Surviving Volcanic Environments—Interaction of Soil Mineral Content and Plant Element Composition
- 1Institute for Plant Biology, Technische Universität Braunschweig, Braunschweig, Germany
- 2Professur für Baumphysiologie, Institut für Forstwissenschaften, Albert-Ludwigs-Universität Freiburg, Freiburg, Germany
- 3Department of Zoology, King Saud University, Riyadh, Saudi Arabia
- 4Institute for Crop and Soil Science, Federal Research Centre for Cultivated Plants (JKI), Braunschweig, Germany
Different plant species were investigated from two Aeolian Islands located in close vicinity, one with fumarolic activity (Vulcano) and one without (Lipari). On Vulcano, elevated concentrations of SO2/H2S determined in ambient air indicated the need of plants to adapt to harmful sulphur concentrations by detoxification strategies. The current study was focused on evaluating the element composition of plant leaves in relation to soil mineral contents. The soil of Volcano was characterised by a significantly lower pH on all three sampling sites as well as very high amounts of sulphur and plant available sulphate due to volcanic activities, compared to Lipari. By contrast, a general difference in the composition of trace elements in the soil was not observed between the islands, apart from arsenic, which was increased at all three sampling sites on Vulcano. Element accumulation in the leaves differed between the two islands. The tested species showed a significant higher accumulation of numerous elements (Al, B, Fe, K, Mg, Mn, Ni, and Zn) on Vulcano compared to Lipari, while excluding Ca and Mo. These differences in element accumulation in the leaves between the islands may be caused by the lower soil pH on Vulcano. Extreme sulphur accumulation was found for all tested species on Vulcano, but was lower in woody species with higher dry matter content compared to herbaceous species with lower dry matter content. This caused a significantly negative correlation between plant sulphur and dry matter content. From these results, it is concluded that species with higher dry matter contents possess a more effective protection against extreme sulphur accumulation. Strategies to cope with other potentially toxic elements in the soil ranged from exclusion to hyper-accumulation. Hierarchical cluster analyses of the leaf element content revealed a clear separation between two groups: First, herbaceous perennial plants as strong accumulators; and second, woody perennial plants such as shrubs or trees as less strong accumulators, with the primordial species Fumaria capreolata representing an outside group.
Introduction
Plants growing in vicinity of active volcanoes are forced to cope with extreme abiotic stress caused by fumarolic gases that can affect plant growth and development. The predominant discharge constituents of fumaroles are water vapour and CO2 as well as harmful concentrations of H2S and SO2 (Giggenbach et al., 2001). H2S and SO2 enter plants via the stomata and are converted to toxic bisulphite and sulphite in the aqueous environment of the apoplast which need to be detoxified (Brychkova et al., 2007; Hamisch et al., 2012; Randewig et al., 2012). The high toxicity of sulphite is based on its nucleophilic ability to break disulphite bridges resulting in degradation of proteins (Menzel et al., 1986; Heber and Hüve, 1998). In a previously published study, three different evolutionary strategies to detoxify sulphite were identified; these strategies enable plants to cope with environmental stress caused by fumarolic gases high in SO2 and H2S (Baillie et al., 2016). As main strategy, the first group used sulphite oxidation via plant sulphite oxidase (pSO). This molybdoenzyme was highly up-regulated in plants exposed to fumarolic gases. The second group also showed up-regulated pSO activity, but additionally used reductive processes to detoxify excess sulphite. In addition, the third group was able to oxidize and to reduce sulphite, but in these species no up-regulation of pSO was observed. Fumaria capreolata, a phylogenetically more primordial species, showed no detectable reaction at all to fumarolic gases (Baillie et al., 2016). These findings underline the view that plant species have developed diverse strategies to detoxify excess sulphur rather than just a single pathway.
Plants growing close to volcanoes have to cope not only with high atmospheric contaminations above ground, but also potentially toxic compounds in the rhizosphere. Accordingly, plants have to adapt to the harmful environment near volcanoes in more than one aspect. Due to the continuous emission of sulphur-containing gases, important soil parameters such as the pH, nutrition composition and/or the soil microbial community can be affected. Previous studies showed that high atmospheric SO2 concentrations resulted in the formation of acid rain causing soil degradation and acidification (e.g., Stoddard et al., 1999; Kochergina et al., 2017). The same is true for local and continuous emissions of fumarolic gases. Additionally, volcanic gases contain potentially toxic elements such as Al, As, Cu, Hg, Mn, Ni, Pb, and Zn (Seaward and Richardson, 1989; Signorelli et al., 1998; Nagajyoti et al., 2010). Thus, the bioavailability of nutrients and toxic elements change in environments exposed to fumarolic gases (Kochergina et al., 2017).
To evaluate the effect of long-term volcanic activity on element composition of different plant species, leaf samples were collected on two Aeolian Islands: one set from Vulcano, which is characterized by fumarolic activity, and one set from the neighbouring island Lipari as a control, where no volcanic activity has been recorded recently. This set of leaf samples provided the possibility to investigate mechanisms of stress adaptation to an environment affected by active volcanism. The same set of leaf samples was used in a previous study to evaluate differences in stress adaptation to high SO2/H2S concentrations (Baillie et al., 2016). In the current investigation, we addressed the following questions: (1) Does the strong volcanic activity on Vulcano result in specific soil element composition? (2) Are there differences in plant mineral content in the leaves of the collected species due to the volcanic activity? (3) Do the tested species show additional adaptation mechanisms to potentially toxic elements in the soil besides sulphite detoxification strategies? (4) Is it possible to identify different strategies to cope with stress in the volcanic environment based on element composition?
Materials and Methods
Sample Collection
Plant and soil samples were collected on the two Aeolian Islands Lipari and Vulcano, which are located close to Sicily (Italy) in the Tyrrhenian Sea. Both islands are of volcanic origin. Vulcano is characterized by the volcano La Fossa (501 m high) with the active centre, the Gran Cratere, erupting last in 1888–1890 and since then continuously emitting fumaroles (Giggenbach et al., 2001). On Lipari, volcanic gases cannot be detected in ambient air. The islands are closely located to each other (distance of about 1 km, Figure 1A) and share the same climatic conditions [Vulcano: 634 mm yearly rainfall and 18.2°C mean temperature (https://de.climate-data.org/location/446833/); Lipari: 634 mm yearly rainfall and 18.3°C mean temperature (https://de.climate-data.org/location/13870/)]. Therefore, collecting plant samples of the same species on both islands was on the one hand a great challenge but provides the possibility to investigate mechanisms of stress adaptation to an environment affected by active volcanism. Leaves from a range of species were collected including herbaceous and woody plants. Only species that grew on both islands in reasonable amounts were used in this study. Plant samples were collected from the 2nd to the 4th of April in 2014. At this time, all plant species were in their main vegetative growth phase and herbaceous plants were already flowering. For comparative studies it is important to investigate plants in their main vegetative growth as differences in nutrient and trace element acquisition will be most pronounced under such conditions. The different species were harvested on three sites on Vulcano permanently fumigated with SO2/H2S, while on Lipari the same species were collected as controls in a greater area on five locations. Finally, eight plant species were chosen for the present investigation (Table 1), according to the strategies to detoxify harmful amounts of sulphur containing gases identified by Baillie et al. (2016). Leaf samples were collected in three replicates and were dried for 48 h at 65°C, before grinding to a fine powder.
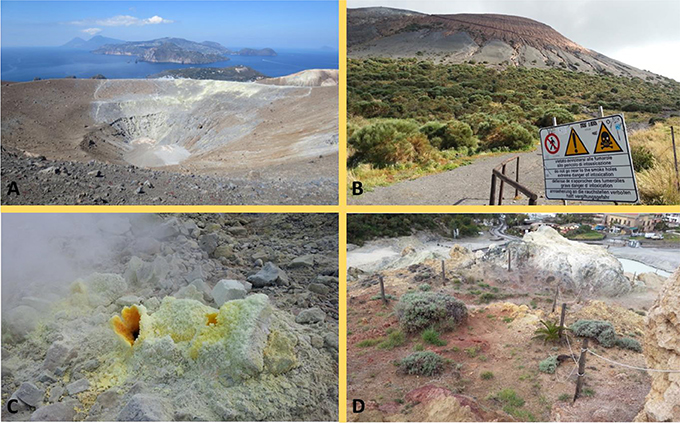
Figure 1. The Aeolian Island Vulcano. (A) Top of the Gran Cratere on Vulcano. In the background, the island Lipari with the respective control sampling sites is visible (distance ≈1 km). (B) Side view of the Gran Cratere with vegetation up to a hostile zone with critical concentrations of fumarolic gases and danger sign alerting to toxicity. (C) Fumaroles near the sampling sites with sulphur deposits. (D) Sampling site close to the fumarolic active “Dead Field” at the outer rim of Vulcano where plant samples on Vulcano were collected.
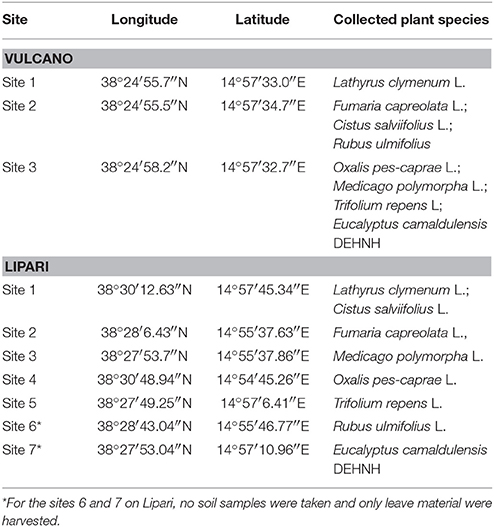
Table 1. Sampling site of soil and plant samples collected on the Aeolian Islands Vulcano and Lipari in April 2014.
At the locations of leaf sampling, also samples of the topsoil (0–10 cm) were collected in three replicates. Vulcano and Lipari are composed exclusively of volcanic rocks (Bargagli et al., 1991). The history and pedogenesis of the volcanic substrates on the Aeolian Islands is explained in detail by De Rosa et al. (2016). Most of the sampled soils were shallow soils with rock material close to the surface. The collected soils were mainly sandy loam or loamy sand in texture containing a great proportion of coarse soil particles (>2 mm). Site 3 on Vulcano, the so-called “Dead Field,” was a strongly disturbed soil where it was hardly possible to collect a sample. At the leaf sampling sites 6 and 7 on Lipari appropriate soil material could not be collected. As a deep-rooting tree species (Burgess et al., 2001), Eucalyptus camaldulensis takes up nutrients not only in the studied 0–10 cm top soil, but also from deeper soil layers. Therefore, a comparison between leaf and 0–10 cm top soil element contents did not appear to be appropriate. Rubus ulmifolius was collected on Lipari at a site with physically no chance to collect reasonable amounts of soil (stone wall). As a consequence, these two species (R. ulmifolius and E. camaldulensis) were omitted from the comparative analysis between the mineral composition of leaves and the soil.
Analysis of Soil Samples
Soil samples were air-dried, homogenized and sieved through a 2 mm sieve. The pH of soil samples was determined potentiometrically, according to DIN ISO 10390 after mixing 10 g of soil with 25 mL 0.01 M CaCl2 and an equilibration time of 60 min (DIN ISO 10390, 1997).
After determination of pH, the total contents of macro elements (C, Ca, K, Mg, N, P, S) as well as of trace elements (Al, As, B, Cd, Cr, Cu, Fe, Mn, Mo, Ni, Pb, Se, Zn) were measured in soil extracts. This measurement—total (acid soluble) macro elements and potentially toxic elements—was performed by ICP-OES (iCAP 6000, Thermo) and ICP-MS (Element XR, Thermo), respectively. For this purpose, 5 g of air-dried soil was extracted with 25 mL aqua regia (1 part HNO3 + 3 part HCl) according to VDLUFA (1991).
The total C and N content of the soil samples was determined by a C/N element analyser (Vario MAX CNS, Elementar, Germany). Soil sulphate content was determined according to Bloem et al. (2002) by ion chromatography (Methrom 761 Compact IC equipped with a Metrosep Anion Dual 2 column).
Analysis of Plant Samples
For the extraction of macro (Ca, K, Mg, P, S) and trace elements (Al, B, Cd, Cr, Cu, Fe, Mn, Mo, Ni, Pb, Se, Zn), 0.1 g oven dried plant material was digested with 2.4 mL HNO3 + 0.6 mL H2O2 in a microwave oven (MARS 6, CEM, Matthews, USA). Samples were heated within 5 min to 120°C at 600 Watt and kept for 2 min at 120°C. Subsequently, the temperature was increased within 5 min to 200°C and kept for 15 min at 200°C. Then the samples were cooled down for 30 min and 25 mL distilled water were added. The element concentrations were determined in the extracts by ICP-OES (iCAP 6000, Thermo) and ICP-MS (Element XR, Thermo), respectively.
Sulphate was extracted according to Novozamsky et al. (1986) and the measurement was performed by ion chromatography (Methrom 761 Compact IC equipped with a Metrosep Anion Dual 2 column).
Total C and N contents of leaf samples were determined as previously described (Simon et al., 2010; Hu et al., 2015). Aliquots of 2.0–2.5 mg of dried plant material were weighed into tin capsules (IVA Analysetechnik, Meerbusch, Germany) and analyzed with a C/N element analyzer (NA 2500, CE Instruments, Milan, Italy). Working standards were analysed after every 10th sample to rule out potential instrumental drift over time.
On Site SO2 and H2S Measurement
The volcanic gases present in ambient air of the sampling sides on the Island Vulcano and the ambient air on the Island Lipari were measured with an APSA-370 H2S/SO2-Immission Monitor (Horiba, Leichlingen, Germany). The range of gas-concentration are monitored over the time period of plant harvest. The instrument has a lower detection limit of 0.5 nL L−1.
Statistical Analysis
The data were statistically evaluated by analysis of variance (ANOVA) and by Tukey's test using the COSTAT software package. Significant differences were determined at p < 0.05.
Correlation of the collected data and clustering was performed using the statistical analysis software R (R Core Team, 2014) based on calculated ratios with values from Vulcano divided by values from Lipari. Further transformation of the generated values was performed to ensure equal distance distribution of both lower and higher values in comparison. A distance matrix was generated using the dist function and hierarchical cluster analysis of this distance matrix was performed using the hclust function of the R base package. The parameters taken into account for clustering were Al, B, Ca, K, Mg, Mn, Mo, and Zn. A clustering including the macro elements (C, N, P, and S) did not show a conclusive picture and was not taken into account.
Results and Discussion
When visiting the islands Vulcano and Lipari situated in the Tyrrhenian Sea north of Sicily (Italy) the difference in volcanic activity is obvious. On Lipari, neither fumaroles nor any smell of S-containing gases is noticeable. On Vulcano, on the other hand, there is an impressive cone with the Gran Cratere on top (Figures 1A,B) where fumaroles are releasing H2S and SO2 continuously and only pioneer plants can be found (Figure 1C). The sampling sites on Vulcano are closely located to the so-called “Dead Field” (Figure 1D) another area on the island where fumaroles are emitting S-containing gas near a mud hole.
The gases of fumarolic origin on Vulcano are strongly fluctuating depending on volcanic activities, weather conditions including wind speed and direction as well as humidity. On average the gas concentrations at the sampling sites on Vulcano for the given time period in 2014 varied between 0.2 and 0.8 μL L−1 H2S with a maximum of 2 μL L−1 H2S. The average SO2 concentrations measured ranged from 6 to 8 nL L−1, with a maximum of 22 nL L−1 SO2 during the sampling period. These values are much lower than the SO2 concentration of 0.5 μL L−1 measured 20 years before by Graziani et al. (1997) which is even harmful for animals and humans and reflects the high temporal variability of the release of S containing fumaroles. On the control Island Lipari, however, the concentrations of volcanic gases were below the detection limit (Baillie et al., 2016).
Differences in Soil Composition Due to Volcanic Activity
At the plant sampling locations, top soil (0–10 cm) samples were collected. In Table 2 macro element composition of the soil at different sampling sites, pH and sulphate content are summarized.
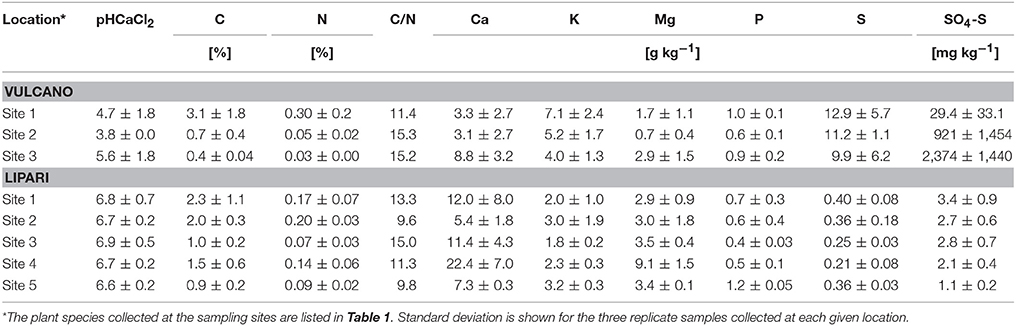
Table 2. Macro element composition, pH and sulphate content of the top soil samples (0–10 cm) collected at the plant sampling locations on the Aeolian Islands Vulcano and Lipari.
The data clearly indicate soil acidification due to the fumarolic gases on Vulcano with the lowest pH value of 3.8 at sampling site 2 and an overall strong acidification on Vulcano with a mean of pH 4.7 compared to a mean of pH 6.7 on Lipari. Acidification is accompanied on Vulcano by an accumulation of sulphur in the soil with a high proportion of sulphate (up to 24% at site 3 on Vulcano). As a consequence, Vulcano soil is depleted of Ca and Mg, especially at site 1 and 2 with high soil acidification (Table 2). Potassium (K) and phosphor (P) are the only macro elements, which were not depleted at any site on Vulcano compared to the sites on Lipari.
In Table 3, trace element composition and selected metal(loid)s are summarised for the sampling sites. The data demonstrate the high variability of soil characteristics on each of the two islands studied. For example, site 3 on Vulcano was extremely high in Cu with 334 mg per kg soil compared to all other sampling sites. Also Cd, Mn, Mo, and As contents were quite high at this sampling site in comparison to other sampling sites on Vulcano and Lipari. Apparently, site 3 on Vulcano differs from the other sampling sites on both island. In the present study, an accumulation of As is observed, which is reported frequently in areas surrounding active volcanoes (Kabata-Pendias and Pendias, 1985). High As concentrations in the range of 2.1–25 ppm were previously determined in fumaroles from Vulcano in the years of 1978 to 1993 (Signorelli et al., 1998).
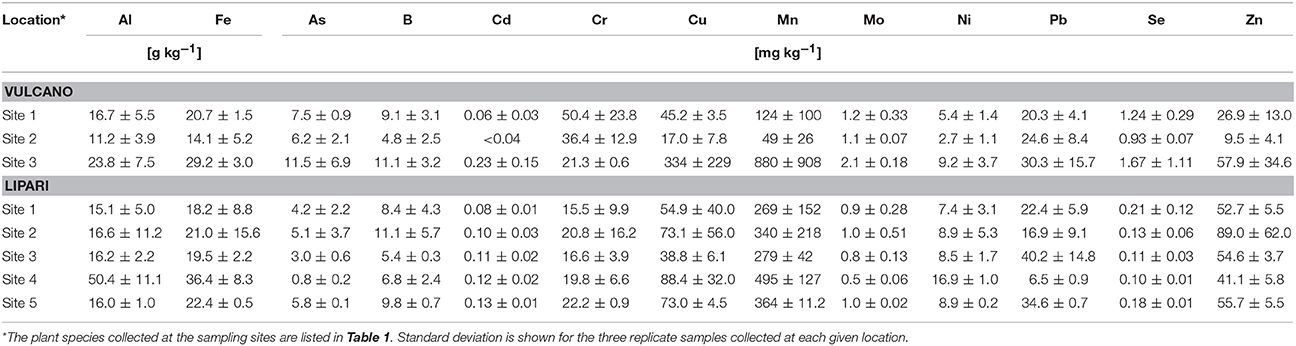
Table 3. Trace element composition of the soil samples collected on the Aeolian Islands Vulcano and Lipari.
Table 4 visualises soil data in correspondence to specific plant species, which were sampled both, from sites on Vulcano and Lipari. For each species, a ratio of means was calculated of soil element contents with the sampling site on Lipari set to 100%. The colours indicate the shift of this ratio with green depicting a depletion of an element in the Vulcano soil with values of 90% and lower. Red highlights an enrichment in soil contents on Vulcano with 110% and higher. This way the extreme amount of S in the soil of Vulcano is visualized for each sampling site. For example, on site 3 on Vulcano (location of O. pes-caprae, M. polymorpha, and T. repens) a maximal enrichment of 3,905% was observed compared to the Lipari sampling site. Other elements that are present in much higher amounts on Vulcano over all sampling sites include K, Mo and Cr, for the latter element with the exception of the sampling sites of O. pes-caprae and T. repens. Some elements revealed a depletion in the Vulcano soil relative to the control island Lipari. Mg is depleted at all sampling sites with the lowest amount of 22% for F. capreolata on sampling 2 on Vulcano compared to the Lipari control. Apparently, the volcanic activity has no significant influence on most trace elements in the soil except for As and Cr, but shows strong effects on the macro element composition in the topsoil.
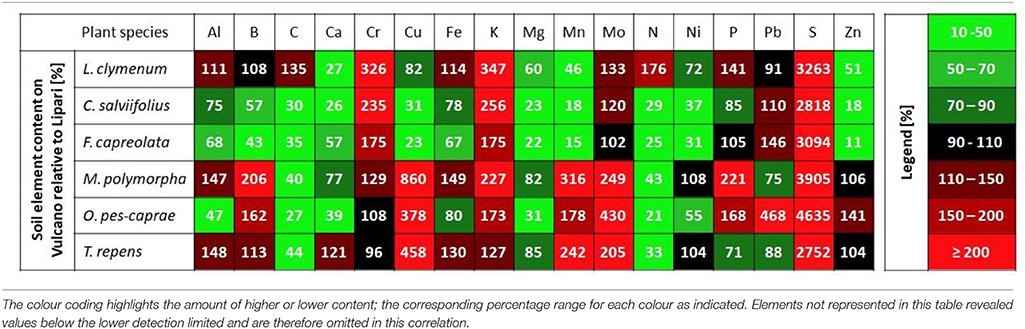
Table 4. Soil element content on Vulcano relative to Lipari sites (%) grouped to the species, which were harvested from both islands.
Significance of Volcanic Activity for Plant Species Element Composition
Having seen prominent differences between the sampling sites in macro element, but not in the trace element composition of the soil, it was tested whether these differences are reflected in the plant material. This may be expected because plants as sessile organisms cannot escape from high pollution, but rather cope with adverse environmental factors by adaptation strategies to ensure survival. Therefore, element analysis was performed with the plant material from all sampling locations (Tables 5, 6). The data were subjected to a two-factorial ANOVA in order to assess differences in nutrient acquisition between the plant species investigated and differences in element accumulation in relation to the specific element composition of the sampling site. On Vulcano, plants accumulated significantly more K, Mg, and S (Table 5) as well as Fe, Mn, Al, B, Ni, and Zn (Table 6). Significantly lower amounts of Ca were found in the leaves of plants on Vulcano, which coincides with the lower Ca contents determined in the soil (Table 2). F. capreolata leaves possess a significantly higher Ca concentration than C. salviifolius, E. camaldulensis, R. ulmifolius, and O. pes-caprae, while Ca concentrations in L. clymenum, M. polymorpha, and T. repens were not significantly different. The highly significant interaction indicates a correlation between plant species and sampling site: plant species with a higher Ca concentration on Vulcano such as F. capreolata show a further increased Ca concentration when grown on Lipari. The foliar Mg content was found to be independent from the low availability in the soil.
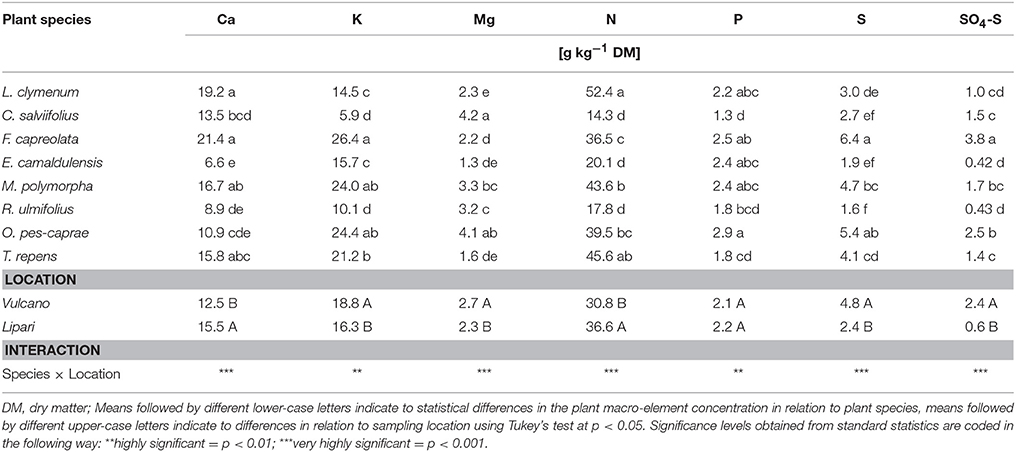
Table 5. Macro element composition of the eight investigated plant species collected on the Aeolian islands Vulcano and Lipari: Two-factorial ANOVA of the elements in relation to plant species and the plant composition correlating to the location of the sampling sites was performed.
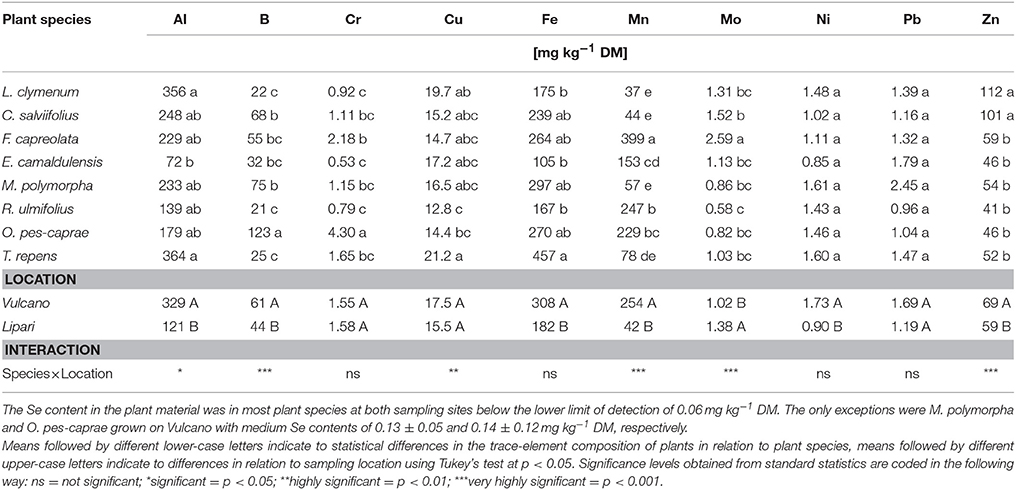
Table 6. Trace element composition of the investigated plant species collected on the Aeolian Islands Vulcano and Lipari: Two-factorial ANOVA of the elements in relation to plant species and the plant composition correlating to the location of the sampling sites was performed.
The most important differences in macro element accumulation were found for sulphur. In general, F. capreolata showed the highest sulphur content of 6.4 g kg−1 dry mass (DM) averaged over both sampling locations. Furthermore, high sulphur contents were determined in leaves of the herbaceous species O. pes-caprae with 5.4, M. polymorpha with 4.7 and T. repens with 4.1 g kg−1 DM. However, woody plants such as the shrub or the tree species studied showed lower average sulphur contents, i.e., C. salviifolius 2.7 and E. camaldulensis 1.9 g kg−1 DM. All herbaceous species showed very low dry matter contents (13–23% of fresh weight), whereas C. salviifolius had significantly higher dry matter content (27–39% of fresh weight; for details see Baillie et al., 2016). As a result, a significant negative correlation was found between the sulphur content of the plant species studied and their dry matter content (Figure 2). Plants with a higher dry matter content seem to have more effective avoidance mechanisms (Rennenberg, 2014), to protect themselves from an extreme sulphur accumulation. This also correlates with these species being perennial, which means they need to cope with the excess sulphur over more than one growth period leading to the necessity of physiological adaptation.
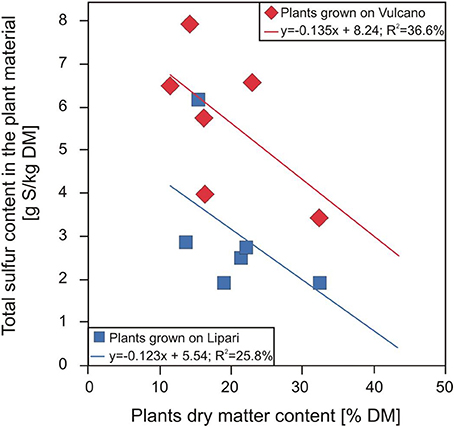
Figure 2. Relationship between the total sulphur content in the plant material and the plant dry matter content of different plant species grown on Lipari (blue dots) and Vulcano (red dots). (E. camaldulensis and R. ulmifolius data are excluded because R. ulmifolius grew on a stone wall and E. camaldulensis as a tree behave differently to herbaceous crops).
Foliar accumulation of the trace elements Al, B, Fe, Mn, Ni, and Zn was significantly increased in plants grown on Vulcano, despite comparable amounts in the soil (Tables 6, 7). Mo was the only trace element which was significantly decreased in the leaves of plants grown on Vulcano compared to Lipari despite the fact that soil Mo concentrations were similar or (slightly) increased on Vulcano. In addition, due to the low soil pH Al toxicity might easily occur at Vulcano sampling sites. Al3+ mobilization increases, when the soil pH drops below 4.5 and the saturation of the effective cation exchange capacity (CECeff) with Al3+ is increasing (Scheffer and Schachtschabel, 2016).
Corresponding to the calculation of soil element ratios on both islands, depicted in Table 4, ratios of the foliar element contents between the Islands Vulcano and Lipari were calculated to visualise differences in element accumulation between the two islands. The results of this calculation for all plant species shown in Table 7, highlight increases in element content on Vulcano in shades of red and decreases in shades of green. All plant species accumulated significantly more sulphur on Vulcano. Only F. capreolata accumulated sulphur on both islands in similar amounts, independent of the environment (Table 5). The accumulation of sulphur on Vulcano was very highly significant (p < 0.001***) in case of M. polymorpha and O. pes-caprae, highly significant (p < 0.01**) in case of L. clymenum and only significant (p < 0.05*) in case of C. salviifolius and T. repens. Additionally, prominent enrichment of foliar Al, Mn, Ni, and Zn was found in most species on Vulcano. Only C. salviifolius showed lower contents of Mn and Zn. On the other hand, accumulation of Mo and P in the leaves was decreased in many species on Vulcano.
Different environmental factors strongly affect the foliar metal accumulation in plants. These factors include the metal concentration in the soil, the soil type and pH as well as the plant species (Hooda et al., 1997). Plant availability of most metals increases with decreasing pH, only Mo is more available at higher soil pH (Kabata-Pendias, 2004; Kaiser et al., 2005). This is very well reflected by the higher foliar accumulation of Al, Cu, Fe, and Zn in plants grown on Vulcano compared to Lipari (Table 7) with Mo representing an exception. Furthermore, the interaction between species and location in element accumulation (Tables 5, 6) indicates a strong relationship between site parameters and element availability and consequently, plant uptake.
The soil-to-plant metal transfer factor (TF value = the concentration of a metal in plants divided by its concentration in the soils) provides an indication of plant responses to enhanced soil metal contents. Based on this factor, plants can be classified as excluders (low metal uptake), indicators (metal uptake reflects the soil concentration) or accumulators (high metal uptake) (Metz et al., 2001). In the present study, the metal concentrations of the different species are in the same range as reported for different agricultural crops and grassland species (Kühnen and Goldbach, 2004). Hooda et al. (1997) reported TFs in the range of 0.002–0.02 for Pb, 0.03–9.5 for Cu, 0.03–0.46 for Ni and 0.13–2.3 for Zn for different agricultural crops. When calculating metal transfer factors in the present study, most ratios are far below 1.0 indicating a low accumulation of metals from the soil into the plants. Especially low ratios were determined for Fe, Pb, Cr, and Al but also for Ni and Cu (Table 8). F. capreolata showed increased Mn accumulation on Vulcano with TF values of 13.27, while ratios for all other species on the islands were below 1. Zn was accumulated to a higher extent by C. salviifolius, F. capreolata and L. clymenum especially on Vulcano; its TF was above 1.0 also for M. polymorpha and T. repens on this island. Also Ni and Cu were less excluded by C. salviifolius and F. capreolata on Vulcano, but only with TF values of 0.46–0.48 for Ni and 0.72–0.91 for Cu (Table 8). The plant with the highest dry matter content in this study, C. salviifolius (>32% DM), accumulated higher metal contents on Lipari compared to the herbaceous species, but this did not result in increased foliar metal concentrations compared to the other species studied (Table 6).
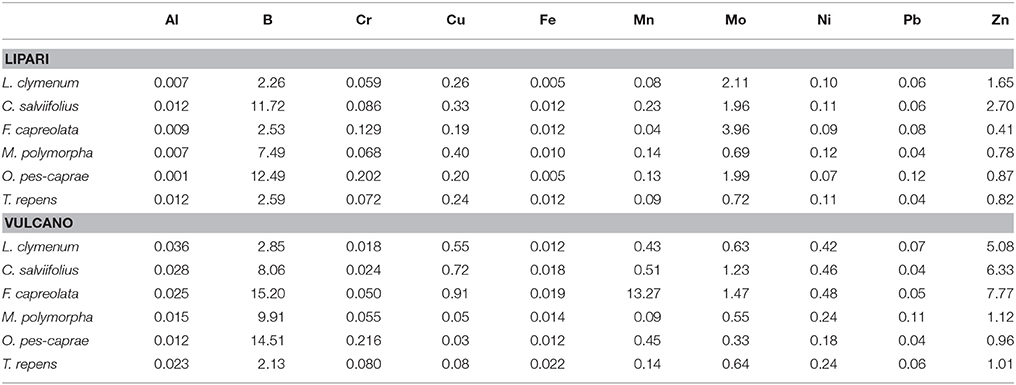
Table 8. Metal transfer factors (TF values) calculated by dividing the metal concentration in the dry plant material through the metal concentration in the soil (values >1 indicate to an accumulation of the respective element in the plants).
Apparently, volcanic activity had a strong effect on the element composition of plant leaves, mostly as a consequence of the acidification of the soil on Vulcano, which leads to enhanced metal(loid) mobility.
Cross-Species Interactions of Element Composition With Volcanic Activity
To assess cross-species interactions of plant element composition with changes in soil element composition by volcanic activity, the entire data set on plant element composition was subjected to correlation analysis with the data set on soil element composition, soil pH and soil carbon content (Table 9). The analysis showed a strong S to S and sulphate to sulphate interaction between plants and soil, but no general plant to soil interaction for the other elements studied. No other result can be expected as from the total element concentrations in the soil only a very small proportion is usually plant available for most elements (e.g., Bloem et al., 2017). Moreover, plants developed avoidance strategies to deal with toxic elements as reviewed for Pb by Sharma and Dubey (2005). Uptake studies in Raphanus sativus have demonstrated that roots have the ability to take up significant quantities of Pb whilst simultaneously greatly restricting its translocation to above ground parts (Lane and Martin, 1977; Samuilov et al., 2016).
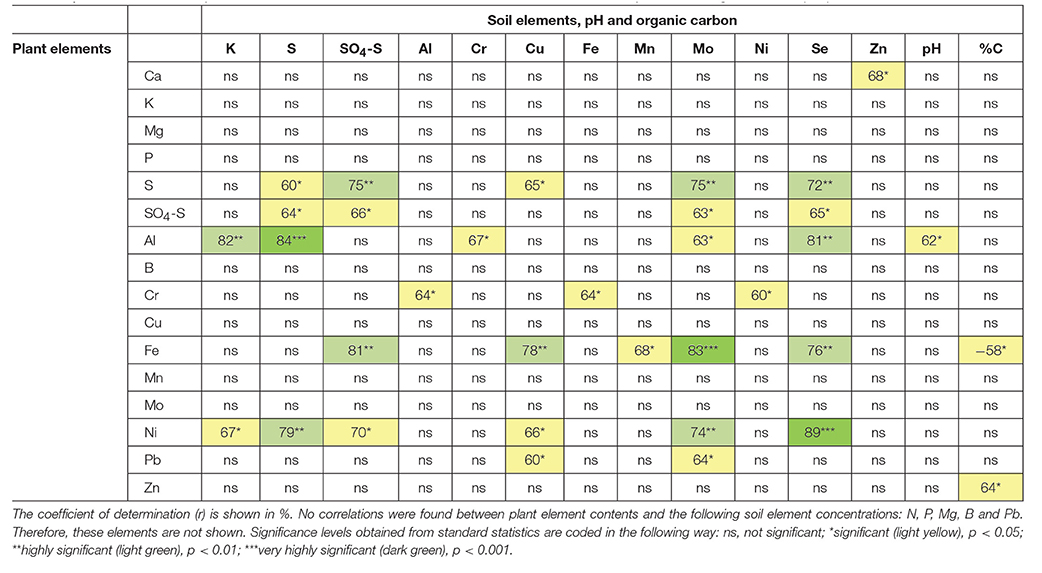
Table 9. Correlation matrix of plant macro- and microelements in relation soil element concentrations, pH and soil organic carbon (%C).
Our results clearly show the dominance of sulphur emissions for the interaction between the element composition in plants and soil. Apparently, plant element composition beyond sulphur/sulphate is largely independent of differences in element composition in the soil. Still several element contents in the soil affected other elements in the plants. For example, (1) the S content of the soil correlated with the Al and Ni content of the plants, (2) the Se and Mo content of the soil with the S, sulphate, Al, Fe, and Ni content of the plants and (3) the Cu content of the soil with the S, Fe, Ni, and Pb content of the plants. The mechanistic basis for this cross-species, cross-element interactions remains to be elucidated in particular, because the present approach provides circumstantial evidence rather than causal relationships. Se is taken up by the plants via the same transporters as S because both elements show chemical similarities and Se can replace S in assimilation reactions and compounds (Pilon-Smits and Quinn, 2010). This is most likely the reason why comparable correlations were found between soil-S and soil-Se with plant element concentrations (Table 9). Se was present in distinctly higher concentrations in the soil of Vulcano compared to Lipari (Table 3), but was not accumulated to a much higher extent by most plant species. Se concentrations above the detection limit of 0.06 mg kg−1 DM were only observed in plant material of M. polymorpha and O. pes-caprae grown on Vulcano. Interestingly, F. capreolata took up elevated amounts of S but not of Se, indicating a preferential uptake of S from the atmosphere, as plants that accumulate high amounts of S from the soil also accumulate Se to a higher extent in general (Pilon-Smits and Quinn, 2010).
A cross species assessment of element composition with C and dry matter accumulation (Table 10) showed a strong negative correlation of dry matter accumulation with the P content of the plants and a strong negative correlation of the C accumulation with the K, S, sulphate, Fe, Mn, and Ni contents. From these results it may be concluded that the latter elements negatively affected photosynthetic CO2 fixation of the plants studied and that K accumulation counteracted the loss of dry weight of these negative effects at the study sites. Again, more detailed studies are required to elucidate whether this is a specific situation of the study sites or of more general significance.
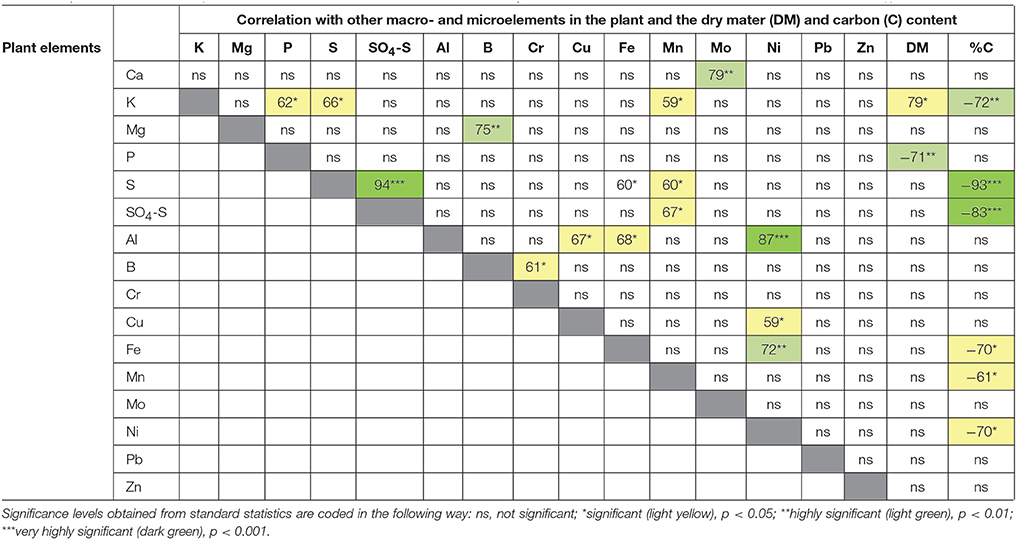
Table 10. Correlation matrix of plant macro- and microelements and in relation to dry mater and carbon content. The coefficient of determination (r) is shown in %.
Different Plant Strategies to Cope With Environmental Stress by Volcanic Activity
Plants have developed different strategies to cope with high SO2/H2S concentrations in the atmosphere due to volcanic activities (Baillie et al., 2016). Therefore, it may be assumed that such strategies to cope with the volcanic environment are reflected by the plants' element composition as well.
The relative primordial plant species, F. capreolata did not reveal any avoidance strategy to high SO2/H2S concentration (Baillie et al., 2016) and took up high amounts of S on both islands. In addition, F. capreolata accumulated many other elements to a higher extent than the other species investigated. Especially Ca, K, Mn, and Mo were accumulated in higher concentrations by F. capreolata (Tables 5, 6). Only K and Mn show an increase on Vulcano when comparing the foliar contents between the sampling sites on the two islands for this species (Table 7).
Based on the element composition, the grouping of the species according to their sulphite detoxification strategies in response to high SO2/H2S concentration described by Baillie et al. (2016) cannot be transferred directly to foliar element concentrations. Therefore, a clustering of selected parameters was performed (Figure 3) including the leaf element contents of the metals Ca, K and Mg and the trace elements Al, B, Mn, Mo, and Zn. A clustering including the macro elements (C, N, P, S) did not show a conclusive picture and was therefore not taken into account. The trace elements were selected not only due to significantly higher amounts in at least two species on both Islands, but also based on significant correlations between the sampling sites revealed by ANOVA (Tables 5, 6). Thus, the selected trace elements show strong variance between the samplings sides.
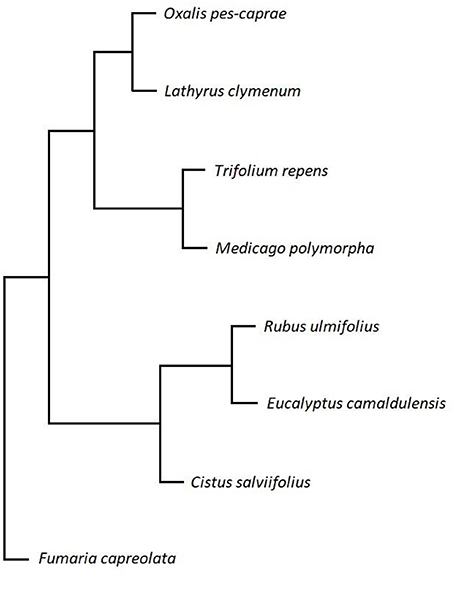
Figure 3. Hierarchical clustering of the tested species based on the pattern of element composition under volcanic stress. To allow a correlative analysis of all given leave data values from control Island Lipari within one species were set to 100%. Values from Vulcano were calculated in accordance to Lipari, resulting in calculated ratios for each element and each species, respectively. For clustering calculated ratios of the plant material of the following elements were used: Al, B, Ca, K, Mg, Mn, Mo, and Zn. A clustering including the macro elements (C, N, P, S) did not show a conclusive picture and was not taken into account. To ensure an equally spread distribution the percentage values were transformed into a distance matrix and the clustering was performed with the statistical analysis software R (R Core Team, 2014) and the base package hclust function.
The previous study of Baillie et al. (2016) focussed on SO2/H2S-detoxification parameters of the same plant species and revealed three different groups based on their sulphite detoxification strategies. In contrast, hierarchical clustering based on the element parameters in the present study reveals a separation into two main groups of plants with different reaction to the volcanic environment on Vulcano (Figure 3). F. capreolata again constituted an outside group in the cluster. The species O. pes-caprae, M. polymorpha, T. repens, and L. clymenum form one group and the species R. ulmifolius, C. salviifolius, and E. camaldulensis the other. Apparently, hierarchical clustering distinguished between perennial herbaceous plants on one side and woody plants such as shrubs and trees on the other side. Thus, clustering of the same species based on element parameters does not show the same distribution as clustering according to sulphite detoxification strategies, but follows evolutionary likeliness. Apparently, there is no direct interaction of element composition and sulphur detoxification strategies in the species studied.
Conclusion
Environmental stress caused by volcanic activity affects plants by gaseous compounds, which enter the plants via the stomata, or by accumulation of compounds from the rhizosphere. A previously published paper showed that the investigated plants are negatively affected by the high SO2/H2S concentrations emitted on Vulcano. Due to the proximity of the tested species to the fumaroles on Vulcano a strong soil acidification occurred which lead to an enhanced accumulation of potentially toxic elements and negative effects on plant element composition. This is reflected by the high amounts of Al in the plants, most likely mediated by the pH shift in the soil. The content of Al in the soil itself, however, was lower on Vulcano for some sampling locations compared to the control soil on Lipari. Herbaceous species accumulated more sulphur than woody plant species. However, the transfer of heavy metals from the soil into vegetative plant parts was less pronounced than the transfer of sulphur, indicating the significance of avoidance strategies to cope with heavy metals in the environment.
The cluster analyses based on element contents revealed two groups of plants with F. capreolata as an outside group. The analyses separated the perennial herbaceous plants (O. pes-caprae, M. polymorpha, T. repens, and L. clymenum) from higher shrubs or trees (R. ulmifolius, C. salviifolius, and E. camaldulensis). This signifies that the species, which showed different strategies to detoxify excess volcanic sulphur, also cluster by their element contents. Thus, both avoidance and accumulation strategies for particular elements as well as detoxification strategies for excess sulphur contribute to the adaptation of plants to the stressful environment in the vicinity of volcanoes.
Author Contributions
C-KB, HR, RH, and EB planned and designed the project. RH collected the samples on Vulcano and Lipari. C-KB, EB, and HR performed the experiments and C-KB and EB handled statistical analysis. All authors analysed and discussed the results. C-KB, EB, RH, and DK were primarily involved in drafting the manuscript and C-KB, EB, and DK produced figures and tables. HR and RM critically read the manuscript and improved the text, all authors finalised it. EB and RH coordinated the work.
Conflict of Interest Statement
The authors declare that the research was conducted in the absence of any commercial or financial relationships that could be construed as a potential conflict of interest.
Acknowledgments
This work was financially supported by the Deutsche Forschungsgemeinschaft (grant Hä3107-5/1 and RE515/40-1).
Supplementary Material
The Supplementary Material for this article can be found online at: https://www.frontiersin.org/articles/10.3389/fenvs.2018.00052/full#supplementary-material
References
Baillie, C.-K., Kaufholdt, D., Karpinski, L. H., Schreiber, V., Hänsch, S., Evers, C., et al. (2016). Detoxification of volcanic sulfur surplus in planta: three different strategies of survival. Environ. Exp. Bot. 126, 44–56. doi: 10.1016/j.envexpbot.2016.02.007
Bargagli, R., Barghigiani, C., Siegel, B. Z., and Siegel, S. M. (1991). Trace metal anomalies in surface soils and vegetation on two active island volcanoes: Stromboli and Vulcano (Italy). Sci. Total Environ. 102, 209–222. doi: 10.1016/0048-9697(91)90315-6
Bloem, E., Haneklaus, S., Haensch, R., and Schnug, E. (2017). EDTA application on agricultural soils affects microelement uptake of plants. Sci. Total Environ. 577, 166–173. doi: 10.1016/j.scitotenv.2016.10.153
Bloem, E., Haneklaus, S., and Schnug, E. (2002). Optimization of a method for soil sulphur extraction. Commun. Soil Sci. Plant Anal. 33, 41–51. doi: 10.1081/CSS-120002376
Brychkova, G., Xia, Z., Yang, G., Yesbergenova, Z., Zhang, Z., Davydov, O., et al. (2007). Sulfite oxidase protects plants against sulfur dioxide toxicity. Plant J. 50, 696–709. doi: 10.1111/j.1365-313X.2007.03080.x
Burgess, S. S. O., Adams, M. A., Turner, N. C., White, D. A., and Ong, C. K. (2001). Tree roots: conduits for deep recharge of soil water. Oecologia 126, 158–165. doi: 10.1007/s004420000501
De Rosa, R., Donato, P., and Scarciglia, F. (2016). On the origin and post-depositional history of widespread massive ash deposits: the case of Intermediate Brown Tuffs (IBT) of Lipari (Aeolian Islands, Italy). J. Volcanol. Geotherm. Res. 327, 135–151. doi: 10.1016/j.jvolgeores.2016.07.007
DIN ISO 10390 (1997). Handbuch Bodenuntersuchung, Bodenbeschaffenheit: Bestimmung des pH Wertes. Beuth; Berlin; Wien; Zürich: Wiley-VCH.
Giggenbach, W. F., Tedesco, D., Sulistiyo, Y., Caprai, A., Cioni, R., Favara, R., et al. (2001). Evaluation of results from the fourth and fifth IAVCEI field workshops on volcanic gases, Vulcano islands, Italy and Java, Indonesia. J. Volcanol. Geotherm. Res. 108, 157–172. doi: 10.1016/S0377-0273(00)00283-3
Graziani, G., Martilli, A., Pareschi, M. T., and Valenza, M. (1997). Atmospheric dispersion of natural gases at Vulcano island. J. Volcanol. Geotherm. Res. 75, 283–308. doi: 10.1016/S0377-0273(96)00067-4
Hamisch, D., Randewig, D., Schliesky, S., Bräutigam, A., Weber, A. P., Geffers, R., et al. (2012). Impact of SO2 on Arabidopsis thaliana transcriptome in wildtype and sulfite oxidase knockout plants analyzed by RNA deep sequencing. New Phytol. 196, 1074–1085. doi: 10.1111/j.1469-8137.2012.04331.x
Heber, U., and Hüve, K. (1998). Action of SO2 on plants and metabolite detoxification of SO2. Int. Rev. Cytol. 177, 255–286.
Hooda, P. S., Mc Nulty, D., Alloway, B. J., and Aitken, M. N. (1997). Plant availability of heavy metals in soils previously amended with heavy applications of sewage sludge. J. Sci. Food Agric. 73, 446–454. doi: 10.1002/(SICI)1097-0010(199704)73:4<446::AID-JSFA749>3.0.CO;2-2
Hu, B., Simon, J., Günthardt-Goerg, M. S., Arend, M., Kuster, T. M., and Rennenberg, H. (2015). Changes in the dynamics of foliar n metabolites in oak saplings by drought and air warming depend on species and soil type. PLOS ONE 10:e0126701. doi: 10.1371/journal.pone.0126701
Kabata-Pendias, A. (2004). Soil-plant transfer of trace elements – an environmental issue. Geoderma 122, 143–149. doi: 10.1016/j.geoderma.2004.01.004
Kabata-Pendias, A., and Pendias, H. (1985). Trace Elements in Soils and Plants. Boca Raton, FL: CRC Press, Inc.
Kaiser, B. N., Gridley, K. L., Brady, J. N., Phillips, T., and Tyerman, S. D. (2005). The role of molybdenum in agricultural plant production. Ann. Bot. Lond. 96, 745–754. doi: 10.1093/aob/mci226
Kochergina, Y. V., Udatný, M., Penížek, V., and Mihaljevic, M. (2017). Mobility of Pb, Zn, Cu and As in disturbed forest soils affected by acid rain. Environ. Monit. Assess. 189:570. doi: 10.1007/s10661-017-6306-7
Kühnen, V., and Goldbach, H. E. (2004). Schwermetallbilanzen Verschiedener Betriebstypen: Eintragswege, Flüsse, Minderungspotential. Forschungsbericht, Nr. 118; Lehr- und Forschungsschwerpunkt “Umweltverträgliche und Standortgerechte Landwirtschaft,” Landwirtschaftliche Fakultät der Rheinischen Friedrich-Wilhelms-Universität Bonn.
Lane, S. D., and Martin, E. S. (1977). A histochemical investigation of lead uptake in Raphanus sativus. New Phytol. 79, 281–286. doi: 10.1111/j.1469-8137.1977.tb02206.x
Menzel, D. B., Keller, D. A., and Leung, K. H. (1986). “Covalent reactions in the toxicity of SO2 and sulfite,” in Biological Reactive Intermediates III, eds J. J. Kocsis, D. J. Jollow, C. M. Witmer, J. O. Nelson, and R. Snyder (Boston, MA: Springer), 477–492.
Metz, R., Böken, H., Witten, J., and Hoffmann, C. (2001). Schwermetalle im Pfad Boden-Pflanze-Tier-Mensch. VDLUFA Schriftenreihe 57, 776–781.
Nagajyoti, P. C., Lee, K. D., and Sreekanth, T. V. M. (2010). Heavy metals, occurrence and toxicity for plants: a review. Environ. Chem. Lett. 8, 199–216. doi: 10.1007/s10311-010-0297-8
Novozamsky, I., Van Ech, R., Van der Lee, J. J., Houba, V. J. G., and Temminghoff, E. (1986). Determination of total sulphur and extractable sulphate in plant material by inductively-coupled plasma atomic emission spectroscopy. Commun. Soil Sci. Plant Anal. 17, 1147–1157. doi: 10.1080/00103628609367780
Pilon-Smits, E. A. H., and Quinn, C. F. (2010). “Selenium metabolism in plants,” in Cell Biology of Metals and Nutrients, Plant Cell Monographs 17, eds R. Hell and R. R. Mendel (Berlin; Heidelberg: Springer-Verlag), 225–241. doi: 10.1007/978-3-642-10613-2_10
Randewig, D., Hamisch, D., Herschbach, C., Eiblmeier, M., Gehl, C., Jurgeleit, J., et al. (2012). Sulfite oxidase controls sulfur metabolism under SO2 exposure in Arabidopsis thaliana. Plant Cell Environ. 35, 100–115. doi: 10.1111/j.1365-3040.2011.02420.x
R Core Team (2014). R: A Language and Environment for Statistical Computing. Vienna: R Foundation for Statistical Computing. Available online at: http://www.R-project.org/
Rennenberg, H. (2014). “Communities and ecosystem functioning,” in Ecological Biochemistry – Environmental and Interspecies Interactions, eds G.-J. Krauss and D. H. Nies (Weinheim: Wiley-VCH), 77–91.
Samuilov, S., Lang, F., Djukic, M., Diunisijevic-Bojovic, D., and Rennenberg, H. (2016). Lead uptake increases drought tolerance of wild type and transgenic poplar (Populus tremula x P. alba) overexpressing gsh 1. Environ. Pollut. 216, 773–785. doi: 10.1016/j.envpol.2016.06.047
Seaward, M. R. D., and Richardson, D. H. S. (1989). “Atmospheric sources of metal pollution and effects on vegetation,” in Heavy Metal Tolerance in Plants: Evolutionary Aspects, eds A. J. Shaw (Boca Raton, FL: CRC Press), 75–94.
Sharma, P., and Dubey, R. S. (2005). Lead toxicity in plants. Braz. J. Plant Physiol. 17, 35–52. doi: 10.1590/s1677-04202005000100004
Signorelli, S., Buccianti, A., Martini, M., and Piccardi, G. (1998). Arsenic in fumarolic gases of Vulcano (Aeolian Islands, Italy) from 1978 to 1993: geochemical evidence from multivariate analysis. Geochem. J. 32, 367–382. doi: 10.2343/geochemj.32.367
Simon, J., Waldhecker, P., Brüggemann, N., and Rennenberg, H. (2010). Competition for nitrogen sources between European beech (Fagus sylvatica) and sycamore maple (Acer pseudoplatanus) seedlings. Plant Biol. 12, 453–458. doi: 10.1111/j.1438-8677.2009.00225.x
Keywords: abiotic stress, fumaroles, Lipari, metal accumulation, mineral transfer factors, Vulcano
Citation: Baillie C-K, Kaufholdt D, Meinen R, Hu B, Rennenberg H, Hänsch R and Bloem E (2018) Surviving Volcanic Environments—Interaction of Soil Mineral Content and Plant Element Composition. Front. Environ. Sci. 6:52. doi: 10.3389/fenvs.2018.00052
Received: 19 March 2018; Accepted: 25 May 2018;
Published: 13 June 2018.
Edited by:
Urs Feller, Universität Bern, SwitzerlandReviewed by:
Antonio Lidón, Universitat Politècnica de València, SpainBingcheng Xu, Institute of Soil and Water Conservation (CAS), China
Copyright © 2018 Baillie, Kaufholdt, Meinen, Hu, Rennenberg, Hänsch and Bloem. This is an open-access article distributed under the terms of the Creative Commons Attribution License (CC BY). The use, distribution or reproduction in other forums is permitted, provided the original author(s) and the copyright owner are credited and that the original publication in this journal is cited, in accordance with accepted academic practice. No use, distribution or reproduction is permitted which does not comply with these terms.
*Correspondence: Robert Hänsch, r.haensch@tu-braunschweig.de