Effects of Dietary Glycerol Monolaurate on Growth, Antioxidant Capacity and Lipid Metabolism in Cage-Farmed Pompano (Trachinotus ovatus) Juveniles
- 1College of Fisheries, Guangdong Ocean University, Zhanjiang, China
- 2Aquatic Animals Precision Nutrition and High Efficiency Feed Engineering Research Center of Guangdong Province, College of Fisheries, Guangdong Ocean University, Zhanjiang, China
- 3Guangdong Provincial Key Laboratory of Aquatic Animal Disease Control and Healthy Culture, College of Fisheries, Guangdong Ocean University, Zhanjiang, China
Glycerol monolaurate (GML), a representative fatty acid glyceride, was used to promote growth. This study investigated the effects of GML in diets on growth, serum biochemical parameters, antioxidant capacity, liver morphology and lipid metabolism for juvenile pompano Trachinotus ovatus. Six groups of T. ovatus (mean weight = 14.00 ± 0.70 g) were fed with six diets, containing varying GML levels of 0.00% (G0), 0.05% (G5), 0.10% (G10), 0.15% (G15), 0.20% (G20), and 0.25% (G25) for 8 weeks. Fish were reared for in floating cages (length: 1 m; width: 1 m; and height: 2 m). The highest weight gain rate (WGR), specific growth rate (SGR), and protein deposit rate (PDR) were observed in the G15 group (P < 0.05). Hepatic glutathione peroxidase, total antioxidant capacity and superoxide dismutase activities were significantly increased in the G10 and G15 groups compared with the G0 group (P < 0.05), whereas hepatic malondialdehyde (MDA) was significantly reduced in the G10 and G15 groups (P < 0.05). The alkaline phosphatase activity was significantly higher in the G5, G10, G15, and G20 groups than in the G0 group (P < 0.05), and the highest AKP activity was observed in the G15 group. Alanine aminotransferase (ALT) activity was significantly lower in the G10, G15, G20, and G25 groups than in the G0 group (P < 0.05). The addition of GML to the feed significantly reduced triglyceride (TG) content (P < 0.05). In comparison with the G0 group, the G15, G20, and G25 groups had significantly lower total cholesterol content and significantly higher high-density lipoprotein content (P < 0.05). Low-density lipoprotein content was significantly lower in the G5 and G10 groups than in the G0 group (P < 0.05). Serious vacuolation occurred in the G0 group, but the cell boundaries in all added groups were obvious, and the rate of intracellular vacuolization decreased. As the GML level increased, carnitine palmitoyl-transferase Ι mRNA level was significantly up-regulated (P < 0.05). The expression of fatty acid synthesis in the G10, G15, G20, and G25 groups was significantly lower than that in the G0 group (P < 0.05). The expression of sterol regulatory element-binding protein-Ι was significantly lower in the G10, G15, G20, and G25 groups than in the G0 group (P < 0.05). Based on the broken-line model of WGR and PDR corresponding to GML levels, the optimum addition level of dietary GML was 0.14%-0.16% for juvenile T. ovatus. In conclusion, 0.15% GML significantly improved the growth performance, hepatic antioxidant ability, and lipid metabolism and protected the liver for juvenile pompano T. ovatus.
Introduction
Lipids are important nutrients for the growth of aquatic animals. It provides energy for growth, and promotes the absorption of various fat-soluble substances (Leaver et al., 2008). In aquaculture, lipids are used to save feed protein and reduce nitrogen excretion (Liu et al., 2018a). When the fat content in the feed is insufficient to meet the energy required for aquatic animal growth, the protein in the body serves as an energy source, thus reducing its anabolic capacity. The deficiency of essential fatty acids will negatively affect the growth and cause some. This condition also causes the lack of essential fatty acids, which affects the normal growth of aquatic animals and even causes some nutritional and metabolic diseases (Sun et al., 2018). However, excessive lipid content in feed results in excessive fat intake by aquatic animals and increases fat deposition in the muscle and liver, resulting in liver damage and metabolic disorders (Bonvini et al., 2015) and a decrease in the antioxidant capacity of the body and the quality of aquatic products. Therefore, the development of feed additives to alleviate fatty liver is importanct.
Glycerol monolaurate (GML), a representative fatty acid glyceride of the medium-chain fatty acid monoglycerides, is easy to digest, is well absorbed, and has high antioxidant properties (Han et al., 2018). GML is transported to the liver, where it is efficiently used for energy production via beta-oxidation in the mitochondria for rapid energy supply (Turner et al., 2009). Therefore, GML could be used as a feed additive to accelerate growth and promote lipid metabolism in the liver (Liu et al., 2018b). GML is widely used in livestock and poultry nutrition because of its metabolic and functional characteristics (Van Immerseel et al., 2004; Shokrollahi et al., 2014; Wang et al., 2015). In aquaculture, GML could significantly improve the growth of Litopenaeus vannamei (Wang et al., 2020c), Larimichthys croceus (Jiang, 2021) and Danio rerio (Wang et al., 2021). In addition, GML plays a vital role in promoting fat metabolism and reducing fat accumulation in Lateolabrax maculatus (Sun et al., 2021) and Salmo salar (Belghit et al., 2019).
Pompano Trachinotus ovatus, a valuable food fish with tender flesh and delicious flavor, is known as the golden pompano (Osteichthyes, Perciformes, Carangidae) and is widely distributed in China, Japan, and Australia (Tutman et al., 2004). Considering the fast growth, high nutritional value, delicious flesh, thornless flesh, brilliant body color and special aroma of trevally, it has traditionally been classified as a valuable food fish among farmers (Wang et al., 2017a; Zhang et al., 2019). The annual production of T. ovatus in China exceeded 100,000 tons in 2020, promising candidates for intensive aquaculture in Southern China with an annual production of approximately 120,000 tons (Li et al., 2020). In recent years, as the scale of aquaculture expands, production results in low cost and high output, whereas high density and excessive baiting could cause impaired lipid metabolism and reduced antioxidant capacity of the organism in fish (Guo et al., 2021). The chance of inducing fatty liver is greatly increased, thus affecting growth and restricting the healthy and sustainable development of pompano T. ovatus farming (Fang et al., 2021).
Although GML has been widely used in livestock farming, limited studies have focused on farmed fish, and no research had been reported on its application in pompano T. ovatus farming. In the present study, the effects of GML on growth, serum biochemical parameters, antioxidant capacity, liver morphology, and lipid metabolism for T. ovatus were investigated by feeding different doses of GML to provide an experimental basis for the application of GML as a functional feed additive in the feed of T. ovatus.
Materials and Methods
Diet Formulation and Preparation
Six iso-nitrogenous and iso-lipidic diets (Table 1) were randomly allocated to 18 floating cages (length: 1 m; width: 1 m; height: 2 m): the basal diet with 0.00% (G0), 0.05% (G5), 0.10% (G10), 0.15% (G15), 0.20% (G20), and 0.25% (G25) GML (powdery, purity: 85%, Guangdong Lipid Science and Application Engineering Technology Research Centre, South China University of Technology) with three replicates each. Fish meal, chicken meal, soybean meal, peanut meal, and corn gluten meal were the main sources of protein in the diet.
Different doses of GML were weighed and completely dissolved in sterile water, respectively. All raw material was crushed through a 60-mesh sieve, mixed through a V-type vertical mixer (JS-14S; Zhejiang Zhengtai Electric Co., Ltd.), followed by adding with oil and water, and then pelleted (2.5 mm diameter) making use of a double screw extruder (F-75; South China University of Technology) (Song et al., 2010; Liang et al., 2020a). After the prepared experimental feed was naturally dried to about 10% moisture, it was sealed in a vacuum-packed bag and stored at -20°C until it was fed (He et al., 2021; Lin et al., 2021a).
Experimental Animals and Breeding Management
The experiment was conducted at an experimental site in Zhanjiang, Guangdong, China. Juvenile T. ovatus was procured from a seedling farm in Hainan Province for this investigation. The juvenile T. ovatus were acclimated to experimental conditions for two weeks. After two weeks, the total number of the experimental fish was 720, and there were 40 fish (initial mean weight: 14.00 ± 0.70 g) in each floating cage, respectively. The 18 groups were randomly assigned to the six test diets, with three replicates each. All fishes were fed twice daily (7:00 and 18:00) to visual satiety for 8 weeks. During the experiment, water temperature ranged from 29.0 to 31.0°C and the salinity was 24-26 ppt.
All procedures involving live animals were approved by the Guangdong Ocean University Institutional Animal Care and Use Committee.
Sample Collection
Fish samples were kept fast for 24 h before collection at the end of the trial and anesthetized with MS-222 (1:10,000) (Sigma, St Louis, MO, USA) (Wu et al., 2021). The fish samples of each experimental group were calculated and weighed, and it was determined that weight gain rate, survival rate and specific growth rate (Liang et al., 2020b). Afterward, three fish samples of each floating cage were randomly selected and then stored at -20°C to detect the whole-body composition (Wang et al., 2020a). Blood samples were gotten from the tail veins of seven randomly selected fish samples from each floating cage and then stored at 4°C for 12 h (Chen et al., 2016). After centrifuging (4000 ×g, 4°C, 10 min), the serum of fish samples was obtained and immediately preserved at -80°C for analyzing serum indicators (Gyan et al., 2020). After blood collection, muscle and liver of three fish randomly selected from each floating cage were stored frozen at -20°C to detect the composition. Furthermore, the liver samples of six randomly selected fish each floating cage were quickly removed, one part (three fish) was stored for enzymes activity analysis, whereas the others (three fish) were stored in RNA-later (Ambion, Texas, America) at -80°C before RNA isolation (Lin et al., 2022).
Formula for Calculations
Weight gain rate (WGR, %) = 100 × (final body weight − initial body weight)/initial body weight;
Specific growth rate (SGR, %/d) = 100 × [ln (final body weight) − ln (initial body weight)]/days;
Feed coefficient rate (FCR) = feed intake/(final body weight − initial body weight);
Survival rate (SR, %) = 100 × (final fish number/initial fish number);Hepatic somatic indices (HSI, %) = 100 × hepatic weight/body weight;
Viscera somatic index (VSI, %) = 100 × viscera weight/body weight;
Lipid deposition rate (LDR, %) = 100 × fish lipid deposition/total lipid intake of feed;
Protein deposition rate (PDR, %) = 100 × fish protein deposition/total protein intake of feed.
Chemical Analyses
The ingredients (crude protein, crude lipid, moisture and crude ash) of the samples were measured by using AOAC procedures (Horwitz, 2010). The content of crude protein was assayed by means of Kjeldahl method (N × 6.25) and crude lipid was measured using the method of Soxhlet extraction. Moisture was measured by the way of drying whole-body samples at 105°C until it reached a changeless weight. The content of ash was measured by a muffle furnace instrument burning at 550°C for 12 h. Crude Fiber was measured using the method of Van Soest (Yin et al., 2018). Gross energy in diets was determined by using oxygen bomb calorimetry (IKA-C2000, Germany) (Wang et al., 2017b).
The enzyme activities in serum were determined by using a detection kit (Nanjing Jian Cheng Bioengineering Institute, China) (Xie et al., 2013). Total protein was measured with Zhu et al. (2021b). Alanine aminotransferase (ALT) and aspartate aminotransferase (AST) were determined following Zhu et al. (2012) and Wang et al. (2020b). Alkaline phosphatase (AKP) activity was assayed according to Liu et al. (2021) and Li et al. (2009). High-density lipoprotein (HDL) and low-density lipoprotein (LDL) were measured with the methods of Li and C (2022) and Ray et al. (2020). Total cholesterol (CHOL) was determined following An et al. (2018). Triglyceride (TG) was assayed according to Gui et al. (2019).
The liver of three fish each floating cage was removed from the -80°C refrigerator and thawed on 4°C ice. It was accurately weighed and homogenized with 9 times of chilled saline and then centrifuged (3,000 rpm for 15 min) at 4°C, and the collection of the supernatant was pending subsequent analysis (Liu et al., 2013; Zhu et al., 2021c). The protein content of the samples was determined by using a total protein quantitative assay kit provided (Nanjing Jian Cheng Bioengineering Institute, China) (Liang et al., 2019). The superoxide dismutase (SOD) was measured by the xanthine oxidase method according to Wang et al. (2011). The activity of glutathione peroxidase (GSH-Px) was determined using the xanthine oxidase method (Ma et al., 2014). The total antioxidant capacity (T-AOC) was measured by Yang et al. (2017). The malondialdehyde (MDA) content was assayed as described by Lin et al. (2020).
Histological Morphology
The livers of three fish each floating cage were quickly removed, and one part was stored in 4% paraformaldehyde solution for histological analysis using hematoxylin-eosin (H & E) and Oil red staining (Lin et al., 2021b). The tissue sections of H & E staining and Oil red staining were respectively observed and photographed under an electron microscope scanner (VS 120-S6, Olympus, Norway) and light microscopy (Nikon Eclipse Ti-E, Japan) according to the method of Wang et al. (2020a).
Real-Time PCR Analysis of the Organization
Total RNA from the liver of three randomly selected fish from each floating cage was extracted with an RNA extraction kit (TransZol Up Plus RNA Kit, Beijing, China). PrimeScript™ RT-PCR Kit (TaKaRa, Kusatsu, Japan) was used to synthesize complementary DNA (cDNA) according to the manufacturers’ instructions. The PCR primers were listed in the Table 2. The PCR cycling protocol was used according to Liang et al. (2021) and Wang et al. (2018), and all the real-time PCR reactions were performed on a Roche LightCycler480II (Switzerland) using an SYBR @ Premix Ex TaqTMKit (Takara) (Zhu et al., 2021b) and program consisted of one cycle at 95°C for 30 s followed by 40 cycles at 95°C for 5 s and 60°C for 30 s, a melting curve of 0.5°C increments from 65 to 95°C was performed. The amplification efficiency of all genes was approximately equal, ranging from 98.1% to 100.0%. The relative mRNA expressions were calculated using the 2−ΔΔCT method (Livak and Schmittgen, 2002).
Statistical Analysis
All data were subjected to ANOVA using SPSS 21.0 (SPSS Inc., Chicago, IL, USA). Duncan’s multiple range test was performed in the case of a significant overall difference between the experimental group and the control (P < 0.05). The results were shown as the mean ± SEM (standard error of the mean).
Results
Effects of GML on the Growth Performance for Juvenile T. ovatus
The effect of GML on the growth performance for juvenile T. ovatus is shown in Figure 1. WGR and SGR were significantly higher in the G15 and G20 groups than in the G0 group (P < 0.05). PDR was significantly higher in the G10, G15, G20, and G25 groups than in the G0 group (P < 0.05). The LDR and VSI were significantly decreased in all added groups than in the control group (P < 0.05). No significant differences were observed in SR and FCR (P >0.05). The HSI in the G10, G15, G20, and G25 groups was significantly lower than in the G0 group (P < 0.05).
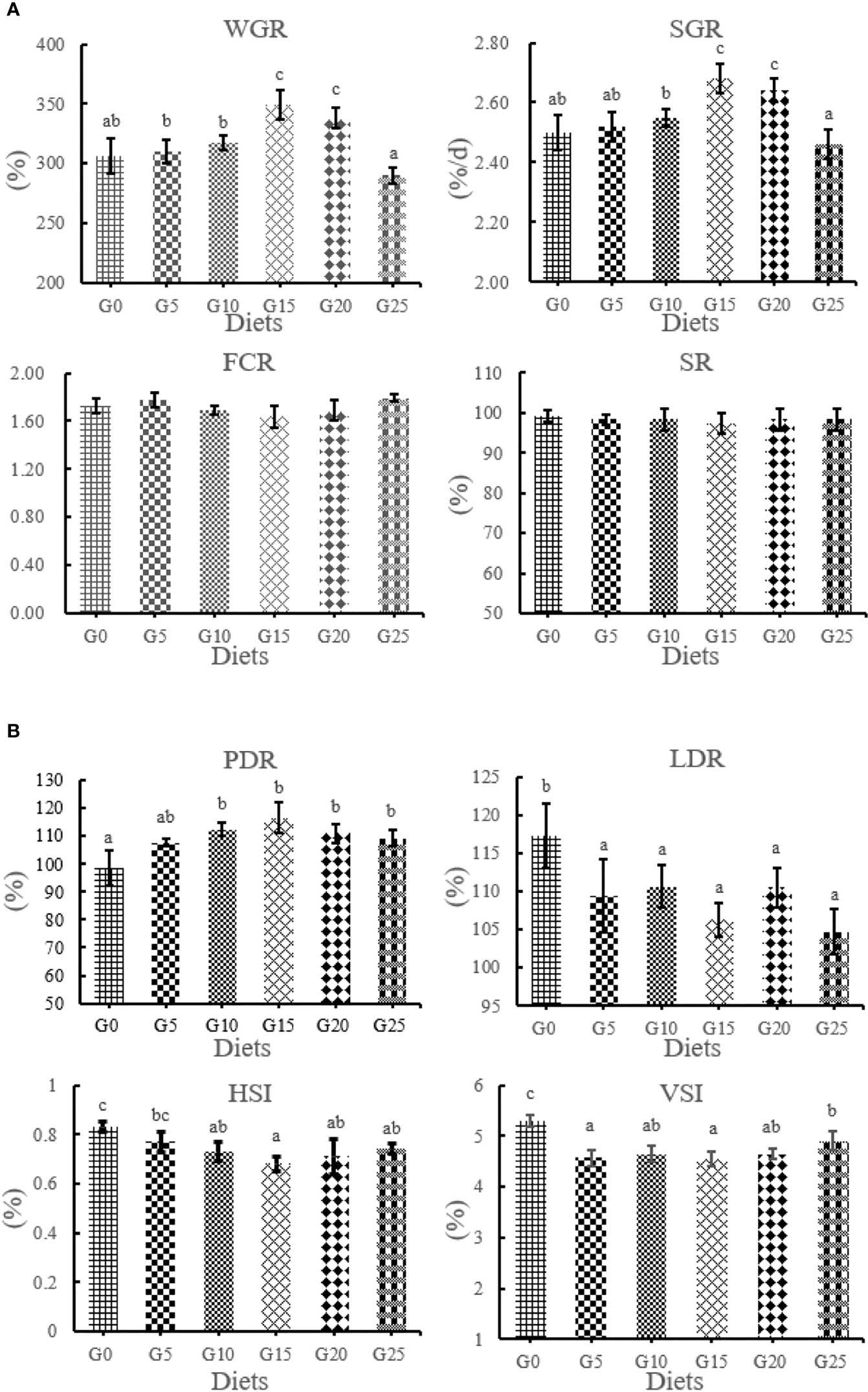
Figure 1 (A, B) Effect of GML on growth performance for juvenile T. ovatus. Vertical bars represented the mean ± S.E.M. (n = 3). Data marked with letters differ significantly (P < 0.05) among groups. WGR, weight gain rate; SGR, specific growth rate; FCR, feed coefficient rate; SR, survival rate; PDR, protein deposition rate; LDR; lipid deposition rate; HSI; hepatosomatic index; VSI; viscerosomatic index.
Based on the broken-line model of WGR and PDR corresponding to GML levels, the break points were evident at 0.14% and 0.16% GML of dry diet, respectively (Figures 2, 3). The WGR (YWGR) and PDR (YPDR) to dietary concentration of GML relationship were estimated using the following broken-line model regression equations:
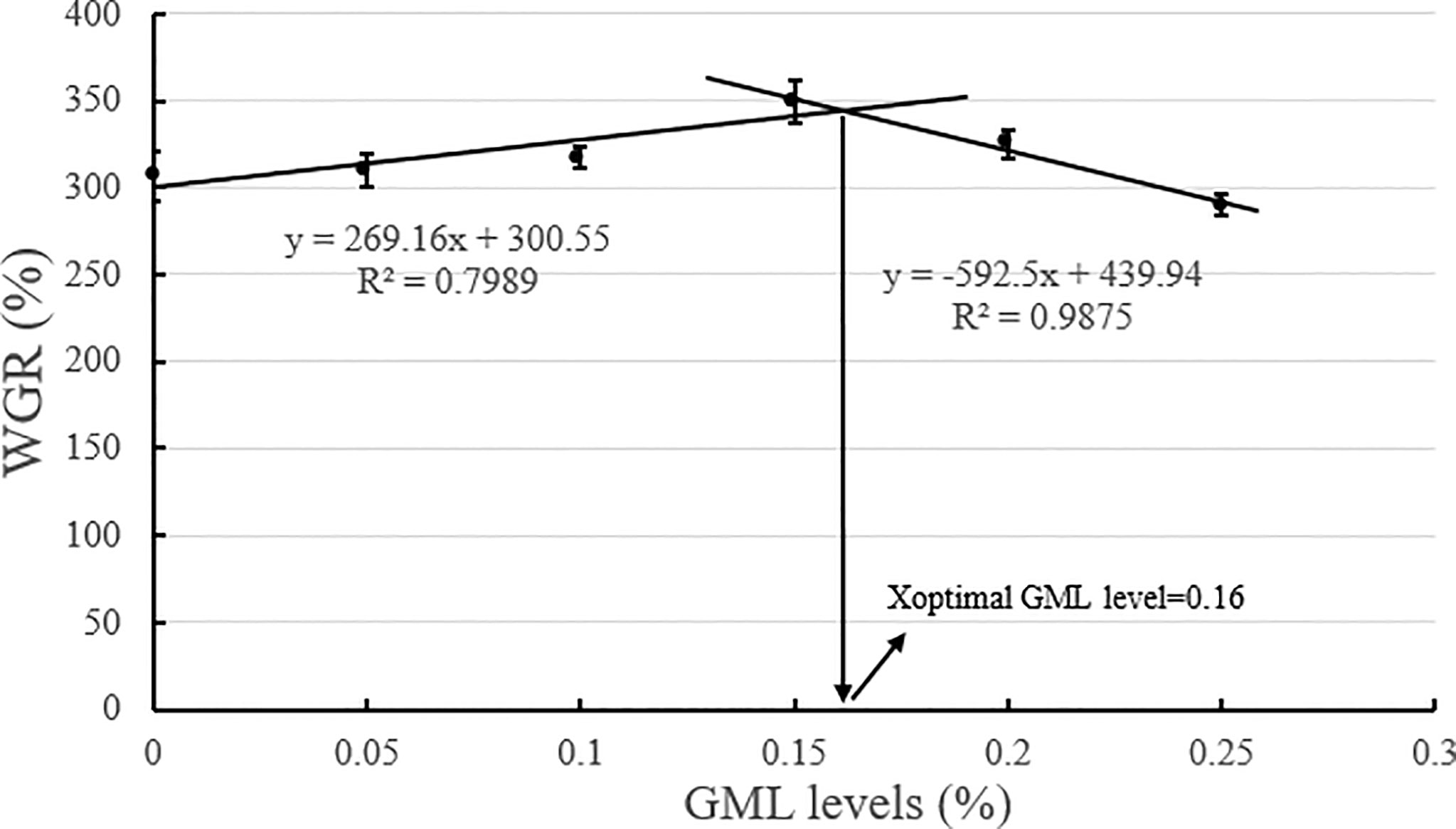
Figure 2 Effects of different GML levels on the WGR for juvenile pompano T. ovatus (n = 3). Based on the broken-line model of WGR corresponding to GML levels (y = 269.16x + 300.55, R2 = 0.7989; y = −592.5x + 439.94, R2 = 0.9875), the optimum addition level of dietary GML was estimated to be 0.16%.
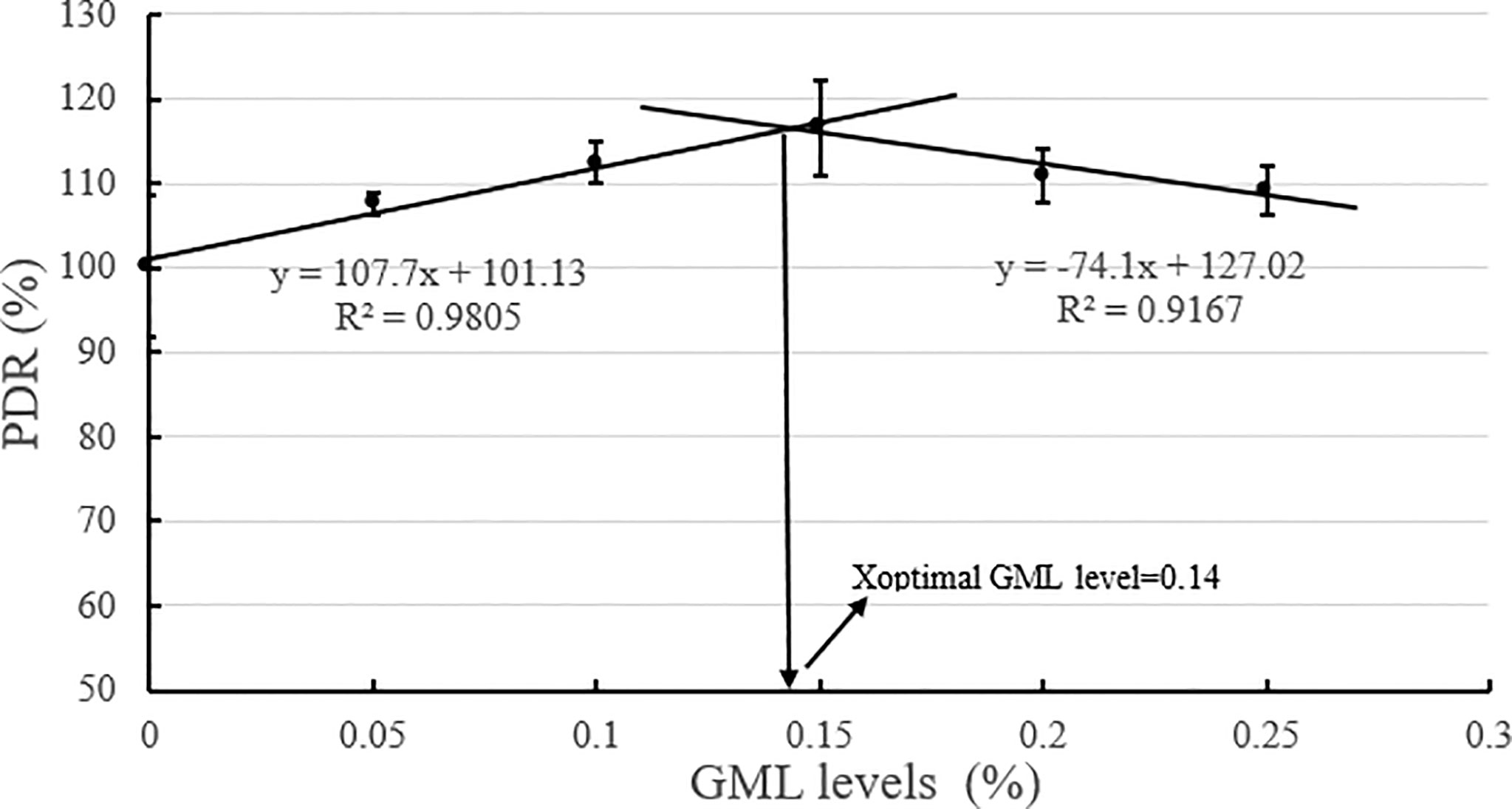
Figure 3 Effects of different GML levels on the PDR for juvenile pompano T. ovatus (n = 3). Based on the broken-line model of PDR corresponding to GML levels (y =107.7x + 101.13, R2 = 0.9805; y = −74.1x + 127.02, R2 = 0.9167), the optimum addition level of dietary GML was estimated to be 0.14%.
YWGR: y1 = 269.16x + 300.55, R2 = 0.7989; y2 = −592.5x + 439.94, R2 = 0.9875;
YPDR: y1 = 107.7x + 101.13, R2 = 0.9805; y2 = −74.1x + 127.02, R2 = 0.9167.
Effects of GML on the Body Composition for Juvenile T. ovatus
The crude protein of body composition was significantly higher in the G15 group than in the other groups (P < 0.05; Table 3). The crude lipid of body composition was significantly lower in the G10, G15, and G20 groups than in the G0 group (P < 0.05). The crude lipid in the livers in the G10, G15, G20, and G25 groups was significantly lower than in the G0 group (P < 0.05), whereas no significant differences were observed in muscle crude lipid (P > 0.05). No significant differences were found in the crude ash and moisture of the body composition (P > 0.05).
Effects of GML on Serum Biochemical Indicators for Juvenile T. ovatus
In terms of serum indicators (Table 4), TP was significantly higher in the G5, G10, and G15 groups than in the G0 group (P < 0.05). The AKP activity was significantly higher in the G5, G10, G15, and G20 groups than in the G0 group (P < 0.05), and the highest AKP activity was observed in the G15 group. ALT activity was significantly lower in the G10, G15, G20, and G25 groups than in the G0 group (P < 0.05). No significant difference was observed in AST activity (P > 0.05).
Effects of GML on Antioxidant Parameters in the Liver for Juvenile T. ovatus
The T-AOC activity in the added group was significantly higher than that in the control group, while the MDA content in the added group was significantly lower than that in the control group (P < 0.05, Figure 4). The GSH-Px activity was significantly higher in the G15, G20, and G25 groups than in the G0 group (P < 0.05). SOD activity was significantly higher in the G10, G15, G20, and G25 groups than in the G0 group (P < 0.05). The highest T-AOC, GSH-Px, and SOD activities were obtained in the G15 group.
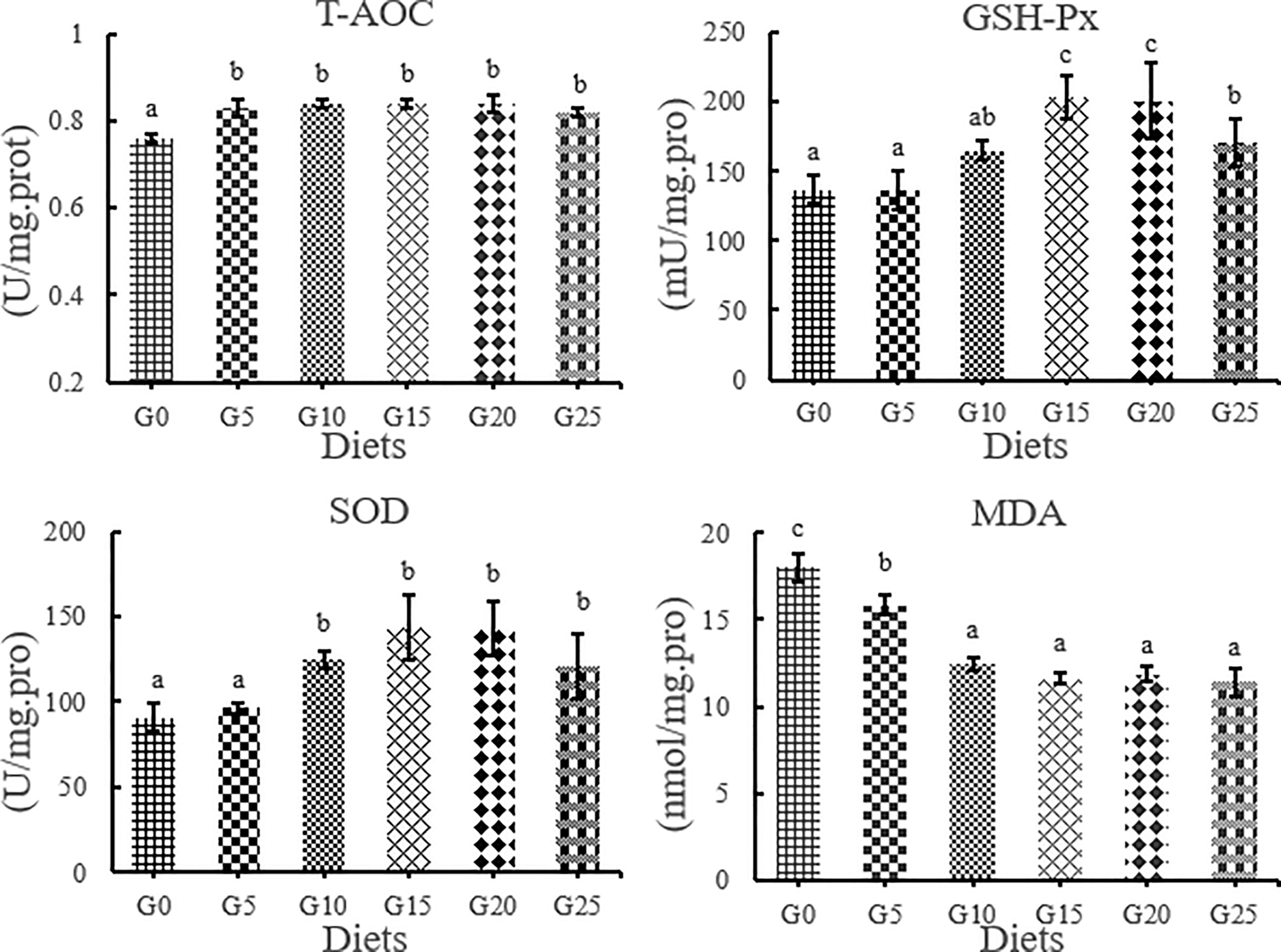
Figure 4 Effect of GML on liver antioxidant parameters for juvenile T. ovatus. T-AOC, total antioxidant capacity GSH-Px, glutathione peroxidase; MDA, malondialdehyde; SOD, superoxide dismutase.
Effects of GML on Lipid Metabolism-Related Enzyme Activity in Serum for Juvenile T. ovatus
TG levels were significantly lower in the G10, G15, G20, and G25 groups than in the G0 group (P < 0.05; Figure 5), in which the lowest TG levels were observed in the G15 group. The total cholesterol content tended to decrease as the amount of GML added increased. The CHOL levels were significantly lower in the G15, G20, and G25 groups than in the G0 group (P < 0.05). However, HDL content increased with increasing GML addition. HDL levels were significantly higher in the G15, G20, and G25 groups than in the G0 group (P < 0.05). LDL was significantly lower in the G5 and G10 groups than in the G0 group (P < 0.05).
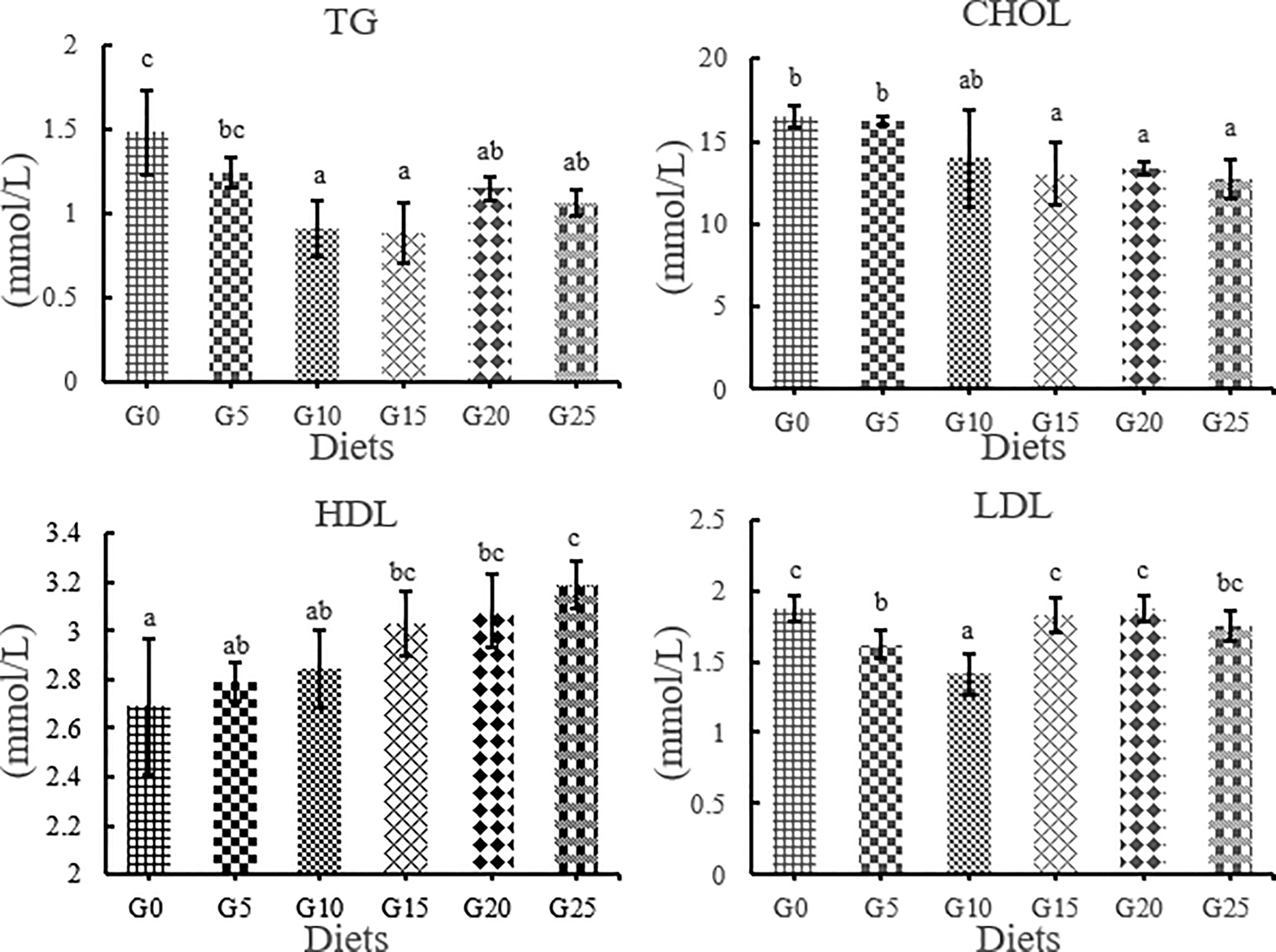
Figure 5 Effect of GML on serum lipid metabolism related enzyme activity for juvenile T. ovatus. TG, triglyceride; CHOL, total cholesterol; HDL, high-density lipoprotein; LDL, low-density lipoprotein.
Effects of GML on Liver Morphology for Juvenile T. ovatus
Based on the observation of liver tissue (Figure 6), serious vacuolation occurred in the G0 group, but the cell boundaries in all added groups were obvious, and the rate of intracellular vacuolization decreased. We stained the liver with oil red to stain the fat in red and thus observe fat deposition in the liver (Figure 7). Fat deposition tended to decrease with increasing GML levels. The fat droplets in the control group were large and dense, whereas the experimental group had significantly smaller and fewer fat droplets.
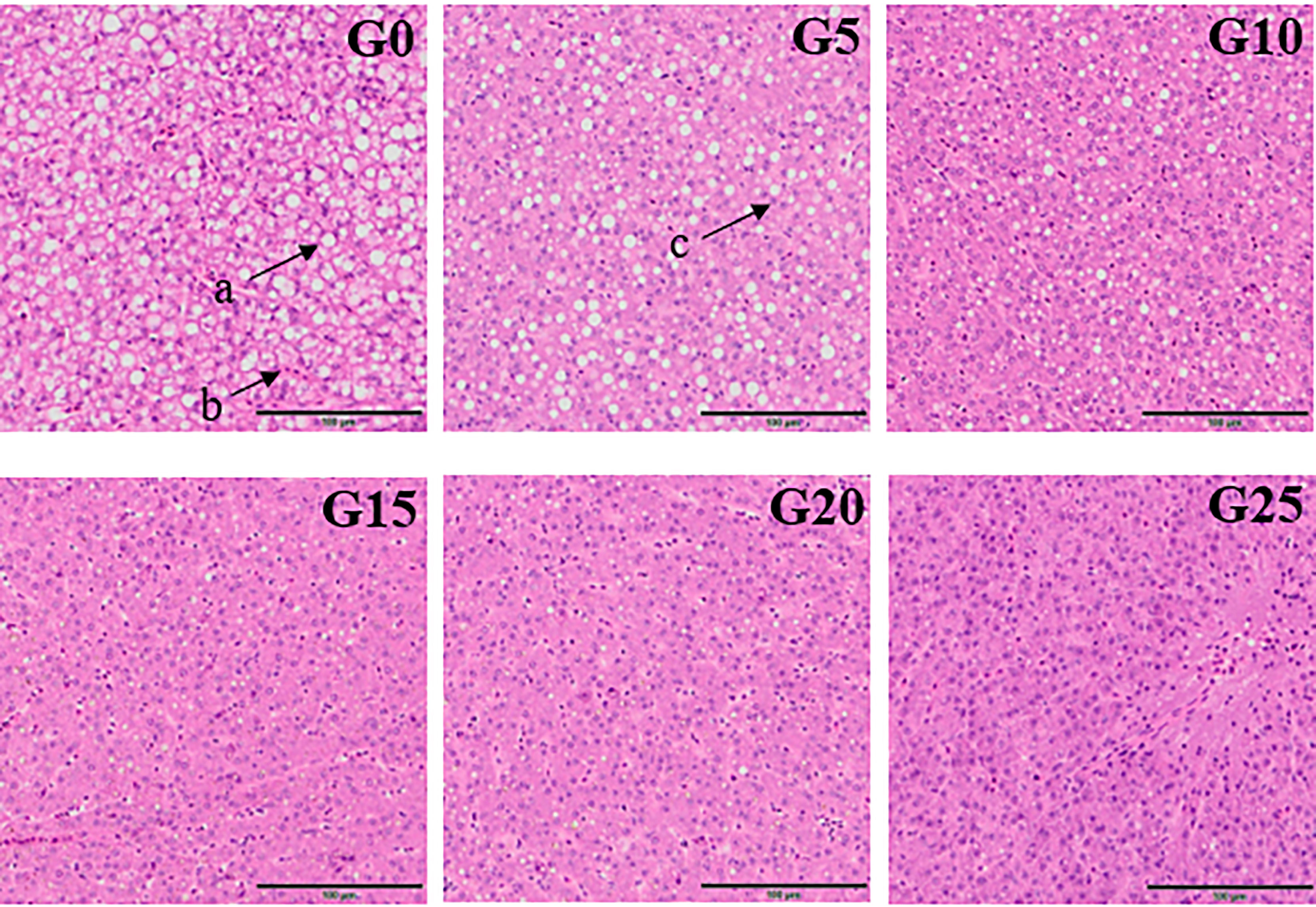
Figure 6 Electron microscope scanner (VS 120-S6, Olympus, Norway) of the liver tissue morphology for juvenile pompano T. ovatus fed diets with different GML diets (H & E staining). (a) Fat cavitation; (b) Hepatic sinusoid; (c) Nucleus.
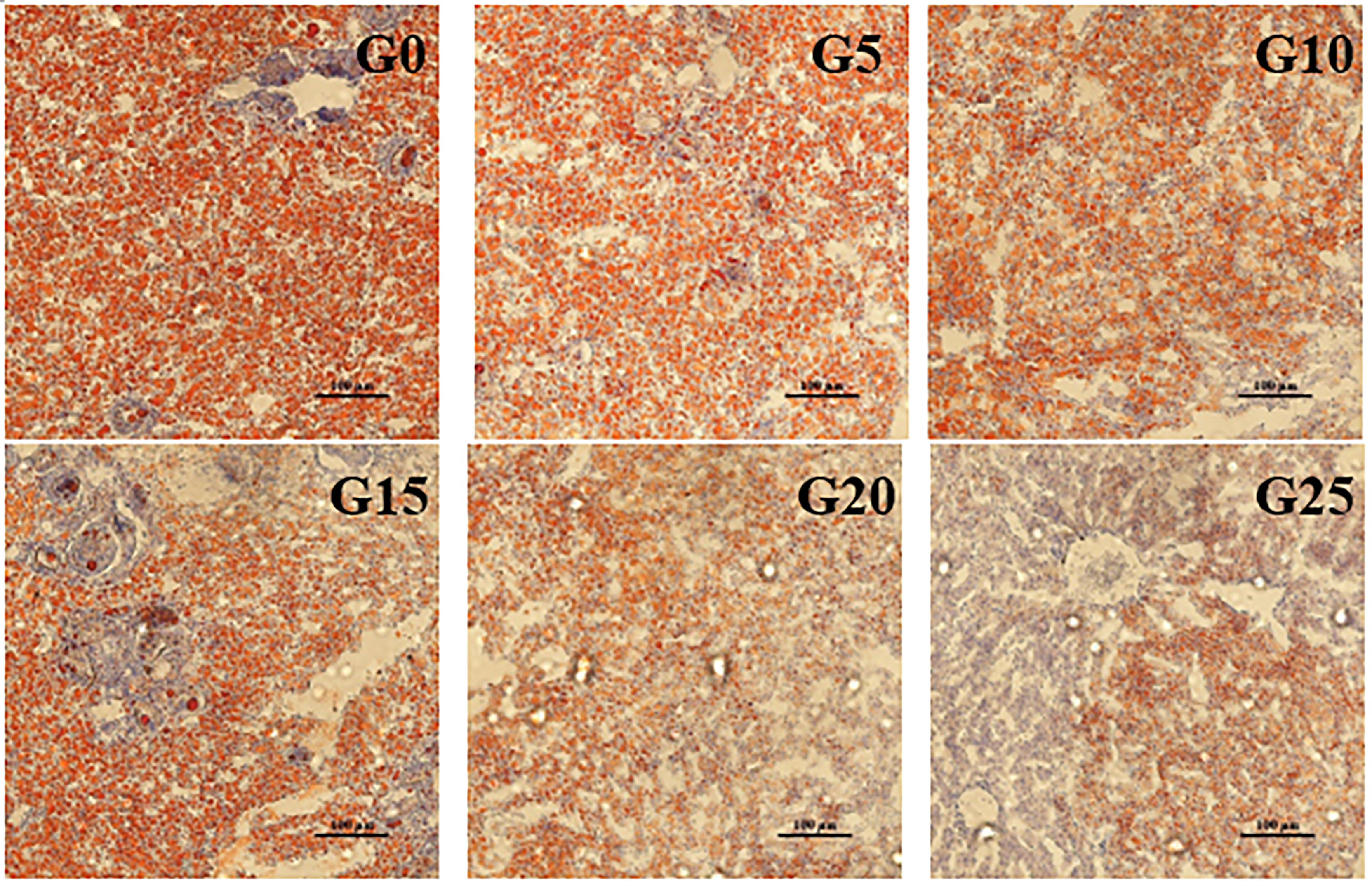
Figure 7 Light microscopy of the liver tissue morphology for juvenile pompano T. ovatus fed diets with different GML diets (Oil red staining).
Effects of GML on Expression of Lipid Metabolism-Related Genes in the Liver for Juvenile T. ovatus
As GML level increased, carnitine palmitoyl-transferase Ι (cptΙ) mRNA level was significantly upregulated (P < 0.05; Figure 8). The expression of fatty acid synthesis (fasn) in the G10, G15, G20, and G25 groups was significantly lower than that in the G0 group (P < 0.05), whereas the expression of fasn in the G5 group was not significantly different from that in G0 group (P > 0.05). The expression of apolipoprotein b-100 (apob100) was significantly higher in the G10 and G15 groups than in the G0 group (P < 0.05), whereas the other additive groups were not significantly different from the control group. The expression of sterol regulatory element-binding protein-Ι (srebp-Ι) was significantly lower in the G10, G15, G20, and G25 groups than in the G0 group (P < 0.05). No significant difference was observed in fatty acid-binding protein Ι (fabp1) mRNA level (P > 0.05).
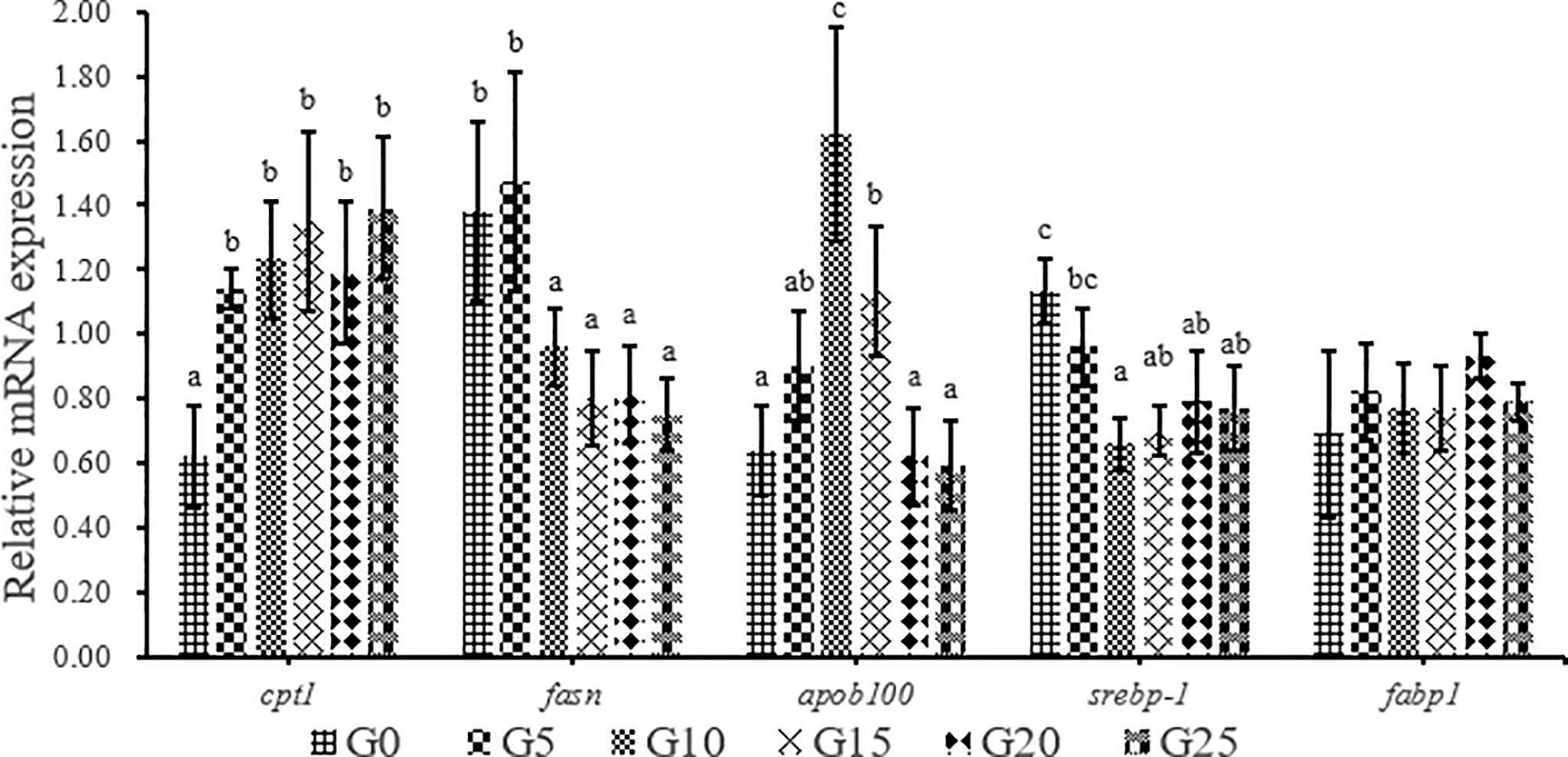
Figure 8 Relative expression levels of lipid metabolism-related genes in liver for juvenile T. ovatus. Data were expressed as means ± S.E.M. (n = 3). Different letters above a bar were statistically significant different among treatments (P < 0.05). cptΙ, carnitine palmitoyl transferase Ι; fsan, fatty acid synthesis; apob100, apolipoprotein B-100; srebp-Ι, sterol-regulatory element binding protein-Ι; fabpΙ, fatty acid binding protein Ι.
Discussion
GML is a source of energy for the body and can be oxidized as it enters the mitochondria of liver cells, thus providing energy quickly (Wojtczak and Schonfeld, 1993; Turner et al., 2009). Hence, GML is a widespread concern in animal growth (Baltić et al., 2017; Jackman et al., 2020). In Danio rerio (Wang et al., 2021), 0.075% GML supplementation significantly improved WGR and SGR, and FCR decreased along with the improvements in intestinal lipase and protease activities after 8 weeks of feeding trial. Supplementation with 0.075% GML was effectively improved the WGR of L. croceus (Jiang, 2021), increasing the yellow color of the abdominal skin and the synthesis of some nutritionally relevant amino acids, thus increasing the nutritional value of the fish. In addition, the dietary supplementation of 0.07% and 0.105% GML remarkably improved the growth performance and changed their β-diversity and composition of the gut microbiota for Litopenaeus vannamei (Wang et al., 2020c). These results are consistent with previous studies, which indicate the potential of GML as a novel growth promoter in aquafeeds (Rimoldi et al., 2018). Similarly, the results of the present study show that the addition of GML in feeds significantly improved WGR, SGR, and PDR for juvenile T. ovatus. GML is a typical medium-chain fatty acid (C6-C12) derivative, and its hydrolysis product lauric acid could enter the liver directly in free form through the portal system to participate in the body’s metabolism (Guillot et al., 1994). In the absence of apolipoproteins, lauric acid could also enter the mitochondria for oxidation and rapid energy production for the body (Hashim and Tantibhedyangkul, 1987). Therefore, GML significantly promoted the growth performance of juvenile T. ovatus. Based on the broken-line model of WGR and PDR corresponding to GML levels, the optimum addition level of dietary GML was estimated to be 0.14%-0.16%.
Serum biochemical indicators reflect the physiological status and the health and nutritional level of the body (Tu et al., 2012). The total protein content of the serum reflects the absorption, synthesis, and breakdown of protein in the body (Chen et al., 2015). In the present study, the addition of a certain amount of GML facilitated the promotion of serum TP levels. An increase of serum TP content enhanced the animals’ metabolic level, promoting the synthesis of protein and increasing nitrogen deposition (Tan et al., 2016). In the present study, the addition of GML significantly increased AKP activity, which was consistent with the significant increase in WGR for juvenile T. ovatus. This result was similar to that Pelodiscus sinensis (Wang et al., 2019). Serum ALT and AST are two sensitive indicators of the body’s liver function. When the liver is damaged by various factors, ALT and AST infiltrated the blood, thus increasing the serum concentrations of both. Therefore, increased serum ALT and AST activity indicates some degree of impairment of liver function. In the present study, GML could remarkably reduce serum ALT activity. The above serum indicators indicated that GML is non-toxic to the liver and significantly improves liver function in juvenile T. ovatus.
Oxygen-demanding organisms produce large amounts of free radicals during lipid metabolism, which was one of the main factors in peroxidative damage and antioxidant imbalances in the body (Amin and Hashem, 2012). Free radicals scavenging is a function of the antioxidant system in the body (Liang et al., 2020a). T-AOC is the total level of various antioxidant macromolecules, antioxidant small molecules and enzymes within a system (Lin et al., 2021a). SOD and GSH-Px are antioxidant enzyme systems that are closely related to free radical scavenging functions and protect important cellular macromolecules and organelles from oxidative damage (Hu et al., 2019). MDA is the end-product of lipid peroxidation triggered by a free radical attack on polyunsaturated fatty acids in biological membranes, and its concentration reflects the degree of cellular damage (Gyan et al., 2021). A study on the effect of GML on the antioxidant capacity for Lateolabrax maculatus (Sun et al., 2021) shows that GML significantly increases SOD activity and GSH-Px concentration and significantly reduces MDA concentration. The results of the present study support those of the above-mentioned studies, in which GML significantly increases SOD and GSH-Px levels and significantly decreases MDA concentrations in the liver for juvenile pompano T. ovatus. Under stress, the activity of antioxidant enzymes decreases, oxidative metabolites accumulate, and an imbalance is observed between the production and scavenging of free radicals in the body, thus triggering oxidative damage. However, in the present study, as GML increased, the structural boundaries of liver tissue cells were evident, thus improving the condition of the liver. The increased antioxidant capacity of GML can be attributed to the fact that as a medium-chain fatty acid, GML could supply energy to juvenile pompano T. ovatus, thus reducing energy consumption for the metabolism of other nutrients, reducing mitochondrial energy production and free radical production, reducing oxidative stress in the body, and restoring antioxidant capacity (An et al., 2014).
GML could significantly improve fat metabolism, reduce fat accumulation, and improve the condition of the liver (Wein et al., 2009; Nagao and Yanagita, 2010). Fat deposits in fish are mainly concentrated in the liver and muscles, and large accumulations of liver fat could affect fish nutrient metabolism, and reduce growth performance and immunity, leading to disease outbreaks and even massive fish mortality (Hixson, 2014). In the present study, the addition of GML significantly reduced the liver fat content. This finding is in line with the findings of Sun et al. (2021) and Belghit et al. (2019), who found that the addition of GML to the diet reduced liver fat content in both Lateolabrax maculatus and Salmo salar, respectively. In the present study, no significant difference was observed in muscle crude fat content, which was similar to that in Pelodiscus sinensis (Wang et al., 2019), but different from that reported for GML to reduce fat deposition in animals (Noguchi et al., 2002). This finding was obtained possibly because GML primarily accelerated lipid metabolism in juvenile T. ovatus.
The serum levels of TG, CHOL, HDL-C, and LDL-C are important indicators of the body’s lipid metabolism (He et al., 2021). Considering that lipids are mainly transported in the body by the blood, the serum concentrations of CHOL and TG could, to a certain extent, reflect the degree of lipid metabolism in the body (Wang et al., 2020b). Lipids are transported between the liver and blood in the form of HDL-C and LDL-C by using lipoproteins as carriers (Lin and Luo, 2011). A decrease in HDL-C or increase in LDL-C in the serum would affect normal lipid metabolism in the liver. In the present study, serum TG, CHOL and LDL-C levels in the GML-added group decreased with increasing levels of GML, whereas HDL-C levels increased significantly compared with the control group, suggesting that GML might affect the lipid transport process by lowering LDL-C levels, thereby reducing serum concentrations of TG and CHOL. This finding is consistent with the results obtained for Lateolabrax maculatus (Sun et al., 2021), Pelodiscus sinensis (Wang et al., 2019) and broiler (Liu et al., 2018b), where GML reduced serum TG and CHOL levels. Therefore, the addition of GML could significantly affect the changes of serum indexes, and the appropriate amount of GML could significantly reduce blood lipids and improve serum lipid metabolism in juvenile pompano T. ovatus.
The liver is the main site of lipid metabolism in fish, where the metabolic processes of lipid synthesis, catabolism, and transport proceed (Weng et al., 2012). The fasn is the key enzyme for the de novo fatty acid biosynthesis, and it catalyzes the synthesis of malonyl-CoA and acetyl coenzyme A into long-chain saturated fatty acids (He et al., 2021). Srebp1 is one of the important transcription factors that regulate adipogenesis by inducing the expression of adipogenic genes (e.g. fasn). The cptΙ is the key and rate-limiting enzyme that regulates the beta-oxidation of fatty acids, which is the main catabolic process in the body (Nilsson-Ehle et al., 1980). In the present study, GML significantly downregulated fasn and srebp1 expression and upregulated cptΙ, indicating that GML increased hepatic lipolytic enzyme activity and decreased lipid synthase levels. In addition, fat deposition is related to fat transport rate. An increase in the very-low-density lipoprotein (VLDL) secretion rate could reduce hepatic lipid deposition (Nagayoshi et al., 2008). Hussain et al. (2008) reported that apob100 is an essential component in the assembly of VLDL particles. In the present study, GML significantly upregulated apob100, thus affecting the rate of hepatic fat transport. In combination with liver histomorphology, severe lipid deposition and vacuolation of hepatocytes were observed in the control group, but the addition of GML moderated the situation in the experimental group. Moreover, the fat content of the liver was significantly reduced in the present study. Therefore, GML can regulate the liver fat metabolism related to juvenile T. ovatus. A similar conclusion was reached in Lateolabrax maculatus (Sun et al., 2021), indicating that GML could promote lipid metabolism and prevent lipid deposition in fish.
Conclusion
In conclusion, our study indicated that 0.15% GML significantly improved growth performance and enhanced hepatic antioxidant capacity for juvenile pompano T. ovatus. In addition, GML promoted lipid metabolism in juvenile T. ovatus by regulating serum lipid metabolism-related enzyme activity, significantly reducing liver fat deposition and regulating liver lipid metabolism-related genes, thereby protecting the liver condition for juvenile T. ovatus. Based on the broken-line model of WGR and PDR corresponding to GML levels, the optimum addition level of dietary GML was estimated to be 0.14%-0.16%.
Data Availability Statement
The original contributions presented in the study are included in the article/supplementary material. Further inquiries can be directed to the corresponding author.
Ethics Statement
The animal study was reviewed and approved by Guangdong Ocean University.
Author Contributions
HL: Responsible for breeding experiments, data analysis and article writing. QY: Responsible for directing experiments, paper revisions, and funding acquisition. BT: Responsible for experimental data guidance and funding acquisition. GW: Responsible for the guidance of breeding experiments, and paper revisions. SC: Responsible for the guidance of breeding experiments. HW: Responsible for the maintenance of laboratory equipment. YW: Responsible for the purchase of experimental consumables. MY: Responsible for the guidance of breeding experiments, and paper revisions. All authors contributed to the article and approved the submitted version.
Funding
National Natural Science Foundation (31802316); Correspondent for science and technology of Guangdong Province (GDKTP 2021048400); National Key Research and Development Program (2019YFD0900200); Postgraduate Education Innovation Project of Guangdong Ocean University (202155).
Conflict of Interest
The authors declare that the research was conducted in the absence of any commercial or financial relationships that could be construed as a potential conflict of interest.
Publisher’s Note
All claims expressed in this article are solely those of the authors and do not necessarily represent those of their affiliated organizations, or those of the publisher, the editors and the reviewers. Any product that may be evaluated in this article, or claim that may be made by its manufacturer, is not guaranteed or endorsed by the publisher.
Acknowledgments
We thank BT, SSC, HW, and YW for taking responsibility for some works (e.g. raw materials) to ensure that the experiment was carried out correctly. QY, GW, and MY reviewed and revised the manuscript. Additional thanks to Chunfeng Yao (Guangdong Yuehai Feed Group Company Limited) for providing the experimental site.
References
Amin K. A., Hashem K. S. (2012). Deltamethrin-Induced Oxidative Stress and Biochemical Changes in Tissues and Blood of Catfish (Clarias Gariepinus): Antioxidant Defense and Role of Alpha-Tocopherol. BMC Vet. Res. 8 (1), 45. doi: 10.1186/1746-6148-8-45
An Y. P., Cui B., Wang Y. T., Jin W. P., Geng X. P., Yan X. X., et al. (2014). Functional Properties of Ovalbumin Glycosylated With Carboxymethyl Cellulose of Different Substitution Degree. Food Hydrocolloids. 40, 1–8. doi: 10.1016/j.foodhyd.2014.01.028
An W. Q., Lai W. W., Tan B. P., Yang Q. H., Dong X. H., Liu H. Y., et al. (2018). Optimum Calcium and Phosphorus Supplemental Levels in Diets of Large Size Litopenaeus Vannamei. J. Guangdong. Ocean. Univ. 38 (04), 8–19. doi: 10.3969/j.issn.1673-9159.2018.04.002
Baltić; B., Starčević; M., Đorđević; J., Mrdović; B., Marković; R. (2017). Importance of Medium Chain Fatty Acids in Animal Nutrition. IOP. Conf. Series.: Earth Environ. Sci. 85 (1). doi: 10.1088/1755-1315/85/1/012048
Belghit I., Waagbo R., Lock E. J., Liland N. S. (2019). Insect-Based Diets High in Lauric Acid Reduce Liver Lipids in Freshwater Atlantic Salmon. Aquacult. Nutr. 25 (2), 343–357. doi: 10.1111/anu.12860
Bonvini E., Parma L., Mandrioli L., Sirri R., Brachelente C., Mongile F., et al. (2015). Feeding Common Sole (Solea Solea) Juveniles With Increasing Dietary Lipid Levels Affects Growth, Feed Utilization and Gut Health. Aquaculture 449, 87–93. doi: 10.1016/j.aquaculture.2015.01.013
Chen Y. Y., Li C. L., Huang X. H. (2015). Effects of Microcystin on Activities of Immune Enzymes in the White Shrimp Litopenaeus Vannamei. J. Guangdong. Ocean. Univ. 35 (06), 35–39. doi: 10.3969/j.issn.1673-9159.2015.06.007
Chen Q., Liu H. Y., Tan B. P., Dong X. H., Chi S. Y., Yang Q. H., et al. (2016). Effects of Dietary Cholesterol Level on Growth Performance, Blood Biochemical Parameters and Lipid Metabolism of Juvenile Cobia (Rachycentron Canadum). J. Guangdong. Ocean. Univ. 36 (01), 35–43. doi: 10.3969/j.issn.1673-9159.2016.01.007
Fang H. H., Xie J. J., Zhao W., Liu Z. L., Liu Y. J., Tian L. X., et al. (2021). Study Supplementation of Astaxanthin in High-Fat Diet on Growth Performance, Antioxidant Ability, Anti-Inflammation, non-Specific Immunity and Intestinal Structure of Juvenile Trachinotus Ovatus. Aquacult. Nutr. 27 (6), 2575–2586. doi: 10.1111/anu.13386
Guillot E., Lemarchal P., Dhorne T., Rerat A. (1994). Intestinal Absorption of Medium Chain Fatty Acids: In Vivo Studies in Pigs Devoid of Exocrine Pancreatic Secretion. Br. J. Nutr. 72 (4), 545–553. doi: 10.1079/bjn19940058
Gui L., Mai H., Chi S., Zhou W., Li Y., Tan B., et al. (2019). Effects of Yeast Culture on Growth Performance, Hematological Parameters, Immunity and Disease Resistance of Litopenaeus Vannamei. J. Guangdong. Ocean. Univ. 39 (03), 30–37. doi: 10.3969/j.issn.1673-9159.2019.03.005
Guo H. J., Chen C. Y., Yan X., Li Y. Y., Wen X. B., You C. H., et al. (2021). Effects of Different Dietary Oil Sources on Growth Performance, Antioxidant Capacity and Lipid Deposition of Juvenile Golden Pompano Trachinotus Ovatus. Aquaculture 530, 735923. doi: 10.1016/j.aquaculture.2020.735923
Gyan W. R., Yang Q. H., Tan B. P., Dong X. H., Chi S. Y., Liu H. Y., et al. (2021). Effects of Replacing Fishmeal With Dietary Dried Distillers Grains With Solubles on Growth, Serum Biochemical Indices, Antioxidative Functions, and Disease Resistance for Litopenaeus Vannamei Juveniles. Aquacult. Rep. 21, 100821. doi: 10.1016/j.aqrep.2021.100821
Gyan W. R., Yang Q., Tan B., Jan S. S., Jiang L., Chi S., et al. (2020). Effects of Antimicrobial Peptides on Growth, Feed Utilization, Serum Biochemical Indices and Disease Resistance of Juvenile Shrimp, Litopenaeus Vannamei. Aquacult. Res. 51 (3), 1222–1231. doi: 10.1111/are.14473
Han Y. S., Tang C. H., Zhao Q. Y., Zhan T. F., Zhang K., Han Y. M., et al. (2018). Effects of Dietary Supplementation With Combinations of Organic and Medium Chain Fatty Acids as Replacements for Chlortetracycline on Growth Performance, Serum Immunity, and Fecal Microbiota of Weaned Piglets. Livestock. Sci. 216, 210–218. doi: 10.1016/j.livsci.2018.08.013
Hashim S. A., Tantibhedyangkul P. (1987). Medium Chain Triglyceride in Early Life: Effects on Growth of Adipose Tissue. Lipids 22 (6), 429–434. doi: 10.1007/BF02537274
He S., Ding M., Watson Ray G., Yang Q., Tan B., Dong X., et al. (2021). Effect of Dietary Vitamin D Levels on Growth, Serum Biochemical Parameters, Lipid Metabolism Enzyme Activities, Fatty Acid Synthase and Hepatic Lipase mRNA Expression for Orange-Spotted Grouper (Epinephelus Coioides) in Growth Mid-Stage. Aquacult. Nutr. 27 (3), 655–665. doi: 10.1111/anu.13212
Hixson S. M. (2014). Fish Nutrition and Current Issues in Aquaculture: The Balance in Providing Safe and Nutritious Seafood, in an Environmentally Sustainable Manner. J. Aquacult. Res. Dev. 03 (03). doi: 10.4172/2155-9546.1000234
Horwitz W. (2010). “Official Methods of Analysis of AOAC International,” in Volume I, Agricultural Chemicals, Contaminants, Drugs. Ed. Horwitz W. (Gaithersburg (Maryland: AOAC International).
Hu B., Song L. P., Mao S. Q., Xu P. (2019). Effects of Four Chinese Herbal Preparations on Growth Performance and Antioxidant Activity in Juvenile Micropterus Salmoides. J. Guangdong. Ocean. Univ. 39 (04), 101–107. doi: 10.3969/j.issn.1673-9159.2019.04.015
Hussain M. M., Rava P., Pan X., Dai K., Dougan S. K., Iqbal J., et al. (2008). Microsomal Triglyceride Transfer Protein in Plasma and Cellular Lipid Metabolism. Curr. Opin. Lipidol. 19 (3), 277–284. doi: 10.1097/MOL.0b013e3282feea85
Jackman J. A., Hakobyan A., Zakaryan H., Elrod C. C. (2020). Inhibition of African Swine Fever Virus in Liquid and Feed by Medium-Chain Fatty Acids and Glycerol Monolaurate. J. Anim. Sci. Biotechnol. 11 (1), 114. doi: 10.1186/s40104-020-00517-3
Jiang H. Q. (2021). The Effect of Glycerol Monolaurate on Growth, Health and Food Quality of Cultured Large Yellow Croaker ( Zhejiang University).
Leaver M. J., Bautista J. M., Bjornsson B. T., Jonsson E., Krey G., Tocher D. R., et al. (2008). Towards Fish Lipid Nutrigenomics: Current State and Prospects for Fin-Fish Aquaculture. Rev. Fish. Sci. 16 (sup1), 73–94. doi: 10.1080/10641260802325278
Liang D. Z., Ma H. Y., Yang Q. H., Tan B. P., Dong X. H., Chi S. Y., et al. (2019). Efects of Selenium Sources and Selenium Levels on Growth Performance, Immune Enzyme Activities and Selenium Contents of Whole Fish and Vertebrate for Juvenile Grouper (Epinephelus Coioide). Chin. J. Anim. Nutr. 31 (06), 2777–2787. doi: 10.3969/j.issn.1006-267x.2019.06.038
Liang D. Z., Wang J. X., Gyan W. R., Yang Q. H., Tan B. P., Dong X. H., et al. (2020a). Effects of Different Dietary Levels of Soybean Protein Hydrolysates on the Growth Performance, Antioxidant Capacity and Relative mRNA Expression Levels of Juvenile Hybrid Grouper (Epinephelus Fuscoguttatus ♀ × Epinephelus Lanceolatus ♂). Aquacult. Nutr. 26 (6), 1857–1870. doi: 10.1111/anu.13134
Liang D., Yang Q., Tan B., Dong X., Chi S., Liu H., et al. (2020b). Dietary Vitamin A Deficiency Reduces Growth Performance, Immune Function of Intestine, and Alters Tight Junction Proteins of Intestine for Juvenile Hybrid Grouper (Epinephelus Fuscoguttatus ♀ × Epinephelus Lanceolatus ♂). Fish. Shellfish. Immunol. 107 (Pt A), 346–356. doi: 10.1016/j.fsi.2020.10.016
Liang D., Zheng Q., Yang Q., Tan B., Dong X., Chi S., et al. (2021). Alterations on Growth Performance, Antioxidant Responses and Lipid Metabolism in Liver for Juvenile Hybrid Grouper (Epinephelus Fuscoguttatus ♀ ×Epinephelus Lanceolatus ♂) Fed Dietary Vitamin E. Aquacult. Rep. 21, 100862. doi: 10.1016/j.aqrep.2021.100862
Li S. Y., C Z. Z. (2009). Effect of Dietary Rhubarb Levels on Growth and Non-Specific Immune Response of White Shrimp, Litopenaeus Vannamei. J. Guangdong Ocean Univ. 29(06), 36−41.
Li W., Li L., Liu H., Tan B., Dong X., Yang Q., et al. (2022). Effects of Clostridium Butyricum on Growth, Antioxidant Capacity and non-Specific Immunology of Litopenaeus Vannamei Fed With Concentrated Cottonseed Protein Replacement of Fishmeal. J. Guangdong. Ocean. Univ. 42 (2), 1–10.
Lin H., Ding M., Ray G. W., Tan B., Yang Q. (2022). Vitamin D Promotes Growth, Feed Utilization and Gene Expression Related to Lipid Metabolism for Juvenile Orange-Spotted Grouper Epinephelus Coioides. Aquacult. Rep. 23, 101074. doi: 10.1016/j.aqrep.2022.101074
Lin S. M., Luo L. (2011). Effects of Different Levels of Soybean Meal Inclusion in Replacement for Fish Meal on Growth, Digestive Enzymes and Transaminase Activities in Practical Diets for Juvenile Tilapia, Oreochromis Niloticus × O. Aureus. Anim. Feed. Sci. Technol. 168 (1-2), 80–87. doi: 10.1016/j.anifeedsci.2011.03.012
Lin H., Tan B., Watson G., Zeng M., Li M., Chi S., et al. (2021b). A Challenge to Conventional Fishmeal: Effects of Soy Protein Peptides on Growth, Histomorphology, Lipid Metabolism and Intestinal Health for Juvenile Pompano Trachinotus Ovatus. Front. Mar. Sci. 2135. doi: 10.3389/fmars.2021.815323
Lin A. Y., Wang W. Z., Chen G., Zhang J. D., Huang J. S., Pan C. H., et al. (2020). Effects of Two Lactic Acid Bacteria on Growth Performance and Activities of Digestive and non-Specific Immune Enzymes of Juvenile Cobia (Rachycentron Canadum). J. Guangdong. Ocean. Univ. 40 (05), 112–117. doi: 10.3969/j.issn.1673-9159.2020.05.014
Lin H., Yang Q., Wang A., Wang J., Tan B., Ray G. W., et al. (2021a). Effects of Fish Meal Under Different Storage Conditions on Growth, Serum Biochemical Indices and Antioxidant Capacity for Juvenile Grouper Epinephelus Coioides. Aquacult. Nutr. 27 (3), 723–733. doi: 10.1111/anu.13218
Liu M. Y., Chen X. Q., Zhao H. B., Feng F. Q. (2018b). Effect of Dietary Supplementation With Glycerol Monolaurate on Growth Performance, Digestive Ability and Chicken Nutritional Components of Broilers. Food Sci. 39 (15), 67–71. doi: 10.7506/spkx1002-6630-201815010
Liu H. Y., Li L. X., Ayiku A., Tang Z., Fan W., Tan B. P., et al. (2021). Effects of Dietary Yeast Culture Supplementation on Growth, Intestinal Morphology, Immune, and Disease Resistance in Epinephelus Fuscoguttatus ♀ × Epinephelus Lanceolatu ♂. J. Guangdong. Ocean. Univ. 41 (03), 1–11. doi: 10.3969/j.issn.1673-9159.2021.03.001
Liu K., Liu H. Y., Chi S. Y., Dong X. H., Yang Q. H., Tan B. P. (2018a). Effects of Different Dietary Lipid Sources on Growth Performance, Body Composition and Lipid Metabolism-Related Enzymes and Genes of Juvenile Golden Pompano, Trachinotus Ovatus. Aquacult. Res. 49 (2), 717–725. doi: 10.1111/are.13502
Liu J. H., Yang H. S., Wang H. (2013). Effects of Continuous High Temperature on Digestive Enzyme and Lysozyme Activities of Young GIFT Oreochromis Niloticus. J. Guangdong. Ocean. Univ. 33 (06), 47–51.
Livak K. J., Schmittgen T. D. (2002). Analysis of Relative Gene Expression Data Using Real-Time Quantitative PCR and the 2–ΔΔCT Method. Methods 25, 402–408. doi: 10.1006/meth.2001.1262
Li M., Xu C., Ma Y., Ye R., Chen H., Xie D., et al. (2020). Effects of Dietary N-3 Highly Unsaturated Fatty Acids Levels on Growth, Lipid Metabolism and Innate Immunity in Juvenile Golden Pompano (Trachinotus Ovatus). Fish. Shellfish. Immunol. 105, 177–185. doi: 10.1016/j.fsi.2020.06.060c
Ma H. Y., Yang Q. H., Dong X. H., Tan B. P., Chi S. Y., Liu H. Y. (2014). Dietary Requirement of Juvenile Grouper, Epinephelus Coioides for Hydroxy Methionine Copper. J. Guangdong. Ocean. Univ. 34 (04), 19–26.
Nagao K., Yanagita T. (2010). Medium-Chain Fatty Acids: Functional Lipids for the Prevention and Treatment of the Metabolic Syndrome. Pharmacol. Res. 61 (3), 208–212. doi: 10.1016/j.phrs.2009.11.007
Nagayoshi A., Matsuki N., Saito H., Tsukamoto K., Kaneko K., Wakashima M., et al. (2008). Defect in Assembly Process of Very-Low-Density Lipoprotein in Suncus Liver: An Animal Model of Fatty Liver. Japanese. Biochem. Soc. 117 (4), 787−793.
Nilsson-Ehle P., Garfinkel A. S., Schotz M. C. (1980). Lipolytic Enzymes and Plasma Lipoprotein Metabolism. Annu. Rev. Biochem. 49, 667–693. doi: 10.1146/annurev.bi.49.070180.003315
Noguchi O., Takeuchi H., Kubota F., Tsuji H., Aoyama T. (2002). Larger Diet-Induced Thermogenesis and Less Body Fat Accumulation in Rats Fed Medium-Chain Triacylglycerols Than in Those Fed Long-Chain Triacylglycerols. J. Nutr. Sci. Vitaminol. (Tokyo). 48 (6), 524–529. doi: 10.3177/jnsv.48.524
Ray G. W., Liang D., Yang Q., Tan B., Dong X., Chi S., et al. (2020). Effects of Replacing Fishmeal With Dietary Soybean Protein Concentrate (SPC) on Growth, Serum Biochemical Indices, and Antioxidative Functions for Juvenile Shrimp Litopenaeus Vannamei. Aquaculture 516, 734630. doi: 10.1016/j.aquaculture.2019.734630
Rimoldi S., Gliozheni E., Ascione C., Gini E., Terova G. (2018). Effect of a Specific Composition of Short- and Medium-Chain Fatty Acid 1-Monoglycerides on Growth Performances and Gut Microbiota of Gilthead Sea Bream (Sparus Aurata). Peerj 6, e5355. doi: 10.7717/peerj.5355
Shokrollahi B., Yavari Z., Kordestani A. H. (2014). Effects of Dietary Medium-Chain Fatty Acids on Performance, Carcass Characteristics, and Some Serum Parameters of Broiler Chickens. Br. Poult. Sci. 55 (5), 662–667. doi: 10.1080/00071668.2014.955836
Song L. P., Hu B., Wang A. Y., Cao S. Q., Zhang Y. H., Pan X. L. (2010). Effects of Antibacterial Peptide on Growth and Immunity of Penaeus Vannamei. J. Guangdong. Ocean. Univ. 30 (03), 28–32.
Sun C. Y., Dong H. B., Wang W. H., Li Y., Gu Q. H., Duan Y. F., et al. (2021). Effect of Glycerol Monolaurate(GML) on Lipid Metabolism of Lateolabrax Maculatus. South. Fish. Sci. 17 (01), 67–75. doi: 10.12131/20200130
Sun P., Jin M., Ding L. Y., Lu Y., Ma H. N., Yuan Y., et al. (2018). Dietary Lipid Levels Could Improve Growth and Intestinal Microbiota of Juvenile Swimming Crab, Portunus Trituberculatus. Aquacult. 490., 208–216. doi: 10.1016/j.aquaculture.2018.02.018
Tan X. H., Lin H. Z., Huang Z., Zhou C. P., Wang A. L., Qi C. L., et al. (2016). Effects of Dietary Leucine on Growth Performance, Feed Utilization, non-Specific Immune Responses and Gut Morphology of Juvenile Golden Pompano Trachinotus Ovatus. Aquaculture 465, 100–107. doi: 10.1016/j.aquaculture.2016.08.034
Tu G. X., Chen G., Zhou H., Wang S., Ji D. L., Zhang J. D., et al. (2012). Effects of Partial Replacement Dietary Fish Meal by Three Kinds of Protein Sources on Haematological Indices of Juvenile Epinephelus Fuscoguttatus. J. Guangdong. Ocean. Univ. 32 (4), 12–19.
Turner N., Hariharan K., TidAng J., Frangioudakis G., Beale S. M., Wright L. E., et al. (2009). Enhancement of Muscle Mitochondrial Oxidative Capacity and Alterations in Insulin Action are Lipid Species Dependent: Potent Tissue-Specific Effects of Medium-Chain Fatty Acids. Diabetes 58 (11), 2547–2554. doi: 10.2337/db09-0784
Tutman P., Glavić N., Skaramuca B., Glamuzina B. (2004). Preliminary Information on Feeding and Growth of Pompano, Trachinotus Ovatus (Linnaeu) (Pisces; Carangidae) in Captivity. Aquacult. Int. 12 (4/5), 387–393. doi: 10.1023/B:AQUI.0000042135.88381.f4
Van Immerseel F., De Buck J., Boyen F., Bohez L., Pasmans F., Volf J., et al. (2004). Medium-Chain Fatty Acids Decrease Colonization and Invasion Through hilA Suppression Shortly After Infection of Chickens With Salmonella Enterica Serovar Enteritidis. Appl. Environ. Microbiol. 70 (6), 3582–3587. doi: 10.1128/AEM.70.6.3582-3587.2004
Wang Y., Abdullah, Zhang C., Li Y., Zhang H., Wang J., et al. (2020c). Effects of Dietary Glycerol Monolaurate on the Growth Performance, Digestive Enzymes, Body Composition and non-Specific Immune Response of White Shrimp (Litopenaeus Vannamei). Aquacult. Rep. 18. doi: 10.1016/j.aqrep.2020.100535
Wang Y., Abdullah, Zhong H., Wang J., Feng F., , (2021). Dietary Glycerol Monolaurate Improved the Growth, Activity of Digestive Enzymes and Gut Microbiota in Zebrafish (Danio Rerio). Aquacult. Rep. 20, 100670. doi: 10.1016/j.aqrep.2021.100670
Wang Y., Du Z., Li Y., Zhang H., Feng F. (2019). Effect of Glycerol Monolaurate on Growth, Health and Nutritional Quality of Chinese Soft-Shelled Turtle (Pelodiscus Sinensis). Chin. J. Anim. Nutr. 31 (01), 428–436. doi: 10.3969/j.issn.1006-267x.2019.01.050
Wang J., Liang D., Yang Q., Tan B., Dong X., Chi S., et al. (2020a). The Effect of Partial Replacement of Fish Meal by Soy Protein Concentrate on Growth Performance, Immune Responses, Gut Morphology and Intestinal Inflammation for Juvenile Hybrid Grouper (Epinephelus Fuscoguttatus Female × Epinephelus Lanceolatus Male). Fish. Shellfish. Immunol. 98 (C), 619–631. doi: 10.1016/j.fsi.2019.10.025
Wang Y., Ma X. Z., Wang F., Wu Y. B., Qin J. G., Li P. (2017a). Supplementations of Poultry by-Product Meal and Selenium Yeast Increase Fish Meal Replacement by Soybean Meal in Golden Pompano (Trachinotus Ovatus) Diet. Aquacult. Res. 48 (4), 1904–1914. doi: 10.1111/are.13028
Wang G. X., Sun Y. P., Niu F. C., He F., Mo W. Y., Zhu X. F., et al. (2017b). Effects of Exogenous Enzyme Supplementation on Digestive Enzyme Activity, Apparent Digestibility and Fecal Nitrogen and Phosphorus Content of Juvenile Yellow Catfish. J. Guangdong. Ocean. Univ. 37 (06), 19–25. doi: 10.3969/j.issn.1673-9159.2017.06.004
Wang J., Wang X., Li J., Chen Y., Yang W., Zhang L. (2015). Effects of Dietary Coconut Oil as a Medium-Chain Fatty Acid Source on Performance, Carcass Composition and Serum Lipids in Male Broilers. Asian-Australas. J. Anim. Sci. 28 (2), 223–230. doi: 10.5713/ajas.14.0328
Wang A. J., Yang Q. H., Tan B. P., Xiao W. W., Jia J., Dong X. H., et al. (2018). Effects of Enzymolytic Soybean Meal on Growth Performance, Serum Biochemical Indices, non-Specific Immunity and Disease Resistance of Juvenile Litopenaeus Vannamei. J. Guangdong. Ocean. Univ. 38 (01), 14–21. doi: 10.3969/j.issn.1673-9159.2018.01.003
Wang J. X., Zhang H. T., Yang Q. H., Tan B. P., Dong X. H., Chi S. Y., et al. (2020b). Effects of Replacing Soybean Meal With Cottonseed Meal on Growth, Feed Utilization and non-Specific Immune Enzyme Activities for Juvenile White Shrimp, Litopenaeus Vannamei. Aquacult. Rep. 16 (C), 1–6. doi: 10.1016/j.aqrep.2019.100255
Wang S. J., Zhang H. F., Zhao J., Yang Y. Q., Yang S. S. (2011). Effects of Different Salinities on the Growth and Physiology of Orange-Spotted Grouper, Epinephelus Coioides. J. Guangdong. Ocean. Univ. 31 (06), 39–44.
Wein S., Wolffram S., Schrezenmeir J., Gasperikova D., Klimes I., Sebokova E. (2009). Medium-Chain Fatty Acids Ameliorate Insulin Resistance Caused by High-Fat Diets in Rats. Diabetes Metab. Res. Rev. 25 (2), 185–194. doi: 10.1002/dmrr.925
Weng X. J., Wu X. F., Tian J., Li X. Q., Guan L., Weng D. C. (2012). Molecular Cloning of Fatty Acid Synthase From Grass Carp (Ctenopharyngodon Idella) and the Regulation of its Expression by Dietary Fat Level. Aquacult. Nutr. 18 (5), 551–558. doi: 10.1111/j.1365-2095.2011.00917.x
Wojtczak L., Schonfeld P. (1993). Effect of Fatty Acids on Energy Coupling Processes in Mitochondria. Biochim. Biophys. Acta 1183 (1), 41–57. doi: 10.1016/0005-2728(93)90004-y
Wu Y. C., Li R. M., Shen G. R., Huang F., Yang Q. H., Tan B. P., et al. (2021). Effects of Dietary Small Peptides on Growth, Antioxidant Capacity, Nonspecific Immunity and Ingut Microflora Structure of Litopenaeus Vannamei. J. Guangdong. Ocean. Univ. 41 (05), 1–9. doi: 10.3969/j.issn.1673-9159.2021.05.001
Xie J., Fang H., Liao S., Guo T., Yin P., Liu Y., et al. (2019). Study on Schizochytrium Sp. Improving the Growth Performance and non-Specific Immunity of Golden Pompano (Trachinotus Ovatus) While Not Affecting the Antioxidant Capacity. Fish. Shellfish. Immunol. 95, 617–623. doi: 10.1016/j.fsi.2019.10.028
Xie J. H., Qiu D. Q., Liu C. X., Zhu W. W., Zeng L. (2013). Effcets of Vibrio Alginolyticus Peptidoglycan on Astaxanthin Level, Immune Indicators and Protection in Litopenaeus Vannamei. J. Guangdong. Ocean. Univ. 33 (01), 50–55.
Yang Q., Ding M., Tan B., Dong X., Chi S., Zhang S., et al. (2017). Effects of Dietary Vitamin A on Growth, Feed Utilization, Lipid Metabolism Enzyme Activities, and Fatty Acid Synthase and Hepatic Lipase mRNA Expression Levels in the Liver of Juvenile Orange Spotted Grouper. Epinephelus. coioides. Aquacult. 479, 501–507. doi: 10.1016/j.aquaculture.2017.06.024
Yin F. C., Wu Z. M., Wang C. J., Wu H. H., Nawab A., Zhuang G. F., et al. (2018). Effects of Different Concentrate to Forage Ratio on Growth Performance, Blood Biochemistry and Rumen Microbial Diversity of Leizhou Goats. J. Guangdong. Ocean. Univ. 38 (01), 80–86. doi: 10.3969/j.issn.1673-9159.2018.01.011
Zhang M., Chen C. Y., You C. H., Chen B. J., Wang S. Q., Li Y. Y. (2019). Effects of Different Dietary Ratios of Docosahexaenoic to Eicosapentaenoic Acid (DHA/EPA) on the Growth, non-Specific Immune Indices, Tissue Fatty Acid Compositions and Expression of Genes Related to LC-PUFA Biosynthesis in Juvenile Golden Pompano Trachinotus Ovatus. Aquaculture 505, 488–495. doi: 10.1016/j.aquaculture.2019.01.061
Zhu X. F., Deng Q. X., Guo H., Li G. L., Zhu C. H. (2021a). Effect of Hydrolyzable Tannins on Hemolymph and Cellular Immunological Responses of Litopenaeus Vannamei Challenged by Vibrio Parahaemolyticus. J. Guangdong. Ocean. Univ. 41 (03), 12–19. doi: 10.3969/j.issn.1673-9159.2021.03.002
Zhu Q. G., Lin J. B., Huang C. J., Chen D. H., Liang P., Qin C. Q., et al. (2012). Effects of Dietary N-3 Highly Unsaturated Fatty Acids on Growth and Muscle Fatty Acid Composition of Juvenile Grouper (Epinephlus Coioides). J. Guangdong. Ocean. Univ. 32 (04), 20–27.
Zhu Z. H., Li R. M., Yang Q. H., Tan B. P., Dong X. H., Chi S. Y., et al. (2021c). The Potential Advantage of Myo-Inositol as a Dietary Supplement for Juvenile Hybrid Grouper (Brown-Marbled Grouper Epinephelus Fuscoguttatus Female × Giant Grouper Epinephelus Lanceolatus Male). North Am. J. Aquacult. 84 (1), 74–82. doi: 10.1002/naaq.10217
Zhu Z. H., Yang Q. H., Tan B. P., Zhou X. Q., Dong X. H., Chi S. Y., et al. (2021b). Effects of Replacing Fishmeal With Soybean Protein Concentrate (SPC) on Growth, Blood Biochemical Indexes, non-Specific Immune Enzyme Activity, and Nutrient Apparent Digestibility for Juvenile Litopenaeus Vannamei. Aquacult. Int. 29 (6), 2535–2554. doi: 10.1007/s10499-021-00765-8
Keywords: Trachinotus ovatus, glycerol monolaurate, growth performance, antioxidant capacity, lipid metabolism
Citation: Lin H, Tan B, Yang Q, Chi S, Wei H, Wu Y, Ray GW and Yohana MA (2022) Effects of Dietary Glycerol Monolaurate on Growth, Antioxidant Capacity and Lipid Metabolism in Cage-Farmed Pompano (Trachinotus ovatus) Juveniles. Front. Mar. Sci. 9:914134. doi: 10.3389/fmars.2022.914134
Received: 06 April 2022; Accepted: 07 June 2022;
Published: 12 July 2022.
Edited by:
Eduardo Almansa, Spanish Institute of Oceanography (IEO), SpainReviewed by:
Hany M. R. Abdel-Latif, Alexandria University, EgyptMingchun Ren, Chinese Academy of Fishery Sciences, China
Copyright © 2022 Lin, Tan, Yang, Chi, Wei, Wu, Ray and Yohana. This is an open-access article distributed under the terms of the Creative Commons Attribution License (CC BY). The use, distribution or reproduction in other forums is permitted, provided the original author(s) and the copyright owner(s) are credited and that the original publication in this journal is cited, in accordance with accepted academic practice. No use, distribution or reproduction is permitted which does not comply with these terms.
*Correspondence: Qihui Yang, qihuiyang03@163.com
†ORCID ID: Qihui Yang, orcid.org/0000-0001-9107-2541
Huaxing Lin, orcid.org/0000-0003-2333-6327