Corrigendum: Microbiota dynamics, metabolic and immune interactions in the cervicovaginal environment and their role in spontaneous preterm birth
- 1Department of Pathology, Aga Khan University, Nairobi, Kenya
- 2Centre of Excellence Women and Child Health, Aga Khan University, Nairobi, Kenya
- 3Faculty of Life Sciences and Medicine, Department of Women and Children’s Health, School of Life Course and Population Sciences, King’s College London, London, United Kingdom
Differences in the cervicovaginal microbiota are associated with spontaneous preterm birth (sPTB), a significant cause of infant morbidity and mortality. Although establishing a direct causal link between cervicovaginal microbiota and sPTB remains challenging, recent advancements in sequencing technologies have facilitated the identification of microbial markers potentially linked to sPTB. Despite variations in findings, a recurring observation suggests that sPTB is associated with a more diverse and less stable vaginal microbiota across pregnancy trimesters. It is hypothesized that sPTB risk is likely to be modified via an intricate host-microbe interactions rather than due to the presence of a single microbial taxon or broad community state. Nonetheless, lactobacilli dominance is generally associated with term outcomes and contributes to a healthy vaginal environment through the production of lactic acid/maintenance of a low pH that excludes other pathogenic microorganisms. Additionally, the innate immunity of the host and metabolic interactions between cervicovaginal microbiota, such as the production of bacteriocins and the use of proteolytic enzymes, exerts a profound influence on microbial populations, activities, and host immune responses. These interplays collectively impact pregnancy outcomes. This review aims to summarize the complexity of cervicovaginal environment and microbiota dynamics, and associations with bacterial vaginosis and sPTB. There is also consideration on how probiotics may mitigate the risk of sPTB and bacterial vaginosis.
1 Introduction
Extensive research has detailed microbiota residing in many human body sites and their contribution to health and disease (1–4). However, within the cervicovaginal despite much research (5, 6) there remains a lack of clinical impact in terms of improving pregnancy outcomes.
Many have reported differences in the cervicovaginal environment in relation to risk of spontaneous preterm birth (sPTB). Mechanistically, a more diverse and inflammatory environment may lead to cervical shortening and an increased risk of microbial invasion of the gravid uterus (7, 8). However, many of the studies of cervicovaginal microbiota are geographically confined, limited in terms of clinical phenotyping, and include study of heterogenous populations; these limitations may have led to overly simplified interpretation of findings. For example, a Lactobacillus crispatus dominant environment in pregnancy is often reported as driver of a healthy pregnancy (9–13), with a more diverse environment (associated with the transition to Lactobacillus iners dominance) associated with sPTB (11, 14). This may be the case for European dominated populations (15, 16), but it is unclear whether a diverse cervicovaginal microbiota confers a similar risk in other geographically dispersed populations. As such, there is a need to study women from a range of geographical locations and environments, and to develop a deeper understanding of the nuances of bacterial community structure. This is particularly important as no one bacterial species in presence or absence has been consistently associated with poor pregnancy outcomes such as sPTB. The intricate dynamics within a bacterial community, the potential presence of viruses and the distinct metabolic strategies employed by different bacterial species might collectively exert influence over the determination of risk status (13, 17). Such knowledge would provide a greater understanding and context for treatment strategies, such as targeted probiotic interventions. In addition, future studies could integrate knowledge of cervicovaginal environment status with other maternal exposures and deeper phenotyping to acknowledge sPTB as a syndrome, and that the impact of the vaginal microbiota on sPTB risk may be limited to a sub-group of women only.
This review centers on: (1) cervicovaginal microbiota composition and patterns; (2) cervicovaginal microbial metabolites; (3) cervicovaginal bacteria defense mechanisms like bacteriocins, hydrogen peroxide, and biosurfactants in influencing pregnancy outcomes, including sPTB; and (4) effectiveness of interventions such as probiotics for preventing adverse pregnancy outcomes like preterm births.
1.1 Cervicovaginal microbiota and environment
The cervicovaginal environment, along with female genital tract as a whole accommodates a microbial biomass, estimated to be 1010–1011 bacteria for women of reproductive age (18), and comprises many bacterial species (13, 18, 19). The classification of these microbes into five distinct community state types (CSTs) (20–22) has undergone refinement in response to advances in metagenomics sequencing and enhanced representation of samples (23, 24). Four CSTs are dominated by lactobacilli namely; Lactobacillus crispatus (CST I), Lactobacillus gasseri (CST II), Lactobacillus iners (CST III), or Lactobacillus jensenii (CST V), while the fifth (CST IV) is characterized by lower proportions of lactic acid-producing bacteria and higher proportions of strict anaerobes (9, 20, 22, 25). Numerous studies have shown the cervicovaginal microbiota of most women is primarily dominated by lactobacilli (20, 24, 26, 27). A study of the vaginal bacterial communities of 396 asymptomatic North American women from diverse ethnic backgrounds (White, Black, Hispanic, and Asian populations) identified a lactobacilli dominance in 73% of women (20). In instances where lactobacilli are not dominant, other lactic acid-producing microorganisms like Atopobium, Megasphaera, Eggerthella-like bacterium, and/or Leptotrichia species may establish colonization (27, 28). These opportunistic pathogens have demonstrated distinct associations with conditions like bacterial vaginosis (BV), aerobic vaginosis (AV), and sPTB (12, 29–31), although their mere presence or absence is insufficient to explain causality. In a sub-optimal cervicovaginal microbiota environment, lactobacilli may lose their protective capacity to increased inflammation from opportunistic pathogens (32). Certain key genera, including members of Gardnerella clades, Atopobium, Prevotella, Peptostreptococcus, Mobiluncus, Sneathia, Leptotrichia, Megasphera spp, mycoplasma, have been identified as potential causative agents of sPTB (12, 33).
The cervicovaginal microbial environment is a summative reflection of microbial community and host interactions where several factors tend to influence the cervicovaginal microbiota and, by extension, sPTB (Figure 1). Among these is the cervicovaginal pH which is usually maintained in an acidic pH range (3.8 - 4.5) by microbial (85%) and cervicovaginal epithelial (15% [L-lactic acid]) production of lactic acid (34, 35). The interplay between cervicovaginal microbiota and other host environmental factors such as warm, humid, anaerobic, and nutrient-rich cervicovaginal environment, significantly modulates the growth and survival of the cervicovaginal microbiota (18). Cervicovaginal microbiota can equally be influenced by factors related to the host innate immunity (e.g., antimicrobial peptides, cytokines, neutrophils, and monocytes) (36), as well as conditions like diabetes (37), autoimmune disease (38), hormonal fluctuations (39, 40), age (41), sexual activity (42) and the use of antibiotics, the effects of which have been extensively documented (43). Any disruption to the ‘normal’ cervicovaginal microbiota can stimulate the overgrowth of opportunistic pathogens. This overgrowth can outweigh the beneficial microbiota, subsequently increasing the risk of infection (e.g. BV) and sPTB (31, 33).
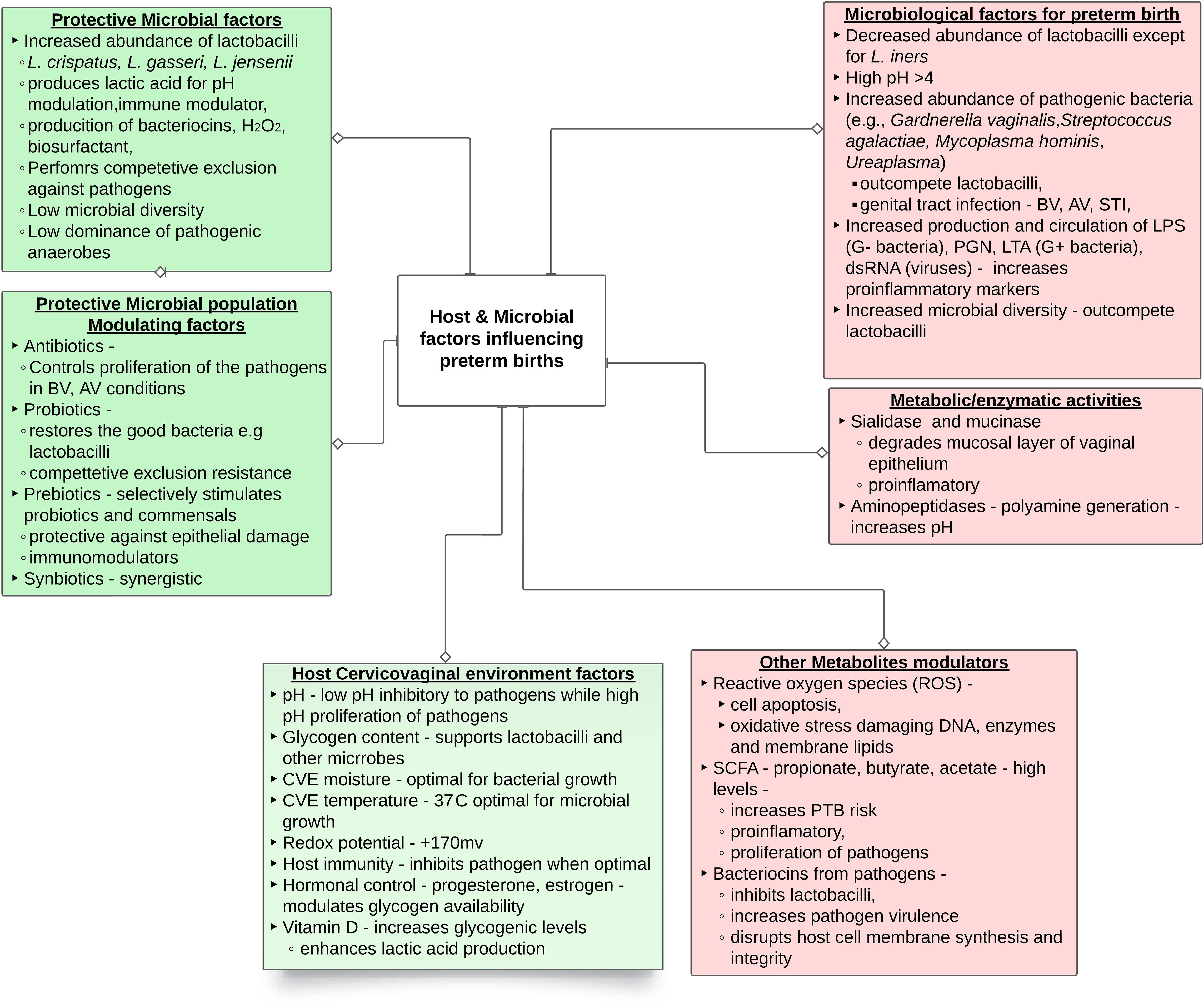
Figure 1 Summary of female genital microbiological factors influencing preterm birth. The abbreviations are dsRNA, double-stranded RNA; LPS, lipopolysaccharide; LTA, lipoteichoic acid; PGN, peptidoglycan; CVE – cervicovaginal environment; SCFA short chain fatty acid.
The menstrual cycle represents a significant influencing factor on the cervicovaginal microbiota (44, 45). Notably, the alkaline pH of the cervicovaginal environment during menstruation, has been linked to the promotion of suboptimal microbiota composition in most women (46). It has been observed that women with a predisposition to pregnancy failure, including those with recurrent miscarriage and recurrent implantation failure, consistently exhibit a reduced abundance of lactobacilli throughout all phases of the ovulation cycle (46). This phenomenon may contribute to increased bacterial diversity, particularly in conditions such as AV and BV which have been associated with increased sPTB risk (31, 33).
While extensive research has been directed towards bacteria, the cervicovaginal microbiota encompasses a dynamic and intricate ecosystem including protozoa, archaea, viruses, and fungi (47). Among these, viruses, including human immunodeficiency virus (HIV), herpes simplex virus-2, and human papilloma virus (HPV), appear to contribute to PTB (47–49); while the role of other viruses remains unclear. Similarly, most fungi, across genera such as Candida, Cladosporium, Pichia, Aspergillus, and Rhodotorula predominantly inhabit the cervicovaginal and may be pathogenic (50, 51). Evidently, further research is essential to comprehend the roles of protozoa, viruses, fungi, and archaea within the cervicovaginal microbiota. The ensuing sections of this review will focus predominantly on bacteria, to shed light on the intricate mechanisms underpinning interactions, shifts, and potential disruptions within cervicovaginal environment during pregnancy and their consequential effects on sPTB, as diagrammatically represented in Figure 1.
1.2 Cervicovaginal microbiota dynamics during pregnancy
The cervicovaginal microbial community is dynamic and undergoes significant changes during pregnancy (9, 52). These shifts, a result of microbial competition, along with hormonal, immunological, and metabolic interactions, hold potential to ultimately impact pregnancy outcomes. Women with normal pregnancies tend to exhibit a higher dominance of lactobacilli in their cervicovaginal microbiota compared to non-pregnant women (53). A retrospective longitudinal case-control study demonstrated a higher abundance of Limosilactobacillus vaginalis, L. crispatus, L. gasseri, and L. jensenii and a lower abundance of 22 other phylotypes in pregnant women (9). The microbiota composition, particularly the presence of lactobacilli, exhibited greater stability in pregnant women when compared to women who were not pregnant (9). This may explain the connection between lactobacilli and full-term pregnancy.
Within a predominantly African-American cohort, (n=474 women), longitudinal characterization of vaginal microbiota during pregnancy determined a decrease in richness and evenness of the vaginal microbiota from first through the third term of gestation (24). In addition, advancing gestational age corresponded with lactobacilli dominance, including a positive correlation with over 30 lactobacilli species and a negative correlation with opportunistic pathogens (24). This correlation highlights the critical role of lactobacilli in promoting term birth. However, a small study of 12 women identified a stable cervicovaginal microbiota, with no changes in lactobacilli (particularly L. crispatus and L. iners) observed before and during pregnancy (54).
Across trimesters, a lactobacilli-dominant microbiota by the second trimester has been proposed to be a marker for a stable environment conducive to term birth, irrespective of whether the starting cervicovaginal microbiota was simple or complex (55). DiGiulio and colleagues demonstrated a stable vaginal community state on the time scale of weeks but noted that some women maintained a stable CST throughout pregnancy, whereas others had relatively frequent transitions between CSTs. However, these shifts did not influence term and sPTB (25). The study further discovered that CSTs dominated by lactobacilli exhibited greater stability compared with those with frequent transitions. Moreover, both the duration and proportion of time spent in frequent transition were correlated with sPTB (25). These findings highlight the intricate interplay of vaginal microbiota without a direct correlation to delivery outcomes, prompting further exploration into the multifaceted factors that influence pregnancy and childbirth. In another longitudinal study, even though its primary focus was not on sPTB, the cervicovaginal microbiota of 57 women was sequenced at 24, 36 weeks of gestation and at birth. With advancing gestational age, the study unveiled an increase in the relative abundance of diverse bacterial taxa. A gradual decline in lactobacilli abundance which coincided with an increase in Enterococcus, Streptococcus, and bacteria within the Enterobacteriaceae family was also reported (56). These bacteria, commonly defines AV and has also been linked to sPTB (57).
Term birth has consistently been correlated with an abundance of lactobacilli, mainly due to their lactic acid production. This lactic acid plays a crucial role in maintaining an acidic pH within the cervicovaginal environment, promoting the growth of beneficial lactobacilli while inhibiting opportunistic pathogens (9). In the cervicovaginal environment, lactobacilli are the primary producers of lactic acid, which exists in two forms: L-type and D-type (58) from glycogen and its hydrolysates (59, 60). The former is produced by both cervicovaginal epithelial cells and bacteria, while the latter is predominantly bacterial-derived, accounting for over 85% of cervicovaginal lactic acid (35). Among the lactobacilli species, L. crispatus and L. gasseri are prominent in cervicovaginal metabolism, capable of producing both D and L-type lactic acid, while L. jensenii exclusively produces the D-type, and L. iners generates the L-type (60), emphasizing the influence of predominant lactobacilli species on lactic acid composition and the unique metabolic profile of the cervicovaginal environment. This intricate interplay between lactobacilli and lactic acid has wider implications for pregnancy outcomes. Elevated levels of L-lactic acid and the L:D-lactic acid ratio stimulate extracellular matrix metalloproteinase inducer (EMMPRIN, also known as CD147) and matrix metalloproteinase MMP-8 (60). EMMPRIN, a crucial protein in fetal development, modulates MMP-8 expression (61). MMP-8, functioning as an enzyme, degrades the extracellular matrix of cervicovaginal epithelial cells (60), potentially compromising cervical integrity and facilitating bacterial movement, thereby increasing the risk of sPTB (60). In cervicovaginal environments with lower lactobacilli dominance but an abundance of L. iners, L-lactic acid levels tend to be higher (60). This observation provides valuable insights into the relationship between L. iners and sPTB.
1.2.1 Hormonal influence on cervicovaginal microbiota and pregnancy
Throughout pregnancy up to the delivery of the placenta, the concentrations of progesterone and estrogen continue to rise [Farage, Miller, & Sobel, (62)]. Lactobacilli dominance in the cervicovaginal environment, influenced by estradiol and/or progesterone, is prevalent during reproductive years. However, in early stage of puberty and post menopause women often exhibit a more diverse microbiota with lactobacilli deficiency [Kaur et al., 45; Muhleisen & Herbst-Kralovetz, (63)] correlating with an increased risk of sPTB. Elevated estrogen levels stimulate glycolytic and lactic acid fermentation which play a crucial role in fostering lactobacilli growth and normal microbiota [Farage et al., (62); Kim & Park, (64)]. Conversely, in postmenopausal women, reduced estrogen secretion leads to a decline in protective lactobacilli such as L. crispatus, resulting in microbiota imbalance and increased colonization by microorganisms like G. vaginalis and Candida [Gustafsson et al., (65); Kim & Park, (64)].
The delicate balance between progesterone and estrogen is paramount. Progesterone withdrawal triggers increased expression of estrogen-dependent receptors, including oxytocin, gap junction protein connexin 43 (CX-43), cyclooxygenase-2 (COX-leading to prostaglandin production), prostaglandin, and myosin light chain kinase (MLCK) receptors (66). This cascade promotes uterine contractility, emerging as a potential risk factor for sPTB. Notably, vitamin D, with progesterone-like activity, is implicated in maintaining and reinforcing the cervicovaginal epithelium through the induction of the antimicrobial peptide LL-37 (67). Additionally, vitamin D regulates insulin synthesis, a crucial hormone for glycogen metabolism. Vitamin D deficiency during pregnancy is associated with an increased risk of sPTB due to its impact on glycogen metabolism (67, 68).
1.3 Preterm birth and infection
Preterm birth (PTB), defined as childbirth occurring before 37 weeks of gestation, constitutes approximately 10-15% of global births (69, 70). PTB contributes significantly to neonatal morbidity and mortality, and its consequences can extend into adulthood (71). Approximately one-third of PTBs are medically indicated, often due to maternal or fetal conditions like preeclampsia or growth restriction, while the remaining are spontaneous PTBs, with 25-30% involving spontaneous preterm labor, with or without prelabor rupture of fetal membranes (PPROM) (72).
Our primary focus is how cervicovaginal microbiota modulate the risk of sPTB, recognizing that sPTB is a complex syndrome influenced by multiple factors, including intra-amniotic infections, ascending infections, cervical insufficiency, stress, vascular disorders, maternal age, multiple pregnancies, nutrition, immunity, and lifestyle behaviors (72–74). Cervicovaginal infections during pregnancy often manifest as polymicrobial rather than single bacterial species. About 35% of sPTB cases are associated with infections and inflammation (75). Key microorganisms in this context include C. trachomatis, Enterococcus spp., Gardnerella vaginalis (G. vaginalis), Mycoplasma hominis, Ureaplasma parvum (U. parvum), and Ureaplasma urealyticum (U. urealyticum), which can significantly influence cervical health and pregnancy outcomes (12, 57, 76–79). Shedding light on this intricate relationship, a study conducted in pregnant Caucasian women identified a microbial community (CST IV) characterized by an enrichment of facultative anaerobes and a low abundance of lactobacilli (80). This specific microbial composition has been associated with both cervical shortening and an elevated risk of sPTB (80). In this context, U. urealyticum emerges as the commonly isolated microorganism in the cervix of patients experiencing PTB, with a prevalence estimated at 34.5%, followed closely by Enterococcus spp. at 27.6% and Mycoplasma hominis at 17.2%. Additionally, PCR analysis has identified U. parvum as the dominant pathogen in the cervix, accounting for 85.5%, followed by Chlamydia trachomatis (C. trachomatis) at 8.0% (81), confirming the importance of Ureaplasma spp. in endocervical infection and its association with placental inflammation (82), pPROM and sPTB (83). Similarly, the prevalence of C. trachomatis has been observed to increase among women experiencing preterm labor, particularly those with PTB, compared with women who gave birth at term (84). This became apparent when women with C. trachomatis receiving antibiotic treatment showed a reduced incidence of sPTB (85). However, there is some inconsistency regarding the impact of C. trachomatis on sPTB. In a case-control study involving 75 Iranian women with sPTB, no significant association with C. trachomatis cervical infection was observed when compared with 75 women with term births (86). Nevertheless, women with cervical C. trachomatis infection who received antibiotic treatment had lower rates of pPROM, sPTB, and small-for-gestational-age infants compared with those who did not receive antibiotic treatment (87, 88), providing insight into the role of C. trachomatis in promoting sPTB.
G. vaginalis is another important cervical microorganism, and its abundance has been linked with microbial invasion of the amniotic cavity, consequently promoting pPROM (89). Interestingly, a case-control study involving 203 pregnant Korean women in mid-pregnancy revealed a contradictory finding, associating G. vaginalis with term birth (90). This study also highlighted a positive correlation between Ureaplasma and Prevotella and sPTB but only in the absence of lactobacilli (90); highlighting the need to examine the cervicovaginal microbiota as a community.
Moreover, there is a significant variation in the risk of sPTB linked to cervicovaginal infections at different times during pregnancy. In the second trimester, when the cervix is typically stable, such infections do not significantly raise the sPTB risk. However, infections in the third trimester, when the cervix naturally prepares for labor, show a marked increase in the risk, especially when they coincide with premature cervical ripening (91). This temporal distinction underscores the critical importance of considering pregnancy stage when assessing the impact of cervicovaginal infections on sPTB risk.
1.4 Cervicovaginal microbiota, race, and ethnicity, as sPTB risk factors
As stated, elevated risk of sPTB has been associated with specific microbiota compositions in the cervicovaginal, including reduced abundance of L. crispatus and increased dominance of L. iners in European and American populations (6, 15, 16). Recent studies have revealed distinct cervicovaginal microbiota based on geographical locations, emphasizing the importance of considering geographical diversity in cervicovaginal microbiota research to better comprehend sPTB risk factors. When comparing the cervicovaginal microbiota profiles of African American and Caucasian women, 30-60% of African-American, African, and Hispanic women are more likely to possess diverse cervicovaginal microbial communities with low lactobacilli dominance, increasing their risk of sPTB (15, 31, 92). This knowledge gap is significant because 80% of sPTB occur in developing countries, possibly due to the high prevalence of vaginal infections like AV and BV, coupled with inadequate healthcare facilities, as reported among sub-Saharan African women (93).
The influence of cervicovaginal microbiota on pregnancy outcomes in African women remains unclear. The cervicovaginal environment of approximately 16% of pregnant women in Pemba Island, Tanzania, are colonized by at least three of four STI (sexually transmitted infection) infectious agents (i.e., Chlamydia trachomatis, Trichomonas vaginalis, Neisseria gonorrhoeae, and Mycoplasma genitalium); therefore, adverse pregnancy outcomes may not necessarily be instigated by a single infectious agent (79). In Kenya, more than fifty percent of the vaginal microbiota consisted of Gardnerella and/or Prevotella, with lactobacilli ranging from 1% – 99%, but without a clear link between variation in composition and term or sPTB (94). In a longitudinal study involving pregnant Nigerian women (n=38), the prevalent cervicovaginal bacterium was L. iners (40). Noteworthy, this prevalence of L. iners was particularly pronounced in most women who ultimately delivered at full term, challenging a direct association between L. iners and sPTB (40). The presence of L. iners in the cervicovaginal environment indicates it is a regular part of a healthy vaginal microbiota.
1.5 Cervicovaginal metabolic interaction and link with sPTB
It is important to note that the causality of sPTB cannot be solely attributed to the presence or absence of specific bacteria. The composition of the cervicovaginal microbiota and the diversity of microbial species within it, plays a significant role in shaping the metabolite profile within the cervicovaginal environment (13, 60, 95) owing to the inherent metabolic capability of microbiota (96). These metabolites can influence cervicovaginal homeostasis (60, 95), yet the precise mechanisms by which their interaction impacts sPTB remain elusive. The dynamic metabolic ecosystem within the cervicovaginal environment encompasses diverse pathways involving carbohydrates and amino acids, contributing to its complex nature, believed to influence pregnancy outcomes. For example, the metabolomes of women who experienced sPTB and those who carried their pregnancies to term differ in 30 of 310 (9.7%) identified metabolites. Notably, mannitol and methylphosphate were upregulated, while 28 other metabolites, including medium-chain fatty acids and collagen degradation markers, were downregulated in women who had sPTB compared with term births (97). In a separate investigation involving 300 healthy pregnant women, women with a higher presence of L. jensenii CST V, as opposed to L. crispatus CST I (both considered health-promoting bacteria), in their vaginal fluid exhibited lower levels of lactate and glutamate. This subgroup, characterized by low glutamate and D-lactate levels and high acetate levels, was notably more prone to sPTB compared to those dominated by L. crispatus CST I, as confirmed by both a study involving this subgroup (98) and a prospective study with asymptomatic singleton women (99). Furthermore, the composition of metabolites in the cervicovaginal space changes over gestation; at 20-24 weeks and 24-28 weeks there are significant differences in 313 amino acids, carbohydrates, and peptide metabolites between women experiencing sPTB and term birth (100). However, the number of distinct metabolites reduced from 82 at 20-24 weeks to 48 at 24-28 weeks (100).
Some studies, like Melinda et al. [91], did not find significant metabolite differences between term birth and sPTB, potentially due to early sample collection. However, Gerson et al. [92] identified 11 metabolites associated with sPTB, including stearoyl-linoleoyl-glycerol, palmitoyl dihydrosphingomyelin, and others. Amino acid catabolites (biogenic amines) were increased in women with CST IV microbiota profiles, while asparagine was reduced compared to CST I profiles. These findings were corroborated in another study, where women with CST IV had elevated amino acid catabolites and 12-HETE [93]. In contrast, levels of certain amino acids, specifically glutamate and tyrosine, as well as dipeptides, were found to be low in this context, with implications for signaling cascades for host inflammation and disruption of epithelial barrier integrity; collectively these may contribute to the occurrence of sPTB (101). Another study involving 346 pregnant women examined during the first two trimesters, including 60 cases of sPTB, did not reveal disparities in metabolite profiles between women who experienced sPTB and those who carried their pregnancies to term (13). Nonetheless, the authors identify metabolite features that increased in cases of sPTB such as betaine, acetate, and calcium levels, whereas lactate and leucine were positively correlated with full-term births (13). Interestingly, aspartate seemed to have a protective effect in the presence of L. acidophilus, but increased the risk of sPTB when Bifidobacterium breve and L. delbrueckii were present (13).
While limited studies have explored metabolic relationships among microbes in the cervicovaginal environment concerning sPTB, a significant in vitro study by Horrocks et al. revealed a symbiotic and commensal relationship among bacteria commonly associated with BV infection and sPTB (102). Notably, Prevotella spp., such as P. bivia, a group of bacteria frequently linked to BV infections and sPTB, exhibited distinctive symbiotic connections with Peptostreptococcus anaerobius and G. vaginalis (102). P. bivia plays a pivotal role in this relationship by significantly releasing metabolites actively utilized by other BV-associated bacteria. These metabolites include succinate, fumarate, alanine, glutamate, glycine, methionine, phenylalanine, proline, valine, and uracil, highlighting the crucial role of P. bivia in cross-feeding within the cervicovaginal environment. Furthermore, P. bivia and P. anaerobius maintain a commensal relationship within cervicovaginal environment where P. bivia provides amino acids (threonine, tyrosine, phenylalanine, and proline), especially proline, to P. anaerobius, promoting increased glucose uptake and acetate production from glucose (102). It is thought that G. vaginalis, by aiding the ascension of P. bivia, enhances its invasive potential in the uterus (103). Additionally, P. bivia and G. vaginalis exhibit a mutualistic relationship, leading to increased production of acetate and aspartate, which has been linked to BV and sPTB (13, 99, 102). G. vaginalis closely associates with L. iners and less with other lactobacilli species, including L. crispatus, a profile known to be correlated with BV and sPTB (11, 13). Interestingly, L. iners is unable to produce acetate, suggesting that acetate production originates from other bacteria, including G. vaginalis (102). Therefore, it remains unclear how L. iners and G. vaginalis interact to promote sPTB.
Despite certain limitations in study design (e.g., non-representative media, microbiota, and host immunity), it is important to consider the diversity of metabolic relationships within and between bacterial species, rather than relying solely on ecological consistency based on marker genes in BV infection and sPTB.
1.6 Cervicovaginal microbiota instigated immune modulation on sPTB
The cervicovaginal microbiota significantly influences host immunity pregnancy outcomes, with interactions implicated in intrauterine infections (104). Microbial species, such as Enterobacteriaceae spp, Streptococcus spp, Staphylococcus spp, Escherichia coli, and Gram‐negative bacteria, may migrate through anatomical barriers, such as cervicovaginal or endometrial epithelia, triggering elevated production of microbial products like lipopolysaccharides and inflammasomes, altering cervicovaginal inflammatory responses (105). This heightened immune response, involves the activation of immune components such as the complement system (C3b and C5), mannose-binding lectin (MBL), immunoglobulins (IgM and IgG), alongside the production of pro-inflammatory cytokines (IL-8, IL-6, and IL-1β) (36). Immune alterations, particularly the association between the dominance of L. iners and elevated levels of IgM, C3b, C5, C5a, and IL-6, collectively contribute to an increased susceptibility to short cervix, compromised endometrial receptivity, implantation rates and increased susceptibility to sPTB (36, 105). Prevotella and Gardnerella, integral to BV, have also been shown to mediate cervicovaginal epithelium immune response. They have been demonstrated to activate NF-κB, induce the release of proinflammatory cytokines, including TNF-α, particularly through TLR2/4 pathways (106, 107), and elicit the NLRP3 inflammasome release via NOD-like receptors (NLRs) signaling pathways (108). This dual influence on TLR2/4 and NLRs expression apart from contributing to cervical inflammation, may also increase the risk of sPTB.
Neutrophils, which are involved in cervical remodeling can infiltrate the myometrium, potentially leading to cervical shortening during labor, to equally increase the susceptibility to sPTB (109, 110). From limited data, it appears that neutrophil counts in cervicovaginal fluid decrease as gestational age increases (111). Notably, women with a low diversity cervicovaginal microbiota, characterized by the dominance of L. iners (CST III), tend to exhibit an increased presence of neutrophils in the cervicovaginal environment during pregnancy (111, 112). While one study revealed a positive correlation between G. vaginalis (CST IV) and neutrophils, suggesting increased neutrophil presence in samples from women who eventually experienced sPTB (111), a study by Molina et al. study provided contrasting results (112). These conflicting results underscore the complexity of the relationship between the cervicovaginal microbiota and the neutrophil response during pregnancy.
Other than the presence or absence of key microbial species, cervicovaginal microbiota can also modulate immunity and pregnancy outcome via the production of lactic and short-chain fatty acids (SCFAs). Lactic acid can exert immune suppressive effects by inhibiting monocyte and cytotoxic CD8+ T cell differentiation, dendritic maturation, and macrophage polarization towards the M2 type (113). This potentially maintains the integrity of the cervix to promote term birth. Reduced lactic acid levels, often associated with a low presence of lactobacilli, can further contribute to elevated levels of inflammatory immune factors such as IL-6, IL-8, IL-1α, IL-1β, and MIP-1α/β (32) which can together compromise the cervix, potentially enabling sPTB.
Although SCFAs are recognized for their beneficial effects on the gut such as enhancing the integrity of the gut epithelial membrane (114), their impact on the cervicovaginal environment appears to be detrimental. SCFAs are organic fatty acids typically containing fewer than six carbon atoms. Common SCFAs include acetic acid (C=2), propionic acid (C=3), and butyric acid (C=4) (115). Elevated levels of SCFAs, such as propionate, succinate, acetate, and butyrate, are linked to increased inflammatory markers including IL-1β, IL-2, IL-6, IL-8, IL-10, TNF-α, Interferon (IFN)-γ, and RANTES (59, 116). Some of these inflammatory markers, for example acetate in early pregnancy, have been associated with sPTB in cases where the cervicovaginal microbiota is less dominated by L. crispatus (13). Furthermore, high SCFA levels can dampen the expression of an innate antibacterial factor known as neutrophil gelatinase-associated lipocalin (NGAL), a condition that can promote the growth of the BV community (117) to promote sPTB. In cases of BV, a high concentrations of acetic (100 mM) and butyric (20 mM) acids can specifically stimulate TLR1/2/3 in cervicovaginal epithelial cells, leading to increased secretion of the TNF-α while inhibiting the production of IL-6, RANTES, and IP-10 (34). Despite the observed effects, the exact impact of cervicovaginal microbiota on the immune responses of reproductive cells and its consequences on spontaneous preterm birth (sPTB) are not yet clear and require further clarification.
1.7 Other metabolites – hydrogen peroxide, bacteriocins, biosurfactant, sialic acid and sialidase activity
Beyond lactic acid and SCFAs, beneficial bacteria produce additional metabolites such as hydrogen peroxide, bacteriocins, and biosurfactants, all of which contribute substantially to maintain the health and homeostasis of the cervicovaginal environment (95).
1.7.1 Hydrogen peroxide
Hydrogen peroxide (H2O2) is a reactive oxygen species that is produced by specific lactic acid bacteria such as L. crispatus and L. jensenii, L. gasseri, and L. vaginalis (118) but not L. iners (119). The extent of H2O2 production varies among these bacteria, with higher production being observed with L. jensenii and L. vaginalis (118). Mechanistically, H2O2 can inhibit the growth of pathogenic microbes such as G. vaginalis and C. albicans by the oxidation of sulfhydryl groups in proteins and essential enzymes, DNA, and other cellular components (120). This reduction in viability hinders the ability of these opportunistic pathogens to adhere to the vaginal epithelium and cause ascending uterine infections (118). Moreover, the presence of H2O2-producing lactobacilli can also regulate the immune response by suppressing proinflammatory cytokines including interleukin-1β (121).
1.7.2 Microbiota bacteriocins as an agent of cervicovaginal microbiota modulation and sPTB
Bacteriocins as summarized in Table 1, are small peptides produced in favorable conditions by some cervicovaginal microbes and can kill or inhibit the growth of opportunistic pathogens. Examples include compounds such as crispacin produced by L. crispatus along with lactocin 160 and lactocin AL705 (122). Some disrupt the integrity of the target bacterial cell membrane, causing cell death, while others interfere with essential metabolic pathways, such as DNA, RNA, and protein metabolism inside the cell, thereby killing and/or inhibiting microbial growth (135). They are effective against several pathogenic bacteria, including G. vaginalis and Streptococcus agalactiae, which have closely been associated with BV and sPTB respectively (136). L. rhamnosus may significantly contribute to the reduction of sPTB risk posed by P. bivia and G. vaginalis. It can achieve this by producing lactocin 160 and AL705, which target the cytoplasmic membrane of these bacteria, leading to the efflux of ATP molecules and the dissipation of the proton motive force (132).
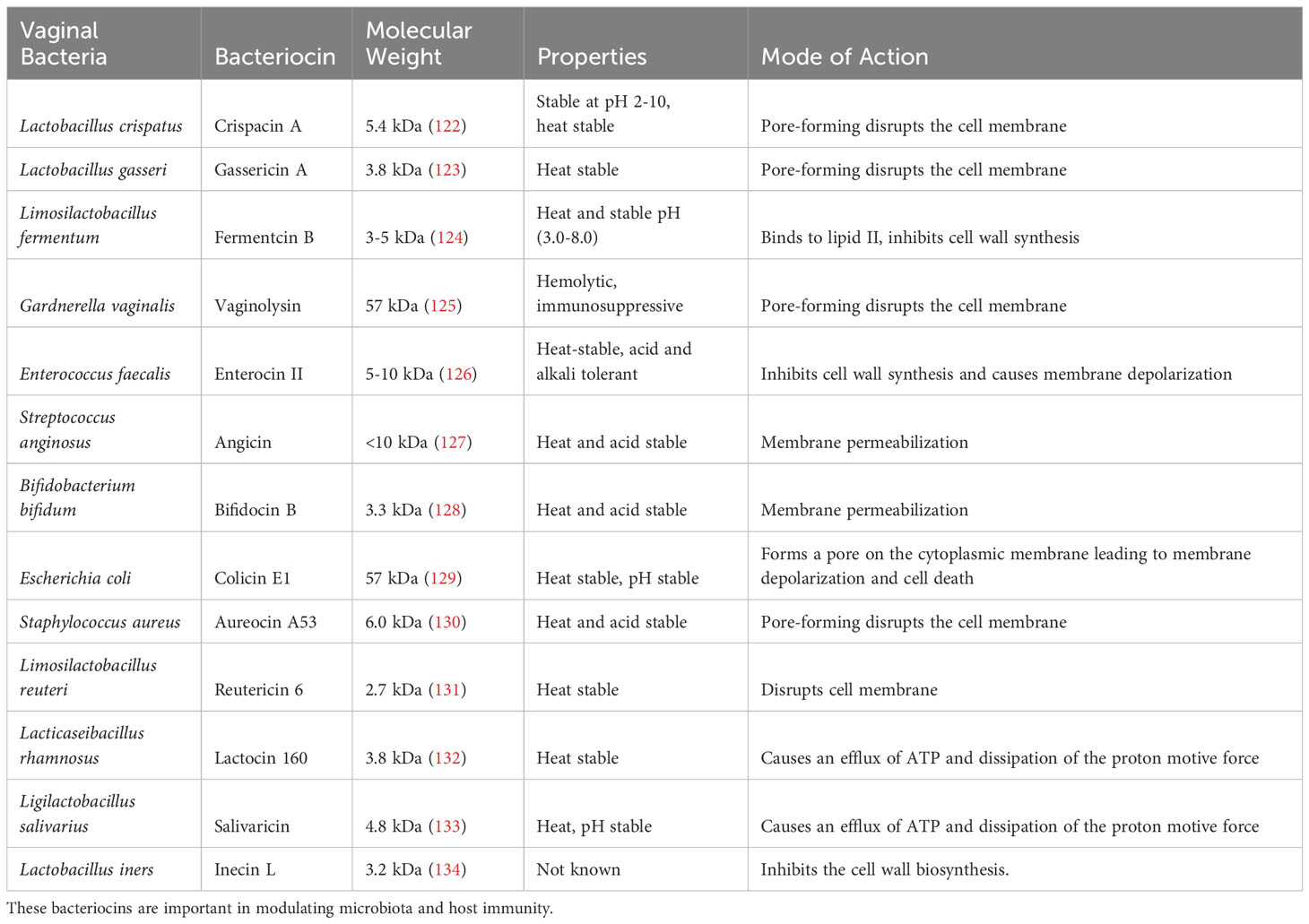
Table 1 A summary of bacteriocins produced by bacteria from the female genital tract, their molecular weight, and mode of action.
It is important to note that the production of bacteriocins is not exclusive to beneficial bacteria. Pathogenic bacteria can similarly produce bacteriocins to enhance their pathogenicity against the host and other resident bacteria. For example, L. iners and G. vaginalis produce cytolysins, namely inerolysin (125, 137) and vaginolysin (137) respectively. These can promote cervicovaginal epithelial damage to increase inflammation to increase the risk of sPTB. The role of bacteriocins remains incompletely understood. However, the type and rate of bacteriocin production, whether from pathogenic or commensal bacteria, is heavily influenced by the conditions within the cervicovaginal environment (125, 137). Further investigations are essential to characterize properties and functions of bacteriocins, to increase our understanding of their role in either preventing or promoting adverse pregnancy outcomes and their broader implications for human health.
1.7.3 Cervicovaginal Biosurfactants and link to sPTB
Biosurfactants are another category of molecules produced by cervicovaginal microbiota and are surface-active molecules generated by microorganisms. These compounds can either reside on the cell surface or be secreted extracellularly. Biosurfactants serve varying functions, microbial quorum sensing, motility, biofilm adhesion and detachment, virulence factors, and antagonism (138). Beneficial bacteria can use biosurfactants to reduce the adhesion of pathogenic bacteria to the cervicovaginal epithelium, thereby discouraging the adherence of pathogenic bacteria (139) to maintain balanced cervicovaginal microbiota promote term birth. Certain bacteria can use biosurfactants to establish a biofilm, adhering to the cervicovaginal surfaces for growth and survival (139). Furthermore, pathogenic bacteria within the biofilm are challenging to control due to the protective layers they form against host defenses and antibiotics (39, 139). This environment may also enhance the survival and growth of opportunistic pathogens capable of initiating sPTB.
1.7.4 Cervicovaginal microbiota and sialic acid on sPTB
Elevated sialidase enzyme activity, which catalyzes the production of sialic acid, has been associated with various adverse pregnancy outcomes, including placental infection, miscarriage, late pregnancy sPTB, and recurrent BV (140, 141). Sialic acid is a sugar molecule located on the terminal residues of glycoproteins and glycolipids present on cell surfaces, including the cervicovaginal epithelial cells (141). Certain bacteria, including G. vaginalis and A. vaginae, secrete sialidase and other mucin-degrading enzymes (142–144) to produce sialic acid. By degrading the terminal sialic acid residues from the protective glycan mucus layer of the cervicovaginal epithelium, these bacteria can trigger inflammation and disrupt the balance of the cervicovaginal microbiota which is associated with sPTB and low birth weight (142). The degradation of the mucus also generates readily available carbon sources that can contribute to the growth of other bacteria within the cervicovaginal environment (144). In a pregnant Chinese cohort (n=819) high sialidase activity and leukocyte levels were linked to diverse vaginal microbiota composition and reduced lactobacilli; however, these factors did not increase the risk of PTB (145). The variation in results could be influenced by other factors, such as ethnicity, which is known to play a significant role in shaping the composition of vaginal microbiota and its effects on pregnancy outcomes.
1.8 Probiotic-based interventions in modulating the cervicovaginal-microbiota-PTB link
The modulation of cervicovaginal microbiota can be achieved through interventions, including the use of probiotics, prebiotics, antibiotics, and pH modulators (146). Perhaps the most common and effective method that has been employed against BV, AV, and associated infectious conditions, is the use of antibiotics. Antibiotics such as metronidazole, clindamycin, and azithromycin are commonly used (43, 77, 147). Antibiotics, while effective against infections, can disrupt the microbiota balance, including beneficial bacteria. This disruption is associated with a higher recurrence rate (43) and an increased risk of sPTB (147).
In response, alternatives to antibiotics such as probiotics have been proposed. Probiotics encompass live beneficial bacteria that can colonize the vagina and improve its microbial composition. For example, L. crispatus, L. jensenii, and L. gasseri are commonly used probiotics (147, 148). They can be administered orally or intravaginally in the form of capsules, suppositories, or creams (146, 149–151).
Most interventions have focused on using Lactobacillus species to address bacterial conditions such as BV and AV, both of which have been associated with an increased risk of sPTB. The results of these interventions have yielded mixed findings, with some studies demonstrating the effectiveness of probiotics while others have not. For instance, in a study involving pregnant women who received vaginal supplementation with Lactobacillus crispatus CTV-05, a reduced rate of sPTB was observed (148). Another study involving pregnant women who tested positive for Group B Streptococcus (GBS) during late pregnancy administered a daily oral probiotic capsule containing L. rhamnosus GR-1 and L. reuteri RC-14 until delivery. This intervention resulted in a modest reduction in GBS compared to the placebo group (Table 2) (153). Additionally, although not conducted in pregnant women, a randomized controlled study found that vaginally applied probiotic capsules containing L. rhamnosus GR-1 and L. reuteri RC-14, once a day for five days, led to a faster BV cure rate compared to twice-daily metronidazole gel application (150). Some studies have also shown that these interventions can reduce inflammation, which is a known risk factor for sPTB. In one study, a capsule containing L. brevis CD2, L. salivarius subsp. salicinius, and L. plantarum was administered vaginally to pregnant women with BV for 30 days, proving effective against BV and reducing vaginal inflammatory cytokines: IL-1β and IL-6 (151).
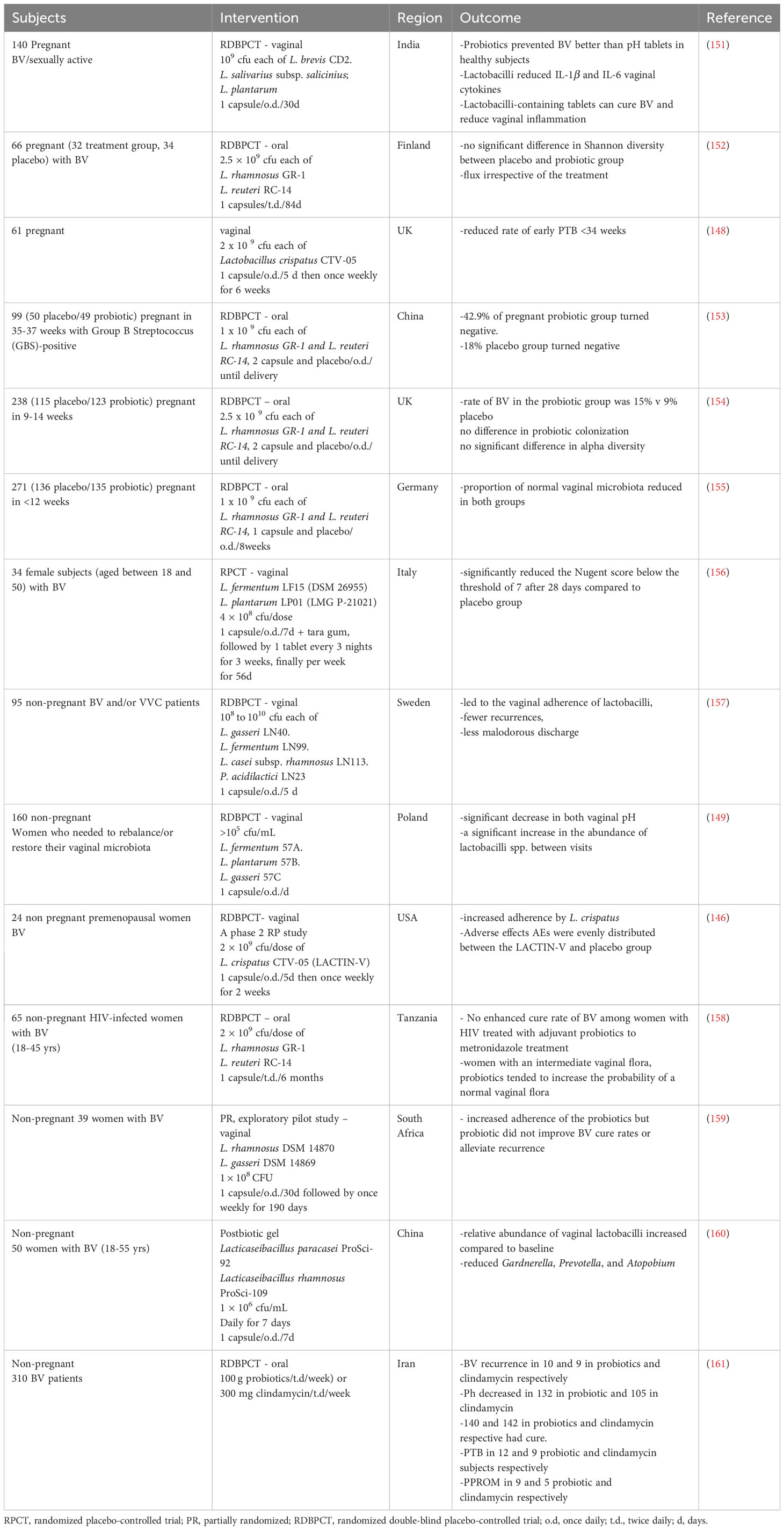
Table 2 A summary of probiotics, prebiotics and synbiotic intervention to influence female genital tract microbiota and consequence on bacterial vaginosis, anaerobic vaginosis, and or pregnancy outcome.
However, other studies have revealed that probiotics do not offer a cure for BV and do not significantly impact pregnancy outcomes when compared to the placebo group (152, 154, 155). The uncertainty regarding their effectiveness arises from the wide variability in routes of administration, dosages, and probiotic choices, making it challenging to draw direct comparisons across studies. For instance, despite efforts to target specific effects and induce compositional changes in the cervicovaginal environment through oral administration, multiple studies have reported no significant differences between the placebo and probiotic-treated groups (Table 2) (154, 155, 161). These collective findings suggest that the oral route of administration may not be effective in achieving the desired effects in the cervicovaginal environment and, consequently, a protective effect against sPTB. The effectiveness of the vaginal route of administration, while appearing promising, is also challenged by the lack of uniformity surrounding its application.
2 Conclusion and future perspective
sPTB is a complex syndrome influenced by various factors. Research efforts have increasingly centered on investigating the role of the cervicovaginal microbiota in adverse pregnancy outcomes. While a definitive causal link is yet to be established, changes in microbial composition, characterized by a decrease in lactobacilli and an increase in opportunistic pathogens such as Gardnerella, Enterobacter, Escherichia coli, Candida, and Prevotella, have been associated with sPTB. The microbiota dynamics encompassing the production of lactic acid as well as bacteriocins, can have antagonistic effects critical for maintaining cervicovaginal microbiota balance that have profound influence on pregnancy outcome.
Moreover, the utilization of SCFAs and biosurfactants by cervicovaginal microbiota to adherence to epithelial surfaces, along with the presence of their proteolytic and glycohydrolase enzymes, may compromise the integrity of the epithelial. This could potentially impact the microbiota composition, increase susceptibility to infections, and ultimately influence the risk of sPTB. Nevertheless, our understanding of the production and function of these metabolites is restricted due to a paucity of comprehensive research.
Existing reports on cervicovaginal microbial composition and structure often rely on isolated time points rather than longitudinal studies, potentially missing crucial changes throughout pregnancy. Besides, the precise role of certain pathogens, even when present in low abundance, in triggering adverse pregnancy outcomes remains obscured. Therefore, conducting detailed microbial profiling throughout the gestational period is imperative to elucidate the impact of alterations in opportunistic pathogens and lactobacilli during gestation on pregnancy outcomes.
Traditionally, antibiotics have been employed to manage conditions like BV, AV, and other cervicovaginal infections. However, the use of antibiotics, with potential effects on lactobacilli, has been linked to high recurrence rates and chronic infections. Biotherapeutics such as probiotics have shown promise in reducing infections and relapses of BV and AV. Nevertheless, uncertainties persist regarding their effectiveness due to inadequate study power, lack of standardization in probiotic selection, dosage, administration routes, and study endpoints. Furthermore, the use of maternal synthetic preImplantation factor (sPIF) prophylaxis during pregnancy, which has shown promise in reducing bacterial lipopolysaccharide-induced preterm births by regulating exaggerated immune responses (162), may offer a viable option for managing, may provide a viable option for managing sPTB.
In conclusion, investigating the cervicovaginal microbiota and its interactions including host immunity during pregnancy has the potential to significantly enhance our understanding of sPTB and contribute to the development of effective prevention and treatment strategies.
Author contributions
SO: Conceptualization, Data curation, Methodology, Visualization, Writing – original draft, Writing – review & editing. JM: Conceptualization, Methodology, Writing – review & editing. AK: Conceptualization, Funding acquisition, Writing – review & editing. PO: Funding acquisition, Supervision, Writing – review & editing. MT: Conceptualization, Funding acquisition, Supervision, Writing – review & editing. PV: Conceptualization, Funding acquisition, Writing – review & editing. RT: Conceptualization, Funding acquisition, Methodology, Supervision, Writing – review & editing. GO: Conceptualization, Funding acquisition, Methodology, Supervision, Writing – review & editing.
Group member of the PRECISE Network
King’s College London (Laura A. Magee, Hiten Mistry, Marie-Laure Volvert, Lucilla Poston, Jane Sandall, Sophie Moore, Tatiana Salisbury); Aga Khan University, Nairobi (Grace Mwashigadi, Consolata Juma, Isaac Mwaniki, Alex Mugo, Joseph Mutunga, Moses Mukhanya, Onesmus Wanje, Marvin Ochieng, Emily Mwadime); Centro de Investigação de Saúde de Manhiça (Esperança Sevene, Corssino Tchavana, Salesio Macuacua, Anifa Vala, Helena Boene, Lazaro Quimice, Sonia Maculuve, Eusebio Macete, Inacio Mandomando, Carla Carillho); Donna Russell Consulting (Donna Russell); London School of Hygiene and Tropical Medicine (Hannah Blencowe, Veronique Filippi, Joy Lawn, Matt Silver, Joseph Waiswa, Ursula Gazeley); Midlands State University (Prestige Tatenda Makanga, Liberty Makacha, Reason Mlambo); MRC Unit The Gambia at LSHTM (Umberto D’Alessandro, Anna Roca, Hawanatu Jah Andrew M. Prentice, Melisa Martinez-Alvarez,Brahima Diallo, Abdul Sesay, Sambou Suso, Baboucarr Njie, Fatima Touray,Yahaya Idris, Fatoumata Kongira, Modou F.S. Ndure, Lawrence Gibba, Abdoulie Bah, Yorro Bah); University of Oxford (Alison Noble, Aris Papageorghiou, Rachel Craik); St George’s, University of London (Judith Cartwright; Guy Whitley, Sanjeev Krishna); University of British Colombia (Marianne Vidler, Jing (Larry) Li, Jeff Bone, Mai-Lei (Maggie) Woo Kinshella, Domena Tu, Ash Sandhu, Kelly Pickerill); Imperial College London (Ben Barratt).
Funding
The author(s) declare financial support was received for the research, authorship, and/or publication of this article. This work was supported by the Medical Research Council Global Maternal and Neonatal Health Scheme [PRECISE-SPTB: MR/T03890X/1], the UK Research and Innovation Global Challenges Research Fund GROW Awards (PRECISE: MR/P027938/1), and the NIHR–Wellcome Partnership for Global Health Research Collaborative Awards (PRECISE-DYAD: 17123/Z/19/Z).
Conflict of interest
The authors declare that the research was conducted in the absence of any commercial or financial relationships that could be construed as a potential conflict of interest.
Publisher’s note
All claims expressed in this article are solely those of the authors and do not necessarily represent those of their affiliated organizations, or those of the publisher, the editors and the reviewers. Any product that may be evaluated in this article, or claim that may be made by its manufacturer, is not guaranteed or endorsed by the publisher.
Glossary
References
1. Eloe-Fadrosh EA, Rasko DA. The human microbiome: from symbiosis to pathogenesis. Annu Rev Med (2013) 64:145–63. doi: 10.1146/annurev-med-010312-133513
2. Flint HJ, Scott KP, Louis P, Duncan SH. The role of the gut microbiota in nutrition and health. Nat Rev Gastroenterol Hepatol (2012) 9(10):577–89. doi: 10.1038/nrgastro.2012.156
3. Peterson J, Garges S, Giovanni M, McInnes P, Wang L, Schloss JA, et al. The nih human microbiome project. Genome Res (2009) 19(12):2317–23. doi: 10.1101/gr.096651.109
4. Dekaboruah E, Suryavanshi MV, Chettri D, Verma AK. Human microbiome: an academic update on human body site specific surveillance and its possible role. Arch Microbiol (2020) 202(8):2147–67. doi: 10.1007/s00203-020-01931-x
5. Freitas AC, Bocking A, Hill JE, Money DM. Increased richness and diversity of the vaginal microbiota and spontaneous preterm birth. Microbiome (2018) 6(1):117. doi: 10.1186/s40168-018-0502-8
6. Dunlop AL, Satten GA, Hu YJ, Knight AK, Hill CC, Wright ML, et al. Vaginal microbiome composition in early pregnancy and risk of spontaneous preterm and early term birth among African American women. Front Cell Infect Microbiol (2021) 11:641005. doi: 10.3389/fcimb.2021.641005
7. Tantengco OAG, Menon R. Breaking down the barrier: the role of cervical infection and inflammation in preterm birth. Front Global Women's Health (2022) 2. doi: 10.3389/fgwh.2021.777643
8. Anton L, Sierra LJ, DeVine A, Barila G, Heiser L, Brown AG, et al. Common cervicovaginal microbial supernatants alter cervical epithelial function: mechanisms by which lactobacillus crispatus contributes to cervical health. Front Microbiol (2018) 9:2181. doi: 10.3389/fmicb.2018.02181
9. Romero R, Hassan SS, Gajer P, Tarca AL, Fadrosh DW, Nikita L, et al. The composition and stability of the vaginal microbiota of normal pregnant women is different from that of non-pregnant women. Microbiome (2014) 2(1):4. doi: 10.1186/2049-2618-2-4
10. Baud A, Hillion K-H, Plainvert C, Tessier V, Tazi A, Mandelbrot L, et al. Microbial diversity in the vaginal microbiota and its link to pregnancy outcomes. Sci Rep (2023) 13(1):9061. doi: 10.1038/s41598-023-36126-z
11. Kindinger LM, Bennett PR, Lee YS, Marchesi JR, Smith A, Cacciatore S, et al. The interaction between vaginal microbiota, cervical length, and vaginal progesterone treatment for preterm birth risk. Microbiome (2017) 5:1–14. doi: 10.1186/s40168-016-0223-9
12. Feehily C, Crosby D, Walsh CJ, Lawton EM, Higgins S, McAuliffe FM, et al. Shotgun sequencing of the vaginal microbiome reveals both a species and functional potential signature of preterm birth. NPJ Biofilms Microbiomes (2020) 6(1):50. doi: 10.1038/s41522-020-00162-8
13. Flaviani F, Hezelgrave NL, Kanno T, Prosdocimi EM, Chin-Smith E, Ridout AE, et al. Cervicovaginal microbiota and metabolome predict preterm birth risk in an ethnically diverse cohort. JCI Insight (2021) 6(16). doi: 10.1172/jci.insight.149257
14. Goodfellow L, Verwijs MC, Care A, Sharp A, Ivandic J, Poljak B, et al. Vaginal bacterial load in the second trimester is associated with early preterm birth recurrence: A nested case-control study. BJOG an Int J obstetrics gynaecol (2021) 128(13):2061–72. doi: 10.1111/1471-0528.16816
15. Fettweis JM, Brooks JP, Serrano MG, Sheth NU, Girerd PH, Edwards DJ, et al. Differences in vaginal microbiome in african american women versus women of European ancestry. Microbiol (Reading) (2014) 160(Pt 10):2272–82. doi: 10.1099/mic.0.081034-0
16. Roachford OSE, Alleyne AT, Nelson KE. Insights into the vaginal microbiome in a diverse group of women of African, Asian and European ancestries. PeerJ (2022) 10:e14449. doi: 10.7717/peerj.14449
17. Serrano MG, Parikh HI, Brooks JP, Edwards DJ, Arodz TJ, Edupuganti L, et al. Racioethnic diversity in the dynamics of the vaginal microbiome during pregnancy. Nat Med (2019) 25(6):1001–11. doi: 10.1038/s41591-019-0465-8
18. Chen C, Song X, Wei W, Zhong H, Dai J, Lan Z, et al. The microbiota continuum along the female reproductive tract and its relation to uterine-related diseases. Nat Commun (2017) 8(1):875. doi: 10.1038/s41467-017-00901-0
19. Mendling W. Vaginal microbiota. Adv Exp Med Biol (2016) 902:83–93. doi: 10.1007/978-3-319-31248-4_6
20. Ravel J, Gajer P, Abdo Z, Schneider GM, Koenig SSK, McCulle SL, et al. Vaginal microbiome of reproductive-age women. Proc Natl Acad Sci (2011) 108(supplement_1):4680–7. doi: 10.1073/pnas.1002611107
21. Zhou X, Bent SJ, Schneider MG, Davis CC, Islam MR, Forney LJ. Characterization of vaginal microbial communities in adult healthy women using cultivation-independent methods. Microbiol (Reading England) (2004) 150(8):2565–73. doi: 10.1099/mic.0.26905-0
22. Leo AP, Oskotsky TT, Oskotsky B, Wibrand C, Roldan A, Tang A, et al. Vmap: vaginal microbiome atlas during pregnancy. medRxiv preprint server Health Sci (2023). doi: 10.1101/2023.03.21.23286947
23. Mancabelli L, Tarracchini C, Milani C, Lugli GA, Fontana F, Turroni F, et al. Vaginotypes of the human vaginal microbiome. Environ Microbiol (2021) 23(3):1780–92. doi: 10.1111/1462-2920.15441
24. Romero R, Theis Kevin R, Gomez-Lopez N, Winters Andrew D, Panzer Jonathan J, Lin H, et al. The vaginal microbiota of pregnant women varies with gestational age, maternal age, and parity. Microbiol Spectr (2023) 11(4):e03429–22. doi: 10.1128/spectrum.03429-22
25. DiGiulio DB, Callahan BJ, McMurdie PJ, Costello EK, Lyell DJ, Robaczewska A, et al. Temporal and spatial variation of the human microbiota during pregnancy. Proc Natl Acad Sci United States America (2015) 112(35):11060–5. doi: 10.1073/pnas.1502875112
26. Petrova MI, Lievens E, Malik S, Imholz N, Lebeer S. Lactobacillus species as biomarkers and agents that can promote various aspects of vaginal health. Front Physiol (2015) 6. doi: 10.3389/fphys.2015.00081
27. Tamrakar R, Yamada T, Furuta I, Cho K, Morikawa M, Yamada H, et al. Association between lactobacillus species and bacterial vaginosis-related bacteria, and bacterial vaginosis scores in pregnant Japanese women. BMC Infect Dis (2007) 7:128. doi: 10.1186/1471-2334-7-128
28. Witkin SS, Linhares IM, Giraldo P. Bacterial flora of the female genital tract: function and immune regulation. Best Pract Res Clin obstetrics gynaecol (2007) 21(3):347–54. doi: 10.1016/j.bpobgyn.2006.12.004
29. Gudnadottir U, Debelius JW, Du J, Hugerth LW, Danielsson H, Schuppe-Koistinen I, et al. The vaginal microbiome and the risk of preterm birth: A systematic review and network meta-analysis. Sci Rep (2022) 12(1):7926. doi: 10.1038/s41598-022-12007-9
30. Gupta P, Singh MP, Goyal K. Diversity of vaginal microbiome in pregnancy: deciphering the obscurity. Front Public Health (2020) 8. doi: 10.3389/fpubh.2020.00326
31. Hyman RW, Fukushima M, Jiang H, Fung E, Rand L, Johnson B, et al. Diversity of the vaginal microbiome correlates with preterm birth. Reprod Sci (2014) 21(1):32–40. doi: 10.1177/1933719113488838
32. Manhanzva MT, Abrahams AG, Gamieldien H, Froissart R, Jaspan H, Jaumdally SZ, et al. Inflammatory and antimicrobial properties differ between vaginal lactobacillus isolates from South African women with non-optimal versus optimal microbiota. Sci Rep (2020) 10(1):6196. doi: 10.1038/s41598-020-62184-8
33. Fettweis JM, Serrano MG, Brooks JP, Edwards DJ, Girerd PH, Parikh HI, et al. The vaginal microbiome and preterm birth. Nat Med (2019) 25(6):1012–21. doi: 10.1038/s41591-019-0450-2
34. Delgado-Diaz DJ, Tyssen D, Hayward JA, Gugasyan R, Hearps AC, Tachedjian G. Distinct immune responses elicited from cervicovaginal epithelial cells by lactic acid and short chain fatty acids associated with optimal and non-optimal vaginal microbiota. Front Cell Infect Microbiol (2019) 9:446. doi: 10.3389/fcimb.2019.00446
35. Boskey ER, Cone RA, Whaley KJ, Moench TR. Origins of vaginal acidity: high D/L lactate ratio is consistent with bacteria being the primary source. Hum Reprod (2001) 16(9):1809–13. doi: 10.1093/humrep/16.9.1809
36. Chan D, Bennett PR, Lee YS, Kundu S, Teoh TG, Adan M, et al. Microbial-driven preterm labour involves crosstalk between the innate and adaptive immune response. Nat Commun (2022) 13(1):975. doi: 10.1038/s41467-022-28620-1
37. Murphy HR, Howgate C, O'Keefe J, Myers J, Morgan M, Coleman MA, et al. Characteristics and outcomes of pregnant women with type 1 or type 2 diabetes: A 5-year national population-based cohort study. Lancet Diabetes Endocrinol (2021) 9(3):153–64. doi: 10.1016/S2213-8587(20)30406-X
38. Stokkeland K, Ludvigsson JF, Hultcrantz R, Ekbom A, Höijer J, Bottai M, et al. Increased risk of preterm birth in women with autoimmune hepatitis – a nationwide cohort study. Liver Int (2016) 36(1):76–83. doi: 10.1111/liv.12901
39. Pekmezovic M, Mogavero S, Naglik JR, Hube B. Host-pathogen interactions during female genital tract infections. Trends Microbiol (2019) 27(12):982–96. doi: 10.1016/j.tim.2019.07.006
40. Odogwu NM, Onebunne CA, Chen J, Ayeni FA, Walther-Antonio MRS, Olayemi OO, et al. Lactobacillus crispatus thrives in pregnancy hormonal milieu in a Nigerian patient cohort. Sci Rep (2021) 11(1):18152. doi: 10.1038/s41598-021-96339-y
41. Uchihashi M, Bergin IL, Bassis CM, Hashway SA, Chai D, Bell JD. Influence of age, reproductive cycling status, and menstruation on the vaginal microbiome in baboons (Papio anubis). Am J Primatol (2015) 77(5):563–78. doi: 10.1002/ajp.22378
42. Günther V, Allahqoli L, Watrowski R, Maass N, Ackermann J, von Otte S, et al. Vaginal microbiome in reproductive medicine. Diagnostics (2022) 12(8). doi: 10.3390/diagnostics12081948
43. Mayer BT, Srinivasan S, Fiedler TL, Marrazzo JM, Fredricks DN, Schiffer JT. Rapid and profound shifts in the vaginal microbiota following antibiotic treatment for bacterial vaginosis. J Infect Dis (2015) 212(5):793–802. doi: 10.1093/infdis/jiv079
44. Krog MC, Hugerth LW, Fransson E, Bashir Z, Nyboe Andersen A, Edfeldt G, et al. The healthy female microbiome across body sites: effect of hormonal contraceptives and the menstrual cycle. Hum Reprod (Oxford England) (2022) 37(7):1525–43. doi: 10.1093/humrep/deac094
45. Kaur H, Merchant M, Haque MM, Mande SS. Crosstalk between female gonadal hormones and vaginal microbiota across various phases of women’s gynecological lifecycle. Front Microbiol (2020) 11. doi: 10.3389/fmicb.2020.00551
46. Vomstein K, Reider S, Böttcher B, Watschinger C, Kyvelidou C, Tilg H, et al. Uterine microbiota plasticity during the menstrual cycle: differences between healthy controls and patients with recurrent miscarriage or implantation failure. J Reprod Immunol (2022) 151:103634. doi: 10.1016/j.jri.2022.103634
47. Gupta S, Kakkar V, Bhushan I. Crosstalk between vaginal microbiome and female health: A review. Microbial pathogenesis (2019) 136:103696. doi: 10.1016/j.micpath.2019.103696
48. Santella B, Schettino MT, Franci G, De Franciscis P, Colacurci N, Schiattarella A, et al. Microbiota and hpv: the role of viral infection on vaginal microbiota. J Med Virol (2022) 94(9):4478–84. doi: 10.1002/jmv.27837
49. Gudza-Mugabe M, Havyarimana E, Jaumdally S, Garson KL, Lennard K, Tarupiwa A, et al. Human immunodeficiency virus infection is associated with preterm delivery independent of vaginal microbiota in pregnant african women. J Infect Dis (2020) 221(7):1194–203. doi: 10.1093/infdis/jiz584
50. Drell T, Lillsaar T, Tummeleht L, Simm J, Aaspõllu A, Väin E, et al. Characterization of the vaginal micro-and mycobiome in asymptomatic reproductive-age Estonian women. PloS One (2013) 8(1):e54379. doi: 10.1371/journal.pone.0054379
51. Lai GC, Tan TG, Pavelka N. The mammalian mycobiome: A complex system in a dynamic relationship with the host. Wiley Interdiscip Reviews: Syst Biol Med (2019) 11(1):e1438. doi: 10.1002/wsbm.1438
52. Nunn KL, Witkin SS, Schneider GM, Boester A, Nasioudis D, Minis E, et al. Changes in the vaginal microbiome during the pregnancy to postpartum transition. Reprod Sci (2021) 28(7):1996–2005. doi: 10.1007/s43032-020-00438-6
53. Sohn K, Underwood MA. Prenatal and postnatal administration of prebiotics and probiotics. Semin fetal neonatal Med (2017) 22(5):284–9. doi: 10.1016/j.siny.2017.07.002
54. Li D, Chi XZ, Zhang L, Chen R, Cao JR, Sun XY, et al. Vaginal microbiome analysis of healthy women during different periods of gestation. Bioscience Rep (2020) 40(7). doi: 10.1042/bsr20201766
55. Dominguez-Bello MG. Gestational shaping of the maternal vaginal microbiome. Nat Med (2019) 25(6):882–3. doi: 10.1038/s41591-019-0483-6
56. Rasmussen MA, Thorsen J, Dominguez-Bello MG, Blaser MJ, Mortensen MS, Brejnrod AD, et al. Ecological succession in the vaginal microbiota during pregnancy and birth. ISME J (2020) 14(9):2325–35. doi: 10.1038/s41396-020-0686-3
57. Donders GG, Van Calsteren K, Bellen G, Reybrouck R, Van den Bosch T, Riphagen I, et al. Predictive value for preterm birth of abnormal vaginal flora, bacterial vaginosis and aerobic vaginitis during the first trimester of pregnancy. BJOG an Int J obstetrics gynaecol (2009) 116(10):1315–24. doi: 10.1111/j.1471-0528.2009.02237.x
58. Pohanka M. D-lactic acid as a metabolite: toxicology, diagnosis, and detection. BioMed Res Int (2020) 2020:3419034. doi: 10.1155/2020/3419034
59. Amabebe E, Anumba DOC. The vaginal microenvironment: the physiologic role of lactobacilli. Front Med (Lausanne) (2018) 5:181. doi: 10.3389/fmed.2018.00181
60. Witkin Steven S, Mendes-Soares H, Linhares Iara M, Jayaram A, Ledger William J, Forney Larry J. Influence of vaginal bacteria and D- and L-lactic acid isomers on vaginal extracellular matrix metalloproteinase inducer: implications for protection against upper genital tract infections. mBio (2013) 4(4):e00460–13. doi: 10.1128/mBio.00460-13
61. Iacono KT, Brown AL, Greene MI, Saouaf SJ. Cd147 immunoglobulin superfamily receptor function and role in pathology. Exp Mol Pathol (2007) 83(3):283–95. doi: 10.1016/j.yexmp.2007.08.014
62. Farage MA, Miller KW, Sobel JD. Dynamics of the vaginal ecosystem—Hormonal influences. Infect Diseases: Res Treat (2010) 3:IDRT–S3903. doi: 10.4137/IDRT.S3903
63. Muhleisen AL, Herbst-Kralovetz MM. Menopause and the vaginal microbiome. Maturitas (2016) 91:42–50. doi: 10.1016/j.maturitas.2016.05.015
64. Kim JM, Park YJ. Probiotics in the prevention and treatment of postmenopausal vaginal infections: review article. J menopausal Med (2017) 23(3):139–45. doi: 10.6118/jmm.2017.23.3.139
65. Gustafsson RJ, Ahrné S, Jeppsson B, Benoni C, Olsson C, Stjernquist M, et al. The lactobacillus flora in vagina and rectum of fertile and postmenopausal healthy Swedish women. BMC women's Health (2011) 11(1):17. doi: 10.1186/1472-6874-11-17
66. Challis JRG, Matthews SG, Gibb W, Lye SJ. Endocrine and paracrine regulation of birth at term and preterm. Endocrine Rev (2000) 21(5):514–50. doi: 10.1210/edrv.21.5.0407
67. Jefferson KK, Parikh HI, Garcia EM, Edwards DJ, Serrano MG, Hewison M, et al. Relationship between vitamin D status and the vaginal microbiome during pregnancy. J Perinatol (2019) 39(6):824–36. doi: 10.3389/fcimb.2021.622474
68. Zhou SS, Tao YH, Huang K, Zhu BB, Tao FB. Vitamin D and risk of preterm birth: up-to-date meta-analysis of randomized controlled trials and observational studies. J Obstetrics Gynaecol Res (2017) 43(2):247–56. doi: 10.1016/S0140-6736(08)60074-4
69. Chawanpaiboon S, Vogel JP, Moller AB, Lumbiganon P, Petzold M, Hogan D, et al. Global, regional, and national estimates of levels of preterm birth in 2014: A systematic review and modelling analysis. Lancet Global Health (2019) 7(1):e37–46. doi: 10.1016/s2214-109x(18)30451-0
70. Blencowe H, Cousens S, Chou D, Oestergaard M, Say L, Moller A-B, et al. Born Too Soon: The Global Epidemiology of 15 Million Preterm Births. Reproductive health (2013) 10(1):S2. doi: 10.1186/1742-4755-10-S1-S2
71. Kumar S, Kumari N, Talukdar D, Kothidar A, Sarkar M, Mehta O, et al. The vaginal microbial signatures of preterm birth delivery in Indian women. Front Cell Infect Microbiol (2021) 11:622474. doi: 10.3389/fcimb.2021.622474
72. Goldenberg RL, Culhane JF, Iams JD, Romero R. Epidemiology and causes of preterm birth. Lancet (2008) 371(9606):75–84. doi: 10.1016/S0140-6736(08)60074-4
73. Mtshali A, San JE, Osman F, Garrett N, Balle C, Giandhari J, et al. Temporal changes in vaginal microbiota and genital tract cytokines among South African women treated for bacterial vaginosis. Front Immunol (2021) 12. doi: 10.1016/j.diagmicrobio.2019.114981
74. Barrientos-Durán A, Fuentes-López A, de Salazar A, Plaza-Díaz J, García F. Reviewing the composition of vaginal microbiota: inclusion of nutrition and probiotic factors in the maintenance of eubiosis. Nutrients (2020) 12(2). doi: 10.3390/nu12020419
75. Manuck TA, Esplin MS, Biggio J, Bukowski R, Parry S, Zhang H, et al. The phenotype of spontaneous preterm birth: application of a clinical phenotyping tool. Am J Obstetrics Gynecol (2015) 212(4):487.e1–.e11. doi: 10.1016/j.ajog.2015.02.010
76. Casari E, Ferrario A, Morenghi E, Montanelli A. Gardnerella, trichomonas vaginalis, candida, chlamydia trachomatis, mycoplasma hominis and ureaplasma urealyticum in the genital discharge of symptomatic fertile and asymptomatic infertile women. New microbiologica (2010) 33 1:69–76. doi: 10.1016/j.ajog.2019.11.1283
77. Wang C, Fan A, Li H, Yan Y, Qi W, Wang Y, et al. Vaginal bacterial profiles of aerobic vaginitis: A case–control study. Diagn Microbiol Infect Dis (2020) 96(4):114981. doi: 10.1016/j.diagmicrobio.2019.114981
78. Kumar L, Dwivedi M, Jain N, Shete P, Solanki S, Gupta R, et al. The female reproductive tract microbiota: friends and foe. Life (Basel Switzerland) (2023) 13(6). doi: 10.3390/life13061313
79. Juliana NCA, Deb S, Juma MH, Poort L, Budding AE, Mbarouk A, et al. The Vaginal Microbiota Composition and Genital Infections During and after Pregnancy among Women in Pemba Island, Tanzania. Microorganisms (2022) 10(3). doi: 10.3390/microorganisms10030509
80. Gerson KD, McCarthy C, Elovitz MA, Ravel J, Sammel MD, Burris HH. Cervicovaginal microbial communities deficient in lactobacillus species are associated with second trimester short cervix. Am J obstetrics gynecology (2020) 222(5):491–e1. doi: 10.12816/0039672
81. Barinov SV, Tirskaya YI, Kadsyna TV, Lazareva OV, Medyannikova IV, Tshulovski YI. Pregnancy and delivery in women with a high risk of infection in pregnancy. J Maternal-Fetal Neonatal Med (2022) 35(11):2122–7. doi: 10.1111/j.1447-0756.1997.tb00814.x
82. Bartkeviciene D, Opolskiene G, Bartkeviciute A, Arlauskiene A, Lauzikiene D, Zakareviciene J, et al. The impact of ureaplasma infections on pregnancy complications. Libyan J Med (2020) 15(1). doi: 10.1080/19932820.2020.1812821
83. González Bosquet E, Gené A, Ferrer I, Borrás M, Lailla JM. Value of endocervical ureaplasma species colonization as a marker of preterm delivery. Gynecologic obstetric Invest (2006) 61(3):119–23. doi: 10.1001/jama.1990.03440230056032
84. Mohamed SS, Rabei NH, Faisal MM, Amin AS. Association between chlamydia trachomatis cervicitis and preterm labor. Egyptian J Hosp Med (2017) 68(3):1342–9. doi: 10.1016/0002-9378(90)90815-O
85. Begum S, Sagawa T, Fujimoto S. Screening for bacterial vaginosis and cervicitis aimed at preventing premature delivery. J Obstetrics Gynaecol Res (1997) 23(1):103–10. doi: 10.1371/journal.pone.0245937
86. Ahmadi SJ, Farhadifar F, Sharami SRY, Zare S, Rezaei M, Soofizadeh N, et al. The role of chlamydia trachomatis in preterm delivery: A case-control study in Besat hospital, Sanandaj, Iran (2018–2019). Iranian J Microbiol (2020) 12(4):325. doi: 10.1038/s41598-022-13871-1
87. Cohen I, Veille J-C, Calkins BM. Improved pregnancy outcome following successful treatment of chlamydial infection. Jama (1990) 263(23):3160–3. doi: 10.1016/0028-2243(91)90185-N
88. Ryan GM Jr., Abdella TN, McNeeley SG, Baselski VS, Drummond DE. Chlamydia trachomatis infection in pregnancy and effect of treatment on outcome. Am J obstetrics gynecology (1990) 162(1):34–9. doi: 10.1016/0002-9378(90)90815-O
89. Kacerovsky M, Pliskova L, Bolehovska R, Lesko D, Gerychova R, Janku P, et al. Cervical gardnerella vaginalis in women with preterm prelabor rupture of membranes. PloS One (2021) 16(1):e0245937. doi: 10.1016/j.ajog.2018.10.014
90. Park S, You Y-A, Kim Y-H, Kwon E, Ansari A, Kim SM, et al. Ureaplasma and prevotella colonization with lactobacillus abundance during pregnancy facilitates term birth. Sci Rep (2022) 12(1):10148. doi: 10.1038/s41598-022-13871-1
91. Chambers S, Pons JC, Richard A, Chiesa M, Bouyer J, Papiernik E. Vaginal infections, cervical ripening and preterm delivery. Eur J Obstetrics Gynecol Reprod Biol (1991) 38(2):103–8. doi: 10.1016/0028-2243(91)90185-N
92. Verstraelen H, Vieira-Baptista P, De Seta F, Ventolini G, Lonnee-Hoffmann R, Lev-Sagie A. The vaginal microbiome: I. Research development, lexicon, defining "Normal" and the dynamics throughout women's lives. J Low Genit Tract Dis (2022) 26(1):73–8. doi: 10.1097/lgt.0000000000000643
93. Bayigga L, Kateete DP, Anderson DJ, Sekikubo M, Nakanjako D. Diversity of vaginal microbiota in sub-saharan africa and its effects on hiv transmission and prevention. Am J Obstet Gynecol (2019) 220(2):155–66. doi: 10.1016/j.ajog.2018.10.014
94. Gulavi E, Mwendwa F, Atandi DO, Okiro PO, Hall M, Beiko RG, et al. Vaginal microbiota in women with spontaneous preterm labor versus those with term labor in Kenya: A case control study. BMC Microbiol (2022) 22(1):270. doi: 10.1186/s12866-022-02681-0
95. Jimenez Nicole R, Maarsingh Jason D, Łaniewski P, Herbst-Kralovetz Melissa M. Commensal lactobacilli metabolically contribute to cervical epithelial homeostasis in a species-specific manner. mSphere (2023) 8(1):e00452–22. doi: 10.1128/msphere.00452-22
96. Onyango SO, Juma J, De Paepe K, Van de Wiele T. Oral and gut microbial carbohydrate-active enzymes landscape in health and disease. Front Microbiol (2021) 12:653448. doi: 10.3389/fmicb.2021.653448
97. Ghartey J, Anglim L, Romero J, Brown A, Elovitz MA. Women with symptomatic preterm birth have a distinct cervicovaginal metabolome. Am J Perinatol (2017) 34(11):1078–83. doi: 10.1055/s-0037-1603817
98. Stafford GP, Parker JL, Amabebe E, Kistler J, Reynolds S, Stern V, et al. Spontaneous preterm birth is associated with differential expression of vaginal metabolites by lactobacilli-dominated microflora. Front Physiol (2017) 8:615. doi: 10.1128/msphere.00166-22
99. Amabebe E, Anumba DOC. A combination of cervicovaginal fluid glutamate, acetate and D-lactate identified asymptomatic low-risk women destined to deliver preterm: A prospective cohort study. Reprod Sci (Thousand Oaks Calif) (2022) 29(3):915–22. doi: 10.1007/s43032-021-00711-2
100. Ghartey J, Bastek JA, Brown AG, Anglim L, Elovitz MA. Women with preterm birth have a distinct cervicovaginal metabolome. Am J Obstet Gynecol (2015) 212(6):776.e1–.e12. doi: 10.1016/j.ajog.2015.03.052
101. Srinivasan S, Morgan Martin T, Fiedler Tina L, Djukovic D, Hoffman Noah G, Raftery D, et al. Metabolic signatures of bacterial vaginosis. mBio (2015) 6(2):e00204–15. doi: 10.1128/mBio.00204-15
102. Horrocks V, Hind Charlotte K, Wand Matthew E, Fady P-E, Chan J, Hopkins Jade C, et al. Nuclear magnetic resonance metabolomics of symbioses between bacterial vaginosis-associated bacteria. mSphere (2022) 7(3):e00166–22. doi: 10.1128/msphere.00166-22
103. Gilbert NM, Lewis WG, Li G, Sojka DK, Lubin JB, Lewis AL. Gardnerella vaginalis and prevotella bivia trigger distinct and overlapping phenotypes in a mouse model of bacterial vaginosis. J Infect Dis (2019) 220(7):1099–108. doi: 10.1093/infdis/jiy704
104. D’Ippolito S, Di Nicuolo F, Pontecorvi A, Gratta M, Scambia G, Di Simone N. Endometrial microbes and microbiome: recent insights on the inflammatory and immune “Players” of the human endometrium. Am J Reprod Immunol (2018) 80(6):e13065. doi: 10.1111/aji.13065
105. Di Simone N, Santamaria Ortiz A, Specchia M, Tersigni C, Villa P, Gasbarrini A, et al. Recent insights on the maternal microbiota: impact on pregnancy outcomes. Front Immunol (2020) 11:528202. doi: 10.3389/fimmu.2020.528202
106. Anton L, Ferguson B, Friedman ES, Gerson KD, Brown AG, Elovitz MA. Gardnerella vaginalis alters cervicovaginal epithelial cell function through microbe-specific immune responses. Microbiome (2022) 10(1):119. doi: 10.4049/jimmunol.1302891
107. Zariffard MR, Novak RM, Lurain N, Sha BE, Graham P, Spear GT. Induction of tumor necrosis factor–α Secretion and toll-like receptor 2 and 4 mrna expression by genital mucosal fluids from women with bacterial vaginosis. J Infect Dis (2005) 191(11):1913–21. doi: 10.1086/429922
108. Xiang N, Yin T, Chen T. Gardnerella vaginalis induces nlrp3 inflammasome−Mediated pyroptosis in macrophages and thp−1 monocytes. Exp Ther Med (2021) 22(4):1–9. doi: 10.3892/etm.2021.10609
109. Osman I, Young A, Ledingham MA, Thomson AJ, Jordan F, Greer IA, et al. Leukocyte Density and Pro-Inflammatory Cytokine Expression in Human Fetal Membranes, Decidua, Cervix and Myometrium before and During Labour at Term. Mol Hum Reprod (2003) 9(1):41–5. doi: 10.3389/fimmu.2020.00279
110. Rinaldi SF, Catalano RD, Wade J, Rossi AG, Norman JE. Decidual neutrophil infiltration is not required for preterm birth in a mouse model of infection-induced preterm labor. J Immunol (2014) 192(5):2315–25. doi: 10.3389/fcimb.2023.1124591
111. Mohd Zaki A, Hadingham A, Flaviani F, Haque Y, Mi JD, Finucane D, et al. Neutrophils dominate the cervical immune cell population in pregnancy and their transcriptome correlates with the microbial vaginal environment. Front Microbiol (2022) 13. doi: 10.3389/fmicb.2022.904451
112. Molina B, Bayar E, Lee Y, Muller I, Botto M, MacIntyre D, et al. Cervicovaginal inflammation and neutrophil infiltration/activation in women at high-risk of prematurity. BJOG: Int J Obstet Gy (2022) 129:47–62. doi: 10.1111/1471-0528.8_17178
113. Ma L-N, Huang X-B, Muyayalo KP, Mor G, Liao A-H. Lactic acid: A novel signaling molecule in early pregnancy? Front Immunol (2020) 11:279. doi: 10.1177/1933719115570914
114. Dong M, Dong Y, Bai J, Li H, Ma X, Li B, et al. Interactions between microbiota and cervical epithelial, immune, and mucus barrier. Front Cell Infection Microbiol (2023) 13. doi: 10.1128/JCM.42.2.713-717.2004
115. Schönfeld P, Wojtczak L. Short- and medium-chain fatty acids in energy metabolism: the cellular perspective. J Lipid Res (2016) 57(6):943–54. doi: 10.1194/jlr.R067629
116. Aldunate M, Srbinovski D, Hearps AC, Latham CF, Ramsland PA, Gugasyan R, et al. Antimicrobial and immune modulatory effects of lactic acid and short chain fatty acids produced by vaginal microbiota associated with eubiosis and bacterial vaginosis. Front Physiol (2015) 6:164. doi: 10.3389/fphys.2015.00164
117. Beghini J, Giraldo PC, Linhares IM, Ledger WJ, Witkin SS. Neutrophil gelatinase-associated lipocalin concentration in vaginal fluid. Reprod Sci (2015) 22(8):964–8. doi: 10.1177/1933719115570914
118. Wilks M, Wiggins R, Whiley A, Hennessy E, Warwick S, Porter H, et al. Identification and H(2)O(2) production of vaginal lactobacilli from pregnant women at high risk of preterm birth and relation with outcome. J Clin Microbiol (2004) 42(2):713–7. doi: 10.1128/jcm.42.2.713-717.2004
119. Ceccarani C, Foschi C, Parolin C, D’Antuono A, Gaspari V, Consolandi C, et al. Diversity of vaginal microbiome and metabolome during genital infections. Sci Rep (2019) 9(1):14095. doi: 10.1038/s41598-019-50410-x
120. O'Hanlon DE, Moench TR, Cone RA. In vaginal fluid, bacteria associated with bacterial vaginosis can be suppressed with lactic acid but not hydrogen peroxide. BMC Infect Dis (2011) 11(1):200. doi: 10.1186/1471-2334-11-200
121. Mitchell C, Fredricks D, Agnew K, Hitti J. Hydrogen peroxide-producing lactobacilli are associated with lower levels of vaginal interleukin-1β, independent of bacterial vaginosis. Sexually transmitted Dis (2015) 42(7):358–63. doi: 10.1097/olq.0000000000000298
122. Tahara T, Kanatani K. Isolation and partial characterization of crispacin a, a cell-associated bacteriocin produced by lactobacillus crispatus jcm 2009. FEMS Microbiol Lett (1997) 147(2):287–90. doi: 10.1111/j.1574-6968.1997.tb10255.x
123. Kawai Y, Saito T, Toba T, Samant SK, Itoh T. Isolation and characterization of a highly hydrophobic new bacteriocin (Gassericin a) from lactobacillus gasseri la39. Bioscience biotechnology Biochem (1994) 58(7):1218–21. doi: 10.1271/bbb.58.1218
124. Yan TR, Lee CS. Characterization of a partially purified bacteriocin, fermentcin B, from lactobacillus fermentum. Biotechnol Lett (1997) 19(8):741–4. doi: 10.1023/A:1018327907435
125. Ragaliauskas T, Plečkaitytė M, Jankunec M, Labanauskas L, Baranauskiene L, Valincius G. Inerolysin and vaginolysin, the cytolysins implicated in vaginal dysbiosis, differently impair molecular integrity of phospholipid membranes. Sci Rep (2019) 9(1):10606. doi: 10.1038/s41598-019-47043-5
126. Alang H, Kusnadi J, Ardyati T. Optimization and characterization of enterocin enterococcus faecalis K2b1 isolated from toraja's belang buffalo milk, south sulawesi, Indonesia. Biodiversitas J Biol Diversity (2020) 21:1236–42. doi: 10.13057/biodiv/d210351
127. Vogel V, Bauer R, Mauerer S, Schiffelholz N, Haupt C, Seibold GM, et al. Angicin, a novel bacteriocin of streptococcus anginosus. Sci Rep (2021) 11(1):24377. doi: 10.1038/s41598-021-03797-5
128. Yildirim Z, Johnson MG. Characterization and antimicrobial spectrum of bifidocin B, a bacteriocin produced by bifidobacterium bifidum ncfb 1454. J Food Prot (1998) 61(1):47–51. doi: 10.4315/0362-028x-61.1.47
129. Masi M, Vuong P, Humbard M, Malone K, Misra R. Initial steps of colicin E1 import across the outer membrane of escherichia coli. J Bacteriol (2007) 189:2667–76. doi: 10.1128/JB.01448-06
130. Netz DJA, Pohl R, Beck-Sickinger AG, Selmer T, Pierik AJ, de Freire Bastos M, et al. Biochemical characterisation and genetic analysis of aureocin A53, a new, atypical bacteriocin from staphylococcus aureus. J Mol Biol (2002) 319(3):745–56. doi: 10.1016/S0022-2836(02)00368-6
131. Kabuki T, Saito T, Kawai Y, Uemura J, Itoh T. Production, purification and characterization of reutericin 6, a bacteriocin with lytic activity produced by lactobacillus reuteri la6. Int J Food Microbiol (1997) 34(2):145–56. doi: 10.1016/S0168-1605(96)01180-4
132. Turovskiy Y, Ludescher RD, Aroutcheva AA, Faro S, Chikindas ML. Lactocin 160, a bacteriocin produced by vaginal lactobacillus rhamnosus, targets cytoplasmic membranes of the vaginal pathogen, gardnerella vaginalis. Probiotics Antimicrobial Proteins (2009) 1:67–74. doi: 10.1093/infdis/jix088
133. Vera Pingitore E, Hébert EM, Nader-Macías ME, Sesma F. Characterization of salivaricin crl 1328, a two-peptide bacteriocin produced by lactobacillus salivarius crl 1328 isolated from the human vagina. Res Microbiol (2009) 160(6):401–8. doi: 10.1016/j.resmic.2009.06.009
134. Li L, Zhang J, Zhou L, Shi H, Mai H, Su J, et al. The first lanthipeptide from lactobacillus iners, inecin L, exerts high antimicrobial activity against human vaginal pathogens. Appl Environ Microbiol (2023) 0(0):e02123–22. doi: 10.1128/aem.02123-22
135. Cotter PD, Ross RP, Hill C. Bacteriocins — a viable alternative to antibiotics? Nat Rev Microbiol (2013) 11(2):95–105. doi: 10.1038/nrmicro2937
136. Shannon B, Gajer P, Yi TJ, Ma B, Humphrys MS, Thomas-Pavanel J, et al. Distinct effects of the cervicovaginal microbiota and herpes simplex type 2 infection on female genital tract immunology. J Infect Dis (2017) 215(9):1366–75. doi: 10.1093/infdis/jix088
137. Gelber SE, Aguilar JL, Lewis KL, Ratner AJ. Functional and phylogenetic characterization of vaginolysin, the human-specific cytolysin from gardnerella vaginalis. J Bacteriol (2008) 190(11):3896–903. doi: 10.1128/jb.01965-07
138. Sharma J, Sundar D, Srivastava P. Biosurfactants: potential agents for controlling cellular communication, motility, and antagonism. Front Mol Biosci (2021) 8. doi: 10.1007/s00203-018-1520-4
139. Hardy L, Cerca N, Jespers V, Vaneechoutte M, Crucitti T. Bacterial biofilms in the vagina. Res Microbiol (2017) 168(9-10):865–74. doi: 10.1016/j.resmic.2017.02.001
140. Zhang X, Xu X, Li J, Li N, Yan T, Ju X. Relationship between vaginal sialidase bacteria vaginosis and chorioammionitis. Zhonghua fu chan ke za zhi (2002) 37(10):588–90. doi: 10.1097/QCO.0000000000000620
141. Agarwal K, Lewis AL. Vaginal sialoglycan foraging by gardnerella vaginalis: mucus barriers as a meal for unwelcome guests? Glycobiology (2021) 31(6):667–80. doi: 10.1093/glycob/cwab024
142. Govinden G, Parker JL, Naylor KL, Frey AM, Anumba DOC, Stafford GP. Inhibition of sialidase activity and cellular invasion by the bacterial vaginosis pathogen gardnerella vaginalis. Arch Microbiol (2018) 200(7):1129–33. doi: 10.1007/s00203-018-1520-4
143. Cavalcante T, Medeiros MM, Mule SN, Palmisano G, Stolf BS. The role of sialic acids in the establishment of infections by pathogens, with special focus on leishmania. Front Cell Infection Microbiol (2021) 11. doi: 10.1371/journal.ppat.1008559
144. Muzny CA, Łaniewski P, Schwebke JR, Herbst-Kralovetz MM. Host-vaginal microbiota interactions in the pathogenesis of bacterial vaginosis. Curr Opin Infect Dis (2020) 33(1):59–65. doi: 10.1097/qco.0000000000000620
145. Ng S, Chen M, Kundu S, Wang X, Zhou Z, Zheng Z, et al. Large-scale characterisation of the pregnancy vaginal microbiome and sialidase activity in a low-risk Chinese population. NPJ Biofilms Microbiomes (2021) 7(1):89. doi: 10.1038/s41522-021-00261-0
146. Hemmerling A, Harrison W, Schroeder A, Park J, Korn A, Shiboski S, et al. Phase 2a study assessing colonization efficiency, safety, and acceptability of lactobacillus crispatus ctv-05 in women with bacterial vaginosis. Sexually transmitted Dis (2010) 37(12):745–50. doi: 10.1097/OLQ.0b013e3181e50026
147. Happel A-U, Kullin B, Gamieldien H, Wentzel N, Zauchenberger CZ, Jaspan HB, et al. Exploring potential of vaginal lactobacillus isolates from South African women for enhancing treatment for bacterial vaginosis. PloS Pathog (2020) 16(6):e1008559. doi: 10.1371/journal.ppat.1008559
148. Bayar E, MacIntyre DA, Sykes L, Mountain K, Parks TP, Lee PP, et al. Safety, tolerability, and acceptability of lactobacillus crispatus ctv-05 (Lactin-V) in pregnant women at high-risk of preterm birth. Beneficial Microbes (2023) 14(1):45–55. doi: 10.3920/bm2022.0084
149. Tomusiak A, Strus M, Heczko PB, Adamski P, Stefański G, Mikołajczyk-Cichońska A, et al. Efficacy and safety of a vaginal medicinal product containing three strains of probiotic bacteria: A multicenter, randomized, double-blind, and placebo-controlled trial. Drug design Dev Ther (2015) 9:5345. doi: 10.1016/j.tjog.2016.06.003
150. Anukam KC, Osazuwa E, Osemene GI, Ehigiagbe F, Bruce AW, Reid G. Clinical study comparing probiotic lactobacillus gr-1 and rc-14 with metronidazole vaginal gel to treat symptomatic bacterial vaginosis. Microbes Infect (2006) 8(12-13):2772–6. doi: 10.1016/j.micinf.2006.08.008
151. Vasundhara D, Raju VN, Hemalatha R, Nagpal R, Kumar M. Vaginal & Gut microbiota diversity in pregnant women with bacterial vaginosis & Effect of oral probiotics: an exploratory study. Indian J Med Res (2021) 153(4):492. doi: 10.4103%2Fijmr.IJMR_350_19
152. Yang S, Reid G, Challis JRG, Gloor GB, Asztalos E, Money D, et al. Effect of oral probiotic lactobacillus rhamnosus gr-1 and lactobacillus reuteri rc-14 on the vaginal microbiota, cytokines and chemokines in pregnant women. Nutrients (2020) 12(2). doi: 10.3390/nu12020368
153. Ho M, Chang Y-Y, Chang W-C, Lin H-C, Wang M-H, Lin W-C, et al. Oral lactobacillus rhamnosus gr-1 and lactobacillus reuteri rc-14 to reduce group B streptococcus colonization in pregnant women: A randomized controlled trial. Taiwanese J Obstetrics Gynecol (2016) 55(4):515–8. doi: 10.1016/j.tjog.2016.06.003
154. Husain S, Allotey J, Drymoussi Z, Wilks M, Fernandez-Felix BM, Whiley A, et al. Effects of oral probiotic supplements on vaginal microbiota during pregnancy: A randomised, double-blind, placebo-controlled trial with microbiome analysis. BJOG: Int J Obstetrics Gynaecol (2020) 127(2):275–84. doi: 10.1111/1471-0528.15675
155. Gille C, Böer B, Marschal M, Urschitz MS, Heinecke V, Hund V, et al. Effect of probiotics on vaginal health in pregnancy. Effpro, a randomized controlled trial. Am J Obstetrics Gynecol (2016) 215(5):608.e1–.e7. doi: 10.1016/j.ajog.2016.06.021
156. Vicariotto F, Mogna L, Del Piano M. Effectiveness of the two microorganisms lactobacillus fermentum lf15 and lactobacillus plantarum lp01, formulated in slow-release vaginal tablets, in women affected by bacterial vaginosis: A pilot study. J Clin Gastroenterol (2014) 48 Suppl 1:S106–12. doi: 10.1097/mcg.0000000000000226
157. Ehrström S, Daroczy K, Rylander E, Samuelsson C, Johannesson U, Anzén B, et al. Lactic acid bacteria colonization and clinical outcome after probiotic supplementation in conventionally treated bacterial vaginosis and vulvovaginal candidiasis. Microbes Infect (2010) 12(10):691–9. doi: 10.3109/14767058.2011.614654
158. Hummelen R, Changalucha J, Butamanya NL, Cook A, Habbema JD, Reid G. Lactobacillus rhamnosus gr-1 and L. Reuteri rc-14 to prevent or cure bacterial vaginosis among women with hiv. Int J gynaecol obstetrics (2010) 111(3):245–8. doi: 10.1016/j.ijgo.2010.07.008
159. Marcotte H, Larsson PG, Andersen KK, Zuo F, Mikkelsen LS, Brandsborg E, et al. An exploratory pilot study evaluating the supplementation of standard antibiotic therapy with probiotic lactobacilli in South African women with bacterial vaginosis. BMC Infect Dis (2019) 19(1):824. doi: 10.1186/s12879-019-4425-1
160. Shen X, Xu L, Zhang Z, Yang Y, Li P, Ma T, et al. Postbiotic gel relieves clinical symptoms of bacterial vaginitis by regulating the vaginal microbiota. Front Cell Infection Microbiol (2023) 13. doi: 10.3389/fcimb.2023.1114364
161. Hantoushzadeh S, Golshahi F, Javadian P, Khazardoost S, Aram S, Hashemi S, et al. Comparative efficacy of probiotic yoghurt and clindamycin in treatment of bacterial vaginosis in pregnant women: A randomized clinical trial. J maternal-fetal neonatal Med (2012) 25(7):1021–4. doi: 10.3109/14767058.2011.614654
162. Spinelli M, Boucard C, Di Nicuolo F, Haesler V, Castellani R, Pontecorvi A, et al. Synthetic preimplantation factor (Spif) reduces inflammation and prevents preterm birth. PloS One (2020) 15(6):e0232493. doi: 10.1371/journal.pone.0232493
163. Zheng J, Wittouck S, Salvetti E, Franz C, Harris HMB, Mattarelli P, et al. A taxonomic note on the genus lactobacillus: description of 23 novel genera, emended description of the genus lactobacillus beijerinck 1901, and union of lactobacillaceae and leuconostocaceae. Int J Syst Evol Microbiol (2020) 70(4):2782–858. doi: 10.1099/ijsem.0.004107
Keywords: lactobacilli, preterm births, bacteriocins, lactic acid, pregnancy, probiotics
Citation: Onyango S, Mi JD, Koech A, Okiro P, Temmerman M, von Dadelszen P, Tribe RM, Omuse G and the PRECISE Network (2023) Microbiota dynamics, metabolic and immune interactions in the cervicovaginal environment and their role in spontaneous preterm birth. Front. Immunol. 14:1306473. doi: 10.3389/fimmu.2023.1306473
Received: 03 October 2023; Accepted: 11 December 2023;
Published: 22 December 2023.
Edited by:
Martin Mueller, University Hospital Bern, SwitzerlandReviewed by:
Nicoletta Di Simone, Humanitas University, ItalyBoris Kramer, University of Western Australia, Australia
Copyright © 2023 Onyango, Mi, Koech, Okiro, Temmerman, von Dadelszen, Tribe, Omuse and the PRECISE Network. This is an open-access article distributed under the terms of the Creative Commons Attribution License (CC BY). The use, distribution or reproduction in other forums is permitted, provided the original author(s) and the copyright owner(s) are credited and that the original publication in this journal is cited, in accordance with accepted academic practice. No use, distribution or reproduction is permitted which does not comply with these terms.
*Correspondence: Stanley Onyango, stanley.onyango@aku.edu
†These authors share senior authorship