A Review of SARS-CoV2: Compared With SARS-CoV and MERS-CoV
- 1National Drug Clinical Trial Center, The First Affiliated Hospital of Bengbu Medical College, Bengbu, China
- 2School of Pharmacy, Bengbu Medical College, Bengbu, China
- 3School of Public Foundation, Bengbu Medical University, Bengbu, China
- 4Key Laboratory of Anti-inflammatory and Immune Medicine, Ministry of Education, Institute of Clinical Pharmacology, Anhui Medical University, Hefei, China
- 5Basic Medical Sciences, Anhui Medical University, Hefei, China
- 6Department of Oncology, The First Affiliated Hospital of Anhui Medical University, Hefei, China
- 7Department of Pharmacology, The Affiliated Hospital of Hangzhou Normal University, Hangzhou, China
The outbreak of coronavirus disease 2019 (COVID-19) has been spreading rapidly in China and the Chinese government took a series of policies to control the epidemic. Studies found that severe COVID-19 is characterized by pneumonia, lymphopenia, exhausted lymphocytes and a cytokine storm. Studies have showen that SARS-CoV2 has significant genomic similarity to the severe acute respiratory syndrome (SARS-CoV), which was a pandemic in 2002. More importantly, some diligent measures were used to limit its spread according to the evidence of hospital spread. Therefore, the Public Health Emergency of International Concern (PHEIC) has been established by the World Health Organization (WHO) with strategic objectives for public health to curtail its impact on global health and economy. The purpose of this paper is to review the transmission patterns of the three pneumonia: SARS-CoV2, SARS-CoV, and MERS-CoV. We compare the new characteristics of COVID-19 with those of SARS-CoV and MERS-CoV.
Introduction
Coronaviruses (CoVs) are enveloped RNA viruses that respond primarily to respiratory and intestinal infections in animals and humans (1, 2). Historically, CoV infection in humans was closely linked to the human CoVs (HCoVs) HCoV-229E and HCoV-OC43-induced mild upper respiratory tract diseases (2). Nevertheless, a new fatal CoV was discovered in 2003, leading to SARS-CoV, and thus redefining historical cognition (3). More importantly, researchers have identified that respiratory diseases were significantly associated with HcoVs. For example, studies demonstrated that HCoV-HKU1 induced chronic pulmonary disease (4). HCoV-NL63 could mediate upper and lower respiratory tract diseases in children and adults around the world (5), and the recently (April 2012) Middle East Respiratory Syndrome (MERS-CoV) has been shown to be involved in acute pneumonia and sporadic renal failure (6). These findings proved that CoVs were important human pathogenic factors, and emphasize the necessity of reverse genetic systems to facilitate the genetic manipulation of the viral genome, thereby studying basic viral processes, developing candidate vaccines, and testing antiviral drugs.
In the past two decades, the world experienced outbreaks of coronavirus infection that threaten the global pandemic in 2002–2003 by SARS-CoV and in 2011 by MERS-CoV. In both cases, the causative agents (SARS-CoV and MERS-CoV, respectively), were newly identified coronavirus in the genus Beta-coronavirus with zoonotic origin the SARS-CoV (2002) and the MERS (2012) (7, 8). Moreover, studies demonstrated that SARS-CoV and MERS-CoV could infect humans, camesl, bats, cows, and civets, and thus infect the acute and fatal respiratory of humans (7, 9).
The recent coronavirus outbreak happened in the Wuhan city of China, which is known as the 2019-nCoV outbreak, recently renamed as SARS-CoV-2 outbreak or COVID-19 (10). The SARS-CoV-2 spread throughout the world is a zoonotic virus and is the seventh coronavirus that infects the human (11). The first case of SARS-CoV-2 infection was reported in Wuhan, China, on 31st December 2019 with the presentation of symptoms of atypical pneumonia. This case was further confirmed to be caused by the novel coronavirus, SARS-CoV-2 (12). According to the WHO, as of 7:54 P.M. CEST, 21 May 2021, 165,158,285 cases of COVID-19 have been reported with associated 3,425,017 deaths worldwide. There were 105,647 confirmed cases of SARS-CoV-2 infections in mainland China, including 4,861 deaths. The biggest potential risk for the spread of COVID-19 around the world was closely associated with travel that caused the disease to spread regionally and globally (13). More importantly, researchers found that SARS and COVID-19 share many similarities in terms of their transmission and pathogenicity. Therefore, COVID-19 firstly transmitted from animal to human at the wet store of the sale of seafood and can transmit from humans to humans (14). At this moment, WHO is investigating if Wuhan was the first place of the first infected human. Coronaviruses, like influenza viruses, spread to various animals in nature. Mammals was infected by α-coronaviruses and β-coronaviruses, and gamma-coronaviruses and delta-coronaviruses tend to infect birds. Moreover, the mammals may also be infected with gamma-coronaviruses and delta-coronaviruses. Although still preliminary, current data suggest that bats are the most likely initial source of the COVID-19 outbreak, which begun in December 2019 in Wuhan, China, apparently spreading from a “wet market” to multiple cities and provinces in China (15).
SARS-CoV-2 is a single-stranded sense RNA virus with a genome size of 27–32 KB (125 nm or 0.125 μm) It has a 5′- cap structure and a 3′–poly-A tail (16). Viruses belonging to the same category have some similar properties. The virus has four main structural proteins including spike (S), membrane (M), envelope (E), and nucleocapsid (N) proteins, which are required to regulate the function and viral structure (17). More importantly, N and S are the most important of these four proteins. N helps the virus to properly form the capsid and the entire viral structure. S helps the virus attach to the host cell (18, 19). The S protein consists of three parts, including a large outer domain, a single channel transmembrane anchor and a short intracellular tail. These three regions can accurately anchor the host cell. In these segments, the outer domain contains two subunits, the S1 receptor binding subunit and the S2 membrane fusion subunit. These subunits locate in the clove-trimer or crown structure, which is why it is named corona virus (corona = crown) (20).
The usual symptoms of COVID-19 include fever (83–98%), cough (59–82%), shortness of breath (19–55%), and muscle ache (11–44%), which are similar to those of SARS and MERS, especially the receptor binding domain (RBD) and the receptor binding motif (RBM) in the viral genome (21, 22). Fever is not the initial symptom in all patients, and some patients experience sore throat, rhinorrhea, headache, and confusion in the days preceding the fever (23). In addition, the characteristics of fever have not been fully defined, with a small proportion of patients presenting with hemoptysis and a large proportion without associated symptoms (24–26). Hemographic aspects, patients have normal or low white blood cell counts, lymphopenia or thrombocytopenia, and elevated C-reactive protein levels (23, 25, 27). Fever and upper respiratory symptoms with leukopenia or lymphopenia should be suspected, especially in patients with a history of travel to epidemic areas or close contact. More importantly, Genomic analysis showed that SARS-CoV-2 is closely related to SARS-CoV, with which it shares about 79% of its genome (28, 29). Angiotensin-converting enzyme 2 (ACE2), as their common receptor, reemerges as a hotspot owing to its indispensable role in facilitating cellular entry of SARS-CoV-2 and SARS-CoV (30), which indicates that a similar pathogenic mechanism is involved in both viral infections. Other similar co-receptors may include Neuropilin 1 (31) and CD4 (32). Interestingly, ACE2 protein is expressed in all organs of the human body, mainly distributed in the main target organs of coronavirus such as lung, kidney and intestine (33, 34). There are clinical reports of male patients experiencing problems such as erectile dysfunction (35) and sexual anhedonia (36) following recovery from infection (low libido). Furthermore, SARS-CoV-2 infects host cells through the ACE2 receptor, resulting in COVID-19-associated pneumonia, as well as causing acute myocardial damage and chronic damage to the cardiovascular system (37).
Noteworthy, it has also been suggested that the mechanism of action of COVID-19 in human infections is similar to SARS. According to the reports, an amino acid residue (Gln493) is the main component RBM of the SARS-CoV-2. Moreover, Gln493 promotes the binding of viral S proteins to viruses and fusion into ACE2 proteins in human cells, particularly in the lungs, which can lead to respiratory infections in humans (38). The structure and binding of S protein and ACE2 are described (Figure 1). The easiest and most direct way to fight SARS-CoV-2 is to prevent the virus from entering the cell because the method has been used in previous same kind viruses (19). The key advantage here is that the host ACE2 protein will not change, so there is no need to worry about favorable mutations that may hinder drug development (39).
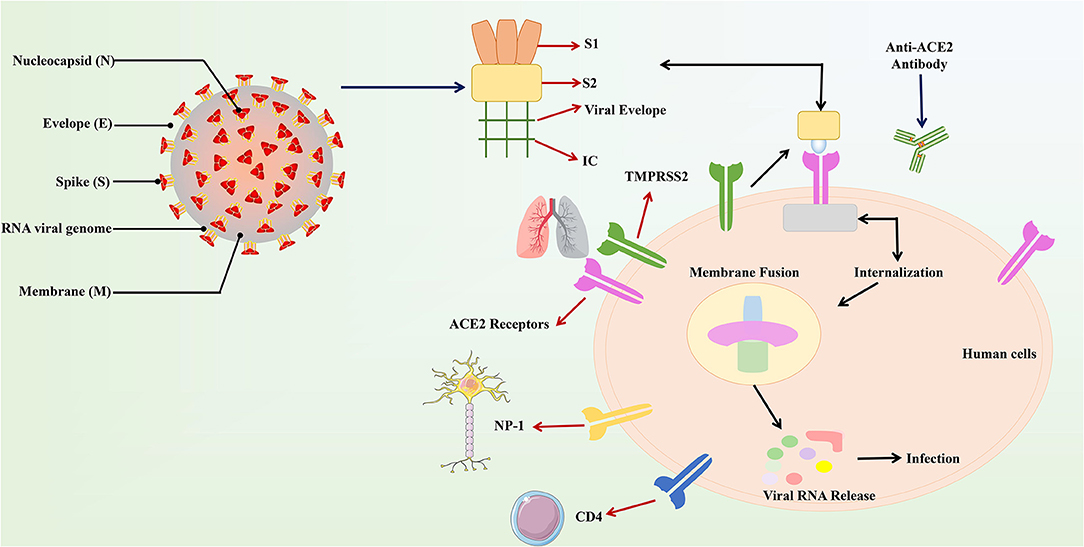
Figure 1. The structure and combination of COVID-19 virus and ACE2. The COVID-19 virus was described in the above figure. In the structure of the virus, the S protein plays a major role in the binding of the virus to the host recipient cell. The S protein is composed of the S1 receptor binding subunit and the S2 membrane fusion subunit. Then S2 subunit is internalized on the ACE2 receptor connected to human host cells and produces the membrane fusion among the viral subunit and the ACE2 receptors. This results in the release of viral RNA into the host cell and causes respiratory infections.
However, due to the novel nature of the virus, there is no effective drug treatment for COVID-19, so it is particularly important to understand the transmission method of COVID-19, and thereby preventing COVID-19 infection. Considering the severity of the COVID-19 outbreak all over the world, it is urgent to understand transmission pathways to control the spread of the disease to susceptible groups and to identify effective treatments. Hence, in order to control infections and develop effective management systems to deal with virus outbreaks, we should understand the ways of COVID-19 infection and evaluate the similarities and dissimilarities of the SARS-CoV-2 with the viruses that have caused outbreaks in the past. Based on the accumulated data and knowledge on the previous coronavirus infection, this review aims to fill the knowledge gap about the spread ways of COVID-19 and may better help prevent COVID-19 infection.
Pathogenesis and Epidemiology
Human Coronaviruses
Coronavirus belong to single-stranded zoonotic RNA virus that initially causes a common cold and further causes respiratory, intestinal, liver, and nervous system symptoms (40, 41), including HCoV-OC43, HCoV-229E, SARS-CoV, HCoV-HKU1, MERS-CoV and HCoVNL63 (42, 43). SARS-CoV-2 is the seventh reported coronavirus in human. Before the SARS-CoV outbreak, these infections were self-limiting in nature. Therefore, HCoV-229E and HCoV-OC43 coronavirus infections have been controlled half a century ago. After the SARS-CoV outbreak, HCoV-NL63 and HCoV-HKU1 were extracted and then controlled (5, 44). Approximately 15–30% of human respiratory infections are caused by these viruses each year. And individuals with weakened immunity are the most vulnerable groups, such as newborns, the elderly, and other chronic comorbidities.
In November 2002, the first known case of SARS occurred in Foshan, China. New cases emerged in mainland China, and by February 2003, more than 300 cases had been reported, around one-third of which were in health care workers (45). In March 2003, the WHO established a network of laboratories to determine the causative agent of SARS. A remarkable global effort led to the identification of SARS coronavirus (SARS-CoV) in early April of that year (46, 47). SARS-CoV is considered to be the most serious disease compared with other coronaviruses. The mortality rate of the SARS-CoV outbreak is 9%. By July 2003 and after a total of 8,096 reported cases, including 774 deaths in 27 countries. More importantly, the mortality rates among the elderly are as high as 50%. SARS not only has a high fatality rate but also causes a severe global economic recession, especially in Southeast Asia and Toronto, Canada (48). After the outbreak of SARS, it gradually spread to more than 24 countries. More importantly, the researchers demonstrated that the SARS-CoV sequence was found in Chinese horseshoe bats, and these bats were infected with a related virus before the outbreak, suggesting that Chinese horseshoe bats are the main reason for the SARS-CoVs outbreak (49, 50). SARS-CoV enters host cells by a mechanism involving the interaction between its S protein and human angiotensin-converting enzyme 2 (ACE2) expressed on host cells in the lung (51). During entry, S is cleaved into an N-terminal S1 region that mediates binding to ACE2 and a fusogenic C-terminal S2 region that mediates viral and host cell membranes (52, 53). The region designated S791 in the study corresponds to amino acids 620–900 of the S protein, which contains the cleavage sites for the S1 and S2 domains (54). S791 also contains an epitope recognized by serum samples from Vietnamese SARS convalescent patients (5). S protein is capable of inducing protective immunity (55–57). However, an antibody targeting the S1 domain enables antibody-dependent enhancement of SARS-CoV entry into host cells when the amino acid sequence of the S protein is altered (58, 59).
In June 2012, 10 years after the first emergence of SARS-CoV, a man in Saudi Arabia died of acute pneumonia and renal failure. A novel coronavirus, MERS-CoV was isolated from his sputum (6). A cluster of cases of the severe respiratory disease had occurred in April 2012 in a hospital in Jordan and was retrospectively diagnosed as MERS (60). According to WHO, since April 2012, 2019 laboratory confirmed cases of MERS-CoVs infection had been reported by January 2020. The lethality rate is 34.3%, with 866 confirmed deaths, the majority occurring in Saudi Arabia (2,121 cases, including 788 deaths, with a lethality rate of 37.1%) (61). Studies found that MERS-CoVs was closely associated with HKU4 and HKU5 (62). Therefore, MERS-CoVs is considered to be derived from bats and is similar to the source of SARS. Serum test results of MERS-CoV antibodies in dromedaries in Middle Eastern countries indicated that the intermediate host of MERS-CoV is camel (63). Importantly, MERS-CoVs were also found on humans and camels in Saudi Arabia and can be transmitted to humans through camels (64, 65). At the end of December 2019, an outbreak of mysterious pneumonia occurred in Wuhan City, Hubei Province, China (66, 67). It was observed that the etiologic agent of the disease was a novel CoV, previously designated 2019-nCoV by WHO and, more recently, SARS-CoV-2 by the International Committee for Taxonomy of Viruses (68). The outbreak appears to have started from a single or multiple zoonotic transmission events at a wet market in Wuhan where game animals and meat were sold (24), and then spread rapidly worldwide. At the latest count on 21st May 2021, the number of confirmed infected cases worldwide has exceeded 165,158,285 in 200 countries with cases reported in all continents and the global reported death toll has risen to 3,425,017. SARS-CoV-2 is a beta-coronavirus like the two other viruses that have caused fatal infections over the last 20 years: SARS-CoVs and MERS-CoVs. More importantly, the transmission from person to person was soon recognized due to the various cases of COVID-19 within families and among people who did not go to the Huanan market (69).
Epidemiology of COVID-19: Comparison With MERS and SARS
As of April 29, 2020, a total of 3,061,615 cases of COVID-19 occurring in at least 170 countries and territories were reported, with ~7% of mortality rate (213,577/3,061,615). A prior overview from China that included 72,314 confirmed, suspected, and asymptomatic patients revealed several important epidemiological features of COVID-19 (70). Most patients were between 35 and 55 years old, and children and infants are less infected (71, 72). A research on the dynamics of early transmission of the virus reported that the median age of patients was 59 years ranging from 15 to 89 years with the majority being males (59%) (13). People with low immune function, especially the elderly, and those with renal and liver dysfunction were vulnerable (13). SARS-CoV2 was found to have higher rates of transmissibility and pandemic risk than SARS-CoV, as the global effective reproductive number (R) of SARS-CoV2 is 2.9, which is much higher than SARS's R (1.77). Another study demonstrated that SARS-CoV2 is between 2.6 and 4.71. The incubation period of SARS-CoV-2 is about 4–8 days after infection (23, 73) (Figure 2).
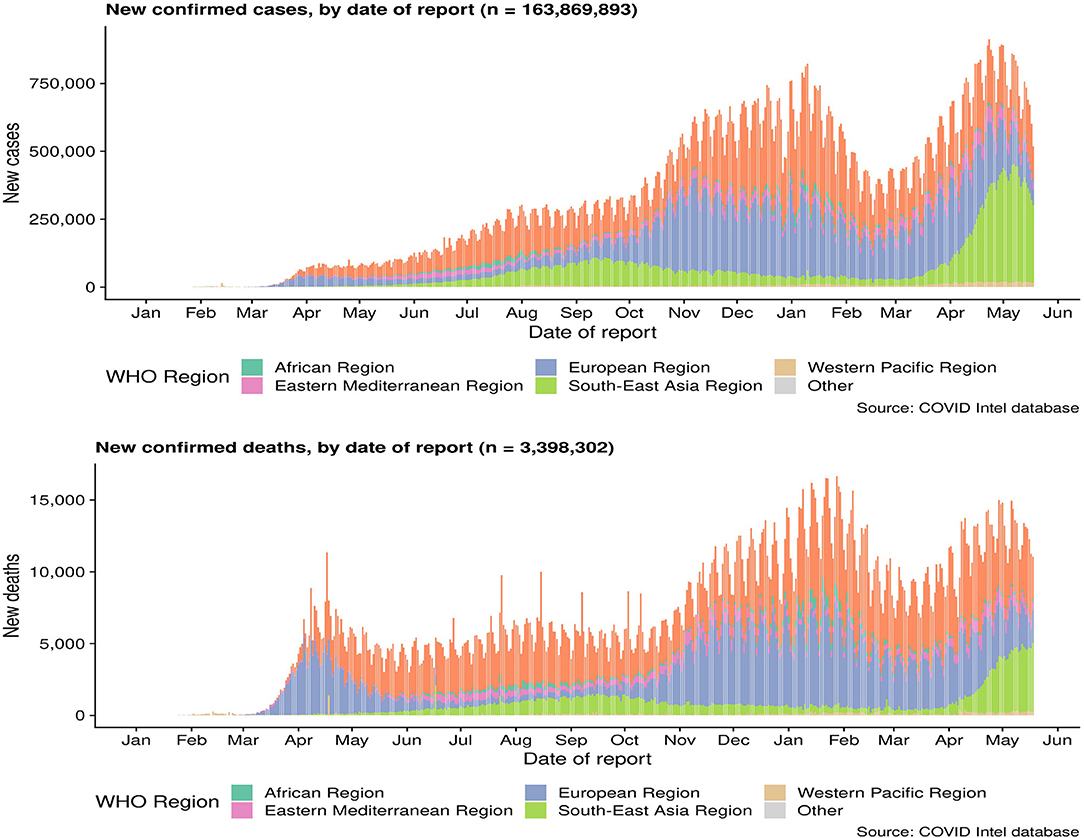
Figure 2. Cumulative confirmed cases and death cases of COVID-19. Data are from the World Health Organization.
Transmission of COVID-19: Comparison With MERS and SARS
Previous reports demonstrated that the interpersonal transmission of SARS-CoV and MERS-CoV was mainly transmitted through hospitals. Forty to one hundred percentage of MERS cases in individual outbreaks were associated with hospitals. In addition, similar observations were performed for some of the SARS clusters (74, 75). Researchers showed that 13–21% of MERS cases and 22–39% of SARS cases were transmitted by family members. Notably, transmission between patients occurred in 60–80% of MERS cases. Moreover, infection of health care workers by SARS-CoV-infected patients was very frequent (33–42%) (74). Therefore, the main reason for hospital spread to become the main mode of transmission is that a large amount of virus shedding only occurs after symptoms appear (76, 77), when most patients are already seeking medical care (78). An analysis of the surface of the hospital after the treatment of MERS-patients revealed that viral RNA is prevalent in the environment within a few days after the patient test is no longer positive (79). In addition, many SARS or MERS patients are infected through super spreaders (74, 77, 80–82).
On the other hand, it is speculated that the wet animal market in Wuhan, where live animals are sold, may be the origin of SARS-CoV2 zoonotic. Therefore, scientists are working hard to find a host reservoir or an intermediate vector in which the infection could spread to humans (11). Previous studies showed that the possible SARS-CoV2 reservoirs were the two snakes. However, the researchers only clearly demonstrated that mammals and birds were the reservoirs of coronavirus to date. SARS-CoV2, the causative agent of this respiratory disease, was identified and its genome is fully sequenced. The genomic sequence of SARS-CoV-2 showed a similar, but distinct genome composition of SARS-CoV and MERS-CoV, indicating that bats are the most likely connection between COVID-19 and humans (83). Several studies have shown that transmission from person to person is a possible way for COVID-19 infection to spread. Furthermore, SARS-CoV2 has infected many people who have not visited the wet animal market in Wuhan, further proving that COVID-19 can be transmitted from person to person (84). Three routes of transmission exist for SARS-CoV-2 in the human population: (1) inhalation of droplets generated by an infected person (2) close contact with an infected person (3) contact with an object surface contaminated with SARS-CoV-2 (85). SARS-CoV2 can be released when coughing, talking and sneezing of infected person. Droplets containing the virus can infect others if they do not follow a safe distance (86). Figure 2 shows the current global infection situation, indicating that the transmission rate of SARS-CoV2 is extremely fast. More importantly, recent studies reported that the newborn from infected mothers were not infected with SARS-CoV-2, suggesting that SARS-CoV-2 could not be transmitted vertically from mother to newborn. Nonetheless, the pregnant mother had a cesarean section, so it was still uncertain whether it would spread during vaginal delivery. This study is extremely important because pregnant women are more susceptible to respiratory infections and extreme pneumonia (83). In addition, previous studies have shown that weather has an important impact on infectious disease transmission, including but not limited to influenza and SARS. For example, sudden changes in ambient temperature increased the risk of SARS transmission (87, 88). Therefore, it is hypothesized that COVID-19 transmission may decrease or even disappear when the temperature and UV radiation increase in the summer. However, Ye et al. demonstrated that the spread ability of COVID-19 would not change with increasing temperature and increasing UV exposure. In addition, they did not find significant associations of relative humidity, maximum temperature and minimum temperature with cumulative incidence rate or R0 of SARS-CoV2, suggesting that it might be premature to count on warmer weather to control COVID-19 (89). SARS-CoV2 binds to the receptor expressed by the host cell is the first step of infection, and then fuse with the cell membrane. The primary target of SARS-CoV2 was the lung epithelial cells. Studies have shown that the combination between ACE2 and the receptor binding domain of the viral peak is the main link for SARS-CoV-2 to achieve human-to-human transmission (83). More importantly, the sequence of receptor binding domain of SARS-CoV2 is similar to SARS-CoV, indicating that the ACE2 receptor is the main factor for SARS-CoV2 to enter the host cell (90) (Figure 3). In addition, recent studies have found that the receptors of SARS-CoV2 may also include neuropilin-1 (NP-1), transmembrane protein serines2 (TMPRSS2), CD4, etc. The existence of these receptors is of great significance to the pathological progress and clinical manifestations of COVID-19. For example, the activation of virus S protein by host cell protease TMPRSS2 is a prerequisite for the invasion of SARS-CoV2 (91). For another example, the sharp decrease of CD4 + T cell count in patients with COVID-19 is associated with poor clinical outcomes (92). Moreover, NP-1 may be a way for SARS-CoV2 to enter the nervous system, which is related to the olfactory loss symptoms in some patients (32).
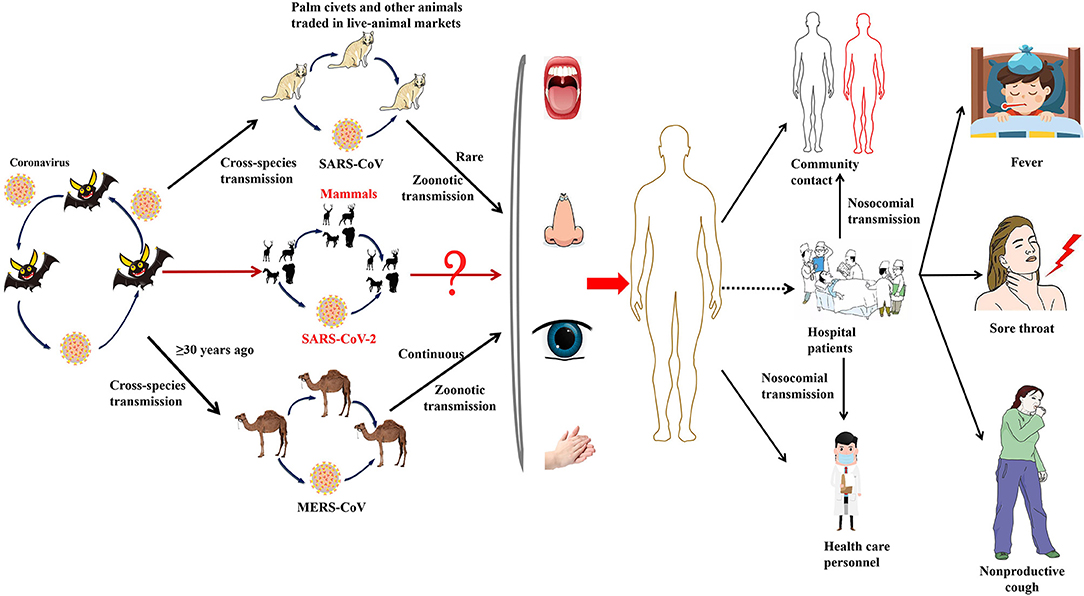
Figure 3. The emergence of SARS-CoV-2, SARS-CoV, and MERS-CoV. Bats harbor a wide range of coronaviruses, including SARS-CoV-like and MERS-CoV-like viruses. SARS-CoV crossed the species barrier into masked palm civets and other animals in live-animal markets in China; genetic analysis suggests that this occurred in late 2002. Several people in close proximity to palm civets became infected with SARS-CoV. A MERS-CoV ancestral virus crossed the species barrier into dromedary camels; serological evidence suggests that this happened more than 30 years ago. Abundant circulation of MERS-CoV in dromedary camels results in frequent zoonotic transmission of this virus. SARS-CoV-2, SARS-CoV, and MERS-CoV spread between humans. However, the main mode of transmission of COVID-19 is unclear.
Clinical Presentation of SARS-CoV-2: Comparison With SARS-CoV and MERS-CoV
The data obtained from different populations in the world and 31 provinces in China indicated that there are many similarities between the clinical symptoms of SARS-CoV-2 and the clinical symptoms of SARS-CoV infection (93, 94). The median age of the infected patients was 47 years. Therefore, males account for 58.2% of infected patients, and the mean incubation time was 3.0 days (range: 0–24.0 days) (95). More importantly, dyspnea develops within a median of 8 days after the onset of illness (range of 5–13 days), while in others, respiratory distress may be absent in some infected patients (73). The most frequently reported symptoms include cough-producing phlegm, runny nose, myalgia, fever, pneumonia, complicated dyspnea, body ache, fatigue, nausea, vomiting, diarrhea, conjunctivitis, skin lesions and anosmia, while the less widely documented symptoms include headache, vomiting and hemoptysis, which were similar to that of SARS-CoV infection (96, 97). Lymphocytes and white blood cells decreased, fever and new pulmonary infiltrates on chest radiography with no improvement in symptoms after 3 days of antibiotic treatment were considered to be the main clinical features that defined COVID-19 (8). Importantly, the intensive care may be required by 3–29% of infected patients. Severe patients may rapidly develop multiple organ dysfunction and eventually lead to death, and infected patients with shortness of breath and hypoxemia may rapidly develop acute respiratory distress syndrome (ARDS), severe sepsis accompanied by shock and even multiple organ dysfunction syndromes (MODS) within a week (73, 93).
Recently chest computed tomography (CT) of case series (n = 18) of infected patients were documented. They reported that typical CT findings included bilateral pulmonary parenchymal ground-glass and consolidative pulmonary opacities, sometimes with a rounded morphology and a peripheral lung distribution (98). Notably, lung cavitation, discrete pulmonary nodules, pleural effusions, and lymphadenopathy were absent (98). In addition, researchers found that fever, followed by fatigue, dry cough, myalgia, and dyspnea are the most common symptoms of infected patients in case series (n = 138) of COVID-19 in a hospital in Wuhan, China. Most infected people are almost 50 years old. The majority of infected patients who were admitted to ICU are the elderly and others with comorbidities, and the fatality rate reached 4.3% among patients in the study (73). Besides, the results of 41 laboratory-confirmed cases of COVID-19 in Wuhan, China showed that males, combined with other comorbidities, and exposed to Huanan seafood market were the main infected person. The symptoms were similar to the previously reported cases (28). It is worth noting that the direct damage of the virus to the intestine leads to the gastrointestinal symptoms of COVID-19, rather than the immunopathogenic response to host lung infection (99). Studies showed that the virus can be shed in the gastrointestinal tract and fecal-oral transmission due to the ACE2 that was expressed in the human gastrointestinal epithelial cells (100). Collectively, the epidemiology, transmission and clinical presentations of the most important coronavirus outbreaks were described in Table 1 (SARS-CoV-2, SARS-CoV, and MERS-CoV).
Prevention and Control of COVID-19
The methods and strategies for prevention and control of COVID-19 are reported at three levels, including national level, case-related population level, and general population level. At the national level, the National Health Commission of China issued “Announcement No. 1,” which formally incorporated COVID-19 into the management of Class B legal infectious diseases and allowed the implementation of prevention and control measures for Class A infectious diseases (101, 102). In order to prevent and control the spread of COVID-19, observation programs and isolation treatment can be carried out according to this policy in medical institutions (103). On January 22, 2020, the National Health Commission of China issued the “Guidelines for the Prevention and Control of New National Coronavirus Infection (COVID-19),” and requires medical institutions to do a good job in the prevention of nosocomial infections (101). On January 28, 2020, the “Guidelines for Rapid Prevention and Control Measures” was issued by the National Health Commission to effectively curb the spread of disease through the “Great Isolation and Disinfection” program during the Chinese New Year (101). Importantly, in order to better suppress the spread of COVID-19, the National Health Commission issued a series of targeted measures for the elderly population (released on January 31, 2020) and rural areas (released on January 28, 2020) (101). At present, the case isolation, identification and monitoring of contacts, environmental disinfection and the use of personal protective equipment were performed to prevent the spread of SARS-CoV-2 in 2019 in China (104). So far, infected patients treated with antiviral therapy have not been confirmed to be infected with COVID-19 again. Moreover, experts recommended that appropriate symptomatic treatment and supportive care should be taken for the treatment of patients with COVID-19. Additionally, studies reported that the psychological health issues and nosocomial infection were linked to COVID-19. It is recommended to take measures to minimize nosocomial infection including prevention and control awareness training, isolation, disinfection, graded protection in infected areas, and case report protection (105). More importantly, in terms of mental health, psychological intervention is recommended for medical personnel, suspected cases and confirmed cases (106).
At present, a total of 7 kinds of COVID-19 vaccines have been certified by the WHO. At present, the number of people vaccinated with the COVID-19 vaccine has exceeded the number of infected people, but it is still far from establishing an effective immune barrier. Therefore, avoiding exposure to SARS-CoV2 is the best preventive measure for the general population. The airborne measures and other protections were discussed and suggested for protection. According to CDC recommendations, the main strategies for preventing the spread of COVID-19, include(1) Covering sneeze or cough with a tissue and discarding the contaminated tissue in a contained trash; (2) Maintain social distance of six feet and above; (3) Avoiding travel to high risk areas; (4) Wash hands with soap and water for at least 20 s; (5) If soap was unavailable, use a disinfectant that contains at least 60% alcohol; (6) Avoiding hand contact with facial components; (7) Cleaning and disinfecting repeatedly touched surfaces (107). Moreover, the detailed recommendations of the use of face masks were issued by WHO within the population (108). Training courses on the correct use of surgical masks or N95 masks and various personal protective equipment were held for the staff. Those who failed to pass the mask use training twice received the training of powered air purification respirator (PAPR). This allows everyone in the team to be ready to respond to cases that may arise (109). Importantly, it is recommended that particulate masks should be used when medical personnels perform aerosol generation procedures, such as licensed N95 or FFP2 masks. The surgical masks should be used when medical personnels provide treatment for suspected or confirmed patients. It is necessary for all individuals, with or without symptoms of respiratory infection, to wear masks in public as there may be a significant number of people with asymptomatic infection in the population (110). In addition to papers published in scientific journals, the Chinese Center for Disease Control and Prevention also provides guidelines to increase public awareness of COVID-19 prevention and control, including causes of illness, how to choose and wear masks, correct hand washing methods, preventive measures taken in different places (such as homes, public transportation and public spaces), disinfection methods and home medical monitoring (110).
Ongoing Clinical Trials
Currently, existing antiviral drugs are shown to be ineffective in treating COVID-19 pneumonia. However, several clinical trials of potential antiviral drugs are initiated. The therapies can be divided into two categories according to their target. The first category is acting on coronavirus directly, either through suppressing viral enzymes, which regulate genome replication or via preventing the virus from entering the host cells. Another aims to regulate the human immune system, either via elevating the innate immune responses or through blocking the inflammatory processes which cause liver injury. These drugs were originally designed to inhibit other pathogens and are rapidly being used in current COVID-19 trials. At the same time, the specificity of specific vaccines and antibodies against SARS-CoV-2 was tested in several trials. Here, we summarize ongoing treatment options that may lead us to combat COVID-19 (Figure 4).
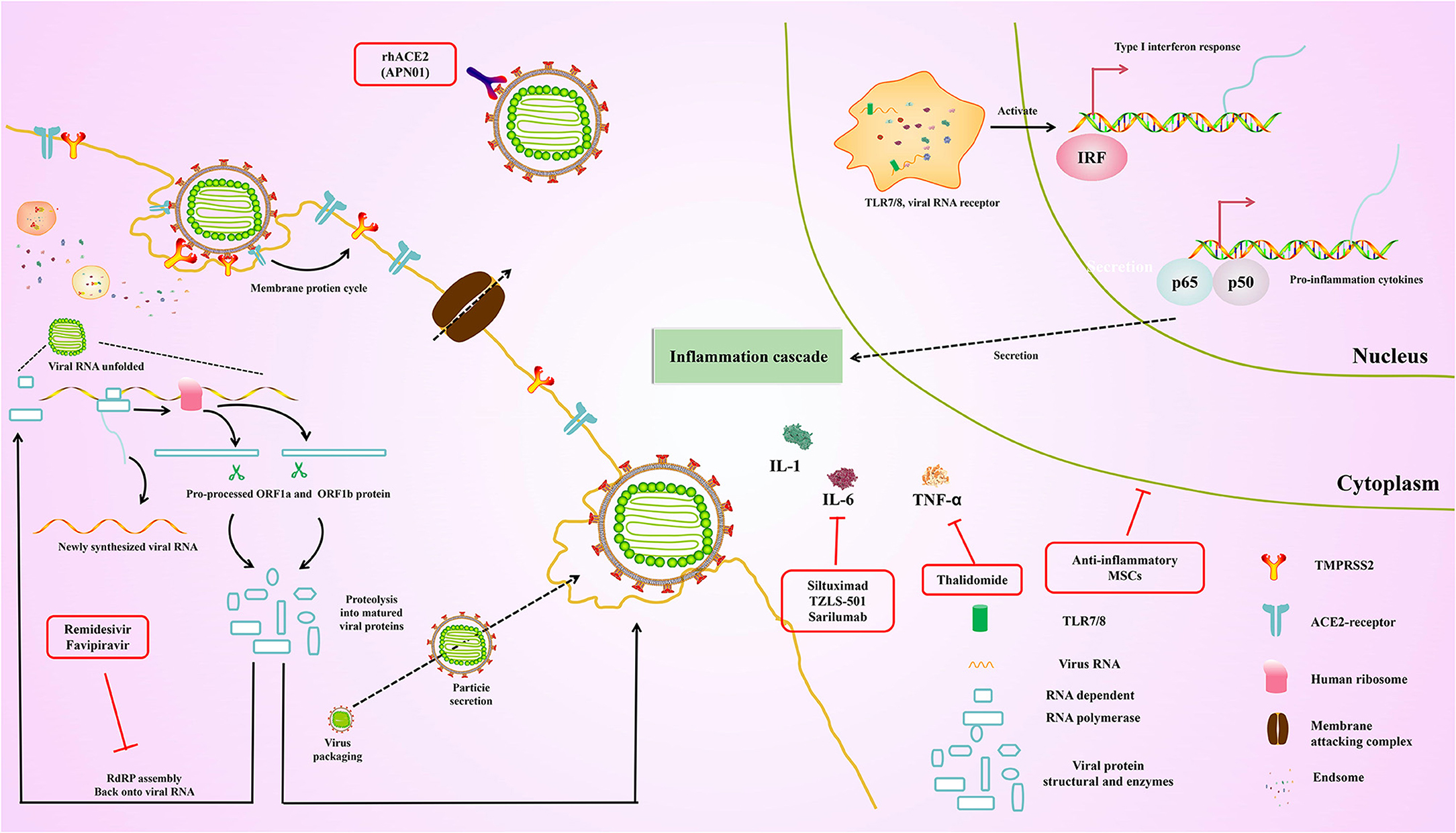
Figure 4. Overview of the repurposed therapeutic drugs undergoing clinical trial against COVID-19 in the context of host pathways and virus replication mechanisms.
Inhibiting the RNA-Dependent RNA Polymerase
Remdesivir
The specific drug, which belong to the adenosine nucleotide analog, is a promising and potential anti-RNA virus broad-spectrum antiviral drug (111). Therefore, it can enter into the nascent viral RNA and further inhibit RNA-dependent RNA polymerase, leading to the premature termination of the viral RNA, thereby inhibiting the replication of the viral genome. Remdesivir was originally used against the Ebola virus which was developed by Gilead Sciences (USA), and has gone through clinical trial during the recent Ebola outbreak in the Democratic Republic of Congo (112). Although there was no significant effect against Ebola in this trial, the drug has been shown to be safe in humans, which was introduced into clinical trials immediately against COVID-19 in an emergency (112). Previous studies have shown that remdesivir could be used in treatments against SARS-CoV and MERS-CoV (113, 114). Importantly, recent studies demonstrated that the SARS-CoV-2 was also inhibited (115). According to the reports of the first COVID-19 patient in the United States, it was proved that remdesivir significantly improved the condition of patients on the 8th day without s any noticeable adverse reactions (116). Another recent study has shown that remidesivir scored 0.77 μM at half-maximal concentration against COVID-19 and blocked viral infection (73, 115, 117). On October 22, 2020, remdesivir was approved by the U.S. FDA as the first COVID-19 drug across the United States.
Favipiravir
Favipiravir is similar to remidesivir which was developed by Toyama Chemical (Fujifilm Division, Japan). Of note, favipiravir is structurally similar to endogenous guanine, thereby resulting in the inhibition of RNA-dependent RNA polymerase. In addition, the competitive inhibition greatly reduces the effectiveness of virus replication (118). Although it is used against influenza, there are still fewer clinical studies supporting favipiravir in treatment for COVID-19 compared with the remdesivir. Nevertheless, favipiravir and interferon-α are given simultaneously to increase immunity and suppress the virus. Hence, the synergistic effect of favipiravir and interferon-α (ChiCTR2000029600) was assessed via recruiting the patients. Indeed, favipiravir was approved by China's National Medical Products Administration in March 2020 as China's first anti-SARS-CoV-2 drug due to its proven efficacy and low side effects, which was demonstrated by the clinical trial.
Blocking Virus–Cell Membrane Fusion
Recombinant Human ACE2 (APN01)
The entry of SARS-CoV-2 is inhibited by the soluble recombinant human ACE2 (rhACE2) via preventing the interaction between S protein and cellular ACE2. Currently, the recent studies reported that the RNA replication of SARS-CoV-2 is blocked by rhACE2 in cellular and embryonic stem cell-derived organoids, and its therapeutic effect by up to 1,000–5,000 times (119). More importantly, the rhACE2 keeps the substrate away from the associated enzyme, leading to the downregulation of serum level of angiotensin II, further inhibiting the ACE2 receptor activation, which could maintain the pulmonary vascular integrity and inhibit ARDS (120). Recently, APN01 was developed by Apeiron Biologics, and the phase II trial for ARDS is being performed. Importantly, the biological and physiological function of rhACE2 in SARS-CoV-2 is now assessed in China (NCT04287686). especially in the treatment of ARDS. In addition, the safety and tolerability of APN01 were also evaluated by Apeiron Biologics. Interestingly, the biological and physiological function of rhACE2 in SARS-CoV-2 is assessed via detecting the plasma level of angiotensin II and angiotensin 1–7 of bioproducts.
Arbidol Hydrochloride (Umifenovir)
Arbidol has been approved in Russia and China as an entry inhibitor against influenza viruses and arboviruses (121). Arbidol inhibits the fusion of viral membrane and endosome after endocytosis by targeting hemagglutinin (HA), which is the main glycoprotein on the surface of influenza virus. Currently, the clinical trial of a signal agent is underway in China (NCT04260594, NCT04255017). Additionally, a randomized clinical trial has shown that arbidol has more evidently therapeutic effects compared with favipiravir (ChiCTR2000030254) (122).
Enhancing the Innate Immune System
Natural Killer Cells
Accumulating reports revealed that the majority were among patients of ≥ 60 years of age, with the ≥ 80 age group characterized by the highest fatality rate (20.3%) among all age groups, which may because of the weakening of the immune system with age. Therefore, enhancing innate antiviral immune response may be an effective antiviral method. Natural killer cells (NK) are an important part of the innate immune system, which promote the sensitivity of immune system to virus. Previous studies have demonstrated that the SARS-CoV2 clearance can be regulated by the pulmonary migration of NK cells and macrophages (123). Additionally, the secretion of cytokines and chemokines are elevated by the innate immune system without the CD8+ T cells and antibodies, thus controlling the infection of COVID-19. The phase I trial is being performed to assess whether viral clearance from COVID-19 pneumonia is facilitated by NK cell, which is expected to be completed by the end of 2020. Currently, the related anti-cancer drugs based on NK cells may be used to treat COVID-19 by some companies.
Recombinant Interferon
The cells infected by virus secretion type I interferons, which have broad-spectrum antiviral effects against HCV, respiratory syncytial virus, SARS-CoV and MERs-CoV when used alone or in combination with other drugs (124, 125). Currently, clinical trials are performed to evaluate the safety and efficacy of type I interferons against SARS-CoV-2 (NCT04293887).
Convalescent Serum
There have been clinical studies showen that in COVID-19 patients convalescent plasma can play a great anti-inflammatory role. The mechanisms include the presence of IgM/IgG neutralizing antibodies that can inhibit viral entry into host cells and activate the host's immune system through antibody dependent cellular immunity. But the choice of the timing of rehabilitation therapy is important. If given in an inappropriate amount of time, cytokine storm syndrome may instead be triggered. When using rehabilitative plasma, the following points should be noted:
(1) Immunoglobulins are not completely homologous between individuals.
(2) Patients may have more viral load than the immunoglobulins transferred to them, that is, patients need more than one dose to be injected.
(3) Transferred Ig may reduce the Ig produced naturally by the patient's immune system.
(4) Antibodies produced by the patients themselves and transferred antibodies may form immune complexes, causing infusion reactions that can lead to nephritis or other serious consequences.
(5) There is a latent phase for the virus.
(6) There may be differences in the subtypes of immunoglobulins between donors and recipients.
(7) There is insufficient information on the predominant IgA in the recipient mucosal tissues.
Monoclonal Antibodies
Monoclonal antibodies are currently used primarily in the treatment of COVID-19 patients, and the first relevant human study was with LY-CoV555 directed against the spike protein, performed by the pharmaceutical company “Lilly,” which is currently in phase II trials. Recently, monoclonal antibodies such as Bamlanivimab and Casirivimab-Imdevimab were licensed for the treatment of outpatients with mild to moderate infection with SARS-CoV2. Of particular note, the early use of monoclonal antibodies (against IL-6, IL-1β, IL-17, interferon-γ TNF-α) is beneficial in controlling the covid-19 inflammatory storm, attenuating the progression of the condition, and reducing mortality. In addition, monoclonal antibodies are also being tried as vaccines and rapid diagnostic reagents with a bright future (126).
Corticosteroids
Studies of corticoid therapy for viral pneumonia and acute respiratory distress syndrome have had mixed results. However, a randomized evaluation of COVID-19 treatment (recovery) trial, which randomized 2,104 patients with COVID-19 to receive 6 mg dexamethasone daily for up to 10 days and 4,321 patients receiving usual care, found that dexamethasone reduced 28-day-all-cause mortality [21.6 vs. 24.6%; Age adjusted rate ratio, 0.83 (95% CI, 0.74–0.92); P < 0.001] By contrast, in patients with a shorter duration of symptoms and no supplemental oxygen requirement, there was no benefit (and potential for harm). A retrospective cohort study of 201 patients diagnosed with COVID-19 and acute respiratory distress syndrome in Wuhan, China, reported that treatment with methylprednisolone was associated with a reduced risk of death [hazard ratio, 0.38 (95% CI, 0.20–0.72)]. Overall, corticoid administration is beneficial for the control of inflammatory storm and is suitable for the treatment of severe COVID-19 patients (127).
Future Perspectives
Some strict measures need to be implemented to reduce the spread of COVID-19 from person to person, especially vulnerable people, including infants, health care providers and the elderly. In addition, the Chinese Center for Disease Control and Prevention issued protective guidelines for medical personnel, health care professionals, public health individuals and researchers associated with COVID-19 (106). Among the cases, the majority were the elderly and characterized by the highest fatality rate due to the poor immune system, which causes the virus infection to progress faster (128). The public facilities should be disinfected regularly. In addition, contact should be avoided when handling infected samples, in order to prevent infection COVID-19 (129). The prevention and control measures were implemented in the world, especially the United States, China, India, and the UAE, such as blockades and travel screening in order to control the spread of COVID-19. In addition, we should closely monitor the epidemiological changes of COVID-19 infection, such as possible transmission routes, subclinical infections, adaptation, evolution, possible intermediate animals and reservoir and transmission between humans. There are still many problems to be solved. Including the identity, the number of people tested, how many of the test people are positive, whether this ratio remains the same or changes. So far, only a few pediatric cases have been reported due to lack of sensitivity or insufficient testing.
Conclusion
SARS originated from a wildlife trade in Shunde, Guangdong, China, where the virus could be transmitted by contact with patient droplets or respiratory secretions. After the virus enters the human body, it binds to the ACE2 receptor of lung epithelial cells, and utilizes the host cell system for replication, transcription, and translation. The way MERS-CoV infects cells is extremely similar to that of SARS-CoV, but unlike the latter, the receptor that MERS-CoV binds to in humans is DPP4. The intermediate host for MERS is the dromedary camel, where the virus is transmitted from bats to dromedaries and then from dromedaries to humans. Like SARS-CoV, the receptor for SARS-CoV-2 also includes ACE2. SARS-CoV-2 can bind to ACE2 receptors of animals such as humans, bats, and pigs, suggesting that these animals may be intermediate hosts of SARS-CoV-2. These virus outbreaks will permanently exist or even undergo secondary outbreaks due to the genetic changes that are inevitable in the process of virus evolution. Therefore, developing effective antiviral therapeutics and vaccines for COVID-19 is urgent. To achieve this goal, we need to fully understand the molecular mechanism and pathogenic principle of the virus. Analysis of the correlation and differences between the SARS-CoV-2 epidemic and previous coronavirus outbreaks, particularly the integrated use of biochemical, proteomic, genetic, structural, bioinformatic, virological, and imaging methods to find pan coronavirus therapeutic targets, may provide us with clues for the development of new COVID-19 therapeutics and vaccines. Several pan coronavirus therapeutic targets such as Tom70, sigma-1 (130), etc. have been reported. As a long-standing effort has been devoted to the development of a universal influenza vaccine in general, the development of a universal coronavirus vaccine should also be put on the agenda, which is perhaps less difficult than that of a universal influenza vaccine.
Author Contributions
JY, HZ, and HF drafted the manuscript. LZ and LW revised the manuscript. BC, XZ, and CZ reviewed and modified the manuscript. SC reviewed and modified the Figure. All authors agreed on the final version.
Funding
This study was supported by the National Natural Science Foundation of China (Grant Nos. 81673444, 81473223, and U1803129).
Conflict of Interest
The authors declare that the research was conducted in the absence of any commercial or financial relationships that could be construed as a potential conflict of interest.
Publisher's Note
All claims expressed in this article are solely those of the authors and do not necessarily represent those of their affiliated organizations, or those of the publisher, the editors and the reviewers. Any product that may be evaluated in this article, or claim that may be made by its manufacturer, is not guaranteed or endorsed by the publisher.
References
1. Lai MM, Cavanagh D. The molecular biology of coronaviruses. Adv Virus Res. (1997) 48:1–100. doi: 10.1016/S0065-3527(08)60286-9
2. Masters PS. The molecular biology of coronaviruses. Adv Virus Res. (2006) 66:193–292. doi: 10.1016/S0065-3527(06)66005-3
3. Stadler K, Masignani V, Eickmann M, Becker S, Abrignani S, Klenk HD, et al. SARS–beginning to understand a new virus. Nat Rev Microbiol. (2003) 1:209–18. doi: 10.1038/nrmicro775
4. Woo PC, Lau SK, Chu CM, Chan KH, Tsoi HW, Huang Y, et al. Characterization and complete genome sequence of a novel coronavirus, coronavirus HKU1, from patients with pneumonia. J Virol. (2005) 79:884–95. doi: 10.1128/JVI.79.2.884-895.2005
5. van der Hoek L, Pyrc K, Jebbink MF, Vermeulen-Oost W, Berkhout RJ, Wolthers KC, et al. Identification of a new human coronavirus. Nat Med. (2004) 10:368–73. doi: 10.1038/nm1024
6. Zaki AM, van Boheemen S, Bestebroer TM, Osterhaus AD, Fouchier RA. Isolation of a novel coronavirus from a man with pneumonia in Saudi Arabia. N Engl J Med. (2012) 367:1814–20. doi: 10.1056/NEJMoa1211721
7. de Wit E, van Doremalen N, Falzarano D, Munster VJ. SARS and MERS: recent insights into emerging coronaviruses. Nat Rev Microbiol. (2016) 14:523–34. doi: 10.1038/nrmicro.2016.81
8. Cui J, Li F, Shi ZL. Origin and evolution of pathogenic coronaviruses. Nat Rev Microbiol. (2019) 17:181–92. doi: 10.1038/s41579-018-0118-9
9. Hilgenfeld R, Peiris M. From SARS to MERS: 10 years of research on highly pathogenic human coronaviruses. Antiviral Res. (2013) 100:286–95. doi: 10.1016/j.antiviral.2013.08.015
10. Wu F, Zhao S, Yu B, Chen YM, Wang W, Song ZG, et al. A new coronavirus associated with human respiratory disease in China. Nature. (2020) 579:265–9. doi: 10.1038/s41586-020-2008-3
11. Bassetti M, Vena A, Giacobbe DR. The novel Chinese coronavirus (2019-nCoV) infections: challenges for fighting the storm. Eur J Clin Invest. (2020) 50:e13209. doi: 10.1111/eci.13209
12. Rabaan AA, Al-Ahmed SH, Haque S, Sah R, Tiwari R, Malik YS, et al. SARS-CoV-2, SARS-CoV, and MERS-COV: a comparative overview. Infez Med. (2020) 28:174–84.
13. Li Q, Guan X, Wu P, Wang X, Zhou L, Tong Y, et al. Early transmission dynamics in Wuhan, China, of novel coronavirus-infected pneumonia. N Engl J Med. (2020) 382:1199–207. doi: 10.1056/NEJMoa2001316
14. Riou J, Althaus CL. Pattern of early human-to-human transmission of Wuhan 2019 novel coronavirus (2019-nCoV), December 2019 to January 2020. Euro Surveill. (2020) 25:2000058. doi: 10.2807/1560-7917.ES.2020.25.4.2000058
15. Rodriguez-Morales AJ, Bonilla-Aldana DK, Balbin-Ramon GJ, Rabaan AA, Sah R, Paniz-Mondolfi A, et al. History is repeating itself: probable zoonotic spillover as the cause of the 2019 novel coronavirus epidemic. Infez Med. (2020) 28:3–5.
16. Chen J. Pathogenicity and transmissibility of 2019-nCoV-A quick overview and comparison with other emerging viruses. Microbes Infect. (2020) 22:69–71. doi: 10.1016/j.micinf.2020.01.004
17. She J, Liu L, Liu W. COVID-19 epidemic: disease characteristics in children. J Med Virol. (2020) 92:747–54. doi: 10.1002/jmv.25807
18. Siu YL, Teoh KT, Lo J, Chan CM, Kien F, Escriou N, et al. The M, E, and N structural proteins of the severe acute respiratory syndrome coronavirus are required for efficient assembly, trafficking, and release of virus-like particles. J Virol. (2008) 82:11318–30. doi: 10.1128/JVI.01052-08
19. Walls AC, Park YJ, Tortorici MA, Wall A, McGuire AT, Veesler D. Structure, function, and antigenicity of the SARS-CoV-2 spike glycoprotein. Cell. (2020) 181:281–92.e6. doi: 10.1016/j.cell.2020.02.058
20. Zumla A, Chan JF, Azhar EI, Hui DS, Yuen KY. Coronaviruses - drug discovery and therapeutic options. Nat Rev Drug Discov. (2016) 15:327–47. doi: 10.1038/nrd.2015.37
21. Jiang S, Du L, Shi Z. An emerging coronavirus causing pneumonia outbreak in Wuhan, China: calling for developing therapeutic and prophylactic strategies. Emerg Microbes Infect. (2020) 9:275–7. doi: 10.1080/22221751.2020.1723441
22. Zhang L, Lin D, Sun X, Curth U, Drosten C, Sauerhering L, et al. Crystal structure of SARS-CoV-2 main protease provides a basis for design of improved alpha-ketoamide inhibitors. Science. (2020) 368:409–12. doi: 10.1126/science.abb3405
23. Huang C, Wang Y, Li X, Ren L, Zhao J, Hu Y, et al. Clinical features of patients infected with 2019 novel coronavirus in Wuhan, China. Lancet. (2020) 395:497–506. doi: 10.1016/S0140-6736(20)30183-5
24. Chan JF, Yuan S, Kok KH, To KK, Chu H, Yang J, et al. A familial cluster of pneumonia associated with the 2019 novel coronavirus indicating person-to-person transmission: a study of a family cluster. Lancet. (2020) 395:514–23. doi: 10.1016/S0140-6736(20)30154-9
25. Fu L, Wang B, Yuan T, Chen X, Ao Y, Fitzpatrick T, et al. Clinical characteristics of coronavirus disease 2019 (COVID-19) in China: a systematic review and meta-analysis. J Infect. (2020) 80:656–65. doi: 10.1016/j.jinf.2020.03.041
26. Wang Z, Yang B, Li Q, Wen L, Zhang R. Clinical features of 69 cases with coronavirus disease 2019 in Wuhan, China. Clin Infect Dis. (2020) 71:769–77. doi: 10.1093/cid/ciaa272
27. Hurley MM, Kream BE, Raisz LG. Structural determinants of the capacity of heparin to inhibit collagen synthesis in 21-day fetal rat calvariae. J Bone Miner Res. (1990) 5:1127–33. doi: 10.1002/jbmr.5650051106
28. Lu R, Zhao X, Li J, Niu P, Yang B, Wu H, et al. Genomic characterisation and epidemiology of 2019 novel coronavirus: implications for virus origins and receptor binding. Lancet. (2020) 395:565–74. doi: 10.1016/S0140-6736(20)30251-8
29. Zhou P, Yang XL, Wang XG, Hu B, Zhang L, Zhang W, et al. A pneumonia outbreak associated with a new coronavirus of probable bat origin. Nature. (2020) 579:270–3.
30. Pandey A, Bigoniya P, Raj V, Patel KK. Pharmacological screening of Coriandrum sativum Linn. for hepatoprotective activity. J Pharm Bioallied Sci. (2011) 3:435–41. doi: 10.4103/0975-7406.84462
31. Daly JL, Simonetti B, Klein K, Chen KE, Williamson MK, Anton-Plagaro C, et al. Neuropilin-1 is a host factor for SARS-CoV-2 infection. Science. (2020) 370:861–5. doi: 10.1126/science.abd3072
32. Chiappelli F, Khakshooy A, Greenberg G. CoViD-19 immunopathology and immunotherapy. Bioinformation. (2020) 16:219–22. doi: 10.6026/97320630016219
33. Ding Y, He L, Zhang Q, Huang Z, Che X, Hou J, et al. Organ distribution of severe acute respiratory syndrome (SARS) associated coronavirus (SARS-CoV) in SARS patients: implications for pathogenesis and virus transmission pathways. J Pathol. (2004) 203:622–30. doi: 10.1002/path.1560
34. Anastasiou IA, Eleftheriadou I, Tentolouris A, Tsilingiris D, Tentolouris N. In vitro data of current therapies for SARS-CoV-2. Curr Med Chem. (2020) 27:4542–8. doi: 10.2174/0929867327666200513075430
35. Sansone A, Mollaioli D, Ciocca G, Limoncin E, Colonnello E, Vena W, et al. Addressing male sexual and reproductive health in the wake of COVID-19 outbreak. J Endocrinol Invest. (2021) 44:223–31. doi: 10.1007/s40618-020-01350-1
36. Shoar S, Khavandi S, Tabibzadeh E, Vaez A, Oskouei AK, Hosseini F, et al. A late COVID-19 complication: male sexual dysfunction. Prehosp Disaster Med. (2020) 35:688–9. doi: 10.1017/S1049023X20001223
37. Zheng M, Song L. Novel antibody epitopes dominate the antigenicity of spike glycoprotein in SARS-CoV-2 compared to SARS-CoV. Cell Mol Immunol. (2020) 17:536–8. doi: 10.1038/s41423-020-0385-z
38. Yin Y, Wunderink RG. MERS. SARS and other coronaviruses as causes of pneumonia. Respirology. (2018) 23:130–7. doi: 10.1111/resp.13196
39. Killerby ME, Biggs HM, Haynes A, Dahl RM, Mustaquim D, Gerber SI, et al. Human coronavirus circulation in the United States 2014-2017. J Clin Virol. (2018) 101:52–6. doi: 10.1016/j.jcv.2018.01.019
40. Aluga MA. Coronavirus disease 2019 (COVID-19) in Kenya: preparedness, response and transmissibility. J Microbiol Immunol Infect. (2020) 53:671–3. doi: 10.1016/j.jmii.2020.04.011
41. Columbus C, Brust KB, Arroliga AC. 2019 novel coronavirus: an emerging global threat. Proceedings. (2020) 33:209–12. doi: 10.1080/08998280.2020.1731272
42. Su S, Wong G, Shi W, Liu J, Lai ACK, Zhou J, et al. Epidemiology, genetic recombination, and pathogenesis of coronaviruses. Trends Microbiol. (2016) 24:490–502. doi: 10.1016/j.tim.2016.03.003
43. Chen Y, Liu Q, Guo D. Emerging coronaviruses: genome structure, replication, and pathogenesis. J Med Virol. (2020) 92:418–23. doi: 10.1002/jmv.25681
44. McIntosh K, Becker WB, Chanock RM. Growth in suckling-mouse brain of “IBV-like” viruses from patients with upper respiratory tract disease. Proc Natl Acad Sci USA. (1967) 58:2268–73. doi: 10.1073/pnas.58.6.2268
45. Zhong NS, Zheng BJ, Li YM, Poon, Xie ZH, Chan KH, et al. Epidemiology and cause of severe acute respiratory syndrome (SARS) in Guangdong, people's republic of China, in February, 2003. Lancet. (2003) 362:1353–8. doi: 10.1016/S0140-6736(03)14630-2
46. Drosten C, Gunther S, Preiser W, van der Werf S, Brodt HR, Becker S, et al. Identification of a novel coronavirus in patients with severe acute respiratory syndrome. N Engl J Med. (2003) 348:1967–76. doi: 10.1056/NEJMoa030747
47. File TM Jr, Tsang KW. Severe acute respiratory syndrome: pertinent clinical characteristics and therapy. Treat Respir Med. (2005) 4:95–106. doi: 10.2165/00151829-200504020-00003
48. Fehr AR, Perlman S. Coronaviruses: an overview of their replication and pathogenesis. Methods Mol Biol. (2015) 1282:1–23. doi: 10.1007/978-1-4939-2438-7_1
49. Lau SK, Woo PC, Li KS, Huang Y, Tsoi HW, Wong BH, et al. Severe acute respiratory syndrome coronavirus-like virus in Chinese horseshoe bats. Proc Natl Acad Sci USA. (2005) 102:14040–5. doi: 10.1073/pnas.0506735102
50. Li W, Shi Z, Yu M, Ren W, Smith C, Epstein JH, et al. Bats are natural reservoirs of SARS-like coronaviruses. Science. (2005) 310:676–9. doi: 10.1126/science.1118391
51. Kuhn JH, Li W, Choe H, Farzan M. Angiotensin-converting enzyme 2: a functional receptor for SARS coronavirus. Cell Mol Life Sci. (2004) 61:2738–43. doi: 10.1007/s00018-004-4242-5
52. Tripet B, Howard MW, Jobling M, Holmes RK, Holmes KV, Hodges RS. Structural characterization of the SARS-coronavirus spike S fusion protein core. J Biol Chem. (2004) 279:20836–49. doi: 10.1074/jbc.M400759200
53. Xu Y, Lou Z, Liu Y, Pang H, Tien P, Gao GF, et al. Crystal structure of severe acute respiratory syndrome coronavirus spike protein fusion core. J Biol Chem. (2004) 279:49414–9. doi: 10.1074/jbc.M408782200
54. Belouzard S, Chu VC, Whittaker GR. Activation of the SARS coronavirus spike protein via sequential proteolytic cleavage at two distinct sites. Proc Natl Acad Sci USA. (2009) 106:5871–6. doi: 10.1073/pnas.0809524106
55. He Y, Zhou Y, Liu S, Kou Z, Li W, Farzan M, et al. Receptor-binding domain of SARS-CoV spike protein induces highly potent neutralizing antibodies: implication for developing subunit vaccine. Biochem Biophys Res Commun. (2004) 324:773–81. doi: 10.1016/j.bbrc.2004.09.106
56. Greenough TC, Babcock GJ, Roberts A, Hernandez HJ, Thomas WD Jr, Coccia JA, et al. Development and characterization of a severe acute respiratory syndrome-associated coronavirus-neutralizing human monoclonal antibody that provides effective immunoprophylaxis in mice. J Infect Dis. (2005) 191:507–14. doi: 10.1086/427242
57. Lai SC, Chong PC, Yeh CT, Liu LS, Jan JT, Chi HY, et al. Characterization of neutralizing monoclonal antibodies recognizing a 15-residues epitope on the spike protein HR2 region of severe acute respiratory syndrome coronavirus (SARS-CoV). J Biomed Sci. (2005) 12:711–27. doi: 10.1007/s11373-005-9004-3
58. Yang ZY, Werner HC, Kong WP, Leung K, Traggiai E, Lanzavecchia A, et al. Evasion of antibody neutralization in emerging severe acute respiratory syndrome coronaviruses. Proc Natl Acad Sci USA. (2005) 102:797–801. doi: 10.1073/pnas.0409065102
59. Kam YW, Kien F, Roberts A, Cheung YC, Lamirande EW, Vogel L, et al. Antibodies against trimeric S glycoprotein protect hamsters against SARS-CoV challenge despite their capacity to mediate FcgammaRII-dependent entry into B cells in vitro. Vaccine. (2007) 25:729–40. doi: 10.1016/j.vaccine.2006.08.011
60. Hijawi B, Abdallat M, Sayaydeh A, Alqasrawi S, Haddadin A, Jaarour N, et al. Novel coronavirus infections in Jordan, April 2012: epidemiological findings from a retrospective investigation. East Mediterr Health J. (2013) 19 (Suppl. 1):S12–8. doi: 10.26719/2013.19.supp1.S12
61. Bialek SR, Allen D, Alvarado-Ramy F, Arthur R, Balajee A, Bell D, et al. First confirmed cases of middle east respiratory syndrome coronavirus (MERS-CoV) infection in the United States, updated information on the epidemiology of MERS-CoV infection, and guidance for the public, clinicians, and public health authorities - May 2014. MMWR Morb Mortal Wkly Rep. (2014) 63:431–6.
62. Debiaggi M, Canducci F, Ceresola ER, Clementi M. The role of infections and coinfections with newly identified and emerging respiratory viruses in children. Virol J. (2012) 9:247. doi: 10.1186/1743-422X-9-247
63. Meyer B, Muller MA, Corman VM, Reusken CB, Ritz D, Godeke GJ, et al. Antibodies against MERS coronavirus in dromedary camels, United Arab emirates, 2003 and 2013. Emerg Infect Dis. (2014) 20:552–9. doi: 10.3201/eid2004.131746
64. Madani TA, Azhar EI, Hashem AM. Evidence for camel-to-human transmission of MERS coronavirus. N Engl J Med. (2014) 371:1360. doi: 10.1056/NEJMc1409847
65. Memish ZA, Cotten M, Meyer B, Watson SJ, Alsahafi AJ, Al Rabeeah AA, et al. Human infection with MERS coronavirus after exposure to infected camels, Saudi Arabia, 2013. Emerg Infect Dis. (2014) 20:1012–1015. doi: 10.3201/eid2006.140402
66. Du Z, Wang L, Cauchemez S, Xu X, Wang X, Cowling BJ, et al. Risk for transportation of coronavirus disease from wuhan to other cities in China. Emerg Infect Dis. (2020) 26:1049–52. doi: 10.3201/eid2605.200146
67. Zhu N, Zhang D, Wang W, Li X, Yang B, Song J, et al. A novel coronavirus from patients with pneumonia in China, 2019. N Engl J Med. (2020) 382:727–33. doi: 10.1056/NEJMoa2001017
68. Gralinski LE, Menachery VD. Return of the coronavirus: 2019-nCoV. Viruses. (2020) 12:135. doi: 10.3390/v12020135
69. da Costa VG, Moreli ML, Saivish MV. The emergence of SARS. MERS and novel SARS-2 coronaviruses in the 21st century. Arch Virol. (2020) 165:1517–26. doi: 10.1007/s00705-020-04628-0
70. Chen N, Zhou M, Dong X, Qu J, Gong F, Han Y, et al. Epidemiological and clinical characteristics of 99 cases of 2019 novel coronavirus pneumonia in Wuhan, China: a descriptive study. Lancet. (2020) 395:507–13. doi: 10.1016/S0140-6736(20)30211-7
71. Zhao Z, Gao D. Precaution of 2019 novel coronavirus infection in department of oral and maxillofacial surgery. Br J Oral Maxillofac Surg. (2020) 58:250–3. doi: 10.1016/j.bjoms.2020.03.001
72. Zimmermann P, Curtis N. Coronavirus infections in children including COVID-19: an overview of the epidemiology, clinical features, diagnosis, treatment and prevention options in children. Pediatr Infect Dis J. (2020) 39:355–68. doi: 10.1097/INF.0000000000002660
73. Wang D, Hu B, Hu C, Zhu F, Liu X, Zhang J, et al. Clinical characteristics of 138 hospitalized patients with 2019 Novel coronavirus-infected pneumonia in Wuhan, China. JAMA. (2020) 323:1061–9. doi: 10.1001/jama.2020.1585
74. Chowell G, Abdirizak F, Lee S, Lee J, Jung E, Nishiura H, et al. Transmission characteristics of MERS and SARS in the healthcare setting: a comparative study. BMC Med. (2015) 13:210. doi: 10.1186/s12916-015-0450-0
75. Hunter JC, Nguyen D, Aden B, Al Bandar Z, Al Dhaheri W, Abu Elkheir K, et al. Transmission of middle east respiratory syndrome coronavirus infections in healthcare settings, Abu Dhabi. Emerg Infect Dis. (2016) 22:647–56. doi: 10.3201/eid2204.151615
76. Anderson RM, Fraser C, Ghani AC, Donnelly CA, Riley S, Ferguson NM, et al. Epidemiology, transmission dynamics and control of SARS: the 2002-2003 epidemic. Philos Trans R Soc Lond B Biol Sci. (2004) 359:1091–105. doi: 10.1098/rstb.2004.1490
77. Cowling BJ, Park M, Fang VJ, Wu P, Leung GM, Wu JT. Preliminary epidemiological assessment of MERS-CoV outbreak in South Korea, May to June 2015. Euro Surveill. (2015) 20:7–13. doi: 10.2807/1560-7917.ES2015.20.25.21163
78. Peiris JS, Chu CM, Cheng VC, Chan KS, Hung IF, Poon LL, et al. Clinical progression and viral load in a community outbreak of coronavirus-associated SARS pneumonia: a prospective study. Lancet. (2003) 361:1767–72. doi: 10.1016/S0140-6736(03)13412-5
79. Bin SY, Heo JY, Song MS, Lee J, Kim EH, Park SJ, et al. Environmental contamination and viral shedding in MERS patients during MERS-CoV outbreak in South Korea. Clin Infect Dis. (2016) 62:755–60. doi: 10.1093/cid/civ1020
80. Korea Centers for Disease Control and Prevention. Middle East Respiratory Syndrome Coronavirus Outbreak in the Republic of Korea, 2015. Osong Public Health Res Perspect. (2015) 6:269–78. doi: 10.1016/j.phrp.2015.08.006
81. Kucharski AJ, Althaus CL. The role of superspreading in Middle East respiratory syndrome coronavirus (MERS-CoV) transmission. Euro Surveill. (2015) 20:14–8. doi: 10.2807/1560-7917.ES2015.20.25.21167
82. Wong G, Liu W, Liu Y, Zhou B, Bi Y, Gao GF. MERS, SARS, and ebola: the role of super-spreaders in infectious disease. Cell Host Microbe. (2015) 18:398–401. doi: 10.1016/j.chom.2015.09.013
83. Jaimes JA, Millet JK, Stout AE, Andre NM, Whittaker GR. A tale of two viruses: the distinct spike glycoproteins of feline coronaviruses. Viruses. (2020) 12:83. doi: 10.3390/v12010083
84. Douedi S, Miskoff J. Novel coronavirus 2019 (COVID-19): a case report and review of treatments. Medicine. (2020) 99:e20207. doi: 10.1097/MD.0000000000020207
85. Liu YC, Kuo RL, Shih SR. COVID-19: The first documented coronavirus pandemic in history. Biomed J. (2020) 43:328–33. doi: 10.1016/j.bj.2020.04.007
86. Zhang M, Wang L, Yu S, Sun G, Lei H, Wu W. Status of occupational protection in the COVID-19 fangcang shelter hospital in Wuhan, China. Emerg Microbes Infect. (2020) 9:1835–42. doi: 10.1080/22221751.2020.1803145
87. Zak J, Povysil C, Pacovsky V, Marek J. [Comparative histomorphometric study of acromegalic spongy bone. Formative static parameters]. Cas Lek Cesk. (1990) 129:1191–4.
88. Tan J, Mu L, Huang J, Yu S, Chen B, Yin J. An initial investigation of the association between the SARS outbreak and weather: with the view of the environmental temperature and its variation. J Epidemiol Community Health. (2005) 59:186–92. doi: 10.1136/jech.2004.020180
89. Yao Y, Pan J, Liu Z, Meng X, Wang W, Kan H, et al. No association of COVID-19 transmission with temperature or UV radiation in Chinese cities. Eur Respir J. (2020) 55:2000517. doi: 10.1183/13993003.00517-2020
90. Wan Y, Shang J, Graham R, Baric RS, Li F. Receptor recognition by the novel coronavirus from wuhan: an analysis based on decade-long structural studies of SARS coronavirus. J Virol. (2020) 94:e00127–20. doi: 10.1128/JVI.00127-20
91. Hoffmann M, Kleine-Weber H, Schroeder S, Kruger N, Herrler T, Erichsen S, et al. SARS-CoV-2 cell entry depends on ACE2 and TMPRSS2 and is blocked by a clinically proven protease inhibitor. Cell. (2020) 181:271–80.e8. doi: 10.1016/j.cell.2020.02.052
92. Peng X, Ouyang J, Isnard S, Lin J, Fombuena B, Zhu B, et al. Sharing CD4+ T Cell loss: when COVID-19 and HIV collide on immune system. Front Immunol. (2020) 11:596631. doi: 10.3389/fimmu.2020.596631
93. Rolf JD. Clinical characteristics of Covid-19 in China. N Engl J Med. (2020) 382:1860. doi: 10.1056/NEJMc2005203
94. Wu TH, Chang CC, Yang CH, Lin WY, Ee TJ, Lin CW. Hybridization chain reactions targeting the severe acute respiratory syndrome coronavirus 2 (SARS-CoV-2). Int J Mol Sci. (2020) 21:3216. doi: 10.3390/ijms21093216
95. Chang CK, Sue SC, Yu TH, Hsieh CM, Tsai CK, Chiang YC, et al. Modular organization of SARS coronavirus nucleocapsid protein. J Biomed Sci. (2006) 13:59–72. doi: 10.1007/s11373-005-9035-9
96. Popp R, Schimmer O. Induction of sister-chromatid exchanges (SCE), polyploidy, and micronuclei by plant flavonoids in human lymphocyte cultures. A comparative study of 19 flavonoids. Mutat Res. (1991) 246:205–13. doi: 10.1016/0027-5107(91)90123-6
97. Yao X, Ye F, Zhang M, Cui C, Huang B, Niu P, et al. In vitro antiviral activity and projection of optimized dosing design of hydroxychloroquine for the treatment of severe acute respiratory syndrome coronavirus 2 (SARS-CoV-2). Clin Infect Dis. (2020) 71:732–9. doi: 10.1093/cid/ciaa237
98. Chung M, Bernheim A, Mei X, Zhang N, Huang M, Zeng X, et al. CT imaging features of 2019 novel coronavirus (2019-nCoV). Radiology. (2020) 295:202–7. doi: 10.1148/radiol.2020200230
99. Hindson J. COVID-19: faecal-oral transmission? Nat Rev Gastroenterol Hepatol. (2020) 17:259. doi: 10.1038/s41575-020-0295-7
100. Xu Y, Li X, Zhu B, Liang H, Fang C, Gong Y, et al. Characteristics of pediatric SARS-CoV-2 infection and potential evidence for persistent fecal viral shedding. Nat Med. (2020) 26:502–5. doi: 10.1038/s41591-020-0817-4
101. Hamid S, Mir MY, Rohela GK. Novel coronavirus disease (COVID-19): a pandemic (epidemiology, pathogenesis and potential therapeutics). New Microbes New Infect. (2020) 35:100679. doi: 10.1016/j.nmni.2020.100679
102. Monteleone PA, Nakano M, Lazar V, Gomes AP, de HM, Bonetti TC. A review of initial data on pregnancy during the COVID-19 outbreak: implications for assisted reproductive treatments. JBRA Assist Reprod. (2020) 24:219–25. doi: 10.5935/1518-0557.20200030
103. Morray BH, Gordon BM, Crystal MA, Goldstein BH, Qureshi AM, Torres AJ, et al. Resource allocation and decision making for pediatric and congenital cardiac catheterization during the novel coronavirus SARS-CoV-2 (COVID-19) pandemic: a U.S. multi-institutional perspective. J Invasive Cardiol. (2020) 32:E103–9.
104. Tibirica E, De Lorenzo A. Increased severity of COVID-19 in people with obesity: are we overlooking plausible biological mechanisms? Obesity. (2020) 28:1374. doi: 10.1002/oby.22887
105. Kelly ME, Murphy E, Bolger JC, Cahill RA. COVID-19 and the treatment of acute appendicitis in Ireland: a new era or short-term pivot? Colorectal Dis. (2020) 22:648–9. doi: 10.1111/codi.15141
106. Decaro N, Lorusso A. Novel human coronavirus (SARS-CoV-2): a lesson from animal coronaviruses. Vet Microbiol. (2020) 244:108693. doi: 10.1016/j.vetmic.2020.108693
107. Bialvaei AZ, Kafil HS, Asgharzadeh M. Role of treatment cost on transmission of multidrug-resistant tuberculosis into Iran. Clin Infect Dis. (2015) 61:1029–30. doi: 10.1093/cid/civ459
108. Ifdil I, Fadli RP, Suranata K, Zola N, Ardi Z. Online mental health services in Indonesia during the COVID-19 outbreak. Asian J Psychiatr. (2020) 51:102153. doi: 10.1016/j.ajp.2020.102153
109. Loke KSH, Tham WY, Bharadwaj P, Keng FYJ, Huang Z, Idu MB, et al. Adapting to a novel disruptive threat: nuclear cardiology service in the time of the coronavirus (COVID-19) Outbreak 2020 (SARS REBOOT). J Nucl Cardiol. (2020) 27:1005–9. doi: 10.1007/s12350-020-02117-0
110. Uddin M, Mustafa F, Rizvi TA, Loney T, Suwaidi HA, Al-Marzouqi AHH, et al. SARS-CoV-2/COVID-19: viral genomics, epidemiology, vaccines, therapeutic interventions. Viruses. (2020) 12:526. doi: 10.3390/v12050526
111. Warren TK, Jordan R, Lo MK, Ray AS, Mackman RL, Soloveva V, et al. Therapeutic efficacy of the small molecule GS-5734 against ebola virus in rhesus monkeys. Nature. (2016) 531:381–5. doi: 10.1038/nature17180
112. Mulangu S, Dodd LE, Davey RT Jr, Tshiani Mbaya O, Proschan M, Mukadi D, et al. A randomized, controlled trial of ebola virus disease therapeutics. N Engl J Med. (2019) 381:2293–303. doi: 10.1056/NEJMoa1910993
113. Sheahan TP, Sims AC, Graham RL, Menachery VD, Gralinski LE, Case JB, et al. Broad-spectrum antiviral GS-5734 inhibits both epidemic and zoonotic coronaviruses. Sci Transl Med. (2017) 9:eaal3653. doi: 10.1126/scitranslmed.aal3653
114. Agostini ML, Andres EL, Sims AC, Graham RL, Sheahan TP, Lu X, et al. Coronavirus susceptibility to the antiviral remdesivir (GS-5734) is mediated by the viral polymerase and the proofreading exoribonuclease. mBio. (2018) 9:e00221–18. doi: 10.1128/mBio.00221-18
115. Wang M, Cao R, Zhang L, Yang X, Liu J, Xu M, et al. Remdesivir and chloroquine effectively inhibit the recently emerged novel coronavirus (2019-nCoV) in vitro. Cell Res. (2020) 30:269–71. doi: 10.1038/s41422-020-0282-0
116. Holshue ML, DeBolt C, Lindquist S, Lofy KH, Wiesman J, Bruce H, et al. First case of 2019 novel coronavirus in the United States. N Engl J Med. (2020) 382:929–36. doi: 10.1056/NEJMoa2001191
117. Wang L, Wang Y, Ye D, Liu Q. Review of the 2019 novel coronavirus (SARS-CoV-2) based on current evidence. Int J Antimicrob Agents. (2020) 55:105948. doi: 10.1016/j.ijantimicag.2020.105948
118. Furuta Y, Komeno T, Nakamura T. Favipiravir (T-705), a broad spectrum inhibitor of viral RNA polymerase. Proc Jpn Acad Ser B Phys Biol Sci. (2017) 93:449–63. doi: 10.2183/pjab.93.027
119. Monteil V, Kwon H, Prado P, Hagelkruys A, Wimmer RA, Stahl M, et al. Inhibition of SARS-CoV-2 infections in engineered human tissues using clinical-grade soluble human ACE2. Cell. (2020) 181:905–13.e7. doi: 10.1016/j.cell.2020.04.004
120. Khan A, Benthin C, Zeno B, Albertson TE, Boyd J, Christie JD, et al. A pilot clinical trial of recombinant human angiotensin-converting enzyme 2 in acute respiratory distress syndrome. Crit Care. (2017) 21:234. doi: 10.1186/s13054-017-1823-x
121. Kadam RU, Wilson IA. Structural basis of influenza virus fusion inhibition by the antiviral drug arbidol. Proc Natl Acad Sci USA. (2017) 114:206–14. doi: 10.1073/pnas.1617020114
122. Tu YF, Chien CS, Yarmishyn AA, Lin YY, Luo YH, Lin YT, et al. A review of SARS-CoV-2 and the ongoing clinical trials. Int J Mol Sci. (2020) 21:2657. doi: 10.3390/ijms21072657
123. Chen J, Lau YF, Lamirande EW, Paddock CD, Bartlett JH, Zaki SR, et al. Cellular immune responses to severe acute respiratory syndrome coronavirus (SARS-CoV) infection in senescent BALB/c mice: CD4+ T cells are important in control of SARS-CoV infection. J Virol. (2010) 84:1289–301. doi: 10.1128/JVI.01281-09
124. Cinatl J, Morgenstern B, Bauer G, Chandra P, Rabenau H, Doerr HW. Treatment of SARS with human interferons. Lancet. (2003) 362:293–4. doi: 10.1016/S0140-6736(03)13973-6
125. Sheahan TP, Sims AC, Leist SR, Schafer A, Won J, Brown AJ, et al. Comparative therapeutic efficacy of remdesivir and combination lopinavir, ritonavir, and interferon beta against MERS-CoV. Nat Commun. (2020) 11:222. doi: 10.1038/s41467-019-13940-6
126. Tabll AA, Shahein YE, Omran MM, Elnakib MM, Ragheb AA, Amer KE. A review on monoclonal antibodies in COVID-19: Role in immunotherapy, vaccine development and viral detection. Hum Antibodies. (2021) 29:179–91. doi: 10.3233/HAB-200441
127. Wiersinga WJ, Rhodes A, Cheng AC, Peacock SJ, Prescott HC. Pathophysiology, transmission, diagnosis, and treatment of coronavirus disease 2019 (COVID-19): a review. JAMA. (2020) 324:782–93. doi: 10.1001/jama.2020.12839
128. Yan Y, Shin WI, Pang YX, Meng Y, Lai J, You C, et al. The first 75 days of novel coronavirus (SARS-CoV-2) outbreak: recent advances, prevention, and treatment. Int J Environ Res Public Health. (2020) 17:2323. doi: 10.3390/ijerph17072323
129. Shidham VB, Frisch NK, Layfield LJ. Severe acute respiratory syndrome coronavirus 2 (the cause of COVID 19) in different types of clinical specimens and implications for cytopathology specimen: an editorial review with recommendations. Cytojournal. (2020) 17:7. doi: 10.25259/Cytojournal_24_2020
Keywords: COVID-19, SARS-CoV, WHO, MERS-CoV, PHEIC 3
Citation: Zhou H, Yang J, Zhou C, Chen B, Fang H, Chen S, Zhang X, Wang L and Zhang L (2021) A Review of SARS-CoV2: Compared With SARS-CoV and MERS-CoV. Front. Med. 8:628370. doi: 10.3389/fmed.2021.628370
Received: 13 January 2021; Accepted: 05 November 2021;
Published: 07 December 2021.
Edited by:
Walid Alali, Kuwait University, KuwaitReviewed by:
Luiz Ricardo Berbert, Federal University of Rio de Janeiro, BrazilYongsheng Ji, University of Science and Technology of China, China
Copyright © 2021 Zhou, Yang, Zhou, Chen, Fang, Chen, Zhang, Wang and Zhang. This is an open-access article distributed under the terms of the Creative Commons Attribution License (CC BY). The use, distribution or reproduction in other forums is permitted, provided the original author(s) and the copyright owner(s) are credited and that the original publication in this journal is cited, in accordance with accepted academic practice. No use, distribution or reproduction is permitted which does not comply with these terms.
*Correspondence: Linding Wang, wanglinding@ahmu.edu.cn; Lingling Zhang, ll-zhang@hotmail.com
†These authors have contributed equally to this work