Treatment of Infections Due to MDR Gram-Negative Bacteria
- 1Clinica Malattie Infettive, Azienda Sanitaria Universitaria Integrata di Udine, Presidio Ospedaliero Universitario Santa Maria della Misericordia, Udine, Italy
- 2Department of Health Sciences, University of Genoa, Genoa, Italy
The treatment of multidrug-resistant Gram-negative bacteria (MDR-GNB) infections in critically ill patients presents many challenges. Since an effective treatment should be administered as soon as possible, resistance to many antimicrobial classes almost invariably reduces the probability of adequate empirical coverage, with possible unfavorable consequences. In this light, readily available patient's medical history and updated information about the local microbiological epidemiology remain critical for defining the baseline risk of MDR-GNB infections and firmly guiding empirical treatment choices, with the aim of avoiding both undertreatment and overtreatment. Rapid diagnostics and efficient laboratory workflows are also of paramount importance both for anticipating diagnosis and for rapidly narrowing the antimicrobial spectrum, with de-escalation purposes and in line with antimicrobial stewardship principles. Carbapenem-resistant Enterobacteriaceae, Pseudomonas aeruginosa, and Acinetobacter baumannii are being reported with increasing frequencies worldwide, although with important variability across regions, hospitals and even single wards. In the past few years, new treatment options, such as ceftazidime/avibactam, meropenem/vaborbactam, ceftolozane/tazobactam, plazomicin, and eravacycline have become available, and others will become soon, which have provided some much-awaited resources for effectively counteracting severe infections due to these organisms. However, their optimal use should be guaranteed in the long term, for delaying as much as possible the emergence and diffusion of resistance to novel agents. Despite important progresses, pharmacokinetic/pharmacodynamic optimization of dosages and treatment duration in critically ill patients has still some areas of uncertainty requiring further study, that should take into account also resistance selection as a major endpoint. Treatment of severe MDR-GNB infections in critically ill patients in the near future will require an expert and complex clinical reasoning, of course taking into account the peculiar characteristics of the target population, but also the need for adequate empirical coverage and the more and more specific enzyme-level activity of novel antimicrobials with respect to the different resistance mechanisms of MDR-GNB.
Introduction
In the last 15 years, intensivists, and infectious diseases consultants have started to face novel peculiar challenges in the treatment of severe infections in critically ill patients in intensive care units (ICU), due to the selection and diffusion of multidrug-resistant Gram-negative bacteria (MDR-GNB) (1, 2).
Indeed, although the development of resistance has accompanied antimicrobial therapy since its dawn, only in recent years GNB have started, in non-negligible numbers, to manifest concomitant resistance to all commonly used classes of antimicrobials. This has forced clinicians to consider treatment approaches based on combinations of drugs with impaired activity, and/or to rediscover old drugs with suboptimal pharmacokinetics and toxicity issues, all in the absence of high-level evidence to firmly guide bedside decisions (3, 4). Fortunately, this situation has started to change very recently, owing to the introduction into the market of novel drugs with potent activity against some MDR-GNB such as carbapenem-resistant Enterobacterales (CRE) and carbapenem-resistant Pseudomonas aeruginosa (CRPA) (5, 6).
Nonetheless, the availability of novel agents does not automatically imply an easy and always successful treatment, for several reasons: (i) most of available novel agents have still suboptimal activity against carbapenem-resistant Acinetobacter baumannii (CRAB); (ii) activity against CRE of novel β-lactam/β-lactamase inhibitors (BL-BLI) is dependent of the type of carbapenemase conferring resistance to carbapenems; (iii) resistance to novel antibacterials has already started to emerge, and widespread use of novel antibacterials should thus be avoided, in order to relieve selective pressure for further development of resistance; (iv) on the other hand, adequate coverage for MDR should be empirically guaranteed in critically-ill patients with severe infections and risk factors for MDR, in order not to delay active treatment (7–9). With so many factors at stake, treatment of MDR-GNB infections in critically-ill patients are becoming a very complex task, which requires dedicated expertise, as well as an always updated knowledge of the patients' medical history and the local microbiology epidemiology, in order to promptly recognize the risk of MDR-GNB and also the most likely resistance mechanisms involved.
This latter factor is particularly important in light of the renewed possibility of treating severe MDR-GNB infections with beta-lactams (some already available such as ceftazidime/avibactam, ceftolozane/tazobactam, and meropenem/vaborbactam, and others that will be available in the near future), that inevitably raise the question as to whether the type of suspected resistance determinant (e.g., the type of carbapenemase) should not only guide the choice of the better agent/s to be administered, but also the decisions about escalation and de-escalation, in order to follow antimicrobial stewardship purposes also at the enzyme-level.
In this review, we discuss both current and future therapeutic approaches to severe MDR-GNB infections in critically-ill patients in ICU.
Methods
As the basis for the present narrative review, a literature search was performed in the PubMed/MEDLINE database using various combinations of pertinent keywords (e.g., “ICU,” “Gram-negative,” “therapy,” “management,” “novel antibiotics,” “novel drugs,” “Pseudomonas,” “Acinetobacter,” “Klebsiella,” “MDR”). Subsequently, retrieved papers were discussed and further iterative searches were conducted. Ultimately, two main narrative chapters were organized as follows: (i) current treatment options for MDR-GNB in critically-ill patients; (ii) future treatment options for MDR-GNB in critically-ill patients.
Current Treatment Options for MDR-GNB in Critically-ill Patients
The main characteristics of the currently available agents for the treatment of severe MDR-GNB infections in critically-ill patients are briefly introduced in the following paragraphs, while an example of possible clinical reasoning for guiding the treatment of MDR-GNB infections in critically-ill patients with currently available options is shown in Figure 1.
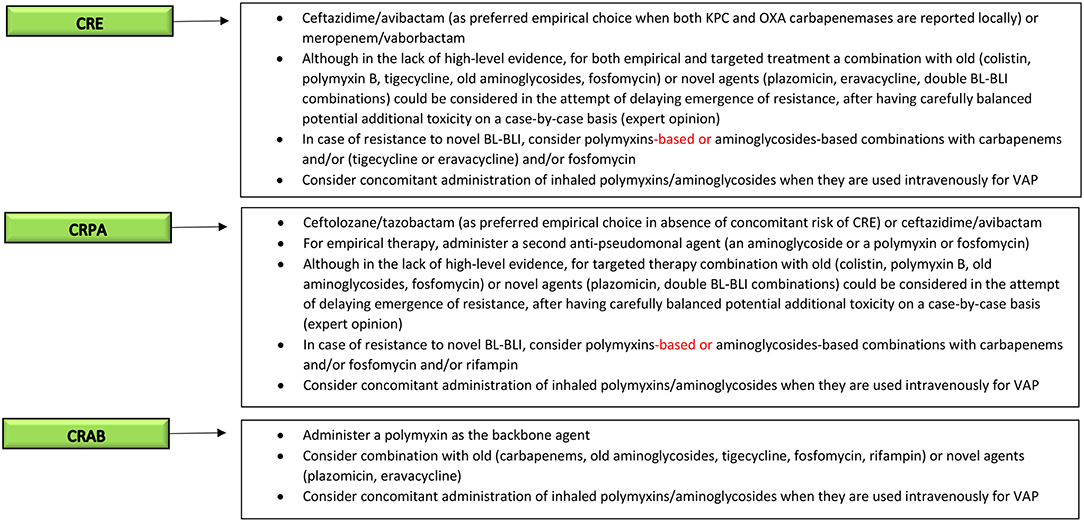
Figure 1. Current clinical reasoning for the treatment of serious MDR-GNB infections in critically-ill patients. MDR-GNB, Multi-drug resistant Gram-negative bacteria; CRE, carbapenem-resistant Enterobacterales; CRPA, carbapenem-resistant Pseudomonas aeruginosa; CRAB, carbapenem-resistant Acinetobacter baumannii; BL-BLI, β-lactam/β-lactamase inhibitors; VAP, ventilator-associated pneumonia.
Polymyxins
Polymyxins acts as detergents of the outer membrane of GNB, exerting bactericidal activity. Among those available for use in humans are colistin (polymyxin E) and polymyxin B. They were frequently used for the treatment of MDR-GNB infections in the past few years, when they often remained among the few (sometimes the only one) dependable options for CRE, CRPA, and CRAB (5, 10, 11). Nowadays, they are still among the first-line treatment options for CRAB infections (pending approval of more effective agents), whereas for CRE and CRPA already available novel agents should be preferred whenever possible, owing to the potential polymyxin-associated risks either of nephrotoxicity or of suboptimal concentrations (especially in the lung) (12). Furthermore, worrisome trends of increasing resistance have been reported in some countries (e.g., Italy and Greece) (13, 14). Consequently, the use of polymyxins should be optimized as much as possible in terms of dosages and indications, in order both to maximize effectiveness and to curb the emergence of further polymyxin resistance. For this reason, an international consensus document has been developed very recently, for guiding the proper use of polymyxins on all those occasions (e.g., CRAB infections, CRE and CRPA resistant to novel BL-BLI) when they still remain essential (12).
Aminoglycosides
Aminoglycosides are concentration-dependent, bactericidal agents which inhibit the bacterial S30 ribosomal subunit. They have been frequently used in recent years for the treatment of various carbapenem-resistant GNB, frequently in case of polymyxin resistance (15, 16). However, very similar to polymyxins, two factors hamper the effective use of classical aminoglycosides (i.e., gentamicin, amikacin, tobramycin) for the treatment of MDR-GNB infections: (i) potential nephrotoxicity and reduced lung concentrations (17); (ii) increasing rates of resistance (18). With regard to this latter point, low rates of resistance have conversely been reported in vitro for plazomicin, a novel aminoglycoside derivative of sisomicin, which retains stability against several aminoglycoside-modifying enzymes (19). Its activity in vitro seems higher against CRE than against CRPA and CRAB, although possible resistance has been described in some NDM-1-producing CRE, conferred by co-expression of plazomicin-inactivating methyltransferases in these strains (5, 20). Plazomicin is currently approved by the Food and Drug Administration (FDA) for the treatment of complicated urinary tract infection (cUTI), based on the results of the phase 3 EPIC trial, in which superiority of plazomicin vs. meropenem was shown (21). In a smaller randomized study, lower mortality was observed in patients with severe CRE infections receiving plazomicin than in those receiving colistin plus tigecycline or meropenem, although the evidence was not considered substantial to achieve FDA approval for bloodstream infections (BSI) (22). An application has been recently submitted to European Medicines Agency (EMA) for approval of plazomicin for cUTI and other severe infections.
Tigecycline
Tigecycline, a glycylcycline antibiotic which binds to the bacterial 30S ribosomal subunit, usually display activity against CRE and CRAB, but not CRPA, since P. aeruginosa is inherently resistant (23). Tigecycline has been used in combination with other agents for the treatment of severe CRE and CRAB infections, based mainly on favorable in vitro studies and observational experiences (24, 25). Of note, caution is needed in using tigecycline for ventilator-associated pneumonia (VAP), in line with an FDA warning based on pooled data from randomized clinical trials, reporting possible increased mortality in comparison with other regimens (26). When alternatives are not available and tigecycline is used for pneumonia, higher dosages should be employed for achieving sufficient PK/PD targets (27).
Carbapenems
Although apparently paradoxical, the use of carbapenems in combinations with other agents for the treatment of carbapenem-resistant MDR-GNB was frequent in the past (before the availability of novel BL-BLI). This approach was based on the possibility of achieving sufficient carbapenem concentrations against some resistant organisms with only slightly increased carbapenem MICs, and also because of possible synergistic effects (28–31). According to the results of large observational studies, this approach seemed ultimately favorable for severe CRE infections caused by KPC-producing strains, whereas a recent randomized, controlled trial (RCT) did not find differences in survival between meropenem plus colistin vs. colistin alone for the treatment of severe CRAB infections (10, 11, 32). In this regard, the high mortality reported in the trial in our opinion further stress the need for novel effective agents against CRAB (5). There are very few small observational studies on carbapenem-including combinations for CRPA, and no firm conclusions can be drawn.
Fosfomycin
Fosfomycin is an analog of phosphoenolpyruvate which interferes with the formation of UDP N-acetylmuramic acid, a peptidoglycan precursor. Its intravenous formulation has been used for the treatment of MDR-GNB, often in combinations with other agents, when few or none active alternatives were available (16, 33). In a small RCT including 94 patients with CRAB infections treated with colistin plus fosfomycin vs. colistin alone, higher rates of microbiological response were observed in the combination arm, but no appreciable differences were observed in survival time (34). In a small series of 48 critically-ill patients with MDR-GNB infections who received fosfomycin (mostly in combination with tigecycline or colistin) at the dosage of 8 g every 8 h for 14 days, all-cause 28-day mortality was 37.5% (35). In our opinion, because of the absence of larger studies and the reported risk of rapid selection of resistance, it might still be prudent to reserve the use of fosfomycin for selected cases (16).
Ceftazidime/Avibactam
Ceftazidime/avibactam is a recently marketed BL-BLI combination which is active against class A (e.g., KPC) and class D (e.g., OXA) carbapenemase-producing CRE, and demonstrated activity against some CRPA isolates (36). Ceftazidime/avibactam is approved by FDA and EMA for cUTI, complicated intra-abdominal infections (cIAI), hospital-acquired pneumonia (HAP), and VAP. Furthermore, ceftazidime/avibactam received approval by EMA for infections due to GNB in adults with limited treatment options. Although efficacy of ceftazidime-avibactam in randomized clinical trials was demonstrated against ceftazidime-resistant isolates, whereas CRE were not included, its activity against the latter is supported by the favorable results of observational studies (37–39). For example, a lower 30-day mortality was observed among 104 patients with BSI due to KPC-producing K. pneumoniae BSI treated with ceftazidime-avibactam (within the compassionate use program) than among a matched cohort of 104 patients receiving other agents (36.5 vs. 55.7%, respectively, p 0.005) (39). Consequently, ceftazidime/avibactam is an important, effective, and already available option for the treatment of CRE, the use of which should be necessarily optimized according to antimicrobial stewardship principles. Indeed, some cases of resistance to ceftazidime/avibactam, conferred by blaKPC mutations, have already been reported (8, 40).
Meropenem/Vaborbactam
Meropenem/vaborbactam is another novel BL-BLI, exerting potent and specific activity against class A (e.g., KPC) carbapenemase-producing CRE. After having received approval by FDA for cUTI, meropenem/vaborbactam was recently approved by EMA for cUTI, cIAI, HAP, VAP, and infections due to aerobic Gram-negative organisms in adult patients with limited treatment options. In the double-blind, double-dummy TANGO-I trial, meropenem/vaborbactam demonstrated superiority vs. piperacillin/tazobactam for the treatment of cUTI, including acute pyelonephritis (41). The open-label TANGO-II trial, in which meropenem/vaborbactam was compared with best therapy available for CRE infections, was terminated early because of demonstrated superiority of meropenem/vaborbactam. Patients had mostly bacteremia, and rates of clinical cure were 65.6% in patients treated with meropenem/vaborbactam (21/32) and 33.3% in those receiving the comparators (5/15) (42). Against this backdrop, meropenem/vaborbactam is another novel and very effective option for KPC-producing CRE. Nonetheless, similarly to ceftazidime/avibactam, it should be used wisely, since resistance might develop (although possibly less frequently) (6, 9). In this light, we think future therapeutic algorithms for CRE infections should carefully take into account the peculiar characteristics and spectrum of activity of each of these two novel compounds, in order to maximize the effectiveness of anti-CRE therapies in each selected situation, as well as to preserve the activity of both drugs in the long-term.
Ceftolozane/Tazobactam
Ceftolozane/tazobactam is probably the novel, available BL-BLI with the most potent in vitro activity against CRPA (although not against carbapenemase-producing strains), whereas it is not active against CRE (43). Ceftolozane/tazobactam is approved by FDA and EMA for the treatment of cIAI and cUTI on the basis of the ASPECT-cIAI and ASPECT-cUTI trials (44, 45). However, the most attractive use of ceftolozane/tazobactam at the present time is perhaps the treatment of CRPA infections, also for off-label indications. Indeed, this is confirmed by the growing amount of observational post-marketing data regarding the use of ceftolozane/tazobactam for CRPA infections, in turn reflecting the frequent lack of more active in-label alternatives (46, 47). In the future, ceftolozane/tazobactam could receive approval also for HAP and VAP, since achievement of non-inferiority vs. meropenem in the ASPECT-NP trial (NCT02070757) has been recently announced (48). Finally, the possibility of using ceftolozane/tazobactam as a carbapenem-sparing option for infections due to extended-spectrum β-lactamases (ESBL)-producing Enterobacterales has also been proposed, that might be useful in selected scenarios pending confirmatory clinical and economic data (43).
Eravacycline
Eravacycline (TP-434) is a novel synthetic fluorocycline, structurally similar to tigecycline, that has been recently FDA and EMA approved for the treatment of cIAIs. Eravacycline has been synthetized to evade many resistance mechanisms observed for tetracycline. It has shown to be active against most bacteria expressing the tetracycline targeting efflux channels or containing ribosomal protection mechanisms and β-lactamases (49). As such, eravacycline has been shown to be broadly active against Gram-positive, Gram-negative, and anaerobic bacteria with the exception of P. aeruginosa and Burkholderia cenopacia (50). Importantly, it has been found to be particularly successful against CRAB, and in one in vitro study it was the more potent than any drug tested (51). Eravacycline is highly bioavailable after oral administration (more than 90%), has a high metabolic stability and low potential drug-drug interactions. It can also be administered intravenously (51).
Clinically, eravacycline has been studies in four phase 3 clinical trials in the setting of cIAI and cUTI with conflicting results: good performance in cIAI and suboptimal in cUTI. In the IGNITE 1 study, a randomized, double blind, non-inferiority trial, intravenous eravacycline at a dose of 1.0 mg/kg every 12 h was compared with ertapenem 1 gr every 24 h. Among patients in the intent-to-treat populations, clinical cures were 86.8% for eravacycline and 87.6% for ertapenem, showing non-inferiority of eravacycline (52). In the IGNITE 2 (NCT019783938) (53) and IGNITE 3 (NCT03032510) (54) eravacycline was compared with levofloxacin and ertapenem, respectively, for the treatment of cUTI. In both studies eravacycline did not reach non-inferiority, and issues regarding the possible poor urinary tract penetration have been raised. Another randomized, phase 3 trial (IGNITE 4) of eravacycline in comparison to meropenem for the treatment of cIAI demonstrated a favorable microbiological response of 88.9 and 100% for eravacycline against infections due to Enterobacteriaceae and A. baumannii, respectively (55).
In our opinion, eravacycline may offer an important option for patients with cIAI caused by MDR-GNB bacteria, including CRAB. In addition, its high oral bioavailability (>90%) allows for the switch from an intravenous to an oral formulation.
Other Treatment Options
Variable in vitro synergy has been reported for rifampin in combination with other agents for the treatment of MDR-GNB infections. Possible improvements in microbiological response by adding rifampin were observed in an RCT trial in which 210 patients with A. baumannii infections were randomized to receive either colistin or colistin plus rifampin (56). However, similar mortality was observed in the two arms (56).
Omadacycline is an aminomethylcycline recently approved by the FDA for the treatment of community-acquired bacterial pneumonia and acute skin and skin structure infections. In vitro activity against some MDR-GNB has been reported (57), although clinical post-marketing experience is needed to clarify as to whether this drug will have a role in future treatment algorithms for MDR-GNB.
Future Treatment Options for MDR-GNB in Critically-ill Patients
In this section we will discuss newly antibiotics against MDR Gram-negative pathogens in late stage of development. An example of possible future clinical reasoning for guiding the choice of antimicrobials to treat MDR-GNB infections in critically-ill patients, also including agents currently in phase 3, is shown in Figure 2.
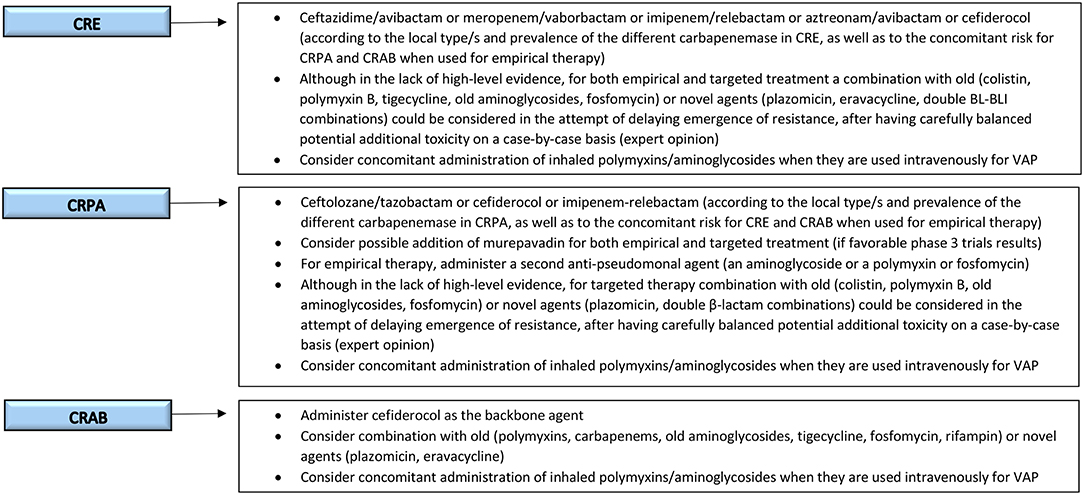
Figure 2. Possible future clinical reasoning for the treatment of serious MDR-GNB infections in critically-ill patients. MDR-GNB, Multi-drug resistant Gram-negative bacteria; CRE, carbapenem-resistant Enterobacterales; CRPA, carbapenem-resistant Pseudomonas aeruginosa; CRAB, carbapenem-resistant Acinetobacter baumannii; VAP, ventilator-associated pneumonia.
Cefiderocol
Cefiderocol is a novel siderophore antibiotic with a catechol moiety on the third position side chain which chelates free iron and provides to the new drug a unique mechanism of action (58). The catechol side chain binds to ferric iron and is actively transported across the outer membrane via the bacterial iron transporters, which are up-regulated during host immune response to acute infection (58). In addition, cefiderocol is highly active against all classes of carbapenemase (59). This combination of efficient cell entry and stability to carbapenemases allows cefiderocol to be highly active against a variety of multidrug or extremely drug resistant gram-negative bacteria, including KPC and VIM producing Enterobacteriaceae, P. aeruginosa producing MBL, Stenotrophomonas maltophilia, and A. baumannii producing OXA-type β-lactamase (59).
In a multicenter, double-blind phase 2 trial concerning treatment of cUTI, cefiderocol (2 g every 24 h) was compared with imipenem/cilastatin (1 g every 8 h) (60). Overall, 183/252 (73%) patients treated with cefiderocol and 65/119 (55%) of those treated with imipenem-cilastatin met the primary efficacy endpoint of clinical cure and microbiologic eradication at test of cure (weighted difference 18.6%, 95% CI 8.2–28.9). Moreover, cefiderocol was safe and well tolerated. Only five patients (<2%) discontinued cefiderocol because of either C. difficile, hypersensitivity, increased liver enzyme or diarrhea (60). Other two phase 3 clinical trials assessing the efficacy of cefiderocol for nosocomial pneumonia and serious infections caused by carbapenem- resistant gram negative pathogens are ongoing. The APEKS-NP (NCT03032380) is a randomized, double blind trial comparing all-cause mortality in adult patients with HAP/VAP due to gram-negative pathogens receiving either cefiderocol or meropenem (both in association with linezolid). The estimated date for completion of the study is June 2019. Another randomized, open label phase 3 trial (CREDIBLE-CR, NCT02714595) has been initiated in 2017 in order to provide the evidence of efficacy of cefiderocol in patients with serious infections (healthcare-associated pneumonia [HCAP], HAP, VAP, cUTI, and BSI) caused by carbapenem-resistant GNB. In this trial, cefiderocol is compared with best available therapy including up to 3 antibacterial agents for carbapenem-resistant GNB with either a polymyxin-based or non-polymyxin-based regimen.
Although definitive results from phase 3 trials are still not available, we believe that cefiderocol represents one of the most promising future therapeutic option for infections caused by serious carbapenem-resistant MDR-GNB, including CRE, CRPA, and CRAB.
Imipenem/Relebactam
Imipenem/relebactam is a combination of an existing carbapenem (imipenem-cilastatin) with a new potent non β-lactam, bicyclic diazabicyclooctan, β-lactamase inhibitor (relebactam). Structurally related to avibactam, relebactam inhibits the activity of class A, and C beta-lactamase, but does not have activity against metallo-β-lactamases (MBL) and class D carbapenemases (61). With this profile, relebactam primarily restores the clinical activity of imipenem against imipenem-resistant isolates such as KPC- producing Enterobacteriaceae or against P. aeruginosa isolates showing carbapenem resistance due to porin loss in combination with AmpC expression (62, 63). However, imipenem/relebactam is not active against imipenem-resistant Enterobacteriaceae producing VIM, IMP, or NDM type of MBL as well as against A. baumannii, or IMP- or VIM-producing P. aeruginosa (62).
Relebactam' s safety, tolerability, and efficacy have been originally studied in two phase 2 clinical trials including patients with cIAI or with cUTI. In those studies, the combination of imipenem (500 mg every 6 h) with relebactam at different dosage (125 mg every 6 h and 250 mg every 6 h) was non-inferior to imipenem alone. Indeed, imipenem/relebactam showed high clinical response (>96%) in both infections and for both dosages (61, 64). In addition, the two doses (125 mg every 6 h and 250 mg every 6 h) of relebactam were well tolerated and imipenem/relebactam had the same safety profile compared with imipenem-cilastatin alone.
The RESTORE-IMI1 study was a multicenter, randomized, double-blind trial designed to assess the safety and efficacy of imipenem/relebactam compared with colistin plus imipenem for treating patients with HAP/VAP, cIAI or cUTI due to imipenem-non-susceptible bacteria (but colistin and imipenem/relebactam-susceptible). A favorable overall response for each different infection type in microbiological intent-to-treat (mMITT) population was evaluated as a primary endpoint. Preliminary results from the RESTORE-IMI1 trial have been recently presented (65). In the mMITT population, the favorable overall response was similar in the imipenem/relebactam arm (n = 15; 71.4%) and the colistin plus imipenem arm (n = 7; 70%). At day 28, imipenem/relebactam was associated with higher favorable clinical response (71.4 vs. 40%) and lower all-cause mortality (9.5 vs. 30%) compared with colistin plus imipenem. Moreover, in a safety analysis, fewer patients who received imipenem/relebactam had a drug-related adverse event (16.1 vs. 31.3%, p = 0.001), including treatment-emergent nephrotoxicity (10 vs. 56%, p = 0.001). A second pivotal phase 3 trial (RESTORE-IMI2) comparing the efficacy of imipenem/relebactam with piperacillin/tazobactam for the treatment of HAP and VAP is currently ongoing (NCT02493764).
Murepavadin
Murepavadin (POL7080) is a protein epitope mimetic that belongs to a new class of antibiotics called outer membrane protein targeting antibiotics (OMPTA). It possesses a novel, non-lytic mechanism of action that targets the lipopolysaccharide transport protein D (LptD), involved in lipopolysaccharide biogenesis of the outer membrane of P. aeruginosa (66). Therefore, murepavadin is highly active against P. aeruginosa (66) with an expected limited impact on normal gastrointestinal flora or resistance selection in other bacterial pathogens. In vitro, it has demonstrated potent antimicrobial activity against P. aeruginosa, including carbapenemase-producing, colistin-resistant, extremely drug-resistant and pan drug-resistant isolates (67, 68) and other tested Pseudomonas species, but is not active against other non-fermenting Gram-negative pathogens or Enterobacteriaceae (69). The drug has a high volume of tissue distribution with linear and dose-proportional pharmacokinetics, and a half-life of 2–5 h (69).
Clinically, murepavadin has been studied in patients with VAP due to suspected or documented P. aeruginosa. In an open-label phase 2 study, murepavadin plus standard of care was administered in 25 patients with suspected of confirmed P. aeruginosa VAP (NCT02096328). Among 12 patients with confirmed P. aeruginosa VAP (nine of which the infection was caused by a MDR or extremely drug-resistant isolate), clinical cure was achieved in 91% of the patients at test of cure and 28-day all-cause mortality was of 8%, far below the 20–40% expected mortality rate (70). No development of treatment-emergent resistance to murepavadin was observed during the study. The PRISM-UDR trial assessing the efficacy of murepavadin with ertapenem in the treatment of nosocomial pneumonia (NCT03582007) and the PRIMS-MDR trial comparing murepavadin plus one anti-pseudomonas antibiotic with two anti-pseudomonas antibiotics for VAP are ongoing (NCT03409679).
In conclusion, if the promising results observed in phase 2 trials will be confirmed in phase 3 studies, murepavadin could represent an important option in combination with other antibiotics for empirical treatment in patients with strong risk factors for P. aeruginosa infections, as well as for targeted treatment. Efficacy data for other type of infection, including bloodstream infections, cUTI or cIAI, are clearly needed.
Aztreonam/Avibactam
Aztreonam is the only available monobactam antibiotic that has been approved for treatment of gram infection since 1986. It is active against MBL-producing bacteria, but it is hydrolyzed by Ambler class A beta-lactamases (e.g., ESBL and KPCs) and class C (e.g., AmpC) beta-lactamases. Therefore, the combination of aztreonam with avibactam is able to inhibit cell wall synthesis in MBL-producing strains despite the presence of other co-carried beta-lactamases or carbapenemases (59). Aztreonam/avibactam showed a potent in-vitro activity against ESBL, class C b-lactamase, MBL, and KPC-producing strains with an activity 10 times that of aztreonam alone. Despite this, limited activity has been shown against A. baumannii or P. aeruginosa compared with aztreonam alone (59).
A phase III clinical trial to compare aztreonam/avibactam (with or without metronidazole) with meropenem (with or without colistin) for the treatment of HAP, VAP, and cIAI due to Gram-negative bacteria for which there are limited, or no treatment options is ongoing (REVISIT study, NCT03329092). Another phase 3 study to determine the efficacy, safety, and tolerability of aztreonam/avibactam vs. best available therapy in the treatment of serious infections (cIAI, NP, HAP, VAP, cUTI, or BSI) due to MBL–producing Gram-negative bacteria will be performed starting in April 2020 (NCT03580044).
Aztreonam/avibactam may represent an interesting alternative for infections caused by MBL-producing strains.
Agents in Earlier Stages of Development
Agents in earlier stages of development are not the focus of the present review and have been comprehensively summarized elsewhere (5, 71). Nonetheless, it should be reminded that they could represent another precious addition to the clinicians' armamentarium in the future. For example, cefepime/zidebactam has shown promising activity against CRE, CRPA, and CRAB, and both cefepime/zidebactam and meropenem/nacubactam have demonstrated in vitro activity against MBL-producing CRE (5, 72–76). Ceftaroline/avibactam, cefepime/AAI101, TP-6076, VNXR-5133, WCK-5153, MEDI3902, COT-143, and RX-P2382 are other promising agents to be further evaluated for their activity against MDR-GNB during clinical development (5, 77, 78).
Conclusions
The treatment of MDR-GNB infections in critically ill patients presents many challenges: (i) an effective treatment should be administered as soon as possible, but resistance to many antimicrobial classes invariably reduces the probability of adequate empirical coverage, with possible unfavorable consequences; (ii) there is an increasing need for rapid diagnostics and efficient laboratory workflows to anticipating diagnosis rapidly narrowing the antimicrobial spectrum, in line with antimicrobial stewardship principles; (ii) infection-control initiatives are also of paramount important, since CRE, CRPA, and CRAB are being reported with increasing frequencies worldwide, although with important variability across regions and even hospitals.
Novel treatment options have become available in recent years, providing some much-awaited resources for effectively counteracting some severe MDR-GNB infections. However, their optimal use should be guaranteed in the long term, for delaying as much as possible the emergence and diffusion of resistance to novel agents. Despite important progresses, PK/PD optimization of dosages and treatment duration in critically ill patients has still some areas of uncertainty requiring further study, that should take into account also resistance selection as a major endpoint. Treatment of severe MDR-GNB infections in critically ill patients in the near future will require an expert and complex clinical reasoning, of course taking into account the peculiar characteristics of the target population, but also the need for adequate empirical coverage and the more and more specific enzyme-level activity of novel antimicrobials with respect to the different resistance mechanisms of MDR-GNB.
Author Contributions
All authors contributed equally to the conception and overview of the manuscript content, and also contributed equally to the writing of the manuscript. In addition, MB provided critical revision for intellectual content, and oversight. All the authors approved the final version of the manuscript.
Conflict of Interest Statement
Outside the submitted work, MB has received funding for scientific advisory boards, travel and speaker honoraria from Angelini, AstraZeneca, Bayer, Cidara, Cubist, Pfizer, Menarini, MSD, Nabriva, Paratek, Roche, Shionogi, Tetraphase, The Medicine Company and Astellas Pharma Inc. Outside the submitted work, DRG reports honoraria from Stepstone Pharma GmbH and an unconditioned grant from MSD Italia.
The remaining authors declare that the research was conducted in the absence of any commercial or financial relationships that could be construed as a potential conflict of interest.
Abbreviations
ICU, intensive care units; MDR, multidrug-resistant; GNB, Gram-negative bacteria; CRE, carbapenem-resistant Enterobacterales, CRPA, carbapenem-resistant Pseudomonas aeruginosa; CRAB, carbapenem-resistant Acinetobacter baumannii; β-lactam/β-lactamase inhibitors (BL-BLI); FDA, Food and Drug Administration; cUTI, complicated urinary tract infections; BSI, bloodstream infections; EMA, European Medicines Agency; RCT, randomized controlled trial; VAP, ventilator-associated pneumonia; cIAI, complicated intraabdominal infections; HAP, hospital-acquired pneumonia; ESBL, extended-spectrum β-lactamases; HCAP, healthcare-associated pneumonia; metallo-β-lactamases (MBL); mMITT, microbiological intent-to-treat; OMPTA, outer membrane protein targeting antibiotics; LptD, lipopolysaccharide transport protein D; NP, nosocomial pneumonia.
References
1. Bassetti M, Welte T, Wunderink RG. Treatment of Gram-negative pneumonia in the critical care setting: is the beta-lactam antibiotic backbone broken beyond repair? Crit Care. (2016) 20:19. doi: 10.1186/s13054-016-1197-5
2. Munoz-Price LS, Poirel L, Bonomo RA, Schwaber MJ, Daikos GL, Cormican M, et al. Clinical epidemiology of the global expansion of Klebsiella pneumoniae carbapenemases. Lancet Infect Dis. (2013) 13:785–96. doi: 10.1016/S1473-3099(13)70190-7
3. Giacobbe DR, di Masi A, Leboffe L, Del Bono V, Rossi M, Cappiello D, et al. Hypoalbuminemia as a predictor of acute kidney injury during colistin treatment. Sci Rep. (2018) 8:11968. doi: 10.1038/s41598-018-30361-5
4. Garonzik SM, Li J, Thamlikitkul V, Paterson DL, Shoham S, Jacob J, et al. Population pharmacokinetics of colistin methanesulfonate and formed colistin in critically ill patients from a multicenter study provide dosing suggestions for various categories of patients. Antimicrob Agents Chemother. (2011) 55:3284–94. doi: 10.1128/AAC.01733-10
5. Giacobbe DR, Mikulska M, Viscoli C. Recent advances in the pharmacological management of infections due to multidrug resistant gram-negative bacteria. Expert Rev Clin Pharmacol. (2018) 11:1219–36. doi: 10.1080/17512433.2018.1549487
6. Pogue JM, Bonomo RA, Kaye KS. Ceftazidime/avibactam, Meropenem/vaborbactam or both? Clinical and formulary considerations. Clin Infect Dis. (2018) 68:519–24. doi: 10.1093/cid/ciy576
7. Bassetti M, Righi E, Vena A, Graziano E, Russo A, Peghin M. Risk stratification and treatment of ICU-acquired pneumonia caused by multidrug- resistant/extensively drug-resistant/pandrug-resistant bacteria. Curr Opin Crit Care. (2018) 24:385–93. doi: 10.1097/MCC.0000000000000534
8. Shields RK, Chen L, Cheng S, Chavda KD, Press EG, Snyder A, et al. Emergence of ceftazidime-avibactam resistance due to plasmid-borne blaKPC-3 Mutations during Treatment of Carbapenem-Resistant Klebsiella pneumoniae Infections. Antimicrob Agents Chemother. (2017) 61: e02097–16. doi: 10.1128/AAC.02097-16
9. Sun D, Rubio-Aparicio D, Nelson K, Dudley MN, Lomovskaya O. Meropenem-vaborbactam resistance selection, resistance prevention, and molecular mechanisms in mutants of KPC-producing klebsiella pneumoniae. Antimicrob Agents Chemother. (2017) 61:e01694–17. doi: 10.1128/AAC.01694-17
10. Daikos GL, Tsaousi S, Tzouvelekis LS, Anyfantis I, Psichogiou M, Argyropoulou A, et al. Carbapenemase-producing Klebsiella pneumoniae bloodstream infections: lowering mortality by antibiotic combination schemes and the role of carbapenems. Antimicrob Agents Chemother. (2014) 58:2322–8. doi: 10.1128/AAC.02166-13
11. Tumbarello M, Trecarichi EM, De Rosa FG, Giannella M, Giacobbe DR, Bassetti M, et al. Infections caused by KPC-producing Klebsiella pneumoniae: differences in therapy and mortality in a multicentre study. J Antimicrob Chemother. (2015) 70:2133–43. doi: 10.1093/jac/dkv086
12. Tsuji BT, Pogue JM, Zavascki AP, Paul M, Daikos GL, Forrest A, et al. International consensus guidelines for the optimal use of the polymyxins: endorsed by the American College of Clinical Pharmacy (ACCP), European Society of Clinical Microbiology and Infectious Diseases (ESCMID), Infectious Diseases Society of America (IDSA), International Society for Anti-infective Pharmacology (ISAP), Society of Critical Care Medicine (SCCM), and Society of Infectious Diseases Pharmacists (SIDP). Pharmacotherapy. (2019) 39:10–39. doi: 10.1002/phar.2209
13. Giacobbe DR, Del Bono V, Trecarichi EM, De Rosa FG, Giannella M, Bassetti M, et al. Risk factors for bloodstream infections due to colistin-resistant KPC-producing Klebsiella pneumoniae: results from a multicenter case-control-control study. Clin Microbiol Infect. (2015) 21:1106.e1–8. doi: 10.1016/j.cmi.2015.08.001
14. Giamarellou H. Epidemiology of infections caused by polymyxin-resistant pathogens. Int J Antimicrob Agents. (2016) 48:614–21. doi: 10.1016/j.ijantimicag.2016.09.025
15. Gonzalez-Padilla M, Torre-Cisneros J, Rivera-Espinar F, Pontes-Moreno A, Lopez-Cerero L, Pascual A, et al. Gentamicin therapy for sepsis due to carbapenem-resistant and colistin-resistant Klebsiella pneumoniae. J Antimicrob Chemother. (2015) 70:905–13. doi: 10.1093/jac/dku432
16. Bassetti M, Giacobbe DR, Giamarellou H, Viscoli C, Daikos GL, Dimopoulos G, et al. Management of KPC-producing Klebsiella pneumoniae infections. Clin Microbiol Infect. (2018) 24:133–44. doi: 10.1016/j.cmi.2017.08.030
17. Panidis D, Markantonis SL, Boutzouka E, Karatzas S, Baltopoulos G. Penetration of gentamicin into the alveolar lining fluid of critically ill patients with ventilator-associated pneumonia. Chest. (2005) 128:545–52. doi: 10.1378/chest.128.2.545
18. Kadri SS, Adjemian J, Lai YL, Spaulding AB, Ricotta E, Prevots DR, et al. National institutes of health antimicrobial resistance outcomes research, difficult-to-treat resistance in gram-negative bacteremia at 173 US hospitals: retrospective cohort analysis of prevalence, predictors, and outcome of resistance to all first-line agents. Clin Infect Dis. (2018) 67:1803–14. doi: 10.1093/cid/ciy378
19. Zhanel GG, Lawson CD, Zelenitsky S, Findlay B, Schweizer F, Adam H, et al. Comparison of the next-generation aminoglycoside plazomicin to gentamicin, tobramycin and amikacin. Expert Rev Anti Infect Ther. (2012) 10:459–73. doi: 10.1586/eri.12.25
20. Livermore DM, Mushtaq S, Warner M, Zhang JC, Maharjan S, Doumith M, et al. Activity of aminoglycosides, including ACHN-490, against carbapenem-resistant Enterobacteriaceae isolates. J Antimicrob Chemother. (2011) 66:48–53. doi: 10.1093/jac/dkq408
21. Cloutier DJ, Miller LG, Komirenko AS, et al. Plazomicin Versus Meropenem for the Treatment of Complicated Urinary Tract Infection and Acute Pyelonephritis: Results of the EPIC Study. (Vienna) (2017). doi: 10.1056/NEJMoa1801467
22. Wagenlehner FME, Cloutier DJ, Komirenko AS, Cebrik DS, Krause KM, Keepers TR, et al. Once-daily plazomicin for complicated urinary tract infections. N Engl J Med. (2019) 380:729–40. doi: 10.1056/NEJMoa1801467
24. Rao GG, Ly NS, Diep J, Forrest A, Bulitta JB, Holden PN, et al Combinatorial pharmacodynamics of polymyxin B and tigecycline against heteroresistant Acinetobacter baumannii. Int J Antimicrob Agents. (2016) 48:331–6. doi: 10.1016/j.ijantimicag.2016.06.006
25. Tumbarello M, Viale P, Viscoli C, Trecarichi EM, Tumietto F, Marchese A, et al. Predictors of mortality in bloodstream infections caused by Klebsiella pneumoniae carbapenemase-producing K. pneumoniae: importance of combination therapy. Clin Infect Dis. (2012) 55:943–50. doi: 10.1093/cid/cis588
26. FDA Drug Safety Communication. FDA Warns of Increased Risk of Death With IV Antibacterial Tygacil (tigecycline) and Approves New Boxed Warning. Available online at: https://www.fda.gov/Drugs/DrugSafety/default.htm
27. Wiskirchen DE, Koomanachai P, Nicasio AM, Nicolau DP, Kuti JL. In vitro pharmacodynamics of simulated pulmonary exposures of tigecycline alone and in combination against Klebsiella pneumoniae isolates producing a KPC carbapenemase. Antimicrob Agents Chemother. (2011) 55:1420–7. doi: 10.1128/AAC.01253-10
28. Cancelli F, Oliva A, De Angelis M, Mascellino MT, Mastroianni CM, Vullo V. Role of double-carbapenem regimen in the treatment of infections due to carbapenemase producing carbapenem-resistant enterobacteriaceae: a single-center, observational study. Biomed Res Int. (2018) 2018:2785696. doi: 10.1155/2018/2785696
29. Del Bono V, Giacobbe DR, Marchese A, Parisini A, Fucile C, Coppo E, et al. Meropenem for treating KPC-producing Klebsiella pneumoniae bloodstream infections: Should we get to the PK/PD root of the paradox? Virulence. (2017) 8:66–73. doi: 10.1080/21505594.2016.1213476
30. Pea F, Della Siega P, Cojutti P, Sartor A, Crapis M, Scarparo C, et al. Might real-time pharmacokinetic/pharmacodynamic optimisation of high-dose continuous-infusion meropenem improve clinical cure in infections caused by KPC-producing Klebsiella pneumoniae? Int J Antimicrob Agents. (2017) 49:255–8. doi: 10.1016/j.ijantimicag.2016.10.018
31. Zusman O, Avni T, Leibovici L, Adler A, Friberg L, Stergiopoulou T, et al. Systematic review and meta-analysis of in vitro synergy of polymyxins and carbapenems. Antimicrob Agents Chemother. (2013) 57:5104–11. doi: 10.1128/AAC.01230-13
32. Paul M, Daikos GL, Durante-Mangoni E, Yahav D, Carmeli Y, Benattar YD, et al. Colistin alone versus colistin plus meropenem for treatment of severe infections caused by carbapenem-resistant Gram-negative bacteria: an open-label, randomised controlled trial. Lancet Infect Dis. (2018) 18:391–400. doi: 10.1016/S1473-3099(18)30099-9
33. Grabein B, Graninger W, Rodriguez Bano J, Dinh A, Liesenfeld DB. Intravenous fosfomycin-back to the future. Systematic review and meta-analysis of the clinical literature. Clin Microbiol Infect. (2017) 23:363–72. doi: 10.1016/j.cmi.2016.12.005
34. Sirijatuphat R, Thamlikitkul V. Preliminary study of colistin versus colistin plus fosfomycin for treatment of carbapenem-resistant Acinetobacter baumannii infections. Antimicrob Agents Chemother. (2014) 58:5598–601. doi: 10.1128/AAC.02435-13
35. Pontikis K, Karaiskos I, Bastani S, Dimopoulos G, Kalogirou M, Katsiari M, et al. Outcomes of critically ill intensive care unit patients treated with fosfomycin for infections due to pandrug-resistant and extensively drug-resistant carbapenemase-producing Gram-negative bacteria. Int J Antimicrob Agents. (2014) 43:52–9. doi: 10.1016/j.ijantimicag.2013.09.010
36. Montravers P, Bassetti M. The ideal patient profile for new beta-lactam/beta-lactamase inhibitors. Curr Opin Infect Dis. (2018) 31:587–93. doi: 10.1097/QCO.0000000000000490
37. Carmeli Y, Armstrong J, Laud PJ, Newell P, Stone G, Wardman A, et al. Ceftazidime-avibactam or best available therapy in patients with ceftazidime-resistant Enterobacteriaceae and Pseudomonas aeruginosa complicated urinary tract infections or complicated intra-abdominal infections (REPRISE): a randomised, pathogen-directed, phase 3 study. Lancet Infect Dis. (2016) 16:661–73. doi: 10.1016/S1473-3099(16)30004-4
38. Shields RK, Nguyen MH, Chen L, Press EG, Potoski BA, Marini RV, et al. Ceftazidime-avibactam is superior to other treatment regimens against carbapenem-resistant klebsiella pneumoniae bacteremia. Antimicrob Agents Chemother. (2017) 61:e00883–17. doi: 10.1128/AAC.00883-17
39. Tumbarello M, Trecarichi EM, Corona A, De Rosa FG, Bassetti M, Mussini C, et al. Efficacy of ceftazidime-avibactam salvage therapy in patients with infections caused by KPC-producing klebsiella pneumoniae. Clin Infect Dis. (2019) 68:355–64. doi: 10.1093/cid/ciy492
40. Gaibani P, Campoli C, Lewis RE, Volpe SL, Scaltriti E, Giannella M, et al. In vivo evolution of resistant subpopulations of KPC-producing Klebsiella pneumoniae during ceftazidime/avibactam treatment. J Antimicrob Chemother. (2018) 73:1525–9. doi: 10.1093/jac/dky082
41. Kaye KS, Bhowmick T, Metallidis S, Bleasdale SC, Sagan OS, Stus V, et al. Effect of meropenem-vaborbactam vs piperacillin-tazobactam on clinical cure or improvement and microbial eradication in complicated urinary tract infection: the TANGO I randomized clinical trial. JAMA. (2018) 319:788–99. doi: 10.1001/jama.2018.0438
42. Wunderink RG, Giamarellos-Bourboulis EJ, Rahav G, Mathers AJ, Bassetti M, Vazquez J, et al. Effect and safety of meropenem-vaborbactam versus best-available therapy in patients with carbapenem-resistant enterobacteriaceae infections: the TANGO Ii randomized clinical trial. Infect Dis Ther. (2018) 7:439–455. doi: 10.1007/s40121-018-0214-1
43. Giacobbe DR, Bassetti M, De Rosa FG, Del Bono V, Grossi PA, Menichetti F, et al. Ceftolozane/tazobactam: place in therapy. Expert Rev Anti Infect Ther. (2018) 16:1–14. doi: 10.1080/14787210.2018.1447381
44. Solomkin J, Hershberger E, Miller B, Popejoy M, Friedland I, Steenbergen J, et al. Ceftolozane/tazobactam plus metronidazole for complicated intra-abdominal infections in an era of multidrug resistance: results from a randomized, double-blind, phase 3 trial. (ASPECT-cIAI). Clin Infect Dis. (2015) 60:1462–71. doi: 10.1093/cid/civ097
45. Wagenlehner FM, Umeh O, Steenbergen J, Yuan G, Darouiche RO. Ceftolozane-tazobactam compared with levofloxacin in the treatment of complicated urinary-tract infections, including pyelonephritis: a randomised, double-blind, phase 3 trial (ASPECT-cUTI). Lancet. (2015) 385:1949–56. doi: 10.1016/S0140-6736(14)62220-0
46. Bassetti M, Castaldo N, Cattelan A, Mussini C, Righi E, Tascini C, et al. Ceftolozane/tazobactam for the treatment of serious P. aeruginosa infections: a multicenter nationwide clinical experience. Int J Antimicrob Agents. (2018) 5:ofy280. doi: 10.1016/j.ijantimicag.2018.11.001
47. Munita JM, Aitken SL, Miller WR, Perez F, Rosa R, Shimose LA, et al. Multicenter evaluation of ceftolozane/tazobactam for serious infections caused by carbapenem-resistant pseudomonas aeruginosa. Clin Infect Dis. (2017) 65:158–61. doi: 10.1093/cid/cix014
48. Merck's ZERBAXA® (ceftolozane and tazobactam). Met Primary Endpoints of Non-Inferiority Compared to Meropenem in Pivotal Phase 3 Study of Adult Patients with Hospital-Acquired Bacterial Pneumonia or Ventilator-Associated Bacterial Pneumonia. Available onlinev at: https://investors.merck.com/news/press-release-details/2018/Mercks-ZERBAXA-ceftolozane-and-tazobactam-Met-Primary-Endpoints-of-Non-Inferiority-Compared-to-Meropenem-in-Pivotal-Phase-3-Study-of-Adult-Patients-with-Hospital-Acquired-Bacterial-Pneumonia-or-Ventilator-Associated-Bacterial-Pneumonia/default.aspx
49. Sutcliffe JA, O'Brien W, Fyfe C, Grossman TH. Antibacterial activity of eravacycline (TP-434), a novel fluorocycline, against hospital and community pathogens. Antimicrob Agents Chemother. (2013) 57:5548–58. doi: 10.1128/AAC.01288-13
50. Bassetti M, Righi E, Carnelutti A. New therapeutic options for respiratory tract infections. Curr Opin Infect Dis. (2016) 29:178–86. doi: 10.1097/QCO.0000000000000251
51. Seifert H, Stefanik D, Sutcliffe JA, Higgins PG. In-vitro activity of the novel fluorocycline eravacycline against carbapenem non-susceptible Acinetobacter baumannii. Int J Antimicrob Agents. (2018) 51:62–4. doi: 10.1016/j.ijantimicag.2017.06.022
52. Solomkin J, Evans D, Slepavicius A, Lee P, Marsh A, Tsai L, et al. Assessing the efficacy and safety of eravacycline vs ertapenem in complicated intra-abdominal infections in the Investigating Gram-Negative Infections Treated With Eravacycline (IGNITE 1) trial: a randomized clinical trial. JAMA Surg. (2017) 152:224–32. doi: 10.1001/jamasurg.2016.4237
53. IGNITE2. Eravacycline Inferior to Levofloxacin, but IV Formulation Shows Promise Healio. Available online at: https://www.healio.com
54. Tetraphase Announces Top-Line Results From IGNITE3 Phase 3 Clinical Trial of Eravacycline in Complicated Urinary Tract Infections (cUTI). Tetraphase. Available online at: https://ir.tphase.com/news-releases/news-release-details/tetraphase-announces-top-line-results-ignite3-phase-3-clinical
55. Solomkin JS, Gardovskis J, Lawrence K, Montravers P, Sway A, Evans D, et al. IGNITE4: results of a phase 3, randomized, multicenter, prospective trial of eravacycline vs. meropenem in the treatment of complicated intra-abdominal infections. Clin Infect Dis. (2018). doi: 10.1093/cid/ciy1029
56. Durante-Mangoni E, Signoriello G, Andini R, Mattei A, De Cristoforo M, Murino P, et al. Colistin and rifampicin compared with colistin alone for the treatment of serious infections due to extensively drug-resistant Acinetobacter baumannii: a multicenter, randomized clinical trial. Clin Infect Dis. (2013) 57:349–58. doi: 10.1093/cid/cit253
57. Pfaller MA, Huband MD, Rhomberg PR, Flamm RK. Surveillance of omadacycline activity against clinical isolates from a global collection (North America, Europe, Latin America, Asia-Western Pacific), 2010-2011. Antimicrob Agents Chemother. (2017) 61:e00018–17. doi: 10.1128/AAC.00018-17
58. Ito A, Nishikawa T, Matsumoto S, Yoshizawa H, Sato T, Nakamura R, et al. Siderophore cephalosporin cefiderocol utilizes ferric iron transporter systems for antibacterial activity against pseudomonas aeruginosa. Antimicrob Agents Chemother. (2016) 60:7396–401. doi: 10.1128/AAC.01405-16
59. Wright H, Bonomo RA, Paterson DL. New agents for the treatment of infections with Gram-negative bacteria: restoring the miracle or false dawn? Clin Microbiol Infect. (2017) 23:704–12. doi: 10.1016/j.cmi.2017.09.001
60. Portsmouth S, van Veenhuyzen D, Echols R, Machida M, Ferreira JCA, Ariyasu M, et al. Cefiderocol versus imipenem-cilastatin for the treatment of complicated urinary tract infections caused by Gram-negative uropathogens: a phase 2, randomised, double-blind, non-inferiority trial. Lancet Infect Dis. (2018) 18:1319–28. doi: 10.1016/S1473-3099(18)30554-1
61. Lucasti C, Vasile L, Sandesc D, Venskutonis D, McLeroth P, Lala M, et al. Phase 2, dose-ranging study of relebactam with imipenem-cilastatin in subjects with complicated intra-abdominal infection. Antimicrob Agents Chemother. (2016) 60:6234–43. doi: 10.1128/AAC.00633-16
62. Livermore DM, Warner M, Mushtaq S. Activity of MK-7655 combined with imipenem against Enterobacteriaceae and Pseudomonas aeruginosa. J Antimicrob Chemother. (2013) 68:2286–90. doi: 10.1093/jac/dkt178
63. Olsen I. New promising beta-lactamase inhibitors for clinical use. Eur J Clin Microbiol Infect Dis. (2015) 34:1303–8. doi: 10.1007/s10096-015-2375-0
64. Sims M, Mariyanovski V, McLeroth P, Akers W, Lee YC, Brown ML, et al. Prospective, randomized, double-blind, Phase 2 dose-ranging study comparing efficacy and safety of imipenem/cilastatin plus relebactam with imipenem/cilastatin alone in patients with complicated urinary tract infections. J Antimicrob Chemother. (2017) 72:2616–26. doi: 10.1093/jac/dkx139
65. Motsch J, de Oliveira C, Stus V, Koksal I, Lyulko O, Boucher H, et al. RESTORE-IMI 1: a multicenter, randomized, double-blind, comparator-controlled trial comparing the efficacy and safety of imipenem/relebactam versus colistin plus imipenem in patients with imipenem-non-susceptible bacterial infections. In: Presented in 28th European Congress of Clinical Microbiology and Infectious Diseases (ECCMID). Madrid (2018).
66. Srinivas N, Jetter P, Ueberbacher BJ, Werneburg M, Zerbe K, Steinmann JB, et al. Peptidomimetic antibiotics target outer-membrane biogenesis in Pseudomonas aeruginosa. Science. (2010) 327:1010–3. doi: 10.1126/science.1182749
67. Sader HS, Dale GE, Rhomberg PR, Flamm RK. Antimicrobial activity of murepavadin tested against clinical isolates of pseudomonas aeruginosa from the United States, Europe, and China. Antimicrob Agents Chemother. (2018) 62:e00311–18. doi: 10.1128/AAC.00311-18
68. Sader HS, Flamm RK, Dale GE, Rhomberg PR, Castanheira M. Murepavadin activity tested against contemporary. (2016-17) clinical isolates of XDR Pseudomonas aeruginosa. J Antimicrob Chemother. (2018) 73:2400–04. doi: 10.1093/jac/dky227
69. Martin-Loeches I, Dale GE, Torres A. Murepavadin: a new antibiotic class in the pipeline. Expert Rev Anti Infect Ther. (2018) 16:259–268. doi: 10.1080/14787210.2018.1441024
71. Bassetti M, Righi E, Russo A, Carnelutti A. New antibiotics for pneumonia. Clin Chest Med. (2018) 39:853–69. doi: 10.1016/j.ccm.2018.08.007
72. Moya B, Barcelo IM, Bhagwat S, Patel M, Bou G, Papp-Wallace KM, et al. Potent beta-Lactam Enhancer Activity of Zidebactam and WCK 5153 against acinetobacter baumannii, including carbapenemase-producing clinical isolates. Antimicrob Agents Chemother. (2017) 61:e01238–17. doi: 10.1128/AAC.01238-17
73. Moya B, Barcelo IM, Bhagwat S, Patel M, Bou G, Papp-Wallace KM, et al. WCK 5107 (Zidebactam) and WCK 5153 are novel inhibitors of PBP2 showing potent “beta-Lactam Enhancer” activity against pseudomonas aeruginosa, Including Multidrug-Resistant Metallo-beta-Lactamase-Producing High-Risk Clones. Antimicrob Agents Chemother. (2017) 61:e02529–16. doi: 10.1128/AAC.02529-16
74. Sader HS, Castanheira M, Huband M, Jones RN, Flamm RK. WCK 5222 (Cefepime-Zidebactam) antimicrobial activity against clinical isolates of gram-negative bacteria collected Worldwide in 2015. Antimicrob Agents Chemother. (2017) 61:e072–17. doi: 10.1128/AAC.00072-17
75. Sader HS, Rhomberg PR, Flamm RK, Jones RN, Castanheira M. WCK 5222 (cefepime/zidebactam) antimicrobial activity tested againstGram-negative organisms producing clinically relevant beta-lactamases. J Antimicrob Chemother. (2017) 72:1696–703. doi: 10.1093/jac/dkx050
76. Mushtaq S, Vickers A, Woodford N, Haldimann A, Livermore DM. Activity of nacubactam (RG6080/OP0595) combinations against MBL-producing Enterobacteriaceae. J Antimicrob Chemother. (2018) 74:953–60. doi: 10.1093/jac/dky522
77. Castanheira M, Williams G, Jones RN, Sader HS. Activity of ceftaroline-avibactam tested against contemporary Enterobacteriaceae isolates carrying beta-lactamases prevalent in the United States. Microb Drug Resist. (2014) 20:436–40. doi: 10.1089/mdr.2013.0181
Keywords: gram-negative, ICU, MDR, antimicrobial resistance, Pseudomonas, Acinetobacter, Klebsiella
Citation: Bassetti M, Peghin M, Vena A and Giacobbe DR (2019) Treatment of Infections Due to MDR Gram-Negative Bacteria. Front. Med. 6:74. doi: 10.3389/fmed.2019.00074
Received: 04 February 2019; Accepted: 27 March 2019;
Published: 16 April 2019.
Edited by:
George Dimopoulos, University General Hospital Attikon, GreeceReviewed by:
Despoina Koulenti, University General Hospital Attikon, GreeceValter Viana Andrade-Neto, Fundação Oswaldo Cruz (Fiocruz), Brazil
Copyright © 2019 Bassetti, Peghin, Vena and Giacobbe. This is an open-access article distributed under the terms of the Creative Commons Attribution License (CC BY). The use, distribution or reproduction in other forums is permitted, provided the original author(s) and the copyright owner(s) are credited and that the original publication in this journal is cited, in accordance with accepted academic practice. No use, distribution or reproduction is permitted which does not comply with these terms.
*Correspondence: Matteo Bassetti, matteo.bassetti@asuiud.sanita.fvg.it