- 1Faculty of Medicine and Health, The University of Sydney, Sydney, NSW, Australia
- 2Cancer Genetics, Kolling Institute of Medical Research, Sydney, NSW, Australia
- 3Department of Endocrinology, Royal North Shore Hospital, Sydney, NSW, Australia
- 4Melanoma Institute Australia, The University of Sydney, Sydney, NSW, Australia
- 5Department of Medical Oncology, Royal North Shore Hospital, Sydney, NSW, Australia
- 6Department of Medical Oncology, Mater Hospital, Sydney, NSW, Australia
Immune checkpoints are small molecules present on the cell surface of T-lymphocytes. They maintain self-tolerance and regulate the amplitude and duration of T-cell responses. Antagonism of immune checkpoints with monoclonal antibodies (immune checkpoint inhibitors) is a rapidly evolving field of anti-cancer immunotherapy and has become standard of care in management of many cancer subtypes. Immune checkpoint inhibition is an effective cancer treatment but can precipitate immune related adverse events (irAEs). Thyroid dysfunction is the most common endocrine irAE and can occur in up to 40% of treated patients. Both thyrotoxicosis and hypothyroidism occur. The clinical presentation and demographic associations of thyrotoxicosis compared to hypothyroidism suggest unique entities with different etiologies. Thyroid irAEs, particularly overt thyrotoxicosis, are associated with increased immune toxicity in other organ systems, but also with longer progression-free and overall survival. Polygenic risk scores using susceptibility loci associated with autoimmune thyroiditis predict development of checkpoint inhibitor associated irAEs, suggesting potentially shared mechanisms underpinning their development. Our review will provide an up-to-date summary of knowledge in the field of thyroid irAEs. Major focus will be directed toward pathogenesis (including genetic factors shared with autoimmune thyroid disease), demographic associations, clinical presentation and course, treatment, and the relationship with cancer outcomes.
Introduction
Immune checkpoint inhibitors (ICIs) are a rapidly expanding class of medications. At present, over 2000 clinical trials have been completed or are in progress to evaluate more than 30 different ICIs (1). The main immune checkpoint targets are cytotoxic T-lymphocyte antigen-4 (CTLA-4) and programmed cell death protein-1 (PD-1) or its ligand programmed cell death ligand-1 (PD-L1). The introduction of ICIs into cancer treatment algorithms has been transformative and durable responses are now possible in patients with previously limited therapeutic options. However, immune activation following ICI-treatment is associated with off target effects known as immune related adverse events (irAEs) (2). Any organ system may be affected and thyroid dysfunction is one of the most common ICI-related toxicities (3).
Immune Checkpoint Physiology
Immune checkpoints are small molecules present on the cell surface of T-lymphocytes (4). Normally, T-cell activation induces immune checkpoint expression to limit the amplitude and duration of immune responses (4). Immune checkpoints are pivotal to regulate the immune response and prevent inappropriate immune activation, such as occurs in autoimmune disease (4). However, in some situations, immune checkpoints can be counterproductive, such as malignant tumors which activate immune checkpoints to evade immune mediated tumor lysis (3). Monoclonal antibodies antagonizing CTLA-4, PD-1, and other immune checkpoints have been developed to counteract such tumor evasion strategies and stimulate anti-tumor immune responses (4). These agents convey significant therapeutic benefit but are associated with wide ranging irAEs (2–4).
Immune Related Adverse Events
The etiology of irAEs is yet to be fully elucidated. Potential mechanisms include activation of polyclonal T-cell populations, loss of regulatory T-cell function, and expansion/activation of self-reactive, antigen-specific T-cells (5). Irrespective of mechanism, ICI-associated irAEs are common, affecting up to 76% of treated patients (3). Any organ system can be affected, although there are distinct pharmacological class-specific differences between ICIs targeting CTLA-4 and those targeting PD-1 (3). Endocrine organs are commonly affected, with thyroid dysfunction, hypophysitis, insulin deficient diabetes, primary adrenal insufficiency, and hypoparathyroidism all reported following ICI-treatment (2, 6). In this review we will highlight the key features of thyroid irAEs and how our understanding of thyroid irAEs has informed our understanding of autoimmune thyroid disease more generally.
Thyroid Immune Related Adverse Events
Epidemiology
Thyroid irAEs are the most common endocrine toxicity related to ICI-treatment (6). From clinical trial data, over 10% of patients treated with ICIs develop a thyroid irAE (7). Higher rates are observed following treatment with PD-1 inhibitors relative to CTLA-4 inhibitors, with the highest rates following combined anti-PD-1 and anti-CTLA-4 treatment (7). Observational studies typically report higher rates of thyroid irAEs, usually due to inclusion of subclinical thyroid dysfunction that may be overlooked in clinical trials adverse events reporting (8–11). In the two largest observational studies of thyroid irAEs to date, 42-53% experienced an ICI-associated thyroid irAE (Table 1) (11, 12). Thyroid dysfunction was most common following combined anti-PD-1 and anti-CTLA-4 treatment (56%) and less frequent following anti-PD-1 (38%) and anti-CTLA-4 (25%) monotherapy (11).
Clinical Manifestations
Thyroid irAEs are typically identified incidentally during routine monitoring of thyroid function as part of ICI-treatment protocols (7). Most thyroid irAEs present as a painless thyroiditis with transient thyrotoxicosis (6, 7, 11, 13). In patients with more severe (ie. biochemically overt) thyrotoxicosis, a hypothyroid phase often follows the initial thyrotoxicosis and over 40% of these patients will develop permanent hypothyroidism requiring thyroid hormone replacement (11). A smaller number of patients can present with primary hypothyroidism without a preceding thyrotoxic phase.
Onset of thyrotoxicosis usually occurs within 3 months of first ICI-exposure (6, 7). Overt and subclinical thyrotoxicosis have phenotypic differences in their presentation and disease associations. Onset of overt thyrotoxicosis typically occurs earlier and persists longer than subclinical cases (11). Overt thyrotoxicosis has also been associated with higher baseline levels of anti-thyroid antibodies which increase during ICI-inhibitor treatment (14). Treatment related changes in antibody titer have not been observed in other subtypes of thyroid irAE (14). Patients who develop overt thyrotoxicosis are more likely to develop severe irAEs and to experience two or more irAEs in extra-thyroidal organ systems (11).
Isolated hypothyroidism without a preceding thyrotoxic phase can occur. Most cases of isolated hypothyroidism are biochemically subclinical and may be related to non-ICI factors such as non-thyroidal illness syndrome. Subclinical hypothyroidism without a preceding thyrotoxic phase typically normalizes spontaneously without need for thyroid hormone replacement (11, 15). Overt hypothyroidism can have a late-onset months to years after starting ICI-treatment (7). In contrast with subclinical hypothyroidism, overt hypothyroidism is usually permanent and lifelong treatment with thyroxine is required in most cases (6, 7, 11).
Risk Factors and Disease Associations
Identification of reliable thyroid irAE risk factors has been elusive. To date, no reliable risk factors of thyroid irAEs have been identified, although clinical and biochemical associations have been documented in retrospective cohort studies (7). Female sex was associated with thyroid irAEs in the two largest studies to date (Table 1), although to a lesser degree than the roughly 8:1 female preponderance observed in autoimmune thyroid disease developing outside of the ICI-treatment setting (11, 12). Prevalence of baseline TPOAb and TgAb positivity is higher in patients who develop a thyroid irAE compared to those who do not (11, 16). Anti-thyroid antibody positivity is particularly associated with overt thyroid dysfunction (11, 16) and titers of TPOAb and TgAb can increase significantly during ICI-treatment in patients that develop overt thyrotoxicosis, which is not observed in patients with other subtypes of thyroid irAE (14). A complete list of demographic and biochemical factors that have been associated with development of thyroid irAEs are summarized in Table 2.
Pathophysiology
The etiology and biological drivers of irAEs remain elusive. It is possible that patients who experience irAEs may share a premorbid immunological state prior to ICI-initiation. A recent study using mass cytometry time of flight analysis identified higher levels of activated CD4+ memory T-cells and increased diversity in the T-cell receptor as pretreatment predictors of severe irAEs irrespective of the organ involved (20). These same factors also occurred with higher frequency in non-ICI treated patients with autoimmune disease relative to healthy controls, suggesting that irAEs may result from unmasking a latent or subclinical autoimmune process which predates ICI-initiation (20).
Traditionally, thyroid irAEs were thought to be a single entity spanning a spectrum of disease including isolated mild (asymptomatic or minimally symptomatic) thyrotoxicosis to biphasic thyroiditis to isolated overt and severe hypothyroidism. This indeed may be the case, although more likely thyroid irAEs arise via multiple mechanisms as evidenced by phenotypic differences in presentation, antithyroid antibody positivity rates, and differential association with cancer survival outcomes (11).
Genetic factors associated with lifetime risk of autoimmune thyroid disease have been implicated in the risk of developing thyroid irAEs during ICI-treatment (21, 22). Key loci have been implicated from genes regulating the immune response such as CD69 (T-cell activation), LPP (B-cell maturation), CTLA-4 (T-cell priming) and PTPN22 (T-cell and B-cell receptor signaling). When these genes and others are combined into a polygenic risk score they can identify subgroups of patients at >6-fold increased risk of thyroid irAEs and identify patients at a lower risk of cancer death (21).
The PD-1/PD-L1 axis is significantly implicated in autoimmune thyroid disease (23). Patients with autoimmune thyroiditis and Graves’ disease experience a moderate increase in circulating PD-1 positive T-cells and marked increase in intrathyroidal PD-1 positive T-cells (23). Thyroid follicular cells also show high levels of PD-L1 expression, whereas multinodular goiter (non-autoimmune thyroid disease) has negligible basal expression of PD-L1 (23). The presence of high PD-L1 expression in autoimmune thyroid disease may explain why most cases are slowly progressive, as the immune stimulating effects of intrathyroidal PD-1 positive T-cells are kept in check. PD-1 positive lymphocytes and PD-L1 expression are similarly increased following ICI-treatment. However, different to autoimmune thyroid disease, blockade of the PD-1/PD-L1 axis blunts the protection against progressive immune mediated destruction via and therefore thyroid dysfunction is more rapidly progressive following ICI-treatment than in other autoimmune thyroid conditions (23, 24).
Management
Screening
Thyroid function should be measured at baseline and repeated at 6-weekly intervals following commencement of ICI-treatment in asymptomatic patients. Additional measures of TSH and FT4 can be undertaken for case detection in patients that develop signs or symptoms of thyroid dysfunction outside this window (25). Most cases of clinically significant thyroid dysfunction will occur within 6-months of ICI-commencement (7). Therefore, monitoring frequency can be relaxed after the 6-month mark although continued periodic monitoring (3-6 monthly) to detect late onset cases is recommended.
Diagnosis
As most cases of thyroid irAE are asymptomatic, diagnosis is most commonly via thyroid function monitoring in asymptomatic patients (7). Thyrotoxicosis is diagnosed in the setting of a low TSH with a normal or elevated FT4. Thyrotoxicosis often occurs as the initial phase of a biphasic thyroiditis and is followed by progression to hypothyroidism (elevated TSH, low FT4). More rarely, isolated hypothyroidism without a preceding thyrotoxic phase can occur. Unlike thyrotoxicosis, isolated hypothyroidism may present months to years after initiation of ICI-treatment. Special attention should be paid to the finding of an inappropriately low TSH in combination with a low or low-normal FT4. This pattern of results should alert the clinician to the possibility of hypophysitis, particularly in the setting of a CTLA-4 inhibitor. When hypophysitis (central hypothyroidism) is suspected, the remaining pituitary hormone axes should be interrogated urgently to exclude cortisol and other hormone deficiencies prior to initiation of thyroid hormone replacement.
Treatment
For asymptomatic or minimally symptomatic thyrotoxicosis, treatment is usually not required. Temporary use of beta blockers such as propranolol may be considered in symptomatic patients. ICI-treatment can be continued, and most cases will resolve spontaneously within days to weeks. Thyrotoxicosis lasting longer than 6-weeks or with additional features (goiter, thyroid bruit, exophthalmos) should have TSH-receptor antibody level (TRAB) measured to exclude Graves’ disease (25). In severe or atypical presentations, imaging with a thyroid uptake scan can also be performed to differentiate between ICI-mediated thyroiditis and other etiologies of thyrotoxicosis. Thyrotoxicosis with marked symptoms or life-threatening complications is rare and necessitates suspension of ICI-treatment to allow for prompt investigation and specialist led treatment (25). Following identification of thyrotoxicosis, TSH and FT4 should be monitored every 2-3 weeks until recovery of normal thyroid function, as many patients will experience a biphasic illness which progresses to hypothyroidism. Regular monitoring allows early detection of hypothyroidism, which does not recover in the majority of cases (11). In the setting of overt hypothyroidism, treatment with thyroxine can be initiated, particularly when the TSH is >10 mIU/L, as recovery is highly unlikely in this setting (11). Alternatively, TSH and FT4 can be repeated after 4-6 weeks and if hypothyroidism persists, treatment can be initiated at that point (25). Thyroxine doses are typically higher for patients with ICI-mediated hypothyroidism than for Hashimoto’s thyroiditis, suggesting that gland destruction is complete in most cases of ICI-mediated thyroiditis whereas it is slowly progressive with some residual production of endogenous thyroid hormone in patients with Hashimoto’s thyroiditis (26). A simplified algorithm for the classification and management of thyroid irAEs is presented in Figure 1.
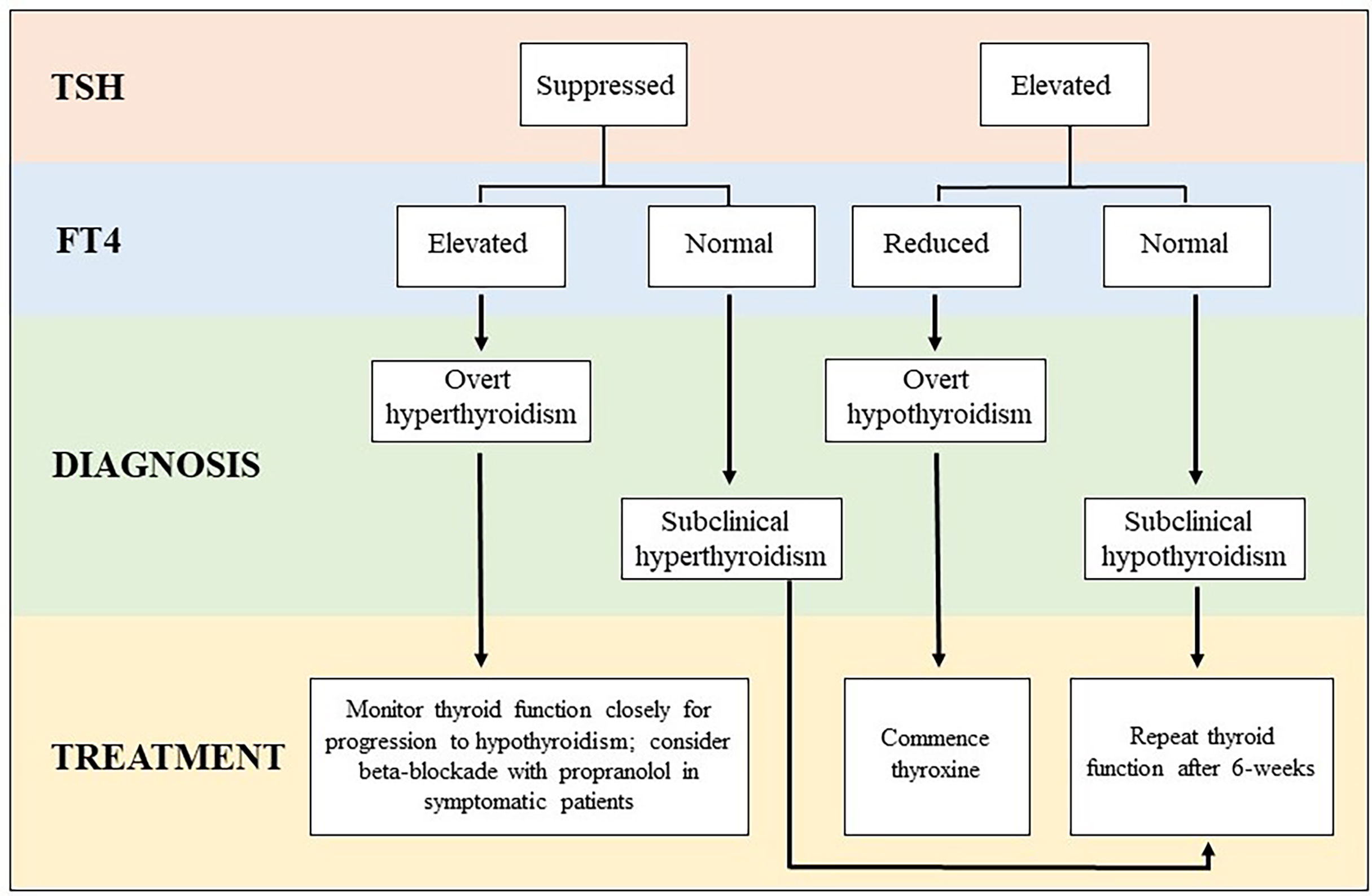
Figure 1 Algorithm for classification and management of thyroid irAE subtypes. *When overt thyrotoxicosis is prolonged or severe, additional investigation with TSH receptor antibody (TRAB) and a thyroid uptake scan should be considered to exclude other etiologies of thyrotoxicosis (ie. Graves’ disease, toxic adenoma, etc).
Special Situations
Pre-existing Hypothyroidism
Patients with established hypothyroidism may experience transient effects on thyroid function following ICI-treatment (7). Compensated hypothyroidism can be exacerbated requiring initiation or increased doses of thyroxine. More rarely, patients with hypothyroidism may paradoxically experience a transient period of thyrotoxicosis requiring temporary suspension of thyroid hormone (27).
Graves’ Disease and Thyroid Eye Disease
Graves’ disease (GD) has been reported following ICI-treatment (6). Whether it occurs de novo related to ICI-treatment, is unmasked by ICI-treatment, or is a coincidental occurrence remains unknown. Similarly, thyroid eye disease (TED) has been reported (28). Reactivation or progression of GD and TED is possible following initiation of ICI-treatment and in patients with known GD or TED, close consultation with an experienced endocrinologist and ophthalmologist is important for early identification and treatment.
Prognostic Implications
Observational studies repeatedly document an association between irAEs and improvement in cancer outcomes (29, 30). Owing to the frequency of thyroid irAEs, they are among the most well studied and strongly associated with improvement in progression free survival (PFS) and overall survival (OS) (31–33). Reported benefits are large with robust hazard ratios that are maintained following correction for immortal time bias (31, 34). However, emerging data suggest thyroid irAE subtypes are not equivalent in their association with cancer outcomes. When thyroid irAEs are classified by severity, biochemically overt thyroid dysfunction is more strongly associated with improved PFS and OS than subclinical thyroid dysfunction (11, 35, 36). Our previous work suggests this may be limited to overt thyrotoxicosis, as no survival benefit was observed in patients with overt hypothyroidism without a preceding thyrotoxic phase (11). Permanent thyroid dysfunction requiring initiation of thyroid hormone replacement has also been associated with improved cancer outcomes (12, 37). However, an ultimate requirement for thyroxine may have been a surrogate marker for a preceding phase of overt thyrotoxicosis rather than a beneficial prognostic factor in of itself. The significance of antithyroid antibodies on cancer outcomes following ICI-treatment is unknown and current data has conflicting results (35, 38). Given the high prevalence of anti-thyroid antibodies in patients who develop overt thyrotoxicosis, an association is likely and prospective study into potential utility as a predictive biomarker is warranted (14).
Interestingly, abnormal TSH level (elevated or low) prior to initiation of ICI-treatment has been associated with inferior cancer outcomes irrespective of thyroid function changes post-ICI initiation in one study (12). However, these results may have been confounded as significantly more patients with abnormal baseline TSH were receiving tyrosine kinase inhibitor treatment for metastatic renal cell cancer, which is known to significantly affect thyroid function (12). Abnormal TSH prior to ICI-treatment may also be a function of sicker patients displaying the non-thyroidal illness syndrome. Therefore, at present it is not known whether baseline thyroid function is truly associated with worse outcomes or if it simply reflects patients with a more aggressive cancer or a physiologic response to chronic illness in a less well patient at commencement of ICI-treatment.
Future Directions
Further research is required to better inform our understanding of thyroid irAEs and their relationship to thyroid autoimmune conditions occurring outside the cancer immunotherapy setting. Enhanced characterization of the immune phenotype and T-cell subsets driving thyroid irAEs may allow for identification of patients at risk of thyroid (and other) irAEs and inform future research to uncouple the efficacy of ICIs from the risk of developing treatment related irAEs. Understanding the effects of immune checkpoint inhibition on the thyroid gland could also inform use of these agents for treatment of primary thyroid malignancies and prevention of autoimmune thyroid disease.
Conclusions
Thyroid irAEs are a frequent complication of anti-PD-1 based ICI-treatment. Recent work has begun to unravel the molecular mechanisms underpinning development and their relationship to other autoimmune thyroid conditions. Regular monitoring of thyroid function during ICI-treatment is required, as although most cases of thyroid irAEs are asymptomatic and manageable, severe presentations with thyroid storm or myxedema can occur. Development of thyroid irAEs is associated with improved PFS and OS, especially overt thyrotoxicosis which may be a surrogate marker of patients experiencing a more robust immune response to ICI-treatment. When hypothyroidism occurs, it is usually permanent and treatment with thyroid hormone replacement will be required. Familiarity with thyroid irAEs among health professionals is important to facilitate efficient diagnosis and appropriate treatment of this increasingly common thyroid disease.
Author Contributions
All authors listed have made a substantial, direct, and intellectual contribution to the work. All authors approved it for publication.
Conflict of Interest
The authors declare that the research was conducted in the absence of any commercial or financial relationships that could be construed as a potential conflict of interest.
Publisher’s Note
All claims expressed in this article are solely those of the authors and do not necessarily represent those of their affiliated organizations, or those of the publisher, the editors and the reviewers. Any product that may be evaluated in this article, or claim that may be made by its manufacturer, is not guaranteed or endorsed by the publisher.
Acknowledgments
CM gratefully acknowledges support from the National Health & Medical Research Council postgraduate scholarship. AM gratefully acknowledges support from Nicholas and Helen Moore and Melanoma Institute Australia and a National Health & Medical Research Council investigator grant.
References
1. Beaver JA, Pazdur R. The Wild West of Checkpoint Inhibitor Development. N Engl J Med (2021) 386:1297–1301. doi: 10.1056/NEJMp2116863
2. Postow MA, Sidlow R, Hellmann MD. Immune-Related Adverse Events Associated With Immune Checkpoint Blockade. New Engl J Med (2018) 378(2):158–68. doi: 10.1056/NEJMra1703481
3. Ramos-Casals M, Brahmer JR, Callahan MK, Flores-Chavez A, Keegan N, Khamashta MA, et al. Immune-Related Adverse Events of Checkpoint Inhibitors. Nat Rev Dis Primers (2020) 6(1):1–21. doi: 10.1038/s41572-020-0160-6
4. Pardoll DM. The Blockade of Immune Checkpoints in Cancer Immunotherapy. Nat Rev Cancer (2012) 12(4):252–64. doi: 10.1038/nrc3239
5. Korman AJ, Garrett-Thomson SC, Lonberg N. The Foundations of Immune Checkpoint Blockade and the Ipilimumab Approval Decennial. Nat Rev Drug Discov (2021), 1–20. doi: 10.1038/s41573-021-00345-8
6. Chang L-S, Barroso-Sousa R, Tolaney SM, Hodi FS, Kaiser UB, Min L. Endocrine Toxicity of Cancer Immunotherapy Targeting Immune Checkpoints. Endocr Rev (2019) 40(1):17–65. doi: 10.1210/er.2018-00006
7. Muir CA, Menzies AM, Clifton-Bligh R, Tsang VH. Thyroid Toxicity Following Immune Checkpoint Inhibitor Treatment in Advanced Cancer. Thyroid (2020) 30(10):1458–69. doi: 10.1089/thy.2020.0032
8. Morganstein DL, Lai Z, Spain L, Diem S, Levine D, Mace C, et al. Thyroid Abnormalities Following the Use of Cytotoxic T-Lymphocyte Antigen-4 and Programmed Death Receptor Protein-1 Inhibitors in the Treatment of Melanoma. Clin Endocrinol (2017) 86(4):614–20. doi: 10.1111/cen.13297
9. Scott ES, Long GV, Guminski A, Clifton-Bligh RJ, Menzies AM, Tsang VH. The Spectrum, Incidence, Kinetics and Management of Endocrinopathies With Immune Checkpoint Inhibitors for Metastatic Melanoma. Eur J Endocrinol (2018) 178(2):173–80. doi: 10.1530/EJE-17-0810
10. Pollack RM, Kagan M, Lotem M, Dresner-Pollak R. Baseline Tsh Level Is Associated With Risk of Anti-Pd-1-Induced Thyroid Dysfunction. Endocr Pract (2019) 25(8):824–9. doi: 10.4158/EP-2018-0472
11. Muir CA, Clifton-Bligh RJ, Long GV, Scolyer RA, Lo S, Carlino MS, et al. Thyroid Immune-Related Adverse Events Following Immune Checkpoint Inhibitor Treatment. J Clin Endocrinol Metab (2021) 106(9):e3704–e13. doi: 10.1210/clinem/dgab263
12. von Itzstein MS, Gonugunta AS, Wang Y, Sheffield T, Lu R, Ali S, et al. Divergent Prognostic Effects of Pre-Existing and Treatment-Emergent Thyroid Dysfunction in Patients Treated With Immune Checkpoint Inhibitors. Cancer Immunol Immunother (2022), 1–13. doi: 10.1007/s00262-022-03151-2
13. Iyer PC, Cabanillas ME, Waguespack SG, Hu MI, Thosani S, Lavis VR, et al. Immune-Related Thyroiditis With Immune Checkpoint Inhibitors. Thyroid (2018) 28(10):1243–51. doi: 10.1089/thy.2018.0116
14. Muir CA, Wood CCG, Clifton-Bligh RJ, Long GV, Scolyer RA, Carlino MS, et al. Association of Anti-Thyroid Antibodies in Checkpoint Inhibitor Associated Thyroid Immune Related Adverse Events. J Clin Endocrinol Metab (2022) 107(5):e1843–9. doi: 10.1210/clinem/dgac059
15. Peiro I, Palmero R, Iglesias P, Diez JJ, Simo-Servat A, Marin JA, et al. Thyroid Dysfunction Induced by Nivolumab: Searching for Disease Patterns and Outcomes. Endocrine (2019) 64(3):605–13. doi: 10.1007/s12020-019-01871-7
16. Yoon JH, Hong AR, Kim HK, Kang H-C. Characteristics of Immune-Related Thyroid Adverse Events in Patients Treated With PD-1/PD-L1 Inhibitors. Endocrinol Metab (2021) 36(2):413. doi: 10.3803/EnM.2020.906
17. Pollack R, Ashash A, Cahn A, Rottenberg Y, Stern H, Dresner-Pollak R. Immune Checkpoint Inhibitor-Induced Thyroid Dysfunction Is Associated With Higher Body Mass Index. J Clin Endocrinol Metab (2020) 105(10):e3620–7. doi: 10.1210/clinem/dgaa458
18. Kimbara S, Fujiwara Y, Iwama S, Ohashi K, Kuchiba A, Arima H, et al. Association of Antithyroglobulin Antibodies With the Development of Thyroid Dysfunction Induced by Nivolumab. Cancer Sci (2018) 109(11):3583–90. doi: 10.1111/cas.13800
19. Kurimoto C, Inaba H, Ariyasu H, Iwakura H, Ueda Y, Uraki S, et al. Predictive and Sensitive Biomarkers for Thyroid Dysfunctions During Treatment With Immune-Checkpoint Inhibitors. Cancer Sci (2020) 111(5):1468. doi: 10.1111/cas.14363
20. Lozano AX, Chaudhuri AA, Nene A, Bacchiocchi A, Earland N, Vesely MD, et al. T Cell Characteristics Associated With Toxicity to Immune Checkpoint Blockade in Patients With Melanoma. Nat Med (2022) 28:1–10. doi: 10.1038/s41591-021-01623-z
21. Khan Z, Hammer C, Carroll J, Di Nucci F, Acosta SL, Maiya V, et al. Genetic Variation Associated With Thyroid Autoimmunity Shapes the Systemic Immune Response to PD-1 Checkpoint Blockade. Nat Commun (2021) 12(1):1–12. doi: 10.1038/s41467-021-23661-4
22. Luo J, Martucci VL, Quandt Z, Groha S, Murray MH, Lovely CM, et al. Immunotherapy-Mediated Thyroid Dysfunction: Genetic Risk and Impact on Outcomes With PD-1 Blockade in Non–Small Cell Lung Cancer. Clin Cancer Res (2021) 27(18):5131–40. doi: 10.1158/1078-0432.CCR-21-0921
23. lvarez-Sierra A D, Marin-Sanchez A, Ruiz-Blazquez P, Gil CJ, Iglesias-Felip C, Gonzalez O, et al. Analysis of the PD-1/PD-L1 Axis in Human Autoimmune Thyroid Disease: Insights Into Pathogenesis and Clues to Immunotherapy Associated Thyroid Autoimmunity. J Autoimmun (2019) 103:102285. doi: 10.1016/j.jaut.2019.05.013
24. Kotwal A, Gustafson MP, Bornschlegl S, Kottschade L, Delivanis DA, Dietz AB, et al. Immune Checkpoint Inhibitor-Induced Thyroiditis Is Associated With Increased Intrathyroidal T Lymphocyte Subpopulations. Thyroid (2020) 30(10):1440–50. doi: 10.1089/thy.2020.0075
25. Schneider BJ, Naidoo J, Santomasso BD, Lacchetti C, Adkins S, Anadkat M, et al. Management of Immune-Related Adverse Events in Patients Treated With Immune Checkpoint Inhibitor Therapy: ASCO Guideline Update. J Clin Oncol (2021) 39(36):4073–126. doi: 10.1200/JCO.21.01440
26. Mosaferi T, Tsai K, Sovich S, Wilhalme H, Kathuria-Prakash N, Praw SS, et al. Optimal Thyroid Hormone Replacement Dose in Immune Checkpoint Inhibitor-Associated Hypothyroidism Is Distinct From Hashimoto’s Thyroiditis. Thyroid (2022). doi: 10.1089/thy.2021.0685
27. Patel NS, Oury A, Daniels GA, Bazhenova L, Patel SP. Incidence of Thyroid Function Test Abnormalities in Patients Receiving Immune-Checkpoint Inhibitors for Cancer Treatment. Oncologist (2018) 23(10):1236–41. doi: 10.1634/theoncologist.2017-0375
28. Min L, Vaidya A, Becker C. Thyroid Autoimmunity and Ophthalmopathy Related to Melanoma Biological Therapy. Eur J Endocrinol (2011) 164(2):303–7. doi: 10.1530/EJE-10-0833
29. Haratani K, Hayashi H, Chiba Y, Kudo K, Yonesaka K, Kato R, et al. Association of Immune-Related Adverse Events With Nivolumab Efficacy in Non–Small-Cell Lung Cancer. JAMA Oncol (2018) 4(3):374–8. doi: 10.1001/jamaoncol.2017.2925
30. Fan Y, Xie W, Huang H, Wang Y, Li G, Geng Y, et al. Association of Immune Related Adverse Events With Efficacy of Immune Checkpoint Inhibitors and Overall Survival in Cancers: A Systemic Review and Meta-Analysis. Front Oncol (2021) 11:1081. doi: 10.3389/fonc.2021.633032
31. Cheung Y-MM, Wang W, McGregor B, Hamnvik O-PR. Associations Between Immune-Related Thyroid Dysfunction and Efficacy of Immune Checkpoint Inhibitors: A Systematic Review and Meta-Analysis. Cancer Immunol Immunother (2022) 1–18. doi: 10.1007/s00262-021-03128-7
32. Kotwal A, Ryder M. Survival Benefit of Endocrine Dysfunction Following Immune Checkpoint Inhibitors for Nonthyroidal Cancers. Curr Opin Endocrinol Diabetes Obes (2021) 28(5):517–24. doi: 10.1097/MED.0000000000000664
33. Ferreira JL, Costa C, Marques B, Castro S, Victor M, Oliveira J, et al. Improved Survival in Patients With Thyroid Function Test Abnormalities Secondary to Immune-Checkpoint Inhibitors. Cancer Immunol Immunother (2021) 70(2):299–309. doi: 10.1007/s00262-020-02664-y
34. Kotwal A, Kottschade L, Ryder M. PD-L1 Inhibitor-Induced Thyroiditis is Associated With Better Overall Survival in Cancer Patients. Thyroid (2020) 30(2):177–84. doi: 10.1089/thy.2019.0250
35. Basak EA, van derMeer JWM, Hurkmans DP, Schreurs MWJ, Oomen-de Hoop E, van derVeldt AAM, et al. Overt Thyroid Dysfunction and Anti-Thyroid Antibodies Predict Response to Anti-PD-1 Immunotherapy in Cancer Patients. Thyroid (2020) 30(7):966–73. doi: 10.1089/thy.2019.0726
36. Yamauchi I, Yasoda A, Matsumoto S, Sakamori Y, Kim YH, Nomura M, et al. Incidence, Features, and Prognosis of Immune-Related Adverse Events Involving the Thyroid Gland Induced by Nivolumab. PloS One (2019) 14(5):e0216954. doi: 10.1371/journal.pone.0216954
37. Inaba H, Ariyasu H, Iwakura H, Kurimoto C, Takeshima K, Morita S, et al. Distinct Clinical Features and Prognosis Between Persistent and Temporary Thyroid Dysfunctions by Immune-Checkpoint Inhibitors. Endocr J (2020), EJ20–0371. doi: 10.1507/endocrj.EJ20-0371
Keywords: immune related adverse events, checkpoint inhibitor, thyrotoxicosis, hypothyroidism, thyroid
Citation: Muir CA, Tsang VHM, Menzies AM and Clifton-Bligh RJ (2022) Immune Related Adverse Events of the Thyroid – A Narrative Review. Front. Endocrinol. 13:886930. doi: 10.3389/fendo.2022.886930
Received: 01 March 2022; Accepted: 25 April 2022;
Published: 26 May 2022.
Edited by:
Dario Giuffrida, Mediterranean Institute of Oncology (IOM), ItalyReviewed by:
Agnese Barnabei, Azienda Sanitaria Locale Roma 1, ItalyMelissa G Lechner, University of California, Los Angeles, United States
Copyright © 2022 Muir, Tsang, Menzies and Clifton-Bligh. This is an open-access article distributed under the terms of the Creative Commons Attribution License (CC BY). The use, distribution or reproduction in other forums is permitted, provided the original author(s) and the copyright owner(s) are credited and that the original publication in this journal is cited, in accordance with accepted academic practice. No use, distribution or reproduction is permitted which does not comply with these terms.
*Correspondence: Christopher A. Muir, cmui7357@uni.sydney.edu.au