- 1Department of Physiology, University of Toronto, Toronto, ON, Canada
- 2Department of Obstetrics and Gynaecology, University of Toronto, Toronto, ON, Canada
- 3Department of Medicine, University of Toronto, Toronto, ON, Canada
Phoenixin (PNX) is a newly identified reproductive peptide required for the estrous cycle. It is most highly expressed in the hypothalamus, where it is a positive regulator of gonadotropin-releasing hormone (GnRH) and kisspeptin. However, it is unknown what signals lie upstream of Pnx to coordinate its effects on GnRH and kisspeptin. We investigated the effects of the hormones, estrogen and leptin; the fatty acids, palmitate, docosahexaenoic acid (DHA), oleate and palmitoleate; and the endocrine disrupting chemical BPA on Pnx mRNA levels. We also examined whether the signaling pathways of nitric oxide, lipopolysaccharide, cAMP and protein kinase C could alter Pnx expression. Immortalized hypothalamic neurons were treated from 2 to 24 h with these compounds and Pnx mRNA levels were measured with RT-qPCR. Unexpectedly, only BPA as well as the fatty acids, palmitate, DHA and oleate, could alter Pnx expression; therefore suggesting that Pnx may fulfill a nutrient-sensing role in the hypothalamus. Our study is the first to delineate potential regulators of this novel neuropeptide, and our findings provide some insight into the functional role of PNX in the hypothalamus.
Introduction
Reproductive function is coordinated by the release of gonadotropin-releasing hormone (GnRH) from the hypothalamus, which stimulates the release of luteinizing hormone (LH) and follicle-stimulating hormone (FSH) from the pituitary, which in turn trigger release of steroid hormones from the gonads (Clarke and Cummins, 1982; Radovick et al., 1991). The steroid hormones provide feedback to the hypothalamus and pituitary, thereby forming the hypothalamic-pituitary-gonadal (HPG) axis. While GnRH is critical to control puberty and the estrous cycle (Barbieri, 2014; Plant, 2015), it is becoming increasingly clear that other peptides contribute to the neural control of reproduction. For example, kisspeptin, a now well-studied peptide, acts as an afferent regulator of GnRH, controlling pulsatile GnRH secretion as well as the preovulatory GnRH surge (Gottsch et al., 2006; Clarkson et al., 2008). Extensive research on kisspeptin has led to its use in clinical trials for in vitro fertilization, with promising results (Abbara et al., 2015; Prague and Dhillo, 2015). Input from other peptides, such as gonadotropin-inhibitory hormone (GnIH) and neuropeptide Y (NPY), to GnRH neurons is also involved in normal reproductive function (Dhillon et al., 2009; Roa and Herbison, 2012; Ubuka et al., 2013).
One newly identified peptide crucial for reproductive function is phoenixin (PNX) (Yosten et al., 2013). It is conserved amongst humans, rodents, bovine, pigs, and gallus (Yosten et al., 2013). PNX is cleaved from small integral membrane protein 20 (SMIM20) into an amidated 14 or 20 amino acid chain, referred to as PNX-14 and PNX-20, respectively (Yosten et al., 2013). Yosten et al. (2013) showed siRNA knockdown of the peptide extended the rat estrous cycle by an average of 2.3 days, demonstrating it is indispensable for normal estrous cycling (Yosten et al., 2013). This effect may occur through its actions at two levels of the HPG axis: the hypothalamus and pituitary. In the hypothalamus, where PNX is most highly expressed, PNX positively regulates GnRH expression and secretion, as well as kisspeptin expression (Treen et al., 2016). In the pituitary, PNX enhances GnRH-stimulated LH release (Yosten et al., 2013; Stein et al., 2016). These actions are mediated by the PNX receptor, GPR173, which is a conserved G protein coupled receptor, expressed highly in the brain and ovaries (Matsumoto et al., 2000; Stein et al., 2016; Treen et al., 2016). However, how this positive regulator of the HPG axis is itself controlled remains unknown.
Determining the signals that stimulate PNX to ultimately alter GnRH and kisspeptin is critical to understanding the role of PNX in the hypothalamus. The expression of Pnx at the hypothalamic level may allow it to be modulated by peripheral signals, including hormones and fatty acids, that cross the blood–brain barrier at the median eminence and are known to regulate hypothalamic peptide expression. We took two approaches to determine such regulators of Pnx expression. The first was, as mentioned, to determine Pnx gene expression after exposure to hormones and fatty acids. Given that Pnx is involved in the HPG axis, it may be regulated by estrogen, which provides feedback to the axis (Barraclough and Haller, 1970; Radovick et al., 1991). Another hormone, leptin, is secreted from adipose tissue and is thought to signal the HPG axis as a measure of nutritional status (Barash et al., 1996; Perez-Perez et al., 2015). Furthermore, it is possible that Pnx would be affected by hormone mimics, such as the endocrine disrupting chemical, bisphenol A (BPA), which is thought to have estrogenic activity (Acconcia et al., 2015). Additionally, Pnx may be affected by nutritional signals like fatty acids, which can regulate expression of the reproductive peptides, GnRH and LH (Garrel et al., 2011; Tran et al., 2016). Our second approach was to identify common signaling pathways that potentially regulate Pnx in an attempt to identify other regulatory pathways controlling Pnx expression. Such signaling molecules include cAMP, protein kinase C (PKC), nitric oxide (NO) and lipopolysaccharide (LPS).
The hypothalamus contains a heterogeneous array of neurons that are modulated differentially by hormones and fatty acids. Our laboratory has generated immortalized hypothalamic cell lines to study the responses of individual neuronal populations (Belsham et al., 2004). Therefore, to study the regulation of Pnx in the hypothalamus, we used the immortalized clonal Pnx-expressing mHypoE-46 cell line, as it has been used on numerous occasions to study the effects of leptin, insulin and palmitate in the hypothalamus (Mayer and Belsham, 2009; Dhillon and Belsham, 2011; Ye et al., 2016). We also used cell lines representing male, female, adult and embryonic neurons, allowing for the study of sex and developmental differences. Here, we demonstrate that the fatty acids palmitate, docosahexaenoic acid (DHA) and oleate, as well as BPA modulate Pnx expression in immortalized hypothalamic cell models. We also demonstrate that 17-ß estradiol (E2), leptin, palmitoleate, cAMP, NO, PKC and LPS do not regulate Pnx gene expression. Together, these results provide the first evidence of compounds that can and cannot regulate the newly identified peptide, PNX, in the hypothalamus.
Materials and Methods
Cell Culture
Clonal hypothalamic cell lines were immortalized using SV40 T-antigen, as previously described (Belsham et al., 2004, 2009). These cell lines express Pnx and Gpr173, along with receptors and neuropeptides, and represent neurons from adult, embryonic, male and female mice (Table 1). The effects of all compounds were tested in the embryonic male cell line, mHypoE-46. Given that estrogen has differential effects during development and between sexes, E2 and BPA, which has estrogenic activity, were also studied in the other cell lines.
Cells to be treated with E2 or BPA were cultured in Dulbecco’s Modified Eagle Medium (DMEM) with 25 mM glucose (Sigma-Aldrich), supplemented with 2% fetal bovine serum (FBS; Gibco, Life Technologies) and 1% penicillin/streptomycin (P/S; Gibco, Life Technologies). To eliminate influence of steroids contained in DMEM and FBS, treatments with E2 and BPA were performed in phenol-red free DMEM (Hyclone, Fisher Scientific), supplemented with 1% charcoal:dextran-stripped FBS (Gemini Bio Products through Cedarlane, Inc.) and 1% P/S. Cells to be treated with all other compounds were grown and treated in 5.5 mM glucose DMEM, supplemented with 2% FBS and 1% P/S.
Primary Culture
Eight-week-old CD1 female or male mice were euthanized with CO2 and their hypothalamic extracted. This study was carried out in accordance with the recommendations of the Ontario’s Animals for Research Act, and the federal Canadian Council on Animal Care. All animal procedures were approved by the University of Toronto animal care committee. Cells were grown in Neurobasal A medium (Gibco, Life Technologies) containing 1 × GlutaMAX supplement (Gibco), 1 × B27 serum-free supplement (Gibco), 10% FBS, 5% normal horse serum, and 1% P/S (Gibco, Life Technologies) for 1 week, with 10 ng/μL ciliary neurotrophic factor (CNTF) addition each day. Primary culture was treated with 100 μM BPA for 8 h or 50 μM palmitate for 16 h in DMEM as described below.
Reagents
E2 (Tocris Bioscience) was dissolved in 100% ethanol and diluted to 10 or 100 μM in sterile-filtered Hypure (Hyclone, Fisher Scientific) or UltraPure (Thermofisher Scientific) water followed by a 1:1,000 dilution in culture media to a final concentration of 10 or 100 nM in 0.0005% ethanol. 200 mM BPA (Sigma-Aldrich) was dissolved in 100% ethanol and diluted to 100 mM with sterile-filtered water; a 1:1,000 dilution was then performed in culture media to obtain a final concentration of 100 μM BPA containing 0.05% ethanol. Sodium palmitate and sodium oleate (Sigma-Aldrich) were dissolved by heating to 60°C in water, and added to media at 50 μM. Sodium nitroprusside (SNP) (Sigma-Aldrich) was dissolved in sterile-filtered water and added to media to obtain 100 μM SNP.
All stock solutions were diluted 1:1,000 in culture media to obtain the final concentration. Palmitoleate (Sigma-Aldrich) 200 mM stock solution was prepared in 50% ethanol and by heating to 60°C. Immediately before use, palmitoleate was diluted to 100 mM in 50% ethanol and 100 μM in media. DHA (Sigma-Aldrich) was dissolved in DMSO to obtain a 100 mM stock solution, followed by dilution to 100 μM in media. 100 μM leptin (National Hormone and Peptide Program) stock solution was prepared in 1× PBS and diluted to 100 nM in media. 100 μg/mL LPS (O55:B5; Sigma-Aldrich) stock solution was prepared in sterile-filtered water, and diluted to 100 ng/mL in media. 10 mM Forskolin and 100 μM TPA (Sigma-Aldrich) stock solutions were prepared in DMSO, then diluted to 10 μM and 100 nM in media, respectively. Cells were grown to 70–80% confluency for treatment.
Quantitative Real-Time PCR
Total RNA was isolated using the PureLink RNA Mini Kit (Ambion, Life Technologies) and its purity and concentration were quantified using the Nanodrop 2000c spectrophotometer. cDNA was synthesized with the High Capacity cDNA Reverse Transcription Kit (Applied Biosystems). For qRT-PCR, 12.5 ng of cDNA was amplified with gene-specific primers and Platinum SYBR Green qPCR SuperMix-UDG with ROX (Life Technologies, Thermofisher Scientific) in the Applied Biosystems 7900 HT Real-Time PCR machine. The primers are listed in Table 2 and were designed using the online tool, PrimerBLAST (Ye et al., 2012). Data was analyzed using the standard curve method and normalized to the standardization gene, Histone 3A, except BPA-treated samples which were normalized to Rpl7.
Statistical Analysis
Results were expressed as mean ± SEM and analyzed with GraphPad Prism Software 6.0 (GraphPad Software, Inc.). Statistical significance was determined with a two-way ANOVA or a T-test, as appropriate, followed by a Bonferroni post hoc test, where ∗P < 0.05, ∗∗P < 0.01, ∗∗∗P < 0.001 and ∗∗∗∗P < 0.0001.
Results
E2 Does Not Alter Pnx mRNA Levels in Immortalized Hypothalamic Neurons
Mounting evidence demonstrates that the newly identified peptide, PNX, is integral to the HPG axis at the level of the hypothalamus (Yosten et al., 2013; Stein et al., 2016; Treen et al., 2016), but precisely how it is involved in the HPG axis is unknown. To define its role, we examined how the gonadal hormone E2, which is vital to the HPG axis in both females and males (Radovick et al., 1991; Hayes et al., 2000), affects Pnx gene expression. The mHypoE-46, mHypoE-41 and mHypoA-59 immortalized clonal hypothalamic neuronal cell lines were treated with E2 and the mRNA levels of Pnx were measured with RT-qPCR. In addition to estrogen receptors, these cell lines express both the Pnx gene and receptor, similar to GnRH neurons, which co-express the GnRH receptor (Cheng and Leung, 2005), making them appropriate models for analyzing changes in gene expression in response to E2. 10 nM E2 treatment for 4 or 8 h in the male mHypoE-46 cell line, and female mHypoE-41 and mHypoA-59 cell lines had no significant effect on mRNA levels of Pnx (Figure 1Ai). E2 treatment did, however, change Npy mRNA levels in the mHypoA-59 cell line, as previously reported (Titolo et al., 2006) (Figure 1Aii), demonstrating the responsiveness of these neurons to E2. Further, longer E2 treatment from 12 to 48 h did not alter Pnx mRNA levels in the mHypoE-41 (Figure 1Aiii) and mHypoA-59 cell lines (Figure 1Aiv). Therefore, it appears that Pnx is expressed independently of E2 feedback in these hypothalamic cell lines.
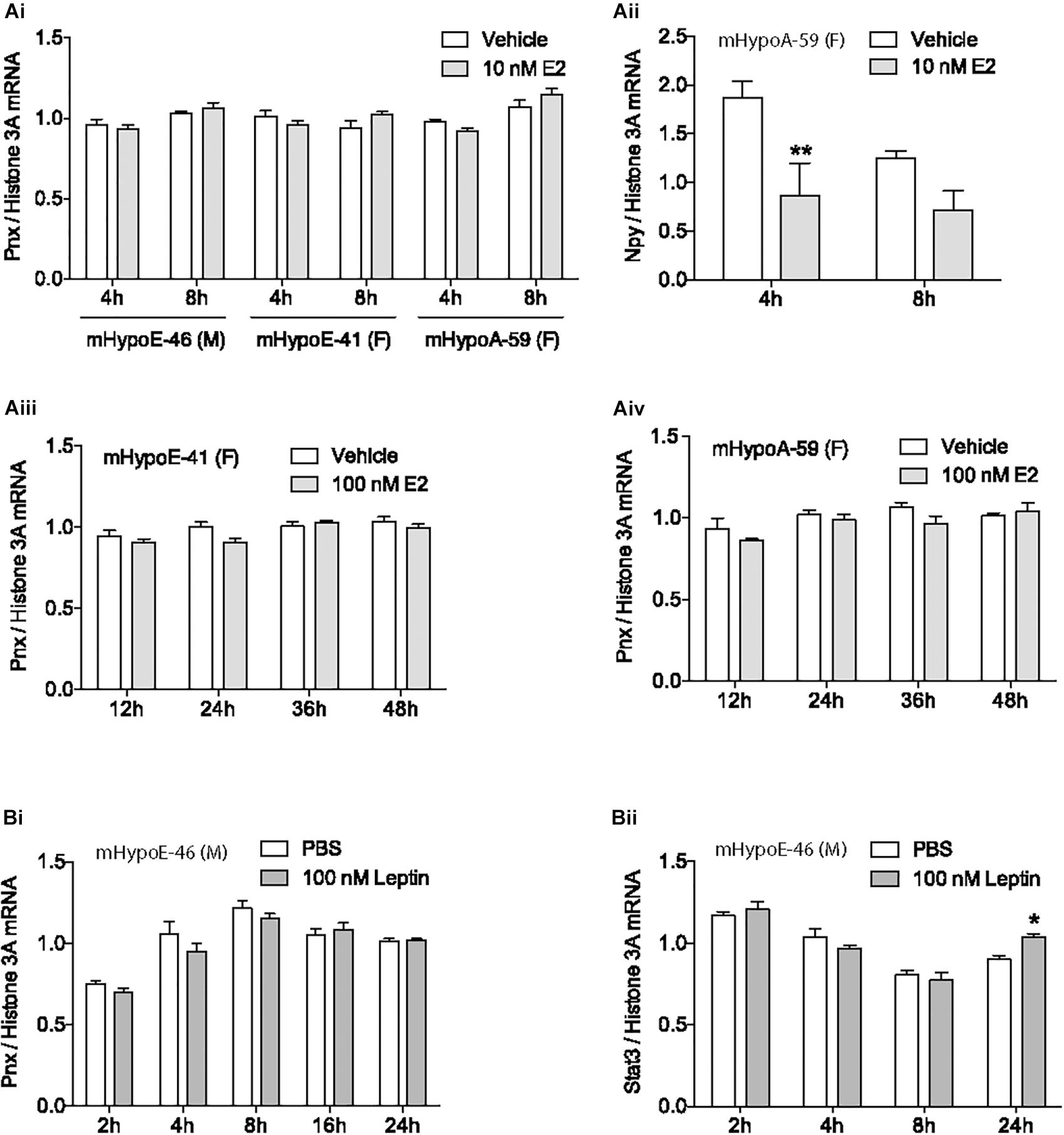
FIGURE 1. Estrogen and leptin do not alter Pnx gene expression. (Ai) Treatment with 10 nM of 17β-estradiol (E2) for 4 or 8 h in the mHypoE-46, mHypoE-41 and mHypoA-59 cell lines (n = 4). (Aii) Npy expression after treatment with 10 nM E2 in the mHypoA-59 cell line (n = 4). (Aiii) Treatment with 100 nM E2 for 12, 24, 36, and 48 h in the mHypoE-41 cell line. (Aiv) Treatment with 100 nM E2 for 12–48 h in the mHypoA-59 cell line. (Bi) Treatment over 24 h with 100 nM leptin in the mHypoE-46 cell line (n = 3). (Bii) Stat3 expression after treatment with 100 nM leptin in the mHypoE-46 cell line (n = 4) (mean ± SEM, ∗P < 0.05 and ∗∗P < 0.01, by two-way ANOVA).
Leptin Does Not Alter Pnx mRNA Levels
Another hormone with input to the reproductive axis is leptin. Secreted from adipose tissue, leptin signals to the hypothalamus to reduce food intake and increase energy expenditure (Sahu, 2003). Leptin is tightly coupled to reproductive capacity, for example, leptin infusion increases secretion of GnRH and LH in rats (Watanobe, 2002; Hausman et al., 2012). However, treatment with 100 nM leptin over 24 h did not alter Pnx expression in the mHypoE-46 cell line (Figure 1Bi), signifying that Pnx expression may not be related to adipose stores. In contrast, as a positive control, we found that leptin increased Stat3 mRNA levels at 24 h (Figure 1Bii).
The Fatty Acids Palmitate, DHA and Oleate, but Not Palmitoleate, Upregulate Pnx mRNA
In addition to hormonal input, the HPG axis receives feedback from nutritional signals. It has been reported that hypothalamic neurons can directly sense nutrients, such as free fatty acids, from the blood (Migrenne et al., 2006; Jo et al., 2009). To investigate the effect of the saturated fatty acid palmitate on Pnx expression, its mRNA levels were measured over 24 h (Figure 2A). 50 μM palmitate significantly increased Pnx expression in the male mHypoE-46 cell line. To determine whether this Pnx increase in this male clonal cell line was representative of the whole hypothalamus, we treated male hypothalamic primary culture with 50 μM palmitate for 24 h (Figure 2B). An increase in Pnx was observed, suggesting Pnx is responsive to palmitate across an array of neuronal populations.
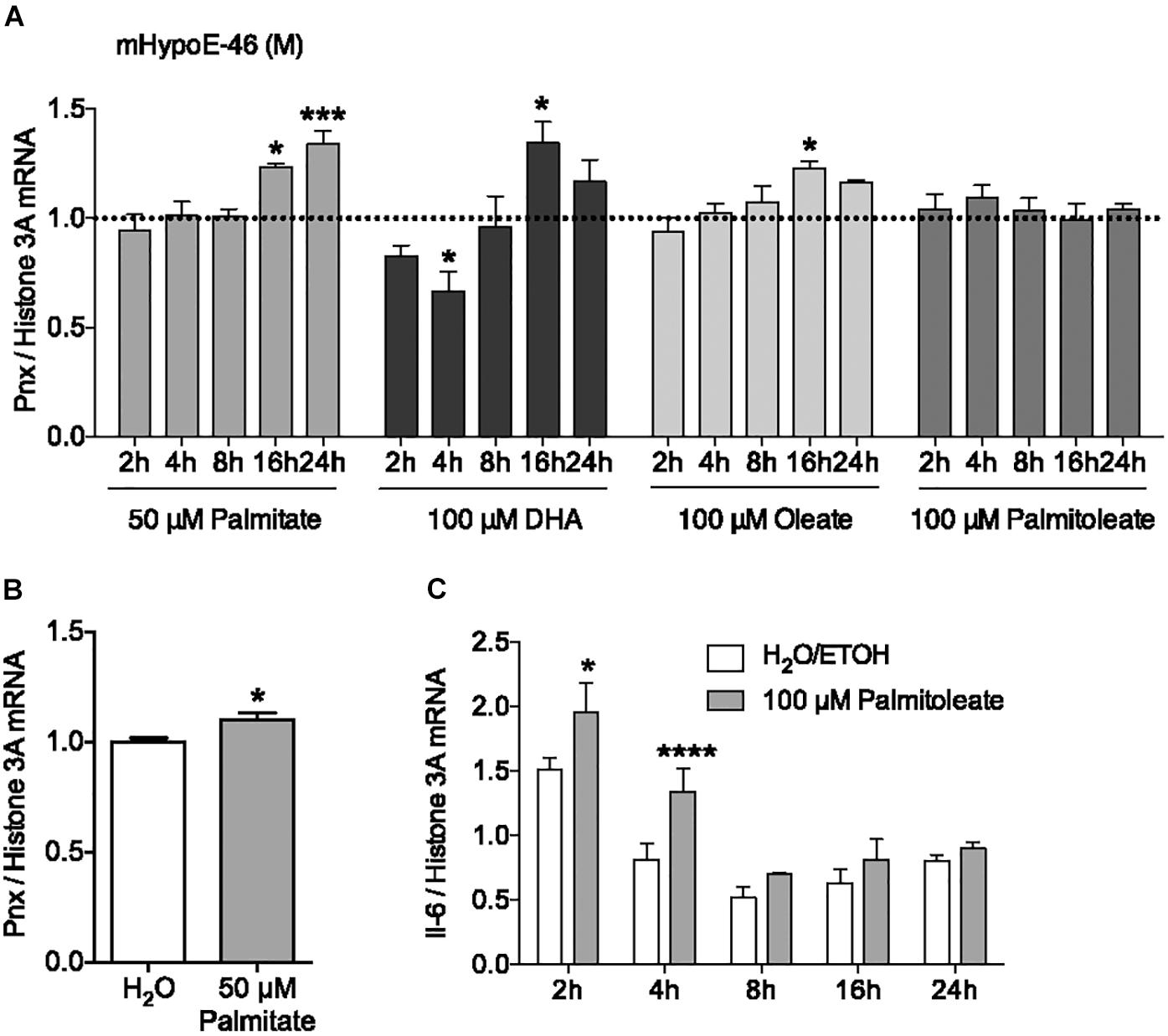
FIGURE 2. Fatty acids regulate Pnx mRNA levels in cell lines and hypothalamic primary culture. (A) The mHypoE-46 cell line was treated with palmitate, DHA, oleate and palmitoleate over 24 h (n = 4). Bars represent treatments normalized to 1 (dotted line indicates time-matched controls set to 1). (B) Male murine primary culture was treated with 50 μM palmitate for 24 h (n = 4; normalized to 1). (C) Il-6 mRNA levels after treatment with 100 μM palmitoleate in the mHypoE-46 cell line (n = 4) (mean ± SEM, ∗P < 0.05, ∗∗∗P < 0.001, and ∗∗∗∗P < 0.0001, by (A,C) two-way ANOVA or (B) T-test).
To determine if unsaturated fatty acids could also affect Pnx mRNA, the effects of the monounsaturated fatty acids, oleate and palmitoleate, and the polyunsaturated fatty acid, DHA, were measured from 2 to 24 h in the mHypoE-46 neurons (Figure 2A). 100 μM oleate and DHA increased Pnx expression, while palmitoleate did not significantly alter Pnx. The expression of the cytokine, interleukin-6 (Il-6), was altered in response to palmitoleate, demonstrating neuronal response to the palmitoleate (Figure 2C). Together, this suggests that Pnx is responsive to multiple fatty acids.
BPA Reduces Pnx Expression in Male Immortalized and Primary Hypothalamic Cell Culture
In addition to determining what physiological compounds could regulate Pnx, we assessed whether compounds could dysregulate Pnx in the hypothalamus. One environmental chemical with potential to disrupt Pnx is BPA, due to its association with reproductive dysfunction such as impairing embryo implantation and reducing sperm quality (Peretz et al., 2014; Tomza-Marciniak et al., 2018). Exposure to BPA in utero or in adult mice demonstrates that it has actions in the hypothalamus, for example, it alters the expression of GnRH and kisspeptin (Bai et al., 2011; Kurian et al., 2015). We therefore assessed whether it also alters Pnx mRNA. To determine the effect of BPA on Pnx gene expression, mRNA levels were measured after 2, 4, 8, 16 and 24 h of 100 μM BPA treatment (Figure 3A). This dosage has been previously found to be effective in our cell lines with the modulation of pro-opiomelanocortin (POMC) and therefore was used here (Salehi et al., 2018). In the mHypoE-46 embryonic male-derived cell line, Pnx expression was downregulated at 16 h. Pnx expression was also decreased at 16 and 24 h in the adult male-derived mHypoA-2/12 cell line. Pnx expression in the embryonic female-derived mHypoE-41 cell line increased after exposure to 100 μM BPA at 4 h, but was unaffected in the adult female mHypoA-59 cell line. Therefore there are potentially differential responses of Pnx to BPA in male-derived and female-derived cell lines.
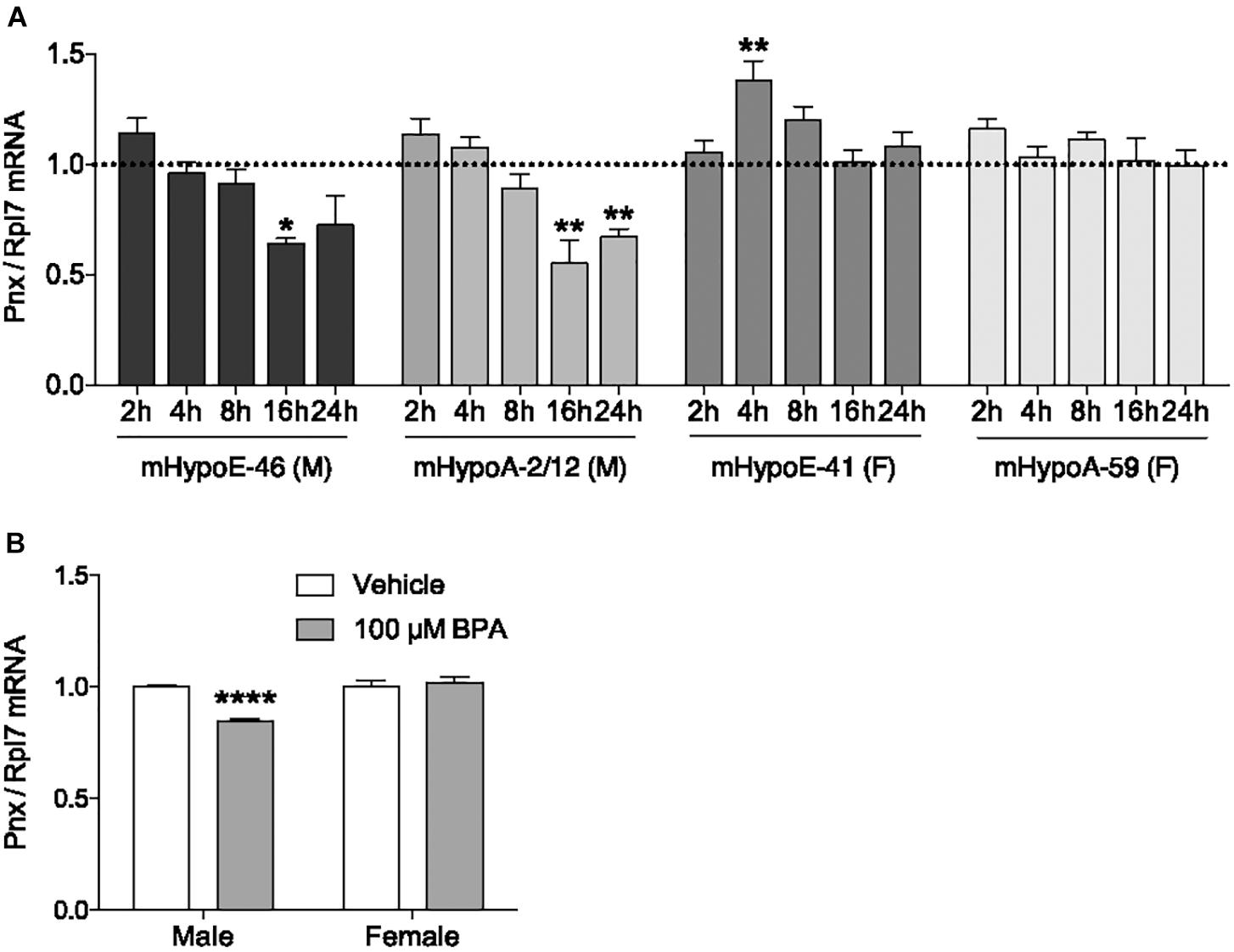
FIGURE 3. Bisphenol A reduces Pnx expression in male cell lines and primary culture. (A) Treatment with 100 μM BPA for 2, 4, 8, 16, and 24 h in the mHypoE-46, mHypoA-2/12, mHypoE-41 and mHypoA-59 cell lines (n = 4). Bars represent treatment normalized to 1 (dotted line indicates time-matched controls set to 1). (B) Male and female primary culture treated with 100 μM BPA for 8 h (n = 7; normalized to 1) (mean ± SEM, ∗P < 0.05, ∗∗P < 0.01, ∗∗∗P < 0.001, ∗∗∗∗P < 0.0001, by (A) two-way ANOVA or (B) T-test).
To investigate how BPA affects Pnx in a heterogeneous non-immortalized population of cells, male and female primary hypothalamic culture were treated with BPA. Consistent with the observations in clonal cell lines, 8-h treatment with 100 μM BPA decreased expression of Pnx in male primary culture, while having no effect on Pnx expression in female primary culture (Figure 3B). Taken together, these results suggest that BPA disrupts production of Pnx in the male murine hypothalamus.
Activation of Inflammation and PKC, and Increasing NO and cAMP Levels Have No Effects on Pnx Expression
In addition to identifying hormones and nutrients that regulate Pnx, identifying signaling molecules upstream of the Pnx gene could also provide information about its regulation. Treatment with LPS to induce the inflammatory signaling pathway did not change Pnx expression (Figure 4Ai), but did alter Il-6 mRNA levels at 2 h, indicating that the inflammatory pathway was activated within the cells (Han et al., 2013) (Figure 4Aii). Activating PKC with 12-O-tetradecanoylphorbol-13-acetate (TPA) also did not change Pnx mRNA levels (Figure 4Bi), but it did significantly alter Il-6 expression at 2 and 4 h (Chen et al., 2012) (Figure 4Bii). Increasing NO levels with the NO donor, SNP, also did not alter Pnx expression (Figure 4Ci); however, inducible NO synthase (iNOS) expression was reduced as a result (Chang et al., 2004) (Figure 4Cii). Induction of cAMP through activation of adenylyl cyclase by forskolin did not alter Pnx expression (Figure 4Di), but did alter the positive control Bmal1 (Chalmers et al., 2008) (Figure 4Dii). Therefore, although the cells were responsive to the signaling molecules tested, it appears that Pnx is not regulated by cAMP, NO, PKC or neuroinflammation. Signaling pathways that do regulate Pnx remain to be identified.
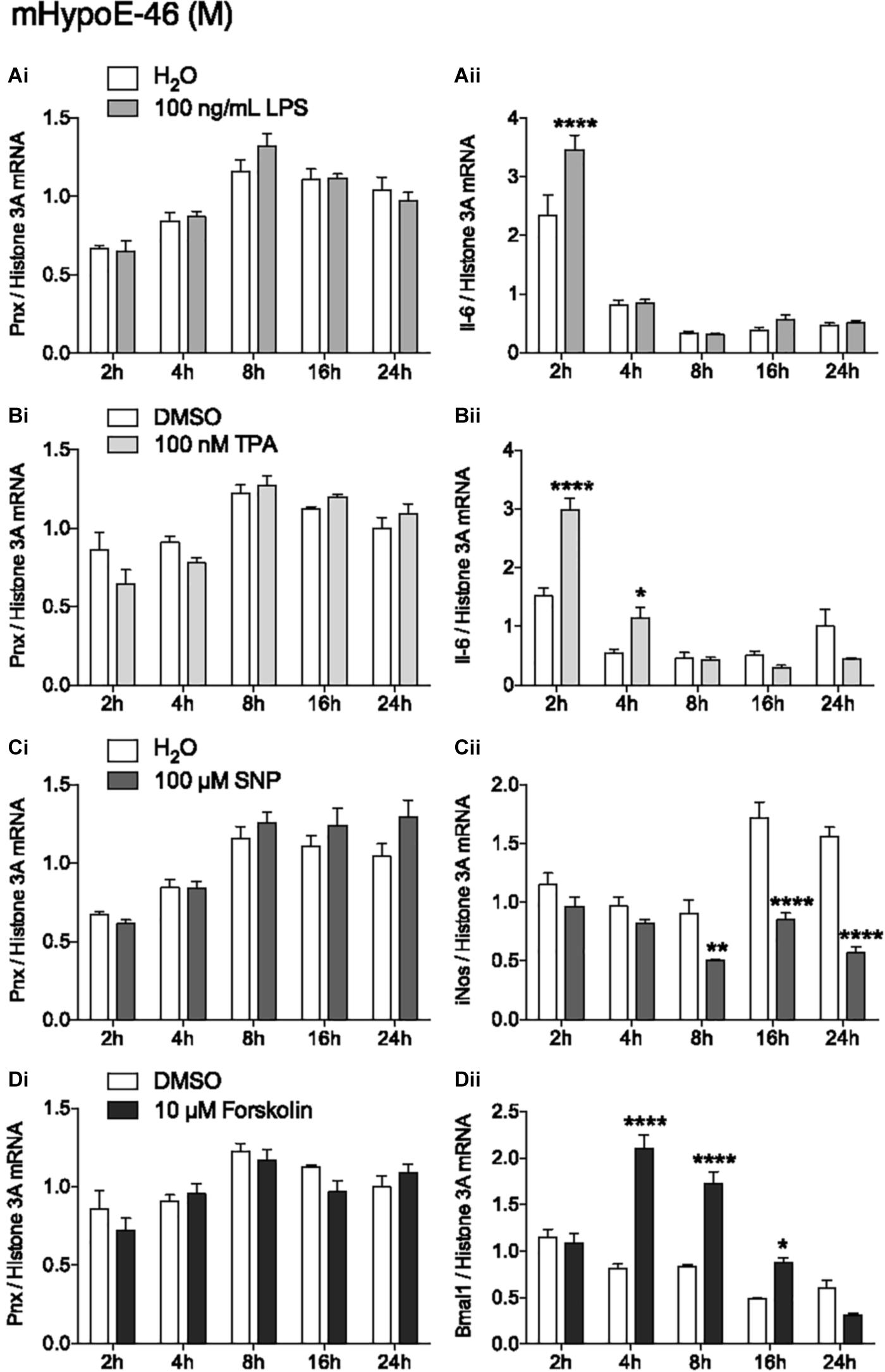
FIGURE 4. None of the classic signal transduction pathways influence Pnx mRNA levels. Treatment over 24 h in the mHypoE-46 cell line with (Ai,ii) 100 ng/mL LPS, (Bi,ii) 100 nM TPA, (Ci,ii) 100 μM SNP and (Di,ii) 10 μM forskolin (n = 4) (mean ± SEM, ∗P < 0.05 and ∗∗∗∗P < 0.0001, by two-way ANOVA).
Discussion
Phoenixin is a recently identified peptide involved in the HPG axis through positive regulation of GnRH, kisspeptin and LH (Yosten et al., 2013; Treen et al., 2016). However, the regulation of PNX itself was unknown. We investigated whether several hormones, fatty acids and signaling pathways could affect mRNA levels of the Pnx gene in the area it is most populous, hypothalamic neurons. Remarkably, only four compounds altered Pnx gene expression: palmitate, DHA, oleate and BPA. These findings may point to the specific role of PNX in regulating the reproductive axis.
The fatty acids palmitate, DHA and oleate upregulated Pnx expression in the neurons, suggesting a role for PNX in nutritional sensing. Neurons in the hypothalamus, particularly the arcuate nucleus, project to the median eminence where the larger fenestrations allow for the passage of large molecules. In particular, GnRH neurons project directly to the median eminence and have been shown to directly respond to palmitate and DHA in vitro (Yin et al., 2009). Without sufficient nutritional resources, it is beneficial for organisms to delay reproduction; therefore Pnx production increasing in response to these fatty acids could be a signal to promote reproduction. Indeed, other peptides that respond to metabolic cues have reproductive input. For example, kisspeptin neurons receive input from leptin, ghrelin and insulin (Forbes et al., 2009; Cravo et al., 2013; Qiu et al., 2013), while neurons expressing the feeding neuropeptides, POMC and NPY, project to GnRH neurons (Roa and Herbison, 2012). Furthermore, mice with POMC neurons lacking both the leptin and insulin receptors have reduced fertility (Hill et al., 2010). As Pnx appears to be specifically responsive to fatty acids, it may play a larger role in the nutritional control of reproduction than these other peptides that are also responsive to hormonal signals. Alternatively, although PNX is primarily associated with reproductive signaling, it is also related to feeding, therefore its ability to respond to fatty acid signaling may be due to this function. PNX appears to be orexigenic as it modestly increases light phase food intake in rats (Schalla et al., 2017). Furthermore, PNX serum levels increase postprandially (Rocca et al., 2017) and with fasting in the hypothalamus (Wang et al., 2018), suggesting the peptide is responsive to metabolic or hormonal nutritional cues. Therefore, the response of Pnx to fatty acids could also be due to its association with satiety and satiation.
The mechanism underlying the effects of palmitate, DHA and oleate may involve multiple signaling pathways. One possibility is that palmitate, DHA and oleate may be acting through the long chain fatty acid receptors, GPR40 and GPR120 (Oh et al., 2010; Nakamoto et al., 2013). Palmitate also activates neuroinflammatory signaling (Shi et al., 2006), however, its effects on Pnx mRNA appear to be independent of neuroinflammation as treatment with LPS, which activates toll-like receptor 4, caused no change in expression. Moreover, DHA and oleate, unlike palmitate, have anti-inflammatory actions but also increased Pnx (Kwon et al., 2014). Therefore, the effects of fatty acids on Pnx appear to be in response to nutritional cues rather than inflammatory cues.
The endocrine disrupting chemical BPA selectively decreased Pnx expression in male-derived cell lines and primary culture. This sex difference is not unusual for BPA, as it has also been associated with sex dependent changes in hormones and reproductive functions (Tomza-Marciniak et al., 2018). For example, perinatal exposure to BPA has been noted to alter methylation enzymes and ERα expression differentially in the cortex and hypothalamus of male and female rats, establishing permanent differences in gene expression (Kundakovic et al., 2013). Additionally, neonatal exposure to BPA reduces arcuate kisspeptin fiber density in female, but not male rats (Patisaul et al., 2009).
Bisphenol A may act through multiple mechanisms to alter Pnx expression. Although the chemical is a putative estrogen mimic and has been shown to activate nuclear and membrane bound estrogen receptors in a variety of cellular models (Welshons et al., 2006), it appears to be acting through an estrogen-independent pathway in the cell models used in this study, as E2 had no effect on Pnx expression. Other mechanisms implicated in the action of BPA include binding and activating the transcription factors estrogen related receptor gamma (ERRγ) or peroxisome proliferator-activated receptor gamma (PPARγ), or activating the inflammatory or endoplasmic reticulum stress pathways (Takayanagi et al., 2006; Asahi et al., 2010; Zhu et al., 2015; Ahmed and Atlas, 2016). BPA is widely known to disrupt the reproductive axis, and our studies provide evidence of yet another way this endocrine disrupting chemical can impact reproduction by altering the expression of a peptide that regulates the HPG axis.
Interestingly, the majority of compounds tested did not alter Pnx mRNA levels. E2, a critical component of the HPG axis, did not affect Pnx mRNA levels, which was particularly unexpected since PNX is predominantly associated with reproduction and the estrous cycle. Therefore, unlike kisspeptin and GnRH (Roy et al., 1999; Dubois et al., 2014), PNX may influence the HPG axis in an estrogen-independent manner. Instead, based on its response to fatty acids, it may modulate the HPG axis in a nutrient-dependent manner. Investigating other reproductive hormones, such as androgens, for their effects on Pnx would be required to further elucidate how PNX is involved in the HPG axis. Leptin, another hormone that did not alter Pnx, is secreted from adipose tissue and signals to alter expression of both appetite regulating and reproductive peptides (Barash et al., 1996; Baver et al., 2014). Here, its inability to change Pnx may again suggest Pnx is more involved with sensing nutrients such as fatty acids, rather than as a sensor of long-term energy stores. Additionally, the general signaling molecules investigated did not alter Pnx expression. cAMP and PKC are downstream of the Gαs and Gαq G proteins, respectively. This suggests that compounds that signal through GPCRs coupled to Gαs and Gαq may not affect Pnx mRNA levels. These G proteins, however, do activate other pathways, so they may nevertheless affect gene expression and furthermore, they could affect PNX secretion, which was not examined. NO, which can act as an extracellular and intracellular messenger (Tuteja and Chandra, 2004) and has been shown, in immortalized GnRH neurons, to stimulate GnRH secretion (Mahachoklertwattana et al., 1994) did not affect Pnx. Additionally, the bacterial endotoxin, LPS, had no effect on Pnx mRNA levels, even though it is known to affect other reproductive peptides, such as decreasing GnRH mRNA in ewes (Herman and Tomaszewska-Zaremba, 2010). This enigma of very few compounds regulating Pnx may be explained by the fact that it forms part of the mitochondrial chaperone-like protein complex, MITRAC7 (Dennerlein et al., 2015). Overexpression or knock down of MITRAC7 blocks assembly of cytochrome oxidase c. Therefore it is possible that due to the importance of maintaining SMIM20 levels constant, very few compounds can alter its expression at the gene level. The fact that BPA had the strongest effect is further evidence of the detrimental effects of endocrine disrupting chemicals on physiological processes. Overall, this suggests that the role of PNX is highly precise and therefore very few compounds can alter its expression.
An important consideration, and perhaps a limitation to our study, is whether mRNA levels correlate directly to PNX protein levels. We chose to use mRNA analysis due to the increased reproducibility of the data compared to protein analysis that is notoriously difficult to quantify, both through protein arrays and Western/ELISA experiments. The literature is discrepant when it comes to a consensus. There have been numerous studies that indicate that mRNA levels correlate well to protein in steady-state conditions, with a gene-specific lag in protein synthesis (Liu et al., 2016). The tools to assess PNX protein have not yet been sufficiently tested and validated, thus extensive analysis would have to be undertaken. Optimally all of the treatments should be validated at the protein level; however, this is difficult due to the unique lag time of protein expression and it would be prohibitive to analyze the PNX protein over a full time course for each of the 11 compounds tested in this study.
In summary, we provide evidence that BPA and the fatty acids palmitate, DHA and oleate stimulate Pnx gene expression, while E2 does not. Elevated levels of cAMP, NO and activation of PKC and neuroinflammation also had no effect on Pnx mRNA. The mechanisms by which BPA and palmitate alter Pnx expression have yet to be elucidated and will be the subject of further study. Determining how PNX is regulated is necessary for clarifying its physiological role and identifying if it has therapeutic potential. Knowledge of GnRH and kisspeptin has led to treatment of infertility (Suda et al., 1990; Abbara et al., 2015), so it is conceivable that PNX may also lead to novel treatment opportunities.
Author Contributions
EM and NL performed the experiments, analyzed the data, and wrote the manuscript. DB provided funding, wrote and edited the paper, and provided project guidance.
Funding
We acknowledge funding from the Canadian Institutes for Health Research (CIHR), National Science and Engineering Research Council (NSERC), Canada Foundation for Innovation, and Canada Research Chairs Program (DB). EM and NL are supported by NSERC CGS Studentships.
Conflict of Interest Statement
The authors declare that the research was conducted in the absence of any commercial or financial relationships that could be construed as a potential conflict of interest.
References
Abbara, A., Jayasena, C. N., Christopoulos, G., Narayanaswamy, S., Izzi-Engbeaya, C., Nijher, G. M. K., et al. (2015). Efficacy of kisspeptin-54 to trigger oocyte maturation in women at high risk of ovarian hyperstimulation syndrome (OHSS) during in vitro fertilization (IVF) therapy. J. Clin. Endocrinol. Metab. 100, 3322–3331. doi: 10.1210/jc.2015-2332
Acconcia, F., Pallottini, V., and Marino, M. (2015). Molecular mechanisms of action of BPA. Dose-Response 13:1559325815610582. doi: 10.1177/1559325815610582
Ahmed, S., and Atlas, E. (2016). Bisphenol S- and bisphenol A-induced adipogenesis of murine preadipocytes occurs through direct peroxisome proliferator-activated receptor gamma activation. Int. J. Obes. 40, 1566–1573. doi: 10.1038/ijo.2016.95
Asahi, J., Kamo, H., Baba, R., Doi, Y., Yamashita, A., Murakami, D., et al. (2010). Bisphenol A induces endoplasmic reticulum stress-associated apoptosis in mouse non-parenchymal hepatocytes. Life Sci. 87, 431–438. doi: 10.1016/j.lfs.2010.08.007
Bai, Y., Chang, F., Zhou, R., Jin, P. P., Matsumoto, H., Sokabe, M., et al. (2011). Increase of anteroventral periventricular kisspeptin neurons and generation of E2-induced LH-surge system in male rats exposed perinatally to environmental dose of bisphenol-A. Endocrinology 152, 1562–1571. doi: 10.1210/en.2010-1042
Barash, I. A., Cheung, C. C., Weigle, D. S., Ren, H., Kabigting, E. B., Kuijper, J. L., et al. (1996). Leptin is a metabolic signal to the reproductive system. Endocrinology 137, 3144–3147. doi: 10.1210/endo.137.7.8770941
Barbieri, R. L. (2014). The endocrinology of the menstrual cycle. Methods Mol. Biol. 1154, 145–169. doi: 10.1007/978-1-4939-0659-8_7
Barraclough, C. A., and Haller, E. W. (1970). Positive and negative feedback effects of estrogen on pituitary LH synthesis and release in normal and androgen-sterilized rats. Endocrinology 86, 542–551. doi: 10.1210/endo-86-3-542
Baver, S. B., Hope, K., Guyot, S., Bjorbaek, C., Kaczorowski, C., and O’connell, K. M. (2014). Leptin modulates the intrinsic excitability of AgRP/NPY neurons in the arcuate nucleus of the hypothalamus. J. Neurosci. 34, 5486–5496. doi: 10.1523/JNEUROSCI.4861-12.2014
Belsham, D. D., Cai, F., Cui, H., Smukler, S. R., Salapatek, A. M., and Shkreta, L. (2004). Generation of a phenotypic array of hypothalamic neuronal cell models to study complex neuroendocrine disorders. Endocrinology 145, 393–400. doi: 10.1210/en.2003-0946
Belsham, D. D., Fick, L. J., Dalvi, P. S., Centeno, M. L., Chalmers, J. A., Lee, P. K., et al. (2009). Ciliary neurotrophic factor recruitment of glucagon-like peptide-1 mediates neurogenesis, allowing immortalization of adult murine hypothalamic neurons. FASEB J. 23, 4256–4265. doi: 10.1096/fj.09-133454
Chalmers, J. A., Lin, S. Y., Martino, T. A., Arab, S., Liu, P., Husain, M., et al. (2008). Diurnal profiling of neuroendocrine genes in murine heart, and shift in proopiomelanocortin gene expression with pressure-overload cardiac hypertrophy. J. Mol. Endocrinol. 41, 117–124. doi: 10.1677/JME-08-0050
Chang, K., Lee, S. J., Cheong, I., Billiar, T. R., Chung, H. T., Han, J. A., et al. (2004). Nitric oxide suppresses inducible nitric oxide synthase expression by inhibiting post-translational modification of IkappaB. Exp. Mol. Med. 36, 311–324. doi: 10.1038/emm.2004.42
Chen, C., Du, J., Feng, W., Song, Y., Lu, Z., Xu, M., et al. (2012). beta-adrenergic receptors stimulate interleukin-6 production through Epac-dependent activation of PKCdelta/p38 MAPK signalling in neonatal mouse cardiac fibroblasts. Br. J. Pharmacol. 166, 676–688. doi: 10.1111/j.1476-5381.2011.01785.x
Cheng, C. K., and Leung, P. C. (2005). Molecular biology of gonadotropin-releasing hormone (GnRH)-I, GnRH-II, and their receptors in humans. Endor. Rev. 26, 283–306. doi: 10.1210/er.2003-0039
Clarke, I. J., and Cummins, J. T. (1982). The temporal relationship between gonadotropin releasing hormone (GnRH) and luteinizing hormone (LH) secretion in ovariectomized ewes. Endocrinology 111, 1737–1739. doi: 10.1210/endo-111-5-1737
Clarkson, J., D’anglemont, De Tassigny, X., Moreno, A. S., Colledge, W. H., and Herbison, A. E. (2008). Kisspeptin-GPR54 signaling is essential for preovulatory gonadotropin-releasing hormone neuron activation and the luteinizing hormone surge. J. Neurosci. 28, 8691–8697. doi: 10.1523/JNEUROSCI.1775-08.2008
Cravo, R. M., Frazao, R., Perello, M., Osborne-Lawrence, S., Williams, K. W., Zigman, J. M., et al. (2013). Leptin signaling in Kiss1 neurons arises after pubertal development. PLoS One 8:e58698. doi: 10.1371/journal.pone.0058698
Dennerlein, S., Oeljeklaus, S., Jans, D., Hellwig, C., Bareth, B., Jakobs, S., et al. (2015). MITRAC7 acts as a COX1-specific chaperone and reveals a checkpoint during cytochrome c oxidase assembly. Cell Rep. 12, 1644–1655. doi: 10.1016/j.celrep.2015.08.009
Dhillon, S. S., and Belsham, D. D. (2011). Leptin differentially regulates NPY secretion in hypothalamic cell lines through distinct intracellular signal transduction pathways. Regul. Pept. 167, 192–200. doi: 10.1016/j.regpep.2011.01.005
Dhillon, S. S., Gingerich, S., and Belsham, D. D. (2009). Neuropeptide Y induces gonadotropin-releasing hormone gene expression directly and through conditioned medium from mHypoE-38 NPY neurons. Regul. Pept. 156, 96–103. doi: 10.1016/j.regpep.2009.04.005
Dubois, S. L., Acosta-Martínez, M., Dejoseph, M. R., Wolfe, A., Radovick, S., Boehm, U., et al. (2014). Positive, but not negative feedback actions of estradiol in female mice require estrogen receptor α (ERα) in kisspeptin neurons. Endocrinology 156, 1111–1120. doi: 10.1210/en.2014-1851
Forbes, S., Li, X. F., Kinsey-Jones, J., and O’byrne, K. (2009). Effects of ghrelin on Kisspeptin mRNA expression in the hypothalamic medial preoptic area and pulsatile luteinising hormone secretion in the female rat. Neurosci. Lett. 460, 143–147. doi: 10.1016/j.neulet.2009.05.060
Garrel, G., Simon, V., Denoyelle, C., Cruciani-Guglielmacci, C., Migrenne, S., Counis, R., et al. (2011). Unsaturated fatty acids stimulate LH secretion via novel PKCepsilon and -theta in gonadotrope cells and inhibit GnRH-induced LH release. Endocrinology 152, 3905–3916. doi: 10.1210/en.2011-1167
Gottsch, M. L., Clifton, D. K., and Steiner, R. A. (2006). Kisspepeptin-GPR54 signaling in the neuroendocrine reproductive axis. Mol. Cell. Endocrinol. 25, 91–96. doi: 10.1016/j.mce.2006.04.030
Han, H. E., Kim, T. K., Son, H. J., Park, W. J., and Han, P. L. (2013). Activation of autophagy pathway suppresses the expression of iNOS, IL6 and cell death of LPS-stimulated microglia cells. Biomol. Ther. (Seoul) 21, 21–28. doi: 10.4062/biomolther.2012.089
Hausman, G. J., Barb, C. R., and Lents, C. A. (2012). Leptin and reproductive function. Biochimie 94, 2075–2081. doi: 10.1016/j.biochi.2012.02.022
Hayes, F. J., Seminara, S. B., Decruz, S., Boepple, P. A., and Crowley, W. F. (2000). Aromatase inhibition in the human male reveals a hypothalamic site of estrogen feedback. J. Clin. Endocrinol. Metab. 85, 3027–3035. doi: 10.1210/jc.85.9.3027
Herman, A. P., and Tomaszewska-Zaremba, D. (2010). Effect of endotoxin on the expression of GnRH and GnRHR genes in the hypothalamus and anterior pituitary gland of anestrous ewes. Anim. Reprod. Sci. 120, 105–111. doi: 10.1016/j.anireprosci.2010.03.011
Hill, J. W., Elias, C. F., Fukuda, M., Williams, K. W., Berglund, E. D., Holland, W. L., et al. (2010). Direct insulin and leptin action on pro-opiomelanocortin neurons is required for normal glucose homeostasis and fertility. Cell Metab. 11, 286–297. doi: 10.1016/j.cmet.2010.03.002
Jo, Y. H., Su, Y., Gutierrez-Juarez, R., and Chua, S. Jr., (2009). Oleic acid directly regulates POMC neuron excitability in the hypothalamus. J. Neurophysiol. 101, 2305–2316. doi: 10.1152/jn.91294.2008
Kundakovic, M., Gudsnuk, K., Franks, B., Madrid, J., Miller, R. L., Perera, F. P., et al. (2013). Sex-specific epigenetic disruption and behavioral changes following low-dose in utero bisphenol A exposure. Proc. Natl. Acad. Sci. U.S.A. 110, 9956–9961. doi: 10.1073/pnas.1214056110
Kurian, J. R., Keen, K. L., Kenealy, B. P., Garcia, J. P., Hedman, C. J., and Terasawa, E. (2015). Acute influences of bisphenol A exposure on hypothalamic release of gonadotropin-releasing hormone and kisspeptin in female rhesus monkeys. Endocrinology 156, 2563–2570. doi: 10.1210/en.2014-1634
Kwon, B., Lee, H. K., and Querfurth, H. W. (2014). Oleate prevents palmitate-induced mitochondrial dysfunction, insulin resistance and inflammatory signaling in neuronal cells. Biochim. Biophys. Acta 1843, 1402–1413. doi: 10.1016/j.bbamcr.2014.04.004
Liu, Y., Beyer, A., and Aebersold, R. (2016). On the dependency of cellular protein levels on mRNA abundance. Cell 165, 535–550. doi: 10.1016/j.cell.2016.03.014
Mahachoklertwattana, P., Black, S. M., Kaplan, S. L., Bristow, J. D., and Grumbach, M. M. (1994). Nitric oxide synthesized by gonadotropin-releasing hormone neurons is a mediator of N-methyl-D-aspartate (n.d.)-induced GnRH secretion. Endocrinology 135, 1709–1712. doi: 10.1210/endo.135.4.7523101
Matsumoto, M., Saito, T., Takasaki, J., Kamohara, M., Sugimoto, T., Kobayashi, M., et al. (2000). An evolutionarily conserved G-protein coupled receptor family, SREB, expressed in the central nervous system. Biochem. Biophys. Res. Commun. 272, 576–582. doi: 10.1006/bbrc.2000.2829
Mayer, C. M., and Belsham, D. D. (2009). Insulin directly regulates NPY and AgRP gene expression via the MAPK MEK/ERK signal transduction pathway in mHypoE-46 hypothalamic neurons. Mol. Cell. Endocrinol. 307, 99–108. doi: 10.1016/j.mce.2009.02.031
Migrenne, S., Cruciani-Guglielmacci, C., Kang, L., Wang, R., Rouch, C., Lefèvre, A.-L., et al. (2006). Fatty acid signaling in the hypothalamus and the neural control of insulin secretion. Diabetes Metab. Res. Rev. 55, S139–S144. 10.2337/db06-S017
Nakamoto, K., Nishinaka, T., Sato, N., Mankura, M., Koyama, Y., Kasuya, F., et al. (2013). Hypothalamic GPR40 signaling activated by free long chain fatty acids suppresses CFA-induced inflammatory chronic pain. PLoS One 8:e81563. doi: 10.1371/journal.pone.0081563
Oh, D. Y., Talukdar, S., Bae, E. J., Imamura, T., Morinaga, H., Fan, W., et al. (2010). GPR120 is an omega-3 fatty acid receptor mediating potent anti-inflammatory and insulin-sensitizing effects. Cell 142, 687–698. doi: 10.1016/j.cell.2010.07.041
Patisaul, H. B., Todd, K. L., Mickens, J. A., and Adewale, H. B. (2009). Impact of neonatal exposure to the ERalpha agonist PPT, bisphenol-A or phytoestrogens on hypothalamic kisspeptin fiber density in male and female rats. Neurotoxicology 30, 350–357. doi: 10.1016/j.neuro.2009.02.010
Peretz, J., Vrooman, L., Ricke, W. A., Hunt, P. A., Ehrlich, S., Hauser, R., et al. (2014). Bisphenol A and reproductive health: update of experimental and human evidence, 2007–2013. Environ. Health Perspect. 122, 775–786. doi: 10.1289/ehp.1307728
Perez-Perez, A., Sanchez-Jimenez, F., Maymo, J., Duenas, J. L., Varone, C., and Sanchez-Margalet, V. (2015). Role of leptin in female reproduction. Clin. Chem. Lab. Med. 53, 15–28. doi: 10.1515/cclm-2014-0387
Plant, T. M. (2015). Neuroendocrine control of the onset of puberty. Front. Neuroendocrinol. 38, 73–88. doi: 10.1016/j.yfrne.2015.04.002
Prague, J. K., and Dhillo, W. S. (2015). Potential clinical use of kisspeptin. Neuroendocrinology 102, 238–245. doi: 10.1159/000439133
Qiu, X., Dowling, A. R., Marino, J. S., Faulkner, L. D., Bryant, B., Bruning, J. C., et al. (2013). Delayed puberty but normal fertility in mice with selective deletion of insulin receptors from Kiss1 cells. Endocrinology 154, 1337–1348. doi: 10.1210/en.2012-2056
Radovick, S., Ticknor, C. M., Nakayama, Y., Notides, A. C., Rahman, A., Weintraub, B. D., et al. (1991). Evidence for direct estrogen regulation of the human gonadotropin-releasing hormone gene. J. Clin. Invest. 88, 1649–1655. doi: 10.1172/JCI115479
Roa, J., and Herbison, A. E. (2012). Direct regulation of GnRH neuron excitability by arcuate nucleus POMC and NPY neuron neuropeptides in female mice. Endocrinology 153, 5587–5599. doi: 10.1210/en.2012-1470
Rocca, C., Scavello, F., Granieri, M. C., Pasqua, T., Amodio, N., Imbrogno, S., et al. (2017). Phoenixin-14: detection and novel physiological implications in cardiac modulation and cardioprotection. Cell. Mol. Life Sci. 75, 743–756. doi: 10.1007/s00018-017-2661-3
Roy, D., Angelini, N. L., and Belsham, D. D. (1999). Estrogen directly respresses gonadotropin-releasing hormone (GnRH) gene expression in estrogen receptor-alpha (ERalpha)- and ERbeta-expressing GT1-7 GnRH neurons. Endocrinology 140, 5045–5053. doi: 10.1210/endo.140.11.7117
Sahu, A. (2003). Leptin signaling in the hypothalamus: emphasis on energy homeostasis and leptin resistance. Front. Neuroendocrinol. 24, 225–253. 10.1016/j.yfrne.2003.10.001
Salehi, A., Loganathan, N., and Belsham, D. D. (2018). Bisphenol A induces Pomc gene expression through neuroinflammatory and PPARgamma nuclear receptor-mediated mechanisms in POMC-expressing hypothalamic neuronal models. Mol. Cell. Endocrinol. doi: 10.1016/j.mce.2018.08.009 [Epub ahead of print].
Schalla, M., Prinz, P., Friedrich, T., Scharner, S., Kobelt, P., Goebel-Stengel, M., et al. (2017). Phoenixin-14 injected intracerebroventricularly but not intraperitoneally stimulates food intake in rats. Peptides 96, 53–60. doi: 10.1016/j.peptides.2017.08.004
Shi, H., Kokoeva, M. V., Inouye, K., Tzameli, I., Yin, H., and Flier, J. S. (2006). TLR4 links innate immunity and fatty acid-induced insulin resistance. J. Clin. Invest. 116, 3015–3025. doi: 10.1172/JCI28898
Stein, L. M., Tullock, C. W., Mathews, S. K., Garcia-Galiano, D., Elias, C. F., Samson, W. K., et al. (2016). Hypothalamic action of phoenixin to control reproductive hormone secretion in females: importance of the orphan G protein-coupled receptor Gpr173. Am. J. Physiol. Regul. Integr. Comp. Physiol. 311, R489–R496. doi: 10.1152/ajpregu.00191.2016
Suda, T., Balakier, H., Powell, W., and Casper, R. F. (1990). Use of gonadotropin-releasing hormone agonist to trigger follicular maturation for in vitro fertilization. J. Clin. Endocrinol. Metab. 71, 918–922. doi: 10.1210/jcem-71-4-918
Takayanagi, S., Tokunaga, T., Liu, X., Okada, H., Matsushima, A., and Shimohigashi, Y. (2006). Endocrine disruptor bisphenol A strongly binds to human estrogen-related receptor ?? (ERR??) with high constitutive activity. Toxicol. Lett. 167, 95–105. doi: 10.1016/j.toxlet.2006.08.012
Titolo, D., Cai, F., and Belsham, D. D. (2006). Coordinate regulation of neuropeptide Y and agouti-related peptide gene expression by estrogen depends on the ratio of estrogen receptor (ER) alpha to ERbeta in clonal hypothalamic neurons. Mol. Endocrinol. 20, 2080–2092. doi: 10.1210/me.2006-0027
Tomza-Marciniak, A., Stepkowska, P., Kuba, J., and Pilarczyk, B. (2018). Effect of bisphenol A on reproductive processes: a review of in vitro, in vivo and epidemiological studies. J. Appl. Toxicol. 38, 51–80. doi: 10.1002/jat.3480
Tran, D. Q., Ramos, E. H., and Belsham, D. D. (2016). Induction of Gnrh mRNA expression by the omega-3 polyunsaturated fatty acid docosahexaenoic acid and the saturated fatty acid palmitate in a GnRH-synthesizing neuronal cell model, mHypoA-GnRH/GFP. Mol. Cell. Endocrinol. 426, 125–135. doi: 10.1016/j.mce.2016.02.019
Treen, A. K., Luo, V., and Belsham, D. D. (2016). Phoenixin activates immortalized GnRH and kisspeptin neurons through the novel receptor GPR173. Mol. Endocrinol. 30, 872–888. doi: 10.1210/me.2016-1039
Tuteja, N., and Chandra, M. (2004). Nitric oxide as a unique bioactive signaling messenger in physiology and pathophysiology. J. Biomed. Biotechnol. 2004, 227–237. doi: 10.1155/S1110724304402034
Ubuka, T., Son, Y. L., Bentley, G. E., Millar, R. P., and Tsutsui, K. (2013). Gonadotropin-inhibitory hormone (GnIH), GnIH receptor and cell signaling. Gen. Comp. Endocrinol. 190, 10–17. doi: 10.1016/j.ygcen.2013.02.030
Wang, M., Deng, S. P., Chen, H. P., Jiang, D. N., Tian, C. X., Yang, W., et al. (2018). Phoenixin participated in regulation of food intake and growth in spotted scat, Scatophagus argus. Comp. Biochem. Physiol. B. Biochem. Mol. Biol. 226, 36–44. doi: 10.1016/j.cbpb.2018.07.007
Watanobe, H. (2002). Leptin directly acts within the hypothalamus to stimulate gonadotropin-releasing hormone secretion in vivo in rats. J. Physiol. 545, 255–268. doi: 10.1113/jphysiol.2002.023895
Welshons, W. V., Nagel, S. C., and Vom Saal, F. S. (2006). Large effects from small exposures. III. Endocrine mechanisms mediating effects of bisphenol A at levels of human exposure. Endocrinology 147, S56–S69. doi: 10.1210/en.2005-1159
Ye, J., Coulouris, G., Zaretskaya, I., Cutcutache, I., Rozen, S., and Madden, T. L. (2012). Primer-BLAST: a tool to design target-specific primers for polymerase chain reaction. BMC Bioinform. 13:134. doi: 10.1186/1471-2105-13-134
Ye, W., Ramos, E. H., Wong, B. C., and Belsham, D. D. (2016). Beneficial effects of metformin and/or salicylate on palmitate- or TNFalpha-induced neuroinflammatory marker and neuropeptide gene regulation in immortalized NPY/AgRP neurons. PLoS One 11:e0166973. doi: 10.1371/journal.pone.0166973
Yin, W., Mendenhall, J. M., Monita, M., and Gore, A. C. (2009). Three-dimensional properties of GnRH neuroterminals in the median eminence of young and old rats. J. Comp. Neurol. 517, 284–295. doi: 10.1002/cne.22156
Yosten, G. L., Lyu, R. M., Hsueh, A. J., Avsian-Kretchmer, O., Chang, J. K., Tullock, C. W., et al. (2013). A novel reproductive peptide, phoenixin. J. Neuroendocrinol. 25, 206–215. doi: 10.1111/j.1365-2826.2012.02381.x
Keywords: phoenixin, hypothalamus, fatty acids, endocrine-disrupting chemical bisphenol A, gene expression, signal transduction
Citation: McIlwraith EK, Loganathan N and Belsham DD (2018) Phoenixin Expression Is Regulated by the Fatty Acids Palmitate, Docosahexaenoic Acid and Oleate, and the Endocrine Disrupting Chemical Bisphenol A in Immortalized Hypothalamic Neurons. Front. Neurosci. 12:838. doi: 10.3389/fnins.2018.00838
Received: 12 September 2018; Accepted: 29 October 2018;
Published: 15 November 2018.
Edited by:
Pierrette Gaudreau, Université de Montréal, CanadaReviewed by:
Laurent Gautron, University of Texas Southwestern Medical Center, United StatesAlexandre Benani, Centre National de la Recherche Scientifique (CNRS), France
Copyright © 2018 McIlwraith, Loganathan and Belsham. This is an open-access article distributed under the terms of the Creative Commons Attribution License (CC BY). The use, distribution or reproduction in other forums is permitted, provided the original author(s) and the copyright owner(s) are credited and that the original publication in this journal is cited, in accordance with accepted academic practice. No use, distribution or reproduction is permitted which does not comply with these terms.
*Correspondence: Denise D. Belsham, d.belsham@utoronto.ca