Higher Levels of Plasma Hyaluronic Acid and N-terminal Propeptide of Type III Procollagen Are Associated With Lower Kidney Function in Children With Non-alcoholic Fatty Liver Disease
- 1Hepatology, Gastroenterology and Nutrition Unit, Bambino Gesù Children's Hospital, IRCCS, Rome, Italy
- 2Section of Endocrinology, Diabetes and Metabolism, Department of Medicine, University and Azienda Ospedaliera Universitaria Integrata of Verona, Verona, Italy
- 3Research Unit of Molecular Genetics of Complex Phenotypes, Bambino Gesù Children's Hospital, IRCCS, Rome, Italy
- 4Unit of Pathology, Bambino Gesù Children's Hospital, IRCCS, Rome, Italy
- 5Southampton National Institute for Health Research Biomedical Research Centre, Southampton General Hospital, University Hospital Southampton, Southampton, United Kingdom
- 6Nutrition and Metabolism, Faculty of Medicine, University of Southampton, Southampton, United Kingdom
Objective: Hyaluronic acid (HA) and N-terminal propeptide of type III procollagen (PIIINP) are two non-invasive biomarkers of liver fibrosis in non-alcoholic fatty liver disease (NAFLD). We examined the relationships of plasma levels of HA and PIIINP with kidney function in children with NAFLD.
Methods: Plasma HA and PIIINP levels were measured using two commercially available enzyme-linked immunosorbent assay kits in a cohort of 106 Caucasian overweight or obese children with biopsy-proven NAFLD. Glomerular filtration rate (eGFR) was estimated using the Bedside Schwartz equation. Genotyping for the patatin-like phospholipase domain-containing protein-3 (PNPLA3) rs738409 variant was performed using an allelic discrimination assay.
Results: Children with fibrosis F2 had significantly higher plasma PIIINP and HA levels than those with F0 or F1 fibrosis. Liver fibrosis was positively associated with plasma HA and PIIINP, as well as with the presence of the risk allele G of PNPLA3 rs738409 variant, and negatively with eGFR. Moreover, eGFR showed significant inverse associations with HA and PIIINP levels, as well as the presence of G of PNPLA3 rs738409, and liver fibrosis stage. Notably, our multivariable regression models showed that higher plasma PIIINP (standardized beta coefficient: −0.206, P = 0.011) and HA levels (standardized beta coefficient: −0.531, P < 0.0001) were associated with lower eGFR values, even after adjustment for age, sex, systolic blood pressure, PNPLA3 rs738409 genotype, and any stage of liver fibrosis.
Conclusions: Higher levels of HA and PIIINP were associated with lower eGFR values in Caucasian children with biopsy-proven NAFLD, independently of PNPLA3 rs738409 genotype and other potential confounding factors.
Introduction
Pediatric non-alcoholic fatty liver disease (NAFLD) has become an important public health problem in several developed countries, and with the exponential increase in childhood obesity, the estimated prevalence of NAFLD is near to 25% (1, 2). NAFLD is a multifactorial disease that encompasses simple steatosis, non-alcoholic steatohepatitis (NASH), advanced fibrosis and, ultimately, cirrhosis (3).
To date, accumulating evidence indicates that NAFLD is not only associated with adverse liver-related outcomes, but also with cardiovascular and kidney complications in both adults and children or adolescents (4–8). Several studies suggest that the relationship between NAFLD and chronic kidney disease could start in childhood (9). In particular, Targher et al. reported a significant association between patatin-like phospholipase domain-containing protein-3 (PNPLA3) rs738409 polymorphism (i.e., the major genetic variant associated with greater susceptibility to NAFLD development and progression) and kidney dysfunction in a pediatric population with histologically confirmed NAFLD. In this study the authors showed that the presence of the risk allele (G) of rs738409 was closely associated with decreasing estimated glomerular filtration rate (eGFR) values and increasing 24-h urinary protein excretion in Caucasian overweight children and adolescents with biopsy-proven NAFLD (10). Furthermore, Yodoshi et al. found that the renal impairment was significantly associated with liver disease severity in 179 children and adolescents with NAFLD. Twenty percent of these patients had glomerular hyper-filtration and 15% had a decreased eGFR within 3 months of their liver biopsy. Besides, glomerular hyper-filtration was associated with a higher histological NAFLD Activity score (NAS), independent of traditional renal risk factors, such as obesity, type 2 diabetes, and hypertension (11).
We hypothesized that concomitant liver and kidney disease in NAFLD could share common pathogenic mechanisms. We propose that liver and kidney disease could share common biomarkers of extracellular matrix (ECM) remodeling, such as increased hyaluronic acid (HA) and N-terminal propeptide of type III procollagen (PIIINP), which are two circulating biomarkers closely associated with the severity of liver fibrosis (12–14).
Hence, the main aim of our cross-sectional study was to evaluate the association of plasma HA and PIIINP levels with kidney dysfunction in children with biopsy-proven NAFLD.
Methods
Patients
A total of 106 Caucasian overweight or obese children and adolescents with ultrasound-defined hepatic steatosis (irrespective of serum aminotransferase levels) were enrolled to the Hepato-Metabolic Department of IRCCS “Bambino Gesù” Children's Hospital in Rome. All patients underwent liver biopsy for diagnosing and staging NAFLD. This practice was in agreement with the diagnostic flow chart proposed by the European Society for Pediatric Gastroenterology, Hepatology, and Nutrition Committee (15).
All children were tested to exclude secondary causes of hepatic steatosis, including alcohol consumption, total parenteral nutrition and chronic use of drugs known to induce hepatic steatosis (e.g., valproate, amiodarone, or prednisone). Hepatitis A, B, and C, cytomegalovirus, Epstein-Barr virus infections, and coeliac disease were also excluded using appropriate serological tests. Autoimmune liver disease, metabolic liver disease, Wilson's disease, and alpha-1-antitrypsin-associated liver disease were ruled out using standard clinical, laboratory, and histological criteria.
The study was carried out according to the rules of the Helsinki Declaration. Written informed consent was obtained from the parents of each child.
Anthropometric, Clinical and Biochemical Measurements
Body mass index (BMI) and waist circumference (WC) were calculated as previously described (14). Blood pressure was measured in the right arm using a standard sphygmomanometer; and an average of three blood pressure values was reported. High blood pressure was defined by systolic or diastolic blood pressure >95th percentile for age, height and sex.
Venous blood samples were collected in the morning after an overnight fast of at least 8 h. Serum liver enzymes [aspartate aminotransferase (AST), alanine aminotransferase (ALT) and gamma-glutamyltransferase (GGT)], lipids [i.e., total cholesterol, high-density lipoprotein (HDL)-cholesterol, low-density lipoprotein (LDL)-cholesterol and triglycerides], glucose, insulin, high-sensitivity C-reactive protein (hs-CRP), uric acid, blood urea nitrogen and creatinine were measured in all children using standard laboratory procedures. The homeostasis model assessment-insulin resistance [HOMA-IR = insulin (mIU/L) x glucose (mmol/L)/22.5] was used to estimate a measure of insulin sensitivity. A cut-off value of HOMA-IR score >2.5 was considered as an index of insulin resistance (16). The estimated glomerular filtration rate (eGFR) was estimated using the Bedside Schwartz equation (eGFR = 0.413 x [height (cm)/serum creatinine (mg/dL)]), which has been documented to be accurate for estimating GFR in pediatric populations (17).
Liver Histology
A diagnosis of NASH was established from liver biopsy, as previously reported (14). The characterized histological features of NAFLD were steatosis, portal and lobular inflammation, hepatocyte ballooning and fibrosis that were evaluated with the use of the scoring system developed by the National Institutes of Health-sponsored NASH Clinical Research Network (18). Steatosis was graded on a four-point scale: 0 = steatosis involving fewer than 5% of hepatocytes, 1 = steatosis involving up to 33% of hepatocytes, 2 = steatosis involving 33–66% of hepatocytes, and 3 = steatosis involving more than 66% of hepatocytes. Lobular inflammation was graded on a four-point scale: 0 = no foci, 1 = fewer than two foci per 200 × field, 2 = two to four foci per 200 × field, and 3 = more than four foci per 200 × field. Portal inflammation was graded on a four-point scale: 0 = none, 1 = mild, 2 = moderate, and 3 = severe. Hepatocyte ballooning was graded on a three-point scale: 0 = no ballooned cells, 1 = few ballooned cells and 2 = many/prominent ballooned cells. The stage of fibrosis was quantified using a five-point scale: 0 = no fibrosis, 1 = peri-sinusoidal or periportal fibrosis [(1a) mild, zone 3, perisinusoidal; (1b) moderate, zone 3, perisinusoidal; and (1c) portal/periportal], 2 = peri-sinusoidal and portal/periportal fibrosis, 3 = bridging fibrosis, and 4 = cirrhosis (19). The NAFLD activity score (NAS) was defined as the sum of the scores for steatosis, lobular inflammation, and ballooning. Cases with NAS of 3 and 4 were diagnosed as borderline NASH, whereas cases with scores of ≥5 were diagnosed as definite NASH. This diagnosis was reviewed and confirmed by an expert pathologist (RDV). Histological characteristics of children with NAFLD are reported in Supplementary Table 1.
PIIINP and HA Measurements
For assessment of plasma PIIINP and HA levels, 3–4 ml of venous blood samples were collected in EDTA buffered tubes by each subject after an overnight fast. Blood samples were centrifuged at 2,000 g by 10 min using a refrigerated centrifuge and plasma samples were stored at −80°C. Plasma concentrations of HA and PIIINP were measured by commercially available ELISA kits (Hyaluronan Quantikine ELISA, R&D, code: DHYAL0, R&D Systems, Minneapolis, MN, United States; Human Procollagen type III N-terminal Propeptide ELISA Kit, code: NBP2-76434, Centennial, CO, United States), according to the manufacturer's instructions.
PNPLA3 Genotyping
The rs738409 C>G polymorphism in PNPLA3 gene has been genotyped by TaqMan 5'-nuclease (Applied Biosystems, Foster City, CA, United States) by staff unaware of the clinical status of patients. Briefly, genomic DNA was isolated from venous blood using a Blood DNA Extraction Kit (Qiagen, Valencia, CA, United States). The absorbance ratio at 260/280 nm of all the samples ranged from 1.8 to 2 indicating they were all free from contaminants. Real-time PCR was performed using Applied Biosystems 7900HT Fast Real-Time PCR System (Applied Biosystems, Carlsbad, CA, United States). Positive and negative controls were included on each reaction plate to verify the reproducibility of the results.
Statistical Analysis
Data are expressed as means ± SD, medians and interquartile ranges (IQR), or percentages. Differences in anthropometric, clinical and biochemical characteristics among the patient groups were tested by using the Fisher's exact test for categorical variables, the one-way ANOVA for normally distributed continuous variables, or the Kruskal-Wallis test for non-normally distributed continuous variables. Pearson's correlation coefficients were calculated to examine univariable linear associations between plasma HA or PIIINP levels (after logarithmic transformation) and liver fibrosis or eGFR values.
Mann-Whitney U-test with Bonferroni adjustment was used to compare differences between independent samples. The medians for continuous parameters and the values si = 1 and no = 0 for ordinals were considered.
Subsequently, multivariable linear regression modeling was used to test the independence of associations between these two circulating biomarkers as key exposures, with eGFR values as the key outcome (as a continuous measure), after adjusting for potential confounding factors, such as age, sex, systolic blood pressure, PNPLA3 rs738409 genotype, and presence of any stage of liver fibrosis. Covariates included in all multivariable regression models were selected as potential confounders based on their significance in univariable regression analyses or based on their biological plausibility.
Statistical analyses were performed using STATA software, version 14.2 (STATA, College Station, TX, United States). A P < 0.05 was considered statistically significant.
Results
We evaluated a cohort composed of 106 overweight and obese children with biopsy-proven NAFLD. Main clinical and biochemical characteristics of these children are reported stratified by increasing eGFR tertiles in Supplementary Table 2. Next, we also evaluated the anthropometric, biochemical and clinical characteristics of these children, stratified by increasing stages of liver fibrosis. Specifically, as shown in Table 1, age, sex, body weight, BMI, blood pressure, total and LDL-cholesterol, fasting glucose, insulin, HOMA-IR, hs-CRP, and liver enzymes did not differ significantly among the three groups of children. On the contrary, WC, HDL-cholesterol, triglycerides, uric acid, blood urea nitrogen, creatinine, and eGFR were significantly different between the three patient groups (P ≤ 0.05). Of note, as shown in Table 2, children with NAFLD and fibrosis F ≥ 2 also had markedly higher levels of plasma PIIINP and HA than those with fibrosis F0 or F1 (P < 0.001 for the trend). In addition, children with fibrosis F ≥ 2 also had a higher prevalence of the genotype GG and CG PNPLA3 rs738409 (P = 0.0048).
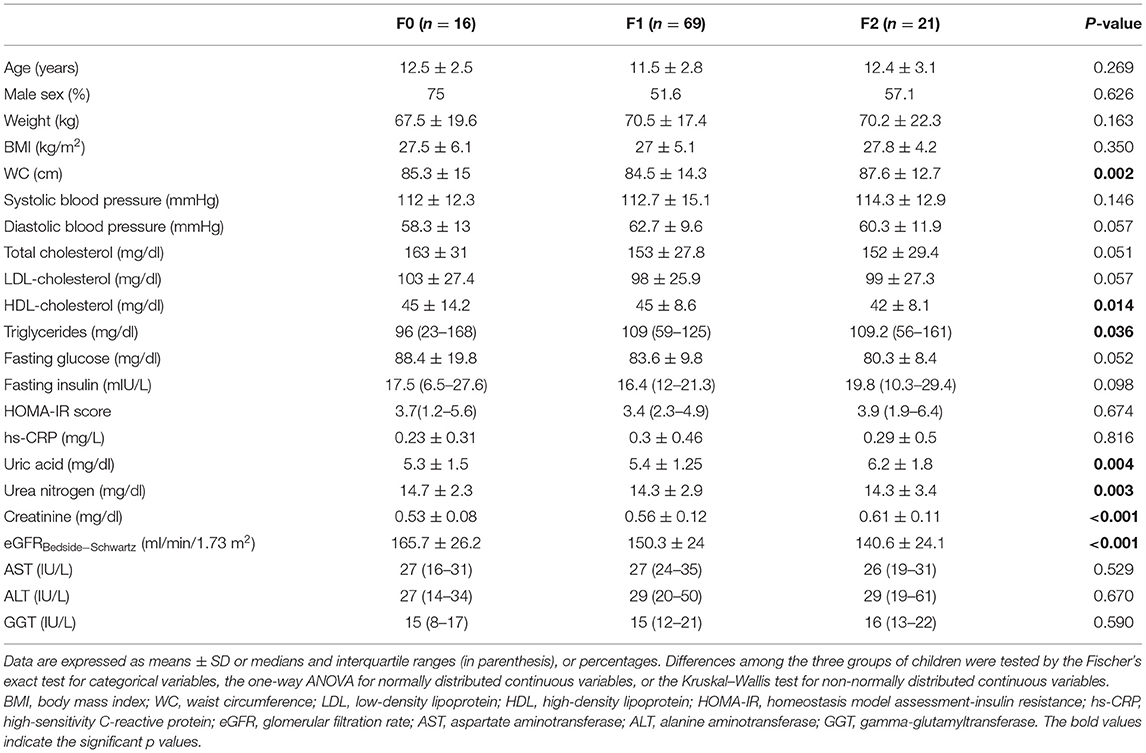
Table 1. Main anthropometric and clinical characteristics of children with biopsy-confirmed NAFLD, stratified by increasing histological stages of liver fibrosis.
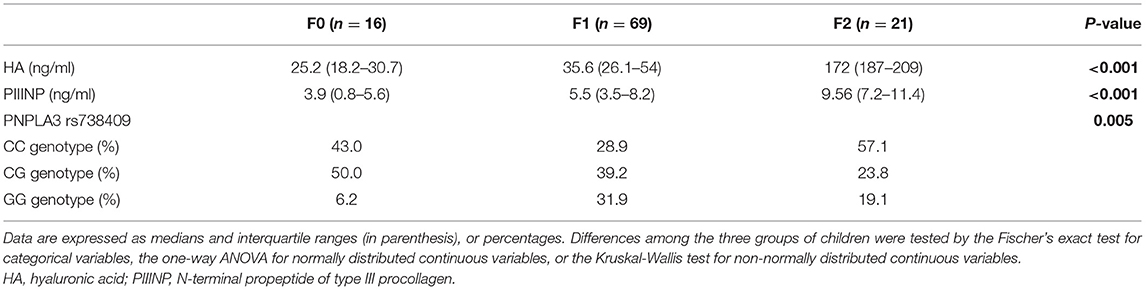
Table 2. Biomarkers of extracellular matrix remodeling and genetic parameters of children with biopsy-confirmed NAFLD, stratified by increasing histological stages of liver fibrosis.
As shown in Table 3, there was a negative correlation between stages of fibrosis and eGFR values (r = −0.22; P = 0.03). Furthermore, increasing fibrosis stage (F ≥ 1) was positively correlated with levels of both HA (r = 0.56; P = 0.0001) and PIIINP (r = 0.38; P = 0.0001), as well as with the presence of the risk allele G of PNPLA3 rs738409 variant (r = 0.28; P = 0.003). In contrast, eGFR values were negatively correlated with levels of HA (r = −0.42; P < 0.0001) and PIIINP (r = −0.33; P = 0.006), as well as with the presence of the risk allele G of PNPLA3 rs738409 variant (r = −0.33; P = 0.006).
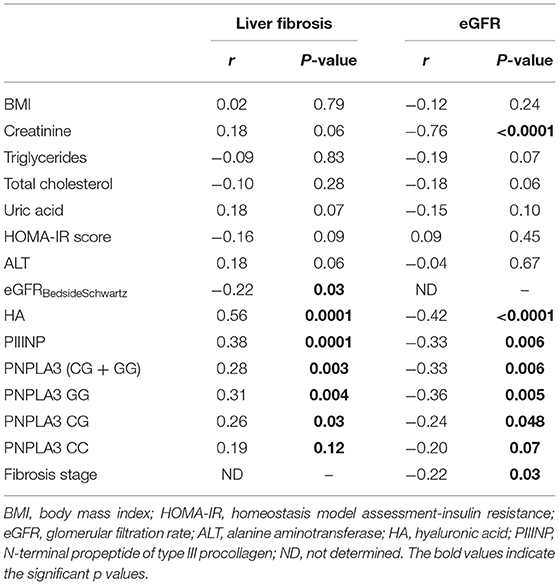
Table 3. Univariate associations between clinical/biochemical and genetic variables with levels of liver fibrosis or eGFR, in children with biopsy-proven NAFLD.
The U-test also showed (Supplementary Table 3) that PNPLA3-CG+GG, PIIINP and HA are also associated with fibrosis (z-score = 2.01, = 2.88; = 3.11; P < 0.05), while PIIINP and HA are also associated with portal inflammation (z-score = 5.8, = 5.5, P < 0.05).
In Table 4 are reported the independent associations between either plasma PIIINP or HA levels and eGFR values (included as a continuous measure) after adjusting for the risk allele G of PNPLA3 rs738409 variant, any stage of liver fibrosis and other potential confounding factors.
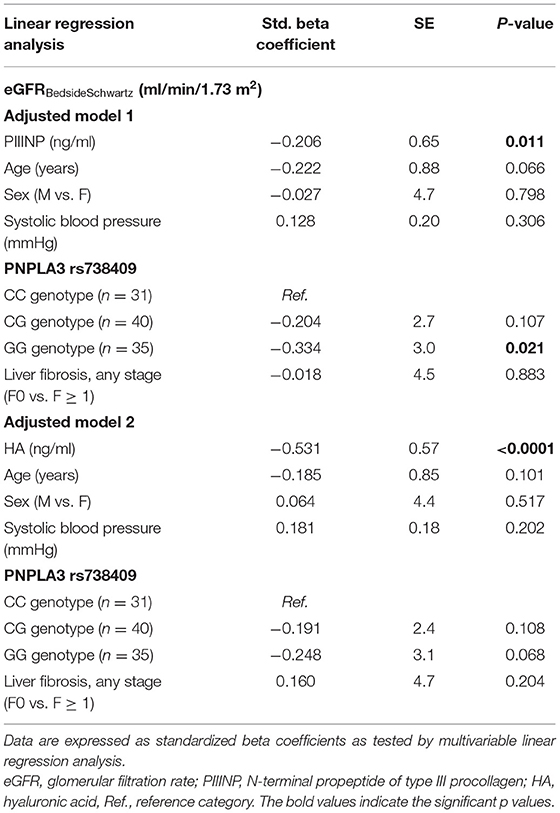
Table 4. Independent associations between eGFR values (included as a continuous measure) and plasma levels of either PIIINP (model 1) or HA (model 2), adjusted for age, sex, systolic blood pressure, PNPLA3 rs738409 genotype, and stages of liver fibrosis in children with NAFLD.
In adjusted model 1, we found that increasing plasma PIIINP levels (included as logarithmically transformed variable) were associated with lower eGFR values (standardized beta coefficient: −0.206; P = 0.01), independent of age, sex, PNPLA3 rs738409 genotype and histologic stage of liver fibrosis (F0 vs. F1 ≥ 1). In this model, the GG PNPLA3 rs738409 genotype was also independently associated with lower eGFR values. Conversely, in the adjusted model 2, only increasing plasma HA levels were significantly associated with lower eGFR values (standardized beta coefficient: −0.531; P < 0.0001) after adjustment for the aforementioned covariates.
Discussion
Our observational study reports for the first time a significant association between higher plasma HA or PIIINP levels and lower kidney function parameters in a pediatric NAFLD population. In particular, the main finding of our study was that plasma levels of both HA and PIIINP were negatively associated with eGFRBedsideSchwartz values.
While the role of circulating levels of HA and PIIINP as biomarkers of liver fibrosis has been well established in children with NAFLD (14, 20, 21), the specific contribution of these two biomarkers in predicting lower eGFR values in a setting of pediatric NAFLD has not been investigated. HA is a high molecular weight glycosaminoglycan with linear polysaccharide structure that is synthesized by several cell types, including fibroblasts (22). Over the last decade, it has become increasingly evident that circulating levels of HA are strongly related to the severity of liver fibrosis in patients with various chronic liver diseases (including NAFLD), probably owing to the increased extracellular matrix deposition in hepatic fibrosis and the reduced clearance of HA by sinusoidal endothelial cells in the liver (23). Increased levels of HA have been found in renal tissue and serum in both experimental models and human samples of several kidney diseases (24, 25). Moreover, in a case-control study of 164 critically ill adult patients and 61 healthy controls, Yagmur et al. (26) reported that circulating HA levels were associated with impaired kidney parameters (i.e., eGFR and cystatin C) and liver function (i.e., albumin and pseudocholinesterase). Our study confirms these findings in a cohort of overweight or obese children and/or adolescents with biopsy-confirmed NAFLD, showing that higher plasma levels of HA were closely associated with lower values of eGFRBedsideSchwartz. The independent and inverse association we observed between plasma HA levels and eGFR in pediatric NAFLD might have two possible explanations. Firstly, as HA is involved in regulating the development of inflammation and fibrosis in the kidney, the increase in circulating levels of this biomarker might represent a trait of ECM remodeling occurring during development and progression of kidney disease (27). Alternatively, as suggested by Pecoits-Filho et al. (28), as HA is partially cleared by the kidney, circulating levels of this biomarker might reflect renal dysfunction.
PIIINP is a procollagen III cleavage product that can be used as a circulating biomarker of ECM remodeling during liver fibrogenesis (29, 30), but a role for this molecule in kidney disease has also been reported in adult patients with various stages of chronic kidney disease (CKD) (31). In that study, Ghoul et al. (31) documented that a higher urinary PIIINP/creatinine ratio (UPIIINP/Cr) was associated with lower eGFR values, higher CKD stage, and greater interstitial renal fibrosis determined by kidney histology. Subsequently, other studies showed that higher PIIINP levels, both in urine and serum, were associated with increasing stages of CKD and with interstitial renal fibrosis in both adults and children (32, 33). According with these findings, we have shown that higher levels of plasma PIIINP were strongly associated with lower eGFR values in our children with NAFLD, even if the strength of this association was slightly lower than that observed for HA. A potential mechanistic explanation for this association might also be linked to increased ECM turnover in the damaged organ. This may occur as a result of breakdown of renal interstitial collagen III by multiple collagenolytic enzymes, released by infiltrating inflammatory cells as impaired renal function occurs (32). Future mechanistic studies are required to better understand the precise molecular mechanisms underpinning the association between plasma HA and PIIINP levels and kidney dysfunction in NAFLD. Moreover, it could be informative to investigate other surrogate markers, such as lumican, which may provide a direct measure of ECM protein remodeling rate and collagen turnover occurring in the fibrotic liver (34).
Finally, we found that the major risk PNPLA3 allele G for NAFLD was also associated with higher liver fibrosis stage and lower eGFR, thereby confirming observations from previous studies, showing the potential impact of this genetic polymorphism on the link between NAFLD and CKD (35).
Our study has some important limitations that should be mentioned. Firstly, this study has a cross-sectional design that does not allow us to establish the temporality and causality of the observed associations. Secondly, it is possible that the single ethnicity of our study participants may cause selection bias; thus our results might not be generalizable to other pediatric populations of different ethnicity. Thirdly, we were unable to directly measure GFR (by plasma iohexol disappearance) and, therefore, we used the most widely accepted prediction formula for estimating GFR in our pediatric population (i.e., the Bedside Schwartz formula) (17). Despite these limitations, our study has several important strengths, including the relatively large sample size, the consecutive enrolment of participants, the database completeness, and the use of liver biopsy to diagnose and stage NAFLD.
In conclusion, our study shows for the first time that higher circulating levels of HA and PIIINP are significantly associated with lower eGFR values in overweight or obese children with biopsy-proven NAFLD. Notably, these associations remained significant even after adjustment for common renal risk factors, presence of liver fibrosis and the PNPLA3 rs738409 genotype.
Data Availability Statement
The original contributions presented in the study are included in the article/Supplementary Material, further inquiries can be directed to the corresponding author/s.
Ethics Statement
The studies involving human participants were reviewed and approved by Bambino Gesù Children's Hospital Ethics Commitee. Written informed consent to participate in this study was provided by the participants' legal guardian/next of kin.
Author Contributions
AA, GT, and CB contributed to study design, data interpretation, wrote the manuscript, and reviewed and edited the manuscript. AMo, AMa, AC, NP, and MB contributed to the analysis and interpretation of data and wrote the manuscript. DC and RD contributed to data collection and clinical interpretation. All authors approved the final submitted version of the manuscript.
Funding
This study was supported by the 5x1000 2022 to AA; CB was supported in part by the Southampton NIHR Biomedical Research Center (IS-BRC-20004), United Kingdom. GT was supported in part by grants from the University School of Medicine of Verona, Verona, Italy.
Conflict of Interest
The authors declare that the research was conducted in the absence of any commercial or financial relationships that could be construed as a potential conflict of interest.
Publisher's Note
All claims expressed in this article are solely those of the authors and do not necessarily represent those of their affiliated organizations, or those of the publisher, the editors and the reviewers. Any product that may be evaluated in this article, or claim that may be made by its manufacturer, is not guaranteed or endorsed by the publisher.
Acknowledgments
We thank the Nicolò' Valenti ONLUS, for supporting AC fellowship.
Supplementary Material
The Supplementary Material for this article can be found online at: https://www.frontiersin.org/articles/10.3389/fped.2022.917714/full#supplementary-material
References
1. Nobili V, Alisi A, Valenti L, Miele L, Feldstein AE, Alkhouri N, et al. In children: new genes, new diagnostic modalities and new drugs. Nat Rev Gastroenterol Hepatol. (2019) 16:517–30. doi: 10.1038/s41575-019-0169-z
2. Shapiro WL, Noon SL, Schwimmer JB. Recent advances in the epidemiology of non-alcoholic fatty liver disease in children. Pediatr Obes. (2021) 16:e12849. doi: 10.1111/ijpo.12849
3. Mann JP, Valenti L, Scorletti E, Byrne CD, Nobili V. Non-alcoholic fatty liver disease in children. Semin Liver Dis. (2018) 38:1–13. doi: 10.1055/s-0038-1627456
4. Targher G, Byrne CD, Tilg H. NAFLD and increased risk of cardiovascular disease: clinical associations, pathophysiological mechanisms and pharmacological implications. Gut. (2020) 69:1691–705. doi: 10.1136/gutjnl-2020-320622
5. Pacifico L, Bonci E, Andreoli GM, Di Martino M, Gallozzi A, De Luca E, et al. The impact of non-alcoholic fatty liver disease on renal function in children with overweight/obesity. Int J Mol Sci. (2016) 17:1218. doi: 10.3390/ijms17081218
6. Manco M, Ciampalini P, DeVito R, Vania A, Cappa M, Nobili V. Albuminuria and insulin resistance in children with biopsy proven non-alcoholic fatty liver disease. Pediatr Nephrol. (2009) 24:1211–7. doi: 10.1007/s00467-009-1134-9
7. Di Costanzo A, Pacifico L, D'Erasmo L, Polito L, Martino MD, Perla FM, et al. Non-alcoholic fatty liver disease (NAFLD), but not its susceptibility gene variants, influences the decrease of kidney function in overweight/obese children. Int J Mol Sci. (2019) 20:4444. doi: 10.3390/ijms20184444
8. Mantovani A, Scorletti E, Mosca A, Alisi A, Byrne CD, Targher G. Complications, morbidity and mortality of non-alcoholic fatty liver disease. Metabolism. (2020) 111S:154170. doi: 10.1016/j.metabol.2020.154170
9. Di Sessa A, Guarino S, Melone R, De Simone RF, Marzuillo P, Miraglia Del Giudice E. Relationship between non-alcoholic fatty liver disease and chronic kidney disease could start in childhood. World J Gastroenterol. (2021) 27:5793–5. doi: 10.3748/wjg.v27.i34.5793
10. Targher G, Mantovani A, Alisi A, Mosca A, Panera N, Byrne CD, et al. Relationship between PNPLA3 rs738409 polymorphism and decreased kidney function in children with NAFLD. Hepatology. (2019) 70:142–53. doi: 10.1002/hep.30625
11. Yodoshi T, Arce-Clachar AC, Sun Q, Fei L, Bramlage K, Xanthakos SA, et al. Glomerular hyperfiltration is associated with liver disease severity in children with non-alcoholic fatty liver disease. J Pediatr. (2020) 222:127–33. doi: 10.1016/j.jpeds.2020.03.038
12. Sharma N, Sircar A, Anders HJ, Gaikwad AB. Crosstalk between kidney and liver in non-alcoholic fatty liver disease: mechanisms and therapeutic approaches. Arch Physiol Biochem. (2020) 1–15. doi: 10.1080/13813455.2020.1745851
14. Mosca A, Comparcola D, Romito I, Mantovani A, Nobili V, Byrne CD, et al. Plasma N-terminal propeptide of type III procollagen accurately predicts liver fibrosis severity in children with non-alcoholic fatty liver disease. Liver Int. (2019) 39:2317–29. doi: 10.1111/liv.14225
15. Vajro P, Lenta S, Socha P, Dhawan A, McKiernan P, Baumann U, et al. Diagnosis of non-alcoholic fatty liver disease in children and adolescents: position paper of the ESPGHAN Hepatology Committee. J Pediatr Gastroenterol Nutr. (2012) 54:700–13. doi: 10.1097/MPG.0b013e318252a13f
16. Conwell LS, Trost SG, Brown WJ, Batch JA. Indexes of insulin resistance and secretion in obese children and adolescents: a validation study. Diabetes Care. (2004) 27:314–9. doi: 10.2337/diacare.27.2.314
17. Schwartz GJ, Muñoz A, Schneider MF, Mak RH, Kaskel F, Warady BA, et al. New equations to estimate GFR in children with CKD. J Am Soc Nephrol. (2009) 20:629–37. doi: 10.1681/ASN.2008030287
18. Kleiner DE, Brunt EM, Van Natta M, Behling C, Contos MJ, Cummings OW, et al. Design and validation of a histological scoring system for non-alcoholic fatty liver disease. Hepatology. (2005) 41:1313–21. doi: 10.1002/hep.20701
19. Brunt EM, Kleiner DE, Wilson LA, Belt P, Neuschwander-Tetri BA, NASH Clinical Research Network (CRN). Non-alcoholic fatty liver disease (NAFLD) activity score and the histopathologic diagnosis in NAFLD: distinct clinicopathologic meanings. Hepatology. (2011) 53:810–20. doi: 10.1002/hep.24127
20. Nobili V, Parkes J, Bottazzo G, Marcellini M, Cross R, Newman D, et al. Performance of ELF serum markers in predicting fibrosis stage in pediatric non-alcoholic fatty liver disease. Gastroenterology. (2009) 136:160–7. doi: 10.1053/j.gastro.2008.09.013
21. Nobili V, Alisi A, Torre G, De Vito R, Pietrobattista A, Morino G, et al. Hyaluronic acid predicts hepatic fibrosis in children with non-alcoholic fatty liver disease. Transl Res. (2010) 156:229–34. doi: 10.1016/j.trsl.2010.05.008
22. Albeiroti S, Soroosh A, de la Motte CA. Hyaluronan's role in fibrosis: a pathogenic factor or a passive player? Biomed Res Int. (2015) 2015:790203. doi: 10.1155/2015/790203
23. Matsuda M, Seki E. The liver fibrosis niche: novel insights into the interplay between fibrosis-composing mesenchymal cells, immune cells, endothelial cells, and extracellular matrix. Food Chem Toxicol. (2020) 143:111556. doi: 10.1016/j.fct.2020.111556
24. Honkanen E, Fröseth B, Grönhagen-Riska C. Serum hyaluronic acid and procollagen III amino terminal propeptide in chronic renal failure. Am J Nephrol. (1991) 11:201–6. doi: 10.1159/000168304
25. Stridh S, Palm F, Hansell P. Renal interstitial hyaluronan: functional aspects during normal and pathological conditions. Am J Physiol Regul Integr Comp Physiol. (2012) 302:R1235–49. doi: 10.1152/ajpregu.00332.2011
26. Yagmur E, Koch A, Haumann M, Kramann R, Trautwein C, Tacke F. Hyaluronan serum concentrations are elevated in critically ill patients and associated with disease severity. Clin Biochem. (2012) 45:82–7. doi: 10.1016/j.clinbiochem.2011.10.016
27. Clotet-Freixas S, Konvalinka A. Too little or too much? Extracellular matrix remodeling in kidney health and disease. J Am Soc Nephrol. (2021) 32:1541–3. doi: 10.1681/ASN.2021050654
28. Pecoits-Filho R, Heimbürger O, Bárány P, Suliman M, Fehrman-Ekholm I, Lindholm B, et al. Associations between circulating inflammatory markers and residual renal function in CRF patients. Am J Kidney Dis. (2003) 41:1212–8. doi: 10.1016/S0272-6386(03)00353-6
29. Adams LA. Biomarkers of liver fibrosis. J Gastroenterol Hepatol. (2011) 26:802–9. doi: 10.1111/j.1440-1746.2010.06612.x
30. Martínez SM, Crespo G, Navasa M, Forns X. Non-invasive assessment of liver fibrosis. Hepatology. (2011) 53:325–35. doi: 10.1002/hep.24013
31. Ghoul BE, Squalli T, Servais A, Elie C, Meas-Yedid V, Trivint C, et al. Urinary procollagen III aminoterminal propeptide (PIIINP): a fibrotest for the nephrologist. Clin J Am Soc Nephrol. (2010) 5:205–10. doi: 10.2215/CJN.06610909
32. Jianguo W, Zhenzhen L, Xianghua L, Zhanzheng Z, Suke S, Suyun W. Serum and urinary procollagen III aminoterminal propeptide as a biomarker of obstructive nephropathy in children. Clin Chim Acta. (2014) 434:29–33. doi: 10.1016/j.cca.2014.04.005
33. Taranta-Janusz K, Moczulska A, Nosek H, Michaluk-Skutnik J, Klukowski M, Wasilewska A. Urinary procollagen III aminoterminal propeptide and β-catenin - new diagnostic biomarkers in solitary functioning kidney? Adv Med Sci. (2019) 64:189–94. doi: 10.1016/j.advms.2018.10.002
34. Decaris ML, Li KW, Emson CL, Gatmaitan M, Liu S, Wang Y, et al. Identifying non-alcoholic fatty liver disease patients with active fibrosis by measuring extracellular matrix remodeling rates in tissue and blood. Hepatology. (2017) 65:78–88. doi: 10.1002/hep.28860
Keywords: children, NAFLD, kidney function, procollagen, hyaluronic acid
Citation: Mosca A, Mantovani A, Crudele A, Panera N, Comparcola D, De Vito R, Bianchi M, Byrne CD, Targher G and Alisi A (2022) Higher Levels of Plasma Hyaluronic Acid and N-terminal Propeptide of Type III Procollagen Are Associated With Lower Kidney Function in Children With Non-alcoholic Fatty Liver Disease. Front. Pediatr. 10:917714. doi: 10.3389/fped.2022.917714
Received: 11 April 2022; Accepted: 03 May 2022;
Published: 06 June 2022.
Edited by:
Claudia Mandato, AORN Santobono-Pausilipon, ItalyReviewed by:
Nicola Santoro, Yale University, United StatesGiulia Paolella, IRCCS Ca' Granda Foundation Maggiore Policlinico Hospital, Italy
Copyright © 2022 Mosca, Mantovani, Crudele, Panera, Comparcola, De Vito, Bianchi, Byrne, Targher and Alisi. This is an open-access article distributed under the terms of the Creative Commons Attribution License (CC BY). The use, distribution or reproduction in other forums is permitted, provided the original author(s) and the copyright owner(s) are credited and that the original publication in this journal is cited, in accordance with accepted academic practice. No use, distribution or reproduction is permitted which does not comply with these terms.
*Correspondence: Anna Alisi, anna.alisi@opbg.net; Giovanni Targher, giovanni.targher@univr.it
†These authors have contributed equally to this work and share first authorship