Alpine wetland degradation reduces carbon sequestration in the Zoige Plateau, China
- 1Wetland Research Center, Institute of Ecological Conservation and Restoration, Chinese Academy of Forestry, Beijing, China
- 2Beijing Key Laboratory of Wetland Services and Restoration, Beijing, China
- 3Sichuan Zoige Wetland Ecosystem Research Station, Tibetan Autonomous Prefecture of Aba, Sichuan, China
- 4University of Chinese Academy of Sciences, Beijing, China
- 5State Key Laboratory of Vegetation and Environmental Change, Institute of Botany, Chinese Academy of Sciences (CAS), Beijing, China
- 6College of Life Sciences, University of Chinese Academy of Sciences, Beijing, China
Alpine wetland plays an important role in the global carbon balance but are experiencing severe degradation under climate change and human activities. With the aim to clarify the effect of alpine wetland degradation on carbon fluxes (including net ecosystem CO2 exchange, NEE; ecosystem respiration, ER; gross ecosystem productivity, GEP, and CH4 flux), we investigated 12 sites and measured carbon fluxes using the static chamber method in the Zoige alpine wetland during August 2018, including undegraded wetland (UD), lightly degraded wetland (LD), moderately degraded wetland (MD), and severely degraded wetland (SD). The results showed that carbon sink strengths differ among the Zoige wetlands with different degradation stages during the growing season. From UD to LD, the rate of carbon sequestration (mean value of NEE) increased by 25.70%; however, from LD to SD, it decreased by 81.67%. Wetland degradation significantly reduced soil water content (SWC), soil organic carbon (SOC), microbial biomass carbon (MBC), and microbial biomass nitrogen (MBN). NEE was significantly correlated with MBC and MBN, while ER was positively correlated with ST but negatively correlated with SOC (P < 0.01). Among all measured environmental factors, GEP was positively correlated with pH (P < 0.01), while CH4 flux was most closely correlated with SOC, SWC, MBC, MBN, and ST (P < 0.001), and was also affected by pH and NO3– content (P < 0.01). These results suggest that the capacity of carbon sequestration in the Zoige wetlands reduced with intensification of the degradation. This study provides a reference for sustainably managing and utilizing degraded wetlands under climate change.
Introduction
Wetlands are one of the most important natural ecosystems on earth and are formed from the interaction between terrestrial and aquatic ecosystems (Li Y. et al., 2021). Wetlands account for only 5–8% of the land surface area but store 25–30% of soil organic carbon (SOC) in the terrestrial ecosystem (Mitsch and Gosselink, 2011). Wetlands are sensitive to climate change and human activities (Yan et al., 2022), and play pivotal roles in flood prevention, runoff regulation, pollution control, climate regulation, and sustaining ecosystems and global carbon balance (Spieles, 2022). Located on the Qinghai-Tibet Plateau, alpine wetland accumulates a large amount of herbaceous litter with a slow decomposition rate, resulting in higher soil carbon density than other ecosystems (Yarwood, 2018). Meanwhile, these alpine wetlands also contain large amounts of SOC (Wang et al., 2002). However, the increase in temperature, drainage, and overgrazing in recent years has led to the degradation of alpine wetland (Zhu et al., 2022), which have become alpine meadows (Li et al., 2018). Therefore, the carbon cycle changed with the degradation of the alpine wetland (Rui et al., 2011). Degradation changes the hydrothermal conditions in alpine wetland, which directly affects the complex physical, chemical, and biological factors of wetland soil, thereby changing the physiological characteristics of wetland plants. The contents of SOC, nitrogen, phosphorus, and water decrease with intensification of the alpine wetland’s degradation. The distribution of plant functional groups is more sensitive to variations in soil water content (SWC), total nitrogen (TN) content, and other factors. Vegetation cover and richness are also affected by the degradation of alpine wetland (Wang et al., 2015; Yuan et al., 2020), which leads to variations in root exudates and soil litter, and affects the carbon and nitrogen conversion rate by soil microorganisms.
Many studies have investigated the changes in carbon fluxes in alpine wetland during different seasons, and most studies have shown that alpine wetland are significant carbon sinks in summer (Zhao et al., 2005; Shi et al., 2006). However, flux observations in the Luanhaizi and Haibei alpine wetlands showed that alpine wetlands are CO2 sources (Zhao et al., 2010; Zhu et al., 2020). Moreover, atmospheric methane (CH4) also plays a key role in climate change and the carbon cycle. Natural CH4 emissions from wetlands are the largest single source in the global CH4 budget (Ringeval et al., 2010), whereas wetland CH4 emissions dominate the interannual variability in the global CH4 source (Walter et al., 2001; Bousquet et al., 2006). Some researchers have reported that degradation of the Zoige peatland due to drainage has greatly reduced CH4 emissions (Zhang et al., 2019). Degradation not only affects net ecosystem CO2 exchange (NEE) but also affects ecosystem respiration (ER) (Chen et al., 2013). Furthermore, previous studies have reported that gross ecosystem productivity (GEP) is significantly higher in slightly degraded than in severely degraded land (Peng et al., 2015). The rates of NEE are significantly lower in degenerated wetlands than in non-degraded wetlands (Xia et al., 2019). Xu et al. (2022) reported that extremely land degradation significantly decreases NEE and ER by 60.61% and 67.53%, respectively. However, another study showed that the average ER flux of degrading vegetation (218.04 ± 95.08 mg C m–2 h–1 in 2013 and 219.03 ± 140.96 mg C m–2 h–1 in 2014) is considerably larger than that of healthy vegetation (163.78 ± 87.51 mg C m–2 h–1 in 2013 and 143.91 ± 84.44 mg C m–2 h–1 in 2014) (Ma et al., 2020). Thus, degradation has a variable effect on carbon fluxes. Studies have shown that although degradation affects carbon sequestration in alpine wetland, the degree of the impact varies with the intensity of degradation (Pei et al., 2022). Therefore, it is important to explore the impact of degradation on carbon cycle variables (including CH4) in the Zoige alpine wetland along four different degradation gradients.
The Zoige alpine wetland are the largest plateau peat swamp in the world, located in the upper reaches of the Yellow River in the northeastern Qinghai-Tibet Plateau (Jiang et al., 2017). The unique climatic, geological and geographical conditions of the Zoige alpine wetland provide the environmental conditions for the survival of a large number of wetland wild animals and plants and make it one of the huge SOC pools in China (Huo et al., 2013). However, frequent extreme climate events and overgrazing since the 1970s have caused serious degradation of the Zoige alpine wetland (Qiu et al., 2009). Drainage and ditching have aggravated the degradation of the Zoige wetlands to varying degrees (Mei et al., 2012). Degradation leads to a reduction in the area of alpine wetland and a drop in water table, which in turn leads to accelerated peat decomposition and carbon release (Moorcroft et al., 2008), thereby exacerbating the global greenhouse effect (Rey et al., 2011). The aims of the present study were to (1) measure the variation of NEE, ER, GEP, and CH4 flux and determined the environmental factors along different wetland degradation gradients; (2) analyze the relationship between environmental factors and carbon fluxes; and (3) clarify the effect of alpine wetland degradation on carbon fluxes. This study will improve our understanding of the degradation impacts on alpine wetland, and provide data to support the theoretical basis for scientifically evaluating the system carbon budget of degraded alpine wetland.
Materials and methods
Study site
The Zoige wetlands (33° 55′ 42″ N, 102° 52′ 14″ E) are located in the northeastern Qinghai-Tibet Plateau with an average altitude of 3,600 m above sea level (Figure 1). The wetlands are in Zoige County, Aba Prefecture, Sichuan Province, and represent the largest plateau peatland in the world (Chen et al., 2009). The wetland area is 6,180 km2, accounting for 31.5% of the total area of the Zoige Plateau (Hao et al., 2011). Due to the high altitude, the air pressure is low at about 668.8 hPa. The region is in the humid climate of the plateau cold temperate zone, with a short spring and autumn, and annual average temperatures of about –1.7–3.3°C (Kang et al., 2018). The annual average rainfall is 650–750 mm, and the rainy season is warm and humid (May to September), whereas the dry season is cold and dry (October–April) (Liu et al., 2020a; Kang et al., 2022). The dominant species are Kobresia tibetica, Carex lasiocarpa, Stipa capillata, and other marsh plants (Liu et al., 2020b). The soil type in the study area was mainly meadow soil, and the vegetation type was mainly alpine meadow vegetation. The depth of the peat in the vertical profile of the Zoige alpine wetland is 2–5 m (Gao et al., 2011) with dry weight of 2.9 Pg and pH of 6.8–7.2 (Chen et al., 2014; Yan et al., 2021). Since the 1960s, the peatland has experienced artificial drainage and expanded pasture (Xiang et al., 2009), which has drained nearly 41% of the total area of the Zoige peatland and has changed the plant community, vegetation cover, and soil properties (Wu et al., 2017).
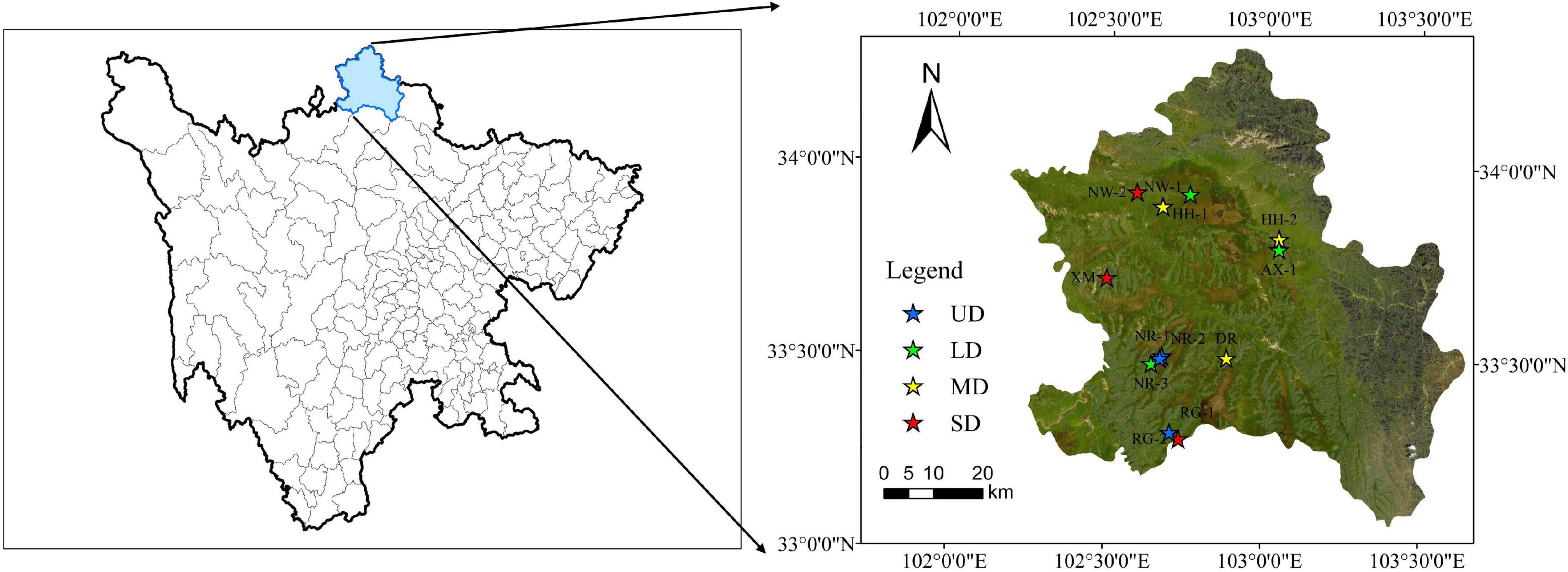
Figure 1. Locations of the sampling sites in the Zoige Plateau. The left panel is the location of Zoige in Sichuan Province, and the right panel is the location of the sampling point in Zoige. UD, undegraded wetland; LD, lightly degraded wetland; MD, moderately degraded wetland; SD, severely degraded wetland.
Experimental design
We investigated 12 sites including four degradation grades, i.e., undegraded wetlands (UD), lightly degraded wetlands (LD), moderately degraded wetlands (MD), and severely degraded wetlands (SD) in the Zoige Plateau during August 2018. Three sites were selected for each degradation gradient, and three 0.5 m × 0.5 m quadrats representing replicates were randomly selected within each site. We investigated the VC in the quadrats, collected soil samples, and measured CO2 and CH4 fluxes in situ during the growing season. The degradation gradients of the alpine wetland were determined according to the vegetation cover (VC) referred from the classification of alpine wetland degradation grade by Ma et al. (2002). The basic information of each degradation gradient is shown in Table 1.
Measurement of carbon fluxes
The carbon fluxes included NEE, ER, GEP, and CH4. The NEE, ER, and CH4 fluxes were measured using the static chamber method (Niu et al., 2010) carried out between 9:00 and 16:00 on a clear and cloudless day. Acrylic materials with light transmittance > 95% were used to make the cubic chamber (size = 0.5 m × 0.5 m × 0.5 m). Two fans were installed on the inner side of the chamber to ensure the uniform mixing of gases in the chamber, and the chamber had an air pipe connected with an LGR-ICOS groove portable greenhouse gas analyzer (UGGA-30p, LGR, United States) to measure the net exchange rate of the CO2 and CH4 at the different degraded sampling sites (Supplementary Figure 1). The base of the cubic chamber was an aluminum alloy bottom groove of 0.5 m × 0.5 m, which vertically hit the ground at 5 cm and maintained the base level. A water seal was used to ensure air tightness inside the cubic chamber during measurements. The cubic chamber was buckled on the bottom of an aluminum alloy tank, and counting began after the system stabilized. The instrument recorded the concentrations of CO2 and CH4 with the frequency of 1 Hz for 120 s continuously. According to the variations in the CO2 and CH4 concentrations during the measurement period (5–118 s), the change in the slopes of CO2 and CH4 with time was calculated, and the CO2 and CH4 fluxes in the cubic chamber per unit time were determined by combining the slope with air temperature, water pressure, and air pressure during the measurement period (Equation 1). The NEE (a positive value is CO2 emissions, a negative value is net CO2 uptake by the ecosystem, and the following methods for measuring ER and CH4 fluxes were the same) and CH4 was determined under the lighting conditions. The ER fluxes were measured with the cubic chamber covered with a dark opaque cloth. The NEE, ER, and CH4 fluxes were calculated from Equation 1. GEP was the difference between ER and NEE.
Where Fc is the gas flux (mg⋅m–2⋅h–1). where dc/dt is the slope of the linear regression for gas concentration gradient through time; where M and V0 are the molar mass of the gas (g mol–1) and the standard molar volume is 22.4 L mol–1, respectively; P and P0 are the measured atmospheric pressure and the standard atmospheric pressure, respectively; T and T0 (°C) represent the average value and the absolute temperature of the air temperature in the chamber, respectively; and H (cm) is the height of the static chamber.
Environmental factors
Soil temperature (ST) at a depth of 5 cm was measured in each sample plot with a portable digital thermometer (JM624, Jinming Institute Co., Tianjing, China), and SWC at a depth of 5 cm was determined with a TDR300 moisture meter (Spectrum Technologies, Inc., Aurora, IL, United States). Three soil samples at a depth of 5 cm were randomly collected from each sample plot and stored at 4°C until the soil properties were determined. The soil samples were passed through a 2 mm sieve to divide the soil into two parts. Ammonia (NH4+), nitrate (NO3–), microbial biomass carbon (MBC), microbial biomass nitrogen (MBN), and dissolved organic carbon (DOC) were determined in fresh soil samples. Soil pH, SOC, and TN were determined in air-dried samples. Soil pH was measured with a pH electrode in a slurry solution with a ratio of soil to water of 1:2.5 (PHS 29, China). SOC and TN were analyzed by a C/N analyzer (multi-N/C 3100, Analytik Jena AG, Jena, Germany). Soil NH4+ content was analyzed using the salicylic acid-sodium nitroprusside method with a spectrophotometer (PharmaSpec UV1700, Shimadzu, Tokyo, Japan), and soil NO3– was analyzed using a Technicon automated analyzer and by the cadmium reduction method with a 2 M KCl solution. Soil MBC and soil MBN were measured by the chloroform fumigation extraction technique with a fumigation time of 20 h and a 25 mM K2SO4 extract. Soil DOC was extracted by adding 50 mL of 0.5 M K2SO4 to 12.5 g of a homogenized subsample followed by stirring on a 120 rpm orbital shaker for 1 h. The filtrate was subjected to a total organic carbon analyzer (multi-55 N/C 3100, Analytik Jena). After digestion with perchloric acid, soil TN was determined by the Kjeldahl method.
Statistical analysis
The effects of the degradation gradient on the NEE, ER, GEP, and CH4 fluxes were tested by one-way analysis of variance. The LSD (least significant difference) post-test was applied to test for differences among the degradation gradients. Additionally, redundancy analysis (RDA) was used to explore the relationships between the different degradation gradients and environmental factors in the Zoige alpine wetland. The heatmap was prepared to show the relationships between the carbon fluxes and environmental factors. All analyses were conducted in R v4.2.0 software (R Core Team, 2020).
Results
Environmental factors
The environmental factors were classified into soil physical properties (including SWC, pH, and ST; Figures 2A–C), soil carbon content (including SOC, DOC, and MBC; Figures 2D–F), and soil nitrogen content (including TN, MBN, NH4+, and NO3–; Figures 2G–J). Degradation significantly altered SWC, ST, SOC, MBC, and MBN in the alpine wetland (Figure 2). SWC decreased with degradation severity, from 82.35% in UD to 26.59% in SD (Figure 2A). The pH at the UD sites was significantly lower than that at the other three degradation gradient sites (Figure 2B). In contrast, ST was significantly higher at the MD and SD sites than that at the UD and LD sites, with the highest value of 20.53°C in the SD and the lowest value of 16.16°C in the UD (Figure 2C). SOC, MBC, and MBN were in ranges of 49.43–348.33 g⋅kg–1, 314.53–513.10 mg⋅kg–1, and 52.20–84.49 mg⋅kg–1, respectively, and all decreased significantly with the degradation gradient (Figures 2D,F,H). SOC at the LD, MD, and SD sites decreased by 31.53%, 59.34%, and 85.81%, compared with that at the UD sites, respectively. The MBC and MBN decreased by 38.70% and 38.22%, respectively, from UD to SD with the degradation of the wetlands. DOC content was the highest in LD, with a value of 448.58 mg⋅kg–1, but it decreased significantly from LD to SD (Figure 2E). TN decreased from UD to MD and increased from MD to SD (Figure 2G), and ranged from 10.47 to 17.20 g⋅kg–1. NH4+content was not significantly different among the four degradation gradients (Figure 2I). NO3– content was lowest in UD, while it was much higher at the sites where degradation occurred (Figure 2J).
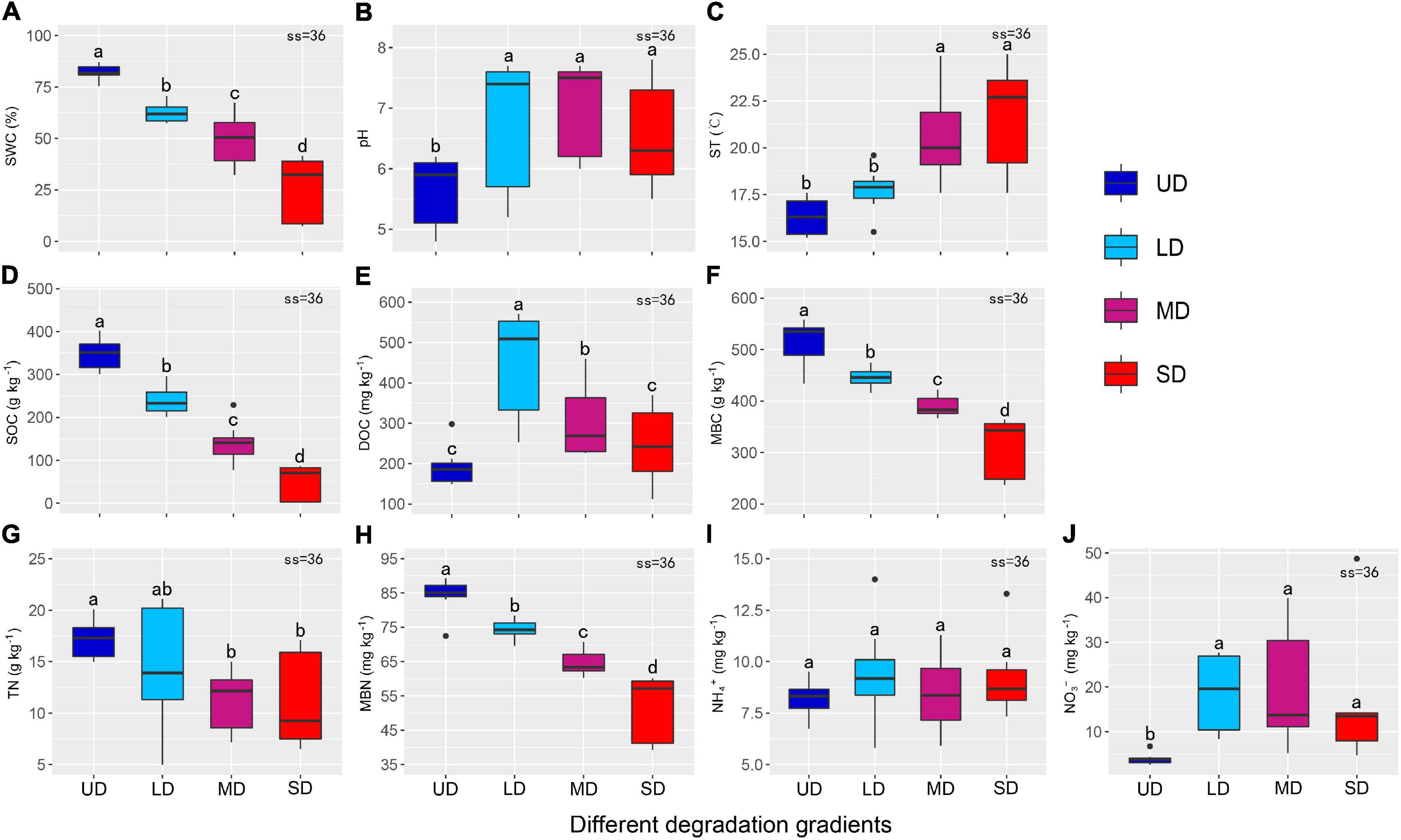
Figure 2. Environmental factors at the different degradation gradient sites. (A–J) Represent the trends of SWC, pH, ST, SOC, DOC, MBC, TN, MBN, NH4+, and NO3– content with degradation gradient, respectively. Different lowercase letters indicate significant differences at the P < 0.05 level (error bars indicate standard errors). UD, undegraded wetland; LD, lightly degraded wetland; MD, moderately degraded wetland; SD, severely degraded wetland; SWC, soil water content; ST, soil temperature at the 5 cm depth; SOC, soil organic carbon; MBN, microbial biomass nitrogen; MBC, microbial biomass carbon; DOC, dissolved organic carbon; NO3–, nitrate; NH4+, ammonia; TN, total nitrogen.
Variations in the ecosystem carbon fluxes
The NEE of the Zoige alpine wetland ranged from –216.31 to –1,180.24 mg CO2 m–2⋅h–1, with an average of –747.53 ± 102.24 mg CO2 m–2⋅h–1, indicating a carbon sink (Figure 3A). NEE weakened from LD to SD with increased wetland degradation. ER increased with degradation severity, the increase in alpine wetland degradation, ranging from 567.96 in UD to 1,524.60 mg CO2 m–2⋅h–1 in MD, with an average value of 1,067.90 ± 95.70 mg CO2 m–2⋅h–1. The ER values of LD, MD, and SD increased by 66.50%, 168.44%, and 117.17% compared with the UD, respectively (Figure 3B). GEP ranged from 1,449.73 to 2,125.86 mg CO2 m–2⋅h–1 and reaching the maximum value in LD, although the difference among the four degrees of degradation was not significant (Figure 3C). The alpine wetland acted as a strong CH4 source in UD (Figure 3D). The UD had a strong CH4 source (38.09 ± 5.02 mg CH4 m–2⋅h–1). However, CH4 flux at the LD, MD, and SD sites were only 0.37, 1.62, and –0.06 mg CH4 m–2⋅h–1, respectively, and no significant differences were observed among the LD, MD, or SD sites.
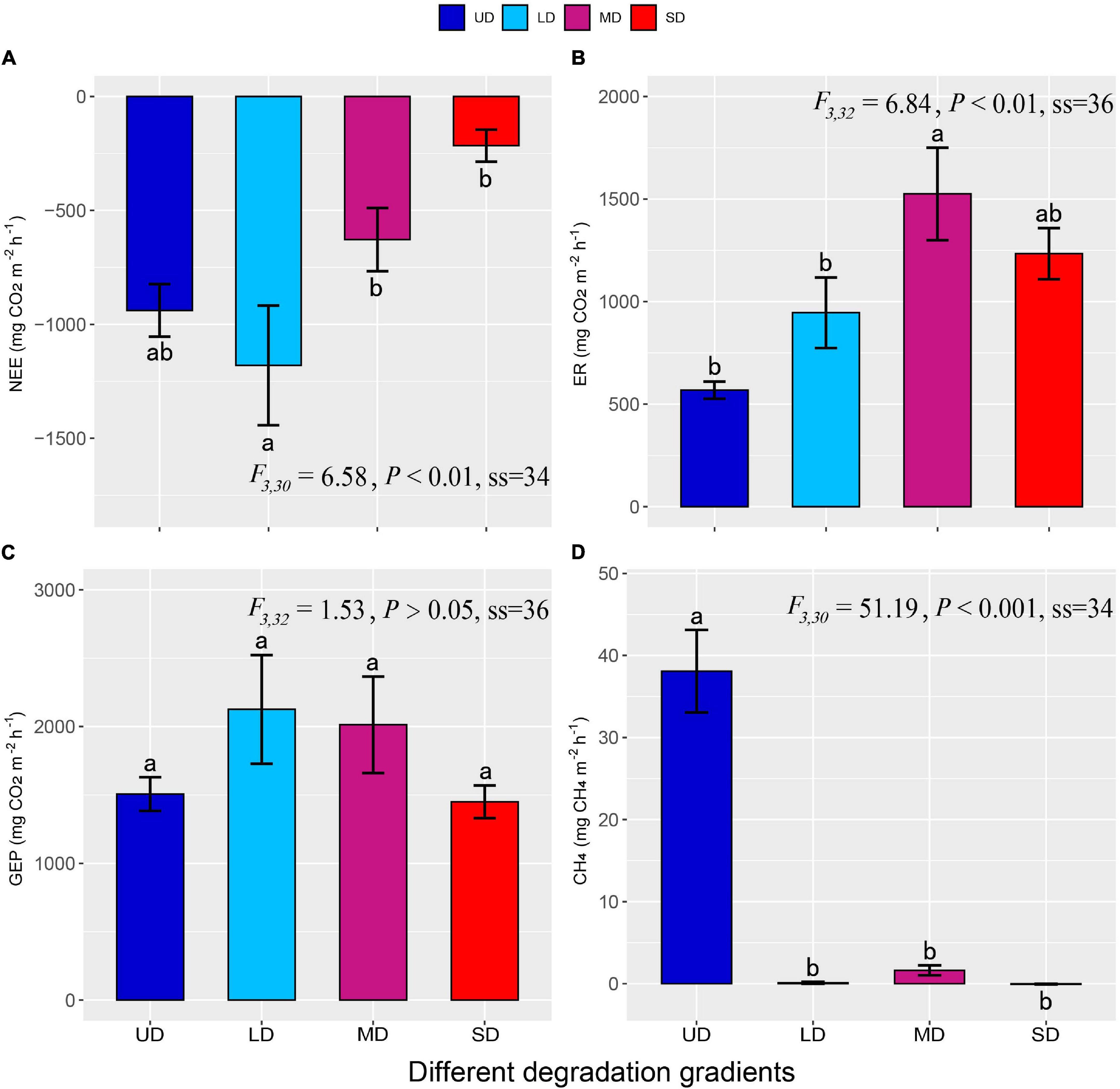
Figure 3. The NEE (A), ER (B), GEP (C), and CH4 (D) fluxes in the alpine wetlands degraded to different degrees. Different lowercase letters indicate a significant difference at the P < 0.05 level (error bars indicate the standard errors). UD, undegraded wetland; LD, lightly degraded wetland; MD, moderately degraded wetland; SD, severely degraded wetland; NEE, net ecosystem CO2 exchange; ER, ecosystem respiration; GEP, gross ecosystem productivity; CH4 flux, methane flux.
Correlations between the environmental variables and carbon fluxes
The heatmap shows that NEE was significantly correlated with MBC and MBN, respectively (Figure 4). ER was positively correlated with ST (r2 = 0.58), and negatively correlated with SOC (r2 = –0.57, P < 0.001). However, no correlation was detected between GEP and the measured environmental factors in this study, except pH (r2 = 0.47, P < 0.01). CH4 flux has significantly positive relationships with SOC, SWC, MBC, and MBN (P < 0.001). A negative correlation was observed between CH4 and ST with NO3– (P < 0.001). A negative correlation was detected between CH4 and ST and pH with correlation coefficients of –0.54 and –0.52, respectively. In addition to CH4, the carbon flux components of the Zoige alpine wetland under different degradation succession gradients sites were not correlated with TN, NH4+, or NO3– (P > 0.05, Figure 4).
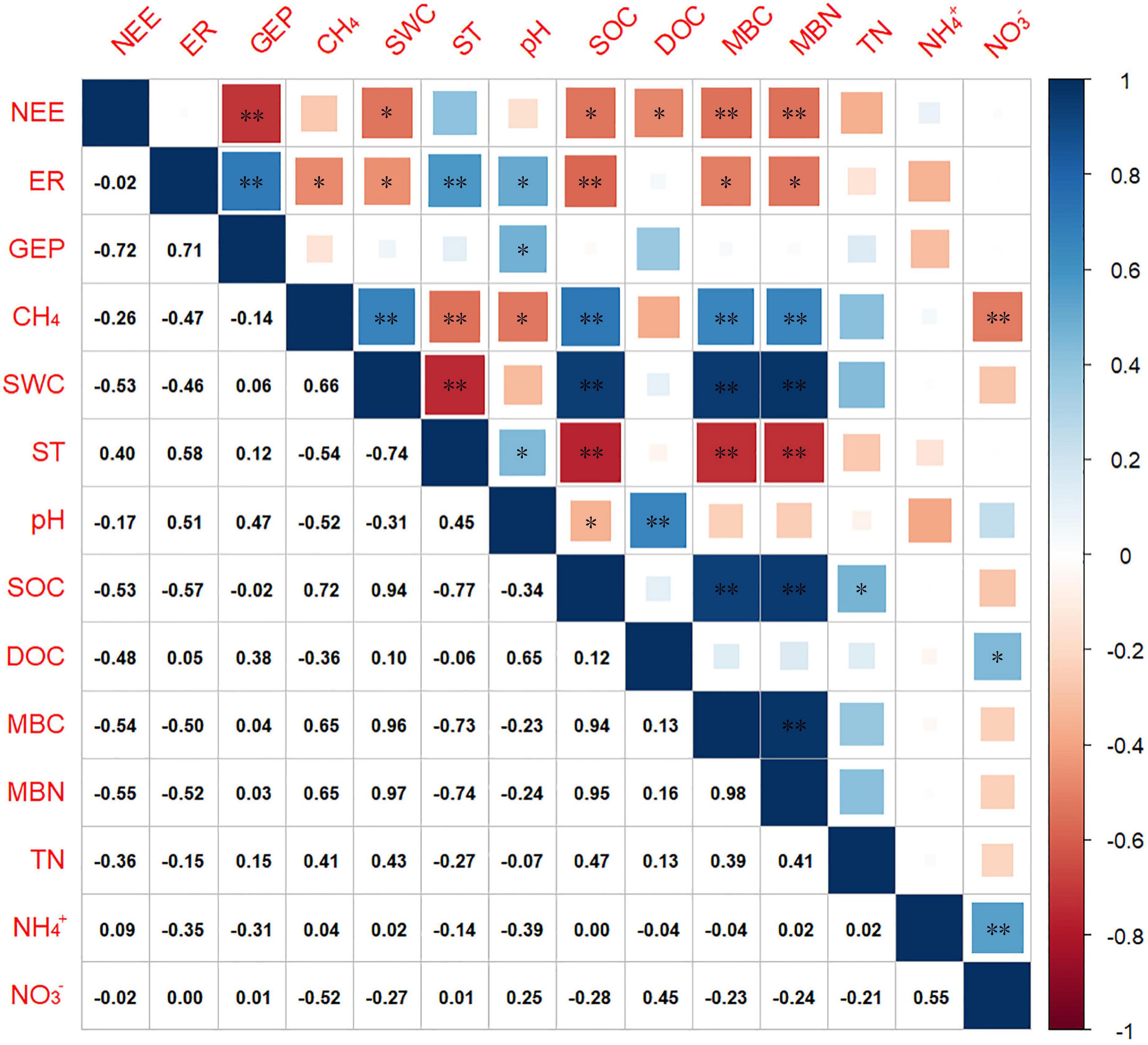
Figure 4. Heatmap of the correlations between NEE, ER, GEP, and CH4 and the environmental factors. A blue square indicates that the correlation coefficient is positive, and a red circle indicates that the correlation coefficient is negative. The correlation coefficient decreased as the color became lighter and the size became smaller. NEE, net ecosystem CO2 exchange; ER, ecosystem respiration; CH4 flux, methane flux; SWC, soil water content; ST, soil temperature at the 5 cm depth; SOC, soil organic carbon; DOC, dissolved organic carbon; MBC, microbial biomass carbon; MBN, microbial biomass nitrogen; TN, total nitrogen; NH4+, ammonia; NO3–, nitrate; “*” and “**” represent significant differences at the 0.01 and 0.001 levels, respectively.
The RDA results showed that the ten environmental factors explained 73.76% of the variation in the carbon fluxes (Figure 5A). SOC, MBN, MBC, and SWC were the main factors affecting the change in gas fluxes in the different degradation succession gradients, and they were highly correlated. The correlation coefficients between the four soil properties and the carbon fluxes under the different degradation succession gradients were 0.67, 0.67, 0.64, and 0.70, respectively (Figure 5B). ST and TN were correlated with the carbon fluxes of the four degradation gradients in the Zoige alpine wetland (P < 0.01).
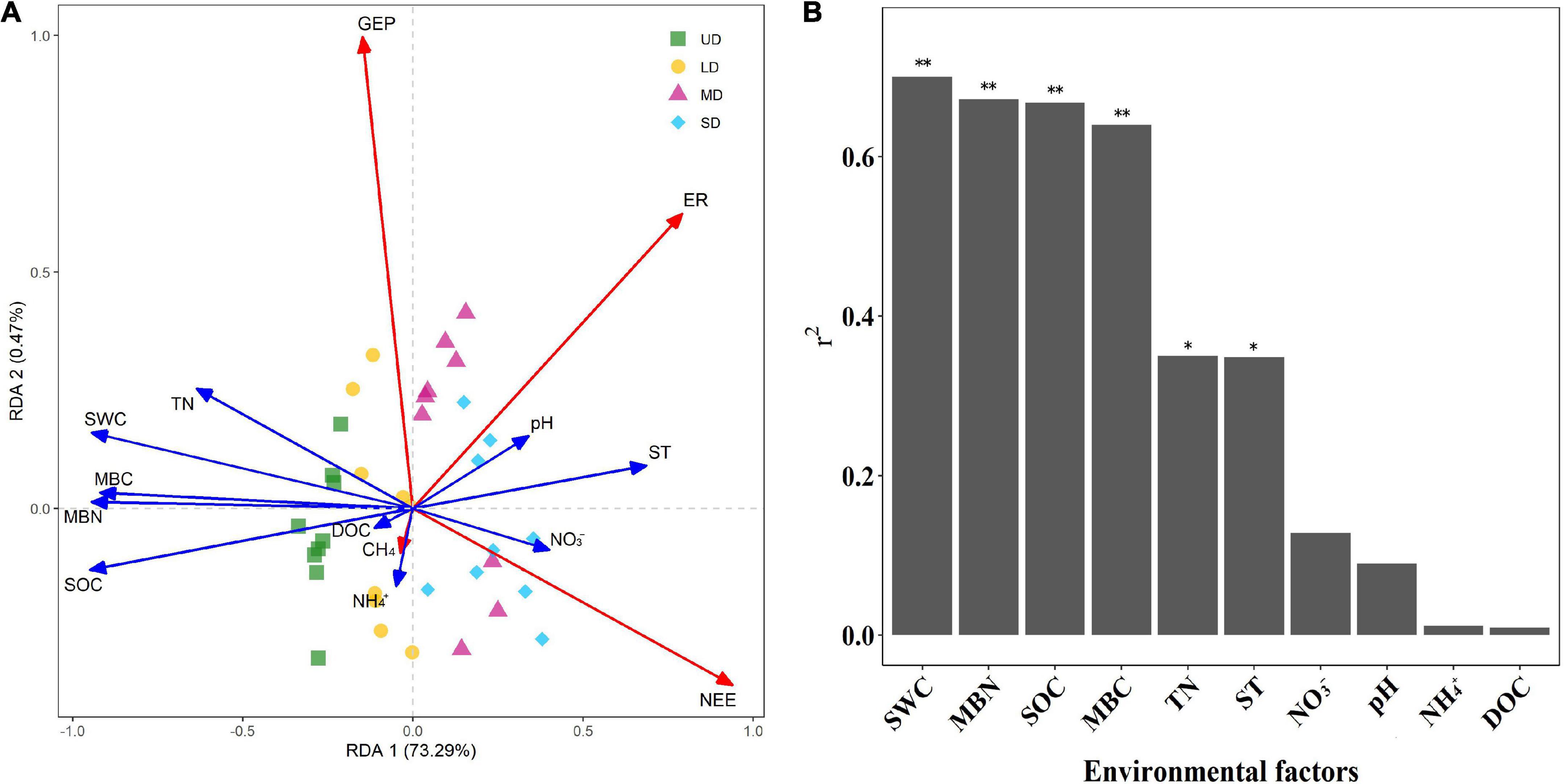
Figure 5. (A) Ordination plots of the redundancy analysis (RDA) for all plots and environmental factors across all sites and (B) a histogram of the explanatory rates of the different environmental factors for carbon fluxes. UD, undegraded wetland; LD, lightly degraded wetland; MD, moderately degraded wetland; SD, severely degraded wetland; SWC, soil water content; ST, soil temperature at a depth of 5 cm; SOC, soil organic carbon; DOC, dissolved organic carbon; MBC, microbial biomass carbon; MBN, microbial biomass nitrogen; TN, total nitrogen; NH4+, ammonia; NO3–, nitrate; “*” and “**” represent significant differences at P < 0.01 and P < 0.001, respectively.
Discussion
Effects of degradation on the environmental factors
The environmental factors were different under the different degradation gradients. As the degree of degradation intensified, the water table level in the Zoige alpine wetland decreased, and SWC decreased significantly (Figure 2A). The lack of water inhibits plant growth and affects nutrient absorption by the plants (Vargas et al., 2020). Therefore, the lower SWC resulted in inadequate growth conditions, which further degraded the alpine wetland. As an indicator of soil properties, SOC is a mixture of plant, animal, and microbial remains, excreta, secretions, their partial decomposition products, and soil humus (Piñeiro et al., 2010). Here, wetland degradation significantly reduced SOC content (Figure 2D). Some studies (Tang et al., 2010) have shown that simple vegetation composition and low diversity reduce the number of aboveground vegetation residues entering the soil; thus, resulting in lower SOC content. We found the same pattern for MBC and MBN (Figures 2F, H). Soil MBC and MBN contents increase in areas where plants grow well and decrease in areas where plants do not grow (Yan et al., 2012). When alpine wetland degrades to the severe degradation stage, the soil organic matter, MBC, and MBN are lost in large quantities.
Temperature is a key factor promoting the changes in root distribution and stock (Shen et al., 2016). The soil surface temperature of the Zoige alpine wetland increased gradually as degradation of the wetlands increased. Previous studies have shown that soil surface temperature, bulk density, and other properties increase with increasing alpine wetland degradation gradients (Li C. Y. et al., 2021). The reason may be that the high-altitude Zoige alpine wetland have stronger solar radiation, which makes the temperature of the soil with low water content increase rapidly, so the more severe the degradation, the higher the surface temperature (5 cm). Meanwhile, another reason for the increase of soil surface temperature may be the reduction of vegetation coverage caused by degradation, which increases the bare soil area and water loss through evaporation (Cui et al., 2005). Due to less vegetation cover and the “heat island” effect of bare land, the soil surface temperature (5 cm) increases with the degradation gradient (Cao et al., 2004).
Soil pH affects the absorption of soil nutrients by plants (Ma et al., 2017), and plant growth and physiological and biological characteristics affect soil pH in turn. In this study, soil pH did not change significantly with the degradation gradient. However, the pH values in the LD, MD, and SD degraded areas were higher than those in the UD area, indicating that wetland degradation reduces soil acidity. The more serious the degradation, the less the vegetation cover (Li C. Y. et al., 2021), and the less vegetation cover interferes with the soil pH (Li et al., 2008), so the soil becomes neutral. However, the specific effects of soil pH and the different degradation gradients in the Zoige alpine wetland remain unclear; thus, further research is necessary on the specific mechanism.
Effects of degradation on carbon fluxes
Significant differences in CO2 fluxes were observed in the Zoige alpine wetland with different degrees of degradation, except GEP (P > 0.05), and NEE and GEP reached their maximum values at the LD sites (Figure 3A). The water table level at the UD sites was high and flooded, which led to low coverage and low photosynthetic capacity. However, a drop in the water table level reduced the flooding stress for plants at the LD sites and increased biomass and photosynthetic capacity. Therefore, the NEE and GEP values at the LD sites were higher than those at the UD sites, suggesting that the carbon sequestration capacity in the wetlands improved under the non-flooded LD condition (Supplementary Figure 2). However, the NEE and GEP rates decreased gradually with the increase in the degradation gradient (Figures 3A,C). The rate of carbon sequestration increased by 25.70% from UD to LD but decreased by 81.67% from LD to SD. The reason was attributed to the factors affecting photosynthesis. The photosynthetic ability of an ecosystem is closely related to environmental factors, such as light intensity, soil moisture, air humidity, air temperature, and atmospheric CO2 concentration (Moore et al., 2006). Photosynthetic ability is also affected by the vegetation type, coverage, and leaf area index (Feng et al., 2008). When the alpine wetland is transformed into an extreme degradation stage, the plant community becomes smaller and sparse, the compositional structure changes, the coverage decreases, grass yield decreases, and the number of species and the amount of fine forage grass decrease (Zhou et al., 2005). The environmental conditions deteriorated rapidly, which made the photosynthetic rate of the community decrease rapidly, and the NEE and GEP also decreased. ER showed a trend of increasing first and then decreasing (Figure 3B) and was positively related to the degree of degradation. As the soil surface temperature of the degraded alpine wetland is high, the higher surface temperature led to an increase in microbial activity, which enhanced ER. As a result, ER was stronger and NEE was weaker and the more severe the degradation of the alpine wetland, the lower the GEP of its ecosystem.
CH4 flux is closely associated with soil moisture (Yu et al., 2013). The SWC at the UD sites was sufficient and the water table level was high; thus, the CH4 flux was very significant and the UD sites were an extremely important source of CH4. In contrast, at the LD, MD, and SD sites, the decline in the water table level resulted in a significant decrease in CH4 flux. Soil organic matter (crop straw of previous crop and photosynthetic products of the current season) is decomposed into methane by methanogenic bacteria (Conrad, 2007), and the high-water table level at the UD sites in this study created the conditions for generating methane. The Zoige alpine wetland exhibited a transformation from a CH4 source to a CH4 sink at the sites with a low MD to SD water table level, but the rate was extremely low. The CO2-eq net flux of CH4 was shown in Supplementary Figure 3. The Zoige alpine wetland had carbon absorption potential in the different degradation stages, and its carbon sink capacity varied with the degree of degradation (Figure 6), which was consistent with previous studies. Our study shows that the carbon sink function gradually weakened with the increase in the degradation gradient.
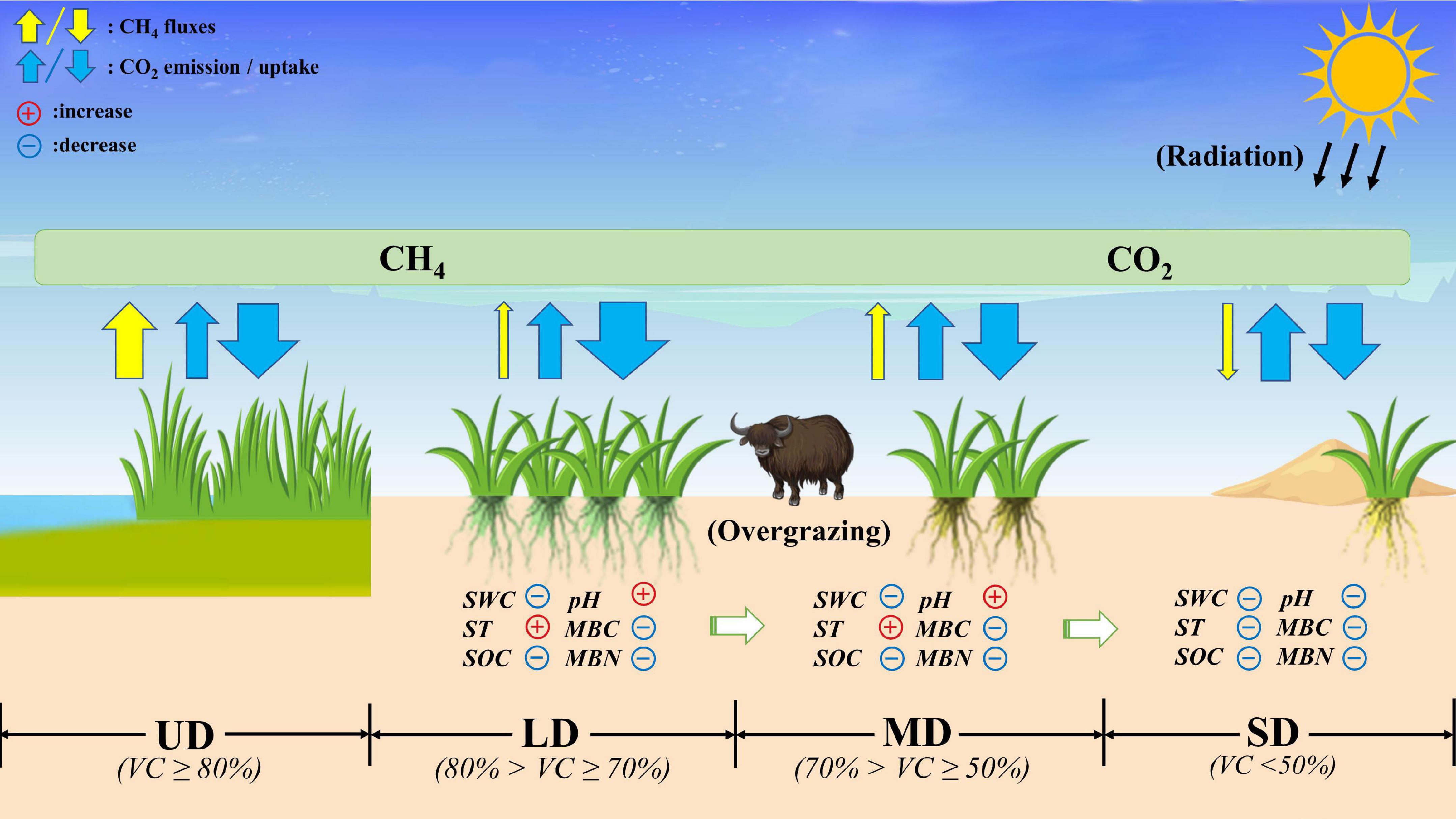
Figure 6. Carbon budget and environmental factors in the Zoige alpine wetlands under different degradation gradients. UD, undegraded wetland; LD, lightly degraded wetland; MD, moderately degraded wetland; SD, severely degraded wetland; SWC, soil water content; ST, soil temperature at the 5 cm depth; SOC, soil organic carbon; MBN, microbial biomass nitrogen; MBC, microbial biomass carbon; VC, vegetation cover.
Effects of the environmental factors on the carbon fluxes
Carbon fluxes in the Zoige alpine wetland were regulated by a variety of factors. Temperature was the primary factor controlling the ecosystem carbon fluxes (Figure 4). Previous studies have shown that temperature is one of the main factors affecting the respiratory rates of different soil use types (Hursh et al., 2016), as variations in temperature control carbon flux to a certain extent. Higher soil surface temperature stimulates the activity of microorganisms, enhances soil enzyme activities, and accelerates litter decomposition (Updegraff et al., 2001) so ST was the dominant ER controlling factor. Notably, NEE had a strong relationship with MBN, MBC, SOC, and SWC (Figure 4). MBC and MBN represent microbial activity, which was directly affected by soil fertility, water holding capacity, soil erosion resistance, and soil bulk density. The variations in MBC and MBN indirectly indicate the degree of wetland degradation, and they have an important effect on soil respiration and carbon balance in an alpine ecosystem. Therefore, MBC and MBN were strongly correlated with the carbon flux (Figure 4), and the carbon flux were high when MBC and MBN contents were high. Moreover, vegetation degradation and soil degradation are interrelated (Tang et al., 2015). One of the main reasons for vegetation degradation in the Zoige alpine wetland was the increase in grazing intensity and the increased rate of activities on the plateau (Liu et al., 2020b). Grazing decreases vegetation coverage and increases the amount of bare soil exposed to the air (Sun et al., 2011), which aggravates the weathering of surface soil. At the same time, a large number of rodents invaded the plateau. Soil quality continued to decline as the rodents gnawed on the roots of plants and increased the number of underground caves, which shifted the Zoige alpine wetland to a severe degree of degradation. Ecosystem respiration decreases when the vegetation becomes severely damaged. Therefore, the carbon exchange rate of the ecosystem was lowest at the SD sites (Figures 3A,D). Therefore, further research on the degradation of the Zoige alpine wetland vegetation caused by grazing and rodent activities should be conducted.
Emissions of the greenhouse gas CH4 account for 15% of the total greenhouse effect (Soebandiono et al., 2021). Here, our results show that CH4 emissions at the UD sites were much higher than those at the LD, MD, or SD sites, and CH4 flux was significantly correlated with SWC, ST, SOC, MBC, and MBN (Figure 4). Studies have shown that nitrates, sulfates, and others act as electron acceptors in wetland sediments, where they promote the anaerobic oxidation of CH4 and reduce CH4 emissions (Zhu et al., 2010). Moreover, soil microorganisms, such as methane-oxidizing bacteria, play an important role in inhibiting methane emissions. pH is an important environmental factor affecting microbial growth. Previous studies have reported that neutral and weak alkaline environments are more conducive to growing such microorganisms (Conrad, 2007). The pH of the soil in this study was 5.7–7.1, which was slightly acidic at the UD sites and was not beneficial for the survival of methane-oxidizing bacteria, suggesting why the CH4 flux at this site was higher than the other three sites.
However, carbon fluxes in alpine wetland are influenced by many factors, such as climate conditions, vegetation type, and soil conditions (Luo and Zhou, 2006). Single-factor analyses of some main soil physical and chemical factors in this study only explained part of the question. Therefore, other factors such as climate conditions and vegetation types, should be considered more in follow-up research, and their effects on carbon fluxes in the alpine wetland should be comprehensively analyzed by multiple factors.
Conclusion
In this study, we comprehensively investigated the carbon fluxes and environmental factors under 12 sites including four degradation grades in Zoige alpine wetland. Our finding indicated that the carbon fluxes of the Zoige alpine wetland during the growing season were closely related to the successional degradation gradients, and Zoige alpine wetland acted as a carbon sink under the four degradation gradients. Meanwhile, we found that degradation changed the environmental factors of alpine wetland, which weakened Zoige alpine wetland carbon sequestration capacity. Considering the complexity of the alpine wetland and the uncertainty of global climate change, further studies on the mechanisms of degradation on carbon sequestration in alpine wetland are needed in the future.
Data availability statement
The original contributions presented in this study are included in the article/Supplementary material, further inquiries can be directed to the corresponding author.
Author contributions
LY and XK conceived and designed the experiments. AY, XZ, XK, YL, ZY, ML, EK, XW, and YN conducted the experiments. AY analyzed the data. AY and XK wrote the manuscript. KZ, LY, XK, and YL revised the manuscript. All authors have read and agreed to the published version of the manuscript.
Funding
This study was supported by the Fundamental Research Funds of CAF (CAFYBB2019QB009), the National Natural Science Foundation of China (32171597, 32171598, and 42041005), the Second Tibetan Plateau Scientific Expedition and Research Program (STEP) (2019 QZKK0304).
Conflict of interest
The authors declare that the research was conducted in the absence of any commercial or financial relationships that could be construed as a potential conflict of interest.
Publisher’s note
All claims expressed in this article are solely those of the authors and do not necessarily represent those of their affiliated organizations, or those of the publisher, the editors and the reviewers. Any product that may be evaluated in this article, or claim that may be made by its manufacturer, is not guaranteed or endorsed by the publisher.
Supplementary material
The Supplementary Material for this article can be found online at: https://www.frontiersin.org/articles/10.3389/fevo.2022.980441/full#supplementary-material
References
Bousquet, P., Ciais, P., Miller, J. B., Dlugokencky, E. J., Hauglustaine, D. A., Prigent, C., et al. (2006). Contribution of anthropogenic and natural sources to atmospheric methane variability. Nature 443, 439–443. doi: 10.1038/nature05132
Cao, G. M., Tang, Y. H., Mo, W. H., Wang, Y. S., Li, Y. N., and Zhao, X. Q. (2004). Grazing intensity alters soil respiration in an alpine meadow on the Tibetan plateau. Soil Biol. Biochem. 36, 237–243. doi: 10.1016/j.soilbio.2003.09.010
Chen, H., Wu, N., Gao, Y. H., Wang, Y. F., Luo, P., and Tian, J. Q. (2009). Spatial variations on methane emissions from Zoige alpine wetlands of Southwest China. Sci. Total Environ. 407, 1097–1104. doi: 10.1016/j.scitotenv.2008.10.038
Chen, H., Yang, G., Peng, C. H., Zhang, Y., Zhu, D., Zhu, Q. Y., et al. (2014). The carbon stock of alpine peatlands on the Qinghai–Tibetan Plateau during the Holocene and their future fate. Quat. Sci. Rev. 95, 151–158. doi: 10.1016/j.quascirev.2014.05.003
Chen, W. W., Zheng, X. H., Chen, Q., Wolf, B., Butterbach-Bahl, K., Brüggemann, N., et al. (2013). Effects of increasing precipitation and nitrogen deposition on CH4 and N2O fluxes and ecosystem respiration in a degraded steppe in Inner Mongolia. China. Geoderma. 192, 335–340. doi: 10.1016/j.geoderma.2012.08.018
Conrad, R. (2007). Microbial ecology of methanogens and methanotrophs. Adv. Agron. 96, 1–63. doi: 10.1016/S0065-2113(07)96005-8
Cui, X., Wang, Y., Niu, H., Wu, J., Wang, S., Schnug, E., et al. (2005). Effect of long-term grazing on soil organic carbon content in semiarid steppes in Inner Mongolia. Ecol. Res. 20, 519–527. doi: 10.1007/s11284-005-0063-8
Feng, Y. L., Fu, G. L., and Zheng, Y. L. (2008). Specific leaf area relates to the differences in leaf construction cost, photosynthesis, nitrogen allocation, and use efficiencies between invasive and noninvasive alien congeners. Planta 228, 383–390. doi: 10.1007/s00425-008-0732-2
Gao, J. Q., OuYang, H., Lei, G. C., Xu, X. L., and Zhang, M. X. (2011). Effects of temperature, soil moisture, soil type and their interactions on soil carbon mineralization in Zoigê alpine wetland. Qinghai-Tibet Plateau. Chin. Geogr. Sci. 21, 27–35. doi: 10.1007/s11769-011-0439-3
Hao, Y. B., Cui, X. Y., and Kang, X. M. (2011). Predominance of precipitation and temperature controls on ecosystem CO2 exchange in Zoige alpine Wetlands of Southwest China. Wetlands 31, 413–422. doi: 10.1007/s13157-011-0151-1
Huo, L. L., Chen, Z. K., Zou, Y. C., Lu, X. G., Guo, J. W., and Tang, X. G. (2013). Effect of Zoige alpine wetland degradation on the density and fractions of soil organic carbon. Ecol. Eng. 51, 287–295. doi: 10.1016/j.ecoleng.2012.12.020
Hursh, A., Ballantyne, A., Cooper, L., Maneta, M., Kimball, J., and Watts, J. (2016). The sensitivity of soil respiration to soil temperature, moisture, and carbon supply at the global scale. Glob. Chang. Biol. 23, 2090–2103. doi: 10.1111/gcb.13489
Jiang, W., Lv, J., Wang, C., Chen, Z., and Liu, Y. (2017). Marsh wetland degradation risk assessment and change analysis: A case study in the Zoige Plateau. China. Ecol. Indic. 82, 316–326. doi: 10.1016/j.ecolind.2017.06.059
Kang, E. Z., Li, Y., Zhang, X. D., Yan, Z. Q., Zhang, W. T., Zhang, K. R., et al. (2022). Extreme drought decreases soil heterotrophic respiration but not methane flux by modifying the abundance of soil microbial functional groups in alpine peatland. Catena 212:106043. doi: 10.1016/j.catena.2022.106043
Kang, X. M., Yan, L., Cui, L. J., Zhang, X. D., Hao, Y. B., Wu, H. D., et al. (2018). Reduced carbon dioxide sink and methane source under extreme drought condition in an alpine peatland. Sustainability 10:4285. doi: 10.3390/su10114285
Li, C. Y., Peng, F., Xue, X., Lai, C. M., Zhang, W. J., You, Q. G., et al. (2021). Degradation stage effects on vegetation and soil properties interactions in alpine steppe. J. Mt. Sci. 18, 646–657. doi: 10.1007/s11629-020-6192-2
Li, C., Hao, X., Zhao, M., Han, G., and Willms, W. D. (2008). Influence of historic sheep grazing on vegetation and soil properties of a Desert Steppe in Inner Mongolia. Agr. Ecosyst. Environ. 128, 109–116. doi: 10.1016/j.agee.2008.05.008
Li, F., Liu, Z., Jia, T., Li, S., Bai, Y., Guo, C., et al. (2018). Functional diversity of soil microbial community carbon metabolism with the degradation and restoration of alpine wetlands and meadows. Acta Ecol. Sin. 38, 6006–6015. doi: 10.5846/stxb201706161096
Li, Y., Ma, J., Gao, C., Li, Y., Shen, X., Zhang, S., et al. (2021). Anaerobic ammonium oxidation (anammox) is the main microbial N loss pathway in alpine wetland soils of the Qinghai-Tibet Plateau. Sci. Total Environ. 787:147714. doi: 10.1016/j.scitotenv.2021.147714
Liu, M., Zhang, Z. C., Sun, J., Ming, X. U., BaiBing, M. A., Baba, T. S., et al. (2020a). The response of vegetation biomass to soil properties along degradation gradients of alpine meadow at Zoige Plateau. Chin. Geogr. Sci. 30, 446–455. doi: 10.1007/s11769-020-1116-1
Liu, M., Zhang, Z. C., Sun, J., Wang, Y., Wang, J. N., Tsunekawa, A., et al. (2020b). One-year grazing exclusion remarkably restores degraded alpine meadow at Zoige, eastern Tibetan Plateau. Glob. Ecol. Conserv. 22:e00951. doi: 10.1016/j.gecco.2020.e00951
Luo, Y. Q., and Zhou, X. H. (2006). Soil respiration and the environment. Amsterdam: Elsevier, 257–305. doi: 10.1016/b978-0-12-088782-8.x5000-1
Ma, M. J., Baskin, C. C., Yu, K. L., Ma, Z., and Du, G. Z. (2017). Wetland drying indirectly influences plant community and seed bank diversity through soil pH. Ecol. Indic. 80, 186–195. doi: 10.1016/j.ecolind.2017.05.027
Ma, W. W., Li, G., Wu, J. H., Xu, G. R., and Wu, J. Q. (2020). Respiration and CH4 fluxes in Tibetan peatlands are influenced by vegetation degradation. Catena 195:104789. doi: 10.1016/j.catena.2020.104789
Ma, Y. S., Lang, B. N., Li, Q. Y., Shi, J. J., and Dong, Q. M. (2002). Study on rehabilitating and rebuilding technologies for degenerated alpine meadow in the Changjiang and Yellow river source region. Pratacultural Sci. 19, 1–5. doi: 10.3969/j.issn.1001-0629.2002.09.001
Mei, L., Li, L., Liao, H., Huang, Z., and Huang, W. (2012). The influence of drainage on wetland degradation in Zoige Plateau. Dis. Adv. 5, 659–666.
Moorcroft, P. R., Wofsy, S. C., Dunn, A. L., and Ise, T. (2008). High sensitiviy of peat decomposition to climate change through water-table feedback. Nat. Geosci. 1, 763–766. doi: 10.1038/ngeo331
Moore, T. R., Lafleur, P. M., Poon, D. M., Heumann, B. W., Seaquist, J. W., and Roulet, N. T. (2006). Spring photosynthesis in a cool temperate bog. Glob. Chang. Biol. 12, 2323–2335. doi: 10.1111/j.1365-2486.2006.01247.x
Niu, S., Wu, M. Y., Han, Y. I., Xia, J., Zhe, Z., Yang, H., et al. (2010). Nitrogen effects on net ecosystem carbon exchange in a temperate steppe. Glob. Chang. Biol. 16, 144–155. doi: 10.1111/j.1365-2486.2009.01894.x
Pei, J. M., Yan, D., Li, J. Q., Qiong, L., Yang, Y. W., Fang, C. M., et al. (2022). Alpine meadow degradation enhances the temperature sensitivity of soil carbon decomposition on the Qinghai-Tibetan plateau. Appl. Soil Ecol. 170:104290. doi: 10.1016/j.apsoil.2021.104290
Peng, F., Quangang, Y., Xue, X., Guo, J., and Wang, T. (2015). Effects of rodent-induced land degradation on ecosystem carbon fluxes in an alpine meadow in the Qinghai–Tibet Plateau. China Solid Earth 6, 303–310. doi: 10.5194/se-6-303-2015
Piñeiro, G., Paruelo, J. M., Oesterheld, M., and Jobbágy, E. G. (2010). Pathways of Grazing Effects on Soil Organic Carbon and Nitrogen. Rangeland Ecol. Manag. 63, 109–119. doi: 10.2111/08-255.1
Qiu, P. F., Wu, N., Luo, P., Wang, Z. Y., and Li, M. H. (2009). Analysis of dynamics and driving factors of wetland landscape in Zoige. Eastern Qinghai-Tibetan Plateau. J. Mt. Sci. 6, 42–55. doi: 10.1007/s11629-009-0230-4
R Core Team (2020). A language and environment for statistical computing. Vienna, Austria: R Foundation for Statistical Computing.
Rey, A., Pegoraro, E., Oyonarte, C., Were, A., Escribano, P., and Raimundo, J. (2011). Impact of land degradation on soil respiration in a steppe (Stipa tenacissima L.) semi-arid ecosystem in the SE of Spain. Soil Biol. Biochem. 43, 393–403. doi: 10.1016/j.soilbio.2010.11.007
Ringeval, B., de Noblet-Ducoudré, N., Ciais, P., Bousquet, P., Prigent, C., Papa, F., et al. (2010). An attempt to quantify the impact of changes in wetland extent on methane emissions on the seasonal and interannual time scales. Glob. Biogeochem. Cy. 24. doi: 10.1029/2008gb003354
Rui, Y. C., Wang, S. P., Xu, Z. H., Wang, Y. F., Chen, C. R., Zhou, X. Q., et al. (2011). Warming and grazing affect soil labile carbon and nitrogen pools differently in an alpine meadow of the Qinghai–Tibet Plateau in China. J. Soil. Sediment. 11, 903. doi: 10.1007/s11368-011-0388-6
Shen, Z. X., Wang, J. W., Sun, W., Li, S. W., Fu, G., Zhang, X. Z., et al. (2016). The soil drying along the increase of warming masks the relation between temperature and soil respiration in an alpine meadow of Northern Tibet. Pol. J. Eco. 64, 125–129. doi: 10.3161/15052249pje2016.64.1.011
Shi, P. L., Sun, X. M., Xu, L. L., Zhang, X. Z., He, Y. T., Zhang, D. Q., et al. (2006). Net ecosystem CO2 exchange and controlling factors in a steppe—Kobresia meadow on the Tibetan Plateau. Sci. China Ser. D Earth Sci. 49, 207–218. doi: 10.1007/s11430-006-8207-4
Soebandiono, S., Muhibuddin, A., Purwanto, E., and Purnomo, D. (2021). The effect of indigenous organic fertilizer on paddy field methane emissions. Org. Agr. 11, 393–407. doi: 10.1007/s13165-020-00345-9
Spieles, D. J. (2022). Wetland construction, restoration, and integration: A comparative review. Land 11:554. doi: 10.3390/land11040554
Sun, D. S., Wesche, K., Chen, D. D., Zhang, G. L., Wu, G. Z., Du, N. B., et al. (2011). Grazing depresses soil carbon storage through changing plant biomass and composition in a Tibetan alpine meadow. Plant. Soil. Environ. 57, 271–278. doi: 10.17221/7/2011-pse
Tang, H. J., Qiu, J. J., Wang, L. G., Li, H., Li, C.-S., and Ranst, E. V. (2010). Modeling soil organic carbon storage and its dynamics in croplands of China. Agr. Sci. China 9, 704–712. doi: 10.1016/s1671-2927(09)60146-2
Tang, L., Dong, S. K., Sherman, R., Liu, S. L., Liu, Q. R., Wang, X. X., et al. (2015). Changes in vegetation composition and plant diversity with rangeland degradation in the alpine region of Qinghai-Tibet Plateau. Rangeland J. 37, 107–115. doi: 10.1071/rj14077
Updegraff, K., Bridgham, S. D., Pastor, J., Weishampel, P., and Harth, C. (2001). Response of CO2 and CH4 emissions from peatlands to warming and water table manipulation. Ecol. Appl. 11, 311–326. doi: 10.1111/gcb.12041
Vargas, A. I., Schaffer, B., and Sternberg, L. (2020). Plant water uptake from soil through a vapor pathway. Phys. Plant. 170, 433–439. doi: 10.1111/ppl.13173
Walter, B. P., Heimann, M., and Matthews, E. (2001). Modeling modern methane emissions from natural wetlands: 2. Interannual variations 1982–1993. J. Geophys. Res. 106, 34207–34219. doi: 10.1029/2001jd900164
Wang, G., Qian, J., Cheng, G., and Lai, Y. (2002). Soil organic carbon pool of grassland soils on the Qinghai-Tibetan Plateau and its global implication. Sci. Total Environ. 291, 207–217. doi: 10.1016/S0048-9697(01)01100-7
Wang, X. X., Sherman, R., Liu, Q. R., Liu, S. L., Li, Y. Y., and Wu, Y. (2015). A comparison of biodiversity–ecosystem function relationships in alpine grasslands across a degradation gradient on the Qinghai–Tibetan Plateau. Rangeland J. 37:45. doi: 10.1071/rj14081
Wu, X. W., Cao, R., Wei, X., Xi, X. Q., Shi, P. L., Eisenhauer, N., et al. (2017). Soil drainage facilitates earthworm invasion and subsequent carbon loss from peatland soil. J. Appl. Ecol. 54, 1291–1300. doi: 10.1111/1365-2664.12894
Xia, X., Jiang, H. L., Tian, X. Y., Guan, M. X., and Wang, L. F. (2019). Response of the plant and soil features to degradation grades in semi-arid grassland of the inner Mongolia. China. IOP Conf. Ser. Mater. Sci. Eng. 484:012039. doi: 10.1088/1757-899X/484/1/012039
Xiang, S., Guo, R. Q., Wu, N., and Sun, S. C. (2009). Current status and future prospects of Zoige Marsh in Eastern Qinghai-Tibet Plateau. Ecol. Eng. 35, 553–562. doi: 10.1016/j.ecoleng.2008.02.016
Xu, D., Mou, W., Wang, X., Zhang, R., Gao, T., Ai, D., et al. (2022). Consistent responses of ecosystem CO2 exchange to grassland degradation in alpine meadow of the Qinghai-Tibetan Plateau. Ecol. Indic. 141:109036. doi: 10.1016/j.ecolind.2022.109036
Yan, M. C., Xu, T. T., Song, P. H., and Dai, J. J. (2012). Effects of different cropping patterns of soybean and maize seedlings on soil enzyme activities and MBC and MBN. J. Northeast Agri. Univ. 19, 42–47. doi: 10.1016/s1006-8104(13)60049-5
Yan, W. C., Wang, Y. Y., Chaudhary, P., Ju, P. J., Zhu, Q. A., Kang, X. M., et al. (2022). Effects of climate change and human activities on net primary production of wetlands on the Zoige Plateau from 1990 to 2015. Glob. Ecol. Conserv. 35:e02052. doi: 10.1016/j.gecco.2022.e02052
Yan, Z. Q., Kang, E. Z., Zhang, K. R., Li, Y., Hao, Y. B., Wu, H. D., et al. (2021). Plant and soil enzyme activities regulate CO2 efflux in alpine peatlands after 5 years of simulated extreme drought. Front. Plant Sci. 12:756956. doi: 10.3389/fpls.2021.756956
Yarwood, S. A. (2018). The role of wetland microorganisms in plant-litter decomposition and soil organic matter formation: A critical review. FEMS Microbiol. Ecol. 94:fiy175. doi: 10.1093/femsec/fiy175
Yu, L., Tang, J., Zhang, R., Wu, Q., and Gong, M. (2013). Effects of biochar application on soil methane emission at different soil moisture levels. Biol. Fert. Soils 49, 119–128. doi: 10.1007/s00374-012-0703-4
Yuan, Z. Q., Wu, Q. B., Song, X., Jiang, X. J., Gao, S. R., Wang, Q. F., et al. (2020). Pasture degradation impact on soil carbon and nitrogen fractions of alpine meadow in a Tibetan permafrost region. J. Soil. Sediment. 20, 2330–2342. doi: 10.1007/s11368-020-02596-1
Zhang, H., Yao, Z., Ma, L., Zheng, X., Wang, R., Wang, K., et al. (2019). Annual methane emissions from degraded alpine wetlands in the eastern Tibetan Plateau. Sci. Total Environ. 657, 1323–1333. doi: 10.1016/j.scitotenv.2018.11.443
Zhao, L., Li, J., Xu, S., Zhou, H., Li, Y., Gu, S., et al. (2010). Seasonal variations in carbon dioxide exchange in an alpine wetland meadow on the Qinghai-Tibetan Plateau. Biogeosciences 7, 1207–1221. doi: 10.5194/bg-7-1207-2010
Zhao, L., Li, Y. N., Zhao, X. Q., Xu, S. X., Tang, Y. H., Yu, G. R., et al. (2005). Comparative study of the net exchange of CO2 in 3 types of vegetation ecosystems on the Qinghai-Tibetan Plateau. Chin. Sci. Bull. 50, 1767–1774. doi: 10.1360/04wd0316
Zhou, H., Zhao, X., Tang, Y., Song, G., and Li, Z. (2005). Alpine grassland degradation and its control in the source region of the Yangtze and Yellow Rivers, China. Grassl. Sci. 51, 191–203. doi: 10.1111/j.1744-697X.2005.00028.x
Zhu, G. B., Jetten, M. S. M., Kuschk, P., Ettwig, K. F., and Yin, C. Q. (2010). Potential roles of anaerobic ammonium and methane oxidation in the nitrogen cycle of wetland ecosystems. Appl. Microbiol. Biot. 86, 1043–1055. doi: 10.1007/s00253-010-2451-4
Zhu, J. B., Zhang, F. W., Li, H. Q., He, H., Li, Y. N., Yang, Y. S., et al. (2020). Seasonal and inter-annual variations of CO2 fluxes over 10 years in an alpine wetland on the Qinghai-Tibetan Plateau. Authorea doi: 10.22541/au.159714934.45073192
Keywords: degradation, carbon sequestration, CH4 flux, environmental factors, alpine wetland
Citation: Yang A, Kang X, Li Y, Zhang X, Zhang K, Kang E, Yan Z, Li M, Wang X, Niu Y and Yan L (2022) Alpine wetland degradation reduces carbon sequestration in the Zoige Plateau, China. Front. Ecol. Evol. 10:980441. doi: 10.3389/fevo.2022.980441
Received: 28 June 2022; Accepted: 26 July 2022;
Published: 16 August 2022.
Edited by:
Chuanyu Gao, Northeast Institute of Geography and Agroecology (CAS), ChinaReviewed by:
Xingliang Xu, Institute of Geographic Sciences and Natural Resources Research (CAS), ChinaJinshu Chi, Hong Kong University of Science and Technology, Hong Kong SAR, China
Copyright © 2022 Yang, Kang, Li, Zhang, Zhang, Kang, Yan, Li, Wang, Niu and Yan. This is an open-access article distributed under the terms of the Creative Commons Attribution License (CC BY). The use, distribution or reproduction in other forums is permitted, provided the original author(s) and the copyright owner(s) are credited and that the original publication in this journal is cited, in accordance with accepted academic practice. No use, distribution or reproduction is permitted which does not comply with these terms.
*Correspondence: Liang Yan, yanliang@caf.ac.cn
†These authors have contributed equally to this work and share first authorship