The Holobiont as a Key to the Adaptation and Conservation of Wild Bees in the Anthropocene
- 1Laboratory of Agrozoology, Department Plants and Crops, Faculty of Bioscience Engineering, Ghent University, Ghent, Belgium
- 2Agroecology Lab, Université libre de Bruxelles (ULB), Brussels, Belgium
- 3Laboratory of Zoology, Research Institute for Biosciences, University of Mons, Mons, Belgium
- 4Laboratory of Microbiology, Department of Biochemistry and Microbiology, Ghent University, Ghent, Belgium
Ever since the development of James Watt's steam engine in 1784, and particularly after the Second World War, human societies have expanded across the world and are now acknowledged to overwhelm the Great Forces of Nature and the planetary boundaries (sensu Rockström et al., 2009). This, in turn, drove changes in the environment and the biogeochemical cycles in unprecedented ways (Gulev et al., 2021). We are experiencing the onset of a new geological epoch coined the “Anthropocene” and characterized by the overexploitation of resources (e.g., extensive soil use changes for agriculture and urbanization, and overfishing), the buildup of CO2 in the atmosphere, massive extinctions of biodiversity, etc., all leading to major and systemic changes at the planetary scale (Crutzen and Stoermer, 2000; Crutzen, 2002; Diaz et al., 2019).
Nowadays, there is undisputable evidence that the rise of Homo sapiens was –and still is– sadly associated with the widespread and often-irreversible demise of multitude of life forms on the planet, including negative population trends, population extirpations and significant range contractions leading eventually to species extinctions (Dirzo et al., 2014; Wagner et al., 2021). The survival of the remaining wildlife through the Anthropocene requires the evolution of new adaptations to today's rapid and extreme changes in environmental conditions and/or biological interactions. However, the plasticity required for species to be able to adapt to the current changing conditions has grown exponentially, and the speed at which species must adapt nowadays is many orders of magnitude faster in comparison with what they have experienced in the course of their evolution (see Otto, 2018; Halsch et al., 2021).
Understanding the plasticity and adaptability of individual species and communities, and how these enable them to survive the “Anthropocene filter” is a very challenging and key issue to predict to what extent the associated shifts and/or losses in biodiversity will translate into changes in ecosystem function and services. Wild bees are often depicted as a textbook case of a trait d'union between biodiversity and ecological processes, as they are actively involved in the sexual reproduction of insect-dependent wildflowers and crops alike, encompassing almost 90% of the angiosperm plants on the planet (Ollerton et al., 2011). They represent an excellent model group to tackle these questions as they show high species diversity with over 20,000 wild bee species described at the worldwide scale (Michener, 2007; Engel et al., 2016; Ascher and Pickering, 2020), >2,000 recorded in Europe (Michez et al., 2011), all with various population trends (Nieto et al., 2014; Cameron and Sadd, 2020). Indeed, different bee species display a large variation in morphological traits (e.g., body size from 3 mm to 4 cm), and contrasting “partial habitat” requirements (Westrich, 1996) that make them more or less successful in the Anthropocene. They also include exotic species that currently thrive in many different regions of the world (e.g., Megachile sculpturalis; Lanner et al., 2020). Recent studies suggest that the diversity of wild bees is increasingly threatened by Anthropocene-associated processes, with reports on the decline of bees emerging in various regions of the world such as in Europe (Nieto et al., 2014), as well as in South and North America (Cameron et al., 2011; Maharramov et al., 2013; Jacobson et al., 2018). One of the core messages of these studies on pollinator decline is that spatial and temporal population trends are not consistent among species: many species are declining (e.g., 33% of species recorded in Belgium are threatened with extinction as reported by Drossart et al., 2019), while others are currently expanding their distribution range (Dellicour et al., 2014; Dew et al., 2019; Ghisbain et al., 2021). Bees are therefore a very appropriate model group to study pollinator insect plasticity and adaptability to the Anthropocene across different ecological and evolutionary contexts, with the potential to uncover key facets of the mechanisms associated with bee population trends and, consequently, to propose accurate mitigation strategies (Engel et al., 2016).
While potential mechanisms of plasticity and adaptation of bees to the threats posed by the Anthropocene are still explored, an increasing number of studies have demonstrated the importance and the key functional role of bee gut microbiome as a toolbox regulating bee nutrition and promoting bee health (Zheng et al., 2018; Steffan et al., 2019; Miller et al., 2021). Bees are characterized by a derived herbivorous diet (i.e., their ancestors were carnivorous) which can be broad (generalist) or narrow (specialist) based on the breadth of the taxonomic spectrum of host plants visited for the collection of floral resources (mainly pollen, nectar and/or oil) by the adult females to feed their larvae (Dötterl and Vereecken, 2010; Vaudo et al., 2015; Michez et al., 2019; Rasmussen et al., 2020). Recent evidence points toward the key role of the bee microbiome in their ability to process their pollen-rich diet (Steffan et al., 2019; Keller et al., 2021; Miller et al., 2021). Furthermore, growing evidence suggests that the fermenting microbial biomass itself contributes to the nutritional value for the brood: a good example is that larval stages of different bee species likely acquire bacteria essential to their development through their diet (Dharampal et al., 2019; Rothman et al., 2019; Voulgari-Kokota et al., 2019a,b). Besides its role in nutrition, the microbiota can also protect the brood cell environment against pathogens and brood spoilage by lowering the pH and producing antimicrobial metabolites, and can help detoxify plant toxins and xenobiotics (Parmentier et al., 2018; Praet et al., 2018; Cuesta-Maté et al., 2021; Keller et al., 2021).
Moreover, bees and microorganisms experience specific and contrasting modes and speeds of genetic evolution (e.g. mutation, gene loss, evolutionary pace; Kwong et al., 2014), but additional drivers of bacterial evolution can also be mediated through gene exchange via homologous recombination and lateral gene transfer. Most bees typically have one generation per year in temperate regions, although some species can have several generations per year (Santos et al., 2019); by contrast, microorganisms reproduce through several thousands of generations in the course of a “bee season” (Gibson et al., 2018). This short generation time and rapid genetic evolution of microorganisms and the possibility of pollinators to use flowers as microbial transfer hubs provide the bee host with a highly dynamic adaptive potential (Keller et al., 2021). Bees with a stable microbiota or those that were able to shift to alternative diets or which can have rapid plastic responses, are more likely to adapt to an “Anthropocene diet”.
In the Anthropocene context of changing landscapes and fluctuating floral resources (e.g. Roger et al., 2016; Gao et al., 2020), the health and survival of bees, particularly the generalist species, which are ecological linchpin species and key for the resilience of ecological networks (e.g., Bascompte and Stouffer, 2009), are likely to be more intimately associated with the plasticity of their microbiota than previously thought. The time is ripe to embrace a “paradigm shift” where the conservation of bees is intertwined with the conservation of their microbiome (i.e. bee holobiont conservation). Both holobiont partners (i.e., bees and microbiome) contribute to its plasticity: the microbiome is primarily involved in processing food, toxins and promoting immunity, while the bee host contributes to thermal tolerance and mobility. Some bee species seem to be able to face environmental changes through plasticity in their thermoregulation (e.g., Martinet et al., 2021a,b), thermal tolerance (Maebe et al., 2021a), phenology (Duchenne et al., 2020; Gérard et al., 2020a), size (Gérard et al., 2020b, 2021), nesting behavior (Ghisbain et al., 2021) or diet (Scheper et al., 2014), which might partly explain their contrasting population trends (reviewed by Maebe et al., 2021b).
With this opinion paper, we advocate that future research should embrace and implement the “holobiont” concept (Bordenstein and Theis, 2015; Carrier and Reitzel, 2017; O'Malley, 2017) also for bees, as well as obtain and disseminate innovative and integrated insights into the plasticity mechanisms and adaptation of the bee holobiont to anthropogenic changes of the environment. This could be done by disentangling the evolutionary associations of plant-pollinator-microbiota networks, how these associations have changed in an Anthropocene context of human-altered habitats (e.g., intensive agriculture and urbanization), and by studying each holobiont partners in isolation. The latter could be achieved by (i) the development of gnotobiotic (or “germ-free”) bees to assess the adaptive responses of the bee without the bee's microbiota, as recently performed with honeybees and bumblebees (Zheng et al., 2018; Hammer et al., 2021), and (ii) by creating an in vitro bee gut simulator to assess the plasticity of the microbiome per se (e.g., sensitivity to xenobiotics). The combined knowledge obtained from both study systems, being which microbiota has a particular importance for a particular bee species in a specific environment, together with the effects of a specific xenobiotic on the microbiota, will provide insights into the key microbiota for the survival of a bee in a specific environment. For instance, negative effects of pesticides on the bees' microbiota – and thus the bee holobiont – may have deleterious effects for the adaptation potential of the host, and thus on a longer-term harbor a sublethal threat to bees that has received virtually no attention to date. Plant phytochemicals also have this potential to negatively impact the host microbiota: for instance, several invasive plants have a high polyphenol content – such as in honey from Japanese knotweed (Fallopia japonica) (Bobiş et al., 2019) or in the pollen of the Himalayan Balsam (Impatiens glandulifera) (Vanderplanck et al., 2019) – which has been shown to decrease the prevalence of Apicystis bombi in bumblebees (Vanderplanck et al., 2019), and could potentially impact also the host microbiota. In turn, this knowledge could set the base for specific new regulatory measures for the use of these xenobiotics and invasive plants, for the conservation of bee populations.
Therefore, a framework to monitor the holobiont as a part of conservation or risk assessment practices should be developed. While it remains still mostly speculative, as future research still needs to generate the essential information to produce such a framework, some general or basic ideas can already be put in place. By identifying “marker” components of the bee microbiota such as “indicator” OUT's (similar to indicator species, see e.g., Dufrêne and Legendre, 1997), and their possible statistical association with function loss (e.g. in pesticide detoxification or food digestion), we will gain insights into the adaptive vs. random nature of the bee microbiota, i.e. the extent to which and how its diverse components impact the survival potential of the bee subject to environmental stressors in a given locale (e.g., in agricultural areas). These results could also be used as a lever toward more sustainable and regenerative land use practices.
To conclude, an integrative and interdisciplinary approach as summarized in Figure 1 will help uncover how and to what extent the bee holobiont is adapted to present-day and future Anthropocene environments. Future research should also help increase our understanding of how response diversity, through the presence of bee species with contrasting and variable microbiota, can contribute to the resilience and maintenance of ecosystem functioning, and the important ecosystem service of pollination. The “holobiont approach” has much potential to understand wild bee population trends and to provide evidence-based recommendations on hitherto overlooked aspects of bee conservation relevant to pollinator protection strategies at all scales, from regional to global.
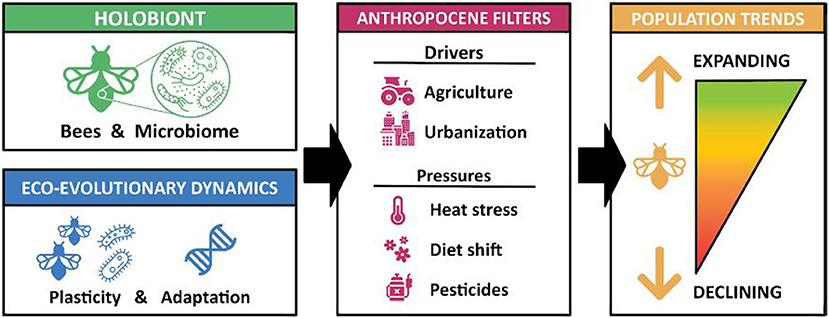
Figure 1. Conceptual diagram showing both holobiont partners with their different eco-evolutionary dynamics, and who together can have a greater chance to pass through the ongoing pressures of Anthropocene filters, which may better explain the observed bee population trends.
Author Contributions
KM and GS conceived this opinion paper. KM, NV, and GS developed and wrote the initial manuscript. NP, SR, DC, DM, PV, and GS participated in the revisions. All authors have read and approved the final manuscript.
Funding
This work was supported through funding from the FWO and F.R.S.-FNRS under the Excellence of Science (EOS) programme for the project climate change and its impact on pollination services (CLiPS, n°3094785).
Conflict of Interest
The authors declare that the research was conducted in the absence of any commercial or financial relationships that could be construed as a potential conflict of interest.
Publisher's Note
All claims expressed in this article are solely those of the authors and do not necessarily represent those of their affiliated organizations, or those of the publisher, the editors and the reviewers. Any product that may be evaluated in this article, or claim that may be made by its manufacturer, is not guaranteed or endorsed by the publisher.
References
Ascher, J. S., and Pickering, J. (2020). Discover life bee species guide and world checklist (Hymenoptera: Apoidea: Anthophila). Available online at: http://www.discoverlife.org/mp/20q?guide=Apoidea_species (accessed September 13, 2021)
Bascompte, J., and Stouffer, D. B. (2009). The assembly and disassembly of ecological networks. Phil. Trans. R. Soc. B. 364, 1781–1787. doi: 10.1098/rstb.2008.0226
Bobiş, O., Dezmirean, D. S., Bonta, V., Moise, A. R., Paşca, C., Domokos, T., et al. (2019). Japanese knotweed (Fallopia japonica): landscape invasive plant versus high quality honey source. Sci. Papers Ser. D, Anim. Sci. 62, 231–235.
Bordenstein, S. R., and Theis, K. R. (2015). Host biology in light of the microbiome: ten principles of holobionts and hologenomes. PLoS Biol. 13:e1002226. doi: 10.1371/journal.pbio.1002226
Cameron, S. A., Lozier, J. D., Strange, J. P., Koch, J. B., Cordes, N., Solter, L. F., et al. (2011). Patterns of widespread decline in North American bumble bees. Proc. Natl. Acad. Sci. USA 108, 662–667. doi: 10.1073/pnas.1014743108
Cameron, S. A., and Sadd, B. M. (2020). Global trends in bumble bee health. Annu. Rev. Entomol. 65, 209–232. doi: 10.1146/annurev-ento-011118-111847
Carrier, T. J., and Reitzel, A. M. (2017). The hologenome across environments and the implications of a host-associated microbial repertoire. Front. Microbiol. 8:802. doi: 10.3389/fmicb.2017.00802
Crutzen, P. J., and Stoermer, E. F. (2000). The ‘Anthropocene'. Int. Geosphere-Biosphere Program. (IGBP) Newslett 41, 17–18.
Cuesta-Maté, A., Renelies-Hamilton, J., Kryger, P., Jensen, A. B., Sinotte, V. M., and Poulsen, M. (2021). Resistance and vulnerability of honeybee (Apis mellifera) gut bacteria to commonly used pesticides. Front. Microbiol. 12, 1–16. doi: 10.3389/fmicb.2021.717990
Dellicour, S., Mardulyn, P., Hardy, O., Hardy, C., Roberts, S., and Vereecken, N. (2014). Inferring the mode of colonisation of a rapid range expansion from multi-locus DNA sequence variation. J. Evol. Biol. 27, 116–132. doi: 10.1111/jeb.12280
Dew, R., Silva, D., and Rehan, S. (2019). Range expansion of an already widespread bee under climate change. Glob. Ecol. Conserv. 17:e00584. doi: 10.1016/j.gecco.2019.e00584
Dharampal, P. S., Carlson, C., Currie, C. R., and Steffan, S. A. (2019). Pollen-borne microbes shape bee fitness. Proc. R. Soc. Lond. B Biol. Sci. 286:20182894. doi: 10.1098/rspb.2018.2894
Diaz, S., Settele, J., Brondízio, E., Ngo, H. T., Agard, J., Arneth, A., et al. (2019). Pervasive human-driven decline of life on Earth points to the need for transformative change. Science 366:6471. doi: 10.1126/science.aax3100
Dirzo, R., Young, H., Galetti, M., Ceballos, G., Isaac, N., and Collen, B. (2014). Defaunation in the anthropocene. Science 345, 401–406. doi: 10.1126/science.1251817
Dötterl, S., and Vereecken, N. (2010). The chemical ecology and evolution of bee–flower interactions: a review and perspectives. Can. J. Zool. 88, 668–697. doi: 10.1139/Z10-031
Drossart, M., Rasmont, P., Vanormelingen, P., Dufrene, M., Folschweiller, M., Pauly, A., et al. (2019). Belgian Red List of Bees. Université de Mons.
Duchenne, F., Thébault, E., Michez, D., Elias, M., Drake, M., Persson, M., et al. (2020a). Phenological shifts alter the seasonal structure of pollinators assemblages in Europe. Nat. Ecol. Evol. 4, 115–121. doi: 10.1038/s41559-019-1062-4
Dufrêne, M, and Legendre, P. (1997). Species assemblages and indicator species: the need for a flexible asymmetrical approach. Ecol. Monogr. 67, 345–366. doi: 10.2307/2963459
Engel, P., Kwong, W., Mcfrederick, Q., Anderson, K., Barribeau, S., Chandler, J., et al. (2016). The bee microbiome: Impact on bee health and model for evolution and ecology of host-microbe interactions. mBio 7, e02164–e02115. doi: 10.1128/mBio.02164-15
Gao, J. G., Liu, H., Wang, N., Yang, J., and Zhang, X.-L. (2020). Plant extinction excels plant speciation in the Anthropocene. BMC Plant Biol. 20:430. doi: 10.1186/s12870-020-02646-3
Gérard, M., Marshal, L., Martinet, B., and Michez, D. (2021). Impact of landscape fragmentation and climate change on body size variation of bumblebees during the last century. Ecography. 44, 255–264. doi: 10.1111/ecog.05310
Gérard, M., Martinet, B., Maebe, K., Marshall, L., Smagghe, G., Vereecken, N. J., et al. (2020b). Shift in size of bumblebee queens over the last century. Glob. Chang. Biol. 26, 1185–1195. doi: 10.1111/gcb.14890
Gérard, M., Vanderplanck, M., Wood, T., and Michez, D. (2020a). Global warming and plant-pollinator mismatches. Emerg. Top. Life Sci. 4, 77–86. doi: 10.1042/ETLS20190139
Ghisbain, G., Gérard, M., Wood, T. J., Hines, H. M., and Michez, D. (2021). Expanding insect pollinators in the Anthropocene. Biol. Rev. 96, 2755–2770. doi: 10.1111/brv.12777
Gibson, B., Wilson, D. J., Feil, E., and Eyre-Walker, A. (2018). The distribution of bacterial doubling times in the wild. Proc. R. Soc. Lond B Biol. Sci. 285:20180789. doi: 10.1098/rspb.2018.0789
Gulev, S. K., Thorne, P. W., Ahn, J., Dentener, F. J., Domingues, C. M., Gerland, S., et al. (2021). Chapter2: Changing State of the Climate System. In: Climate Change 2021: The Physical Science Basis. Contribution of Working Group I to the Sixth Assessment Report of the Intergovernmental Panel on Climate Change, (eds. Masson-Delmotte, V., Zhai, P., Pirani, A., Connors, S. L., Péan, C., Berger, S., et al. Cambridge University Press, UK. In Press. https://www.ipcc.ch/report/ar6/wg1/#FullReport. (accessed September 15, 2021).
Halsch, C. A., Shapiro, A. M., Fordyce, J. A., Nice, C. C., Thorne, J. H., Waetjen, D. P., et al. (2021). Insects and recent climate change. Proc. Natl. Acad. Sci. USA. 118:9. doi: 10.1073/pnas.2002543117
Hammer, T. J., Le, E., and Moran, N. A. (2021). Thermal niches of specialized gut symbionts: the case of social bees. Proc. R. Soc. Lond. B Biol. Sci. 288:20201480. doi: 10.1098/rspb.2020.1480
Jacobson, M., Tucker, E., Mathiasson, M., and Rehan, S. (2018). Decline of bumble bees in northeastern North America, with special focus on Bombus terricola. Biol. Conserv. 217, 437–445. doi: 10.1016/j.biocon.2017.11.026
Keller, A., McFrederick, Q. S., Dharampal, P., Steffan, S., Danforth, B. N., and Leonhardt, S. D. (2021). (More than) Hitchhikers through the network: the shared microbiome of bees and flowers. Curr. Opin. Insect. Sci. 44, 8–15. doi: 10.1016/j.cois.2020.09.007
Kwong, W. K., Engel, P., Koch, H., and Moran, N. A. (2014). Genomics and host specialization of honey bee and bumble bee gut symbionts. Proc. Natl. Acad. Sci. USA 111, 11509–11514. doi: 10.1073/pnas.1405838111
Lanner, J., Huchler, K., Pachinger, B., Sedivy, C., and Meimberg, H. (2020). Dispersal patterns of an introduced wild bee, Megachile sculpturalis Smith, 1853 (Hymenoptera: Megachilidae) in European alpine countries. PLoS ONE. 15:e0236042. doi: 10.1371/journal.pone.0236042
Maebe, K., De Baets, A., Vandamme, P., Vereecken, N., Michez, D., and Smagghe, G. (2021a). Intraspecies variation in thermal tolerance of bumblebees. J. Therm. Biol. 99:103002. doi: 10.1016/j.jtherbio.2021.103002
Maebe, K., Hart, A. F., Marshall, L., Vandamme, P., Vereecken, N. J., Michez, D., et al. (2021b). Bumblebee resilience to climate change, through plastic and adaptive responses. Glob. Change Biol. 27, 4223–4237. doi: 10.1111/gcb.15751
Maharramov, J., Meeus, I., Maebe, K., Arbetman, M., Morales, C., Graystock, P., et al. (2013). Genetic variability of the Neogregarine Apicystis bombi, an etiological agent of an emergent bumblebee disease. PLoS ONE. 8:e81475. doi: 10.1371/journal.pone.0081475
Martinet, B., Dellicour, S., Zambra, E., Przybyla, K., Lecocq, T., Boustani, M., et al. (2021a). Global effects of extreme temperatures on wild bumblebees. Conserv. Biol. 35, 1507–1518. doi: 10.1111/cobi.13685
Martinet, B., Zambra, E., Przybyla, K., Lecocq, T., Nonclercq, D., Rasmont, P., et al. (2021b). Mating under climate change: impact of simulated heatwaves on reproduction of model pollinators. Funct. Ecol. 35, 739–752. doi: 10.1111/1365-2435.13738
Michener, C. D. (2007). The Bees of the World. 2nd Edition, John Hopkins University Press, Baltimore, Maryland, USA.
Michez, D., Rasmont, P., Terzo, M., and Vereecken, N. (2019). Bees of Europe. NAP Editions, Paris, France.
Michez, D., Vanderplanck, M., and Engel, M. S. (2011). Chapter 5. Fossil Bees and Their Plant Associates. In: Evolution of Plant-Pollinator Relationships (Ed. Patiny, S.). Cambridge University press, Cambridge, UK. p. 103–164. doi: 10.1017/CBO9781139014113.006
Miller, D. L., Smith, E. A., and Newton, I. L. G. (2021). A bacterial symbiont protects honey bees from fungal disease. mBio. 12, e00503–e00521. doi: 10.1128/mBio.00503-21
Nieto, A., Roberts, S., Kemp, J., Rasmont, P., Kuhlmann, M., García Criado, M., et al. (2014). “European Red List of Bees,” International Union for Conservation of Nature. Luxembourg: Publication Office of the European Union. doi: 10.2779/77003
Ollerton, J., Winfree, R., and Tarrant, S. (2011). How many flowering plants are pollinated by animals? Oikos. 120, 321–326. doi: 10.1111/j.1600-0706.2010.18644.x
O'Malley, M. A. (2017). From endosymbiosis to holobionts: evaluating a conceptual legacy. J. Theor. Biol. 434, 34–41. doi: 10.1016/j.jtbi.2017.03.008
Otto, S. P. (2018). Adaptation, speciation and extinction in the Anthropocene. Proc. R. Soc. B. 285:20182047. doi: 10.1098/rspb.2018.2047
Parmentier, A., Billiet, A., Smagghe, G., Vandamme, P., Deforce, D., Van Nieuwerburgh, F., et al. (2018). A prokaryotic–eukaryotic relation in the fat body of Bombus terrestris. Environ. Microbiol. Rep. 10, 644–650. doi: 10.1111/1758-2229.12673
Praet, J., Parmentier, A., Schmid-Hempel, R., Meeus, I., Smagghe, G., and Vandamme, P. (2018). Large-scale cultivation of the bumblebee gut microbiota reveals an underestimated bacterial species diversity capable of pathogen inhibition. Environ. Microbiol. 20, 214–227. doi: 10.1111/1462-2920.13973
Rasmussen, C., Engel, M., and Vereecken, N. (2020). A primer of host-plant specialization in bees. Emerg. Topics. Life Sci. 4, 7–17. doi: 10.1042/ETLS20190118
Rockström, J., Steffen, W., Noone, K. J., Persson, Å., Chapin, F. S. III, Lambin, E. F., et al. (2009). A safe operating space for humanity. Nature 461, 472–475. doi: 10.1038/461472a
Roger, N., Moerman, R., Carvalheiro, L. G., Aguirre-Guitiérrez, J., Jacquemart, A.-L., Kleijn, D., et al. (2016). Impact of pollen resources drift on common bumblebees in NW Europe. Glob. Change Biol. 23, 68–76. doi: 10.1111/gcb.13373
Rothman, J. A., Leger, L., Graystock, P., Russell, K., and McFrederick, Q. S. (2019). The bumble bee microbiome increases survival of bees exposed to selenate toxicity. Environ. Microbiol. 21, 3417–3429. doi: 10.1111/1462-2920.14641
Santos, P. K. F., Arias, M. C., and Kapheim, K. M. (2019). Loss of developmental diapause as prerequisite for social evolution in bees. Biol. Lett. 15:20190398. doi: 10.1098/rsbl.2019.0398
Scheper, J., Reemer, M., van Kats, R., Ozinga, W. A., van der Linden, G. T. J., Schaminée, J. H. J., et al. (2014). Museum specimens reveal loss of pollen host plants as key factor driving wild bee decline in the Netherlands. Proc. Natl. Acad Sci. USA. 111, 17552–17557. doi: 10.1073/pnas.1412973111
Steffan, S. A., Dharampal, P. S., Danforth, B. N., Gaines-Day, H. R., Takizawa, Y., and Chikaraishi, Y. (2019). Omnivory in bees: elevated trophic positions among all major bee families. Am. Nat. 194, 414–421. doi: 10.1086/704281
Vanderplanck, M., Roger, N., Moerman, R., Ghisbain, G., Gérard, M., Popowski, D., et al. (2019). Bumble bee parasite prevalence but not genetic diversity impacted by the invasive plant Impatiens Glandulifera. Ecosphere 10:e02804. doi: 10.1002/ecs2.2804
Vaudo, A., Tooker, J., Grozinger, C., and Patch, H. (2015). Bee nutrition and floral resource restoration. Curr. Opin. Insect. Sci. 10, 133–141. doi: 10.1016/j.cois.2015.05.008
Voulgari-Kokota, A., Ankenbrand, M. J., Grimmer, G., Steffan-Dewenter, I., and Keller, A. (2019a). Linking pollen foraging of megachilid bees to their nest bacterial microbiota. Ecol Evol 9, 10788–10800. doi: 10.1002/ece3.5599
Voulgari-Kokota, A., Grimmer, G., Steffan-Dewenter, I., and Keller, A. (2019b). Bacterial community structure and succession in nests of two megachilid bee genera. FEMS Microbiol. Ecol. 95:fiy218 doi: 10.1093/femsec/fiy218
Wagner, D. L., Gramesa, E. M., Foristerb, M. L., Berenbaumc, M. R., and Stopakd, D. (2021). Insect decline in the Anthropocene: death by a thousand cuts. Proc. Natl. Acad. Sci. USA. 118:e2023989118. doi: 10.1073/pnas.2023989118
Westrich, P. (1996). Habitat requirements of central European bees and the problems of partial habitats, in “The Conservation of Bees” (eds., Matheson, A., Buchmann, S. L., O'Toole, C., Westrich, P., Williams, I. H.) Academic Press, London, U.K. pp. 1–16.
Keywords: holobiont, wild bees (Apidae), Anthropocene, ecosystem functioning, conservation
Citation: Maebe K, Vereecken NJ, Piot N, Reverté S, Cejas D, Michez D, Vandamme P and Smagghe G (2021) The Holobiont as a Key to the Adaptation and Conservation of Wild Bees in the Anthropocene. Front. Ecol. Evol. 9:781470. doi: 10.3389/fevo.2021.781470
Received: 22 September 2021; Accepted: 04 November 2021;
Published: 23 November 2021.
Edited by:
Chris Cutler, Dalhousie University, CanadaReviewed by:
Vera Lucia Imperatriz-Fonseca, University of São Paulo, BrazilCopyright © 2021 Maebe, Vereecken, Piot, Reverté, Cejas, Michez, Vandamme and Smagghe. This is an open-access article distributed under the terms of the Creative Commons Attribution License (CC BY). The use, distribution or reproduction in other forums is permitted, provided the original author(s) and the copyright owner(s) are credited and that the original publication in this journal is cited, in accordance with accepted academic practice. No use, distribution or reproduction is permitted which does not comply with these terms.
*Correspondence: Guy Smagghe, guy.smagghe@ugent.be