors declare that the research was conducted in the absence of any commercial or financial relationships that could be construed as a potential conflict of interest.
Publisher’s note
All claims expressed in this article are solely those of the authors and do not necessarily represent those of their affiliated organizations, or those of the publisher, the editors and the reviewers. Any product that may be evaluated in this article, or claim that may be made by its manufacturer, is not guaranteed or endorsed by the publisher.
Supplementary material
The Supplementary Material for this article can be found online at: https://www.frontiersin.org/articles/10.3389/fendo.2022.1042044/full#supplementary-material
Supplementary Table 1 | Primer sequences. The table provided the sequences of primers used for RT-qPCR analysis.
References
1. Miao M, Xiang L. Pharmacological action and potential targets of chlorogenic acid. Adv Pharmacol (2020) 87:71–88. doi: 10.1016/bs.apha.2019.12.002
2. Pimpley V, Patil S, Srinivasan K, Desai N, Murthy PS. The chemistry of chlorogenic acid from green coffee and its role in attenuation of obesity and diabetes. Prep Biochem Biotechnol (2020) 50(10):969–78. doi: 10.1080/10826068.2020.1786699
3. Yan Y, Zhou X, Guo K, Zhou F, Yang H. Use of chlorogenic acid against diabetes mellitus and its complications. J Immunol Res (2020) 2020:9680508. doi: 10.1155/2020/9680508
4. Santana-Gálvez J, Cisneros-Zevallos L, Jacobo-Velázquez DA. Chlorogenic acid: Recent advances on its dual role as a food additive and a nutraceutical against metabolic syndrome. Molecules (2017) 22(3):358. doi: 10.3390/molecules22030358
5. Rodriguez de Sotillo DV, Hadley M. Chlorogenic acid modifies plasma and liver concentrations of: Cholesterol, triacylglycerol, and minerals in (Fa/Fa) zucker rats. J Nutr Biochem (2002) 13(12):717–26. doi: 10.1016/s0955-2863(02)00231-0
6. Xu M, Yang L, Zhu Y, Liao M, Chu L, Li X, et al. Collaborative effects of chlorogenic acid and caffeine on lipid metabolism via the AMPKα-LXRα/SREBP-1c pathway in high-fat diet-induced obese mice. Food Funct (2019) 10(11):7489–97. doi: 10.1039/c9fo00502a
7. Rondanelli M, Riva A, Petrangolini G, Allegrini P, Bernardinelli L, Fazia T, et al. The metabolic effects of cynara supplementation in overweight and obese class I subjects with newly detected impaired fasting glycemia: A double-blind, placebo-controlled, randomized clinical trial. Nutrients (2020) 12(11):3298. doi: 10.3390/nu12113298
8. Ye X, Liu Y, Hu J, Gao Y, Ma Y, Wen D. Chlorogenic acid-induced gut microbiota improves metabolic endotoxemia. Front Endocrinol (Lausanne) (2021) 12:762691. doi: 10.3389/fendo.2021.762691
9. Ong KW, Hsu A, Tan BK. Anti-diabetic and anti-lipidemic effects of chlorogenic acid are mediated by ampk activation. Biochem Pharmacol (2013) 85(9):1341–51. doi: 10.1016/j.bcp.2013.02.008
10. Shao H, Zhang C, Xiao N, Tan Z. Gut microbiota characteristics in mice with antibiotic-associated diarrhea. BMC Microbiol (2020) 20(1):313. doi: 10.1186/s12866-020-01999-x
11. Long CX, Wu JQ, Tan ZJ. Intestinal microbiota disturbance affects the occurrence of African swine fever. Anim Biotechnol (2021) 7:1–10. doi: 10.1080/10495398.2021.2010089
12. Gonthier MP, Verny MA, Besson C, Remesy C, Scalbert A. Chlorogenic acid bioavailability largely depends on its metabolism by the gut microflora in rats. J Nutr (2003) 133(6):1853–9. doi: 10.1093/jn/133.6.1853
13. Zhang X, Zhao Q, Ci X, Chen S, Xie Z, Li H, et al. Evaluation of the efficacy of chlorogenic acid in reducing small intestine injury, oxidative stress, and inflammation in chickens challenged with clostridium perfringens type a. Poult Sci (2020) 99(12):6606–18. doi: 10.1016/j.psj.2020.09.082
14. Wang Z, Lam KL, Hu J, Ge S, Zhou A, Zheng B, et al. Chlorogenic acid alleviates obesity and modulates gut microbiota in high-Fat-Fed mice. Food Sci Nutr (2019) 7(2):579–88. doi: 10.1002/fsn3.868
15. Chen F, Zhang H, Zhao N, Yang X, Du E, Huang S, et al. Effect of chlorogenic acid on intestinal inflammation, antioxidant status, and microbial community of young hens challenged with acute heat stress. Anim Sci J (2021) 92(1):e13619. doi: 10.1111/asj.13619
16. Zhang XY, Chen J, Yi K, Peng L, Xie J, Gou X, et al. Phlorizin ameliorates obesity-associated endotoxemia and insulin resistance in high-fat diet-fed mice by targeting the gut microbiota and intestinal barrier integrity. Gut Microbes (2020) 12(1):1–18. doi: 10.1080/19490976.2020.1842990
17. Shi A, Li T, Zheng Y, Song Y, Wang H, Wang N, et al. Chlorogenic acid improves NAFLD by regulating gut microbiota and GLP-1. Front Pharmacol (2021) 12:693048. doi: 10.3389/fphar.2021.693048
18. Yan Y, Zhou X, Guo K, Zhou F, Yang H. Chlorogenic acid protects against indomethacin-induced inflammation and mucosa damage by decreasing bacteroides-derived LPS. Front Immunol (2020) 11:1125. doi: 10.3389/fimmu.2020.01125
19. Zhou X, He L, Wu C, Zhang Y, Wu X, Yin Y. Serine alleviates oxidative stress via supporting glutathione synthesis and methionine cycle in mice. Mol Nutr Food Res (2017) 61(11):1700262. doi: 10.1002/mnfr.201700262
20. Zhou X, Liu Y, Xiong X, Chen J, Tang W, He L, et al. Intestinal accumulation of microbiota-produced succinate caused by loss of micrornas leads to diarrhea in weanling piglets. Gut Microbes (2022) 14(1):2091369. doi: 10.1080/19490976.2022.2091369
21. Zhou X, Liu Y, Zhang L, Kong X, Li F. Serine-to-Glycine ratios in low-protein diets regulate intramuscular fat by affecting lipid metabolism and myofiber type transition in the skeletal muscle of growing-finishing pigs. Anim Nutr (2021) 7(2):384–92. doi: 10.1016/j.aninu.2020.08.011
22. Chen L, Teng H, Cao H. Chlorogenic acid and caffeic acid from sonchus oleraceus Linn synergistically attenuate insulin resistance and modulate glucose uptake in Hepg2 cells. Food Chem Toxicol (2019) 127:182–7. doi: 10.1016/j.fct.2019.03.038
23. Zuñiga LY, Aceves-de la Mora MCA, González-Ortiz M, Ramos-Núñez JL, Martínez-Abundis E. Effect of chlorogenic acid administration on glycemic control, insulin secretion, and insulin sensitivity in patients with impaired glucose tolerance. J Med Food (2018) 21(5):469–73. doi: 10.1089/jmf.2017.0110
24. Chen L, Lin X, Fan X, Qian Y, Lv Q, Teng H. Sonchus oleraceus Linn extract enhanced glucose homeostasis through the AMPK/Akt/ GSK-3β signaling pathway in diabetic liver and Hepg2 cell culture. Food Chem Toxicol (2020) 136:111072. doi: 10.1016/j.fct.2019
25. Tajik N, Tajik M, Mack I, Enck P. The potential effects of chlorogenic acid, the main phenolic components in coffee, on health: A comprehensive review of the literature. Eur J Nutr (2017) 56(7):2215–44. doi: 10.1007/s00394-017-1379-1
26. Cejas JP, Rosa AS, Nazareno MA, Disalvo EA, Frias MA. Interaction of chlorogenic acid with model lipid membranes and its influence on antiradical activity. Biochim Biophys Acta Biomembr (2021) 1863(1):183484. doi: 10.1016/j.bbamem.2020.183484
27. Xu M, Yang L, Zhu Y, Liao M, Chu L, Li X, et al. Collaborative effects of chlorogenic acid and caffeine on lipid metabolism via the ampkalpha-Lxralpha/SREBP-1c pathway in high-fat diet-induced obese mice. Food Funct (2019) 10(11):7489–97. doi: 10.1039/c9fo00502a
28. Asrih M, Jornayvaz FR. Inflammation as a potential link between nonalcoholic fatty liver disease and insulin resistance. J Endocrinol (2013) 218(3):R25–36. doi: 10.1530/JOE-13-0201
29. Le YJ, He LY, Li S, Xiong CJ, Lu CH, Yang XY. Chlorogenic acid exerts antibacterial effects by affecting lipid metabolism and scavenging ROS in streptococcus pyogenes. FEMS Microbiol Lett (2022) 369(1):fnac061. doi: 10.1093/femsle/fnac061
30. Xu W, Luo T, Chai J, Jing P, Xiong L. Chlorogenic acid alleviates the inflammatory stress of LPS-induced BV2 cell via interacting with TLR4-mediated downstream pathway. Comput Math Methods Med (2022) 2022:6282167. doi: 10.1155/2022/6282167
31. Zamani-Garmsiri F, Ghasempour G, Aliabadi M, Hashemnia SMR, Emamgholipour S, Meshkani R. Combination of metformin and chlorogenic acid attenuates hepatic steatosis and inflammation in high-fat diet fed mice. IUBMB Life (2021) 73(1):252–63. doi: 10.1002/iub.2424
32. Budryn G, Zaczynska D, Zyzelewicz D, Grzelczyk J, Zdunczyk Z, Juskiewicz J. Influence of the form of administration of chlorogenic acids on oxidative stress induced by high fat diet in rats. Plant Foods Hum Nutr (2017) 72(2):184–91. doi: 10.1007/s11130-017-0608-3
33. Hermawati E, Arfian N, Mustofa M, Partadiredja G. Chlorogenic acid ameliorates memory loss and hippocampal cell death after transient global ischemia. Eur J Neurosci (2020) 51(2):651–69. doi: 10.1111/ejn.14556
34. Demidova TY, Lobanova KG, Oinotkinova OS. Gut microbiota is a factor of risk for obesity and type 2 diabetes. Ter Arkh (2020) 92(10):97–104. doi: 10.26442/00403660.2020.10.000778
35. Zhang C, Shao H, Li D, Xiao N, Tan Z. Role of tryptophan-metabolizing microbiota in mice diarrhea caused by folium sennae extracts. BMC Microbiol (2020) 20(1):185. doi: 10.1186/s12866-020-01864-x
36. Li X, Peng X, Guo K, Tan Z. Bacterial diversity in intestinal mucosa of mice fed with dendrobium officinale and high-fat diet. Biotech (2021) 11(1):22. doi: 10.1007/s13205-020-02558-x
37. Jiao A, Yu B, He J, Yu J, Zheng P, Luo Y, et al. Short chain fatty acids could prevent fat deposition in pigs via regulating related hormones and genes. Food Funct (2020) 11(2):1845–55. doi: 10.1039/c9fo02585e
38. Song J, Zhou N, Ma W, Gu X, Chen B, Zeng Y, et al. Modulation of gut microbiota by chlorogenic acid pretreatment on rats with adrenocorticotropic hormone induced depression-like behavior. Food Funct (2019) 10(5):2947–57. doi: 10.1039/c8fo02599a
39. Parkar SG, Trower TM, Stevenson DE. Fecal microbial metabolism of polyphenols and its effects on human gut microbiota. Anaerobe (2013) 23:12–9. doi: 10.1016/j.anaerobe.2013.07.009
40. He X, Zheng S, Sheng Y, Miao T, Xu J, Xu W, et al. Chlorogenic acid ameliorates obesity by preventing energy balance shift in high-fat diet induced obese mice. J Sci Food Agric (2021) 101(2):631–7. doi: 10.1002/jsfa.10675
41. Bambha K, Wilson LA, Unalp A, Loomba R, Neuschwander-Tetri BA, Brunt EM, et al. Coffee consumption in NAFLD patients with lower insulin resistance is associated with lower risk of severe fibrosis. Liver Int (2014) 34(8):1250–8. doi: 10.1111/liv.12379
42. Wijarnpreecha K, Thongprayoon C, Ungprasert P. Coffee consumption and risk of nonalcoholic fatty liver disease: A systematic review and meta-analysis. Eur J Gastroenterol Hepatol (2017) 29(2):e8–e12. doi: 10.1097/MEG.0000000000000776
43. Sewter R, Heaney S, Patterson A. Coffee consumption and the progression of NAFLD: A systematic review. Nutrients (2021) 13(7):2381. doi: 10.3390/nu13072381
44. Wu Y, Liu W, Li Q, Li Y, Yan Y, Huang F, et al. Dietary chlorogenic acid regulates gut microbiota, serum-free amino acids and colonic serotonin levels in growing pigs. Int J Food Sci Nutr (2018) 69(5):566–73. doi: 10.1080/09637486.2017.1394449
45. Bhandarkar NS, Brown L, Panchal SK. Chlorogenic acid attenuates high-carbohydrate, high-fat diet-induced cardiovascular, liver, and metabolic changes in rats. Nutr Res (2019) 62:78–88. doi: 10.1016/j.nutres.2018.11.002
46. Yu F, Han W, Zhan G, Li S, Jiang X, Wang L, et al. Abnormal gut microbiota composition contributes to the development of type 2 diabetes mellitus in Db/Db mice. Aging (Albany NY) (2019) 11(22):10454–67. doi: 10.18632/aging.102469
47. Goldstein EJ, Tyrrell KL, Citron DM. Lactobacillus species: Taxonomic complexity and controversial susceptibilities. Clin Infect Dis (2015) 60 Suppl 2:S98–107. doi: 10.1093/cid/civ072
48. He L, Zhou X, Liu Y, Zhou L, Li F. Fecal mir-142a-3p from dextran sulfate sodium-challenge recovered mice prevents colitis by promoting the growth of lactobacillus reuteri. Mol Ther (2022) 30(1):388–99. doi: 10.1016/j.ymthe.2021.08.02
49. Liu X, Mao B, Gu J, Wu J, Cui S, Wang G, et al. Blautia-a new functional genus with potential probiotic properties? Gut Microbes (2021) 13(1):1–21. doi: 10.1080/19490976.2021.1875796
50. Raman M, Ahmed I, Gillevet PM, Probert CS, Ratcliffe NM, Smith S, et al. Fecal microbiome and volatile organic compound metabolome in obese humans with nonalcoholic fatty liver disease. Clin Gastroenterol Hepatol (2013) 11(7):868–75.e1-3. doi: 10.1016/j.cgh.2013.02.015
Keywords: chlorogenic acid, hyperglycemia, hyperlipidemia, inflammation, oxidative stress
Citation: Yan Y, Li Q, Shen L, Guo K and Zhou X (2022) Chlorogenic acid improves glucose tolerance, lipid metabolism, inflammation and microbiota composition in diabetic db/db mice. Front. Endocrinol. 13:1042044. doi: 10.3389/fendo.2022.1042044
Received: 12 September 2022; Accepted: 31 October 2022;
Published: 17 November 2022.
Edited by:
Xinhua Shu, Glasgow Caledonian University, United KingdomReviewed by:
Haoqing Shao, Hunan University of Medicine, ChinaYuli Li, Hunan University of Chinese Medicine, China
Copyright © 2022 Yan, Li, Shen, Guo and Zhou. This is an open-access article distributed under the terms of the Creative Commons Attribution License (CC BY). The use, distribution or reproduction in other forums is permitted, provided the original author(s) and the copyright owner(s) are credited and that the original publication in this journal is cited, in accordance with accepted academic practice. No use, distribution or reproduction is permitted which does not comply with these terms.
*Correspondence: Xu Zhou, stu.hnucm.edu.cn