Advances in nano-based materials for glioblastoma multiforme diagnosis: A mini-review
- Center of Nanotechnology and Tissue Engineering, Photobiology and Photomedicine Research Group, Department of Chemistry, Faculty of Philosophy, Sciences and Letters of Ribeirão Preto, University of São Paulo, São Paulo, Brazil
The development of nano-based materials for diagnosis enables a more precise prognosis and results. Inorganic, organic, or hybrid nanoparticles using nanomaterials, such as quantum dots, extracellular vesicle systems, and others, with different molecular compositions, have been extensively explored as a better strategy to overcome the blood-brain barrier and target brain tissue and tumors. Glioblastoma multiforme (GBM) is the most common and aggressive primary tumor of the central nervous system, with a short, established prognosis. The delay in early detection is considered a key challenge in designing a precise and efficient treatment with the most encouraging prognosis. Therefore, the present mini-review focuses on discussing distinct strategies presented recently in the literature regarding nanostructures’ use, design, and application for GBM diagnosis.
Nanotechnology applied to brain tumor diagnosis
A cancer diagnosis is commonly confirmed by family history, symptom evaluation, physical examination, testing by imaging, biomarkers, and biopsy assessment in some cases (do Nascimento et al., 2020). Conventionally, histopathology, cytology, and imaging techniques [X-ray, magnetic resonance imaging (MRI), computed tomography (CT), ultrasound, and endoscopy] detect visible morphological changes in degenerated tissue (Zhang et al., 2019). Early tumor diagnosis is a crucial factor for an ideal prognosis. However, there are still many difficulties in differentiating lesions at the beginning of the advanced stage.
Biomarkers are biological signatures of specific physiological or pathologic cell states that serve as intelligent tools for a quantitatively estimated risk condition, reflecting clinical response, progression, prediction, and outcomes (Ganau et al., 2018). Nanoparticles are an efficient tool associated with biomarkers for detecting cancer in the early stages (Smith et al., 2019; do Nascimento et al., 2020). For malignant glioblastoma multiforme (GBM), the most common and malignant brain tumor grade IV, early detection is a fundamental step in establishing better treatment approaches. Investigating biomarkers and nanostructures for GBM and improving their specificity remains a challenge. Regular probes for glioma diagnosis, such as Gd-based contrasts, are limited due to their restricted and low penetration into the brain barriers. Brain tumor diagnosis is also determined by the inability to discriminate between tumor cells and other normal tissues, with a higher level of redox changes, leading to a high false-positive diagnosis (Hanif et al., 2017; Wu et al., 2019).
Nanomaterials exhibit exclusive chemical, physical, and biological properties, small size, large surface area volume ratio, and surface-modulated characteristics (Mukhtar et al., 2020; Hsu et al., 2021). Recently, distinct nanoparticles have shown excellent results in treating and diagnosing complex and aggressive diseases, such as brain cancer (Zottel et al., 2019). Nanoparticles can be easily functionalized with different target molecules, such as PEG, folic acid (Brandt et al., 2019; Hsu et al., 2021), and gelatin (Carvalho et al., 2019), for several applications. Owing to the enhanced permeability and retention (EPR) effect, which describes the spontaneous accumulation of nanoparticles in tumor tissues (Yoshikawa et al., 2019), nanobiotechnology applied to the design of new materials has emerged as a promising method for the early diagnosis and survival of patients with GBM (de Paula et al., 2017; Wu et al., 2019; Zottel et al., 2019).
Inorganic, biological, and hybrid nanoparticles are widely employed as diagnostic agents (Mukherjee et al., 2019; Zhang et al., 2019; Yekula et al., 2020). Nanoparticles overcome the problem of drug transport in brain tumors. They are used as treatment and diagnostic agents because of their high affinity for specific binding sites, acting as target compounds (Zottel et al., 2019; Kirbas Cilingir et al., 2021). Their transposition into biological barriers is accomplished by passive and active targeting. The brain’s passive mechanisms involving nanoparticle accumulation are attributed to the EPR effect. In these cases, the poor tumor vasculature promotes abnormalities and deficiencies in the endothelial cells contributing to particles <400 nm that extravasates and accumulate in perivascular spaces (Su and Hu, 2018). On the other hand, the active nanoparticle accumulation pathway recognizes specific GBM surface receptors, which will be linked to the nanoparticle via functionalization and increase their specificity (Wadajkar et al., 2017).
Herein, we aimed to elucidate the advances in nanoparticles applied in nanomedicine and highlight their design and use as agents for glioblastoma diagnosis. Different nanomaterials that cross the blood-brain barrier (BBB) through distinct pathways are explored, as presented in Figure 1, supporting new strategies with higher sensitivity and specificity for GBM identification and clinical implications. Nowadays, the most used diagnostic tools for GBM are biomarkers, liquid biopsy, and bioimages. Diagnostic biomarkers enable more accurate classification of the tumor and better information about the prognostic, for example, the recurrence or progression. There are many molecular markers under evaluation in the clinical route (Szopa et al., 2017), and the most relevant of them in patients with GBM is the epidermal growth factor receptor (EGFR) (mutation or amplification). Liquid biopsy is used to diagnose brain tumors, once preventing surgery in the brain to perform a genetic tumor profile. The component in the liquid biopsy provides information for prognosis and diagnosis based on molecular profiling. However, the clinical application of the technique is still limited (Saenz-Antoñanzas et al., 2019). The bioimages have been referenced to evaluate GBM progression because it is a non-invasive and standard protocol for monitoring the tumor response. Promising results have been observed for some not yet clinically approved imaging biomarkers. Imaging biomarkers were highlighted as diagnostic tools for monitoring the brain tumor due to the response provided by potential individual patient management (Szopa et al., 2017).
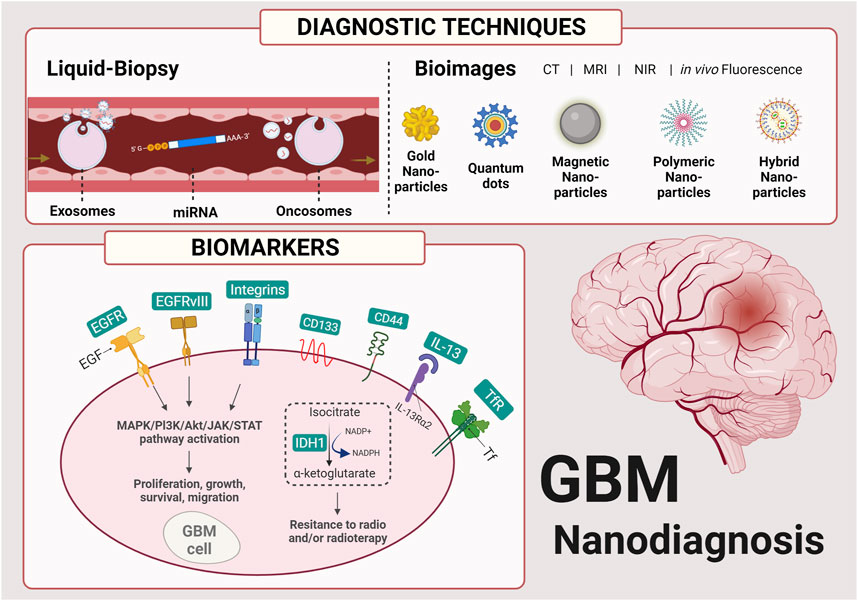
FIGURE 1. Schematic nano-based materials applied in diagnostic techniques and the main biomarkers involved in developing glioblastoma multiforme (GBM). Created with BioRender.com.
Principal types of nanoparticles for GBM diagnosis
Many nanoparticles have been developed and evaluated for therapeutic applications in recent years. For example, organic nanoparticles, such as polymeric, carbon, or lipid nanoparticles, are widely applied. Nanoparticles can accumulate in specific regions due to the EPR effect and act as a drug delivery system (Zhang et al., 2019). In this way, they increase the concentration of the drugs and consequently the therapeutic potential. Furthermore, nanoparticles are studied as potential diagnostic agents contributing to the early detection of many diseases. Mainly inorganic nanoparticles, such as quantum dots, are extensively examined for applications and sensors to detect cancer biomarkers in blood or liquid biopsies. In this minireview, we focus on nano-based materials extensively used as diagnostic agents.
In addition to their well-defined optical, magnetic, electronic, and fluorescent properties, inorganic nanoparticles exhibit high chemical, photochemical, and physical stability (Núñez et al., 2018; Zhou et al., 2020). Conventionally, gadolinium-based particles, which are contrast agents, are used in MRI examinations (Barajas et al., 2019). Besides, iron superparamagnetic iron oxide nanoparticles were recently approved by the Food and Drug Administration (FDA) as a magnetic resonance agent (Tapeinos et al., 2019). With advances in nanoscience, new nanostructured gadolinium-based contrast gold nanoparticles (Choi et al., 2011; Indrasekara et al., 2013), distinct iron-based nanoparticles such as lipid and peptide-based delivery systems, quantum dots (QDs) (Sun et al., 2014; Cabral Filho et al., 2016), carbon-based nanomaterials (CBNs), including carbon nanotubes (CNT), graphene oxide (GO), and graphene quantum dots (GQDs), (Ashrafizadeh et al., 2020; Kirbas Cilingir et al., 2021), and other types of nanomaterials have been extensively evaluated to improve the detection of glioblastomas, especially in the early stages (Kang et al., 2015; Skripka et al., 2021; Song et al., 2021).
Biological nanoparticles are composed of extracellular vesicles (EVs), including microvesicles, exosomes, and apoptotic bodies, isolated from the cell line and used as biological drug delivery systems (Thomas et al., 2020; Simionescu et al., 2021). Biological nanoparticles have essential functions in cells and play a role between cells and tissues. Through body fluids, exosomes transport biological molecules, such as nucleic acids, proteins, and lipids (Zottel et al., 2019). Generally, natural nanoparticles are approximately 40–120 nm and are internalized via endocytosis or cellular fusion (Van Niel et al., 2018). EVs have been used to capture cancer biomarkers to improve the internalization and recognition processes. Accordingly, they are potential tools for real-time cancer detection (Zhang et al., 2019; Yong et al., 2019; He et al., 2021). Although they present low toxicity, biological nanoparticles are not easy to handle on a large scale but are cost-effective (Zhang et al., 2019). In addition, the coated nanoparticle for cancer cells improved targeting due to the homotypic recognition of GBM cells (De Pasquale et al., 2020). The concept of homotypic targeting specifically targets to kill GBM cells in vitro upon the efficient target crossing an in vitro BBB model. Cancer cell membrane-coated nanoparticles typically consist of a core with a cancer cell membrane coat that can carry tumor-specific receptors or antigens for cancer (Jin and Bhujwalla, 2020). The nanoparticle can be applied as a vehicle to obtain an image and is investigated for cancer theranostics.
Nanohybrid particles (HNP) are composed of two or more nanomaterials (∼200 nm), usually polysaccharides, biomolecules, peptides, and fluorescent nanoprobes, such as QDs with high photochemical and biological stability (Carvalho et al., 2019; Zhang et al., 2019). HNPs have been extensively employed to bioimage tumors and perform other functions as optical intracellular nanosensors. Different approaches are employed to synthesize these HNPs: 1) top-down, a physical method where bulk material is used to form the nanostructure; and 2) bottom-up, a more accessible, eco-friendly process that can control the physicochemical properties (e.g., shape, size, surface composition), once NPs are constructed from molecules and/or atoms. Accordingly, HNPs can perform bioimaging analysis of the internalization kinetics process, endocytic pathways, and intracellular tracking of live brain cancer cells (Carvalho et al., 2020; An et al., 2021; Lee et al., 2021). Self-assembled organic−inorganic hybrids are the building blocks that can create hybrid nanosystems. These promising nanosystems show favorable nano-bio interfacial properties while retaining their physical/chemical functionalities. A common approach to solve this issue is to conjugate hydrophilic polymers. For example, PEG, polylactide (PLA), poly (lactic-co-glycolic acid) (PLGA), poly (caprolactone) (PCL), poly-(butylene succinate) are frequently used in self-assembled nano-bio materials, all of which have been approved by FDA for specific in vivo biomedical applications (Li et al., 2018). For instance, the coated manganese (Mn2+)-doped nanoparticles with multiple layers of photosensitizer-conjugated polymers have been used in the nanocomposite for the imaging-guided photodynamic therapy of tumors. According to Ma and coworkers, prepping nanoparticles in which oleic acid is stabilized with cisplatin and Rhodamine B conjugated amphiphilic polymers is possible. The self-assembled micellar structure reduces adverse side effects of cisplatin while simultaneously monitoring the therapeutic process through in vitro and in vivo imaging (Li et al., 2018). Another example is the bio-nano particles conjugated using load enzymes on nanomaterials. The immobilization of enzymes on polymers, inorganic metal oxide, and hybrid compounds provides potential applications in medical applications, such as biosensors and chip or microfluidic sensors (Khan S et al., 2021).
Glioblastoma multiforme
Gliomas are central nervous system tumors that affect normal stromal (glial) cells, such as astrocytes, oligodendrocytes, and ependymal cells (Louis et al., 2016; Finch et al., 2021). The World Health Organization (WHO) classifies astrocytoma according to progressive histological features, such as molecular markers, into four grades (I-IV) (Louis et al., 2016; Weller et al., 2021). Grade IV astrocytoma, or GBM, is the most aggressive diffuse glioma of the astrocytic l lineage, with a poor prognosis and high mortality rate (Poon et al., 2020). Classical treatment includes surgery, radiotherapy, and chemotherapy, with a median survival rate of 12–15 months after diagnosis (Oronsky et al., 2021).
Blood-brain barrier
GBM prognosis is also related to the permeability of drug delivery across the Blood-brain barrier (BBB) (Steeg, 2021). The BBB is one of the essential protection mechanisms in the central nervous system (CNS). Once it is composed of a multicellular structure of endothelial cells that assemble continuous intercellular tight junctions, limiting paracellular and transcellular movement (de Paula et al., 2017; Steeg, 2021), the BBB also protects against pathogens and exogenous compounds (Kadry et al., 2020). Molecules can cross the BBB through specific pathways, including endogenous, receptor-mediated, carrier-mediated, or adsorptive-mediated transport systems. Therefore, biomolecule interactions and particle size play a crucial role in each type of transport. Accordingly, nanomaterials are critical devices for brain medicine applications. Particles smaller than 4 nm can easily penetrate the junctions via passive diffusion (Kirbas Cilingir et al., 2021). As a result, nanomedicine has transformed medical diagnosis and treatment, an essential strategy for overcoming the BBB and delivering drug molecules to a specific brain region. Therefore, nanomaterials that can mediate drug delivery are emerging as an effective and non-invasive system to treat cerebral diseases. Small lipophilic molecules less than 400 Da can diffuse passively through endothelial cells. The penetration of charged molecules such as cationic nanoparticles depends on adsorption-mediated endocytosis.
In contrast, delivering large molecules with high hydrophilicity, such as transferrin, requires an active transport route (Zhou et al., 2018). One of the negative points of nanoparticles is their low penetration efficiency through the BBB because the concentration for som was not high enough to result in therapeutic effects in the CNS. To solve this problem and enhance the penetration efficiency of nanoparticles, hybrids, functionalized, and conjugated nanomaterials with the ligands of receptors located in the BBB were utilized extensively. For example, the most favored strategy is using transferrin, taking advantage of the high affinity between transferrin and its receptor to assist the nanoparticles in penetrating the BBB (Zhou et al., 2018).
Biomarkers and molecular diagnosis
Molecular biomarkers used in diagnosis are essential for predicting and determining the most effective therapy. In this context, the gene mutant isocitrate dehydrogenase (IDH1), a metabolic marker with ubiquitous expression in lower-grade gliomas, can contribute to the diagnosis of tumorigenesis. IDH1 is also related to a better prognosis due to decreased NADPH levels, enhancing radio- and chemotherapy sensitivity (Oronsky et al., 2021). The WHO classified IDH1 gene mutation status, or the lack of mutations, as a biomarker of poor prognosis, including glioblastoma IDH-wildtype and glioblastoma IDH-mutant (Louis et al., 2016). The gene expression and protein profiles of GBM analysis provide a database for significant GBM biomarkers (Verhaak et al., 2010; Qi et al., 2020).
Many studies have approached the EGFR pathway in developing nanoparticle diagnostic tools, as discussed hereafter. Comprehensive GBM genomic analysis revealed complex rearrangements of the EGFR gene signature, considered a hallmark of GBM (approximately 60% of GBM modifications) and a central pathway for GBM maintenance and progression (Brennan et al., 2013; ; Wang et al., 2021). Besides the EGFR pathway, we highlighted biomarkers widely used as a GBM diagnostic tool, such as specific biomarkers of GBM stem-like cells (GSCs) and transferrin (Table 1). In the present mini-review, we have summarized the main biomarkers using different nanoparticles focusing on GBM diagnosis, as shown in Table 1.
EGFR pathway
EGFR is a receptor tyrosine kinase (RTK) on the cell surface with a high affinity for growth factors, cytokines, or hormones, consisting of an extracellular, transmembrane domain and an intracellular cytoplasmic domain. This receptor plays a relevant role in cellular processes, such as cell proliferation, differentiation, survival, cell cycle control, and migration (Talukdar et al., 2020). EGFR overexpression is commonly correlated with cancers associated with progression and poor prognosis due to its crucial role in different processes of cell cycle progression. When activated, EGFR undergoes dimerization of its intracellular cytoplasmic domain, which leads to autophosphorylation of its C-terminal tail, which serves as a docking site for SRC homology 2 (SH2) domain-containing signaling proteins, including phosphoinositide 3-kinase (PI3K) and signal transducers and activators of transcription (STAT) proteins. The main pathways involved in EGFR activation include the RAS/mitogen-activated protein kinase (MAPK)/extracellular signal-regulated kinase (ERK), PI3K/protein kinase B (PKB/AKT), Janus kinase (JAK)/STAT, and protein kinase C (PKC) pathways. These pathways simulate different progression events, such as cell proliferation, growth, differentiation, apoptosis, and migration (Sibilia et al., 2000; Hatanpaa et al., 2010; Oprita et al., 2021).
Several nanoparticles have been developed to target the EGFR pathway for biomarker diagnosis. Mu et al. (2015) developed a monoclonal antibody conjugated with superparamagnetic iron oxide nanoparticles to improve MRI resolution in gliomas. Different genetic rearrangements have been reported to occur in the EGFR gene in GBM. A frequently detected point mutation is EGFR deletion of exons 2-7 (EGFRvIII), which provides a persistent low-level active state, leading to constitutive kinase activity of EGFR, potentiating tumor growth, and increasing survival and invasion in GBM patients (An et al., 2018). This specific point mutation is an essential biomarker for diagnosis and is associated with iron oxide for MRI improvements in GBM (Hadjipanayis et al., 2010). Different nanoparticles target EGFRvIII; for example, PEGylated QDs aptamer-conjugates are used to improve GBM fluorescence images (Tang et al., 2017). Besides EGFRvIII, the interleukin-13 receptor alpha 2 (IL-13Rα2) has also been mentioned as an essential receptor target for diagnosis and treatment of GBM since it was identified as one of the downstream targets of EGFRvIII resulting in the GBM progression (Newman et al., 2017). In this sense, contrast agents based on gadolinium (Gd) in conjunction with IL-13 have been developed for better glioma targeting (Li et al., 2015). Therefore, targets of the EGFR pathway have been considered essential players in GBM for diagnostic strategies to associate nanoparticles.
Glioblastoma stem cells
Many studies have reported the presence of GBM stem-like cells (GSCs) as a GBM biomarker related to poor prognosis, linking malignant relapse and resistance due to the generation of progenitor-like responses that cause the escape of multimodal therapy (Glaser et al., 2017; Hassn Mesrati et al., 2020). GSCs are also important targets for improving cancer theranostics (Tang et al., 2021). They can arise from embryonic stem cell mutations or accumulative mutations in the downstream progenitor over time, contributing to the maintenance of GBM tumors (Glaser et al., 2017). Interestingly, the EGFR signaling pathway is also involved in stemness regulation, controlling treatment resistance (Talukdar et al., 2020). Classical biomarkers for identifying GSCs include CD44, CD15, CD70, Nanog, Sox2, and Oct4, providing a specific profile for isolation and identification of the stemness involved in proliferation, migration, and invasion of GBM. A transmembrane glycoprotein antigen cell surface has been highlighted as a potential target for GBM diagnosis CD133 (Tang et al., 2021). Cho et al. (2017) described small gold-associated peptide nanoparticles as biomarkers with high and efficient biocompatible cell targets for CD133, and immunoliposomes using CD133 antibodies to target GSCs (Kim et al., 2018), improving sensitivity without losing the specificity.
Another strategy is the use using of signs heterodimeric transmembrane adhesion receptors with physiological roles that activate signal transduction pathways and mediate cell signals. Integrins are essential, upregulated biomarkers in GSCs (Su et al., 2020). Integrins trigger the clustering of enzymes and substrates, inducing cross-phosphorylation of RTKs, such as in the EGFR pathway (Miklaszewska et al., 2019). αvβ3 and αvβ5 are classical integrins overexpressed in glioma (Song et al., 2021). Advances in molecular imaging have enabled the exploration of self-assembled peptide nanoparticles targeting αvβ3 integrin for a more precise diagnosis which is considered an important target for early GBM detection (Rizvi et al., 2021).
Transferrin
Transferrin (Tf) is responsible for ferric uptake in the body, and its surface receptor (TfR) enters the cells via TfR-mediated endocytosis. TfR-1 is related to tumorigenesis, inducing iron accumulation in cells by enhancing the progression rate or glutamate production (Calzolari et al., 2010; Ni et al., 2020). Among several tumor cells that are iron-dependent for proliferation, GBM overexpresses TfR, which is less expressed in noncancerous cells (Luo et al., 2019).
Tf-targeted nanoprobes are tools for molecular imaging GBM based on TfR activation, indicating a tumorigenic process and a fluorescent area. As shown in Table 1, distinct nanomaterials such as gold, magnetic nanoparticles, and liposomes have been functionalized with Tf to enhance the specificity of TfR and improve diagnosis. Cabral Filho et al. (2016) conjugated QDs with Tf and observed specificity to U87MG (classical GBM cell line) and DBTRG-05MG (recurrent GBM cell line), suggesting that these inorganic fluorescent nanoprobes (λ exc = 360 nm and λ em = 650 nm) could accurately detect GBM cells. Distinctly, Weerathunge et al. (2019) conjugated superparamagnetic iron oxide nanoparticles with a Tf moiety to develop a simple colorimetric biosensor that can identify U87MG cells.
The BBB penetration mechanism can be classified into active and passive transport routes. The passive transport indicates energy-independent processes are simple diffusion. The passive diffusion of drugs usually occurs in tumor cells via the EPR effect. In contrast, the active transport routes include the receptor and adsorption-mediated endocytosis process, both of which require the hydrolysis of adenosine triphosphate (ATP). Adsorption-mediated endocytosis is a type of active transport that occurs for cationic nanoparticles, such as liposomes and gold nanoparticles. The receptor-mediated endocytosis is another type of active transport that include the TfR, anti-TRf antibody, and others. The most common examples of these carriers are the use of TfR with nanoparticles such as PLGA/PLA coated with polysorbate 80, liposomes, and other nanosystems. The BBB penetration mechanism of the drug delivery for the brain varies according to the nanomaterials. However, endocytosis mediated by TfR is one of the most investigated receptors that facilitate the diagnosis because signaling significantly improves BBB permeation of nanomaterials (Zhou et al., 2018).
Bioimaging
Images with high resolution are crucial for early GBM diagnosis, and CT, positron emission tomography (PET), and MRI are routine procedures and can be used for in vivo applications (Oronsky et al., 2021). Conventional contrasts in these techniques present limitations resulting in a lack of sensibility. They are polydisperse, accumulate in undesired tissues, and show lower dispersion and diffusion into the tissues (Jafari et al., 2018). In the past decades, nanoparticles designed for cancer monitoring and diagnosis as a size-controlled material have attracted attention. Besides being biocompatible, they are homogeneous and easily coated with different and specific biomolecules, leading to a well-resolved and accurate diagnosis (Jin et al., 2020). Then, studies are developing nanotechnology-based diagnostic tools for real-time and cost-effective cancer diagnosis, advancing their clinical trials and providing them soon in the medical market (Zhang et al., 2019). In this context, multimodal imaging has been referred to as the simultaneous production of signals for more than one image technique, such as the construction of nanoprobes associating molecular biology and nanomaterials, increasing specific tissue imaging (Xie et al., 2021).
Usually, MRIs are employed in the assessment and recurrence evaluation of tumors, with gadolinium-based materials as the standard gold contrast owing to their paramagnetic properties (Wu et al., 2019; Mukhtar et al., 2020). However, Gd-contrast has a short half-life. Further, repeated doses are required to enhance the signal for normal adjacent cells. Indeed, primary 2–3 mm lesions could not be detected owing to the low-resolution limit and poor MRI specificity related to other intracranial injuries (Klekner et al., 2019). Clinical Gd-MRI is needed to evaluate the therapeutic efficacy after maximal resection of the brain tumor, followed by chemotherapy. Nevertheless, Gd-based contrasts did not allow differentiation between progression and pseudo-progression in recurrent tumors (Taal et al., 2008; Wen et al., 2010; Barajas et al., 2019).
Designing paramagnetic or Gd-containing nanomaterials is crucial for improving conventional contrast agents. Barajas et al. (2019) combined nanoparticles of iron and Gd to enhance the sharp contrast using different ratios of these paramagnetic to distinguish between IDH1 mutant patients, clarifying GBM pseudoprogression. Gd can be functionalized with growth factors, peptides, and antibodies or encapsulated into liposomes or nanoemulsions to improve targeting and MRI imaging resolution (Mukhtar et al., 2020).
To obtain a better contrast, lower attenuation, higher spatial and temporal resolution, and red and near-infrared (NIR) spectroscopy has become an appropriate strategy for the optical imaging of biological tissues. The wavelength range for near-infrared light is associated with a deeper penetration in tissues, lower absorption and scattering by biomolecules, and a region of minimal cell autofluorescence (Li et al., 2017; Liu et al., 2019; Hettie et al., 2021). Thus, different diagnostic techniques can be exploited based on photoluminescence, optical coherence tomography imaging, and photoacoustic for in vitro and in vivo imaging (Skripka et al., 2021).
Nanoparticles with proper absorption in the infrared window are used for fluorescence and NIR detection, such as gold nanoparticles (Choi et al., 2011), Ag and Cu-doped nanoparticles, and rare Earth nanomaterials (Liu et al., 2019; Skripka et al., 2021). QDs demonstrate outstanding chemical and photochemical stability and a high fluorescence quantum yield in contrast to NIR detection. Tang et al. (2017) engineered biotin-aptamer-conjugated streptavidin-PEG-CdSe/ZnS QDs for in vitro and in vivo fluorescence imaging of gliomas based on specific EGFRvIII signaling. Besides, plasminogen activator receptor (uPAR) conjugated with NIR-II nanoprobe has been reported as a tool for real-time in vivo intraoperative visualization of GBM (Kurbegovic et al., 2018). Their results found a well-defined glioma margin with high fluorescence intensity as a preoperative marker. Among different QDs, carbon dots have attracted attention owing to their high biocompatibility and low toxicity, making them suitable for biomedical applications. Kirbas Cilingir et al. (2021) produced metformin-derived CDs and observed its multivariate bright fluorescence ability to cross the BBB and serve as an imaging agent for brain diseases, including the U87 cell model.
Gold and silver nanoparticles are employed to detect glioblastoma via surface-enhanced Raman scattering (SERS) (Indrasekara et al., 2013). Sonodynamic therapy is a novel promising theranostic for cancer, mainly when employed with oxygen-content nanomaterials, such as MnOx (Liang et al., 2020). Using ultrasound and a sonosensitizer that provides oxygen can solve the primary limitations of photodynamic therapy. Moreover, ultrasound deep and softly penetrates the tissues with controllable conditions to avoid targeted normal tissues and more specific to the tumoral cells (Zhang et al., 2021). Then, ultrasound imaging is a new alternative with the right sensitizers for bioimaging glioblastoma diagnostic studies (Bilmin et al., 2019; Carlos, 2022).
Liquid biopsy
Neuroimaging and biopsy have been extensively explored for the diagnosis and prognosis of brain cancers. Liquid biopsy (LB) using plasma or cerebrospinal fluid (CSF) is an easy and minimally invasive procedure (Ignatiadis et al., 2021). LB is an alternative to tissue extraction with a high invasiveness level, limited to a small tumor region, providing information through body fluids, usually complemented with clinical diagnosis (Saenz-Antoñanzas et al., 2019). LB enables real-time tumor monitoring, facilitating the early diagnosis and effective treatment of brain tumors due to the turnover and constant release of nucleic acids into circulation. In this context, DNA and RNA are used to characterize cancer, acting as biomarkers in LBs and improving the ability to describe the genomic profile of GBM.
LBs permit the identification of alterations in pathways, and the sensitivity of these assays depends on tumor volume, size, and type (Silantyev et al., 2019). For GBM, the BBB remains a challenge because of the decrease in tumor cell DNA and other cellular products in the systemic circulation (Saenz-Antoñanzas et al., 2019). Due to limitations in acquiring blood fluid under ideal conditions, CSF has been evaluated as it is near the brain tissues and presents a higher concentration of biomarkers than plasma. Recent studies have indicated that ultrasound applied with LB increases the concentration of markers, stimulating deep tissue penetration into the tumor noninvasively (Liu et al., 2019; Liang et al., 2020; An et al., 2021).
Many technologies based on microfluidics have been investigated to detect mutations and events that are difficult to identify using conventional imaging techniques (Xu et al., 2019). LB tends to be validated entirely and extensively used in routine clinical trials. Two specific and essential structures in GBM cells that are detectable via LB, namely EVs and microRNAs (miRNAs), are described below.
Extracellular vesicles
EVs play the most critical role in communication between cells. They are naturally occurring vesicles composed of a lipid bilayer with protein membranes containing aqueous core soluble proteins and nucleic acids (Zottel et al., 2019). EVs are considered important mediators of intercellular communication as biomarkers for disease diagnosis and evaluating therapeutic progress. GBM cells secrete many EV types, classified according to their diameter. Exosomes (30–100 nm, microvesicles (50 nm–1.5 μm), oncosomes (100–400 nm), apoptotic bodies (50 nm–2 μm), and large oncosomes (1–10 μm) have different size ranges (Silantyev et al., 2019). They can carry growth factors, receptors, enzymes, proteins, lipids, metabolites, different types of genetic material, immunomodulation molecules, and others, acting as excellent biomarkers. The smallest EVs, exosomes, can permeate different regions via body fluid (Silantyev et al., 2019; Stella et al., 2021). After that, they are internalized by endocytosis or cellular fusion; many molecules can improve these internalization processes. EVs can be used as tools that can cross the BBB, with an excellent capacity for target delivery (Schuurmans et al., 2020). For example, superparamagnetic iron oxide nanoparticles loaded with curcumin in exosomes conjugated with neuropilin-1 improved the imaging and therapy of GBM (Jia et al., 2019). EVs can be isolated from fluids, such as blood, urine, and saliva, presenting many advantages in detection and improving patients’ quality of life before a tumor reaches a more significant malignant potential (Figueroa and Carter, 2018).
MicroRNAs
miRNAs regulate the expression of many proteins of the EGFR signaling pathway and are noncoding RNAs composed of 20–22 nucleotides that negatively regulate gene expression by recognition, transcription, translation, and epigenetic processes (Xu et al., 2021). Different noncoding RNAs have been considered potential biomarkers for GBM as they are upregulated or downregulated in prognosis studies with GBM patients thereby becoming an essential diagnostic biomarker (Min et al., 2016; Xu et al., 2021). As it is detected in biofluids, miRNA stability is critical for control. To overcome this lack of stability, it is transported using nanoparticles. Studies using miRNA combined with a functionalized nanographene oxide conjugated model revealed that it exhibits considerably higher transfection efficiency, better inhibition of cancer cell growth, and is promising for diagnosis via molecular imaging (Cellot et al., 2021; Kutwin et al., 2021). Another strategy to improve miRNA stability is the association with EVs, developing an efficient delivery system through synthetically manufactured nano-sized vehicles (Saadatpour et al., 2016; Ebrahimkhani et al., 2017; Van Niel et al., 2018).
Limitations of using nanoparticles in GBM diagnosis
Significant concerns about the translation of nanoparticles to clinical practice are related to the biodistribution and safety of designing nanotechnology. Mainly because the toxicity of nanoparticles is not entirely understood after cell internalization and metabolization. Then, their physicochemical properties and the behavior of metallic particle liberation, which can change in body distribution, have to be better comprehended for a safe clinical trial with minimal toxic effects (Muthuraman et al., 2018). As mentioned before, in the case of inorganic nanoparticles, besides increasing the specificity, the nanoencapsulation strategy is crucial to decreasing the intrinsic toxicity of metals in different tissues (Mohammadpour et al., 2019; Khan M. A et al., 2021). For example, additional studies indicated that a range of 500–1,000 mg kg−1 of iron oxide could accumulate in vivo for a period between 5–13 weeks in the body and cause hepatic and renal dysfunctions (Katsnelson et al., 2011; Yun et al., 2015; Mohammadpour et al., 2019). However, these are overdoses compared to the minimal dose used for diagnostic purposes, as mentioned by Xie and coworkers, who used 0–50 μg ml−1 for their cytotoxicity studies (Xie et al., 2021).
Although these strategies and the doses differ in clinical translation, rigorous studies are still needed to well-design the clinical trials. Indeed, this is the reason why most nanodrugs for bioimaging are in the experimental stage, and most of the 50 nano probes approved by the FDA are composed of the classic agents: Gd-based, and iron-based contrasts (Bose et al., 2017; Ventola, 2017). To date, there are no nanoparticles approved by FDA as theranostic agents in clinical trials, which means ongoing development is necessary for a feature in which bioimaging nanomedicine can be possible besides the research environment (Han et al., 2019).
Conclusion
The complexity of GBM, its poor prognosis, and invasive biopsy methods highlight the need for new approaches to diagnosis. The design of specific and sensitive nanoparticles that can easily cross the BBB is a promising tool for advances in the early detection of GBM. Nanomaterial effectiveness is directly associated with specific GBM. EGFR is widely known as a crucial pathway for signaling a GBM cell, and several nanoparticles are designed to activate it, enabling multivariate nanodiagnostics using biomarkers, biofluids, and bioimages. Tf and other biomarkers contribute to the detection and poor prognosis of GBM. Nanomaterials are often designed to enhance signal sensibility and resolution in all techniques (CT, MRI, or NIR), such as QDs, carbon dots, and gold nanoparticles. Different diagnostic strategies are potential methods for the early detection of GBM.
Author contributions
LG, FM, and HB have made substantial and equally work, contributing to the writing, conceptualization, preparation of figures and tables, preparation, revision and gave the final approval to the manuscript. AT contributed to the work’s conceptualization, supervision, and final revision.
Acknowledgments
The authors are thankful to the Brazilian Federal Agency for Support and Evaluation of Graduate Education (CAPES), the State of Sao Paulo Research Foundation (FAPESP-Thematic project #2013/50181-1), FINEP project 01.10.0758.01, and the National Council for Scientific and Technological Development CNPq-MS-Innovative Platforms in Advanced Therapies. CNPq #441673/2020-1. We also thank PRONON-SIPAR Grants #25000.077093/2015-86 for the financial support. LG is a recipient of a CNPq doctorate fellowship (#142200/2020-4), FM is a recipient of a CNPq fellowship (#380163/2021-7), and HB is a FAPESP Post-Doc fellowship (#18/17911-0).
Conflict of interest
The authors declare that the research was conducted in the absence of any commercial or financial relationships that could be construed as a potential conflict of interest.
Publisher’s note
All claims expressed in this article are solely those of the authors and do not necessarily represent those of their affiliated organizations, or those of the publisher, the editors and the reviewers. Any product that may be evaluated in this article, or claim that may be made by its manufacturer, is not guaranteed or endorsed by the publisher.
References
An, Y., Fan, F., Jiang, X., and Sun, K. (2021). Recent advances in liquid biopsy of brain cancers. Front. Genet. 12, 720270. doi:10.3389/fgene.2021.720270
An, Z., Aksoy, O., Zheng, T., Fan, Q. W., and Weiss, W. A. (2018). Epidermal growth factor receptor and EGFRvIII in glioblastoma: Signaling pathways and targeted therapies. Oncogene 37, 1561–1575. doi:10.1038/s41388-017-0045-7
Ashrafizadeh, M., Mohammadinejad, R., Kailasa, S. K., Ahmadi, Z., Afshar, E. G., and Pardakhty, A. (2020). Carbon dots as versatile nanoarchitectures for the treatment of neurological disorders and their theranostic applications: A review. Adv. Colloid Interface Sci. 278, 102123–102212. doi:10.1016/j.cis.2020.102123
Barajas, R. F., Hamilton, B. E., Schwartz, D., Mcconnell, H. L., Pettersson, D. R., Horvath, A., et al. (2019). Combined iron oxide nanoparticle ferumoxytol and gadolinium contrast enhanced MRI define glioblastoma pseudoprogression. Neuro. Oncol. 21, 517–526. doi:10.1093/neuonc/noy160
Bilmin, K., Kujawska, T., and Grieb, P. (2019). Sonodynamic therapy for gliomas. Perspectives and prospects of selective sonosensitization of glioma cells. Cells 8, 1428. doi:10.3390/cells8111428
Bose, R. J. C., Ravikumar, R., Karuppagounder, V., Bennet, D., Rangasamy, S., and Thandavarayan, R. A. (2017). Lipid–polymer hybrid nanoparticle-mediated therapeutics delivery: advances and challenges. Drug Discov. Today 22, 1258–1265. doi:10.1016/j.drudis.2017.05.015
Brandt, J. V., Piazza, R. D., dos Santos, C. C., Vega-Chacón, J., Amantéa, B. E., Pinto, G. C., et al. (2019). Synthesis and colloidal characterization of folic acid-modified PEG-b-PCL Micelles for methotrexate delivery. Colloids Surfaces B Biointerfaces 177, 228–234. doi:10.1016/j.colsurfb.2019.02.008
Brennan, C. W., Verhaak, R. G. W., McKenna, A., Campos, B., Noushmehr, H., Salama, S. R., et al. (2013). The somatic genomic landscape of glioblastoma. Cell 155, 462–477. doi:10.1016/j.cell.2013.09.034
Cabral Filho, P. E., Cardoso, A. L. C., Pereira, M. I. A., Ramos, A. P. M., Hallwas, F., Castro, M. M. C. A., et al. (2016). CdTe quantum dots as fluorescent probes to study transferrin receptors in glioblastoma cells. Biochimica Biophysica Acta - General Subj. 1860, 28–35. doi:10.1016/j.bbagen.2015.09.021
Calzolari, A., Larocca, L. M., Deaglio, S., Finisguerra, V., Boe, A., Raggi, C., et al. (2010). Transferrin receptor 2 is frequently and highly expressed in glioblastomas. Transl. Oncol. 3, 123–134. doi:10.1593/tlo.09274
Carlos, L. D. (2022). Theranostic of orthotopic gliomas by core-shell structured nanoplatforms. Light. Sci. Appl. 11, 149. doi:10.1038/s41377-022-00852-2
Carvalho, I. C., Mansur, H. S., Mansur, A. A. P., Carvalho, S. M., de Oliveira, L. C. A., and de Fatima Leite, M. (2020). Luminescent switch of polysaccharide-peptide-quantum dot nanostructures for targeted-intracellular imaging of glioblastoma cells. J. Mol. Liq. 304, 112759. doi:10.1016/j.molliq.2020.112759
Carvalho, J. A., da Silva Abreu, A., Tedesco, A. C., Junior, M. B., and Simioni, A. R. (2019). Functionalized photosensitive gelatin nanoparticles for drug delivery application. J. Biomaterials Sci. Polym. Ed. 30, 508–525. doi:10.1080/09205063.2019.1580664
Cellot, G., Franceschi Biagioni, A., and Ballerini, L. (2021). Nanomedicine and graphene-based materials: advanced technologies for potential treatments of diseases in the developing nervous system. Pediatr. Res.. 11, 1–15. doi:10.1038/s41390-021-01681-6
Chandran, V. I., Welinder, C., Mansson, A. S., Offer, S., Freyhult, E., Pernemalm, M., et al. (2019). Ultrasensitive immunoprofiling of plasma extracellular vesicles identifies syndecan-1 as a potential tool for minimally invasive diagnosis of glioma. Clin. Cancer Res. 25, 3115–3127. doi:10.1158/1078-0432.CCR-18-2946
Cho, J. H., Kim, A. R., Kim, S. H., Lee, S. J., Chung, H., and Yoon, M. Y. (2017). Development of a novel imaging agent using peptide-coated gold nanoparticles toward brain glioma stem cell marker CD133. Acta Biomater. 47, 182–192. doi:10.1016/j.actbio.2016.10.009
Choi, J., Yang, J., Park, J., Kim, E., Suh, J. S., Huh, Y. M., et al. (2011). Specific near-IR absorption imaging of glioblastomas using integrin-targeting gold nanorods. Adv. Funct. Mat. 21, 1082–1088. doi:10.1002/adfm.201002253
Cross, S. J. L., Kay, E., and Leslie, F. M. (2016). Dual functionalized 5-fluorouracil liposomes as highly efficient nanomedicine for glioblastoma treatment as assessed in an in vitro brain tumor model sushant. Physiol. Behav. 176, 100–106. doi:10.1016/j.xphs.2018.07.020
De Pasquale, D., Marino, A., Tapeinos, C., Pucci, C., Rocchiccioli, S., Michelucci, E., et al. (2020). Homotypic targeting and drug delivery in glioblastoma cells through cell membrane-coated boron nitride nanotubes. Mat. Des. 192, 108742. doi:10.1016/j.matdes.2020.108742
de Paula, L. B., Primo, F. L., and Tedesco, A. C. (2017). Nanomedicine associated with photodynamic therapy for glioblastoma treatment. Biophys. Rev. 9, 761–773. doi:10.1007/s12551-017-0293-3
do Nascimento, T., Tavares, M., Monteiro, M. S. S. B., Santos-Oliveira, R., Todeschini, A. R., de Souza, V. T., et al. (2020). Trends in nanotechnology for in vivo cancer diagnosis: Products and patents. Curr. Pharm. Des. 26, 2167–2181. doi:10.2174/1381612826666200219094853
Ebrahimkhani, S., Vafaee, F., Young, P. E., Hur, S. S. J., Hawke, S., Devenney, E., et al. (2017). Exosomal microRNA signatures in multiple sclerosis reflect disease status. Sci. Rep. 7, 14293. doi:10.1038/s41598-017-14301-3
Fechter, P., Cruz Da Silva, E., Mercier, M. C., Noulet, F., Etienne-Seloum, N., Guenot, D., et al. (2019). RNA aptamers targeting integrin α5β1 as probes for cyto- and histofluorescence in glioblastoma. Mol. Ther. - Nucleic Acids 17, 63–77. doi:10.1016/j.omtn.2019.05.006
Figueroa, J. M., and Carter, B. S. (2018). Detection of glioblastoma in biofluids. J. Neurosurg. 129, 334–340. doi:10.3171/2017.3.JNS162280
Fillmore, H. L., Shultz, M. D., Henderson, S. C., Cooper, P., Broaddus, W. C., Chen, Z. J., et al. (2011). Conjugation of functionalized gadolinium metallofullerenes with IL-13 peptides for targeting and imaging glial tumors. Nanomedicine 6, 449–458. doi:10.2217/nnm.10.134
Finch, A., Solomou, G., Wykes, V., Pohl, U., Bardella, C., and Watts, C. (2021). Advances in research of adult gliomas. Int. J. Mol. Sci. 22, 924–936. doi:10.3390/ijms22020924
Ganau, M., Paris, M., Syrmos, N., Ganau, L., Ligarotti, G., Moghaddamjou, A., et al. (2018). How nanotechnology and biomedical engineering are supporting the identification of predictive biomarkers in neuro-oncology. Medicines 5, 23. doi:10.3390/medicines5010023
Glaser, T., Han, I., Wu, L., and Zeng, X. (2017). Targeted nanotechnology in glioblastoma multiforme. Front. Pharmacol. 8, 166–214. doi:10.3389/fphar.2017.00166
Hadjipanayis, C. G., Machaidze, R., Kaluzova, M., Wang, L., Schuette, A. J., Chen, H., et al. (2010). EGFRvIII antibody-conjugated iron oxide nanoparticles for magnetic resonance imaging-guided convection-enhanced delivery and targeted therapy of glioblastoma. Cancer Res. 70, 6303–6312. doi:10.1158/0008-5472.CAN-10-1022
Hallal, S., Azimi, A., Wei, H., Ho, N., Lee, M. Y. T., Sim, H. W., et al. (2020). A comprehensive proteomic SWATH-MS workflow for profiling blood extracellular vesicles: A new avenue for glioma tumour surveillance. Int. J. Mol. Sci. 21, 4754–4826. doi:10.3390/ijms21134754
Han, X., Xu, K., Taratula, O., and Farsad, K. (2019). Applications of nanoparticles in biomedical imaging. Nanoscale 11, 799–819. doi:10.1039/C8NR07769J
Hanif, F., Muzaffar, K., Perveen, K., Malhi, S. M., and Simjee, S. U. (2017). Glioblastoma multiforme: A review of its epidemiology and pathogenesis through clinical presentation and treatment. Asian Pac. J. Cancer Prev. 18, 3–9. doi:10.22034/APJCP.2017.18.1.3
Hassn Mesrati, M., Behrooz, A. B., Y Abuhamad, A., and Syahir, A. (2020). Understanding glioblastoma biomarkers: Knocking a mountain with a hammer. Cells 9, 1236. doi:10.3390/cells9051236
Hatanpaa, K. J., Burma, S., Zhao, D., and Habib, A. A. (2010). Epidermal growth factor receptor in glioma: Signal transduction, neuropathology, imaging, and radioresistance. Neoplasia 12, 675–684. doi:10.1593/neo.10688
He, X., Ma, Y., Xie, H., Rao, G., Yang, Z., Zhang, J., et al. (2021). Biomimetic nanostructure platform for cancer diagnosis based on tumor biomarkers. Front. Bioeng. Biotechnol. 9, 687664. doi:10.3389/fbioe.2021.687664
Hettie, K. S., Klockow, J. L., Moon, E. J., Giaccia, A. J., and Chin, F. T. (2021). A NIR fluorescent smart probe for imaging tumor hypoxia. Cancer Rep. 4, e1384–10. doi:10.1002/cnr2.1384
Hsu, J.-F., Chu, S.-M., Liao, C.-C., Wang, C.-J., Wang, Y.-S., Lai, M.-Y., et al. (2021). Nanotechnology and nanocarrier-based drug delivery as the potential therapeutic strategy for glioblastoma multiforme: An update. Cancers (Basel). 13, 195. doi:10.3390/cancers13020195
Ignatiadis, M., Sledge, G. W., and Jeffrey, S. S. (2021). Liquid biopsy enters the clinic — implementation issues and future challenges. Nat. Rev. Clin. Oncol. 18, 297–312. doi:10.1038/s41571-020-00457-x
Indrasekara, A. S. D. S., Paladini, B. J., Naczynski, D. J., Starovoytov, V., Moghe, P. V., and Fabris, L. (2013). Dimeric gold nanoparticle assemblies as tags for SERS-based cancer detection. Adv. Healthc. Mat. 2, 1370–1376. doi:10.1002/adhm.201200370
Jafari, S. H., Saadatpour, Z., Salmaninejad, A., Momeni, F., Mokhtari, M., Nahand, J. S., et al. (2018). Breast cancer diagnosis: Imaging techniques and biochemical markers. J. Cell. Physiol. 233, 5200–5213. doi:10.1002/jcp.26379
Jhaveri, A., Luther, E., and Torchilin, V. (2019). The effect of transferrin-targeted, resveratrol-loaded liposomes on neurosphere cultures of glioblastoma: implications for targeting tumour-initiating cells. J. Drug Target. 27, 601–613. doi:10.1080/1061186X.2018.1550647
Ji, Z., Ai, P., Shao, C., Wang, T., Yan, C., Ye, L., et al. (2018). Manganese-doped carbon dots for magnetic resonance/optical dual-modal imaging of tiny brain glioma. ACS Biomater. Sci. Eng. 4, 2089–2094. doi:10.1021/acsbiomaterials.7b01008
Jia, Y., Wang, X., Hu, D., Wang, P., Liu, Q., Zhang, X., et al. (2019). Phototheranostics: Active targeting of orthotopic glioma using biomimetic proteolipid nanoparticles. ACS Nano 13, 386–398. doi:10.1021/acsnano.8b06556
Jin, C., Wang, K., Oppong-Gyebi, A., and Hu, J. (2020). Application of nanotechnology in cancer diagnosis and therapy - a mini-review. Int. J. Med. Sci. 17, 2964–2973. doi:10.7150/ijms.49801
Jin, J., and Bhujwalla, Z. M. (2020). Biomimetic nanoparticles camouflaged in cancer cell membranes and their applications in cancer theranostics. Front. Oncol. 9, 1560. doi:10.3389/fonc.2019.01560
Kadry, H., Noorani, B., and Cucullo, L. (2020). A blood–brain barrier overview on structure, function, impairment, and biomarkers of integrity. Fluids Barriers CNS 17, 69–24. doi:10.1186/s12987-020-00230-3
Kang, T., Jiang, M., Jiang, D., Feng, X., Yao, J., Song, Q., et al. (2015). Enhancing glioblastoma-specific penetration by functionalization of nanoparticles with an iron-mimic peptide targeting transferrin/transferrin receptor complex. Mol. Pharm. 12, 2947–2961. doi:10.1021/acs.molpharmaceut.5b00222
Katsnelson, B. A., Degtyareva, T. D., Minigalieva, I. I., Privalova, L. I., Kuzmin, S. V., Yeremenko, O. S., et al. (2011). Subchronic systemic toxicity and bioaccumulation of Fe 3 O 4 nano- and microparticles following repeated intraperitoneal administration to rats. Int. J. Toxicol. 30, 59–68. doi:10.1177/1091581810385149
Khan, M. A., Singh, D., Ahmad, A., and Siddique, H. R. (2021). Revisiting inorganic nanoparticles as promising therapeutic agents: A paradigm shift in oncological theranostics. Eur. J. Pharm. Sci. 164, 105892. doi:10.1016/j.ejps.2021.105892
Khan, S., Babadaei, M. M. N., Hasan, A., Edis, Z., Attar, F., Siddique, R., et al. (2021). Enzyme–polymeric/inorganic metal oxide/hybrid nanoparticle bio-conjugates in the development of therapeutic and biosensing platforms. J. Adv. Res. 33, 227–239. doi:10.1016/j.jare.2021.01.012
Kim, G. B., Aragon-Sanabria, V., Randolph, L., Jiang, H., Reynolds, J. A., Webb, B. S., et al. (2020). High-affinity mutant Interleukin-13 targeted CAR T cells enhance delivery of clickable biodegradable fluorescent nanoparticles to glioblastoma. Bioact. Mat. 5, 624–635. doi:10.1016/j.bioactmat.2020.04.011
Kim, J. S., Shin, D. H., and Kim, J. S. (2018). Dual-targeting immunoliposomes using angiopep-2 and CD133 antibody for glioblastoma stem cells. J. Control. Release 269, 245–257. doi:10.1016/j.jconrel.2017.11.026
Kirbas Cilingir, E., Seven, E. S., Zhou, Y., Walters, B. M., Mintz, K. J., Pandey, R. R., et al. (2021). Metformin derived carbon dots: Highly biocompatible fluorescent nanomaterials as mitochondrial targeting and blood-brain barrier penetrating biomarkers. J. Colloid Interface Sci. 592, 485–497. doi:10.1016/j.jcis.2021.02.058
Klekner, Á., Szivos, L., Virga, J., Árkosy, P., Bognár, L., Birkó, Z., et al. (2019). Significance of liquid biopsy in glioblastoma – a review. J. Biotechnol. 298, 82–87. doi:10.1016/j.jbiotec.2019.04.011
Kurbegovic, S., Juhl, K., Chen, H., Qu, C., Ding, B., Leth, J. M., et al. (2018). Molecular targeted NIR-II probe for image-guided brain tumor surgery. Bioconjug. Chem. 29, 3833–3840. doi:10.1021/acs.bioconjchem.8b00669
Kutwin, M., Sosnowska, M. E., Strojny-Cieślak, B., Jaworski, S., Trzaskowski, M., Wierzbicki, M., et al. (2021). Microrna delivery by graphene-based complexes into glioblastoma cells. Molecules 26, 5804. doi:10.3390/molecules26195804
Lakkadwala, S., and Singh, J. (2019). Co-delivery of doxorubicin and erlotinib through liposomal nanoparticles for glioblastoma tumor regression using an in vitro brain tumor model. Colloids Surfaces B Biointerfaces 173, 27–35. doi:10.1016/j.colsurfb.2018.09.047
Lane, R., Simon, T., Vintu, M., Solkin, B., Koch, B., Stewart, N., et al. (2019). Cell-derived extracellular vesicles can be used as a biomarker reservoir for glioblastoma tumor subtyping. Commun. Biol. 2, 315. doi:10.1038/s42003-019-0560-x
Lee, G. A., Lin, W. L., Kuo, D. P., Li, Y. T., Chang, Y. W., Chen, Y. C., et al. (2021). Detection of pd-l1 expression in temozolomide-resistant glioblastoma by using pd-l1 antibodies conjugated with lipid-coated superparamagnetic iron oxide. Int. J. Nanomedicine 16, 5233–5246. doi:10.2147/IJN.S310464
Li, M., Luo, Z., and Zhao, Y. (2018). Self-assembled hybrid nanostructures: Versatile multifunctional nanoplatforms for cancer diagnosis and therapy. Chem. Mat. 30, 25–53. doi:10.1021/acs.chemmater.7b03924
Li, S., Johnson, J., Peck, A., and Xie, Q. (2017). Near infrared fluorescent imaging of brain tumor with IR780 dye incorporated phospholipid nanoparticles. J. Transl. Med. 15, 18–12. doi:10.1186/s12967-016-1115-2
Li, T., Murphy, S., Kiselev, B., Bakshi, K. S., Zhang, J., Eltahir, A., et al. (2015). A new interleukin-13 amino-coated gadolinium metallofullerene nanoparticle for targeted MRI detection of glioblastoma tumor cells. J. Am. Chem. Soc. 137, 7881–7888. doi:10.1021/jacs.5b03991
Liang, K., Li, Z., Luo, Y., Zhang, Q., Yin, F., Xu, L., et al. (2020). Intelligent nanocomposites with intrinsic blood–brain-barrier crossing ability designed for highly specific MR imaging and sonodynamic therapy of glioblastoma. Small 16, 1906985–1907012. doi:10.1002/smll.201906985
Liu, Z., Ren, F., Zhang, H., Yuan, Q., Jiang, Z., Liu, H., et al. (2019). Boosting often overlooked long wavelength emissions of rare-earth nanoparticles for NIR-II fluorescence imaging of orthotopic glioblastoma. Biomaterials 219, 119364. doi:10.1016/j.biomaterials.2019.119364
Louis, D. N., Perry, A., Reifenberger, G., von Deimling, A., Figarella-Branger, D., Cavenee, W. K., et al. (2016). The 2016 world Health organization classification of tumors of the central nervous system: a summary. Acta Neuropathol. 131, 803–820. doi:10.1007/s00401-016-1545-1
Luo, M., Lewik, G., Ratcliffe, J. C., Choi, C. H. J., Mäkilä, E., Tong, W. Y., et al. (2019). Systematic evaluation of transferrin-modified porous silicon nanoparticles for targeted delivery of doxorubicin to glioblastoma. ACS Appl. Mat. Interfaces 11, 33637–33649. doi:10.1021/acsami.9b10787
Mansur, A. A. P., Caires, A. J., Carvalho, S. M., Capanema, N. S. V., Carvalho, I. C., and Mansur, H. S. (2019). Dual-functional supramolecular nanohybrids of quantum dot/biopolymer/chemotherapeutic drug for bioimaging and killing brain cancer cells in vitro. Colloids Surfaces B Biointerfaces 184, 110507. doi:10.1016/j.colsurfb.2019.110507
Miklaszewska, M., Korohoda, P., Drożdż, D., Zachwieja, K., Tomasik, T., Moczulska, A., et al. (2019). eGFR values and selected renal urine biomarkers in preterm neonates with uncomplicated clinical course. Adv. Clin. Exp. Med. 28, 1657–1666. doi:10.17219/acem/110317
Min, W., Dai, D., Wang, J., Zhang, D., Zhang, Y., Han, G., et al. (2016). Long noncoding RNA miR210HG as a potential biomarker for the diagnosis of glioma. PLoS One 11, e0160451–11. doi:10.1371/journal.pone.0160451
Mohammadpour, R., Dobrovolskaia, M. A., Cheney, D. L., Greish, K. F., and Ghandehari, H. (2019). Subchronic and chronic toxicity evaluation of inorganic nanoparticles for delivery applications. Adv. Drug Deliv. Rev. 144, 112–132. doi:10.1016/j.addr.2019.07.006
Mu, K., Zhang, S., Ai, T., Jiang, J., Yao, Y., Jiang, L., et al. (2015). Monoclonal antibody-conjugated superparamagnetic iron oxide nanoparticles for imaging of epidermal growth factor receptor-targeted cells and gliomas. Mol. Imaging 14, 1–12. doi:10.2310/7290.2015.00002
Mukherjee, A., Waters, A. K., Kalyan, P., Achrol, A. S., Kesari, S., and Yenugonda, V. M. (2019). Lipid–polymer hybrid nanoparticles as a next-generation drug delivery platform: state of the art, emerging technologies, and perspectives. Int. J. Nanomedicine 14, 1937–1952. doi:10.2147/IJN.S198353
Mukhtar, M., Bilal, M., Rahdar, A., Barani, M., Arshad, R., Behl, T., et al. (2020). Nanomaterials for diagnosis and treatment of brain cancer: Recent updates. Chemosensors 8, 117–131. doi:10.3390/chemosensors8040117
Muthuraman, A., Rishitha, N., and Mehdi, S. (2018). “Role of nanoparticles in bioimaging, diagnosis and treatment of cancer disorder,” in Design of nanostructures for theranostics applications (Netherlands: Elsevier), 529–562. doi:10.1016/B978-0-12-813669-0.00013-0
Newman, J. P., Wang, G. Y., Arima, K., Guan, S. P., Waters, M. R., Cavenee, W. K., et al. (2017). Interleukin-13 receptor alpha 2 cooperates with EGFRvIII signaling to promote glioblastoma multiforme. Nat. Commun. 8, 1913. doi:10.1038/s41467-017-01392-9
Ni, X. R., Zhao, Y. Y., Cai, H. P., Yu, Z. H., Wang, J., Chen, F. R., et al. (2020). Transferrin receptor 1 targeted optical imaging for identifying glioma margin in mouse models. J. Neurooncol. 148, 245–258. doi:10.1007/s11060-020-03527-3
Núñez, C., Estévez, S. V., and del Pilar Chantada, M. (2018). Inorganic nanoparticles in diagnosis and treatment of breast cancer. J. Biol. Inorg. Chem. 23, 331–345. doi:10.1007/s00775-018-1542-z
Oprita, A., Baloi, S. C., Staicu, G. A., Alexandru, O., Tache, D. E., Danoiu, S., et al. (2021). Updated insights on EGFR signaling pathways in glioma. Int. J. Mol. Sci. 22, 587–621. doi:10.3390/ijms22020587
Oronsky, B., Reid, T. R., Oronsky, A., Sandhu, N., and Knox, S. J. (2021). A review of newly diagnosed glioblastoma. Front. Oncol. 10, 574012–574110. doi:10.3389/fonc.2020.574012
Poon, M. T. C., Sudlow, C. L. M., Figueroa, J. D., and Brennan, P. M. (2020). Longer-term (≥ 2 years) survival in patients with glioblastoma in population-based studies pre- and post-2005: a systematic review and meta-analysis. Sci. Rep. 10, 11622. doi:10.1038/s41598-020-68011-4
Qi, F., Li, Q., Lu, X., and Chen, Z. (2020). Bioinformatics analysis of high-throughput data to validate potential novel biomarkers and small molecule drugs for glioblastoma multiforme. J. Int. Med. Res. 48, 030006052092454. doi:10.1177/0300060520924541
Ren, F., Liu, H., Zhang, H., Jiang, Z., Xia, B., Genevois, C., et al. (2020). Engineering NIR-IIb fluorescence of Er-based lanthanide nanoparticles for through-skull targeted imaging and imaging-guided surgery of orthotopic glioma. Nano Today 34, 100905–100906. doi:10.1016/j.nantod.2020.100905
Rizvi, S. F. A., Ali, A., Ahmad, M., Mu, S., and Zhang, H. (2021). Multifunctional self-assembled peptide nanoparticles for multimodal imaging-guided enhanced theranostic applications against glioblastoma multiforme. Nanoscale Adv. 3, 5959–5967. doi:10.1039/d1na00597a
Saadatpour, L., Fadaee, E., Fadaei, S., Nassiri Mansour, R., Mohammadi, M., Mousavi, S. M., et al. (2016). Glioblastoma: Exosome and microRNA as novel diagnosis biomarkers. Cancer Gene Ther. 23, 415–418. doi:10.1038/cgt.2016.48
Saenz-Antoñanzas, A., Auzmendi-Iriarte, J., Carrasco-Garcia, E., Moreno-Cugnon, L., Ruiz, I., Villanua, J., et al. (2019). Liquid biopsy in glioblastoma: Opportunities, applications and challenges. Cancers (Basel) 11, 950. doi:10.3390/cancers11070950
Schuurmans, C., Balakrishnan, A., Roy, S., Fleming, T., and Leong, H. S. (2020). The emerging role of extracellular vesicles in the glioma microenvironment: Biogenesis and clinical relevance. Cancers (Basel) 12, 1964–2025. doi:10.3390/cancers12071964
Seleci, D. A., Maurer, V., Barlas, F. B., Porsiel, J. C., Temel, B., Ceylan, E., et al. (2021). Transferrin-decorated niosomes with integrated inp/zns quantum dots and magnetic iron oxide nanoparticles: Dual targeting and imaging of glioma. Int. J. Mol. Sci. 22, 4556. doi:10.3390/ijms22094556
Sibilia, M., Fleischmann, A., Behrens, A., Stingl, L., Carroll, J., Watt, F. M., et al. (2000). The EGF receptor provides an essential survival signal for SOS-dependent skin tumor development. Cell 102, 211–220. doi:10.1016/S0092-8674(00)00026-X
Silantyev, A. S., Falzone, L., Libra, M., Gurina, O. I., Kardashova, K. S., Nikolouzakis, T. K., et al. (2019). Current and future trends on diagnosis and prognosis of glioblastoma: From molecular biology to proteomics. Cells 8, 863. doi:10.3390/cells8080863
Simionescu, N., Zonda, R., Petrovici, A. R., and Georgescu, A. (2021). The multifaceted role of extracellular vesicles in glioblastoma: Microrna nanocarriers for disease progression and gene therapy. Pharmaceutics 13, 988. doi:10.3390/pharmaceutics13070988
Skripka, A., Mendez-Gonzalez, D., Marin, R., Ximendes, E., del Rosal, B., Jaque, D., et al. (2021). Near infrared bioimaging and biosensing with semiconductor and rare-earth nanoparticles: recent developments in multifunctional nanomaterials. Nanoscale Adv. 3, 6310–6329. doi:10.1039/d1na00502b
Smith, E. S., Porterfield, J. E., and Kannan, R. M. (2019). Leveraging the interplay of nanotechnology and neuroscience: Designing new avenues for treating central nervous system disorders. Adv. Drug Deliv. Rev. 148, 181–203. doi:10.1016/j.addr.2019.02.009
Song, C., Ouyang, Z., Gao, Y., Guo, H., Wang, S., Wang, D., et al. (2021). Modular design of multifunctional core-shell tecto dendrimers complexed with copper(II) for MR imaging-guided chemodynamic therapy of orthotopic glioma. Nano Today 41, 101325. doi:10.1016/j.nantod.2021.101325
Steeg, P. S. (2021). The blood–tumour barrier in cancer biology and therapy. Nat. Rev. Clin. Oncol. 18, 696–714. doi:10.1038/s41571-021-00529-6
Stella, M., Falzone, L., Caponnetto, A., Gattuso, G., Barbagallo, C., Battaglia, R., et al. (2021). Serum extracellular vesicle-derived circhipk3 and circsmarca5 are two novel diagnostic biomarkers for glioblastoma multiforme. Pharmaceuticals 14, 618–712. doi:10.3390/ph14070618
Su, C. Y., Li, J. Q., Zhang, L. L., Wang, H., Wang, F. H., Tao, Y. W., et al. (2020). The biological functions and clinical applications of integrins in cancers. Front. Pharmacol. 11, 579068–579114. doi:10.3389/fphar.2020.579068
Su, Y.-L., and Hu, S.-H. (2018). Functional nanoparticles for tumor penetration of therapeutics. Pharmaceutics 10, 193. doi:10.3390/pharmaceutics10040193
Sun, X., Huang, X., Guo, J., Zhu, W., Ding, Y., Niu, G., et al. (2014). Self-illuminating 64 Cu-doped CdSe/ZnS nanocrystals for in vivo tumor imaging. J. Am. Chem. Soc. 136, 1706–1709. doi:10.1021/ja410438n
Szopa, W., Burley, T. A., Kramer-Marek, G., and Kaspera, W. (2017). Diagnostic and therapeutic biomarkers in glioblastoma: Current status and future perspectives. Biomed. Res. Int. 2017, 1–13. doi:10.1155/2017/8013575
Taal, W., Brandsma, D., De Bruin, H. G., Bromberg, J. E., Swaak-Kragten, A. T., Sillevis Smitt, P. A. E., et al. (2008). Incidence of early pseudo-progression in a cohort of malignant glioma patients treated with chemoirradiation with temozolomide. Cancer 113, 405–410. doi:10.1002/cncr.23562
Talukdar, S., Emdad, L., Das, S. K., and Fisher, P. B. (2020). EGFR: An essential receptor tyrosine kinase-regulator of cancer stem cells. 1st ed. Netherlands: Elsevier. doi:10.1016/bs.acr.2020.04.003
Tan, S. K., Pastori, C., Penas, C., Komotar, R. J., Ivan, M. E., Wahlestedt, C., et al. (2018). Serum long noncoding RNA HOTAIR as a novel diagnostic and prognostic biomarker in glioblastoma multiforme. Mol. Cancer 17, 74–77. doi:10.1186/s12943-018-0822-0
Tang, J., Huang, N., Zhang, X., Zhou, T., Tan, Y., Pi, J., et al. (2017). Aptamer-conjugated PEGylated quantum dots targeting epidermal growth factor receptor variant III for fluorescence imaging of glioma. Int. J. Nanomedicine 12, 3899–3911. doi:10.2147/IJN.S133166
Tang, X., Zuo, C., Fang, P., Liu, G., Qiu, Y., Huang, Y., et al. (2021). Targeting glioblastoma stem cells: A review on biomarkers, signal pathways and targeted therapy. Front. Oncol. 11, 701291. doi:10.3389/fonc.2021.701291
Tapeinos, C., Marino, A., Battaglini, M., Migliorin, S., Brescia, R., Scarpellini, A., et al. (2019). Stimuli-responsive lipid-based magnetic nanovectors increase apoptosis in glioblastoma cells through synergic intracellular hyperthermia and chemotherapy. Nanoscale 11, 72–88. doi:10.1039/C8NR05520C
Thomas, L., Florio, T., and Perez-Castro, C. (2020). Extracellular vesicles loaded miRNAs as potential modulators shared between glioblastoma, and Parkinson’s and alzheimer’s diseases. Front. Cell. Neurosci. 14, 590034. doi:10.3389/fncel.2020.590034
Van Niel, G., D’Angelo, G., and Raposo, G. (2018). Shedding light on the cell biology of extracellular vesicles. Nat. Rev. Mol. Cell Biol. 19, 213–228. doi:10.1038/nrm.2017.125
Ventola, C. L. (2017). Progress in nanomedicine: Approved and investigational nanodrugs. P T 42, 742–755. Available at:http://www.ncbi.nlm.nih.gov/pubmed/29234213.
Verhaak, R. G. W., Hoadley, K. A., Purdom, E., Wang, V., Qi, Y., Wilkerson, M. D., et al. (2010). Integrated genomic analysis identifies clinically relevant subtypes of glioblastoma characterized by abnormalities in PDGFRA, IDH1, EGFR, and NF1. Cancer Cell 17, 98–110. doi:10.1016/j.ccr.2009.12.020
Wadajkar, A. S., Dancy, J. G., Hersh, D. S., Anastasiadis, P., Tran, N. L., Woodworth, G. F., et al. (2017). Tumor‐targeted nanotherapeutics: overcoming treatment barriers for glioblastoma. Wiley Interdiscip. Rev. Nanomed. Nanobiotechnol. 9, e1439. doi:10.1002/wnan.1439
Wang, H., Mu, Q., Wang, K., Revia, R. A., Yen, C., Gu, X., et al. (2019). Nitrogen and boron dual-doped graphene quantum dots for near-infrared second window imaging and photothermal therapy. Appl. Mat. Today 14, 108–117. doi:10.1016/j.apmt.2018.11.011
Wang, H., Wang, X., Xu, L., Zhang, J., and Cao, H. (2021). Analysis of the EGFR amplification and CDKN2A deletion regulated transcriptomic signatures reveals the prognostic significance of SPATS2L in patients with glioma. Front. Oncol. 11, 551160–551215. doi:10.3389/fonc.2021.551160
Weerathunge, P., Pooja, D., Singh, M., Kulhari, H., Mayes, E. L. H., Bansal, V., et al. (2019). Transferrin-conjugated quasi-cubic SPIONs for cellular receptor profiling and detection of brain cancer. Sensors Actuators B Chem. 297, 126737. doi:10.1016/j.snb.2019.126737
Weller, M., van den Bent, M., Preusser, M., Le Rhun, E., Tonn, J. C., Minniti, G., et al. (2021). EANO guidelines on the diagnosis and treatment of diffuse gliomas of adulthood. Nat. Rev. Clin. Oncol. 18, 170–186. doi:10.1038/s41571-020-00447-z
Wen, P. Y., Macdonald, D. R., Reardon, D. A., Cloughesy, T. F., Sorensen, A. G., Galanis, E., et al. (2010). Updated response assessment criteria for high-grade gliomas: Response assessment in neuro-oncology working group. J. Clin. Oncol. 28, 1963–1972. doi:10.1200/JCO.2009.26.3541
Wu, X., Yang, H., Yang, W., Chen, X., Gao, J., Gong, X., et al. (2019). Nanoparticle-based diagnostic and therapeutic systems for brain tumors. J. Mat. Chem. B 7, 4734–4750. doi:10.1039/c9tb00860h
Xie, R., Wu, Z., Zeng, F., Cai, H., Wang, D., Gu, L., et al. (2021). Retro-enantio isomer of angiopep-2 assists nanoprobes across the blood-brain barrier for targeted magnetic resonance/fluorescence imaging of glioblastoma. Signal Transduct. Target. Ther. 6, 309. doi:10.1038/s41392-021-00724-y
Xu, B., Mei, J., Ji, W., Huo, Z., Bian, Z., Jiao, J., et al. (2021). MicroRNAs involved in the EGFR pathway in glioblastoma. Biomed. Pharmacother. 134, 111115. doi:10.1016/j.biopha.2020.111115
Xu, Z., Qiao, Y., and Tu, J. (2019). Microfluidic technologies for cfDNA isolation and analysis. Micromachines 10, 672. doi:10.3390/mi10100672
Yekula, A., Minciacchi, V. R., Morello, M., Shao, H., Park, Y., Zhang, X., et al. (2020). Large and small extracellular vesicles released by glioma cells in vitro and in vivo. J. Extracell. Vesicles 9, 1689784. doi:10.1080/20013078.2019.1689784
Yong, T., Zhang, X., Bie, N., Zhang, H., Zhang, X., Li, F., et al. (2019). Tumor exosome-based nanoparticles are efficient drug carriers for chemotherapy. Nat. Commun. 10, 3838. doi:10.1038/s41467-019-11718-4
Yoshikawa, T., Mori, Y., Feng, H., Phan, K. Q., Kishimura, A., Kang, J. H., et al. (2019). Rapid and continuous accumulation of nitric oxide-releasing liposomes in tumors to augment the enhanced permeability and retention (EPR) effect. Int. J. Pharm. X. 565, 481–487. doi:10.1016/j.ijpharm.2019.05.043
Yun, J.-W., Kim, S.-H., You, J.-R., Kim, W. H., Jang, J.-J., Min, S.-K., et al. (2015). Comparative toxicity of silicon dioxide, silver and iron oxide nanoparticles after repeated oral administration to rats. J. Appl. Toxicol. 35, 681–693. doi:10.1002/jat.3125
Zhang, Y., Li, M., Gao, X., Chen, Y., and Liu, T. (2019). Nanotechnology in cancer diagnosis: Progress, challenges and opportunities. J. Hematol. Oncol. 12, 137–213. doi:10.1186/s13045-019-0833-3
Zhang, Y., Zhang, X., Yang, H., Yu, L., Xu, Y., Sharma, A., et al. (2021). Advanced biotechnology-assisted precise sonodynamic therapy. Chem. Soc. Rev. 50, 11227–11248. doi:10.1039/D1CS00403D
Zhou, H., Ge, J., Miao, Q., Zhu, R., Wen, L., Zeng, J., et al. (2020). Biodegradable inorganic nanoparticles for cancer theranostics: Insights into the degradation behavior. Bioconjug. Chem. 31, 315–331. doi:10.1021/acs.bioconjchem.9b00699
Zhou, Y., Peng, Z., Seven, E. S., and Leblanc, R. M. (2018). Crossing the blood-brain barrier with nanoparticles. J. Control. Release 270, 290–303. doi:10.1016/j.jconrel.2017.12.015
Keywords: diagnosis, glioblastoma multiforme, nanotechnology, biomolecular pathways, EGFR
Citation: Gusmão LA, Matsuo FS, Barbosa HFG and Tedesco AC (2022) Advances in nano-based materials for glioblastoma multiforme diagnosis: A mini-review. Front. Nanotechnol. 4:836802. doi: 10.3389/fnano.2022.836802
Received: 15 December 2021; Accepted: 05 August 2022;
Published: 31 August 2022.
Edited by:
Zhi Ping (Gordon) Xu, The University of Queensland, AustraliaReviewed by:
Attilio Marino, Italian Institute of Technology (IIT), ItalyYiqun Zhou, C-Dots, LLC, United States
Bapu Surnar, University of Miami Health System, United States
Copyright © 2022 Gusmão, Matsuo, Barbosa and Tedesco. This is an open-access article distributed under the terms of the Creative Commons Attribution License (CC BY). The use, distribution or reproduction in other forums is permitted, provided the original author(s) and the copyright owner(s) are credited and that the original publication in this journal is cited, in accordance with accepted academic practice. No use, distribution or reproduction is permitted which does not comply with these terms.
*Correspondence: Antonio Claudio Tedesco, atedesco@usp.br
† These authors have contributed equally to this work and share first authorship