- 1Department of Rheumatology and Clinical Immunology, Peking Union Medical College Hospital, Chinese Academy of Medical Sciences & Peking Union Medical College, National Clinical Research Center for Dermatologic and Immunologic Diseases (NCRC-DID), Key Laboratory of Rheumatology and Clinical Immunology, Ministry of Education, Beijing, China
- 2People’s Hospital of Ningxiang City, Ningxiang, China
- 3Key Laboratory of Hunan Province for Integrated Traditional Chinese and Western Medicine on Prevention and Treatment of Cardio-Cerebral Diseases, Hunan University of Chinese Medicine, Changsha, China
- 4The First People's Hospital of Changde City, Changde, China
- 5Fudan University, Shanghai, China
Objective: To evaluate Safety and efficacy of probiotic supplementation in inflammatory arthritis.
Methods: The literature on the treatment of inflammatory arthritis with probiotics has been collected in databases such as CNKI, Pubmed, Cochrane library, Embase, etc. The search time is for them to build the database until May 2022. The included literatures are randomized controlled trials (RCTs) of probiotics in the treatment of hyperuricemia and gout. The Cochrane risk assessment tool was used for quality evaluation, and the Rev Man5.3 software was used for meta-analysis.
Results: A total of 37 records were finally included, involving 34 RCTs and 8 types of autoimmune disease (Hyperuricemia and gout, Inflammatory bowel disease arthritis, juvenile idiopathic arthritis [JIA], Osteoarthritis [OA], Osteoporosis and Osteopenia, Psoriasis, rheumatoid arthritis (RA), Spondyloarthritis). RA involved 10 RCTs (632 participants) whose results showed that probiotic intervention reduced CRP. Psoriasis involved 4 RCTs (214 participants) whose results showed that probiotic intervention could reduce PASI scores. Spondyloarthritis involved 2 RCTs (197 participants) whose results showed that probiotic intervention improved symptoms in patients. Osteoporosis and Ostepenia involving 10 RCTs (1156 participants) showed that probiotic intervention improved bone mineral density in patients. Hyperuricemia and gout involving 4 RCTs (294 participants) showed that probiotic intervention improved serum uric acid in patients. OA involving 1 RCTs (433 participants) showed that probiotic intervention improved symptoms in patients. JIA involving 2 RCTs (72 participants) showed that probiotic intervention improved symptoms in patients. Inflammatory bowel disease arthritis involving 1 RCTs (120 participants) showed that probiotic intervention improved symptoms in patients. All of the above RCTs showed that probiotics did not increase the incidence of adverse events.
Conclusion: Probiotic supplements may improve Hyperuricemia and gout, Inflammatory bowel disease arthritis, JIA, OA, Osteoporosis and Osteopenia, Psoriasis, RA, Spondyloarthritis. However, more randomized controlled trials are needed in the future to determine the efficacy and optimal dosing design of probiotics.
Systematic Review Registration: https://www.crd.york.ac.uk/prospero/display_record.php?ID=CRD42021286425, identifier CRD42021286425.
1 Introduction
Chronic inflammatory arthritis disease is a multifactorial disease characterized by autoantibody production and systemic features. The main pathological features of inflammatory arthritis are persistent synovitis and joint erosion, in which synovitis induces the pannus and joint destruction of arthritis (1). Chronic inflammatory arthritis, as one of the important clinical manifestations of rheumatic immune diseases, often leads to joint pain, limited mobility, and eventually joint deformity and disability (2, 3). The main clinical features of chronic inflammatory arthritis are chronic progression and recurrent attacks, which cause a huge burden of disease and pain in patients. There are many types of inflammatory arthritis, mainly include: (1) rheumatoid arthritis (RA) (4); (2) Gout (gout is characterized by a buildup of uric acid that forms crystals in the joints—especially in the big toe, and sometimes in the hands, wrists, or knees) (5); (3) psoriatic arthritis (about 30 percent of people with psoriasis (an autoimmune disease that causes scaly raised skin bumps) develop psoriatic arthritis, which affects the knees, ankles, wrists, or fingers) (6); (7) osteoarthritis (OA) (8); (4) ankylosing spondylitis (9); (6) arthritis associated with inflammatory bowel disease (10). Among them, epidemiological surveys show that globally, the prevalence of RA is estimated to be 0.24%. Currently, the total number of RA patients in the top 10 global drug markets exceeds 7 million, and this number will exceed 8.5 million by 2023 (11). Gouty arthritis caused by hyperuricemia has also increased rapidly, becoming one of the most common types of arthritis (12, 13). Current treatment drugs mainly include: (1) Non-steroidal anti-inflammatory drugs (NSAIDs) (which reduce the level of prostaglandins – chemicals that promote inflammation) (14). (2) Steroid hormones (which reduce inflammation and suppress the immune system) (15). (3) Anti-rheumatic drugs (DMARDs) (16). (4) Drugs that lower uric acid levels (gout) (17). (5) Other related nutritional supplements. However, the above-mentioned drug-related adverse reactions are common and cannot guarantee remission, and are prone to drug resistance over time leading to treatment failure (18–20). Therefore, there is a need for new related target therapeutic approaches for drug development and treatment of joint inflammation, thereby reducing the disease burden of inflammatory arthritis. A study showed that gut microbial dysbiosis (in combination with environmental triggers) may contribute to inflammatory immune disturbances in inflammatory arthritis in combination with genetically predisposed individuals (21). As shown in a study of a subset of patients with early RA, subclinical intestinal inflammation was present in almost all patients (22). Intestinal inflammation in these patients was characterized by increased numbers of infiltrating monocytes, T cells, B cells, and CD68+ macrophages, as well as the presence of lymphoid follicles. These histological findings suggest that a chronic inflammatory process develops in the gut of patients with early RA (23). Furthermore, in the presence of inflammation, alterations in gut barrier function and concomitant increases in gut permeability and bacterial translocation can promote inflammatory bowel disease and autoimmune responses in genetically susceptible hosts (24). Signs of altered intestinal permeability have been observed in RA patients, which may be related to the possible entry of inflammatory immune cells in the intestinal tissue into joints (24).
Probiotics are defined by the Food and Agriculture Organization of the United Nations (FAO) and the World Health Organization (WHO) as “ live microorganisms that, when administered in adequate amounts, confer a health benefit on the host” (25). Numerous in vivo and in vitro studies have shown that probiotics can exert immunomodulatory effects in several ways: (1) regulate intestinal inflammation and immune function; (2) prevent the increase in intestinal permeability and bacterial translocation that accompanies disruption of intestinal barrier function; (3) reduce the production of autoantibodies in the inflamed intestine; (4) reduce the entry of pro-inflammatory immune cells in the gut tissue into the joints (26). For example, the genera Lactobacillus and Bifidobacterium contain strains with anti-inflammatory properties and are important probiotics. It has been proven that some strains of L. casei can alleviate RA in rats by increasing the body’s anti-inflammatory cytokines (such as IL-10, TGF-β) and inhibiting pro-inflammatory cytokines (such as IL-1β, IL-2, IL-6, IL-12, IL-17) (27). Both L. plantarum LC27 and B. longum LC67 can inhibit the inflammatory response by inhibiting the nuclear factor kappa-B (NF-κB) inflammatory pathway (28). L. casei can promote the differentiation of CD4+ T cells into regulatory T cells (Treg) and inhibit their differentiation into Th17 cells, enhance the function of Treg cells, inhibit the function of Th17, and relieve inflammatory arthritis through immune regulation (29). A number of randomized controlled trials (RCTs) have also shown that probiotics can inhibit inflammatory factors, regulate immunity and improve pain scores in the intervention of arthritis. However, due to different types of probiotics and different types of arthritis, RCTs need to be summarized and summarized to clarify their clinical efficacy and safety. Therefore, this systematic review and meta-analysis summarizes and analyzes the RCTs of probiotics in the treatment of inflammatory arthritis so as to provide reference information for clinical application.
2 Materials and methods
2.1 Protocol
This systematic review and meta-analysis were conducted strictly in accordance with the protocol registered in PROSPERO (CRD42021286425) and PRISMA-guidelines (see Supplementary Material).
2.2 Search criteria
2.2.1 Inclusion criteria
(1) Study type: The included studies were RCTs, with no restrictions on random sequence generation methods, and no restrictions on language and publication time. (2) Participants: patients diagnosed with a type of inflammatory arthritis according to accepted criteria. (3) Intervention methods: The experimental group was an intervention containing a probiotic preparation (preparation type, dose, and type of probiotics are not limited). The control group was the intervention without the probiotic preparation. (4) Outcomes: Disease efficacy indicators, inflammatory indicators and adverse events.
2.2.2 Exclusion criteria
(1) Non-RCT; (2) Animal experiments, reviews, etc.; (3) Unable to view full text for data extraction; (4) The therapy of the control group included probiotics.
2.3 Search databases
Web of Science, China National Knowledge Infrastructure (CNKI), VIP Database for Chinese Technical Periodicals, Sinomed, Pubmed, Embase, Wanfang Database on Academic Institutions in China, Medline Complete, ClinicalTrials.gov and Cochrane Library were searched from the time of their establishment to May 15, 2022. The search strategy was shown in Table S1.
2.4 Search strategy, data extraction and quality assessments
Two researchers independently screened the literature and extract the data according to the established inclusion and exclusion criteria. The literature was initially screened by reading the title and abstract, and those that obviously did not meet the inclusion criteria were excluded. Subsequently, the remaining articles were read in full to determine their final inclusion. In case of disagreement, it was decided by discussion with all researchers.
Regarding literature quality assessment, the final included RCTs were assessed for randomization, allocation concealment, blinding, incompleteness of outcomes, selective reporting, and other risks using risk of bias assessment tools by 2 researchers (30). In case of disagreement, it was decided by discussion with all researchers.
2.5 Statistical analysis
This study used RevMan 5.3 software provided by the Cochrane Collaboration for statistical analysis (31). For continuous variables, mean difference (MD) was used as the pooled effect size. Heterogeneity test was performed for each analysis. If I2≥50%, P<0.1, indicating the existence of heterogeneity, the random-effects model was used for analysis; if it was homogeneous, the fixed-effects model was used for analysis.
3 Results
3.1 Literature search results
A total of 40 relevant studies were obtained in the initial examination, and after screening, 37 records were finally included (32–68), and 3 records were excluded for they are not RCTs (69–71). The literature screening process and results are shown in Figure 1.
3.2 Description of included trials
Three records (32–34) came from the same RCT and were therefore recorded as Alipour et al., 2014 (32–34). Two records (56, 57) came from the same RCT and were therefore recorded as Nilsson et al., 2018 (56, 57). The included RCTs involved 8 inflammatory arthritis (Hyperuricemia and gout, Inflammatory bowel disease arthritis, JIA, OA, Osteoporosis and Osteopenia, Psoriasis, RA, Spondyloarthritis) and were from 14 different countries (Iran, Finland, the U.S.A., Canada, Sweden, China, Brazil, Spanish, Ireland, New Zealand, the U.K., Denmark, Japan, India). The details of study characteristics are presented in Table 1.
3.3 Risk of bias assessments
The summary and graph of risk of bias ware shown in Figures 2, 3.
3.3.1 Sequence generation and allocation concealment
Twenty-three (23) RCTs (32–34, 36–42, 44, 47–54, 56, 57, 60, 63, 64, 66, 68) describe the random sequences generating method and were rated as low risk of bias, while other RCTs did not described the generating methods, and were rated as unclear risk of bias.
Twenty (20) RCTs (32–34, 36–40, 44, 45, 47–53, 56, 57, 59, 61, 62, 66) used allocation concealment (using identical-looking drugs, or allocation methods that were imperceptible to patients and physicians) and were therefore assessed as low risk of bias. Other RCTs did not describe whether allocation concealment was performed, the information was unclear, and therefore were assessed as unclear risk of bias.
3.3.2 Blinding
Twenty (20) RCTs (32–34, 36, 37, 39, 40, 45, 47–60, 66) described blinding of participants and personnel and blinding of outcome assessment, and were therefore assessed as low risk of bias. Other RCTs did not describe whether blinding was performed and were therefore assessed as unclear risk of bias.
3.3.3 Incomplete outcome data and selective reporting
Alipour et al., 2014 (32–34), Esmaeili et al., 2020 (40), Jenks et al., 2010 (48), Wang and Xu 2022 (64), Lei et al., 2017 (65), Shukla et al., 2016 (66) had incomplete outcome data and did not report the use of intent-to-treat, and were therefore assessed as having an unclear risk of bias. The other RCTs do not have incomplete outcome data. All RCTs reported outcomes assessed in the protocol and were therefore assessed as low risk of bias.
3.3.4 Other potential bias
Mandel et al., 2010 (36), Navarro-López et al., 2019 (44), Groeger et al., 2013 (45), Nilsson et al., 2018 (56, 57), Yamanaka et al., 2019 (61), Kamatani et al., 2018 (62) was funded by a pharmaceutical company and was therefore assessed to be at high risk of bias. Brophy et al., 2008 (49) conducted an Internet-based survey without face-to-face patient contact and were therefore assessed to be at high risk of bias. The others were rated as low risk of bias.
3.4 Probiotics for RA
Alipour et al., 2014 treated patients with L. casei 1*10^8 CFU and found improvements in CRP levels, tender and swollen joint counts, global health (GH) scores and DAS28 compared to placebo (P < 0.05). At the end of the study, more patients in the probiotic group had a moderate response to treatment (P < 0.05). Mandel et al., 2010 found a statistically significant improvement in joint pain and a reduction in CRP in patients receiving Bacillus coagulans GBI-30, 6086 2*10^8 CFU compared to placebo. Vadell et al., 2020 found no significant difference in DAS28-ESR compared with controls after administration of anti-inflammatory foods (including Lactobacillus plantarum 299v) (P = 0.116). Esmaeili et al., 2020 found that no significant differences in measured parameters were observed between the probiotic and placebo groups (P>0.05). Cannarella et al., 2021 found that probiotics improved white blood cell counts, TNF-α (P = 0.004) and IL-6 plasma levels (P < 0.05). However, no differences were observed in CRP, ESR, DAS28 between the two groups (P>0.05). Since data from the above RCTs could not be combined for meta-analysis, they are described only. The results of the meta-analysis are shown below.
3.4.1 Efficacy indicators
(1) DAS28: Four (4) RCTs reported the DAS28 data that can be meta-analyzed. The result of heterogeneity analysis was I2 = 97% and P<0.00001, which showed that there was statistical heterogeneity among the 4 studies, so the random effects model was used. The results of Meta analysis showed that the difference of DAS28 between experiment group and control group was of no statistical significance [WMD -0.55 (-1.33, 0.24), P=0.17] (Figure 4).
(2) Tender joint count: Four (4) RCTs reported the tender joint count data that can be meta-analyzed. The result of heterogeneity analysis was I2 = 75% and P=0.007, which showed that there was statistical heterogeneity among the 4 studies, so the random effects model was used. The results of Meta analysis showed that the difference between the experimental group and control group is of no statistical significance [SMD -0.34 (-0.94, 0.27), P=0.27] (Figure 5).
(3) Swollen joint count: Four (4) RCTs reported the swollen joint count data that can be meta-analyzed. The result of heterogeneity analysis was I2 = 69% and P=0.02, which showed that there was statistical heterogeneity among the 4 studies, so the random effects model was used. The results of Meta analysis showed that the difference between the experimental group and control group is of no statistical significance [SMD -0.10 (-0.64, 0.44), P=0.71] (Figure 6).
3.4.2 Inflammatory indicator
(1) ESR: Three (3) RCTs reported the ESR data that can be meta-analyzed. The result of heterogeneity analysis was I2 = 83% and P=0.003, which showed that there was statistical heterogeneity among the 3 studies, so the random effects model was used. The results of Meta analysis showed that the difference between the experimental group and control group is of no statistical significance [SMD -0.63 (-1.56, 0.31), P=0.19] (Figure 7).
(2) CRP: Five (5) RCTs reported the CRP data that can be meta-analyzed. Since The result of heterogeneity analysis was I2 = 95% and P<0.00001, which showed that there was statistical heterogeneity among the 5 studies, so the random effects model was used. The results of Meta analysis showed that there was a statistical difference between the experimental group and the control group (P=0.03), which indicates that curcumin may decrease CRP [SMD -1.57 (-2.98, -0.15)] (Figure 8).
3.4.3 Adverse events
Five RCTs (32–34, 36, 37, 41, 42) reported adverse events. No adverse events were observed in Alipour et al., 2014 (32–34), Mandel et al., 2010 (36), Pineda Mde et al., 2011 (37). Vadell et al., 2020 (41) observed 13 gastrointestinal adverse events in the intervention group and 4 gastrointestinal adverse events in the control group, mainly stomach pain, gas, diarrhea, heartburn and nausea. Gao et al., 2017 (42) found that 1 patient felt mild pain and discomfort in the lower abdomen after 3 days of oral treatment, and 1 patient had increased stool frequency; no serious adverse events were observed in any of the patients. In addition, five RCTs (35, 38–40, 43) did not report whether adverse events were observed, possibly because they did not monitor for adverse events, or did not observe adverse events indeed.
3.5 Probiotics for psoriasis
3.5.1 PASI score
Three RCTs reported PASI score. The results of Navarro-López et al., 2019 showed that the improvement of PASI in the probiotic group was better than that in the placebo group (P>0.05); however, because it did not provide specific values, it could not be integrated into the meta-analysis. The result of heterogeneity analysis was I2 = 0% and P=0.60, which showed that the heterogeneity among the 2 studies was low, so the fixed effects model was used. The summary results showed that the PASI score in probiotic group was lower than the control group (WMD -4.25 [-6.65, -1.85], P=0.0005; fixed effect model) (Figure 9).
3.5.2 CRP, TNF-α and IL-6
Two RCTs reported CRP and IL-6 levels. Both Groeger et al., 2013 and Moludi et al., 2021 found that CRP was reduced after the intervention of probiotics (P<0.05). However, for IL-6, Moludi et al., 2021 found that IL-16 was lower after the intervention of probiotics (P<0.05), while Groeger et al., 2013 showed that there was no significant difference between the probiotics intervention and the placebo group (P>0.05). Groeger et al., 2013 also reported TNF-α level and showed it was lower after the intervention of probiotics (P<0.05).
3.5.3 Adverse events
Two RCTs reported adverse events. Navarro-López et al., 2019 (44) showed a low incidence of adverse events, and no serious adverse events occurred in both groups. Moludi et al., 2021 (47) showed that 12% of patients in the placebo group and 8% of the patients in the probiotic group experienced gastrointestinal reactions, suggesting that patients tolerated probiotics well. In addition, two RCTs (45, 46) did not report whether adverse events were observed, possibly because they did not monitor for adverse events, or did not observe adverse events indeed.
3.6 Probiotics for spondyloarthritis
Only two RCTs reported the results of probiotics for Spondyloarthritis. Jenks et al., 2010 found no significant difference in mean BASFI and BASDAI after probiotic intervention compared with placebo (P>0.05). It also found adverse events in 14 patients in the probiotic group (12 in the placebo group), with no statistically significant difference, including include changes in bowel habits, nausea, vomiting, abdominal pain, and increased breathing. Brophy et al., 2008 used the Internet to recruit patients, send drugs to patients by post, and finally obtain patient feedback through the Internet. It found no significant differences in global health status, severity of intestinal symptoms or arthritis, and incidence of adverse events between the probiotic and control groups (P>0.05).
3.7 Probiotics for hyperuricemia and gout
3.7.1 Serum uric acid
Four RCTs (157 participants in experimental group and 137 participants in control group) reported uric acid. The heterogeneity test showed P=0.05, I2 = 58%, indicating high heterogeneity, and the random effects model was used for analysis. The summary result showed that uric acid in the probiotic group was lower than that in the control group (SMD -0.51 [-0.91, -0.10], P=0.01; random effect model) (Figure 10).
3.7.2 Adverse events
None of the four RCTs reported relevant adverse events, possibly because they were not observed or were not monitored. It is expected that future studies will report in detail the adverse events of probiotics intervention in hyperuricemia or gout.
3.8 Probiotics for osteoporosis and osteopenia
3.8.1 BMD
Seven (7) RCTs reported the absolute value of BMD, and 3 RCTs reported the percentage of BMD improvement. For the absolute value of BMD, the result of heterogeneity analysis showed that the heterogeneity was high in lumbar spine’s BMD (I2 = 71% and P=0.004) but low in total hip’s BMD (I2 = 24% and P=0.27), the random effects model was used. In lumbar spine subgroup, the difference between experimental group and control group was of no statistical significance (WMD 0.04 [-0.00, 0.09], P=0.07; random effect model). In total hip subgroup, the improvement of BMD in experimental group was higher (WMD 0.05 [0.02, 0.08], P=0.0005; random effect model) (Figure 3). The summary results also showed that the improvement of BMD in the experimental group was higher (WMD 0.04 [0.02, 0.07], P=0.001, random effect model) (Figure 11).
For the percentage of BMD improvement, the result of heterogeneity analysis showed that the heterogeneity was high in lumbar spine’s BMD (I2 = 94% and P<0.00001) and total hip’s BMD (I2 = 96% and P<0.00001), the random effects model was used. In lumbar spine subgroup, the improvement of BMD in experimental group was higher (SMD 1.16 [0.21, 2.12], P=0.02; random effect model) (Figure 3). In total hip subgroup, the difference between experimental group and control group was of no statistical significance (SMD 0.52 [-0.69, 1.73], P=0.40; random effect model). The summary results also showed that the difference of BMD between the two groups was of no statistical significance (SMD 0.84 [0.00, 1.68], P=0.05, random effect model) (Figure 12).
3.8.2 Adverse events
Five (5) RCTs reported the adverse events (52–54, 56, 57, 59). Lambert et al., 2017 (53) reported gastrointestinal adverse events in 2 patients in the probiotic group and 1 patient in the control group. Takimoto et al., 2018 (59) showed that no adverse events were observed. Nilsson et al., 2018 (56, 57) showed that there were 36 adverse events in the probiotic group and 39 in the control group, and there was no statistical difference between the two groups (P>0.05). Jansson et al., 2019 (52) showed that there were 30 adverse events in the probiotic group and 32 in the control group, and there was no statistical difference between the two (P>0.05), most of which were gastrointestinal reactions. Li et al., 2021 (54) showed that there were 5 cases of adverse events in the probiotic group and 7 cases in the control group, mainly nausea, vomiting, diarrhea and dizziness, and the difference was not statistically significant (P>0.05). In addition, five RCTs (50, 51, 55, 58, 60) did not report whether adverse events were observed, possibly because they did not monitor for adverse events, or did not observe adverse events indeed.
3.9 Probiotics for OA
Only one RCT reported probiotics for OA. Lei et al., 2017 treated 215 patients with L. casei Shirota and another 218 with placebo. They found that after 6 months of treatment, compared with the placebo group, patients in the probiotic group had significantly improved WOMAC and VAS scores, and decreased serum hs-CRP levels (P<0.05). They also claim that no serious adverse events were observed throughout the study.
3.10 Probiotics for JIA
Only two (2) RCTs reported probiotics for JIA. Shukla et al., 2016 found that the improvement of mJSpADA was not statistically different between the probiotic group and the control group (P>0.05). IL-10 levels were decreased in the probiotic group compared with the placebo group (P < 0.01). It also reported that there was no significant difference in adverse reactions between the probiotic group and the placebo group (11 vs 9, P>0.05), mostly diarrhea, abdominal pain, mild infection and flatulence. Malin et al., 1997 randomly assigned 30 adolescent patients to receive either L. rhamnosus GG or bovine colostrum or a lyophilized powder of bovine immunized colostrum for two weeks. They found that the probiotic group increased the number of immune cells secreting IgA and IgM (P<0.05). Fecal urease activity associated with mucosal tissue damage was decreased in the probiotic group, while increased in the control group (P<0.05). They suggest that oral administration of L. rhamnosus GG has the potential to strengthen the mucosal barrier mechanism of juvenile chronic arthritis. In addition, those two RCTs (66, 67) did not report whether adverse events were observed, possibly because they did not monitor for adverse events, or did not observe adverse events indeed.
3.11 Probiotics for inflammatory bowel disease arthritis
Only Zhang et al., 2020 (68) reported probiotics for inflammatory bowel disease arthritis. They treated 120 patients with probiotic therapy, narrative medical education therapy, probiotics combined with narrative medical education therapy, and primary care therapy. They found that the patients in the probiotics combined with narrative medical education group had the longest total sleep time, the shortest sleep latency, and the highest sleep efficiency, suggesting that this therapy could help improve the sleep quality of patients. In addition, Zhang et al., 2020 (68) did not report whether adverse events were observed, possibly because they did not monitor for adverse events, or did not observe adverse events indeed.
4 Discussion
There is increasing evidence that non-human genetic factors, especially the human microbiota, may contribute to the development of inflammatory arthritis (e.g., RA and spondyloarthritis) in genetically susceptible individuals (72–74). There has been an increasing number of studies investigating the gut community as a determinant of the pathogenesis of inflammatory arthritis. Evidence from multiple epidemiological and translational studies suggests that interactions between mucosal sites and dysregulated microbiota may contribute to the development of inflammatory arthritis (75–78). Studies have shown that in most inflammatory arthritis in the preclinical stage of arthritis, there is already a change in the microbial microbiota of the individual patient. This suggests that gut dysbiosis plays an important role in the development of inflammatory arthritis and systemic inflammation throughout the chronic course (79–86). Therefore, the current hypothesis model is mainly that the inflammatory “gut-arthritis axis” is the pathogenic pathway of inflammatory arthritis. The gut is composed of the most innate and adaptive immune cells in the human body, so it is generally considered to be the largest immune organ in the human body (87). The possible complex interplay between gut microbiota disturbances and genetic factors (the immune system susceptible to autoimmunity) may provide the basis for the development of pathological processes such as systemic inflammation in inflammatory arthritis (88–90). Basic and clinical studies suggest that these alterations in gut microbiota disturbances may occur before disease onset, and to some extent represent hidden triggers of systemic inflammation (24, 91). After initiation of the initiating factors, the links between barrier dysfunction, intestinal inflammation and arthritis reciprocate to form the “gut-arthritis” axis. Among them, dysbiosis may lead to subclinical intestinal inflammation in patients and promote the abnormal activation of specific innate and adaptive immune response pathways. Mucosal lesions due to inflammation exhibit reduced intestinal barrier function, and abnormally activated immune cells are also transported from intestinal sites to secondary lymphoid organs and recirculation of arthritic joints (92, 93) (Figure 13). Therefore, therapies targeting the entero-arthritis axis have emerged as new therapeutic measures for inflammatory arthritis.
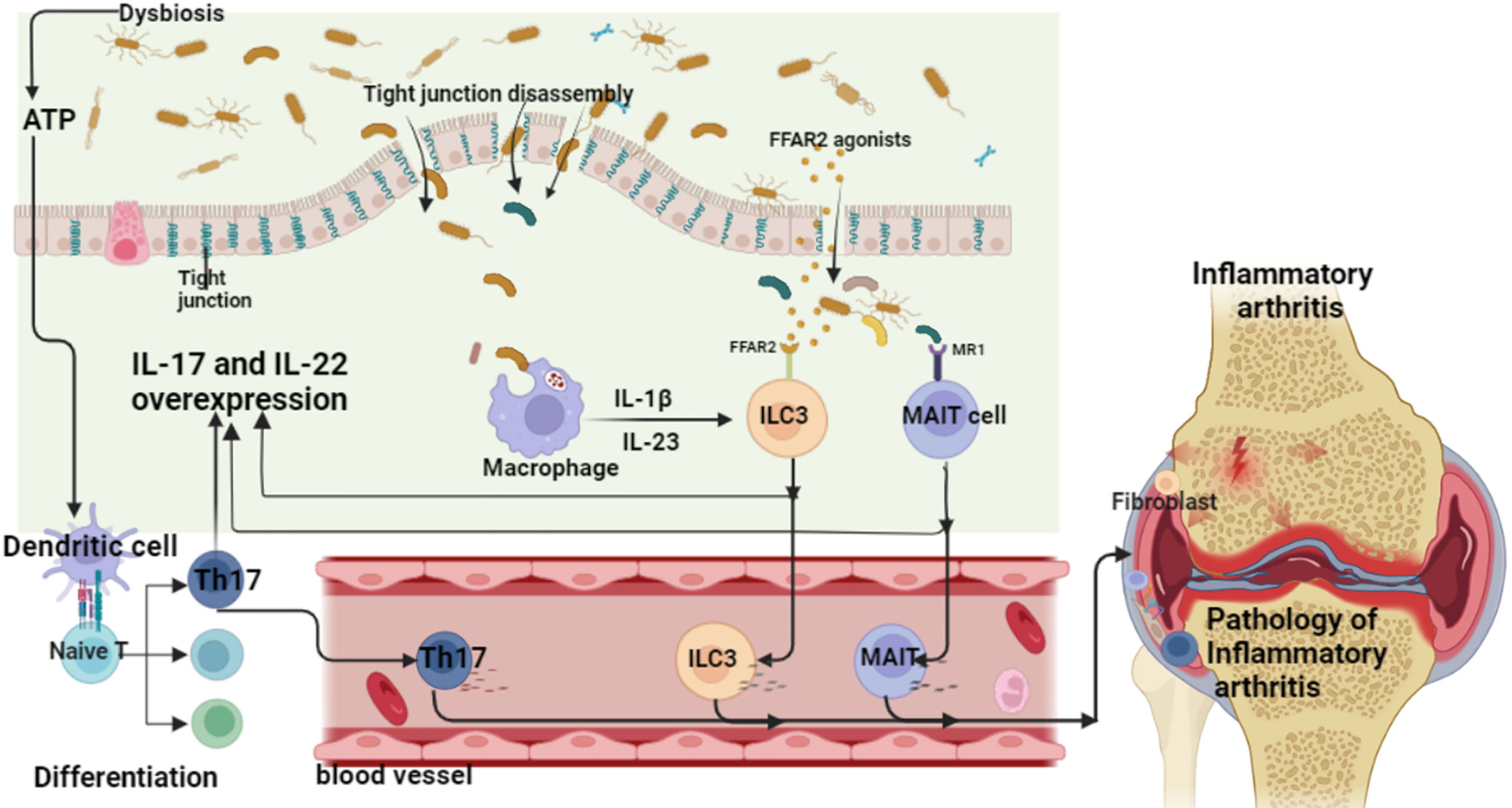
Figure 13 Inflammatory arthritis - the pathogenic mechanism of the “gut-arthritis” axis (ATP, adenosine triphosphate; IL, interleukin; ILC, innate lymphoid cells; MAIT cell, mucosal associated invariant T; Th17, T helper cell 17).
At present, probiotics are live microorganisms that are beneficial to the human body and are of great significance to maintain the intestinal microecological balance. Several clinical studies have shown that probiotics and their metabolites or probiotic fermented foods have received great attention in improving inflammatory arthritis. Among them, Bifidobacterium (such as B. longum, B. breve, B.infantis) and Lactobacillus (such as L. helveticus, L. rhamnosus, L. plantarum and L.casei) are the most widely used probiotics (94). According to the WHO, probiotics, as active microorganisms, can have beneficial effects on the body if taken in appropriate doses (75). Probiotics can restore the balance of microbiota in the gut in various ways, including regulating gut immunity or competing with other gut microbes for nutrients, resulting in a competitive inhibitory effect (76). More and more researchers have found that probiotics can regulate the immune system of humans or animals (77, 78). A systematic review and meta-analysis of chronic intestinal inflammatory diseases showed that in 8 case-control studies and 1 randomized controlled study, more than 45% of patients achieved clinical remission after probiotic intervention (79). Some researchers have also discovered the therapeutic effect of probiotics on inflammatory arthritis. Therefore, we conducted a comprehensive and extensive systematic review and meta-analysis on the efficacy and safety of probiotics on the mechanism of inflammatory arthritis.
4.1 Probiotics for RA
RA is a chronic systemic autoimmune disease characterized by joint lesions, in which autoantibodies are mainly represented by rheumatoid factor and anti-cyclic citrullinated peptide antibody (ACPA) (95). It is characterized by joint swelling and pain, followed by deformity and disability (96), and is accompanied by damage to internal organs, such as lungs, heart, and kidneys. Epidemiological surveys show that the prevalence of RA is 0.3% and 1%, and it is one of the most common autoimmune diseases (97). Current research shows that RA is caused by the combined action of genetic factors and environmental factors. Among environmental factors, immune abnormalities caused by immune cell imbalance have been confirmed to be involved in the occurrence and development of RA (98). These include T, B lymphocytes, macrophages, neutrophils, etc., and imbalances in the proportion of Th1, Th2 and Th17 cells and immune damage caused by cytokines IL-1, IL-2, IL-17, IFN-γ and TNF-α (99). It is a key factor leading to chronic inflammation in RA. In particular, adaptive immunity dominated by CD4+ T cells plays an important role in initiating and maintaining the characteristics of the autoimmune response in rheumatoid arthritis. Among them, Th1 and Th17 cells in CD4+ T cells are important drivers of RA, which activate macrophages and recruit other inflammatory cells to inhibit Treg-mediated immune tolerance (100).
The current study showed significant differences in the fecal microbiota of RA patients compared with healthy subjects. The levels of Bifidobacterium, Bacteroides, Lactobacillus and other probiotics in the feces of RA patients were significantly decreased, while the levels of Escherichia coli and Enterococcus were significantly increased (38, 101, 102). These gut microbiota are involved in the innate immune response pathway and the immune abnormalities of the acquired immune response pathway in the pathogenesis of RA (103). The study found that gavage of Lactobacillus could correct the imbalance of intestinal bacteria in mice with collagen-induced arthritis (CIA), and at the same time reduce the expression of cytokines IL-12, IFN-γ, TGF-β and IL-6 that induce Th1 and Th17 cell differentiation. That is, Lactobacillus can alleviate mouse RA by regulating CD4+ T subset-related cytokines (104). Another study (105) also found that the B cells and Tfh cells associated with antibody production in the inguinal lymph nodes were significantly reduced in CIA mice fed L. helveticus SBT2171. In terms of Treg cells regulating immunity, the alleviating effect of probiotics on CIA rats was related to the time of intervention. Bifidobacterium preventive intervention is more likely to improve the intestinal microecology, increase the concentration of short-chain fatty acids, increase the frequency of Treg cells, and relieve the symptoms of CIA rats than the therapeutic intervention (106). RA symptoms are closely related to the overproduction of pro-inflammatory factors and the activation of intracellular pro-inflammatory signals. In addition, after different doses of compound probiotics (B. breve, L. casei, L. bulgaricus, L. rhamnosus and L. acidophilus) intervened in CIA mice, the degree of joint swelling and pain sensitivity of mice were reduced, and the infiltration of inflammatory cells was also reduced. Serum IL-1β levels decreased, and the activated p38 mitogen-activated protein kinase (MAPK) inflammatory pathway in the spinal cord was inhibited (107). In addition, the reduction of inflammatory factors by gut microbes by regulating redox balance may be one of the mechanisms by which probiotics alleviate rheumatoid arthritis (108). Gavage og L. casei in CIA rats can significantly increase the types and abundance of gut microbes. They found that enterobacterial abundance was inversely correlated with pentose phosphate pathway activating enzyme activity. This indicates that L. casei can inhibit the activation of the pentose phosphate pathway, maintain redox balance, and reduce the production of pro-inflammatory factors IL-1β, IL-6, and IL-17, thereby alleviating rheumatoid arthritis in rats (109).
In this meta-analysis, although only CRP in this meta-analysis showed lower in the probiotic group, results from some studies showed improvement in RA. Alipour et al., 2014 treated patients with L. casei 1*10^8 CFU and found improvements in CRP levels, tender and swollen joint counts, GH scores and DAS28 compared to placebo; meanwhile, more patients in the probiotic group had a moderate response to treatment. Mandel et al., 2010 found a statistically significant improvement in joint pain and a reduction in CRP in patients receiving Bacillus coagulans GBI-30, 6086 2*10^8 CFU compared to placebo. Cannarella et al., 2021 found that probiotics improved white blood cell counts, TNF-α (P = 0.004) and IL-6 plasma levels. This suggests that L. casei, Bacillus coagulans, L. acidophilus, Lactococcus lactis, B. lactis and B. bifidum may have potential curative effects on RA, and more research on the intervention of these probiotics in RA can be carried out in the future. However, since some of these RCTs do not report the details of random sequence generation methods, allocation concealment methods, and blinding methods, the quality was degraded. Hence, the results need to be interpreted with caution. In addition, some RCTs did not report adverse events, so more RCTs reporting efficacy and safety are needed to further explore the treatment of RA with probiotics.
4.2 Probiotics for psoriasis
Psoriasis affects about 2% of the global population and affects all age groups (110). Psoriasis may be related to genetic factors, immune dysfunction, and environmental factors. As a chronic inflammatory disease, psoriasis can be caused by a variety of factors, and the disease is prone to relapse over time. Studies have shown that the pathogenesis of psoriasis is mainly related to the helper T cell (Th cell) 17/IL-23 axis. The intestinal microbiota can participate in the differentiation of T cells, such as segmented filamentous bacteria can induce pro-inflammatory responses in Th17 cells in the intestine (111). Experiments have shown that both short-chain fatty acids (SCFAs)-producing microbiota and SCFAs can act as potent regulators of T cells in the context of T cell-mediated inflammation (112–115). Among them, Clostridium is the main producer of SCFAs, which can induce the production of IL-10 in the colon, and at the same time increase the number of regulatory T cells (Treg cells) in the mucosa, playing a key role in intestinal homeostasis (113). In terms of microbiota distribution, there are certain differences between healthy people and patients with psoriasis. For example, the abundance of Akkermansiamuciniphila is decreased in patients with psoriasis (116). Experiments have shown that increasing A. muciniphila can reduce obesity and repair the damaged intestinal barrier in mice caused by diet (117). Therefore, the decreased abundance of A. muciniphila in the gut of patients with psoriasis can lead to impairment of the intestinal barrier, which in turn induces the development of psoriasis. The gut microbiota of patients with psoriatic arthritis was the same as that of patients with cutaneous psoriasis, and both beneficial bacteria were reduced. It is manifested as Th1 and Th17 cell-driven inflammatory arthritis with new bone formation, suggesting that spondyloarthritis and psoriasis may share a common pathogenic pathway (118). The study found disturbances in the gut microbiota in both people with spondyloarthritis and rodent models. The results of ileal biopsy showed that the Porphyromonas, Lachnospiraceae, Verrucobacterium, Rikenbacteriaceae and Bacteroidetes are rich (119). Meanwhile, transplantation of Escherichia coli from Crohn’s disease patients with spondyloarthritis into germ-free mice induces a Th17 cell response. It also increases the severity of colitis or arthritis in disease-sensitive IL-10-deficient or potassium/BxN mice (120). In summary, the intestinal microbiota is involved in the occurrence and development of psoriasis and psoriatic arthritis. Deng et al. (121) found that reducing B. breve CCFM1078 and B. adolescentis CCFM667 and L. paracasei CCFM1074 and L. reuteri could reduce the levels of IL-23/Th17 axis inflammatory factors in animal models of psoriasis. Its specific action pathway is to promote the production of short-chain fatty acid SCFAs and then reduce the level of IL-23/Th17 axis inflammatory factors, thereby alleviating psoriasis. This meta-analysis also showed that the PASI score of patients with psoriasis decreased after probiotic intervention. However, due to the small number of RCTs, meta-analysis of outcomes such as inflammatory factors could not be performed and the results need to be interpreted with caution. In addition, some RCTs did not report adverse events, hence, more RCTs reporting efficacy and safety are needed to further explore the treatment of psoriasis with probiotics.
4.3 Probiotics for spondyloarthritis
Ankylosing spondylitis (AS) is a chronic progressive rheumatic disease that is mainly characterized by chronic inflammation of the axial joint and can involve internal organs and other tissues. The incidence of AS ranges from 0.1% to 1.6% (122), the age of first onset is 20 to 30 years old, and it is more common in young men (123). AS is a common, highly hereditary immune arthropathy that occurs in genetically susceptible individuals exposed to unknown but possibly prevalent environmental triggers. Animal model studies have shown that germ-free SKG mice cannot develop the disease, but chlamydia infection can lead to axial and peripheral arthritis, psoriasis and uveitis, indicating that the intestinal microbiota plays an important role in the pathogenesis of AS (124). The study found that the intestinal microbiota structure of AS patients was significantly changed compared with the normal population. Compared with healthy controls, the ileum terminal ileum had higher abundances of five bacterial families: Lachnospira, Prevotella, Rikenbacteriaceae, Porphyromonas, and Bacteroidetes (125). Another study found that Bacteroidetes, Firmicutes, Proteobacteria, and Actinobacteria were the four major microbiota in the gut microbiota of AS patients and healthy controls. However, the abundance of actinomycetes in AS patients was significantly higher than that in the control group, especially Bifidobacterium, while the abundance of Clostridium and Verrucobacterium was lower, and Gram-negative Enterobacteriaceae and Citrobacter were relatively less (126). At present, the mechanism of action of gut microbiota in the etiology of AS is not fully understood. It is generally believed that intestinal microbiota may lead to the occurrence and development of AS through molecular simulation of HLA-B27, modification of autoantigens, destruction of tight junction proteins, increase of intestinal permeability and mediating abnormal immune responses of intestinal-associated lymphoid tissues (127). Asquith et al. believed that HLA alleles increased the risk of AS by interacting with the gut microbiome, and suggested that therapies targeting the microbiome may be effective in preventing or treating AS (128). Amdekar et al. have shown that L. casei may prevent arthritis by reducing prostaglandin levels and reducing joint inflammation in rats with collagen-induced arthritis (129). However, in this systematic review and meta-analysis, only two RCTs were included and showed no clinical effect, which indicates that the therapy (Streptococcus salivarius K12 1.6×10^8 CFU + B. lactis LAFTI B94 6.4×10^8 CFU + L. acidophilus LAFTI L10 6.4×10^8 CFU) in Jenks et al., 2010 (48) and the therapy (L. salivarius CUL61 6.25×10^9 CFU + L. paracasei CUL08 1.25×10^9 CFU + B. infantis CUL34 1.25×10^9 CFU + B. bifidum CUL20 1.25×10^9 CFU) in Brophy et al., 2008 (49) may not be effective in AS and potentially other probiotics could be considered for investigation. However, since Brophy et al., 2008 (49) conducted an Internet survey without face-to-face patient contact; and probiotics for spondyloarthritis involved only 2 RCTs, the reliability of the results needs to be interpreted with caution. In addition, some RCTs did not report adverse events, hence, more RCTs reporting efficacy and safety are needed to further explore the treatment of spondyloarthritis with probiotics.
4.4 Probiotics for hyperuricemia and gout
Gout is an inflammatory disease caused by purine metabolism disorder, decreased uric acid excretion or excessive production, resulting in elevated blood uric acid levels and deposition of uric acid crystals in joints and other connective tissues (130). The typical clinical manifestations of joint swelling and pain are not only joint deformities, but also kidney damage, cardiovascular and cerebrovascular diseases, etc., which seriously affect the quality of life of patients (131). The occurrence and development of gout is directly related to the level of blood uric acid in the body, and uric acid is the final metabolite of purine compounds. Therefore, regulating purine metabolism is very important for the pathogenesis and treatment of gout. Studies have found that there are two types of purines in the body, exogenous and endogenous. Reasonable adjustment of dietary structure to reduce the intake of exogenous purines is an effective method for clinically controlling acute gout attacks (132). As an important organ for digestion and absorption of food, the main function of the intestine is that the intestinal microbiota decomposes food into monosaccharides, which are then absorbed into the blood by small intestinal epithelial cells. If the intestinal microenvironment is disturbed, the intestinal microbiota will directly migrate to the extra-intestinal tissues, produce small molecules and participate in the blood circulation, and ultimately interfere with the intestinal Toll-like receptors and lead to the occurrence of rheumatic immune diseases (133, 134). Therefore, intestinal microbiota is closely related to the occurrence and development of gout by participating in the synthesis of purine metabolizing enzymes and the release of inflammatory factors. Studies have confirmed that compared with healthy people, the abundance of intestinal microbiota in gout patients is significantly lower (135). At present, many studies have found that the intestinal microbiota in gout patients is significantly different from that of healthy people. Ren et al. found that there is an imbalance of intestinal microbiota in patients with hyperuricemia, which is mainly manifested as a decrease in the number of physiological microbiota (136). However, the number of Enterobacteriaceae and total anaerobic bacteria increased significantly, indicating that the increase in serum uric acid may be related to the reduction of probiotics such as Bifidobacterium and Lactobacillus (136). Guo et al. found that the number of Bacteroides caccae and Bacteroides xylanisolvens in gout patients was significantly increased, while Faecalibacterium prausnitzii and Bifidobacterium pseudocatenulatum were relatively absent (137). Shao et al. (138) found that the diversity of gut microbiota in most gout patients showed a downward trend, with changes in opportunistic pathogens such as anaerobic bacteria and Bacteroidetes. In addition, Xing et al. (139) found that the number of Clostridium spp. in gout patients was significantly lower than that in healthy people. Some researchers have also used 1 H-NMR and Illumina Miseq technology to study the metabolic profile and microbial community of fecal extracts from gout patients and healthy people. They found that opportunistic pathogens such as Anaerobic bacteria, Bacteroides and Porphyromonas were significantly increased in gout patients (140). Animal studies have shown that under the effects of Proteobacteria, the intestinal nutritional conditions of gout rats are deteriorated, and the permeability of intestinal epithelium and renal endothelium is significantly increased, which promotes the entry of lipopolysaccharide into the blood circulation and significantly aggravates the attack of gout (141). Another study found that compared with normal mice, hyperuricemia mice had significantly less Bifidobacteria and Lactobacilli, while serum xanthine oxidase activity and lipopolysaccharide levels increased. After treatment with probiotics, beneficial bacteria in the intestinal tract increased, and the expression levels of serum lipopolysaccharide and xanthine oxidase decreased significantly (142). This meta-analysis showed that serum uric acid decreased in patients after probiotic intervention. Yamanaka et al., 2019 (61) and Kamatani et al., 2018 (62) used yogurt containing L. delbrueckii ssp. bulgaricus and Streptococcus thermophilus to treat hyperuricemia and gout, but did not show an improvement in serum uric acid, suggesting that the efficacy of these probiotics needs to be further explored, which provides a reference for screening effective microbiota. Furthermore, since only 4 RCTs were involved, and the quality of the literature was degraded because the details of random sequence generation methods, allocation concealment methods, and blinding methods in some RCTs was not reported, the results need to be interpreted with caution. In addition, those RCTs did not report adverse events, hence, more RCTs reporting efficacy and safety are needed to further explore the treatment of psoriasis with probiotics.
4.5 Probiotics for OA
OA is a highly prevalent and disabling disease, affecting more than 7% of the world’s population (about 528 million people), of which knee OA (KOA) accounts for about 85% (141, 143). It is estimated that the prevalence of KOA is about 10% in men and 13% in women among the elderly aged 60 years or older (144). Global life expectancy, changes in dietary habits and lifestyles, aging and obesity prevalence and other social trends have increased the prevalence of KOA, so attention should be paid to the prevention and treatment of KOA (145). In recent years, gut microbes have gradually become a focus of attention in joint inflammatory diseases, and gut microbial imbalance is closely related to the development of OA (146–148). With the progress of microbiome research, modern medicine proposed the concept of “gut-joint” axis. That is, the intestines and joints are closely connected, and diseases of the intestines may induce lesions in the joints. On the contrary, joint diseases can also affect the intestines, and the two are interrelated (149). Gut microbes can positively regulate the host’s immune system, exert immunomodulatory effects (150), protect from pathogenic microorganisms (151), and maintain normal physiological functions. Modulation of dysbiosis and metabolic dysfunction through microbial supplementation may prevent the development of knee injuries (152). For example, prebiotics can reduce systemic inflammatory response and protect articular cartilage in a rodent model of KOA by positively altering the gut microbiota (153). During monoiodoacetate (MIA)-induced KOA, administration of probiotics significantly reduced the expression of proinflammatory cytokines in rat knee articular cartilage (154). In a model of HFS diet-induced knee injury in obese rats, both prebiotic fiber supplementation and aerobic exercise both alone and in combination therapy improved knee injury (153). In a guinea pig model of spontaneous OA, oral administration of B. longum CBi0703 significantly improved cartilage structure and exhibited comprehensive joint protection (155). Therefore, controlling the gut microbiota is considered to be a feasible therapeutic strategy to improve obesity-related OA disease (156). Animal studies have shown that chondroitin sulfate combined with probiotics can prevent oxidative stress in the serum of rats with experimental osteoarthritis (157). Probiotics prevent cartilage damage and osteoarthritis progression in mice (158). Only one RCT reported probiotics for OA in this research. Lei et al., 2017 treated 215 patients with L. casei Shirota and another 218 with placebo. They found that after 6 months of treatment, compared with the placebo group, patients in the probiotic group had significantly improved WOMAC and VAS scores, and decreased serum hs-CRP levels (P<0.05). They also claim that no serious adverse events were observed throughout the study.
4.6 Probiotics for JIA
JIA, defined as arthritis of unknown etiology with onset under the age of 16 and lasting more than 6 weeks, is the most common chronic rheumatic disease in childhood (159–161). At present, more and more studies believe that the pathogenesis of JIA is the result of multiple factors. A genetic susceptible individual is exposed to one or more environmental factors, resulting in local tissue damage, autoantigen release, excessive activation of mononuclear macrophages, neutrophils and other phagocytes, and release of a large number of proinflammatory cytokines, such as IL-1, IL-6, IL-18 and proinflammatory protein S100, which eventually lead to chronic synovitis and systemic multisystem inflammation (162–165). In recent years, the role of gut microbes in regulating the homeostasis of the host has attracted much attention (166). It is gradually recognized that gut microbes may be an important environmental factor involved in the occurrence of JIA and affect disease progression (167). Tejesvi et al. found that the abundance of Firmicutes and Bacteroidetes increased in the gut microbiota of children with primary, untreated JIA (168). With the advancement of experimental technology, the research on gut microbiota has gradually deepened, and more and more studies have found that the gut microbiome structure characteristics of JIA children are significantly different from those of normal children. It can be manifested as changes in microbial richness and diversity, abnormal composition and structure of some bacterial groups, and a decrease in the number of some beneficial microbiota, such as butyrate-producing bacteria (169). These studies suggest that alterations in the structural characteristics of gut microbes are closely related to the occurrence of JIA. Some scholars have found that the abundance of Faecalibacterium in the intestinal microbiota of children with JIA (polyarticular JIA and ERA) is decreased (170). Faecalibacterium is a butyrate producing bacteria (BPB), and its reduction can induce an inflammatory state in the body (167). Due to the difficult treatment of JIA at present, the disease is easy to repeat, the long-term application of glucocorticoids and immunosuppressive agents has serious side effects, and the biological preparations are expensive (160, 171). Therefore, it is urgent for the clinic to summarize the efficacy and safety of the intervention effect of probiotic-based microecological preparations in children with JIA.
Most of the probiotics involved in the included studies were Bifidobacterium, Lactobacillus, Enterococcus and Bacillus, and the doses involved were mostly above 1*10^8 CFU. It is suggested that the intestinal microbiota preparations based on Bifidobacterium, Lactobacillus, Enterococcus and Bacillus may have better effect when the dose is above 1*10^8 CFU.
4.7 Strength and limitations and inspiration for future research
The strength is that this systematic review and meta-analysis evaluated the efficacy and safety of probiotics on 8 types of inflammatory arthritis for the first time, and provided clinical reference.
The limitations of this review are that: (1) The quality of the included RCTs is generally degraded by the lack of detailed random sequence generation, allocation concealment, and blinding information. (2) Vadell et al., 2020 (41) and Lambert et al., 2017 (53) used food containing probiotics and did not give specific strains and doses, while for some of other RCTs, although strains were given, the dosage was uncertain, which further brought heterogeneity. (3) In different diseases, although the same efficacy indicators have been reported, the methods of recording are different. As in psoriasis, Navarro-López et al., 2019 (44) reported the percentage of PASI improvement, but did not provide its specific value, while Lu 2017 (46) and Moludi et al., 2021 (47) reported the specific value of PASI, therefore Navarro-López et al., 2019 (44) was not included in the meta-analysis. (4) Furthermore, most diseases contain fewer than 10 RCTs, and OA even contains only 1 RCT. And only Osteoporosis and Osteopenia included more than 1000 participants (1156), the rest of the diseases included less than 1000 participants. (5) This systematic review only included 8 types of inflammatory arthritis, and the others were not retrieved, which may be due to the fact that probiotics for inflammatory arthritis have just emerged and received less attention. (6) Many RCTs did not report adverse events, perhaps because they were not observed, or were not monitored. These results in the efficacy and safety of probiotics in the treatment of inflammatory arthritis need to be interpreted with caution.
For safety, many RCTs did not report adverse events, raising concerns about the safety of the RCTs included in this review. Based on this, we need to carefully examine many RCTs that do not report adverse events. For example, Tang et al. conducted a search (up to February 2014) of Phase II and IV RCTs of at least one serious adverse event related to drug therapy registered on ClinicalTrials.org, and a total of 1580 RCTs were screened. Then they randomly selected 300 RCTs, and found the corresponding published literature, and compared the agreement between the two serious adverse events, but the results were not completely consistent (172). Specifically, of the 139 RCTs reporting serious adverse events, 44 (32%) RCTs reported a number of serious adverse events in publications that were inconsistent with those recorded on ClinicalTrials.gov. The incidence of serious adverse events reported in the publications of 22 RCTs was lower than the incidence of adverse events recorded on ClinicalTrials.gov, with a difference of more than 30% in each group. Therefore, we consider that the reasons why the included RCTs did not report adverse events may be: (1) RCTs are not registered in the clinical trial center, and the writing is not standardized; (2) There may be cases where only good outcomes are reported, and bad outcomes are not reported; (3) The publications have limited space, etc. This prompts us to interpret the safety results of this study cautiously, and it also suggests that future RCTs should report adverse events in detail. It is also expected that more RCTs in the future can publish the detailed data of the trial in the professional clinical trial database to provide data support for clinical application. In addition, more standardized RCTs reporting on the treatment of other types of inflammatory arthritis with probiotics are needed in the future to further determine the efficacy of probiotics on various inflammatory arthritis.
5 Conclusion
Probiotic supplements may improve Hyperuricemia and gout, Inflammatory bowel disease arthritis, JIA, OA, Osteoporosis and Osteopenia, Psoriasis, RA, Spondyloarthritis. However, lack of evidence and heterogeneity of studies do not allow us to recommend them to patients with inflammatory arthritis to manage their disease. More randomized controlled trials are needed in the future to determine the efficacy and optimal dosing design of probiotics.
Data availability statement
The original contributions presented in the study are included in the article/Supplementary Material. Further inquiries can be directed to the corresponding authors.
Author contributions
LZ and HC are responsible for the study concept and design. LZ, QH, KY, YD, JL, WX, HL, XZ, HC are responsible for the data collection, data analysis and interpretation; LZ and KY drafted the paper; HC supervised the study; all authors participated in the analysis and interpretation of data and approved the final paper.
Conflict of interest
The authors declare that the research was conducted in the absence of any commercial or financial relationships that could be construed as a potential conflict of interest.
Publisher’s note
All claims expressed in this article are solely those of the authors and do not necessarily represent those of their affiliated organizations, or those of the publisher, the editors and the reviewers. Any product that may be evaluated in this article, or claim that may be made by its manufacturer, is not guaranteed or endorsed by the publisher.
Supplementary material
The Supplementary Material for this article can be found online at: https://www.frontiersin.org/articles/10.3389/fimmu.2022.961325/full#supplementary-material
References
1. Hammaker D, Firestein GS. Epigenetics of inflammatory arthritis. CurrOpinRheumatol (2018) 30(2):188–96. doi: 10.1097/BOR.0000000000000471
2. Cronstein BN, Aune TM. Methotrexate and its mechanisms of action in inflammatory arthritis. Nat Rev Rheumatol (2020) 16(3):145–54. doi: 10.1038/s41584-020-0373-9
3. Chiu YM, Chen DY. Infection risk in patients undergoing treatment for inflammatory arthritis: non-biologics versus biologics. Expert Rev Clin Immunol (2020) 16(2):207–28. doi: 10.1080/1744666X.2019.1705785
4. Scherer HU, Häupl T, Burmester GR. The etiology of rheumatoid arthritis. J Autoimmun (2020) 110:102400. doi: 10.1016/j.jaut.2019.102400
5. Zhao J, Wei K, Jiang P, Chang C, Xu L, Xu L, et al. Inflammatory response to regulated cell death in gout and its functional implications. Front Immunol (2022) 13:888306. doi: 10.3389/fimmu.2022.888306
6. Ceribelli A, Motta F, Vecellio M, Isailovic N, Ciccia F, Selmi C. Clinical trials supporting the role of the IL-17/IL-23 axis in axial spondyloarthritis. Front Immunol (2021) 12:622770. doi: 10.3389/fimmu.2021.622770
7. Conway R, Konig MF, Graef ER, Webb K, Yazdany J, Kim AHJ. Inflammatory arthritis in patients with COVID-19. Transl Res (2021) 232:49–59. doi: 10.1016/j.trsl.2021.02.010
8. Thomson A, Hilkens CMU. Synovial macrophages in osteoarthritis: The key to understanding pathogenesis? Front Immunol (2021) 12:678757. doi: 10.3389/fimmu.2021.678757
9. Sun R, Wang X, Sun X, Zhao B, Zhang X, Gong X, et al. Emerging roles of long non-coding RNAs in ankylosing spondylitis. Front Immunol (2022) 13:790924. doi: 10.3389/fimmu.2022.790924
10. Rogler G, Singh A, Kavanaugh A, Rubin DT. Extraintestinal manifestations of inflammatory bowel disease: Current concepts, treatment, and implications for disease management. Gastroenterology. (2021) 161(4):1118–32. doi: 10.1053/j.gastro.2021.07.042
11. Huang J, Fu X, Chen X, Li Z, Huang Y, Liang C. Promising therapeutic targets for treatment of rheumatoid arthritis. Front Immunol (2021) 12:686155. doi: 10.3389/fimmu.2021.686155
12. Singh JA, Gaffo A. Gout epidemiology and comorbidities. Semin Arthritis Rheumatol (2020) 50(3S):S11–6. doi: 10.1016/j.semarthrit.2020.04.008
13. Dalbeth N, Gosling AL, Gaffo A, Abhishek A. Gout. Lancet. (2021) 397(10287):1843–55. doi: 10.1016/S0140-6736(21)00569-9
14. Zavodovsky BV, Sivordova LE. Cardiovascular safety of non-steroidal anti-inflammatory drugs in chronic inflammatory rheumatic diseases. Ter Arkh (2018) 90(8):101–6. doi: 10.26442/terarkh2018908101-106
15. Hardy RS, Raza K, Cooper MS. Therapeutic glucocorticoids: mechanisms of actions in rheumatic diseases. Nat Rev Rheumatol (2020) 16(3):133–44. doi: 10.1038/s41584-020-0371-y
16. Hyrich KL, Machado PM. Rheumatic disease and COVID-19: epidemiology and outcomes. Nat Rev Rheumatol (2021) 17(2):71–2. doi: 10.1038/s41584-020-00562-2
17. Wilson L, Saseen JJ. Gouty arthritis: A review of acute management and prevention. Pharmacotherapy. (2016) 36(8):906–22. doi: 10.1002/phar.1788
18. Hughes CD, Pollard LC, Scott DL. A systematic review of the impact of intensive therapy on remission in rheumatoid arthritis. Rheumatology (2016) 55(suppl 1):i100. doi: 10.1093/rheumatology/kew144.021
19. Rubbert-Roth A, Finckh A. Treatment options in patients with rheumatoid arthritis failing initial TNF inhibitor therapy: a critical review. Arthritis Res Ther (2009) 11 Suppl 1(Suppl 1):S1. doi: 10.1186/ar2666
20. Guzman J, Oen K, Tucker LB, Huber AM, Shiff N, Boire G, et al. The outcomes of juvenile idiopathic arthritis in children managed with contemporary treatments: results from the ReACCh-out cohort. Ann Rheum Dis (2015) 74(10):1854–60. doi: 10.1136/annrheumdis-2014-205372
21. Jethwa H, Abraham S. The evidence for microbiome manipulation in inflammatory arthritis. Rheumatol (Oxford) (2017) 56(9):1452–60. doi: 10.1093/rheumatology/kew374
22. Breban M. Gut microbiota and inflammatory joint diseases. Joint Bone Spine (2016) 83(6):645–9. doi: 10.1016/j.jbspin.2016.04.005
23. Scher JU, Nayak RR, Ubeda C, Turnbaugh PJ, Abramson SB. Pharmacomicrobiomics in inflammatory arthritis: gut microbiome as modulator of therapeutic response. Nat Rev Rheumatol (2020) 16(5):282–92. doi: 10.1038/s41584-020-0395-3
24. Jiao Y, Wu L, Huntington ND, Zhang X. Crosstalk between gut microbiota and innate immunity and its implication in autoimmune diseases. Front Immunol (2020) 11:282. doi: 10.3389/fimmu.2020.00282
25. Yao M, Qv L, Lu Y, Wang B, Berglund B, Li L. An update on the efficacy and functionality of probiotics for the treatment of non-alcoholic fatty liver disease. Engineering (2021) 7(5):679–86. doi: 10.1016/j.eng.2020.01.017
26. Zaiss MM, Joyce Wu HJ, Mauro D, Schett G, Ciccia F. The gut-joint axis in rheumatoid arthritis. Nat Rev Rheumatol (2021) 17(4):224–37. doi: 10.1038/s41584-021-00585-3
27. Bungau SG, Behl T, Singh A, Sehgal A, Singh S, Chigurupati S, et al. Targeting probiotics in rheumatoid arthritis. Nutrients. (2021) 13(10):3376. doi: 10.3390/nu13103376
28. Kim WG, Kim HI, Kwon EK, Han MJ, Kim DH. Lactobacillus plantarum LC27 and bifidobacterium longum LC67 mitigate alcoholic steatosis in mice by inhibiting LPS-mediated NF-κB activation through restoration of the disturbed gut microbiota. Food Funct (2018) 9(8):4255–65. doi: 10.1039/c8fo00252e
29. Wang K, Dong H, Qi Y, Pei Z, Yi S, Yang X, et al. Lactobacillus casei regulates differentiation of Th17/Treg cells to reduce intestinal inflammation in mice. Can J Vet Res (2017) 81(2):122–8.
30. Deeks JJ, Higgins JP, Altman DG. Chapter 8: assessing risk of bias in included studies. In: Higgins JP, Green S, editors. Cochrane handbook or systematic reviews of interventions version 6.1.0, vol. 2020 . UK: The Cochrane Collaboration (2020).
31. Deeks JJ, Higgins JP, Altman DG. Chapter 9: Analyzing data and undertaking meta-analyses. In: Higgins JP, Green S, editors. Cochrane handbook for systematic reviews of interventions, vol. 2020. UK: The Cochrane Collaboration (2020).
32. Alipour B, Homayouni-Rad A, Vaghef-Mehrabany E, Sharif SK, Vaghef-Mehrabany L, Asghari-Jafarabadi M, et al. Effects of lactobacillus casei supplementation on disease activity and inflammatory cytokines in rheumatoid arthritis patients: a randomized double-blind clinical trial. Int J Rheum Dis (2014) 17(5):519–27. doi: 10.1111/1756-185X.12333
33. Vaghef-Mehrabany E, Alipour B, Homayouni-Rad A, Sharif SK, Asghari-Jafarabadi M, Zavvari S. Probiotic supplementation improves inflammatory status in patients with rheumatoid arthritis. Nutrition. (2014) 30(4):430–5. doi: 10.1016/j.nut.2013.09.007
34. Vaghef-Mehrabany E, Homayouni-Rad A, Alipour B, Sharif SK, Vaghef-Mehrabany L, Alipour-Ajiry S. Effects of probiotic supplementation on oxidative stress indices in women with rheumatoid arthritis: A randomized double-blind clinical trial. J Am Coll Nutr (2016) 35(4):291–9. doi: 10.1080/07315724.2014.959208
35. Hatakka K, Martio J, Korpela M, Herranen M, Poussa T, Laasanen T, et al. Effects of probiotic therapy on the activity and activation of mild rheumatoid arthritis–a pilot study. Scand J Rheumatol (2003) 32(4):211–5. doi: 10.1080/03009740310003695
36. Mandel DR, Eichas K, Holmes J. Bacillus coagulans: a viable adjunct therapy for relieving symptoms of rheumatoid arthritis according to a randomized, controlled trial. BMC Complement Altern Med (2010) 10:1. doi: 10.1186/1472-6882-10-1
37. Pineda Mde L, Thompson SF, Summers K, de Leon F, Pope J, Reid G. A randomized, double-blinded, placebo-controlled pilot study of probiotics in active rheumatoid arthritis. Med Sci Monit (2011) 17(6):CR347–54. doi: 10.12659/msm.881808
38. Zamani B, Golkar HR, Farshbaf S, Emadi-Baygi M, Tajabadi-Ebrahimi M, Jafari P, et al. Clinical and metabolic response to probiotic supplementation in patients with rheumatoid arthritis: a randomized, double-blind, placebo-controlled trial. Int J Rheum Dis (2016) 19(9):869–79. doi: 10.1111/1756-185X.12888
39. Zamani B, Farshbaf S, Golkar HR, Bahmani F, Asemi Z. Synbiotic supplementation and the effects on clinical and metabolic responses in patients with rheumatoid arthritis: a randomised, double-blind, placebo-controlled trial. Br J Nutr (2017) 117(8):1095–102. doi: 10.1017/S000711451700085X
40. Esmaeili F, Salesi M, Askari G, Esmaeilisharif A, Maracy M, Karimzadeh H, et al. Efficacy of synbiotic supplementation in improving rheumatoid arthritis. Res Pharm Sci (2020) 15(3):263–72. doi: 10.4103/1735-5362.288432
41. Vadell AKE, Bärebring L, Hulander E, Gjertsson I, Lindqvist HM, Winkvist A. Anti-inflammatory diet in rheumatoid arthritis (ADIRA)-a randomized, controlled crossover trial indicating effects on disease activity. Am J Clin Nutr (2020) 111(6):1203–13. doi: 10.1093/ajcn/nqaa019
42. Gao J, Liu Yi, Liu B, Kong X, Hu H. Effect of bifidobacterium quadruple viable tablet on TNF-α and adiponectin levels in patients with rheumatoid arthritis. J Taishan Med Coll (2017) 38(7):761–4. doi: 10.3969/j.issn.1004-7115.2017.07.014
43. Cannarella LAT, Mari NL, Alcântara CC, Iryioda TMV, Costa NT, Oliveira SR, et al. Mixture of probiotics reduces inflammatory biomarkers and improves the oxidative/nitrosative profile in people with rheumatoid arthritis. Nutrition. (2021) 89:111282. doi: 10.1016/j.nut.2021.111282
44. Navarro-López V, Martínez-Andrés A, Ramírez-Boscá A, Ruzafa-Costas B, Núñez-Delegido E, Carrión-Gutiérrez MA, et al. Efficacy and safety of oral administration of a mixture of probiotic strains in patients with psoriasis: A randomized controlled clinical trial. Acta DermVenereol (2019) 99(12):1078–84. doi: 10.2340/00015555-3305
45. Groeger D, O’Mahony L, Murphy EF, Bourke JF, Dinan TG, Kiely B, et al. Bifidobacterium infantis 35624 modulates host inflammatory processes beyond the gut. Gut Microbes (2013) 4(4):325–39. doi: 10.4161/gmic.25487
46. Lu XY. Therandomized,double blind and control study of probiotics in the treatment of psoriasis vulgaris. China Modern Med (2017) 24(08):47–9.
47. Moludi J, Khedmatgozar H, Saiedi S, Razmi H, Alizadeh M, Ebrahimi B. Probiotic supplementation improves clinical outcomes and quality of life indicators in patients with plaque psoriasis: A randomized double-blind clinical trial. Clin Nutr ESPEN (2021) 46:33–9. doi: 10.1016/j.clnesp.2021.09.004
48. Jenks K, Stebbings S, Burton J, Schultz M, Herbison P, Highton J. Probiotic therapy for the treatment of spondyloarthritis: a randomized controlled trial. J Rheumatol (2010) 37(10):2118–25. doi: 10.3899/jrheum.100193
49. Brophy S, Burrows CL, Brooks C, Gravenor MB, Siebert S, Allen SJ. Internet-Based randomised controlled trials for the evaluation of complementary and alternative medicines: probiotics in spondyloarthropathy. BMC MusculoskeletDisord (2008) 9:4. doi: 10.1186/1471-2474-9-4
50. Guo ZG. Clinical study on the effect of probiotic lactic acid bacteria on osteoporosis in postmenopausal women. Inner Mongolia Medical University (2020).
51. Jafarnejad S, Djafarian K, Fazeli MR, Yekaninejad MS, Rostamian A, Keshavarz SA. Effects of a multispecies probiotic supplement on bone health in osteopenic postmenopausal women: A randomized, double-blind, controlled trial. J Am Coll Nutr (2017) 36(7):497–506. doi: 10.1080/07315724.2017.1318724
52. Jansson PA, Dan C, Ahreén IL, Hansson F, Ohlsson C. Probiotic treatment using a mix of three lactobacillus strains for lumbar spine bone loss in postmenopausal women: a randomised, double-blind, placebo-controlled, multicentre trial. Lancet Rheumatol (2019) 1(3):e154–62. doi: 10.1016/S2665-9913(19)30068-2
53. Lambert MNT, Thybo CB, Lykkeboe S, Rasmussen LM, Frette X, Christensen LP, et al. Combined bioavailable isoflavones and probiotics improve bone status and estrogen metabolism in postmenopausal osteopenic women: a randomized controlled trial. Am J Clin Nutr (2017) 106(3):909–20. doi: 10.3945/ajcn.117.153353
54. Li F, Gong CL, Wang QL, Chen Q, Lu Y. Effects of probiotics on bone metabolism in postmenopausal patients with osteoporosis. Chin J Gerontol (2021) 41(11):2356 2359.
55. Liu HD. Observation of the efficacy of bifidobacterium triple viable enteric-coated capsules in the treatment of type 2 diabetes with osteoporosis. J Gannan Med Coll (2019) 39(04):353–5.
56. Nilsson AG, Sundh D, Bäckhed F, Lorentzon M. Lactobacillus reuteri reduces bone loss in older women with low bone mineral density: a randomized, placebo-controlled, double-blind, clinical trial. J Intern Med (2018) 284(3):307–17. doi: 10.1111/joim.12805
57. Li P, Sundh D, Ji B, Lappa D, Ye L, Nielsen J, et al. Metabolic alterations in older women with low bone mineral density supplemented with lactobacillus reuteri. JBMR Plus (2021) 5(4):e10478. doi: 10.1002/jbm4.10478
58. Song YP, Guo YX, Li JP. Supplementation of bifidobacterium quadruple viable tablets on the effects of bone metabolism in patients with diabetic osteoporosis. Chin J Osteo (2020) 26(02):291–3.
59. Takimoto T, Hatanaka M, Hoshino T, Takara T, Tanaka K, Shimizu A, et al. Effect of bacillus subtilis c-3102 on bone mineral density in healthy postmenopausal Japanese women: a randomized, placebo-controlled, double-blind clinical trial. Biosci Microbio Food Health (2018) 37(4):87–96. doi: 10.12938/bmfh.18-006
60. Wang XS, Liu L, Liu HM. Effects of probiotics on bone biochemical metabolism and osteoporosis in the elderly. Modern digestion and interventional diagnosis and treatment. (2019) A01):1291–2.
61. Yamanaka H, Taniguchi A, Tsuboi H, Kano H, Asami Y. Hypouricaemic effects of yoghurt containing lactobacillus gasseri PA-3 in patients with hyperuricaemia and/or gout: A randomised, double-blind, placebo-controlled study. Mod Rheumatol (2019) 29(1):146–50. doi: 10.1080/14397595.2018.1442183
62. Kamatani N, Motii M, Hashimoto M, Sakurai K, Gokita K. Yoshihara J placebo controlled double blind parallel randomized study to test the urate-lowering effect of yogurt containing lactobacillus gasseri PA-3 in adult males with marginal hyperuricemia. Gout Nucleic Acid Metabol (2016) 40:21–31. doi: 10.6032/gnam.40.21
63. Zhan Y, Wang G, Li X, Zhu X, Tong X. Clinical observation of clostridium butyric viable bacteria tablets combined with febuxostat in the treatment of non-acute gout. Zhejiang J Integrated Tradition Chin West Med (2020) 30(05):385–8. doi: CNKI:SUN:ZJZH.0.2020-05-012
64. Wang P, Xu J. Efficacy of bifidobacterium triple viable bacteria assisted febuxostat in the treatment of patients with gout in the intermittent period. Eval Anal Medication Chin Hospitals (2022) 22(01):47–50. doi: 10.14009/j.issn.1672-2124.2022.01.010
65. Lei M, Guo C, Wang D, Zhang C, Hua L. The effect of probiotic lactobacillus casei shirota on knee osteoarthritis: a randomised double-blind, placebo-controlled clinical trial. Benef Microbes (2017) 8(5):697–703. doi: 10.3920/BM2016.0207
66. Shukla A, Gaur P, Aggarwal A. Effect of probiotics on clinical and immune parameters in enthesitis-related arthritis category of juvenile idiopathic arthritis. Clin Exp Immunol (2016) 185(3):301–8. doi: 10.1111/cei.12818
67. Malin M, Verronen P, Korhonen H, Syväoja EL, Salminen S, Mykkänen H, et al. Dietary therapy with lactobacillus GG, bovine colostrum or bovine immune colostrum in patients with juvenile chronic arthritis: evaluation of effect on gut defence mechanisms. Inflammopharmacology. (1997) 5(3):219–36. doi: 10.1007/s10787-997-0001-1
68. Zhang Y, Xu X, Yang N, Guan X, Peng Yunhui. Effects of narrative medical health education combined with probiotics intervention on sleep in patients with inflammatory bowel disease arthritis. Community Med J (2020) 18(02):117 –120. doi: 10.19790/j.cnki.JCM.2020.02.12
69. Zhang J, Zhong J, Nie D, Lin Z, Gao S. The effect of probiotics on the improvement of bone status in postmenopausal women with osteopenia. Chin J Microecol (2018) 30(12):1440–3.
70. Lee HJ, Waller RD, Stebbings S, Highton J, Orlovich DA, Schmierer D, et al. The effects of an orally administered probiotic on sulfasalazine metabolism in individuals with rheumatoid arthritis: a preliminary study. Int J Rheum Dis (2010) 13(1):48–54. doi: 10.1111/j.1756-185X.2009.01449.x
71. Nenonen MT, Helve TA, Rauma AL, Hänninen OO. Uncooked, lactobacilli-rich, vegan food and rheumatoid arthritis. Br J Rheumatol (1998) 37(3):274–81. doi: 10.1093/rheumatology/37.3.274
72. Levy M, Kolodziejczyk AA, Thaiss CA, Elinav E. Dysbiosis and the immune system. Nat Rev Immunol (2017) 17(4):219–32. doi: 10.1038/nri.2017.7
73. Quirke AM, Perry E, Cartwright A, Kelly C, De Soyza A, Eggleton P, et al. Bronchiectasis is a model for chronic bacterial infection inducing autoimmunity in rheumatoid arthritis. Arthritis Rheumatol (2015) 67(9):2335–42. doi: 10.1002/art.39226
74. Bergot AS, Giri R, Thomas R. The microbiome and rheumatoid arthritis. Best Pract Res Clin Rheumatol (2019) 33(6):101497. doi: 10.1016/j.berh.2020.101497
75. Catrina AI, Deane KD, Scher JU. Gene, environment, microbiome and mucosal immune tolerance in rheumatoid arthritis. Rheumatol (Oxford) (2016) 55(3):391–402. doi: 10.1093/rheumatology/keu469
76. Scher JU, Joshua V, Artacho A, Abdollahi-Roodsaz S, Öckinger J, Kullberg S, et al. The lung microbiota in early rheumatoid arthritis and autoimmunity. Microbiome. (2016) 4(1):60. doi: 10.1186/s40168-016-0206-x
77. Scher JU, Sczesnak A, Longman RS, Segata N, Ubeda C, Bielski C, et al. Expansion of intestinal prevotella copri correlates with enhanced susceptibility to arthritis. Elife. (2013) 2:e01202. doi: 10.7554/eLife.01202
78. Holers VM, Demoruelle MK, Kuhn KA, Buckner JH, Robinson WH, Okamoto Y, et al. Rheumatoid arthritis and the mucosal origins hypothesis: protection turns to destruction. Nat Rev Rheumatol (2018) 14(9):542–57. doi: 10.1038/s41584-018-0070-0
79. Zhang X, Zhang D, Jia H, Feng Q, Wang D, Liang D, et al. The oral and gut microbiomes are perturbed in rheumatoid arthritis and partly normalized after treatment. Nat Med (2015) 21(8):895–905. doi: 10.1038/nm.3914
80. Chen J, Wright K, Davis JM, Jeraldo P, Marietta EV, Murray J, et al. An expansion of rare lineage intestinal microbes characterizes rheumatoid arthritis. Genome Med (2016) 8(1):43. doi: 10.1186/s13073-016-0299-7
81. Marietta EV, Murray JA, Luckey DH, Jeraldo PR, Lamba A, Patel R, et al. Suppression of inflammatory arthritis by human gut-derived prevotella histicola in humanized mice. Arthritis Rheumatol (2016) 68(12):2878–88. doi: 10.1002/art.39785
82. Maeda Y, Kurakawa T, Umemoto E, Motooka D, Ito Y, Gotoh K, et al. Dysbiosis contributes to arthritis development via activation of autoreactive T cells in the intestine. Arthritis Rheumatol (2016) 68(11):2646–61. doi: 10.1002/art.39783
83. Alpizar-Rodriguez D, Lesker TR, Gronow A, Gilbert B, Raemy E, Lamacchia C, et al. Prevotella copri in individuals at risk for rheumatoid arthritis. Ann Rheum Dis (2019) 78(5):590–3. doi: 10.1136/annrheumdis-2018-214514
84. Inamo J. Non-causal association of gut microbiome on the risk of rheumatoid arthritis: a mendelian randomisation study. Ann Rheum Dis (2021) 80(7):e103. doi: 10.1136/annrheumdis-2019-216565
85. Alpizar Rodriguez D, Lesker TR, Gilbert B, Strowig T, Finckh A. Response to: ‘Non-causal association of gut microbiome on the risk of rheumatoid arthritis: a mendelian randomisation study’ by inamo. Ann Rheum Dis (2021) 80(7):e104. doi: 10.1136/annrheumdis-2019-216637
86. Jeong Y, Kim JW, You HJ, Park SJ, Lee J, Ju JH, et al. Gut microbial composition and function are altered in patients with early rheumatoid arthritis. J Clin Med (2019) 8(5):693. doi: 10.3390/jcm8050693
87. Gracey E, Vereecke L, McGovern D, Fröhling M, Schett G, Danese S, et al. Revisiting the gut-joint axis: links between gut inflammation and spondyloarthritis. Nat Rev Rheumatol (2020) 16(8):415–33. doi: 10.1038/s41584-020-0454-9
88. Aarts J, Boleij A, Pieters BCH, Feitsma AL, van Neerven RJJ, Ten Klooster JP, et al. Flood control: How milk-derived extracellular vesicles can help to improve the intestinal barrier function and break the gut-joint axis in rheumatoid arthritis. Front Immunol (2021) 12:703277. doi: 10.3389/fimmu.2021.703277
89. Elsouri K, Arboleda V, Heiser S, Kesselman MM, DemoryBeckler M. Microbiome in rheumatoid arthritis and celiac disease: A friend or foe. Cureus. (2021) 13(6):e15543. doi: 10.7759/cureus.15543
90. De Luca F, Shoenfeld Y. The microbiome in autoimmune diseases. Clin Exp Immunol (2019) 195(1):74–85. doi: 10.1111/cei.13158
91. Clemente JC, Manasson J, Scher JU. The role of the gut microbiome in systemic inflammatory disease Vol. 360. BMJ (2018). p. j5145. doi: 10.1136/bmj.j5145
92. Qaiyum Z, Lim M, Inman RD. The gut-joint axis in spondyloarthritis: immunological, microbial, and clinical insights. Semin Immunopathol (2021) 43(2):173–92. doi: 10.1007/s00281-021-00845-0
93. So J, Tam LS. Gut microbiome and its interaction with immune system in spondyloarthritis. Microorganisms (2020) 8(11):1727. doi: 10.3390/microorganisms8111727
94. Zheng J, Wittouck S, Salvetti E, Franz CMAP, Harris HMB, Mattarelli P, et al. A taxonomic note on the genus lactobacillus: Description of 23 novel genera, emended description of the genus lactobacillus beijerinck 1901, and union of lactobacillaceae and leuconostocaceae. Int J Syst Evol Microbiol (2020) 70(4):2782–858. doi: 10.1099/ijsem.0.004107
95. Malmström V, Catrina AI, Klareskog L. The immunopathogenesis of seropositive rheumatoid arthritis: from triggering to targeting. Nat Rev Immunol (2017) 17(1):60–75. doi: 10.1038/nri.2016.124
96. Li M, Sun Di, Fu B. Research progress on intestinal flora and autoimmune diseases. Chin J Microecol (2015) 27(10):1233–7. doi: 10.13381/j.cnki.cjm.201510032
97. Klareskog L, Padyukov L, Lorentzen J, Alfredsson L. Mechanisms of disease: Genetic susceptibility and environmental triggers in the development of rheumatoid arthritis. Nat Clin Pract Rheumatol (2006) 2(8):425–33. doi: 10.1038/ncprheum0249
98. Brusca SB, Abramson SB, Scher JU. Microbiome and mucosal inflammation as extra-articular triggers for rheumatoid arthritis and autoimmunity. Curr Opin Rheumatol (2014) 26(1):101–7. doi: 10.1097/BOR.0000000000000008
99. Lu DR, McDavid AN, Kongpachith S, Lingampalli N, Glanville J, Ju CH, et al. T Cell-dependent affinity maturation and innate immune pathways differentially drive autoreactive b cell responses in rheumatoid arthritis. Arthritis Rheumatol (2018) 70(11):1732–44. doi: 10.1002/art.40578
100. Kim JE, Chae CS, Kim GC, Hwang W, Hwang JS, Hwang JS, et al. Lactobacillus helveticus suppresses experimental rheumatoid arthritis by reducing inflammatory T cell response. Food Funct (2015) 13(2):350–62. doi: 10.1016/j.jff.2015.01.002
101. Vaahtovuo J, Munukka E, Korkeamäki M, Luukkainen R, Toivanen P. Fecal microbiota in early rheumatoid arthritis. J Rheumatol (2008) 35(8):1500–5. doi: 10.1097/RHU.0b013e31818277fb
102. Mena-Vázquez N, Ruiz-Limón P, Moreno-Indias I, Manrique-Arija S, Tinahones FJ, Fernández-Nebro A. Expansion of rare and harmful lineages is associated with established rheumatoid arthritis. J Clin Med (2020) 9(4):1044. doi: 10.3390/jcm9041044
103. Kinashi Y, Hase K. Partners in leaky gut syndrome: Intestinal dysbiosis and autoimmunity. Front Immunol (2021) 12:673708. doi: 10.3389/fimmu.2021.673708
104. Fan Z, Yang B, Ross RP, Stanton C, Zhao J, Zhang H, et al. The prophylactic effects of different lactobacilli on collagen-induced arthritis in rats. Food Funct (2020) 11(4):3681–94. doi: 10.1039/c9fo02556a
105. Yamashita M, Matsumoto K, Endo T, Ukibe K, Hosoya T, Matsubara Y, et al. Preventive effect of lactobacillus helveticus SBT2171 on collagen-induced arthritis in mice. Front Microbiol (2017) 8:1159. doi: 10.3389/fmicb.2017.01159
106. Fan Z, Yang B, Ross RP, Stanton C, Shi G, Zhao J, et al. Protective effects of bifidobacterium adolescentis on collagen-induced arthritis in rats depend on timing of administration. Food Funct (2020) 11(5):4499–511. doi: 10.1039/d0fo00077a
107. Shadnoush M, Nazemian V, Manaheji H, Zaringhalam J. The effect of orally administered probiotics on the behavioral, cellular, and molecular aspects of adjuvant-induced arthritis. Basic Clin Neurosci (2018) 9(5):325–36. doi: 10.32598/bcn.9.5.325
108. Pan H, Guo R, Ju Y, Wang Q, Zhu J, Xie Y, et al. A single bacterium restores the microbiome dysbiosis to protect bones from destruction in a rat model of rheumatoid arthritis. Microbiome. (2019) 7(1):107. doi: 10.1186/s40168-019-0719-1
109. Fan XX, Pan HD, Li Y, Guo RJ, Leung EL, Liu L. Novel therapeutic strategy for cancer and autoimmune conditions: Modulating cell metabolism and redox capacity. Pharmacol Ther (2018) 191:148–61. doi: 10.1016/j.pharmthera.2018.06.010
110. Boehncke WH, Schön MP. Psoriasis. Lancet (2015) 386(9997):983–94. doi: 10.1016/S0140-6736(14)61909-7
111. Komine M. Recent advances in psoriasis research; the clue to mysterious relation to gut microbiome. Int J Mol Sci (2020) 21(7):2582. doi: 10.3390/ijms21072582
112. Haghikia A, Jörg S, Duscha A, Berg J, Manzel A, Waschbisch A, et al. Dietary fatty acids directly impact central nervous system autoimmunity via the small intestine. Immunity. (2016) 44(4):951–3. doi: 10.1016/j.immuni.2016.04.006
113. Hayashi A, Sato T, Kamada N, Mikami Y, Matsuoka K, Hisamatsu T, et al. A single strain of clostridium butyricum induces intestinal IL-10-producing macrophages to suppress acute experimental colitis in mice. Cell Host Microbe (2013) 13(6):711–22. doi: 10.1016/j.chom.2013.05.013
114. Lucas S, Omata Y, Hofmann J, Böttcher M, Iljazovic A, Sarter K, et al. Short-chain fatty acids regulate systemic bone mass and protect from pathological bone loss. Nat Commun (2018) 9(1):55. doi: 10.1038/s41467-017-02490-4
115. Mariño E, Richards JL, McLeod KH, Stanley D, Yap YA, Knight J, et al. Gut microbial metabolites limit the frequency of autoimmune T cells and protect against type 1 diabetes. Nat Immunol (2017) 18(5):552–62. doi: 10.1038/ni.3713
116. Bellone M, Brevi A, Huber S. Microbiota-propelled T helper 17 cells in inflammatory diseases and cancer. Microbiol Mol Biol Rev (2020) 84(2):e00064–19. doi: 10.1128/MMBR.00064-19
117. Bian X, Wu W, Yang L, Lv L, Wang Q, Li Y, et al. Administration of akkermansia muciniphila ameliorates dextran sulfate sodium-induced ulcerative colitis in mice. Front Microbiol (2019) 10:2259. doi: 10.3389/fmicb.2019.02259
118. Scher JU, Ubeda C, Artacho A, Attur M, Isaac S, Reddy SM, et al. Decreased bacterial diversity characterizes the altered gut microbiota in patients with psoriatic arthritis, resembling dysbiosis in inflammatory bowel disease. Arthritis Rheumatol (2015) 67(1):128–39. doi: 10.1002/art.38892
119. Aggarwal A, Sarangi AN, Gaur P, Shukla A, Aggarwal R. Gut microbiome in children with enthesitis-related arthritis in a developing country and the effect of probiotic administration. Clin Exp Immunol (2017) 187(3):480–9. doi: 10.1111/cei.12900
120. Viladomiu M, Kivolowitz C, Abdulhamid A, Dogan B, Victorio D, Castellanos JG, et al. IgA-coated e. coli enriched in crohn’s disease spondyloarthritis promote TH17-dependent inflammation. Sci Transl Med (2017) 9(376):eaaf9655. doi: 10.1126/scitranslmed.aaf9655
121. Deng Y. Research on the alleviation effect of probiotics on psoriasis vulgaris. Jiangnan University. doi: 10.27169/d.cnki.gwqgu.2021.001780(in chinese)
122. Dean LE, Jones GT, MacDonald AG, Downham C, Sturrock RD, Macfarlane GJ. Global prevalence of ankylosing spondylitis. Rheumatol (Oxford) (2014) 53(4):650–7. doi: 10.1093/rheumatology/ket387
123. Zambrano-Zaragoza JF, Agraz-Cibrian JM, González-Reyes C, Durán-Avelar Mde J, Vibanco-Pérez N. Ankylosing spondylitis: from cells to genes. Int J Inflam (2013) 2013:501653. doi: 10.1155/2013/501653
124. Rehaume LM, Mondot S, Aguirre de Cárcer D, Velasco J, Benham H, Hasnain SZ, et al. ZAP-70 genotype disrupts the relationship between microbiota and host, leading to spondyloarthritis and ileitis in SKG mice. Arthritis Rheumatol (2014) 66(10):2780–92. doi: 10.1002/art.38773
125. Costello ME, Ciccia F, Willner D, Warrington N, Robinson PC, Gardiner B, et al. Brief report: Intestinal dysbiosis in ankylosing spondylitis. Arthritis Rheumatol (2015) 67(3):686–91. doi: 10.1002/art.38967
126. Wen C, Zheng Z, Shao T, Liu L, Xie Z, Le Chatelier E, et al. Quantitative metagenomics reveals unique gut microbiome biomarkers in ankylosing spondylitis. Genome Biol (2017) 18(1):142. doi: 10.1186/s13059-017-1271-6
127. Lin P, Bach M, Asquith M, Lee AY, Akileswaran L, Stauffer P, et al. HLA-B27 and human β2-microglobulin affect the gut microbiota of transgenic rats. PloS One (2014) 9(8):e105684. doi: 10.1371/journal.pone.0105684
128. Maupin-Furlow J. Proteasomes and protein conjugation across domains of life. Nat Rev Microbiol (2011) 10(2):100–11. doi: 10.1038/nrmicro2696
129. Amdekar S, Singh V, Singh R, Sharma P, Keshav P, Kumar A. Lactobacillus casei reduces the inflammatory joint damage associated with collagen-induced arthritis (CIA) by reducing the pro-inflammatory cytokines: Lactobacillus casei: COX-2 inhibitor. J Clin Immunol (2011) 31(2):147–54. doi: 10.1007/s10875-010-9457-7
130. Fan HF, Fang XY, Wu HL, Xu YQ, Gong LC, Yu DR, et al. Effects of stephania hainanensis alkaloids on MSU-induced acute gouty arthritis in mice. BMC Complement Med Ther (2021) 21(1):202. doi: 10.1186/s12906-021-03364-5
131. Molla MD, Bekele A, Melka DS, Teklemariam MD, Challa F, Ayelign B, et al. Hyperuricemia and its associated factors among adult staff members of the Ethiopian public health institute, Ethiopia. Int J Gen Med (2021) 14:1437–47. doi: 10.2147/IJGM.S308158
132. Schlesinger N, Brunetti L, Androulakis IP. Does seasonality of the microbiota contribute to the seasonality of acute gout flare? Clin Exp Rheumatol (2022). doi: 10.55563/clinexprheumatol/hdtge7
133. Larsen JM. The immune response to prevotella bacteria in chronic inflammatory disease. Immunology (2017) 151(4):363–74. doi: 10.1111/imm.12760
134. Buffie CG, Pamer EG. Microbiota-mediated colonization resistance against intestinal pathogens. Nat Rev Immunol (2013) 13(11):790–801. doi: 10.1038/nri3535
135. Ke ST. Distribution characteristics of syndrome elements and correlation with intestinal flora in patients with acute gout attack. Fuzhou: Fujian University of Traditional Chinese Medicine (2020).
136. Ren KY, Yong CM, Jin YC. Analysis of intestinal flora in patients with hyperuricemia in qingdao district. J Chin Physician (2014) 16(12):1649–1651, 1656. doi: 10.3760/cma.j.issn.1008-1372.2014.12.018
137. Guo Z, Zhang J, Wang Z, Ang KY, Huang S, Hou Q, et al. Intestinal microbiota distinguish gout patients from healthy humans. Sci Rep (2016) 6:20602. doi: 10.1038/srep20602
138. Shao T, Shao L, Li H, Xie Z, He Z, Wen C. Combined signature of the fecal microbiome and metabolome in patients with gout. Front Microbiol (2017) 8:268. doi: 10.3389/fmicb.2017.00268
139. Xing SC, Meng DM, Chen Y, Jiang G, Liu XS, Li N, et al. Study on the diversity of bacteroides and clostridium in patients with primary gout. Cell Biochem Biophys (2015) 71(2):707–15. doi: 10.1007/s12013-014-0253-5
140. Liu QP, Yu YR, Li HC. Regulation of QuzhuoTongbiPreseription on gut microbiota of model rats with abnormal uric acid metabolism. Chin J Tradit Chin Med Pharm (2019) 34(4):1722–6. doi: CNKI:SUN:BXYY.0.2019-04-114
141. Xu D, Lv Q, Wang X, Cui X, Zhao P, Yang X, et al. Hyperuricemia is associated with impaired intestinal permeability in mice. Am J Physiol Gastroint Liver Physiol (2019) 317(4):G484–92. doi: 10.1152/ajpgi.00151.2019
142. Jin C, Xu MZ. Association between gut microbiota and hyperuricemia and gout. Chin J Microecol (2019) 31(8):980–4.
143. Hunter DJ, Bierma-Zeinstra S. Osteoarthritis. Lancet. (2019) 393(10182):1745–59. doi: 10.1016/S0140-6736(19)30417-9
144. Vina ER, Kwoh CK. Epidemiology of osteoarthritis: literature update. Curr Opin Rheumatol (2018) 30(2):160–7. doi: 10.1097/BOR.0000000000000479
145. Ferreira RM, Duarte JA, Gonçalves RS. Non-pharmacological and non-surgical interventions to manage patients with knee osteoarthritis: An umbrella review. Acta Reumatol Port (2018) 43(3):182–200.
146. Zhang XZ, Zhang D, Ma YF. Effects of TongluoZhitong gel preparation on knee osteoarthritis in rats. China J Tradit Chin Med Pharm (2019) 5):2184–8. doi: CNKI:SUN:BXYY.0.2019-05-071
147. Ulici V, Kelley KL, Azcarate-Peril MA, Cleveland RJ, Sartor RB, Schwartz TA, et al. Osteoarthritis induced by destabilization of the medial meniscus is reduced in germ-free mice. Osteoarthr Cartilage (2018) 26(8):1098–109. doi: 10.1016/j.joca.2018.05.016
148. Sjögren K, Engdahl C, Henning P, Lerner UH, Tremaroli V, Lagerquist MK, et al. The gut microbiota regulates bone mass in mice. J Bone Miner Res (2012) 27(6):1357–67. doi: 10.1002/jbmr.1588
149. Cypers H, Van Praet L, Varkas G, Elewaut D. Relevance of the gut/joint axis for the management of spondyloarthritis in daily clinical practice. Curr Opin Rheumatol (2014) 26(4):371–6. doi: 10.1097/BOR.0000000000000070
150. Kamada N, Seo SU, Chen GY, Núñez G. Role of the gut microbiota in immunity and inflammatory disease. Nat Rev Immunol (2013) 13(5):321–35. doi: 10.1038/nri3430
151. Kamada N, Chen GY, Inohara N, Núñez G. Control of pathogens and pathobionts by the gut microbiota. Nat Immunol (2013) 14(7):685–90. doi: 10.1038/ni.2608
152. Fortuna R, Hart DA, Sharkey KA, Schachar RA, Johnston K, Reimer RA. Effect of a prebiotic supplement on knee joint function, gut microbiota, and inflammation in adults with co-morbid obesity and knee osteoarthritis: study protocol for a randomized controlled trial. Trials. (2021) 22(1):255. doi: 10.1186/s13063-021-05212-w
153. Rios JL, Bomhof MR, Reimer RA, Hart DA, Collins KH, Herzog W. Protective effect of prebiotic and exercise intervention on knee health in a rat model of diet-induced obesity. Sci Rep (2019) 9(1):3893. doi: 10.1038/s41598-019-40601-x
154. Korotkyi OH, Vovk AA, Dranitsina AS, Falalyeyeva TM, Dvorshchenko KO, Fagoonee S, et al. The influence of probiotic diet and chondroitin sulfate administration on Ptgs2, Tgfb1 and Col2a1 expression in rat knee cartilage during monoiodoacetate-induced osteoarthritis. Minerva Med (2019) 110(5):419–24. doi: 10.23736/S0026-4806.19.06063-4
155. Henrotin Y, Patrier S, Pralus A, Roche M, Nivoliez A. Protective actions of oral administration of bifidobacterium longum CBi0703 in spontaneous osteoarthritis in dunkin Hartley Guinea pig model. Cartilage (2021) 13(2_suppl):1204S–13S. doi: 10.1177/1947603519841674
156. Schott EM, Farnsworth CW, Grier A, Lillis JA, Soniwala S, Dadourian GH, et al. Targeting the gut microbiome to treat the osteoarthritis of obesity. JCI Insight (2018) 3(8):e95997. doi: 10.1172/jci.insight.95997
157. Korotkyi OH, Dvorshchenko KO, Kot LI. The combination of chondroitin sulfate and probiotic prevents oxidative stress in the serum of rats with experimental osteoarthritis. Minerva Biotechnol Biomol Res (2021) 33(2):93–101. doi: 10.23736/S2724-542X.21.02774-7
158. Sophocleous A, Azfer A, Huesa C, Stylianou E, Blanco GR, Ralston SH. Probiotics prevent cartilage damage and progression of osteoarthritis in mice. Bone (2020). doi: 10.1016/j.bonr.2020.100666
159. Sen ES, Ramanan AV. Juvenile idiopathic arthritis-associated uveitis. Clin Immunol (2020) 211:108322. doi: 10.1016/j.clim.2019.108322
160. Onel K, Rumsey DG, Shenoi S. Juvenile idiopathic arthritis treatment updates. Rheum Dis Clin North Am (2021) 47(4):545–63. doi: 10.1016/j.rdc.2021.07.009
161. Martini A, Lovell DJ, Albani S, Brunner HI, Hyrich KL, Thompson SD, et al. Juvenile idiopathic arthritis. Nat Rev Dis Primers (2022) 8(1):5. doi: 10.1038/s41572-021-00332-8
162. Shiff NJ, Beukelman T. Pharmacosurveillance in juvenile idiopathic arthritis. Rheum Dis Clin North Am (2021) 47(4):643–53. doi: 10.1016/j.rdc.2021.07.012
163. McCurdy D, Parsa MF. Updates in juvenile idiopathic arthritis. Adv Pediatr (2021) 68:143–70. doi: 10.1016/j.yapd.2021.05.014
164. Mahmud SA, Binstadt BA. Autoantibodies in the pathogenesis, diagnosis, and prognosis of juvenile idiopathic arthritis. Front Immunol (2019) 9:3168. doi: 10.3389/fimmu.2018.03168
165. Dückers G. Juvenile idiopathic arthritis. DtschArztebl Int (2020) 117(35-36):599. doi: 10.3238/arztebl.2020.0599b
166. De Filippo C, Di Paola M, Giani T, Tirelli F, Cimaz R. Gut microbiota in children and altered profiles in juvenile idiopathic arthritis. J Autoimmun (2019) 98:1–12. doi: 10.1016/j.jaut.2019.01.001
167. Arvonen M, Berntson L, Pokka T, Karttunen TJ, Vähäsalo P, Stoll ML. Gut microbiota-host interactions and juvenile idiopathic arthritis. PediatrRheum Online J (2016) 14(1):44. doi: 10.1186/s12969-016-0104-6
168. Tejesvi MV, Arvonen M, Kangas SM, Keskitalo PL, Pirttilä AM, Karttunen TJ, et al. Faecal microbiome in new-onset juvenile idiopathic arthritis. Eur J Clin Microbiol Infect Dis (2016) 35(3):363–70. doi: 10.1007/s10096-015-2548-x
169. Stoll ML, Kumar R, Morrow CD, Lefkowitz EJ, Cui X, Genin A, et al. Altered microbiota associated with abnormal humoral immune responses to commensal organisms in enthesitis-related arthritis. Arthritis Res Ther (2014) 16(6):486. doi: 10.1186/s13075-014-0486-0
170. Di Paola M, Cavalieri D, Albanese D, Sordo M, Pindo M, Donati C, et al. Alteration of fecal microbiota profiles in juvenile idiopathic arthritis. associations with HLA-B27 allele and disease status. Front Microbiol (2016) 7:1703. doi: 10.3389/fmicb.2016.01703
171. Jacobson JL, Pham JT. Juvenile idiopathic arthritis: A focus on pharmacologic management. J Pediatr Health Care (2018) 32(5):515–28. doi: 10.1016/j.pedhc.2018.02.005
Keywords: inflammatory arthritis, hyperuricemia and gout, inflammatory bowel disease arthritis, juvenile idiopathic arthritis, osteoarthritis, osteoporosis and osteopenia, spondyloarthritis, probiotics
Citation: Zeng L, Deng Y, He Q, Yang K, Li J, Xiang W, Liu H, Zhu X and Chen H (2022) Safety and efficacy of probiotic supplementation in 8 types of inflammatory arthritis: A systematic review and meta-analysis of 34 randomized controlled trials. Front. Immunol. 13:961325. doi: 10.3389/fimmu.2022.961325
Received: 04 June 2022; Accepted: 22 August 2022;
Published: 23 September 2022.
Edited by:
Severine Navarro, QIMR Berghofer Medical Research Institute, The University of Queensland, AustraliaReviewed by:
Arthur C. Ouwehand, International Flavors and Fragrances, FinlandBhagavathi Sundaram Sivamaruthi, Chiang Mai University, Thailand
Copyright © 2022 Zeng, Deng, He, Yang, Li, Xiang, Liu, Zhu and Chen. This is an open-access article distributed under the terms of the Creative Commons Attribution License (CC BY). The use, distribution or reproduction in other forums is permitted, provided the original author(s) and the copyright owner(s) are credited and that the original publication in this journal is cited, in accordance with accepted academic practice. No use, distribution or reproduction is permitted which does not comply with these terms.
*Correspondence: Liuting Zeng, zltab2016@hotmail.com; Kailin Yang, yklab2014@hotmail.com; Ying Deng, yingdeng321@outlook.com; Hua Chen, chhuaa332211@163.com; Xiaofei Zhu, 864084847@qq.com
†These authors share first authorship