- 1Pharmacogenetics Laboratory, Institute of Biochemistry, Faculty of Medicine, University of Ljubljana, Ljubljana, Slovenia
- 2Department of Neurology, University Medical Centre Ljubljana, Ljubljana, Slovenia
Dopaminergic pathway is the most disrupted pathway in the pathogenesis of Parkinson's disease. Several studies reported associations of dopaminergic genes with the occurrence of adverse events of dopaminergic treatment. However, none of these studies adopted a pathway based approach. The aim of this study was to comprehensively evaluate the influence of selected single nucleotide polymorphisms of key dopaminergic pathway genes on the occurrence of motor and non-motor adverse events of dopaminergic treatment in Parkinson's disease. In total, 231 Parkinson's disease patients were enrolled. Demographic and clinical data were collected. Genotyping was performed for 16 single nucleotide polymorphisms from key dopaminergic pathway genes. Logistic and Cox regression analyses were used for evaluation. Results were adjusted for significant clinical data. We observed that carriers of at least one COMT rs165815 C allele had lower odds for developing visual hallucinations (OR = 0.34; 95% CI = 0.16–0.72; p = 0.004), while carriers of at least one DRD3 rs6280 C allele and CC homozygotes had higher odds for this adverse event (OR = 1.88; 95% CI = 1.00–3.54; p = 0.049 and OR = 3.31; 95% CI = 1.37–8.03; p = 0.008, respectively). Carriers of at least one DDC rs921451 C allele and CT heterozygotes had higher odds for orthostatic hypotension (OR = 1.86; 95% CI = 1.07–3.23; p = 0.028 and OR = 2.30; 95% CI = 1.26–4.20; p = 0.007, respectively). Heterozygotes for DDC rs3837091 and SLC22A1 rs628031 AA carriers also had higher odds for orthostatic hypotension (OR = 1.94; 95% CI = 1.07–3.51; p = 0.028 and OR = 2.57; 95% CI = 1.11–5.95; p = 0.028, respectively). Carriers of the SLC22A1 rs628031 AA genotype had higher odds for peripheral edema and impulse control disorders (OR = 4.00; 95% CI = 1.62–9.88; p = 0.003 and OR = 3.16; 95% CI = 1.03–9.72; p = 0.045, respectively). Finally, heterozygotes for SLC22A1 rs628031 and carriers of at least one SLC22A1 rs628031 A allele had lower odds for dyskinesia (OR = 0.48; 95% CI = 0.24–0.98, p = 0.043 and OR = 0.48; 95% CI = 0.25–0.92; p = 0.027, respectively). Gene-gene interactions, more specifically DDC-COMT, SLC18A2-SV2C, and SLC18A2-SLC6A3, also significantly influenced the occurrence of some adverse events. Additionally, haplotypes of COMT and SLC6A3 were associated with the occurrence of visual hallucinations (AT vs. GC: OR = 0.34; 95% CI = 0.16–0.72; p = 0.005) and orthostatic hypotension (ATG vs. ACG: OR = 2.48; 95% CI: 1.01–6.07; p = 0.047), respectively. Pathway based approach allowed us to identify new potential candidates for predictive biomarkers of adverse events of dopaminergic treatment in Parkinson's disease, which could contribute to treatment personalization.
Introduction
Dopamine deficiency resulting from dopaminergic neuron death in the nigrostriatal pathway is the primary chemical disease hallmark of Parkinson's disease (PD). This neurodegenerative disorder presents with motor and non-motor symptoms (Kalia and Lang, 2015; Poewe et al., 2017). Treatment is symptomatic and is based on different dopamine replacement strategies. The most commonly used drugs in PD management are the dopamine receptor agonists (DAs) and the dopamine precursor levodopa (Connolly and Lang, 2014). The treatment of motor symptoms is effective, but adverse events (AEs) are rather common. The most frequent AEs caused by pulsatile levodopa treatment are motor fluctuations and dyskinesia. They are primarily related to levodopa dose and disease severity and usually occur after long-term levodopa treatment, but earlier occurrences may appear. Non-motor AEs, such as excessive daytime sleepiness (EDS) and sleep attacks, visual hallucinations (VHs), nausea/vomiting, orthostatic hypotension (OH), peripheral edema (PE), and impulse control disorders (ICDs) occur less often, usually shortly after treatment initiation and are associated with DAs and less commonly with levodopa (Chou, 2008; Antonini et al., 2009; Wood, 2010; Connolly and Lang, 2014; You et al., 2018). The occurrence of AEs in an individual currently can not be predicted.
Dopaminergic pathway is the most disrupted neurotransmitter pathway in the pathogenesis of PD. Moreover, polymorphic genes encode several enzymes, transporters, and receptors leading to inter-individual variability in the capacity of dopamine synthesis, transport, degradation, and signaling. Dopamine is synthesized in the dopaminergic neurons from tyrosine via levodopa to dopamine by dopa decarboxylase (DDC). It is then transported into synaptic vesicles through vesicular monoamine transporter 2 (SLC18A2). Dopamine is released to the synaptic cleft by exocytosis, where the synaptic vesicle glycoprotein 2C (SV2C) is involved. The signal is then transduced by dopamine receptors (DRD1-5) on postsynaptic neurons or glial cells. Reuptake of dopamine is facilitated via dopamine transporter (SLC6A3) back to the presynaptic neuron, where it gets repackaged into vesicles for future release or metabolized by monoamine oxidase B (MAOB) and catechol-O-methyltransferase (COMT) (Rang et al., 2012; Juarez Olguin et al., 2016; Nishijima and Tomiyama, 2016; You et al., 2018).
Dopamine precursor levodopa as the gold standard treatment of PD is transported through the blood brain barrier by the large neutral amino acid transporter (SLC7A5) and is converted to dopamine in dopaminergic neurons. It can get metabolized also in peripheral tissues by DDC and COMT (Nishijima and Tomiyama, 2016; You et al., 2018). Transport, distribution, and elimination of the drug is facilitated by the transporter SLC22A1 (Becker et al., 2011).
Several pharmacogenomics studies searching for associations between single nucleotide polymorphisms (SNPs) of above mentioned genes and response to dopaminergic treatment have already been performed and some significant results have been reported. DRD1-4 SNPs have already been associated with the occurrence of several AEs of dopaminergic treatment, such as dyskinesia (Zappia et al., 2005; Strong et al., 2006; Lee et al., 2011; Rieck et al., 2012), ICDs (Lee et al., 2009; Zainal Abidin et al., 2015; Krishnamoorthy et al., 2016), nausea/vomiting (Rieck et al., 2016), sleep attacks (Paus et al., 2004; Rissling et al., 2004), and VHs (Goetz et al., 2001). Furthermore, variability of transporter genes has already been associated with drug response. SLC6A3 SNPs have shown associations with dyskinesia (Kaiser et al., 2003; Kaplan et al., 2014), VHs (Schumacher-Schuh et al., 2013), and motor response to acute levodopa challenge (Moreau et al., 2015). SNPs of SLC22A1 and SV2C influenced the levodopa dose (Becker et al., 2011; Altmann et al., 2016). The most studied COMT SNP rs4680 has already been associated with motor fluctuations (Watanabe et al., 2003; Hao et al., 2014; Wu et al., 2014), dyskinesia (Watanabe et al., 2003; Bialecka et al., 2008; de Lau et al., 2012), and daytime sleepiness (Frauscher et al., 2004). Furthermore, MAOB rs1799836 has also been associated with the occurrence of dyskinesia (Hao et al., 2014), while DDC rs921451 and rs3837091 have been associated with the motor response to acute levodopa challenge (Devos et al., 2014). The major limitation of all these studies was that they included only individual genes involved in dopaminergic pathway.
The aim of this study was to comprehensively evaluate the influence of selected SNPs of key dopaminergic pathway genes on the occurrence of motor and non-motor AEs of dopaminergic treatment in PD.
Materials and Methods
Study Participants
A total of 231 unrelated PD patients were enrolled in this retrospective cohort study. Patients were recruited as they were coming for their regular appointment at the Department of Neurology, University Medical Centre Ljubljana, Slovenia between October 2016 and April 2018. Inclusion criteria were (1) diagnosis of PD according to the UK Parkinson Disease Society Brain Bank criteria (Goetz et al., 2008) by an experienced movement disorders specialist, (2) available clinical data, (3) at least 3 months of levodopa and/or DAs treatment duration, (4) ongoing dopaminergic therapy with levodopa and/or DAs. Patients with atypical and secondary forms of parkinsonisms were not included in the study.
Patients and their caregivers underwent a structured interview to obtain demographic and clinical data. Additional information was obtained from the medical records. We focused on eight main AEs of dopaminergic treatment as primary endpoints: motor fluctuations, dyskinesia, EDS and sleep attacks, VHs, nausea/vomiting, OH, PE, and ICDs. The AE was defined as absent or present according to clinical examination, clinical documentation, and patients' answers to specific questions.
The study protocol was approved by the Slovenian Ethics Committee for Research in Medicine (KME 42/05/16). All subjects gave written informed consent in accordance with the Declaration of Helsinki.
SNP Selection
Ten candidate genes were included due to their putative involvement in the dopaminergic pathway (see Supplementary Figure 1) (Redenšek et al., 2018). Several methods were used for SNP selection. First, we searched the literature for SNPs in dopaminergic genes that were already found to be associated with dopaminergic treatment response and the occurrence of AEs. We have also searched for genes involved in dopaminergic pathway (Kanehisa and Goto, 2000) (dopamine synthesis, transport, degradation, and signaling) and their functional SNPs residing in the promoter or coding regions (Sherry et al., 2001). Additionally, we used the SNP function prediction tool (Xu and Taylor, 2009) to select SNPs for the analysis based on their predicted function.
DNA Isolation and Genotyping
Peripheral blood samples were obtained for DNA extraction. Genomic DNA was isolated using the FlexiGene DNA Kit (Qiagen, Hilden, Germany) according to the manufacturer's protocol. Genotyping was performed for 16 SNPs. All of the studied SNPs were genotyped with KASPar assays (KBiosciences, Herts, UK and LGC Genomics, UK) according to manufacturer's instructions. Ten percent of samples were genotyped in duplicate as quality control and all the results were concordant.
Statistical Analysis
Median and 25th to 75th percentile range were used to describe central tendency and variability of continuous variables, while frequencies were used to describe the distribution of categorical variables. The agreement of genotype frequencies with Hardy-Weinberg equilibrium was examined by chi-squared test. Logistic regression was used to calculate odds ratios (ORs) and 95% confidence intervals (95%CIs) to examine the associations of selected SNPs and clinical data with the risk for AEs. Dominant, additive, and recessive genetic models were used for analysis depending on the genotype frequencies. Males and females were analyzed separately for MAOB rs1799836 due to its X chromosome location.
The influence of SNPs on the time to occurrence of motor AEs after levodopa treatment initiation was evaluated by the Cox proportional hazards model to calculate hazard ratio (HR) and the 95%CI.
Gene-gene interactions were examined with logistic regression analysis. The model included two polymorphisms and their interactive term to calculate the OR, 95%CI and p-value for each gene–gene interaction.
A haplotype analysis was carried out to assess the combined effect of multiple SNPs in the same gene. On the basis of genotype data, haplotypes were reconstructed and analyzed using the Thesias program (Tregouet and Garelle, 2007). Only haplotypes with frequencies above 5% were included in the analysis. The most frequent haplotype was used as reference.
All statistical tests were two-sided. Bonferroni correction was used to account for multiple comparisons to prevent false positive results. For genetic data p-values up to 0.0036 (0.05/14) were considered statistically significant, while p values between 0.0036 and 0.0500 were considered nominally significant. For clinical data p-values up to 0.0056 (0.05/9) were considered statistically significant, while p-values between 0.0056 and 0.0500 were considered nominally significant. For an allelic variant with minor allele frequency 0.34 and with a 32% prevalence of an AE, this study had 80% or more power to detect OR of 0.36 or less and OR of 2.31 or more. Power calculation was conducted by the PS Power and sample size calculations, version 3.0. All statistical analyses were carried out by IBM SPSS Statistics, version 21.0 (IBM Corporation, Armonk, NY, USA).
Results
Patients' Characteristics
Patients' median age at enrolment was 72.5 years (65.7–78.0) and median dopaminergic treatment duration was 7.3 years (3.6–13.5). In total, 200 (86.6%) patients experienced at least one of the AEs. The proportion of patients reporting an individual AE varied from 13.9 to 53.2%. On average, 32.2% of patients experienced a specific AE. Demographic and clinical characteristics of patients are presented in Table 1 along with the list and frequencies of AEs.
SNP Genotyping Analysis
Sixteen SNPs were selected for the analysis. COMT rs4680 (p.Val158Met), DRD2 rs1799732 (c.-486_-485insC), and DRD3 rs6280 (p.Gly9Ser) have previously already been associated with the occurrence of AEs in PD treatment according to the literature. Additionally, one functional nonsynonymous SNP rs165815 (p.Arg900Gln) residing in the coding region was selected in the COMT gene. Furthermore, two functional SNPs were selected in genes DRD2 (rs1801028 - p.Ser311Cys) (He et al., 2016), and SLC22A1 (rs628031 - p.Met216Val). Two SNPs in DDC (rs921451 - c.-29+5426A>G and rs3837091 - c.-61_-58delAGAG), one in MAOB (rs1799836 - c.1348-36A>G), and one in SLC6A3 (rs393795 - c.653+4065C>A) were selected due to their previous association with drug response in PD. Additionally, two SLC6A3 SNPs (rs6347 - p.Ser405 = and rs104209 - c.*35T>C), two SLC7A5 SNPs (rs1060253 - c.*438C>G and rs1060257 - c.*1282G>A), one SLC18A2 SNP (rs14240 - c.*294T>A), and one SV2C SNP (rs1423099 - c.-58C>T) were selected with the SNP function prediction tool (Xu and Taylor, 2009).
Investigated SNPs and genotype distributions are presented in Supplementary Table 1. Genotype frequencies did not deviate from Hardy-Weinberg equilibrium (HWE) in the majority of cases (p > 0.05). In the case of DDC rs921451 and rs3837091 the frequencies did not match the HWE requirements. However, frequencies for these two SNPs were not significantly different than frequencies reported in the HapMap-CEU population (p = 0.657 and p = 0.120, respectively). On the other hand, frequencies of SLC7A5 rs1060253 and rs1060257 deviated from HWE and from the HapMap-CEU genotype distributions significantly, so we excluded them from further analysis. None of the SNPs included in the final analysis are in linkage disequilibrium.
Influence of Genetic Variability on the Risk for Adverse Events
Univariate logistic regression identified some possible associations between SNPs and AEs. The significant and nominally significant associations are presented in Table 2, but all associations are given in the Supplementary Tables 2–4. We observed that carriers of at least one COMT rs165815 C allele had lower odds for developing VHs (p = 0.006). Carriers of at least one DRD3 rs6280 C allele had almost two times higher odds (p = 0.033), whereas CC homozygotes had more than three times higher odds for developing VHs (p = 0.006). Genotypes of the DDC SNPs showed associations with the occurrence of OH. Carriers of at least one DDC rs921451 C allele had almost two times higher odds for developing this AE (p = 0.033), whereas under additive model only heterozygotes presented with a nominally significant association (p = 0.009). DDC rs3837091 heterozygotes also had higher odds for OH (p = 0.048). SLC22A1 rs628031 AA carriers had higher odds for developing OH (p = 0.034), peripheral edema (p = 0.003), and ICDs (p = 0.028). On the other hand, SLC22A1 rs628031 heterozygotes had lower odds for presenting with motor fluctuations (p = 0.007) and dyskinesia (p = 0.003). Furthermore, under dominant model carriers of at least one SLC22A1 rs628031 A allele had lower odds for the occurrence of dyskinesia (p = 0.007). Dyskinesia was also more likely to develop in DDC rs3837091 heterozygotes (p = 0.037). Two of the above listed associations with the p = 0.003 were treated as statistically significant results, whereas other associations were treated as nominally significant.
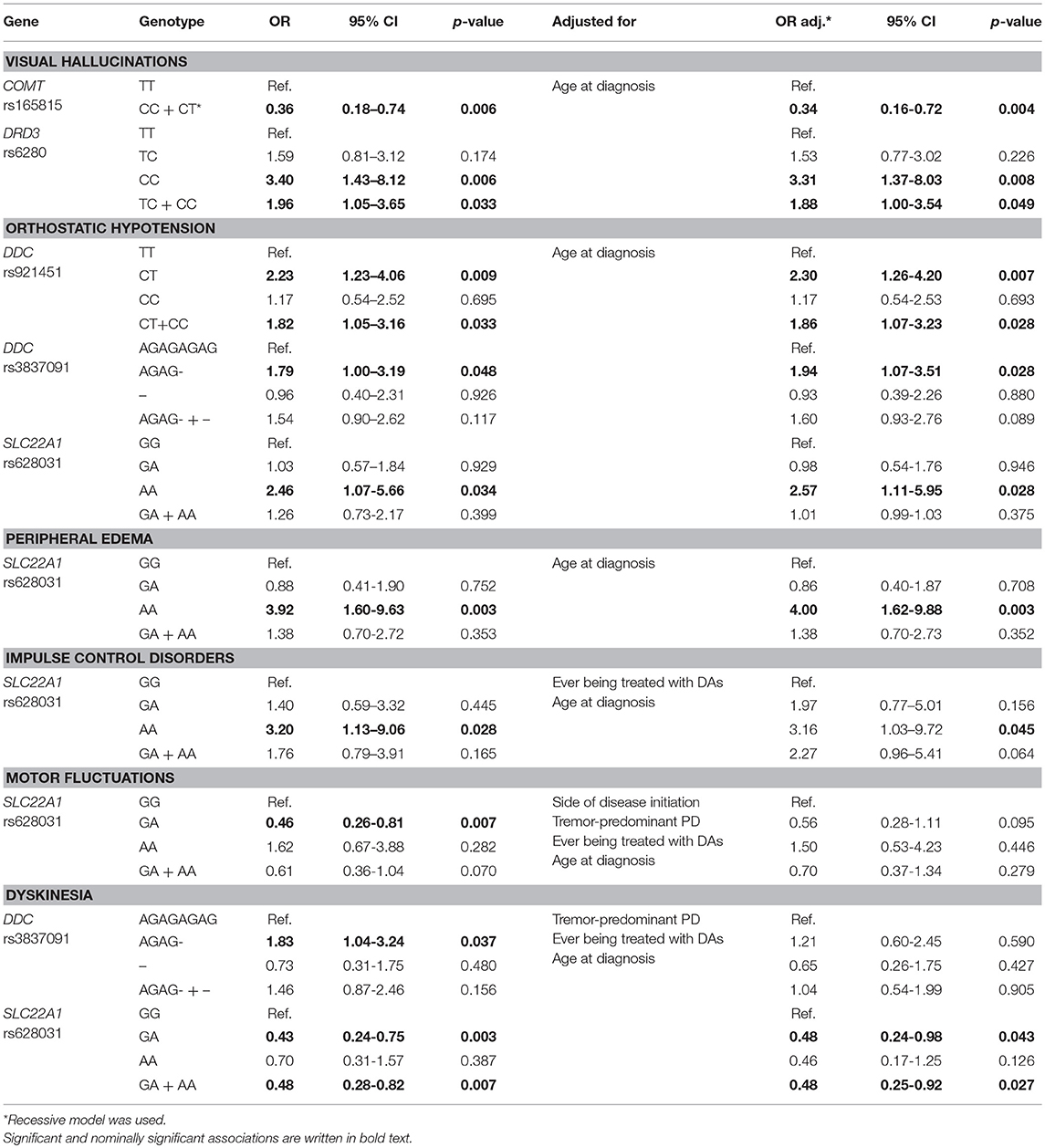
Table 2. Significant and nominally significant associations of the univariate and multivariate logistic analyses of genetic factors with adverse events.
As shown in the Table 3, several clinical parameters were included in our analysis: gender, side of disease initiation, tremor-predominant PD, ever being treated with DAs, age at diagnosis, disease duration, dopaminergic treatment duration, levodopa treatment duration, and LED at enrolment (calculated according to Tomlinson et al., 2010). After univariate logistic regression of these parameters we identified clinical parameters that significantly or nominally significantly affect the occurrence of each AE. These clinical parameters were then used for adjustment of significant or nominally significant genetic factors in multivariate analysis. When considering continuous clinical data only age at diagnosis was used for adjustment due to collinearity of all five continuous parameters. Most of the associations observed in univariate analysis retained their significance level after adjustment as shown in the Table 2.
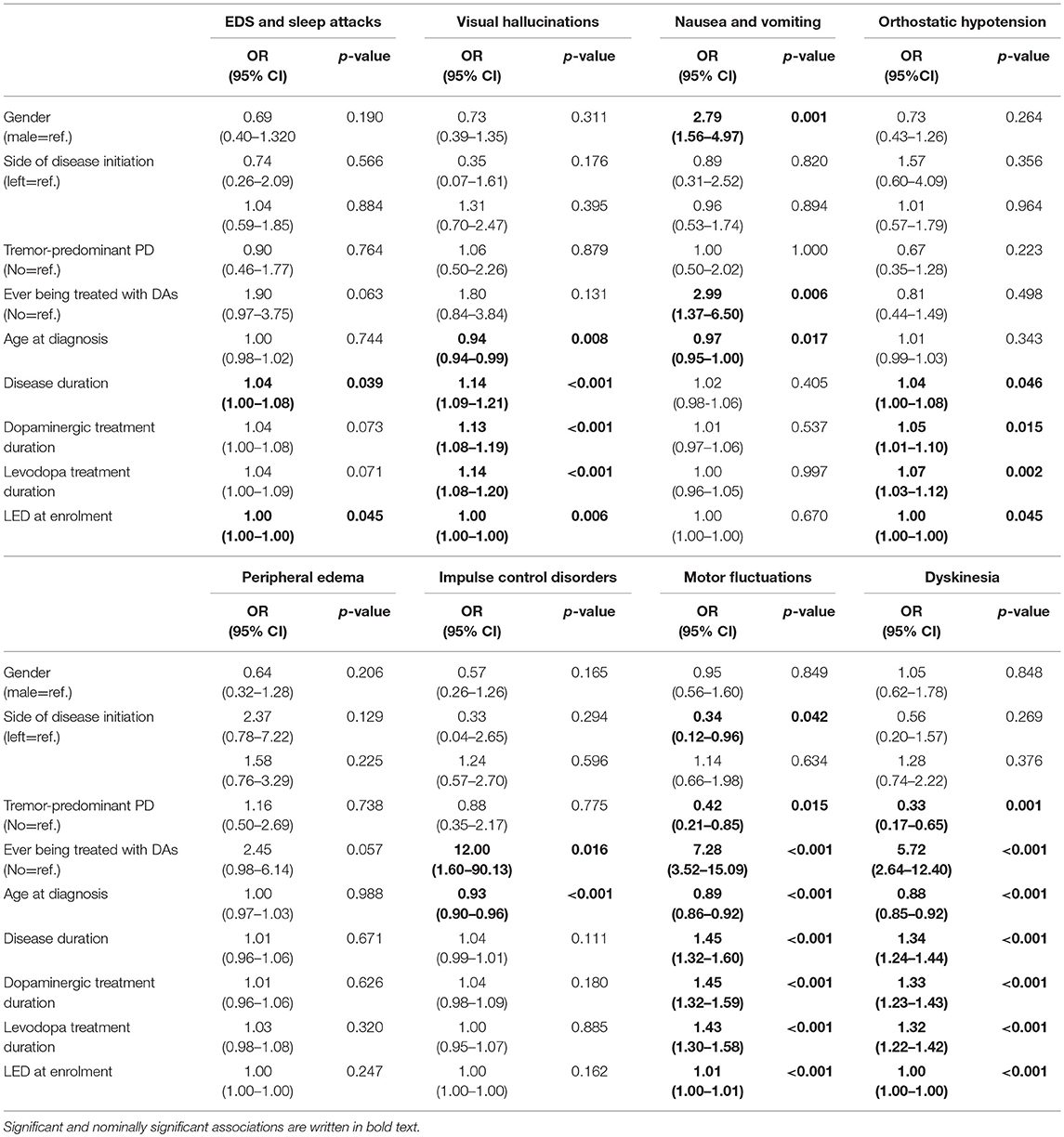
Table 3. Univariate analysis of the influence of clinical parameters on the occurrence of adverse events.
Influence of Genetic Variability on the Time to Occurrence of Motor Adverse Events
We were able to obtain data on the time to occurrence of motor AEs after levodopa treatment initiation. For genetic factors significantly associated with AEs after univariate logistic regression we performed the survival analysis. None of the investigated genetic factors showed any significant effect on the time to occurrence of motor AEs after levodopa treatment initiation. These results are presented in the Supplementary Figure 2.
Influence of Gene-Gene Interactions on the Risk for Adverse Events
According to the structure of dopaminergic pathway, we selected gene pairs that could interact in affecting the occurrence of AEs according to their physiological function. We tested the following gene pairs: COMT-DDC, SLC18A2–SV2C, DRD2–DRD3, and SLC6A3–SLC18A2. Our results suggest that patients with at least one DDC rs921451 C allele and at least one COMT rs165815 C allele had lower odds for developing VHs (OR = 0.16, 95% CI = 0.03–0.78, p = 0.023). Furthermore, patients with at least one DDC rs3837091 deletion allele and at least one COMT rs165815 C allele had lower odds for developing EDS and sleep attacks (OR = 0.28, 95% CI = 0.09–0.94, p = 0.039). Nausea/vomiting were less likely to occur in patients with at least one SLC18A2 rs14240 C allele and at least one SV2C rs1423099 T allele (OR = 0.18, 95%CI = 0.05–0.74, p = 0.017). Moreover, nausea/vomiting were also less likely to occur in patients with at least one SLC18A2 rs14240 C allele and SLC6A3 rs393795 T allele (OR = 0.15, 95% CI = 0.04–0.66, p = 0.012). The presented associations are nominally significant.
Influence of Haplotypes on the Risk for Adverse Events
Only two genes' haplotypes showed nominally significant associations, namely COMT and SLC6A3. All four COMT haplotypes (AT, GT, GC, and AC) were included in the analysis. VHs were less likely to occur in patients with GC haplotype compared to the most common AT haplotype (p = 0.005). Five SLC6A3 haplotypes (ATG, GCG, ATT, ACG, and GTG) were analyzed. They covered 95% of genetic variability. Patients with the ACG haplotype had higher odds for developing OH in comparison to patients with the ATG haplotype (p = 0.047). Three DDC haplotypes (TAGAG, C-, and CAGAG) were included in the analysis and they together covered 100% of the genetic variability, but none of them showed any significant association with any of the AEs. Finally, two DRD2 haplotypes (CC, C-) covering 97% of genetic variability were analyzed, but no significant association was found. Nominally significant results are presented in Table 4, whereas complete results are presented in Supplementary Tables 5–8. No significant associations were observed.
Discussion
In the present study, we examined the association of 14 selected SNPs from dopaminergic pathway genes with AEs related to dopaminergic treatment in PD. We would like to point out two most important findings. First, a very strong effect of genetic variability in the COMT gene on the occurrence of VHs was pointed out in the logistic regression, gene-gene interaction, and haplotype analyses. The COMT rs165815 C allele appeared to be protective against this AE. Another SNP showing strong association was the SLC22A1 rs628031 as its genotype significantly or nominally significantly influenced the occurrence of five AEs: OH, PE, ICDs, motor fluctuations, and dyskinesia. Therefore, these two SNPs seem to be good candidate biomarkers for AEs of dopaminergic treatment in PD.
COMT rs165815 showed a nominally significant protective effect on the VHs' occurrence, whereas COMT rs4680 did not show any association with the studied AEs. The role of the COMT rs4680 has already been thoroughly examined in PD patients. In contrast with our non-significant findings, COMT rs4680 was associated with the occurrence of dyskinesia, motor fluctuations (Watanabe et al., 2003; Bialecka et al., 2008; de Lau et al., 2012; Hao et al., 2014; Wu et al., 2014), and daytime sleepiness (Frauscher et al., 2004) in other studies. The COMT rs165815 has never been studied in PD, but has already been studied in connection to treatment-resistant schizophrenia (Terzić et al., 2016).
Genetic variability of DDC was also associated with the development of AEs. Nominally significant associations were observed between DDC genotypes and OH development in a way that certain genotypes increased risk for the AE's occurrence. Devos et al. (2014) suggested that the studied two SNPs reduce DDC expression or activity. Low enzyme activity leads to low norepinephrine concentration, which may result in vasodilatation and consequently hypotension (Lau et al., 2018). This route of hypotension development is supported by basic agonistic effect of dopaminergic drugs on dopamine receptors, which also leads to vasodilatation. Furthermore, DDC rs3837091 heterozygotes were also more likely to develop dyskinesia. It is plausible that reduced DDC activity may result in higher levodopa bioavailability in the central nervous system and could thus cause dyskinesia. The association did not retain the significance level after adjustment, though. Both DDC SNPs were already associated with motor response to acute levodopa treatment in a previous study (Devos et al., 2014), but have never been associated with AEs of dopaminergic treatment.
Several AEs were associated with genetic variability in the SLC22A1. The rs628031 AA genotype increased risk for OH, PE, and ICDs, whereas GA genotype appeared to be protective against dyskinesia. The association between this SNP and motor fluctuations did not remain significant after adjustment. SLC22A1 rs622342 has been associated with levodopa dose previously (Becker et al., 2011), but SLC22A1 rs628031 has never been examined before in association with PD. Since this SNP appeared to be important in the occurrence of several AEs, it may play a role in the overall drug action and may modify the overall AEs' susceptibility.
DRD3 rs6280 CC genotype was observed as a risk factor for VHs. Similar finding was already reported in the study by Goetz et al. (2001). This SNP may lead to modifications in intracellular signaling via higher binding affinity (Krishnamoorthy et al., 2016; Rieck et al., 2016). We did not confirm the results of some previously published data, such as the association between this SNP and ICDs (Lee et al., 2009; Krishnamoorthy et al., 2016), dyskinesia (Lee et al., 2011), and nausea/vomiting (Rieck et al., 2016), however all of the studied populations differed from our patient cohort in ethnicity (Indian, Korean, and Brazilian, respectively).
We were also not able to confirm the results of some previous studies in other populations. We did not confirm the association between DRD2 rs1799732 and dyskinesia (Rieck et al., 2012) and DRD2 rs1799732 and nausea/vomiting (Rieck et al., 2016) reported in Brazilian patients. We also did not find an association between SLC6A3 rs393795 and dyskinesia as reported in Israeli patients (Kaplan et al., 2014) and the association between MAOB rs1799836 and dyskinesia reported in Chinese patients (Hao et al., 2014).
We also examined the possible influence of significant genetic parameters on the time to occurrence of motor complications after levodopa treatment initiation. No significant associations were found. It is possible that we may have missed this association because the inclusion criterion regarding the treatment duration was set to 3 months, which is rather short. Therefore, some patients could develop AEs later. However, we found some patients experiencing motor complications from the treatment initiation onwards.
We observed a significant influence of the gene-gene interaction COMT-DDC on the occurrence of VHs. Association of COMT rs165815 with VHs was already detected by univariate regression analysis. According to some publications DDC rs921451 may decrease the enzyme's function (Devos et al., 2014), which could lead to reduced dopamine concentrations in the central nervous system. As VHs presumably develop due to elevated dopaminergic stimulation (Rolland et al., 2014), the interaction between DDC and COMT SNPs indeed could lead to reduced odds for this AE. Also EDS and sleep attacks could arise due to elevated dopaminergic stimulation (Knie et al., 2011), which supports the effect of DDC rs3837091 and COMT rs165815 interaction on this AE occurrence as well. The interaction between SLC18A2 rs14240 and SV2C rs1423099 significantly affects the occurrence of nausea/vomiting. Furthermore, the interaction between SLC18A2 rs14240 and SLC6A3 rs393795 significantly influences the occurrence of the same AE. Functional background must still be determined, but might be related to area postrema originating nausea/vomiting (Morris, 1978).
The haplotype analysis additionally supported the observation of COMT genetic variability being involved in VHs' occurrence. According to our results, COMT rs165815 might be a good candidate for a genetic biomarker of protection against this AE. SLC6A3 did not show any associations with AEs in the above described analyses, but a more comprehensive haplotype analysis revealed that the ACG haplotype carriers have more than two times higher odds for developing OH compared to ATG haplotype carriers. This is the first finding of OH being related to this gene. More functional studies are warranted.
Although our study presents some novel findings, some limitations have to be considered. The sample is of moderate size, although it is comparable to the sample sizes of similar PD pharmacogenetic studies and the study power was calculated. Another limitation was that all of the AEs were analyzed as categorical variables. The use of clinical scales to evaluate the severity of various AEs would allow a more in depth analysis of possible associations. A prospective study would have a greater chance to detect even subtler relations between treatment and AEs. It should also be noted that our results should be validated in an independent sample, before they could be applied in a clinical setting.
However, several advantages of this study should be pointed out. All of the patients were recruited from one department, which means that patients were treated according to the same guidelines. Furthermore, these guidelines did not change during the recruitment period. Our study was not biased by genetic heterogeneity since all the patients were recruited in a geographic area with an ethnically homogeneous population (Vidan-Jeras et al., 1998; Mizzi et al., 2016). Study was designed according to a pathway approach, enabling a comprehensive analysis of genetic variability in the dopaminergic pathway.
Conclusions
The results of this study further confirm the role of genetic variability in dopaminergic pathway in the development of AEs of dopaminergic treatment in PD and suggest some new possible predictive biomarkers of the studied AEs, such as COMT rs165815 and SLC22A1 rs628031. Validation of these biomarkers in independent patient cohorts would enable prediction of AEs of dopaminergic treatment in PD and maybe someday also personalized treatment regimens to be implemented in PD management to minimize the burden that AEs present to PD patients.
Author Contributions
All authors have made a substantial intellectual contribution to this work and approved its final version for submission. SR, MT, and VD formed the study focus and organized and executed the study. SR performed the experiments and statistical analysis under the supervision of MT and VD. SR wrote the first draft of the manuscript also under supervision of MT and VD. DF, MK, MG, DG, and ZP helped with patient enrolment and contributed to the final version of the manuscript.
This study was funded by the Slovenian Research Agency (ARRS), grant number P1-0170 and grant for young researchers (SR).
Funding
This study was funded by the Slovenian Research Agency (ARRS), grant number P1-0170 and grant for young researchers (SR).
Conflict of Interest Statement
The authors declare that the research was conducted in the absence of any commercial or financial relationships that could be construed as a potential conflict of interest.
Acknowledgments
We thank all the patients for their participation in the study. We would like to thank Nina Zupančič KriŽnar, MD for her contribution to patient enrolment. We would also like to thank Katja Goričar, Ph.D. for help with statistical analysis, and Tanja Blagus and Savica Soldat for help with the work in the laboratory.
Supplementary Material
The Supplementary Material for this article can be found online at: https://www.frontiersin.org/articles/10.3389/fphar.2019.00008/full#supplementary-material
Abbreviations
PD, parkinson's disease; DAs, dopamine receptor agonists; AEs, adverse events; EDS, excessive daytime sleepiness; VHs, visual hallucinations; OH, orthostatic hypotension; ICDs, impulse control disorders; SNPs, single nucleotide polymorphisms; ORs, odds ratios; CIs, confidence intervals; HR, hazard ratio; HWE, hardy-weinberg equilibrium; PE, peripheral edema; LED, levodopa equivalent dose.
References
Altmann, V., Schumacher-Schuh, A. F., Rieck, M., Callegari-Jacques, S. M., Rieder, C. R., and Hutz, M. H. (2016). Influence of genetic, biological and pharmacological factors on levodopa dose in Parkinson's disease. Pharmacogenomics 17, 481–488. doi: 10.2217/pgs.15.183
Antonini, A., Tolosa, E., Mizuno, Y., Yamamoto, M., and Poewe, W. H. (2009). A reassessment of risks and benefits of dopamine agonists in Parkinson's disease. Lancet Neurol. 8, 929–937. doi: 10.1016/S1474-4422(09)70225-X
Becker, M. L., Visser, L. E., van Schaik, R. H., Hofman, A., Uitterlinden, A. G., and Stricker, B. H. (2011). OCT1 polymorphism is associated with response and survival time in anti-Parkinsonian drug users. Neurogenetics 12, 79–82. doi: 10.1007/s10048-010-0254-5
Bialecka, M., Kurzawski, M., Klodowska-Duda, G., Opala, G., Tan, E. K., and Drozdzik, M. (2008). The association of functional catechol-O-methyltransferase haplotypes with risk of Parkinson's disease, levodopa treatment response, and complications. Pharmacogenet. Genomics 18, 815–821. doi: 10.1097/FPC.0b013e328306c2f2
Chou, K. L. (2008). Adverse events from the treatment of Parkinson's disease. Neurol. Clin. 26, S65-83. doi: 10.1016/j.ncl.2008.05.003
Connolly, B. S., and Lang, A. E. (2014). Pharmacological treatment of Parkinson disease: a review. JAMA 311, 1670–1683. doi: 10.1001/jama.2014.3654
de Lau, L. M., Verbaan, D., Marinus, J., Heutink, P., and van Hilten, J. J. (2012). Catechol-O-methyltransferase Val158Met and the risk of dyskinesias in Parkinson's disease. Mov. Disord. 27, 132–135. doi: 10.1002/mds.23805
Devos, D., Lejeune, S., Cormier-Dequaire, F., Tahiri, K., Charbonnier-Beaupel, F., Rouaix, N., et al. (2014). Dopa-decarboxylase gene polymorphisms affect the motor response to L-dopa in Parkinson's disease. Parkinsonism Relat. Disord. 20, 170–175. doi: 10.1016/j.parkreldis.2013.10.017
Frauscher, B., Högl, B., Maret, S., Wolf, E., Brandauer, E., Wenning, G. K., et al. (2004). Association of daytime sleepiness with COMT polymorphism in patients with parkinson disease: a pilot study. Sleep 27, 733–736. doi: 10.1093/sleep/27.4.733
Goetz, C. G., Burke, P. F., Leurgans, S., Berry-Kravis, E., Blasucci, L. M., Raman, R., et al. (2001). Genetic variation analysis in parkinson disease patients with and without hallucinations: case-control study. Arch. Neurol. 58, 209–213. doi: 10.1001/archneur.58.2.209
Goetz, C. G., Poewe, W., Dubois, B., Schrag, A., Stern, M. B., Lang, A. E., et al. (2008). MDS-UPDRS. Available online at: http://www.movementdisorders.org/MDS-Files1/PDFs/MDS-UPDRS-Rating-Scales/NewUPDRS7308final.pdf (Accessed Sept 18, 2017).
Hao, H., Shao, M., An, J., Chen, C., Feng, X., Xie, S., et al. (2014). Association of Catechol-O-Methyltransferase and monoamine oxidase B gene polymorphisms with motor complications in parkinson's disease in a Chinese population. Parkinsonism Relat. Disord. 20, 1041–1045. doi: 10.1016/j.parkreldis.2014.06.021
He, H., Wu, H., Yang, L., Gao, F., Fan, Y., Feng, J., et al. (2016). Associations between dopamine D2 receptor gene polymorphisms and schizophrenia risk: a PRISMA compliant meta-analysis. Neuropsychiatr. Dis. Treat. 12, 3129–3144. doi: 10.2147/NDT.S118614
Juarez Olguin, H., Calderon Guzman, D., Hernandez Garcia, E., and Barragan Mejia, G. (2016). The Role of dopamine and its dysfunction as a consequence of oxidative stress. Oxid. Med. Cell. Longev. 2016:9730467. doi: 10.1155/2016/9730467
Kaiser, R., Hofer, A., Grapengiesser, A., Gasser, T., Kupsch, A., Roots, I., et al. (2003). L -dopa-induced adverse effects in PD and dopamine transporter gene polymorphism. Neurology 60, 1750–1755. doi: 10.1212/01.WNL.0000068009.32067.A1
Kalia, L. V., and Lang, A. E. (2015). Parkinson's disease. Lancet 386, 896–912. doi: 10.1016/S0140-6736(14)61393-3
Kanehisa, M., and Goto, S. (2000). KEGG: kyoto encyclopedia of genes and genomes. Nucleic Acids Res. 28, 27–30. doi: 10.1093/nar/28.1.27
Kaplan, N., Vituri, A., Korczyn, A. D., Cohen, O. S., Inzelberg, R., Yahalom, G., et al. (2014). Sequence variants in SLC6A3, DRD2, and BDNF genes and time to levodopa-induced dyskinesias in Parkinson's disease. J. Mol. Neurosci. 53, 183–188. doi: 10.1007/s12031-014-0276-9
Knie, B., Mitra, M. T., Logishetty, K., and Chaudhuri, K. R. (2011). Excessive daytime sleepiness in patients with Parkinson's disease. CNS Drugs 25, 203–212. doi: 10.2165/11539720-000000000-00000
Krishnamoorthy, S., Rajan, R., Banerjee, M., Kumar, H., Sarma, G., Krishnan, S., et al. (2016). Dopamine D3 receptor Ser9Gly variant is associated with impulse control disorders in Parkinson's disease patients. Parkinsonism Relat. Disord. 30, 13–17. doi: 10.1016/j.parkreldis.2016.06.005
Lau, A. C. W., Diggle, J. L., and Bring, P. P. (2018). Improvement in severe orthostatic hypotension following carbidopa dose reduction. Can. J. Neurol. Sci. 45, 252–253. doi: 10.1017/cjn.2017.284
Lee, J. Y., Cho, J., Lee, E. K., Park, S. S., and Jeon, B. S. (2011). Differential genetic susceptibility in diphasic and peak-dose dyskinesias in Parkinson's disease. Mov. Disord. 26, 73–79. doi: 10.1002/mds.23400
Lee, J. Y., Lee, E. K., Park, S. S., Lim, J. Y., Kim, H. J., Kim, J. S., et al. (2009). Association of DRD3 and GRIN2B with impulse control and related behaviors in Parkinson's disease. Mov. Disord. 24, 1803–1810. doi: 10.1002/mds.22678
Mizzi, C., Dalabira, E., Kumuthini, J., Dzimiri, N., Balogh, I., Başak, N., et al. (2016). A European spectrum of pharmacogenomic biomarkers: implications for clinical pharmacogenomics. PLoS ONE 11:e0162866. doi: 10.1371/journal.pone.0162866
Moreau, C., Meguig, S., Corvol, J. C., Labreuche, J., Vasseur, F., Duhamel, A., et al. (2015). Polymorphism of the dopamine transporter type 1 gene modifies the treatment response in Parkinson's disease. Brain 138, 1271–1283. doi: 10.1093/brain/awv063
Morris, J. G. (1978). A review of some aspects of the pharmacology of levodopa. Clin. Exp. Neurol. 15, 24–50.
Nishijima, H., and Tomiyama, M. (2016). What mechanisms are responsible for the reuptake of levodopa-derived dopamine in parkinsonian striatum? Front. Neurosci. 10:575. doi: 10.3389/fnins.2016.00575
Paus, S., Seeger, G., Brecht, H. M., Koster, J., El-Faddagh, M., Nöthen, M. M., et al. (2004). Association study of dopamine D2, D3, D4 receptor and serotonin transporter gene polymorphisms with sleep attacks in Parkinson's disease. Mov. Disord. 19, 705–707. doi: 10.1002/mds.20134
Poewe, W., Seppi, K., Tanner, C. M., Halliday, G. M., Brundin, P., Volkmann, J., et al. (2017). Parkinson disease. Nat. Rev. Dis. Primers 3:17013. doi: 10.1038/nrdp.2017.13
Rang, H. P., Dale, M. M., Ritter, J. M., Flower, R. J., and Henderson, G. (2012). Pharmacology. Edinburgh; London: Churchill Livingstone.
Redenšek, S., Trošt, M., and Dolžan, V. (2018). “Effects of genetic variability in dopaminergic pathway on treatment response in Parkinson's disease,” in Parkinson's Disease, ed S. C. Yenisett (Rijeka: IntechOpen), 27–47.
Rieck, M., Schumacher-Schuh, A. F., Altmann, V., Callegari-Jacques, S. M., Rieder, C. R., and Hutz, M. H. (2016). Association between DRD2 and DRD3 gene polymorphisms and gastrointestinal symptoms induced by levodopa therapy in Parkinson's disease. Pharmacogenomics J. 25:79. doi: 10.1038/tpj.2016.79
Rieck, M., Schumacher-Schuh, A. F., Altmann, V., Francisconi, C. L., Fagundes, P. T., Monte, T. L., et al. (2012). DRD2 haplotype is associated with dyskinesia induced by levodopa therapy in Parkinson's disease patients. Pharmacogenomics 13, 1701–1710. doi: 10.2217/pgs.12.149
Rissling, I., Geller, F., Bandmann, O., Stiasny-Kolster, K., Korner, Y., Meindorfner, C., et al. (2004). Dopamine receptor gene polymorphisms in Parkinson's disease patients reporting “sleep attacks”. Mov. Disord. 19, 1279–1284. doi: 10.1002/mds.20245
Rolland, B., Jardri, R., Amad, A., Thomas, P., Cottencin, O., and Bordet, R. (2014). Pharmacology of hallucinations: several mechanisms for one single symptom? Biomed Res. Int. 2014:307106. doi: 10.1155/2014/307106
Schumacher-Schuh, A. F., Francisconi, C., Altmann, V., Monte, T. L., Callegari-Jacques, S. M., Rieder, C. R., et al. (2013). Polymorphisms in the dopamine transporter gene are associated with visual hallucinations and levodopa equivalent dose in Brazilians with Parkinson's disease. Int. J. Neuropsychopharmacol. 16, 1251–1258. doi: 10.1017/S1461145712001666
Sherry, S. T., Ward, M. H., Kholodov, M., Baker, J., Phan, L., Smigielski, E. M., et al. (2001). dbSNP: the NCBI database of genetic variation. Nucleic Acids Res. 29, 308–311. doi: 10.1093/nar/29.1.308
Strong, J. A., Dalvi, A., Revilla, F. J., Sahay, A., Samaha, F. J., Welge, J. A., et al. (2006). Genotype and smoking history affect risk of levodopa-induced dyskinesias in Parkinson's disease. Mov. Disord. 21, 654–659. doi: 10.1002/mds.20785
Terzić, T., Kastelic, M., Dolžan, V., and Plesnicar, B. K. (2016). Genetic polymorphisms in dopaminergic system and treatment-resistant schizophrenia. Psychiatr. Danub. 28, 127–131.
Tomlinson, C. L., Stowe, R., Patel, S., Rick, C., Gray, R., and Clarke, C. E. (2010). Systematic review of levodopa dose equivalency reporting in Parkinson's disease. Mov. Disord. 25, 2649–2653. doi: 10.1002/mds.23429
Tregouet, D. A., and Garelle, V. (2007). A new JAVA interface implementation of THESIAS: testing haplotype effects in association studies. Bioinformatics 23, 1038–1039. doi: 10.1093/bioinformatics/btm058
Vidan-Jeras, B. J. B., Dolžan, V., Jeras, M., Breskvar, K., and Bohinjec, M. (1998). Slovenian Caucasian Normal. Kansas: HLA.
Watanabe, M., Harada, S., Nakamura, T., Ohkoshi, N., Yoshizawa, K., Hayashi, A., et al. (2003). Association between catechol-O-methyltransferase gene polymorphisms and wearing-off and dyskinesia in Parkinson's disease. Neuropsychobiology 48, 190–193. doi: 10.1159/000074637
Wood, L. D. (2010). Clinical review and treatment of select adverse effects of dopamine receptor agonists in Parkinson's disease. Drugs Aging 27, 295–310. doi: 10.2165/11318330-000000000-00000
Wu, H., Dong, F., Wang, Y., Xiao, Q., Yang, Q., Zhao, J., et al. (2014). Catechol-O-methyltransferase Val158Met polymorphism: modulation of wearing-off susceptibility in a Chinese cohort of Parkinson's disease. Parkinsonism Relat. Disord. 20, 1094–1096. doi: 10.1016/j.parkreldis.2014.07.011
Xu, Z., and Taylor, J. A. (2009). SNPinfo: integrating GWAS and candidate gene information into functional SNP selection for genetic association studies. Nucleic Acids Res. 37, W600–5. doi: 10.1093/nar/gkp290
You, H., Mariani, L. L., Mangone, G., Le Febvre de Nailly, D., Charbonnier-Beaupel, F., and Corvol, J. C. (2018). Molecular basis of dopamine replacement therapy and its side effects in Parkinson's disease. Cell Tissue Res. 373, 111–135. doi: 10.1007/s00441-018-2813-2
Zainal Abidin, S., Tan, E. L., Chan, S. C., Jaafar, A., Lee, A. X., Abd Hamid, M. H., et al. (2015). DRD and GRIN2B polymorphisms and their association with the development of impulse control behaviour among Malaysian Parkinson's disease patients. BMC Neurol. 15:59. doi: 10.1186/s12883-015-0316-2
Keywords: Parkinson's disease, genetic polymorphism, dopaminergic pathway, personalized medicine, adverse events
Citation: Redenšek S, Flisar D, Kojović M, Gregorič Kramberger M, Georgiev D, Pirtošek Z, Trošt M and Dolžan V (2019) Dopaminergic Pathway Genes Influence Adverse Events Related to Dopaminergic Treatment in Parkinson's Disease. Front. Pharmacol. 10:8. doi: 10.3389/fphar.2019.00008
Received: 06 November 2018; Accepted: 07 January 2019;
Published: 28 January 2019.
Edited by:
Ulrich M. Zanger, Dr. Margarete Fischer-Bosch Institut für Klinische Pharmakologie (IKP), GermanyReviewed by:
Meenal Gupta, University of Utah, United StatesXi Wu, Shanghai Jiao Tong University, China
Copyright © 2019 Redenšek, Flisar, Kojović, Gregorič Kramberger, Georgiev, Pirtošek, Trošt and Dolžan. This is an open-access article distributed under the terms of the Creative Commons Attribution License (CC BY). The use, distribution or reproduction in other forums is permitted, provided the original author(s) and the copyright owner(s) are credited and that the original publication in this journal is cited, in accordance with accepted academic practice. No use, distribution or reproduction is permitted which does not comply with these terms.
*Correspondence: Vita Dolžan, vita.dolzan@mf.uni-lj.si