- 1Centre National de Référence de la Résistance aux Antibiotiques, CHRU Jean Minjoz, Besançon, France
- 2CNRS, UMR 6249 Chrono-Environnement, Besançon, France
- 3INSERM UMR 1092, Université de Limoges, CHU Limoges, Limoges, France
- 4Département de Microbiologie Clinique, AP-HP, CHU Avicenne, Bobigny, France
A novel carbapenem-hydrolyzing beta-lactamase, called IMP-63, was identified in three clonally distinct strains of Pseudomonas aeruginosa and two strains of Pseudomonas putida isolated within a 4 year timeframe in three French hospitals. The blaIMP–63 gene that encodes this carbapenemase turned out to be located in the variable region of four integrons (In1297, In1574, In1573, and In1572) and to coexist with novel or rare gene cassettes (fosM, gcu170, gcuF1) and insertion elements (ISPsp7v, ISPa16v). All these integrons except one (In1574) were flanked by a copy of insertion sequence ISPa17 next to the orf6 putative gene, and were carried by non-conjugative plasmids (pNECK1, pROUSS1, pROUSS2, pROUE1). These plasmids exhibit unique modular structures and partial sequence homologies with plasmids previously identified in various non-fermenting environmental Gram-negative species. Lines of evidence suggest that ISPa17 promoted en bloc the transposition of IMP-63-encoding integrons on these different plasmids. As demonstrated by genotyping experiments, isolates of P. aeruginosa harboring the 28.9-kb plasmid pNECK1 and belonging to international “high-risk” clone ST308 were responsible for an outbreak in one hospital. Collectively, these data provide an insight into the complex and unpredictable routes of diffusion of some resistance determinants, here blaIMP–63, among Pseudomonas species.
Introduction
The Pseudomonas putida group is composed of several closely related species that live in the soil and surface waters as saprophytes within complex communities (Gomila et al., 2015). Though poorly pathogenic for humans these Gram-negative bacteria can occasionally generate acute or chronic infections in fragile patients. Thus species such as P. putida (sensu stricto), P. monteilii, and P. mosselii are regularly isolated from clinical samples (Yang et al., 1996). Because of the intrinsic susceptibility of these bacteria to many antibiotics and their low virulence, the issue of their resistance to chemotherapy has not been addressed specifically and has not attracted much attention so far. However, the presence of multidrug resistant strains of P. putida (here, used as a generic name for several species or subspecies of the P. putida complex) in the hospital setting is increasingly reported (Raphael and Riley, 2017). As for the well-known human pathogen P. aeruginosa, these species are prone to become resistant to major antibiotic families including aminoglycosides, fluoroquinolones and ß-lactams. More alarming are the observations that some of them produce carbapenem-inactivating ß-lactamases (so-called carbapenemases), and that these enzymes are of the same types as those found in epidemic clones of P. aeruginosa (Oliver et al., 2015; Raphael and Riley, 2017). Indeed metallo-ß-lactamases (MBLs) of VIM- and IMP-types are the most prevalent carbapenemases in carbapenem-resistant P. aeruginosa and P. putida. Their genetic determinants can be borne by the bacterial chromosome or by plasmids (Lee et al., 2002; Docquier et al., 2003; Yomoda et al., 2003; Marchiaro et al., 2010). Given the phylogenetic proximity of fluorescent Pseudomonas, plasmid exchanges are expected to occur freely among these species (Boronin, 1992). However, whether some of these bacteria constitute a reservoir of resistance determinants for the others remain poorly understood (Juan et al., 2010). In this study, we show that the diffusion of a rare variant of MBL IMP-12, named IMP-63, among clinical strains of P. aeruginosa and P. putida occurred through a complex scenario involving several genetic cargos.
Materials and Methods
Bacterial Strains and Microbiology Techniques
Pseudomonas aeruginosa strains Pae1151 to Pae1156, and Pae1133 were collected between November 2011 and July 2012 during an outbreak that occurred in hospital H1, Paris, France. Strain Pae2567 was isolated in the same hospital 2 years later, in 2014. These isolates were found in the following clinical samples: rectal swabs (n = 2), urine (n = 2), tracheal aspirate (n = 1), catheter (n = 1), blood culture (n = 1), and eye swab (n = 1). A strain of P. putida named Pp917 was recovered from the stools of an oncology patient admitted to hospital H2, Paris, in 2012, while a P. aeruginosa called Pae3940 was detected in the same unit of this hospital in 2016, in the rectal swab from another patient. Pseudomonas putida Pp2223 was responsible for a urinary tract infection in a patient hospitalized in hospital H3 (140 km distant from Paris) in 2014. The bacteria were identified at the species level by matrix-assisted laser desorption ionization-time of flight (MALDI-TOF) mass spectrometry using a Bruker MALDI MS equipment. Rifampicin-resistant P. aeruginosa strain PU21 was used in MIC experiments as control and as recipient for plasmid transfers (see below) (Loutit et al., 1968). All the bacterial cultures were performed at 35°C ± 1 on Mueller-Hinton agar medium (MHA, BioRad, Marnes-la-Coquette, France) or in Mueller-Hinton broth (MHB, Becton Dickinson, Microbiology Systems, Cockeysville, MD, United States) containing adjusted concentrations of Mg2+ and Ca2+. MICs of antibiotics were determined in three independent experiments by the microbroth dilution method with Sensititre® microplates containing specifically designed drug panels (ThermoFisher Scientific, Villebon-sur-Yvette, France). Results were recorded after an incubation of 18 h ± 2 and were interpreted according to ongoing EUCAST breakpoints1.
DNA Amplification and Sequencing Methods
Amplification of gene blaIMP–63 was obtained with a PCR targeting the subgroup of IMP beta-lactamases. The 50 μL reaction mixture contained 3 μL DNA extract, 0.06 μM forward primer IMP2010-F (5′-GTTTATGTTCATACWTCGTT-3′) and reverse primer IMP2010-R1 (5′-GCCAAGCTTCTAAATTTGC-3′), 10 μL MyTaq Red Mix (Bioline, France), 1.25 U MyTaq Red DNA Polymerase (Bioline), 3 μL DMSO and 27.75 μL distilled water. After an initial denaturation step of 5 min at 94°C, the gene was amplified through 30 cycles each consisting in 1 min at 94°C, 1 min hybridization at 51°C, followed by an extension of 1 min at 72°C. A final extension of 7 min at 72°C ended the reaction. Genes encoding IMP enzymes are frequently located into class I integrons. Complete nucleotide sequence of blaIMP–63 was obtained by PCR and sequencing on both strands with specific primers Hep58 (5′-TCATGGCTTGTTATGACTGT) and Hep59 (5′-GTAGGGCTTATTATGCAGGC), annealing to 5′-CS and 3′-CS conserved sequences of class I integrons, respectively (White et al., 2000).
The whole DNA content of strains Pae1156, Pae2567, Pp917, Pae3940, PU21(pTROUS1), and Pp2223 was sequenced with the Illumina technology. Briefly, total bacterial DNA was extracted from overnight cultures by using PureLink Genomic DNA Mini Kit (ThermoFisher Scientific), then was quantified with a NanoDrop spectrophotometer (Ozyme, Montigny le Bretonneux, France), and finally sequenced by Microsynth AG (Balgach, Switzerland) in an Illumina NextSeq sequencer with v2 chemistry, using 2 × 150 paired-end reads. DNA libraries were prepared with Nextera XT DNA Library Preparation Kit (Illumina, San Diego, CA, United States). High throughput sequencing yielded from 6039419 to 6690442 reads per strain, with a coverage depth comprised between 80 and 150×. Antimicrobial resistance genes were identified by uploading the resultant reads to ResFinder 3.1 program available at the Center for Genomic Epidemiology server2. De novo assembly of paired-end fragments, search of open reading frames (ORFs), and gene annotation were performed by using Basic Local Alignment Search Tool BLASTn3 and CLC genomics Workbench 10.0.1 software (Qiagen Bioinformatics, Redwood city, CA, United States). Schematic representation of integrons (Figure 2) was drawn with SnapGene4. To establish the plasmid pNECK1 backbone, longer contigs were generated from strain Pae1156 by PacBio SMRT sequencing (CD Genomics, Shirley, NY, United States). The IMP-63-encoding plasmids have been deposited in the NCBI database under the accession numbers MK047609 (pNECK1), MK047610 (pTROUS1), MK047611 (pTROUS2), and MK047608 (pROUE1).
Strain Typing
The sequence types (STs) of P. aeruginosa isolates were determined according to the scheme proposed by van Mansfeld et al. (2009). Internal sequences of housekeeping genes acsA, aroE, guaA, mutL, nuoD, ppsA, and trpE, as determined by whole genome sequencing or specific PCRs (van Mansfeld et al., 2009), were uploaded to MLST web site5 to define STs. In addition, the clonality of the strains involved in hospital H1 outbreak was investigated by MLVA (Multiple-Locus Variable-Number Tandem-Repeat Analysis) targeting microsatellites ms142, ms211, ms212, ms213, ms214, ms215, ms216, ms217, ms222, and ms223 (Vu-Thien et al., 2007). The size of individual amplicons was determined for each strain by agarose gel electrophoresis. The banding patterns of strains Pae1151 to Pae1156 were strictly identical, but different from that of the late isolate Pa2567 (data not shown). When mentioned in the text, strains of P. aeruginosa were serotyped by glass agglutination with O-antiserums from BioRad, according to the Habbs scheme.
Gene Transfer Experiments and Plasmid Characterization
Plasmid transfers between the IMP-63-producing strains and rifampicin-resistant P. aeruginosa PU21 were attempted. Briefly, 100 μL of log-phase donor and recipient cultures in MHB were mixed and deposited on a 0.45 μm pore size nitrocellulose membrane at the surface of a MHA plate. After 3 h at 35°C ± 1, the bacteria were resuspended in MHB and then cultured overnight on MHA plates containing 150 μg/mL ticarcillin and 200 μg/mL rifampicin to counterselect PU21 transconjugants. When present, resistant colonies were subsequently streaked onto the same selective medium and submitted to plasmid extraction by the alkaline lysis method of Kieser (1984). The transfer of plasmid pNECK1 was visually confirmed by 0.8% agarose gel electrophoresis and ethidium bromide staining. The four plasmids from E. coli 39R861 (also named NCTC 50192) were used as calibrators to estimate plasmid size. Transfer of plasmid pTROUS2 from Pa3940 to PU21 was obtained by electro-transformation according to the protocol of Smith and Iglewski (1989).
Results
Outbreak Strains of P. aeruginosa Producing IMP-63 in Hospital H1, Paris
Seven multidrug resistant strains of P. aeruginosa isolated in hospital H1, Paris, between November 2011 and July 2012 were referred to the French National Reference Center for Antibiotic Resistance (NRC-AR, Besançon) for characterization of their resistance mechanisms to ß-lactams. These outbreak isolates (Pae1133, and from Pae1151 to Pae1156) were found to belong to serotype O:11 by slide agglutination and to display the same drug susceptibility profile. According to the EUCAST breakpoints, they were resistant or intermediate to all the ß-lactams and aminoglycosides tested, but remained susceptible to ciprofloxacin and colistin (see isolate Pae1156 in Table 1). They shared the same genotype ST308 and MLVA profile (data not shown). All of them yielded a positive double-disk synergy test between Zn2+ chelator EDTA and imipenem, suggesting the acquisition of a metallo-beta-lactamase (MBL). Use of a PCR targeting known transferable MBL-encoding genes led to the identification of blaIMP–63, which codes for a new variant of IMP-12. This latter carbapenemase was detected in an Italian strain of P. putida in year 2000 (Docquier et al., 2003), and to the best of our knowledge has never been reported since then in the literature. IMP-63 (accession number AOR06148) differs from IMP-12 by a Gly-to-Ser substitution at position 214 and is relatively distant from other IMP-type enzymes, with the closest relatives being IMP-8 and IMP-2 (87.3 and 86.9% sequence identity, respectively). The phylogeny of IMP proteins including IMP-63 is represented Figure 1. The gene blaIMP–63 sequence was deposited in GenBank under the accession number KX821663.1. Interestingly, additional investigations showed that gene oprD which codes for the carbapenem-specific porin OprD in P. aeruginosa was disrupted by a new 1,333-bp IS-like element in Pae1156. This finding supports the notion that both enzymatic and membrane impermeability mechanisms contributed to the carbapenem resistance of H1 outbreak strains.
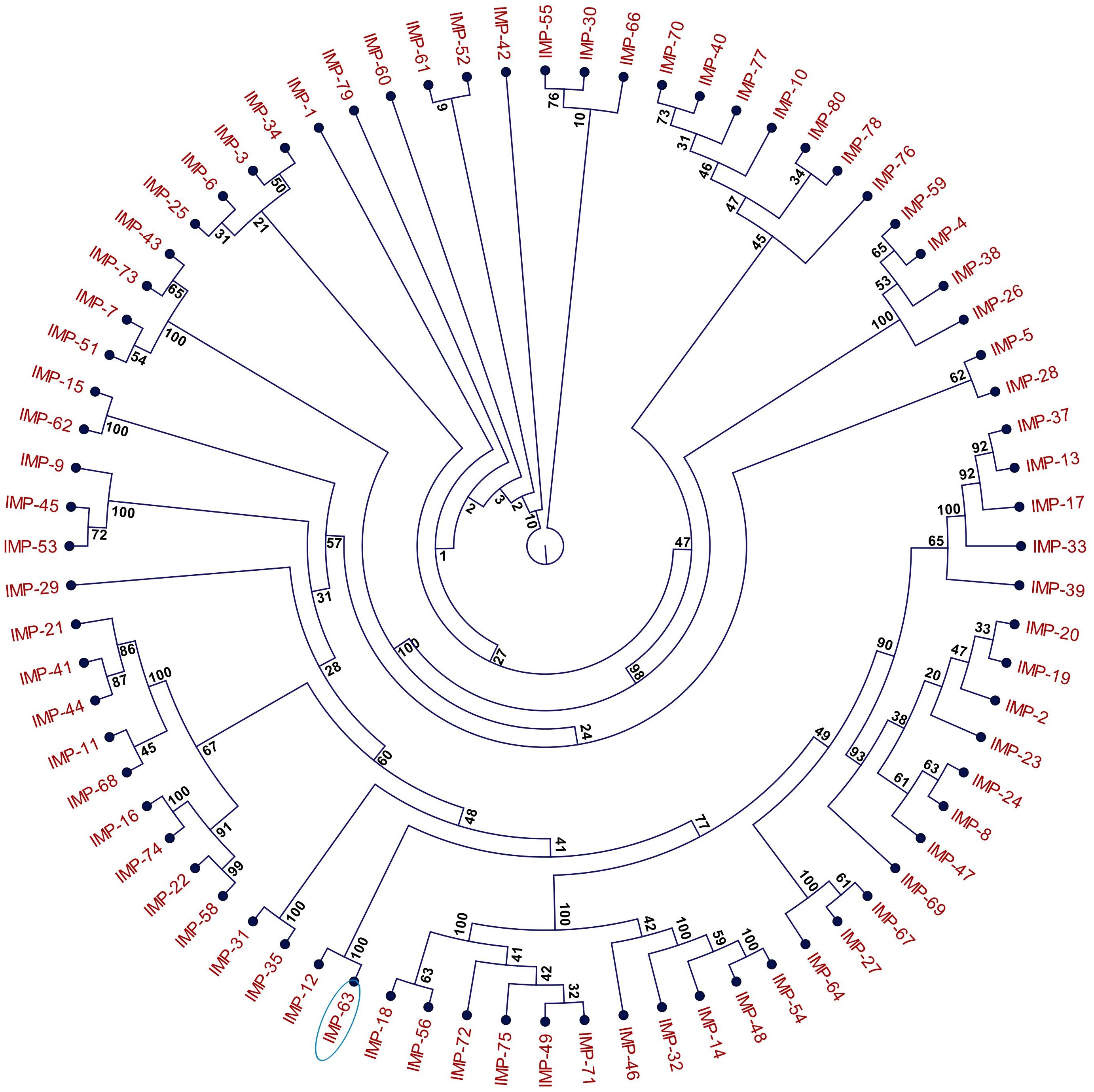
Figure 1. Phylogeny of IMP-type metallo-ß-lactamases (MBLs). The cladogram is based on the amino acid sequences of 76 IMP-type MBLs deposited in the NCBI database (https://www.ncbi.nlm.nih.gov). Construction of the cladogram was performed using the Maximum Likelihood method with 100 replicates. Bootstrap values are indicated next to each branch. In this representation, the length of the branches is irrelevant of evolutionary distance. Enzyme IMP-63 is circled.
Genetic Environment of blaIMP–63 in P. aeruginosa Pae1156
Most blaIMP genes are carried by class I integrons6. Whole genome sequencing of strain Pae1156 confirmed the presence of blaIMP–63 in the variable region of a novel 6-kb integron, dubbed In1297. This integron which contains a unique array of gene cassettes and a truncated integrase gene intI lacking 110-bp at its 3′ end, is interrupted upstream of canonical qacEΔ1 and sul1 genes by a sequence encoding a Thr63Ala, Arg91His, Gly229Ser variant of insertion sequence ISPsp7 (named ISPsp7v in Figure 2). The ISPsp7 element which belongs to the IS30 family was described in the environmental Pseudomonas sp. strain ZM2 recovered in postflotation tailings samples from Poland (Szuplewska et al., 2014). Immediately downstream of the intI truncated sequence (here named intIΔ1), fosM codes for a new fosfomycin resistance determinant sharing 63% amino acid sequence identity with FosI, and that likely accounts for the high fosfomycin MICs of H1 isolates (>1,024 μg/mL). The function of the second cassette, gcu170, remains unknown as those of other gcu genes. Located at the third position of the array, blaIMP–63 is followed by the aminoglycoside-modification gene aac(6′)-Ib′, that is predicted to confer a high resistance to gentamicin, tobramycin and netilmicin, and comparatively low resistance to amikacin. Indeed, the Gln101-Ser102-Leu103-Ala104 motif within the active site of AAC(6′)-Ib enzymes results in an amikacin-to-gentamicin switch in resistance, as compared to the Gln/Ser101-Leu102-Leu103-Ala/Ser104 motif (Rather et al., 1992; Casin et al., 2003). Next in the list of In1297-borne cassettes, gene blaOXA–19 is the determinant of an extended-spectrum variant of oxacillinase OXA-35 harboring a Gly167Asp mutation (DBL numbering) (Mugnier et al., 1998). OXA-19 efficiently hydrolyzes newer cephalosporins including ceftazidime, cefepime and ceftolozane (Mugnier et al., 1998). In contrast to fosM, gcu170, and blaIMP–63 which, to our knowledge, have never been reported in P. aeruginosa before, cassettes aac(6′)-Ib′ and blaOXA–19 are rather common in multidrug resistant strains in France (Hocquet et al., 2010).
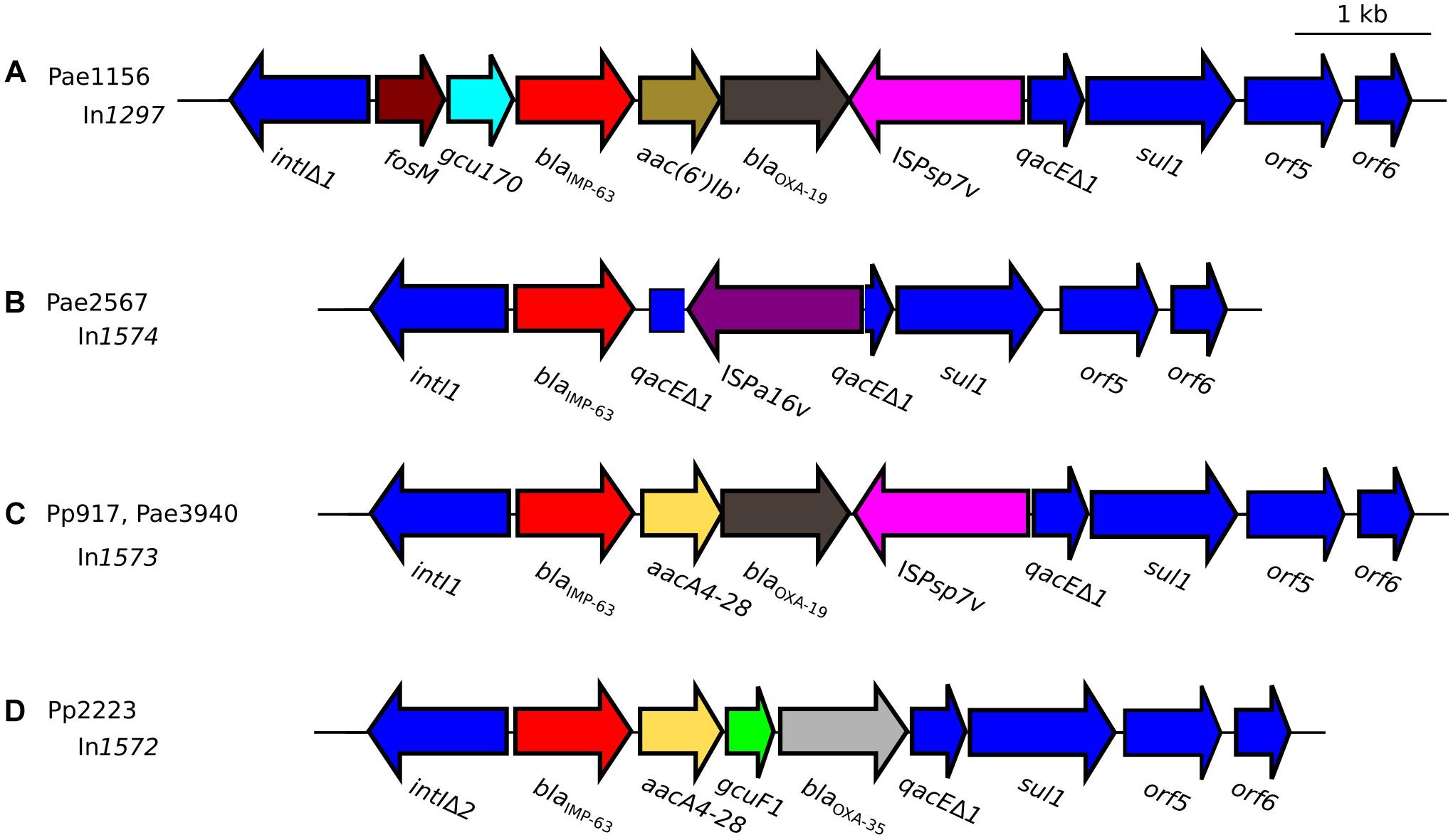
Figure 2. Schematic representation of blaIMP–63-carrying integrons detected in strains of (A) P. aeruginosa Pae1156 isolated in Paris, in 2012 during the hospital H1 outbreak; (B) P. aeruginosa Pae2567 isolated in hospital H1, in 2014; (C) P. putida Pp917 and P. aeruginosa Pae3940 isolated in hospital H2, Paris, in 2012 and 2016, respectively; (D) P. putida Pp2223 isolated in hospital H3, Rouen, in 2014. Genes that form the conserved backbone of these integrons are represented as deep-blue arrows. Gene blaIMP–63 is highlighted in red, while the other cassettes present in the variable regions of the integrons are represented with various colors because of their nucleotide sequence diversity.
Plasmid pNECK1
Analysis of the plasmid content of strain Pae1156 by agarose gel electrophoresis after extraction by the alkaline lysis method, showed the presence of three bands of ca 30-kb, 60-kb and >200-kb, respectively (Figure 3). Mating-out experiments with rifampicin-resistant P. aeruginosa PU21 allowed the transfer at very low frequency (ca 10−7 per donor cell) of the 30-kb plasmid that eventually proved to carry the whole In1297 sequence (see below). The resistance profile of selected transconjugants was similar to that of Pae1156 except for carbapenem MICs, that appeared to be four to eightfold lower for the recipients than for the donor strain [compare PU21(pNECK1) and Pae1156 in Table 1]. These data reinforce the notion that in Pae1156 the activity of carbapenemase IMP-63 is strongly potentiated by the reduced permeability of the outer membrane to carbapenems. The transferred plasmid, dubbed pNECK1, was completely sequenced and assembled in a single contig of 28,859-bp (Figure 4). Its GC content was equal to 63.21%. Search in databases revealed that the replicon is related, though distantly, to several broad-host range IncP plasmids such as pNOR-2000 and pAX22 (Bonnin et al., 2013; Di Pilato et al., 2014). In these plasmids, the defective Tn402-like integron platform is flanked at its 5′ end, immediately downstream of integrase gene intl1, by a copy of insertion sequence ISPa17 and a divergently transcribed resolvase gene (Figure 5). The same module exists in pNECK1 though inserted at the 3′ end of In1297, next to a gene of unknown function, orf6 (Figure 4). As noted elsewhere, ISPa17 exhibits an atypical insertion sequence structure as it contains the genes coding for two putative transposases and a type II toxin-antitoxin system. Its presence has been detected on IncP-1 plasmids harbored by various environmental bacterial species (Heuer et al., 2012). ISPa17 is bounded by 25-bp inverted repeats (IRL and IRR) whose sequence is close and identical, respectively, to IRi and IRt of Tn402-like transposons. While neither ΔTn402::In1297 nor ISPa17 is flanked by direct repeats (DRs) in pNECK1, the same 5′-TATAG sequence is adjacent to IRR of ISPa17 and to IRi of ΔTn402::In1297 (Figure 5). This sequence signature suggests that both ISPa17 and ΔTn402::In1297 have been acquired en bloc by plasmid pNECK1 through a single transposition event, possibly driven by the ISPa17 transposases. A similar scenario based on the use IRL and IRt sites instead of IRL and IRR by ISPa17 transposases has been proposed for acquisition of the ISPa17-ΔTn402::In70 module by plasmid pAX22 (Di Pilato et al., 2014).
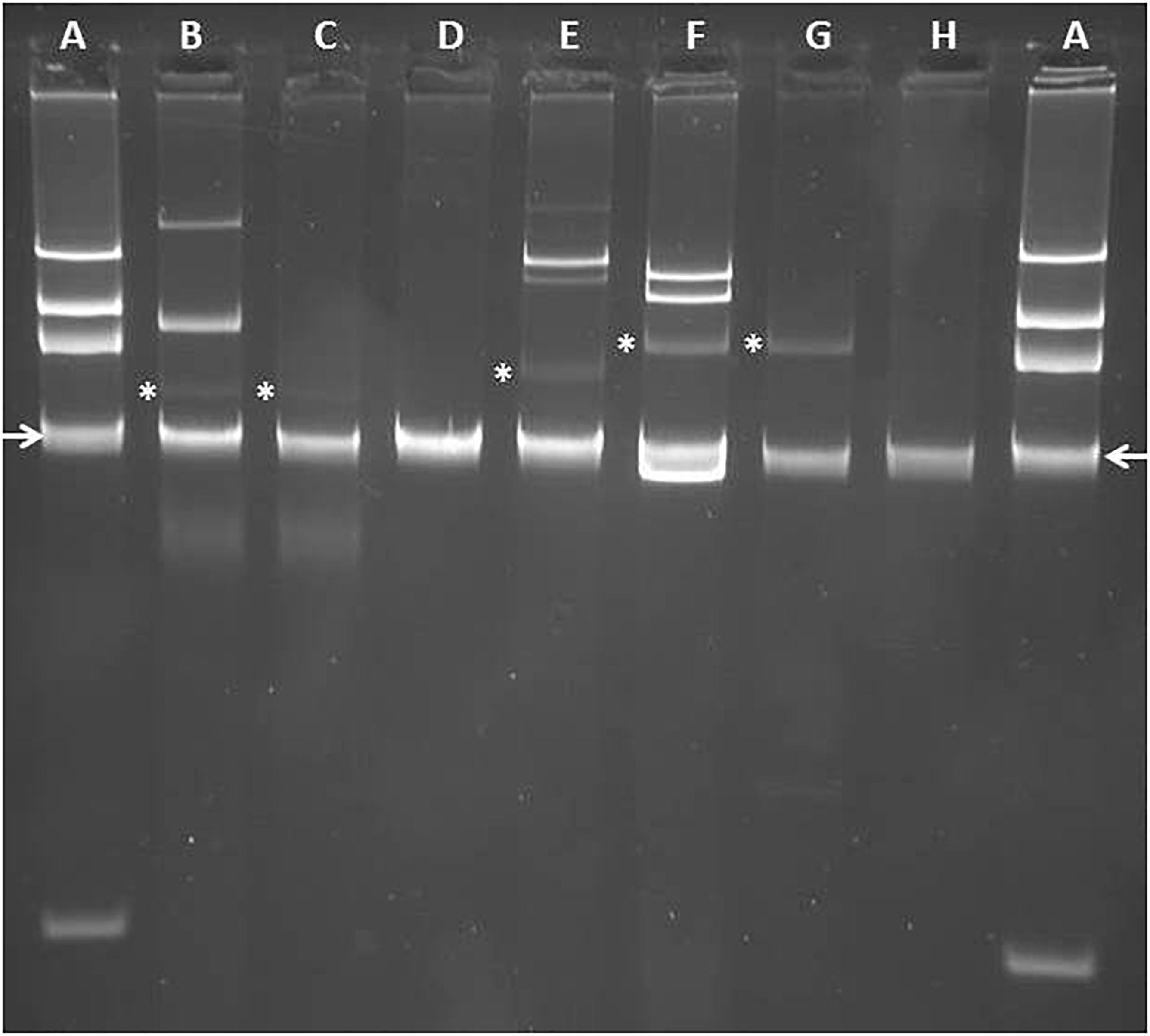
Figure 3. Analysis of plasmid content of IMP-63 positive strains by agarose gel electrophoresis. Plasmids were extracted by using the method of Kieser (1984) and separated by electrophoresis in a 0.8% agarose gel. (A) E. coli strain 39R861 (NCTC 50192) harboring plasmids of ca 154-, 66-, 38-, and 7-kb, respectively, used as size standards for gel calibration; (B) P. aeruginosa strain Pae1156 harboring plasmid pNECK1 (marked with an asterisk); (C) P. aeruginosa strain PU21 transformed with pNECK1; (D) P. aeruginosa strain Pae2567; (E) P. putida strain Pp917 harboring plasmid pTROUS2 (marked with an asterisk); (F) P. aeruginosa strain Pae3940 harboring plasmid pTROUS1 (marked with an asterisk); (G) PU21 transformed with pTROUS1; (H) P. putida strain Pp2223. Location of DNA band corresponding to linear chromosomal fragments is indicated by arrows.
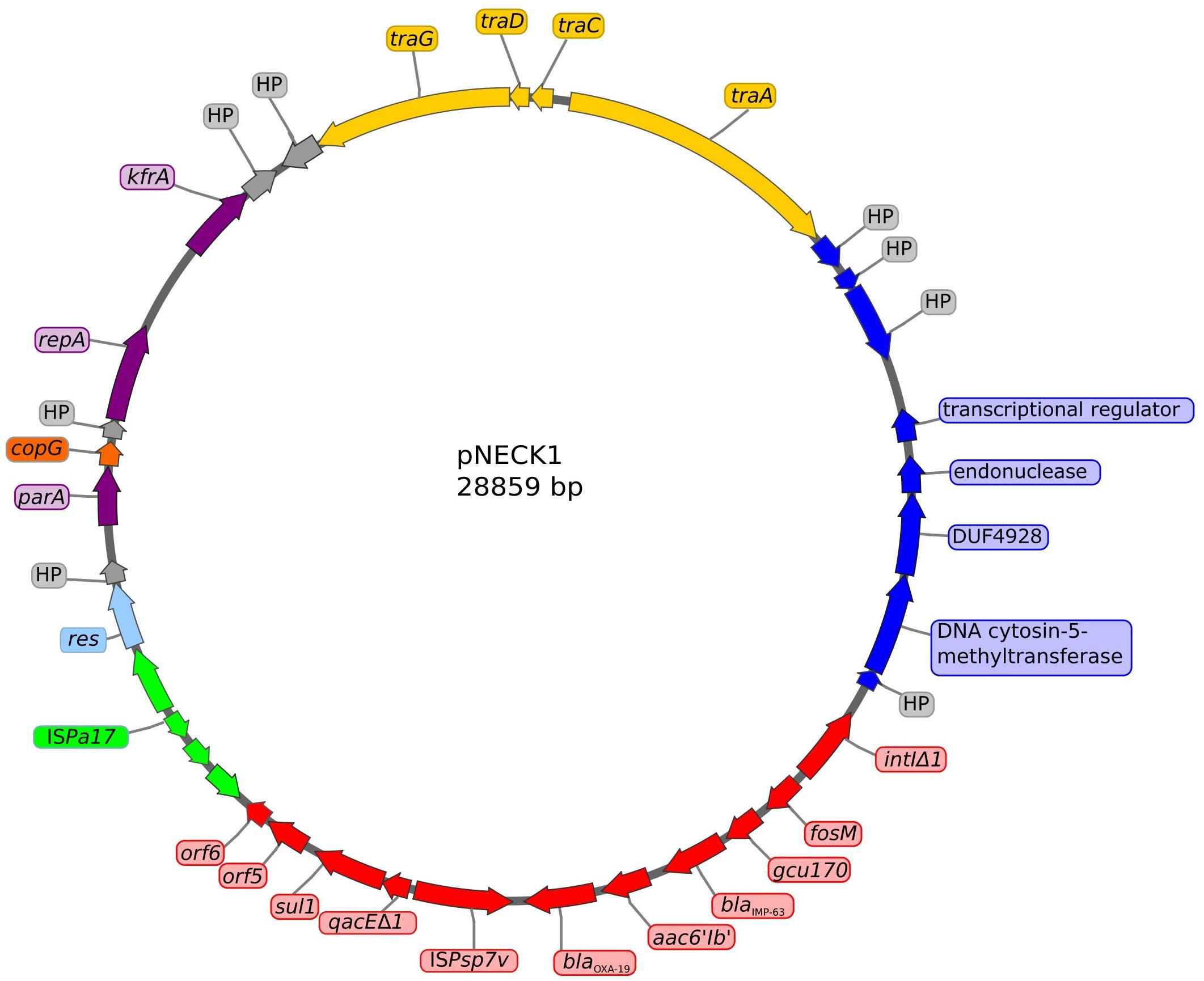
Figure 4. Structure of plasmid pNECK1 harbored by P. aeruginosa strain Pae1156. The GC content of this replicon is equal to 63.21%. Arrows in purple color represent genes involved in plasmid housekeeping functions such as partition, replication, and stability. The integron In1297 structure is indicated in red color, flanked with a copy of insertion sequence ISPa17 in green. Genes involved in plasmid transfer are indicated in yellow. Dark-blue arrows correspond to a set of genes previously found in a Burkholderia cenocepacia plasmid. Genes of unknown function are shown in gray.
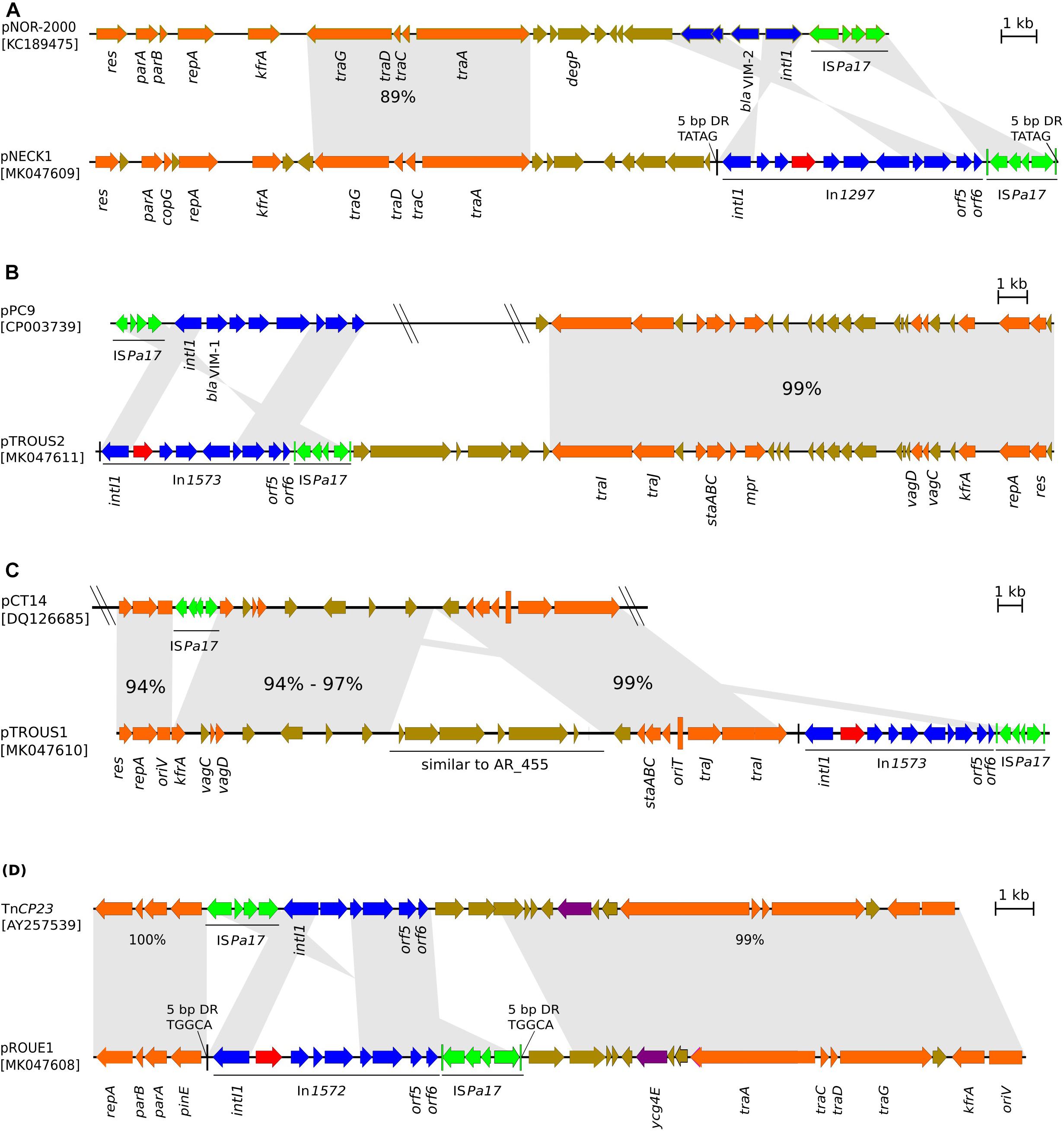
Figure 5. Sequence alignment of the four blaIMP–63-carrying genetic structures, with structurally closest plasmids. (A) Comparison of plasmid pNECK1 with pNOR-2000; (B) pTROUS2 with pPC9; (C) pTROUS1 with pCT14; (D) pROUE1 with TnCP23. Arrows show the direction of transcription. Arrows in orange color represent genes involved in plasmid transfer and housekeeping functions such as replication, partition and stability. DNA regions in plasmid pairs that were found homologous by BLASTn comparison, are connected by gray stretches that take into account gene orientations; the percent nucleotide sequence identity is indicated for relevant homology regions. Genes of integrons are shown in deep-blue color, except cassette blaIMP–63 highlighted in red. Arrows corresponding to genes of unknown function and ISPa17 are colored in brown and in green, respectively. IRR of ISPa17 and IRi of Tn402-like transposon are mapped and represented by vertical lines in green and black colors, respectively.
Annotation analysis also revealed that most pNECK1 backbone genes involved in fundamental functions such as plasmid partioning (parA), replication (repA), maintenance (kfrA), and transfer (traA, traC, traD, traG) have homologs and a similar linear arrangement in various plasmids including pNOR-2000 and pAX22 (Figure 5). However, the closest matches were obtained with DNA contigs from a Sphingomonas histidinilytica strain called UM2 (accession number FUYM01000026) and a Serratia marcescens strain, AR_0027 (accession number CP0267703). While pNECK1 lacks the parB gene present in pNOR-2000 and pAX22, it contains a copG-like gene possibly involved in plasmid replication control (Brantl, 2015), as well as several other ORFs of unknown functions.
Interestingly, pNECK1 shares a 5,376-bp fragment with an unnamed plasmid detected in a cystic fibrosis strain of Burkholderia cenocepacia isolated in Canada (GenBank accession number CP019671.1) (Lee et al., 2017). Among the 8 ORFs borne by this fragment in pNECK1 (represented as blue arrows in Figure 4), three are predicted to encode a DNA cytosin-5-methyltransferase, a peptide related to AMP-binding superfamily, and a DNA mismatch endonuclease of very short patch repairs, respectively. All these DNA restriction/modification proteins have amino acid sequences from 96 to 99% identical to their counterparts from B. cenocepacia. Closely related homologs are also present in S. histidinilytica UM2 and S. marcescens AR_0027 (data not shown). Their functions are unclear but could potentially insure inter-species propagation of plasmids.
A Late IMP-63 Positive P. aeruginosa Strain in Hospital H1
Isolated in hospital H1 2 years after the outbreak strains, serotype O:11 strain Pae2567 belongs to ST1284 and thereby is clonally different from previous H1 isolates. The bacterium exhibited a high resistance to ceftazidime and cefepime associated with an almost wild-type susceptibility to other ß-lactams including carbapenems (Table 1). The origin of this very unusual susceptibility profile -especially to labile penicillins ticarcillin and piperacillin, remains unclear. However, a double-disk synergy test with EDTA and imipenem confirmed production of a metallo-ß-lactamase by this isolate (Supplemental Figure S1). BLASTn analysis of its total DNA at the NCBI website did not allow the detection of plasmidic elements or ISPa17, a result consistent with the absence of visible plasmid band by agarose gel electrophoresis after cell alkaline lysis (Kieser, 1984; Figure 3). Gene blaIMP–63 was the unique resistance cassette of a novel class I integron, named In1574, that in contrast to In1297, contains a full length integrase gene intl. The weak promoter variant PcW that was identified within the intl coding sequence is known to be associated with a low expression and high rates of excision of resistance gene cassettes (Jové et al., 2010), and could at least partly account for the atypical drug susceptibility pattern described above. Of note, a variant of insertion ISPa16 disrupts the qacEΔ1 sequence in In1574. The location of gene blaIMP–63 in Pae2567 was not investigated further. Its transfer to PU21 could not obtained either by conjugation or electro-transformation.
IMP-63 Positive P. aeruginosa and P. putida Strains in Hospital H2, Paris
A multidrug-resistant IMP-63-producing strain of P. putida, named Pp917, was identified in another Parisian hospital, H2, in 2012. Its resistance profile to antibiotics is indicated in Table 1. Sequencing of its whole DNA content revealed the presence of a novel integron structure carrying the blaIMP–63 gene (Figure 2). The cassette array of In1573 resembles those of In1297 as it sequentially contains gene cassettes blaIMP–63, aacA4-28, and blaOXA–19 followed by a divergently oriented copy of ISPsp7v (i.e., the same variant as in In1297). However, the AAC(6′)-Ib modifying enzyme encoded by aacA4-28 differs from that determined by the aac(6′)Ib′ gene of In1297, by the presence of Asn83 and Leu102 residues. As mentioned previously, Leu102 provides AAC(6′)-Ib enzymes with a higher catalytic activity on amikacin than on gentamicin as compared with the Ser102 variants (Rather et al., 1992). Also, while In1297 contains a truncated intl gene, this gene has a full-length sequence of 1,014-bp in In1573. The plasmid content of strain Pp917 was analyzed by agarose gel electrophoresis. Four plasmids of ca. 35-kb, 100-kb, 150-kb, and >200-kbp, respectively, were detected in this bacterium (Figure 3), but transfer of ticarcillin resistance to recipient P. aeruginosa PU21 could not be obtained either by conjugation or by electro-transformation. Integron In1573 was localized by whole DNA sequencing and multi-alignment experiments on the ca 35-kb plasmid. This plasmid of 34,948-bp and 59.29% GC, named pTROUS2, contains 45 ORFs including backbone genes sharing ≥99% sequence identity with homologs present on a 80,360-bp plasmid (called pPC9) previously found in another P. putida strain isolated in the East of France (Molina et al., 2014; Figure 5). Plasmid pPC9 carries an integron encoding the metallo-ß-lactamase VIM-1 and a copy of ISPa17 adjacent to the intl gene. A copy of ISPa17 is also present on pTROUS2 but, as on plasmid pNECK1, this element is located next to orf6.
A resistant strain of P. aeruginosa, Pae3940, isolated in the same hospital H2 4 years later, in 2016, was also found to produce carbapenemase IMP-63 (Table 1). Its sequence type, ST446, was different from that of the H1 isolates (ST308 and ST1284, respectively). Analysis of its DNA content by high throughput sequencing and subsequent contig assembly revealed the presence of a plasmid of 42,035-bp and 56.43% GC, named pTROUS1. This replicon is composed of 39 ORFs and exhibits similarities with plasmid pCT14 from Pseudomonas veronii CT14, a bacterium isolated in the United States from activated sludge (Bramucci et al., 2006; Figure 5). The two replicons share a sequence identity from 94 to 99% over the backbone genes. Plasmid pCT14 which consists of 51 ORFs and is 55,216 nucleotide long, does not harbor integron-like structures but possesses mercury resistance determinants and a copy of ISPa17. More importantly pTROUS1 carries the same integron In1573 as in plasmid pTROUS2, flanked by a copy of ISPa17 next to orf6. No DRs adjacent to IRR of ISPa17 and to IRi of ΔTn402::In1573 could be identified in pTROUS1 and pTROUS2. It is important to mention that these two plasmids differ in their structures and do not derive from each other (data not shown). While the transfer of pTROUS1 to PU21 could not be obtained by conjugational mating, this was possible by electro-transformation. As indicated in Table 1, the susceptibility profile of transformant PU21(pTROUS1) was fairly similar to that of PU21(pNECK1). In strain Pae3940, pTROUS1 coexists with three other plasmids of ca 18-, 80-, and 100-kb, respectively, whose contribution to antibiotic resistance remains unknown (Figure 3).
IMP-63 Positive P. putida Strain in Hospital H3
With the same methods applied to characterize the other IMP-63-producing strains, a drug resistant strain of P. putida, dubbed Pp2223, was identified in 2014 in a third hospital, H3, located 140 km from Paris (Table 1). This bacterium appeared to be genotypically unrelated to P. putida Pp917 from hospital H2 when compared by MLST7. The novel integron (In1572) present in that strain contained a mutation in the intl gene resulting in the synthesis of a predicted peptide of 190 amino acids in length instead of the 337 residues of wild-type integrase (Figure 2). This intl allele, here called intlΔ2, was different from that of integron In1297, previously found to encode a 290 amino acid peptide. It should be noted that both mutated forms of the integrase are non-functional since they lack the Tyr312 catalytic site. Analysis of the aac(6′)Ib gene located downstream of blaIMP–63 in In1572 showed that this cassette, aacA4-28, determines the same AAC(6′)-Ib variant (Asn83, Leu102) as that of In1573 from Pp917. The next gene present in the array, gcuF1, was initially identified upstream of gene blaOXA–28 in the variable region of an integron, In442, carried by a P. aeruginosa strain from France (Hocquet et al., 2012). Interestingly, extended-spectrum ß-lactamase OXA-28 is a single point variant (Trp164Gly, DBL numbering) of OXA-35, an enzyme able to hydrolyze penicillins, aztreonam and cefepime (Aubert et al., 2001; Poirel et al., 2001). Since transcription of blaOXA–28 is negatively influenced by cassette gcuF1 in In442, the same may potentially apply to blaOXA–35 in In1572 (Hocquet et al., 2012). The occurrence of gcuF1 in class I integrons is quite rare, which makes this determinant a good marker for epidemiological studies when present in clinical strains (see text footnote 6). The products of gcuF1 (strain Pp917) and gcu170 (strain Pae1156) have little in common at the sequence level. Alignment of the DNA reads obtained by high throughput sequencing with sequences from the NCBI database allowed us to gain insight into the genetic support and environment of Pp2223 integron. This element is located on a TnCP23-like structure of 25,062-bp and 61.41% GC, distinct from pNECK1 (Figure 5). The backbone genes of this fragment (32 ORFs in total), that was named pROUE1, display sequence identities of 99–100% with their TnCP23 homologs. In some clone C P. aeruginosa, plasmid TnCP23 is embedded in an integrative conjugative element (ICE) called pKLC102, itself inserted in the bacterial chromosome, in a tRNALys gene (Klockgether et al., 2004). However, no pKLC102 specific genes were detected in Pp2223. Given that no plasmid band was visible by agarose gel electrophoresis (Figure 3), the cellular location of pROUE1 remains uncertain but is most likely chromosomal. Attempts to fill the gap between the 5′- and 3′ends of the pROUE1 fragment by long range PCRs failed. Furthermore, transformation and bacterial conjugation experiments even in the presence of helper plasmid pRK2013 (Ditta et al., 1981) were unsuccessful. Like pNECK1, pTROUS1, and pTROUS2 (and unlike TnCP23, pNOR-2000 and pAX22), plasmid pROUE1 carries a copy of insertion sequence ISPa17 next to orf6, at the 3′ terminus of integron In1572 (Figure 5). The observation that IRR of ISPa17 and IRi of this Tn402-like integron are flanked by a same 5′-TGCCA sequence, reinforces the notion that both ISPa17 and the ΔTn402::In1572 element have transposed simultaneously on pROUE1.
Discussion
Since the first description of carbapenemase IMP-1 in a Serratia marcescens strain from Japan in 1991 (Osano et al., 1994), reports on the characterization of novel IMP-type ß-lactamases in various bacterial species including P. aeruginosa have multiplied worldwide. Despite rather similar Zinc-dependent enzymatic activities, these proteins form a heterogeneous group at the sequence level (as represented by Figure 1). However, some of the IMP-types deposited in the NCBI database (see text footnote 3) differ by limited sequence variations and thus are clearly related, as it is the case of IMP-63 and IMP-12.
Sequential acquisition of resistance determinants by epidemiologically successful clones (also called “high-risk clones”) of P. aeruginosa represents a serious public health problem, leaving clinicians with very few therapeutic options to fight nosocomial infections (Del Barrio-Tofino et al., 2017.) By impairing the activity of most antipseudomonal ß-lactams, IMP-type carbapenemases contribute to the evolution of these widespread clones toward pan-drug resistance (Oliver et al., 2015). In this study, the novel metallo-ß-lactamase IMP-63 was identified in one of these clones, involved in an outbreak at H1 hospital, Paris. The detection of other metallo-ß-lactamases such as IMP-1, IMP-8, IMP-13, IMP-45, and VIM-2 in ST308 strains isolated in Japan, Germany, France, China, and India, respectively, confirms the capacity of this international clone to locally collect carbapenemase genes from still unknown environmental reservoirs (reviewed in Oliver et al., 2015). Pseudomonas putida may constitute an epidemiological link between such natural reservoirs and P. aeruginosa as some hospital strains belonging to the P. putida complex were shown to express IMP-1 or IMP-2 in Japan, a country of high endemicity for these ß-lactamases and their variants (Shibata et al., 2003; Yomoda et al., 2003). Simultaneous emergence of IMP-15-positive clinical strains of P. aeruginosa and P. putida was also noticed in Spain (Gilarranz et al., 2013), while co-occurrence of P. aeruginosa and P. putida harboring enzyme IMP-19 was recently demonstrated in a French hospital (Amoureux et al., 2017). Of course, other non-fermenting Gram-negative bacilli may take part in the complex diffusion of IMP and VIM determinants (Yan et al., 2001; Amoureux et al., 2017).
In this study, the blaIMP–63 gene cassette was detected in the variable region of four novel integrons, namely In1297, In1574, In1573, and In1572 (Figure 2). These integrons were borne by different genetic structures (Figure 5) including four distinct plasmids (pNECK1, pTROUS2, pTROUS1, pROUE1), with In1573 occurring on two plasmids (pTROUS2, pTROUS1) in P. aeruginosa and P. putida, respectively. Except the isolates of hospital H1 outbreak, the IMP-63-producing strains of P. aeruginosa were clonally unrelated. Several lines of evidence suggest that the location of integron In1574 in P. aeruginosa strain Pae2567 is chromosomal. All the IMP-63-encoding integrons except In1574 turned out to share the same distinctive feature of being flanked near the putative orf6 gene by a copy of ISPa17, while this element is located next to the integrase gene intl in other ΔTn402-like transposon platforms, such as those carried by plasmids pNOR-2000 (Bonnin et al., 2013), pAX22 (Di Pilato et al., 2014), pPC9 (Molina et al., 2014), TnCP23 (Klockgether et al., 2004), and pJB12 (Botelho et al., 2017; Figure 5). This observation supports the idea that In1297, In1573 and In1572 derive from a common blaIMP–63-carrying transposon whose propagation among Pseudomonas strains occurred through complex genetic transfers, involving ISPa17-mediated transposition events and several plasmid carriers. The presence of DR signatures adjacent to IRR of ISPa17 and IRi of ΔTn402::In1297 (plasmid pNECK1) or ΔTn402::In1572 (plasmid pROUE1), indicates that ISPa17 likely played a major role in the diffusion of blaIMP–63 on different supports.
With its unique blaIMP–63 cassette, integron In1574 might represent the index platform from which the evolution occurred. While displaying different variable regions, the other IMP-63-encoding integrons contain the same sequential arrangement of three gene cassettes, namely (i) blaIMP–63, (ii) aac(6′)Ib′ or aacA4-28, and (iii) blaOXA–19 or blaOXA–35. Genes aac(6′)Ib′ and aacA4-28 code for closely related aminoglycoside modifying enzymes, while extended-spectrum ß-lactamases (ESBLs) OXA-19 and OXA-35 only differ by a single amino acid residue. Thus is it tempting to speculate that the triad blaIMP–63-aacA4-blaOXA was present in In1297, In1573, and In1572 prior to integration of other elements such as fosM, gcu170, gcuF1, and ISPsp7v. The presence of a divergently oriented copy of ISPsp7v in the variable region of two of these integrons also strongly suggests that In1297 evolved from In1573, both by acquisition of fosM and gcu170, and by mutations in gene aac(6′)Ib′. Finally, it should be noted that the integron found to carry gene blaIMP–12, the closest homolog of blaIMP–63, in an Italian strain of P. putida only contained the gene aacA4 in addition to as second resistance cassette, and that its genetic support (plasmid pVA758) was actually different from the IMP-63-encoding plasmids described here. Given that blaIMP–63 is a single nucleotide variant of blaIMP–12, one cannot rule out the possibility that some of the blaIMP–63 genes detected in France have evolved independently from undetected blaIMP–12 precursors, thus giving rise to various evolutionary and diffusion events.
In conclusion, this work illustrates how resistance genes can use very complex and unpredictable routes to diffuse among related species such as those belonging to the fluorescent Pseudomonas group, within a relatively short timeframe (2011–2016). Acquisition of gene blaIMP–63 with other resistance determinants, in particular ESBL-encoding genes (e.g., blaOXA–19 or blaOXA–35) conferred a multidrug resistance phenotype to several hospital strains of P. aeruginosa and P. putida.
Data Availability
The datasets generated for this study can be found in NCBI, MK047608, MK047609, MK047610, MK047611, and KX821663.1.
Ethics Statement
The clinical strains described in the present study were isolated by public hospital laboratories (H1, H2, H3), accredited by the French Ministry of Health, as part as their routine diagnostic activities. These strains were then transmitted to the NRC-AR (Besançon) for further investigations, accompanied with fully anonymized data. The analyses performed by the NRC did not require additional clinical samples or medical intervention on patients; therefore no patient consent or specific agreement by an ethics committee was needed.
Author Contributions
PP and KJ contributed to the conception of the study. MB, EL, PT, TJ, and BV performed the data analyses. PP wrote the manuscript. KJ and J-RZ helped to perform the analysis with constructive discussions.
Funding
The NRC-AR is funded by the French Ministry of Health via the Santé Publique France agency.
Conflict of Interest Statement
The authors declare that the research was conducted in the absence of any commercial or financial relationships that could be construed as a potential conflict of interest.
Acknowledgments
The authors thank medical microbiologists Hélène Guet-Revillet, Emmanuel Bille, Didier Moissenet and Sophie Boyer for referring the IMP-63 positive strains to the French National Reference Center for Antibiotic Resistance (NRC-AR).
Supplementary Material
The Supplementary Material for this article can be found online at: https://www.frontiersin.org/articles/10.3389/fmicb.2019.00404/full#supplementary-material
Footnotes
- ^ http://www.eucast.org
- ^ http://www.genomicepidemiology.org
- ^ https://www.ncbi.nlm.nih.gov
- ^ http://www.snapgene.com
- ^ http://pubmlst.org/paeruginosa/
- ^ http://integrall.bio.ua.pt/
- ^ https://pubmlst.org/pputida/
References
Amoureux, L., Riedweg, K., Chapuis, A., Bador, J., Siebor, E., Pechinot, A., et al. (2017). Nosocomial infections with IMP-19-producing Pseudomonas aeruginosa linked to contaminated sinks, France. Emerg. Infect. Dis. 23, 304–307. doi: 10.3201/eid2302.160649
Aubert, D., Poirel, L., Ali, A. B., Goldstein, F. W., and Nordmann, P. (2001). OXA-35 is an OXA-10-related beta-lactamase from Pseudomonas aeruginosa. J. Antimicrob. Chemother. 48, 717–721. doi: 10.1093/jac/48.5.717
Bonnin, R. A., Poirel, L., Nordmann, P., Eikmeyer, F. G., Wibberg, D., Puhler, A., et al. (2013). Complete sequence of broad-host-range plasmid pNOR-2000 harbouring the metallo-beta-lactamase gene blaVIM-2 from Pseudomonas aeruginosa. J. Antimicrob. Chemother. 68, 1060–1065. doi: 10.1093/jac/dks526
Boronin, A. M. (1992). Diversity of Pseudomonas plasmids: to what extent? FEMS Microbiol. Lett. 100, 461–468. doi: 10.1111/j.1574-6968.1992.tb14077.x
Botelho, J., Grosso, F., and Peixe, L. (2017). Characterization of the pJB12 plasmid from Pseudomonas aeruginosa reveals Tn6352, a novel putative transposon associated with mobilization of the blaVIM-2-harboring In58 integron. Antimicrob. Agents. Chemother. 61:e02532-16. doi: 10.1128/AAC.02532-16
Bramucci, M., Chen, M., and Nagarajan, V. (2006). Genetic organization of a plasmid from an industrial wastewater bioreactor. Appl. Microbiol. Biotechnol. 71, 67–74. doi: 10.1007/s00253-005-0119-2
Brantl, S. (2015). Antisense-RNA mediated control of plasmid replication - pIP501 revisited. Plasmid 78, 4–16. doi: 10.1016/j.plasmid.2014.07.004
Casin, I., Hanau-Bercot, B., Podglajen, I., Vahaboglu, H., and Collatz, E. (2003). Salmonella enterica serovar Typhimurium bla(PER-1)-carrying plasmid pSTI1 encodes an extended-spectrum aminoglycoside 6’-N-acetyltransferase of type Ib. Antimicrob. Agents Chemother. 47, 697–703. doi: 10.1128/AAC.47.2.697-703.2003
Del Barrio-Tofino, E., Lopez-Causape, C., Cabot, G., Rivera, A., Benito, N., Segura, C., et al. (2017). Genomics and susceptibility profiles of extensively drug-resistant Pseudomonas aeruginosa isolates from Spain. Antimicrob. Agents Chemother. 61:e01589-17. doi: 10.1128/AAC.01589-17
Di Pilato, V., Pollini, S., and Rossolini, G. M. (2014). Characterization of plasmid pAX22, encoding VIM-1 metallo-beta-lactamase, reveals a new putative mechanism of In70 integron mobilization. J. Antimicrob. Chemother. 69, 67–71. doi: 10.1093/jac/dkt311
Ditta, G., Stanfield, S., Corbin, D., and Helinski, D. R. (1981). “Cloning DNA from Rhizobium meliloti using a new broad host range, binary vehicle system,” in Basic Life Science, ed. J. M. Lyons, R. C. Valentine, D. A. Phillips, D. W. Rains, and R. C. Huffaker (New York, NY: Plenum Publishing Corp), 31–40.
Docquier, J. D., Riccio, M. L., Mugnaioli, C., Luzzaro, F., Endimiani, A., Toniolo, A., et al. (2003). IMP-12, a new plasmid-encoded metallo-beta-lactamase from a Pseudomonas putida clinical isolate. Antimicrob. Agents Chemother. 47, 1522–1528. doi: 10.1128/AAC.47.5.1522-1528.2003
Gilarranz, R., Juan, C., Castillo-Vera, J., Chamizo, F. J., Artiles, F., Alamo, I., et al. (2013). First detection in Europe of the metallo-beta-lactamase IMP-15 in clinical strains of Pseudomonas putida and Pseudomonas aeruginosa. Clin. Microbiol. Infect. 19, E424–E427. doi: 10.1111/1469-0691.12248
Gomila, M., Pena, A., Mulet, M., Lalucat, J., and Garcia-Valdes, E. (2015). Phylogenomics and systematics in Pseudomonas. Front. Microbiol. 6:214. doi: 10.3389/fmicb.2015.00214
Heuer, H., Binh, C. T., Jechalke, S., Kopmann, C., Zimmerling, U., Krogerrecklenfort, E., et al. (2012). IncP-1epsilon plasmids are important vectors of antibiotic resistance genes in agricultural systems: diversification driven by class 1 integron gene cassettes. Front. Microbiol. 3:2. doi: 10.3389/fmicb.2012.00002
Hocquet, D., Llanes, C., Thouverez, M., Kulasekara, H. D., Bertrand, X., Plésiat, P., et al. (2012). Evidence for induction of integron-based antibiotic resistance by the SOS response in a clinical setting. PLoS Pathog. 8:e1002778. doi: 10.1371/journal.ppat.1002778
Hocquet, D., Plésiat, P., Dehecq, B., Mariotte, P., Talon, D., Bertrand, X., et al. (2010). Nationwide investigation of extended-spectrum beta-lactamases, metallo-beta-lactamases, and extended-spectrum oxacillinases produced by ceftazidime-resistant Pseudomonas aeruginosa strains in France. Antimicrob. Agents Chemother. 54, 3512–3515. doi: 10.1128/AAC.01646-09
Jové, T., Da Re, S., Denis, F., Mazel, D., and Ploy, M. C. (2010). Inverse correlation between promoter strength and excision activity in class 1 integrons. PLoS Genet. 6:e1000793. doi: 10.1371/journal.pgen.1000793
Juan, C., Zamorano, L., Mena, A., Alberti, S., Perez, J. L., and Oliver, A. (2010). Metallo-beta-lactamase-producing Pseudomonas putida as a reservoir of multidrug resistance elements that can be transferred to successful Pseudomonas aeruginosa clones. J. Antimicrob. Chemother. 65, 474–478. doi: 10.1093/jac/dkp491
Kieser, T. (1984). Factors affecting the isolation of CCC DNA from Streptomyces lividans and Escherichia coli. Plasmid 12, 19–36. doi: 10.1016/0147-619X(84)90063-5
Klockgether, J., Reva, O., Larbig, K., and Tummler, B. (2004). Sequence analysis of the mobile genome island pKLC102 of Pseudomonas aeruginosa C. J. Bacteriol. 186, 518–534. doi: 10.1128/JB.186.2.518-534.2004
Lee, A. H., Flibotte, S., Sinha, S., Paiero, A., Ehrlich, R. L., Balashov, S., et al. (2017). Phenotypic diversity and genotypic flexibility of Burkholderia cenocepacia during long-term chronic infection of cystic fibrosis lungs. Genome Res. 27, 650–662. doi: 10.1101/gr.213363.116
Lee, K., Lim, J. B., Yum, J. H., Yong, D., Chong, Y., Kim, J. M., et al. (2002). bla(VIM-2) cassette-containing novel integrons in metallo-beta-lactamase-producing Pseudomonas aeruginosa and Pseudomonas putida isolates disseminated in a Korean hospital. Antimicrob. Agents Chemother. 46, 1053–1058. doi: 10.1128/AAC.46.4.1053-1058.2002
Loutit, J. S., Pearce, L. E., and Marinus, M. G. (1968). Investigation of the mating system of Pseudomonas aeruginosa strain 1. I. Kinetic studies. Genet. Res. 12, 29–36. doi: 10.1017/S0016672300011587
Marchiaro, P., Viale, A. M., Ballerini, V., Rossignol, G., Vila, A. J., and Limansky, A. (2010). First report of a Tn402-like class 1 integron carrying blaVIM-2 in Pseudomonas putida from Argentina. J. Infect. Dev. Ctries. 4, 412–416.
Molina, L., Udaondo, Z., Duque, E., Fernandez, M., Molina-Santiago, C., Roca, A., et al. (2014). Antibiotic resistance determinants in a Pseudomonas putida strain isolated from a hospital. PLoS One 9:e81604. doi: 10.1371/journal.pone.0081604
Mugnier, P., Casin, I., Bouthors, A. T., and Collatz, E. (1998). Novel OXA-10-derived extended-spectrum beta-lactamases selected in vivo or in vitro. Antimicrob. Agents Chemother. 42, 3113–3116. doi: 10.1128/AAC.42.12.3113
Oliver, A., Mulet, X., Lopez-Causape, C., and Juan, C. (2015). The increasing threat of Pseudomonas aeruginosa high-risk clones. Drug Resist. Updat. 21–22, 41–59. doi: 10.1016/j.drup.2015.08.002
Osano, E., Arakawa, Y., Wacharotayankun, R., Ohta, M., Horii, T., Ito, H., et al. (1994). Molecular characterization of an enterobacterial metallo beta-lactamase found in a clinical isolate of Serratia marcescens that shows imipenem resistance. Antimicrob. Agents Chemother. 38, 71–78. doi: 10.1128/AAC.38.1.71
Poirel, L., Girlich, D., Naas, T., and Nordmann, P. (2001). OXA-28, an extended-spectrum variant of OXA-10 beta-lactamase from Pseudomonas aeruginosa and its plasmid- and integron-located gene. Antimicrob. Agents Chemother. 45, 447–453. doi: 10.1128/AAC.45.2.447-453.2001
Raphael, E., and Riley, L. W. (2017). Infections caused by antimicrobial drug-resistant saprophytic Gram-negative bacteria in the environment. Front Med. 4:183. doi: 10.3389/fmed.2017.00183
Rather, P. N., Munayyer, H., Mann, P. A., Hare, R. S., Miller, G. H., and Shaw, K. J. (1992). Genetic analysis of bacterial acetyltransferases: identification of amino acids determining the specificities of the aminoglycoside 6’-N-acetyltransferase Ib and IIa proteins. J. Bacteriol. 174, 3196–3203. doi: 10.1128/jb.174.10.3196-3203.1992
Shibata, N., Doi, Y., Yamane, K., Yagi, T., Kurokawa, H., Shibayama, K., et al. (2003). PCR typing of genetic determinants for metallo-beta-lactamases and integrases carried by gram-negative bacteria isolated in Japan, with focus on the class 3 integron. J. Clin. Microbiol. 41, 5407–5413. doi: 10.1128/JCM.41.12.5407-5413.2003
Smith, A. W., and Iglewski, B. H. (1989). Transformation of Pseudomonas aeruginosa by electroporation. Nucleic Acids Res. 17:10509. doi: 10.1093/nar/17.24.10509
Szuplewska, M., Ludwiczak, M., Lyzwa, K., Czarnecki, J., and Bartosik, D. (2014). Mobility and generation of mosaic non-autonomous transposons by Tn3-derived inverted-repeat miniature elements (TIMEs). PLoS One 9:e105010. doi: 10.1371/journal.pone.0105010
van Mansfeld, R., Willems, R., Brimicombe, R., Heijerman, H., van Berkhout, F. T., Wolfs, T., et al. (2009). Pseudomonas aeruginosa genotype prevalence in Dutch cystic fibrosis patients and age dependency of colonization by various P. aeruginosa sequence types. J. Clin. Microbiol. 47, 4096–4101. doi: 10.1128/JCM.01462-09
Vu-Thien, H., Corbineau, G., Hormigos, K., Fauroux, B., Corvol, H., Clément, A., et al. (2007). Multiple-Locus Variable-Number Tandem-Repeat analysis for longitudinal survey of sources of Pseudomonas aeruginosa infection in cystic fibrosis patients. J. Clin. Microbiol. 45, 3175–3183. doi: 10.1128/JCM.00702-07
White, P. A., McIver, C. J., Deng, Y., and Rawlinson, W. D. (2000). Characterisation of two new gene cassettes, aadA5 and dfrA17. FEMS Microbiol. Lett. 182, 265–269. doi: 10.1111/j.1574-6968.2000.tb08906.x
Yan, J. J., Hsueh, P. R., Ko, W. C., Luh, K. T., Tsai, S. H., Wu, H. M., et al. (2001). Metallo-beta-lactamases in clinical Pseudomonas isolates in Taiwan and identification of VIM-3, a novel variant of the VIM-2 enzyme. Antimicrob. Agents Chemother. 45, 2224–2228. doi: 10.1128/AAC.45.8.2224-2228.2001
Yang, C. H., Young, T., Peng, M. Y., and Weng, M. C. (1996). Clinical spectrum of Pseudomonas putida infection. J. Formos. Med. Assoc. 95, 754–761.
Keywords: Pseudomonas, IMP carbapenemases, plasmids, integrons, diffusion
Citation: Liapis E, Bour M, Triponney P, Jové T, Zahar J-R, Valot B, Jeannot K and Plésiat P (2019) Identification of Diverse Integron and Plasmid Structures Carrying a Novel Carbapenemase Among Pseudomonas Species. Front. Microbiol. 10:404. doi: 10.3389/fmicb.2019.00404
Received: 29 October 2018; Accepted: 15 February 2019;
Published: 04 March 2019.
Edited by:
Dongsheng Zhou, Beijing Institute of Microbiology and Epidemiology, ChinaReviewed by:
Antonio Oliver, Hospital Universitario Son Espases, SpainBenjamin Andrew Evans, University of East Anglia, United Kingdom
Krisztina M. Papp-Wallace, Louis Stokes Cleveland VA Medical Center, United States
Copyright © 2019 Liapis, Bour, Triponney, Jové, Zahar, Valot, Jeannot and Plésiat. This is an open-access article distributed under the terms of the Creative Commons Attribution License (CC BY). The use, distribution or reproduction in other forums is permitted, provided the original author(s) and the copyright owner(s) are credited and that the original publication in this journal is cited, in accordance with accepted academic practice. No use, distribution or reproduction is permitted which does not comply with these terms.
*Correspondence: Patrick Plésiat, patrick.plesiat@univ-fcomte.fr
†These authors have contributed equally to this work