- 1Department of Physiology, College of Medicine, Nanchang University, Nanchang, China
- 2Department of Nurse, Nanchang University Hospital, Nanchang, China
- 3Department of Experimental Teaching Center, Nanchang University, Nanchang, China
Alzheimer disease (AD) is characterized as a chronic neurodegenerative disease associated with aging. The clinical manifestations of AD include latent episodes of memory and cognitive impairment, psychiatric symptoms and behavioral disorders, as well as limited activities in daily life. In developed countries, AD is now acknowledged as the third leading cause of death, following cardiovascular disease and cancer. The pathogenesis and mechanism of AD remain unclear, although some theories have been proposed to explain AD, such as the theory of β-amyloid, the theory of the abnormal metabolism of tau protein, the theory of free radical damage, the theory of the inflammatory response, the theory of cholinergic damage, etc. Effective methods to predict, prevent or reverse AD are unavailable, and thus the development of new, efficient therapeutic drugs has become a current research hot spot worldwide. The isolation and extraction of active components from natural drugs have great potential in treating AD. These drugs possess the advantages of multiple targets in multiple pathways, fewer side effects and a long duration of curative effects. This article summaries the latest research progress regarding the mechanisms of natural drugs in the treatment of AD, providing a review of the literature and a theoretical basis for improving the clinical treatment of AD.
Introduction
Alzheimer disease (AD) is characterized as a chronic neurodegenerative disease associated with aging. The clinical manifestations of AD include latent episodes of memory and cognitive impairment, psychiatric symptoms and behavioral disorders, as well as limited activities in daily life (Sun et al., 2013). As the global population ages, dementia has become an important health problem worldwide. It is estimated that the global prevalence of dementia will increase to 81.1 million in 2040 from 24.3 million in 2005 (Chua et al., 2015). In addition, AD is the most common type of dementia, accounting for 60% of all dementia cases. In developed countries, AD is now considered the third main cause of death, after only cardiovascular disease and cancer (Huang et al., 2016). AD usually has a late onset, and its prevalence will double every 5 years after age 65 (Ballard et al., 2011). AD is caused by genetic and environmental factors. Genetic factors account for approximately 70% of cases and are closely related to three variants of the apolipoprotein E (APOE) gene (ε2, ε3, and ε4) (Verghese et al., 2011). Other diseases, such as hypertension, diabetes, and obesity, can increase the risk of AD to different extents (Xu et al., 2015). The pathogenesis and mechanism of AD remain unclear. However, many pathological features are known. The most important pathological features are amyloid plaques and neurofibrillary tangles, which form following the aggregation of β-amyloid (Aβ) proteins and hyperphosphorylated tau protein, respectively. In addition, other pathological features include cell damage caused by oxidative stress, neuroinflammation and injury to cholinergic neurons, which cause neurodegeneration and a loss of synapses and neurons, leading to macroscopic atrophy of the brain regions associated with learning and memory, including the temporal and frontal areas and the hippocampus (Ryu et al., 2019). Therefore, current research mainly focuses on five theories, namely, the β-amyloid theory, abnormal tau protein metabolism theory, free radical damage theory, inflammatory response theory and cholinergic damage theory. All of these theories provide a basis for improving our understanding and research on AD. Currently, the main clinical treatment for AD is acetyl cholinesterase inhibitors (AChEIs) or antagonists of N-methyl-D-aspartate receptor (NMDAR). AChEIs, such as donepezil, galantamine and rivastigmine, are effective in patients with mild and moderate symptoms. These compounds can inhibit acetylcholine (ACh) degradation in the synapse to increase its availability (Birks, 2006). Antagonists of NMDAR, such as memantine, are effective at treating moderate and severe symptoms in patients. These compounds can reduce the excitatory neurotoxicity of L-glutamate without inhibiting its physiological effects (McShane et al., 2006). Based on accumulating evidence, both types of drug improve patients' behavioral and cognitive impairments, but these clinical drug treatments only relieve the symptoms of the disease and do not prevent progression (Huang and Mucke, 2012; Cheung et al., 2015). Thus, the development of new, effective drugs for treating AD has become a global focus. In this review, we have focused on the potential therapeutic effects of natural drug extracts on AD and solved all the taxonomic ambiguities and errors in original papers (Rivera et al., 2014). Our objective is to assess the accuracy of plants scientific nomenclature and to explore the mechanisms by which these natural drug extracts regulate AD.
Treatments Targeting Aβ
As one of the main features of AD, the Aβ protein is the main component of senile plaques. The accumulation of the Aβ protein is the principal pathological factor of AD and is considered the driving force by some scholars (Moon et al., 2014), becoming the most significant target in the prevention and control of AD.
Inhibition of Aβ Formation
Under normal conditions, the β-amyloid precursor protein (APP) is cleaved by α-secretase and γ-secretase, and no Aβ is formed. If the APP gene is mutated, β-secretase and γ-secretase cleave APP to increase Aβ production. Thus, a treatment that selectively increases the activity of α-secretase and decreases the activity of β-secretase will help reduce the formation of Aβ, subsequently slowing the progression of AD. Among these enzymes, β-secretase is the rate-limiting enzyme that catalyzes the production of the Aβ peptide and is a key factor in the pathogenesis of AD. Natural drug products have been used to treat AD for many years, and some extracts inhibit enzymes required for Aβ production. The natural products of traditional Chinese medicine (TCM), which are widely used in folk medicine, display superior safety (Zhang, 2012).
Some researchers injected AD model mice with Aβ and then administered natural drug extracts, achieving considerable results. As shown in the study by Duan et al. (2016), the administration of Angelica sinensis (Oliv.) Diels (Apiaceae) extracts to rats targets β-secretase in the hippocampus and is associated with reduced Aβ levels according to western blots (Figure 1). Jeong et al. (2013) orally administered schisantherin A [Schisandra chinensis (Turcz.) Baill. (Schisandraceae) extracts] to a model mouse strain and observed reduced β-secretase activity and Aβ1-42 levels in the cerebral cortex and hippocampus (Figure 2). According to Qiu et al. (2014), ginsenoside Rh2 (Rh2) extracted from Panax ginseng C.A.Mey. (Araliaceae) (root) increases the soluble APPα level and carboxyl terminal fragment (CTF) α/β ratio in hippocampal neurons, and decreases the concentrations of Aβ40 and Aβ42 when Rh2 is injected into Tg 2576 AD model mice. In addition, Rh2 regulates APP cleavage by decreasing cholesterol levels and the number of lipid rafts, reducing the formation of senile plaques in the brain of mice at this age. The memory impairment of AD model mice was improved by the treatment, and the Morris water maze test showed that the memory and behavior impairment of AD model mice were even reversed by the treatment.
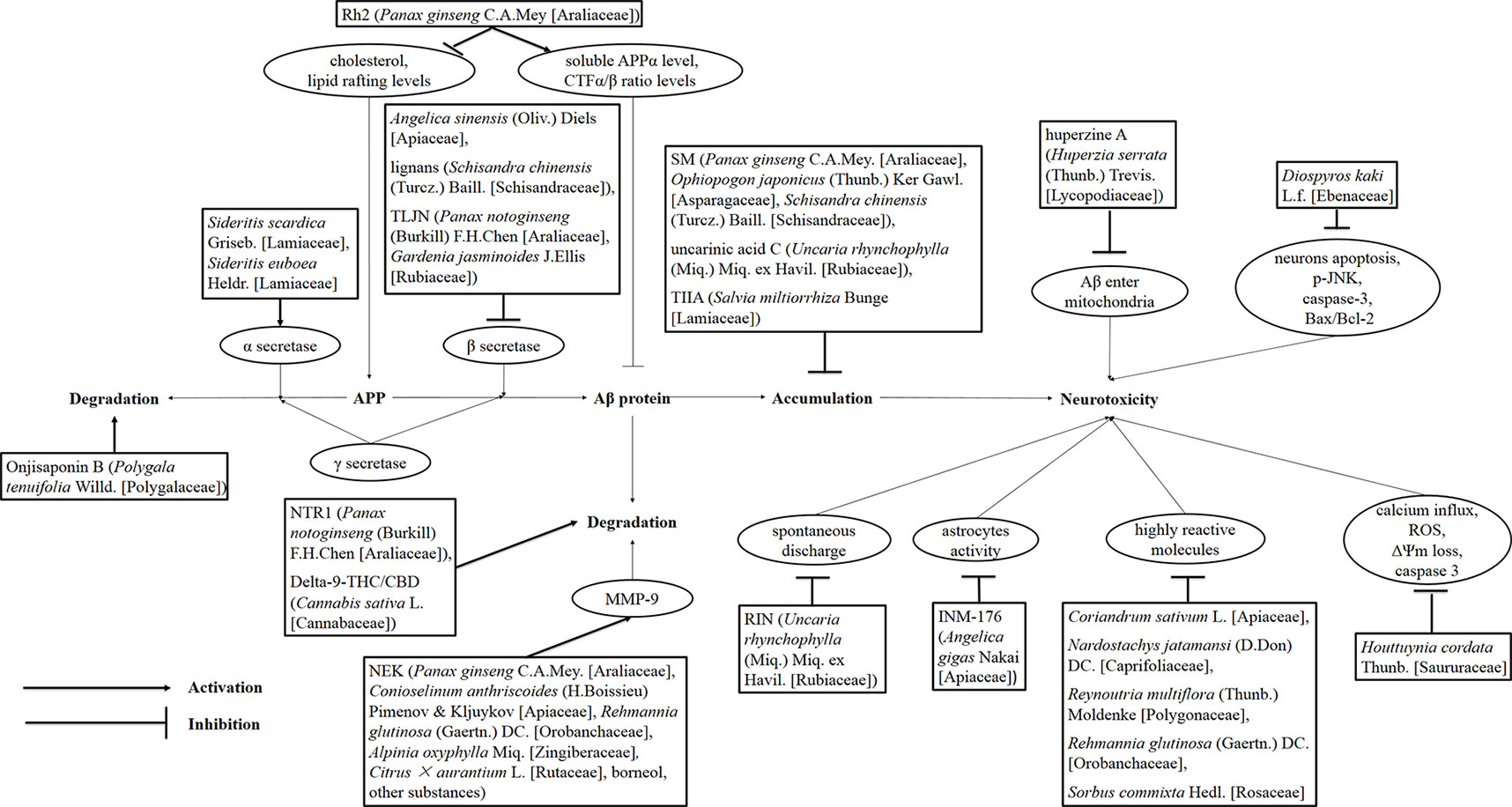
Figure 1 Drug treatment of AD induced by Aβ. Rh2, ginsenoside Rh2; TLJN, TongLuoJiuNao; SM, Shengmai; TIIA, tanshinone IIA; JNK, c-Jun N-terminal kinase; NTR1, notoginsenoside R1; THC, tetrahydrocannabinol; CBD, cannabidiol; NEK, Naoerkang; MMP, matrix metalloproteinase; RIN, rhynchophylline; INM-176, a standardized ethanolic extract of Angelica gigas Nakai (Apiaceae).
On the other hand, APP transgenic mice and nontransgenic aging C57BL/6 mice were orally administered Sideritis scardica Griseb. (Lamiaceae) and Sideritis euboea Heldr. (Lamiaceae) extracts, and the expression of the α-secretase A Desintegrin and Metalloproteinase (ADAM) 10 increased, and the deposition of Aβ42 in APP transgenic mice was substantially reduced. The cognitive ability of elderly, nontransgenic and APP transgenic mice was substantially increased. Compared with the nontransgenic control group, the treatment completely reversed the neuronal loss observed in the APP transgenic mice and even restored the normal number of neurons (Hofrichter et al., 2016). He et al. (2013) chronically administered 6-month-old APP mutant transgenic mice (APP23) the herbal medicine TongLuoJiuNao [TLJN, extracted from Panax notoginseng (Burkill) F.H.Chen (Araliaceae) and Gardenia jasminoides J.Ellis (Rubiaceae) follow by processed and purified in accordance with the protocol of the National Medical Dictionary of China] found that TLJN can decrease the level and activity of β-secretase and the expression of components of the β-secretase complex, significantly reducing the production and deposition of Aβ in the brains of APP23 mice.
Inhibition of Aβ Accumulation
Zhang et al. (2016b) used transgenic Caenorhabditis elegans as a model of AD at the original ecological level and found that the Shengmai (SM) formula water decoction [P. ginseng C.A.Mey. (Araliaceae): Ophiopogon japonicus (Thunb.) Ker Gawl. (Asparagaceae): S. chinensis (Turcz.) Baill. (Schisandraceae)= 9:9:6 (SM), 9:18:9 (SM1), 2:2:1 (SM2), 3:2:1 (SM3), 9:0:0 (SM4), 0:9:0 (SM5), 0:0:6, 9:9:0, 9:0:6, 0:9:6, respectively] and ethanol extract (9:9:6) could improve the paralytic behavior and the pathological characteristics of chemotaxis defects in the transgenic Caenorhabditis by reducing the accumulation of Aβ and inhibiting the expression of the homologous genes hsp16-2, hsp16-41, ace-1, ace-2, TNFA1P1. Based on these results, SM powder has great potential as a treatment for AD. According to Yoshioka et al. (2016), uncarinic acid C from Uncaria rhynchophylla (Miq.) Miq. ex Havil. (Rubiaceae) is a specific inhibitor of the nucleation stage of Aβ42 aggregation. Structure–activity studies revealed that c-27 ferulic acid and c-28 carboxylic acid groups inhibit the activity of related enzymes. Other researchers (Li et al., 2016a) intraperitoneally injected postnatal mice with 25–100 mg/kg tanshinone IIA (TIIA) extracted from the root of Salvia miltiorrhiza Bunge (Lamiaceae) for 30 consecutive days and found that TIIA could reduce the accumulation of Aβ1-42 and CTFs in the hippocampus of the AD model mice. Moreover, the memory impairment in AD mice can be alleviated after treatment with TIIA at doses of 50 and 100 mg/kg.
Promotion of Aβ Degradation
In the APP/Presenilin-1 (PS1) double-transgenic AD model mice, Li et al. (2016b) found that Onjisaponin B, one of active constituent of Polygala tenuifolia Willd. (Polygalaceae), inhibited Aβ production without directly inhibiting the activation of β-site amyloid precursor protein cleaving enzyme 1 (BACE1) and γ-secretase but promoted the degradation of APP to ameliorate Aβ pathology and behavioral defects in a mouse model. Li et al. (2015b) administered 5 or 25 mg/(kg·day) notoginsenoside R1 [NTR1, extracted from P. notoginseng (Burkill) F.H.Chen (Araliaceae)] or vehicle via oral garage to 3-month-old APP/PS1 mice for 3 months. The therapeutic effects on the two groups were compared, and the cognitive function of the NTR1 group was significantly improved. The NTR1 treatment can inhibit the accumulation of Aβ and the expression of insulin-degrading enzyme in APP/PS1 mice and n2a-app695sw cells, suggesting that NTR1 may exert its protective effects by the enhancement of Aβ production (Li et al., 2015b).
Aso et al. (2015) administered delta-9-tetrahydrocannabinol (THC)/cannabidiol (CBD), two phytocannabinoids produced by the plant Cannabis sativa L. (Cannabaceae) to APP/PS1 mice and observed a significant decrease in soluble Aβ42 peptide levels and a change in the plaque composition. Moreover, the administration of the drug at the early symptomatic stage could maintain the memory of the transgenic mice, indicating that the harmful effect of Aβ peptide can be reduced by THC/CBD.
Researchers divided AD model rats into normal control, untreated, piracetam, and Naoerkang (NEK) groups to systematically study the therapeutic effect of NEK on AD model rats. NEK is composed of P. ginseng C.A.Mey. (Araliaceae), Conioselinum anthriscoides (H.Boissieu) Pimenov and Kljuykov (Apiaceae) (rhizome), Rehmannia glutinosa (Gaertn.) DC. (Orobanchaceae) (rhizome), Alpinia oxyphylla Miq. (Zingiberaceae), Citrus × aurantium L. (Rutaceae) (fructus), borneol and other substances. The rats in the NEK groups were treated with a high [60 g/(kg·day)], medium [30 g/(kg·day)] or low dose [15 g/(kg·day)] of NEK. Piracetam (0.375 g/kg, intragastrically) was consecutively administered to the piracetam group. The normal control and untreated groups were treated with the same dose of normal saline. Twenty-eight days after the administration of the different treatments, the learning and memory abilities of AD model rats in the piracetam and NEK groups were significantly improved, the levels of Aβ1-42 in the hippocampus decreased, and matrix metalloproteinase-9 (MMP-9) levels increased compared with the untreated group. Thus, NEK may exert its anti-dementia effect by increasing the expression of MMP-9 and reducing Aβ1-42 production (Li et al., 2011b).
Inhibition of Aβ Neurotoxicity
Some scholars who support Aβ as the driving factor of AD pathogenesis insist that the gradual deposition of Aβ in the cerebral cortex and hippocampal neurons causes a series of cross-cascade reactions, such as glial cell inflammation, oxidative stress, disruption of calcium homeostasis, synaptic dysfunction and loss of a large number of neurons. These changes would ultimately lead to brain atrophy and the destruction of neural structure and function. Recently, a study showed that soluble Aβ1-42 oligomers induced hyperactivity and perturbed the firing patterns of hippocampal neurons. In follow-up studies, Shao et al. (2015) found that treatment with rhynchophylline(RIN) effectively inhibited the soluble Aβ1-42 oligomer-induced increase in spontaneous discharges in a concentration-dependent manner (IC50=9.0 µM). RIN is an active tetracyclic oxindole alkaloid isolated from U. rhynchophylla (Miq.) Miq. ex Havil. (Rubiaceae). It also counteracted the harmful effects of Aβ1-42 on the neural circuits.
Microglia are the main immunocompetent phagocytes in AD and play an important role in regulating neuroinflammatory plaques and neurofibrillary tangles. The overactivation of microglia may produce direct toxic effects on neurons (Huayan et al., 2014). In the AD rat model induced by Aβ, Park et al. (2012) found that INM-176 [ethanolic extract of Angelica gigas Nakai (Apiaceae)] could decrease the activation of astrocytes and the damage of cholinergic neurons in the hippocampal CA3 region and basal ganglia of Meynert that ameliorated memory impairments in model mice. Aβ causes the excessive production of highly reactive molecules, such as reactive oxygen species (ROS), through multiple pathways, which overwhelms the antioxidant capacity and leads to oxidative stress in the brain. Liu et al. (2015) established a rapid in vivo screening system using Drosophila AD models. Among the 23 medicinal plants tested, the extracts from five plants, Coriandrum sativum L. (Apiaceae), Nardostachys jatamansi (D.Don) DC. (Caprifoliaceae), Reynoutria multiflora (Thunb.) Moldenke (Polygonaceae), R. glutinosa (Gaertn.) DC. (Orobanchaceae) and Sorbus commixta Hedl. (Rosaceae) exerted protective effects on Aβ42 neurotoxicity. R. multiflora (Thunb.) Moldenke (Polygonaceae) or S. commixta Hedl. (Rosaceae) extracts were fed to flies expressing Aβ42 with AD-related neurological phenotypes (e.g., decreased survival and motility and increased cell death and reactive oxygen species levels), and the results showed strong neural inhibition. Furthermore, the main component of R. multiflora (Thunb.) Moldenke (Polygonaceae), 2,3,5,4-toluylene-2-O-β-D-glucoside, exerts a similar protective effect on human cells exposed to Aβ42 cytotoxicity. The accumulation of Aβ on the mitochondrial membrane will destroy the mitochondrial membrane potential, which disrupts the electron transport chain and oxidative phosphorylation. Then, excessive ROS are produced in neurons, causing oxidative stress and damaging the normal structure and function of the mitochondria. Finally, the energy metabolism becomes abnormal. Meanwhile, the activation of mitochondrial apoptosis-related proteins can initiate the mitochondrial apoptosis pathway in neurons (Yang and Liu, 2012). Park and Oh (2012) pretreated neurons with Houttuynia cordata Thunb. (Saururaceae) water extracts and then exposed them to Aβ25-35. The water extracts of H. cordata Thunb. (Saururaceae) could inhibit the Aβ(25-35)-induced increase in the intracellular Ca2+ level, overproduction of ROS, disruption of the mitochondrial membrane potential, and activation of caspase 3, which blocked Aβ(25-35)-induced toxicity. Yang et al. (2012) were the first to show the concomitant beneficial effects of huperzine A [a novel alkaloid isolated from Huperzia serrata (Thunb.) Trevis. (Lycopodiaceae)] on mitochondrial dysfunction and memory deficits in AβPP/PS1 double transgenic mice at a time point that acetylcholinesterase (AChE) was not inhibited, suggesting that these effects were independent of its recognized inhibition of AChE. Moreover, using mitochondria isolated from the cortex, huperzine A could reduce ATP production induced by oligomeric Aβ1-42 and decreased mitochondrial swelling and the enzymatic activities of respiratory chain complexes, particularly complexes II–III and IV, which may be attributed to the prevention of oligomeric Aβ1-42 from entering the mitochondria. According to Huang et al. (2016), a peroral treatment with 200 and 400 mg/kg ethyl acetate extract from Diospyros kaki L.f. (Ebenaceae) can reduce the apoptosis of hippocampal neurons in rat AD models induced by Aβ, while reducing the levels of p-JNK, caspase3 and the ratio of Bax to Bcl-2. D. kaki L.f. (Ebenaceae) extract exerts a strong protective effect on the Aβ-induced cognitive dysfunction in rats and this effect is related to the regulation of the antioxidant defense system and mitochondrial apoptosis pathway.
Treatments Targeting the Tau Protein
Inhibition of Tau Protein Hyperphosphorylation
Currently, the hyperphosphorylation of tau protein is believed to play a key role in promoting neurodegeneration in patients with AD by decreasing the biological activity of the tau protein and leading to the loss of microtubule stability. Recently, the administration of some natural drugs to AD models has been shown to inhibit the hyperphosphorylation of the tau protein by regulating the activity of cyclin-dependent kinase-5 (Gao et al., 2014; Ma et al., 2015), glycogen synthase kinase-3 (Li et al., 2011a) or serine/threonine protein phosphatase-2a (Karakani et al., 2015) to improve the symptoms of AD model rats.
Cdk5 Inhibitors
Feng et al. (2017) treated rat models of AD induced by Aβ1-40 and Amanita phalloides with Tongmai Yizhi Decoction (TYD) and huperzine A. TYD included six raw materials: A. oxyphylla Miq. (Zingiberaceae) (fructus, stir-heated), R. multiflora (Thunb.) Moldenke (Polygonaceae) (root, steamed with soya-bean milk), C. anthriscoides (H. Boissieu) Pimenov and Kljuykov (Apiaceae) (root and rhizome), Borneolum syntheticum (crystallization), P. tenuifolia Willd. (Polygalaceae) (root) and Whitmania pigra Whitman (Haemopidae) (entire body), all of them were mixed by 75:50:50:50:15:1. The result showed the escape latency of the model rats was significantly decreased. Compared with the model group, the pathological changes mediated by Aβ1-40 in the hippocampus and ibotenic acid (IBO) were reversed in the treatment group. HE staining revealed significantly decreased Cdk5 and Cdk5 expression in the hippocampus of model rats. Based on these results, the treatment could inhibit Cdk5 expression and reduce protein phosphorylation (Table 1). Ma et al. (2015) found that safflower yellow (SY) from Carthamus tinctorius L. (Asteraceae) could inhibit the activation of the GSK-3 and GSK-5 signaling pathways in AD model rats, thereby inhibiting the morphological changes in neurons and the hyperphosphorylation of the tau protein caused by Aβ1-42 and significantly reducing the learning and memory impairment mediated by Aβ1-42 in the hippocampus of model rats.
GSK3β Inhibitors
A streptozotocin (STZ) injection is known to increase the activity of GSK-3β and cause the hyperphosphorylation of the tau protein. Gao et al. (2014) administered geniposide [50 M, 10 L, isolated from the fruit of G. jasminoides J.Ellis (Rubiaceae)] through oral gavage or unilateral injections into the ventricle of AD model mice treated with STZ and found that geniposide could reduce the hyperactivity of GSK3β induced by STZ by western blotting analysis. Meanwhile, geniposide improved the spatial learning of rats. According to this new evidence, the active monomer of geniposide can be used as a new therapeutic agent for AD (Gao et al., 2014).
PP-2a Activator
Li et al. (2011a) microinjected okadaic acid (OA) (protein phosphatase inhibitor) into both ventricles of adult male SD rats or added it to the cortical neuron culture medium to stimulate the hyperphosphorylation of tau in vivo or in vitro. After 7 days of pretreatment with 10 mg/(kg·day) ginsenoside Rd from Panax ginseng C.A.Mey. (Araliaceae) in SD rats or cultured cortical neurons (2.5 or 5 mol/L, 12 h), the activity of PP-2A was increased and the neurotoxicity induced by OA and the hyperphosphorylation of tau were decreased.
Inhibition of Tau Protein Aggregation
Several synthetic drugs that are currently used to inhibit tau aggregation have moderate beneficial effects, but induce numerous side effects. Therefore, the development of natural drugs with anti-tau aggregation properties is anticipated. The tau protein is stable in fibrils and exhibits a low aggregation tendency in the presence of crocin. Based on transmission electron microscopy, crocin from Crocus sativus L. (Iridaceae) could interfere with the nucleation of the tau protein and inhibits the formation of tau protein filaments (Karakani et al., 2015).
Gong et al. (2014) used insulin as an amyloid protein model to test four simple quinones (1,4-benzoquinone, 1,4-naphthoquinone, 9,10-anthraquinone and 9,10-phenanthraquinone) from medicinal plants or food and four Anthraquinone derivatives (chrysophanol, emodin, aloe-emodin, and rhein) from Rheum rhabarbarum L. (Polygonaceae). After treatment with these quinones, the number of amyloid-mimicking insulin fibers decreased, and the level of hemolysis decreased. Thus, quinones can inhibit the formation of toxic insulin oligomers, and its derivatives may play a potential role in preventing protein misfolding diseases.
The extracts of Glycyrrhiza inflata Batalin (Fabaceae) and P. ginseng C.A. Mey. (Araliaceae) also have the potential to improve the growth of mutant tau protein repeat domain (K280 tauRD DsReSH-SY5Y) and axons in neuronal cell model. G. inflata Batalin (Fabaceae) can further upregulate Ern2 and increase the unfolded protein response by downregulating Serp1 in chaperone Dnajc3 and K280 tauRDDs Red 293 cells (Chang et al., 2016).
In vitro, α-cyperone from rhizomes of Cyperus rotundus L. (Cyperaceae) exerts a significant effect on the tubulin structure by reducing the rate of polymerization and concentration of polymerized tubulin. The interaction between α-cyperone and tubulin can reduce the aggregation of unstable tubulin, which may inhibit inflammation and represent a beneficial treatment for AD (Azimi et al., 2016).
Relevant Therapy Based on Oxidative Stress Inhibition
Promotion of Free Radical Scavenging
Superoxide dismutase (SOD) and glutathione peroxidase (GSH-Px) are important enzymes that scavenge free radicals in cells. The free radical scavenging capacity is increased by increasing the activities of these enzyme. Malondialdehyde (MDA) is one of the products of lipid oxidation. Lipid oxidation can cause the cross-linking polymerization of proteins, nucleic acids and other macromolecules, and thus exerts cytotoxic effects. Therefore, the reduction of intracellular lipid oxidation and MDA production can play an antioxidant role.
The oral administration of an ethyl acetate extract of D. kaki L.f. (Ebenaceae) (Huang et al., 2016), oral administration of Bushen-Yizhi formula (BSYZ) (Hou et al., 2014), intragastric administration of SY (Ma et al., 2015) and inhalation of volatile oil of C. sativum L. (Apiaceae) (Cioanca et al., 2013) could increase SOD and GSH-Px activity and decrease MDA levels in brain tissues from AD model rats, thereby reducing oxidative stress in the brain. At the same time, the memory and cognitive impairments of model rats are significantly improved. In addition, the pathology showed that SY could inhibit the hyperphosphorylation of the tau protein and morphological changes in hippocampal neurons (Ma et al., 2015). BSYZ consisted of six medicinal plants, fruit of Cnidium monnieri (L.) Cusson (Apiaceae), rhizome of P. ginseng C.A. Mey. (Araliaceae), preparata of R. multiflora (Thunb.) Moldenke (Polygonaceae), cortex of Paeonia × suffruticosa Andrews (Paeoniaceae), fruit of Ligustrum lucidum W.T.Aiton (Oleaceae) and Lycium barbarum L. (Solanaceae) mixed in a ratio of 3:3:2:2:2:2, which can inhibit neuronal apoptosis and exert an anti-AD effect as volatile oil of C. sativum L. (Apiaceae) (Table 2). Li et al. (2014) performed the Y-maze test, shuttle box test and Morris water maze test and found that injections of 0.01 or 0.1 mg/kg schisantherin A from S. chinensis (Turcz.) Baill. (Schisandraceae) in the lateral ventricle for 5 consecutive days could significantly reduce learning and memory impairments induced by Aβ1-42. In addition, Mao et al. (2015) injected schisandrin C [extracted from the fruits of S. chinensis (Turcz.) Baill. (Schisandraceae)] into the cerebellar ventricle for 5 consecutive days. Compared with the sham operation group, Aβ1-42 levels and neuronal damage were significantly reversed in Aβ-induced AD model rats; meanwhile, short-term and working memory impairments were also substantially improved.
Singh et al. (2015) established and compared five groups of mice, including a negative control and three groups treated with 25, 50 or 100 mg/kg vanillic acid from A. sinensis (Oliv.) Diels (Apiaceae) for 28 days. At doses of 50 and 100 mg/kg, vanillic acid significantly improved memory and decreased AChE and corticosterone TNF-α levels. In addition, 100 mg/kg vanillic acid exerted a dose-dependent effect on some parameters. Vanillic acid could improve spatial learning and memory retention by preventing oxidative stress.
In a clinical trial, 120 patients with AD were randomly divided into two groups. The control group was orally administered donepezil hydrochloride and piracetam. The observation group was administered 60 Zishenhuoxuefang's synergism treatments and compared with the control group. Zishenhuoxuefang consist of R. glutinosa (Gaertn.) DC. (Orobanchaceae) (30 g), R. multiflora (Thunb.) Moldenke (Polygonaceae) (20 g), C. anthriscoides (H.Boissieu) Pimenov and Kljuykov (Apiaceae) (15 g), L. barbarum L. (Solanaceae) (15 g), C. monnieri (L.) Cusson (Apiaceae) (15 g), P. ginseng C.A.Mey. (Araliaceae) (15 g), S. miltiorrhiza Bunge (Lamiaceae) (15 g), Aulastomum gulo (10 g), L. lucidum W.T.Aiton (Oleaceae) (10 g), Acorus calamus var. angustatus Besser (Acoraceae) (10 g), Polygala sibirica L. (Polygalaceae) (10 g), Glycyrrhiza uralensis Fisch. ex DC. (Fabaceae) (10 g). After treatment, the levels and activity of SOD and GSH-Px increased, and the level of Aβ decreased in the two groups, and the observation group recorded better scores than the control group (Shu et al., 2017).
Inhibition of Free Radicals Production
Excessive production of ROS leads to oxidative stress, which is closely related to the development of AD. Mitochondria are the main site of ROS production in cells, and are vulnerable to oxidative stress. Oxidative stress attacks the mitochondrial DNA, resulting in abnormal electron transport chains and energy production barriers (Ajith and Padmajanair, 2015). Currently, mitochondrial dysfunction is acknowledged to be associated with neurodegenerative diseases, and mitochondrial defects are even regarded as the core factor of AD progression (Cardoso et al., 2017). Serious deficiencies in energy metabolism are observed during the early stage of AD, which may be due to the decrease in activity of complexes in the electron transport chain (ETC) and damage to the mitochondrial DNA induced by Aβ and ROS. The antioxidant coenzyme Q10, α-lipoic acid, selenium, ω-3 fatty acid and vitamin E improve mitochondrial function and reduce oxidative stress. These compounds are accepted as adjuvant drugs for mild to moderate dementia (Ajith and Padmajanair, 2015). Sanghuangporus sanghuang (Sheng H. Wu, T. Hatt. & Y.C. Dai) Sheng H. Wu, L.W. Zhou & Y.C. Dai, 2015 (Sanghuangporus) reduces ROS formation in HepG2 (liver hepatocellular carcinoma) cells. Based on a recent validation study, S. sanghuang (Sheng H. Wu, T. Hatt. & Y.C. Dai) Sheng H. Wu, L.W. Zhou & Y.C. Dai, 2015 (Sanghuangporus) significantly reduced the ROS production induced by tacrine, the damage to the ΔΨm (membrane potential), the damage to the mitochondrial DNA caused by 8-hydroxydeoxyguanosine (8-OHdG) formation, and the toxicity in HepG2 cells to prevent mitochondrial damage (Gao et al., 2013a). As the number of scientific investigations increases, “mitochondrial medicine” has been proposed and has become a new therapeutic strategy to maintain energy production and inhibit neuronal apoptosis.
Regulation of Oxidative Stress Pathway
Heme oxygenase-1 (HO-1) plays a key role in the pathogenesis of neuronal diseases and oxidative stress. According to Lee et al. (2015), the natural compound acerogenin A from Parthenocissus tricuspidata (Siebold and Zucc.) Planch. (Vitaceae) could effectively prevent glutamate-induced oxidative damage in HT22 cells by regulating HO-1 expression through the phosphoinositide 3-kinase/protein kinase B (PI3K/Akt) and nuclear factor-E2-related factor (Nrf2) pathway; this compound exerted a neuroprotective effect.
5,3′-Dihydroxy-3,7,4-trimethoxyflavone (DTMF) from Siegesbeckia pubescens (Makino) Makino (Compositae) can reduce the LPS-induced expression of inducible nitric oxide synthase (iNOS) and cyclooxygenase (COX-2) and promote HO-1 expression, HO activity, Nrf2 nuclear translocation and antioxidant response element (ARE)-luciferase activity, suggesting that it may represent an effective treatment for neurodegenerative diseases caused by oxidative stress (Lee et al., 2016). As shown in the study by Suganthy et al. (2016), quercetin alleviates nerve injury induced by oxidative stress by regulating the expression of Nrf2-dependent antioxidant response elements and inhibiting NF-κB and signal transducer and activator of transcription-1 (STAT-1) signaling to alleviate neuroinflammation by regulating a large number of kinase signaling cascades, such as tyrosine kinase C and protein kinase C (PKC), nerve repair can be enhanced and neuronal survival can be prolonged. Quercetin is widely distributed in plants such as Allium cepa L. (Amaryllidaceae), Asparagus officinalis L. (Asparagaceae), and Lactuca sativa L. (Asteraceae) etc. Zhang et al. (2016a) measured SOD activity, catalase (CAT) activity, MDA activity and ROS levels to assess the oxidative stress level in the brains of Tg2576 mice. The glucoside gastrodin (Gas) from Gastrodia elata Blume (Orchidaceae) could reduce oxidative stress in the hippocampus of Tg2576 transgenic mice and improves learning and memory. In addition, Gas decreases β-secretase expression by inhibiting the activity of protein kinase R/eukaryotic initiation factor-2α (PKR/eIF2α) in SH-SY5Y cells treated with hydrogen oxide.
Relevant Therapies for Inhibiting Neuroinflammatory Factors
Regulation of Inflammatory Cytokines
Many inflammatory factors are involved in the pathogenesis of AD. Tumor necrosis factor (TNF-α), interleukin-1β (IL-1β) and interleukin-6 (IL-6) play prominent roles. Increased TNF-α levels in the cerebrospinal fluid of patients with AD can lead to synaptic dysfunction and amyloid production, resulting in memory impairment.
The expression of TNF-α, IL-1β and IL-6 in the hippocampus of a mouse model of AD induced by Aβ were decrease after treatment with TianDiJingWan (TDJW) (Li et al., 2015c), Compound Danshen Tablets (CDT) (Teng et al., 2014) and the combination of ginkgo flavonoids from Ginkgo biloba L. (Ginkgoaceae) and polysaccharides from Polystictus versicolor (L.) Fr. (Polyporaceae) (Fang et al., 2015) (Table 3). Also, the memory impairment was improved. TDJW is consists of six kinds of TCM including Pheretima aspergillum E. (Geosaurus) (3 g), W. pigra Whitman (Haemopidae) (1 g), rhizome of A. calamus var. angustatus Besser (Acoraceae) (6 g), tuber of G. elata Blume (Orchidaceae) (10 g), rhizome of Polygonatum sibiricum Redouté (Asparagaceae) (10 g), and fruit of L. lucidum W.T.Aiton (Oleaceae) (10 g), which decreased Aβ and p-tau levels and increased acetylcholine and glutamic acid levels in the CA1 region of hippocampus in AD mice. CDT is consisted of S. miltiorrhiza Bunge (Lamiaceae), P. notoginseng (Burkill) F.H.Chen (Araliaceae), borneol in proportions of 450:141:8, which increased the level of brain-derived neurotrophic factor (BDNF), while the combination of ginkgo flavonoids and polysaccharides could increase SOD levels and, subsequently, decrease the intracerebral oxidation level. The ayurvedic plant Bacopa monnieri (L.) Wettst. (Plantaginaceae) can inhibit not only the production of the inflammatory cytokine TNF-α but also the release of IL-6 and the activation of cerebral inflammation-associated enzymes (caspase-3 or -5) in cultured microglia in vitro to exert an anti-inflammatory effect on the central nervous system (Nemetchek et al., 2017). In a clinical trial, Li et al. (2015a) divided participants with moderate AD into three groups, a group treated with Cistanche deserticola Ma (Orobanchaceae) (n=10), a group treated with donepezil hydrochloride tablets (n=8), and the untreated control group (n=6), to evaluate the neuroprotective effect on patients with AD. After 48 weeks of treatment, decreases in T-tau, TNF-α and IL-1β levels were observed in the Cistanche deserticola Ma (Orobanchaceae) group compared with the control group, indicating its neuroprotective potential.
Regulation of NF-κB Signaling Pathway
NF-κB is a nuclear transcription factor that was originally identified as binding to κB binding sites in the promoter region of the κ light chain of immunoglobin, which is present in most plasma samples. The NF-κB signal transduction pathway is activated by stimulation after injury, and the level of NF-κB is increased in many kinds of cells to mediate the inflammatory cell response and increase the expression of proinflammatory factors in the injured area, further exacerbating cerebral neuron damage (Gao et al., 2016).
Akebia saponin D (ASD) is a bioactive triterpenoid saponin extracted from Dipsacus inermis Wall. (Caprifoliaceae). After the intragastric administration of ASD to AD model mice for 4 weeks, Yu et al. (2012) observed the inhibition of both the secretion of protein kinase B (PKB) and the inhibitor of nuclear factor kappa-B kinase (IKK) in the brains of AD model mice. Meanwhile, the activation of NF-κB induced by Aβ1-42 was decreased, the activation of glia and the expression of TNF-α, IL-1β and COX-2 were decreased, whereas the patients' cognitive impairments improved.
Wang et al. (2014) administered Qifu-Yin (8.6–4.3 g/kg dayling for 30 days) to the mouse model of advanced glycation end product (AGE) induced AD, Donepezil (2 mg/kg) was administered as a positive control, and AGE + anti-advanced glycation end product receptor (anti-RAGE) were administered as an additional positive control group. Qifu-Yin is composed of seven raw materials, P. ginseng C.A.Mey. (Araliaceae), R. glutinosa (Gaertn.) DC. (Orobanchaceae), A. sinensis (Oliv.) Diels (Apiaceae), Atractylodes macrocephala Koidz. (Asteraceae), Ziziphus jujuba Mill. (Rhamnaceae), P. tenuifolia Willd. (Polygalaceae) and G. inflata Batalin (Fabaceae) mixed by 6:9:9:5:6:5:3, which could significantly ameliorate the AGE-induced memory impairment in a dose-dependent manner. The production of RAGE and NF-κB as well as the level of the Aβ protein in the hippocampus were substantially reduced, and TNF-α and IL-1β levels were decreased, indicating that Qifu-Yin may exert its neuroprotective effect through both the RAGE/NF-κB pathway and its anti-inflammatory activity (Wang et al., 2014). In addition, the activity of quercetin in scavenging free radicals has been confirmed. Quercetin belong to flavonol-type flavonoid, which are widely present in the diet and play a wide range of roles. By regulating the expression of NRF-2-dependent antioxidant elements, quercetin can protect neurons from oxidative stress. Neuroinflammation is also alleviated by inhibiting NF-κB signal transduction and STAT-1 (Chen et al., 2005).
Treatments Targeting Cholinergic Neurons and Acetylcholine
Cholinergic neurons are widely distributed in the central nervous system. Cholinergic neuron injury plays an important role in AD. Changes in the levels of cholinergic markers in the basal forebrain correlate the severity of AD-related dementia in pathology studies. The activities of AChE and choline acetyltransferase (ChAT) are decreased in the brains of patients with AD, and a noticeable deficiency in ACh is observed. As a result of the decrease in cholinergic activity, ACh and the injury of various types of cholinergic receptors, multiple clinical manifestations may develop, predominantly memory and cognitive impairments (Mahlke et al., 2012; Asaduzzaman et al., 2014).
AChEIs
AChE is the most effective therapeutic target for the treatment of AD (Singh et al., 2013). AChEIs are the most widely used drugs to treat the symptoms of patients with mild to severe Alzheimer's disease (Geromichalos et al., 2012; Babiloni et al., 2013). Cessation may have a negative effect on cognitive and neuropsychiatric symptoms in patients who have taken AChEIs (O'Regan et al., 2015). Based on accumulating evidence, central CACE-IS targeting the blood-brain barrier are associated with a decrease in the rate of cognitive decline (Gao et al., 2013b). The available AChEIs are only effective in 20–30% of patients with AD, and at least two of the four AChEIs currently used are in fact based on ethnopharmacological research. So the development of new anti-AChE drugs from medicinal plants has attracted increasing attention (Russo et al., 2013).
Some scholars (Kaufmann et al., 2016) prepared natural water-containing crude extracts from 80 traditional Chinese medicinal plants and tested their anti-AChE activity in vitro using Hermann's colorimetric method. Berberis bealei Fortune (Berberidaceae), Coptis chinensis Franch. (Ranunculaceae) and Phellodendron chinensis C.K.Schneid. (Rutaceae) contained large amounts of isoquinoline alkaloids, which could effectively inhibit AChE activity. Combinations of individual alkaloids, such as berberine, coptisine and palmatine, synergistically enhance the inhibitory effect on ACh. Based on these results, some Chinese herbs exert synergistic inhibitory effects on AChE through their secondary metabolites.
Ohba et al. (2015) repeatedly administered a H. serrata (Thunb.) Trevis. (Lycopodiaceae) extract to AD model mice. This extract inhibits AChE activity while ameliorating the cognitive impairment of the mice with AD, suggesting broad application prospects of this extract as a treatment for AD symptoms. A team of researchers subsequently isolated highly effective components from H. serrata (Thunb.) Trevis. (Lycopodiaceae) and related plants and synthesized complete lycopodium alkaloids with a special skeleton from a genetic and biological perspective (Yang et al., 2016) (Table 4).
C. sativus L. (Iridaceae) extracts showed moderate AChE inhibitory activity (30%). The IC50 values of crocetin, dimethylcrocetin and safranal were 96.33 µM, 107.1 µM and 21.09 µM, respectively. Safranal only acts at the binding sites of AChE, whereas crocin and dimethyl lycopene simultaneously target binding and peripheral anion sites in the catalytic cleft. The development of new therapeutic agents based on carotenoid dual binding inhibitors will have great value (Geromichalos et al., 2012).
Promotion of ChAT Expression
ChAT is the main rate-limiting enzyme involved in ACh synthesis. ChAT levels are decreased in the brains of patients with AD in a manner proportional to the content of Aβ. Huang et al. (2013) intragastrically administered 500 or 1,000 mg/kg G. elata Blume (Orchidaceae) to a rat model of AD induced by Aβ25-35 daily for 52 days. The AD model rats that received G. elata Blume (Orchidaceae) exhibited significantly improved spatial memory in the Morris water maze test. Congo red staining showed a substantial decrease in the amount of amyloid deposited in the hippocampus, and ChAT expression was substantially increased in medial septum and hippocampus, as evidenced by western blot analysis. The long-term administration of G. elata Blume (Orchidaceae) has therapeutic potential for AD.
In a study of the mouse model of AD induced by Aβ25-35 performed by Teng et al. (2014), CDT could increase the expression of ChAT in the brain, induce BDNF production and activate the PKC receptor. The spatial memory of model mice was substantially improved in the Morris water maze test.
Protection of Cholinergic Neurons
The memory and cognitive impairments in patients with AD are closely related to the loss of cholinergic neurons. Nerve growth factor (NGF) can promote the regeneration of cholinergic neurons in the basal forebrain, and thus targeted transport of NGF has become a potential treatment for AD (Eyjolfsdottir et al., 2016). A variety of traditional Chinese medicine extracts exert a good therapeutic effect on AD, some of which affect the expression of NGF, BDNF and their related receptors (Hou et al., 2015).
After administering the BSYZ to the IBO-induced AD model rats, immunohistochemical staining of the brain revealed an increasing trend in the expression of NGF, TrkA and p75 in hippocampus and cortex, while ChAT and NGF expression were substantially increased. The BSYZ may improve the memory impairment of the IBO-induced AD rats by regulating NGF signal transduction and the anti-apoptotic cholinergic pathway (Hou et al., 2015). According to Moon et al. (2014), 6-shogaol, a bioactive components of Zingiber officinale Roscoe (Zingiberaceae), could increase the levels of NGF and pre-/post-synaptic markers in the hippocampus, and improves the memory impairment induced by Aβ and scopolamine.
Li et al. (2016c) treated the animal model of Aβ25-35-induced AD with Xanthoceras sorbifolium Bunge (Sapindaceae) extracts. The treatment upregulated postsynaptic density protein 95 (PSD95), the main scaffold protein in hippocampal CA1 pyramidal neurons, ameliorated the density of dendritic spines, increased the level of BDNF and decrease the expression of RhoA and its downstream target protein rho associated coiled-coil containing protein kinase 2 (ROCK2). Thus, the X. sorbifolium Bunge (Sapindaceae) extracts can protect dendritic spines through the BDNF signal transduction pathway and improve cognition. As shown in the study by Li et al. (2016a), TIIA could reduce the accumulation of the 1–42 CTF of the β-amyloid protein in an AD model, promote depolarization-induced BDNF synthesis without affecting total BDNF levels, and relieving the memory impairment in the AD mice, which further confirmed the protective effect of BDNF on cholinergic neurons. Moreover, Park HR et al. (Park et al., 2016) orally administered 100 or 500 mg/(kg·day) Polygonum multiflorum Thunberg complex composition-12 (PMC-12) to 5-week-old male C57BL/6 mice. PMC-12 is a mixture of four medicinal herbs, R. multiflora (Thunb.) Moldenke (Polygonaceae) (25.5 kg), P. tenuifolia Willd. (Polygalaceae) (7.5 kg), R. glutinosa (Gaertn.) DC. (Orobanchaceae) (9.5 kg), and Acorus gramineus Aiton (Acoraceae) (7.5 kg). After 2 weeks, the level of BDNF and number of synapses increased in the hippocampus of model mice, the neural precursor cells of dentate gyrus nerves proliferated and the number of surviving newborn cells increased. Thus, PMC-12 can promote hippocampal neurogenesis and improve neurocognitive function.
Summary and Prospects
AD is a complex, inherited, slowly developing and irreversible neurological disease. During decades of asymptomatic progression, multiple interacting systems and molecular mechanisms contribute to the development of the early preclinical stage with situational memory impairment, as well as decline of memory and loss of cognitive function in the dementia phase. At present, it is generally believed that Aβ and Tau proteins are the main causes of AD. However, a large number of studies indicate that oxidative stress, neuroinflammatory factors, cholinergic neurons, and acetylcholine injury are also play important roles in AD. Symptomatic treatment for AD patients is the future trend of treatment.
Modern pharmacology and drug development usually follow a single objective principle, which may have led to the failure of most anti-AD compounds in clinical trials. The advantages of natural drugs are mild curative effect and few side effects, which have become a hot spot for AD treatment. As described above, according to the five pathogenesis hypotheses of AD, many effective natural products have been identified to be able to alleviate the symptoms of AD according to different targets and mechanisms. Although some drugs have entered clinical trials, most are still in the subclinical trial phase, and further evidences are needed to provide the efficacy of these drugs in clinically relevant animal models of AD to find appropriate doses and toxicity etc. Similarly, the pharmaceutical manufacturing industry, including the identification of suitable methods for extracting active ingredients, is equally crucial. In addition, it is still unclear whether they can exhibit or limit drug resistance due to their multi-target properties. In summary, the therapeutic advantages of natural medicines for AD have surpassed existing single-targeted drugs. In order to complete the laboratory-to-clinical conversion, related pharmacology, toxicology, extraction process, and combined use with other drugs need further research and confirmation.
Author Contributions
YM, Ma-wY, X-wL, J-wY, J-zC, and Me-wY wrote the paper. XH, L-lZ contributed to paper revision. F-fH and S-lY are responsible for writing promotion in grammars and languages, the idea, and fund.
Conflict of Interest
The authors declare that the research was conducted in the absence of any commercial or financial relationships that could be construed as a potential conflict of interest.
Acknowledgments
This work was supported by National Natural Science Foundation of China (No. 81660751, 81660151, and 81260504), Key Research and Development Program of Jiangxi Province of China (№ 20161BBG70067), and Jiangxi Provincial Natural Science Foundation of China (No 20171BAB205085).
References
Ajith, T. A., Padmajanair, G. (2015). Mitochondrial pharmaceutics: a new therapeutic strategy to ameliorate oxidative stress in Alzheimer's Disease. Curr. Aging Sci. 8 (3), 235–240. doi: 10.2174/187460980803151027115147
Asaduzzaman, M., Uddin, M. J., Kader, M. A., Alam, A. H., Rahman, A. A., Rashid, M. (2014). In vitro acetylcholinesterase inhibitory activity and the antioxidant properties of Aegle marmelos leaf extract: implications for the treatment of Alzheimer's disease. Psychogeriatrics 14 (1), 1–10. doi: 10.1111/psyg.12031
Aso, E., Sánchez-Pla, A., Vegas-Lozano, E., Maldonado, R., Ferrer, I. (2015). Cannabis-based medicine reduces multiple pathological processes in AβPP/PS1 mice. J. Alzheimers Dis. 43 (3), 977–991. doi: 10.3233/JAD-141014
Azimi, A., Ghaffari, S. M., Riazi, G. H., Arab, S. S., Tavakol, M. M., Pooyan, S. (2016). alpha-Cyperone of Cyperus rotundus is an effective candidate for reduction of inflammation by destabilization of microtubule fibers in brain. J. Ethnopharmacol. 194, 219–227. doi: 10.1016/j.jep.2016.06.058
Babiloni, C., Del Percio, C., Bordet, R., Bourriez, J. L., Bentivoglio, M., Payoux, P. (2013). Effects of acetylcholinesterase inhibitors and memantine on resting-state electroencephalographic rhythms in Alzheimer's disease patients. Clin. Neurophysiol. 124 (5), 837–850. doi: 10.1016/j.clinph.2012.09.017
Ballard, C., Gauthier, S., Corbett, A., Brayne, C., Aarsland, D., Jones, E. (2011). Alzheimer's disease. Lancet 377 (9770), 1019–1031. doi: 10.1016/s0140-6736(10)61349-9
Birks, J. (2006). Cholinesterase inhibitors for Alzheimer's disease. Cochrane Database Syst. Rev. (1), Cd005593. doi: 10.1002/14651858.Cd005593
Cardoso, S., Seica, R. M., Moreira, P. I. (2017). Mitochondria as a target for neuroprotection: implications for Alzheimer s disease. Expert Rev. Neurother. 17 (1), 77–91. doi: 10.1080/14737175.2016.1205488
Chang, K. H., Chen, I. C., Lin, H. Y., Chen, H. C., Lin, C. H., Lin, T. H. (2016). The aqueous extract of Glycyrrhiza inflata can upregulate unfolded protein response-mediated chaperones to reduce tau misfolding in cell models of Alzheimer's disease. Drug Des. Devel Ther. 10, 885–896. doi: 10.2147/dddt.S96454
Chen, J. C., Ho, F. M., Pei-Dawn Lee, C., Chen, C. P., Jeng, K. C., Hsu, H. B. (2005). Inhibition of iNOS gene expression by quercetin is mediated by the inhibition of IkappaB kinase, nuclear factor-kappa B and STAT1, and depends on heme oxygenase-1 induction in mouse BV-2 microglia. Eur. J. Pharmacol. 521 (1-3), 9–20. doi: 10.1016/j.ejphar.2005.08.005
Cheung, T. S., Song, T. H., Ng, T. B., Wu, F. H., Lao, L. X., Tang, S. C. (2015). Therapeutic effects of herbal chemicals in traditional chinese medicine on alzheimer's disease. Curr. Medicinal. Chem. 22 (19), 2392–2403. doi: 10.2174/0929867322666150520095509
Chua, K. K., Wong, A., Kwan, W. L., Song, J. X., Chen, L. L., Chan, L. T. (2015). The efficacy and safety of the Chinese herbal medicine Di-Tan decoction for treating Alzheimer's disease: protocol for a randomized controlled trial. Trials 16, 1, 199. doi: 10.1186/s13063-015-0716-z
Cioanca, O., Hritcu, L., Mihasan, M., Hancianu, M. (2013). Cognitive-enhancing and antioxidant activities of inhaled coriander volatile oil in amyloid beta(1-42) rat model of Alzheimer's disease. Physiol. Behav. 120, 193–202. doi: 10.1016/j.physbeh.2013.08.006
Duan, M. H., Wang, L. N., Jiang, Y. H., Pei, Y. Y., Guan, D. D., Qiu, Z. D. (2016). Angelica sinensis reduced Aβ-induced memory impairment in rats. J. Drug Targeting 24 (4), 340–347. doi: 10.3109/1061186X.2015.1077848
Eyjolfsdottir, H., Eriksdotter, M., Linderoth, B., Lind, G., Juliusson, B., Kusk, P. (2016). Targeted delivery of nerve growth factor to the cholinergic basal forebrain of Alzheimer's disease patients: application of a second-generation encapsulated cell biodelivery device. Alzheimers Res. Ther. 8 (1), 30. doi: 10.1186/s13195-016-0195-9
Fang, X., Jiang, Y., Ji, H., Zhao, L., Xiao, W., Wang, Z. (2015). The synergistic beneficial effects of ginkgo flavonoid and coriolus versicolor polysaccharide for memory improvements in a mouse model of dementia. Evid. Based Complement. Alternat. Med. 2015, 128394. doi: 10.1155/2015/128394
Feng, J. H., Cai, B. C., Guo, W. F., Wang, M. Y., Ma, Y., Lu, Q. X. (2017). Neuroprotective effects of Tongmai Yizhi Decoction () against Alzheimer's disease through attenuating cyclin-dependent kinase-5 expression. Chin J. Integr. Med. 23 (2), 132–137. doi: 10.1007/s11655-016-2507-0
Gao, C., Zhong, L., Jiang, L., Geng, C., Yao, X., Cao, J. (2013a). Phellinus linteus mushroom protects against tacrine-induced mitochondrial impairment and oxidative stress in HepG2 cells. Phytomedicine 20 (8-9), 705–709. doi: 10.1016/j.phymed.2013.02.014
Gao, Y., O’Caoimh, R., Healy, L., Kerins, D. M., Eustace, J., Guyatt, G. (2013b). Effects of centrally acting ACE inhibitors on the rate of cognitive decline in dementia. BMJ Open 3 (7), e002881. doi: 10.1136/bmjopen-2013-002881
Gao, C., Liu, Y., Jiang, Y., Ding, J., Li, L. (2014). Geniposide ameliorates learning memory deficits, reduces tau phosphorylation and decreases apoptosis via GSK3beta pathway in streptozotocin-induced alzheimer rat model. Brain Pathol. 24 (3), 261–269. doi: 10.1111/bpa.12116
Gao, M. D., Lin, J. Q., Tong, L., Zhang, J. Y., Na, H., Liu, X. H. (2016). Research progress on the effect of curcumin on the repair of spinal cord injury by inhibiting NF- B signaling pathway. Chin. J. Pharmacol. Toxicol. 30 (3), 272–277.
Geromichalos, G. D., Lamari, F. N., Papandreou, M. A., Trafalis, D. T., Margarity, M., Papageorgiou, A. (2012). Saffron as a source of novel acetylcholinesterase inhibitors: molecular docking and in vitro enzymatic studies. J. Agric. Food Chem. 60 (24), 6131–6138. doi: 10.1021/jf300589c
Gong, H., He, Z., Peng, A., Zhang, X., Cheng, B., Sun, Y. (2014). Effects of several quinones on insulin aggregation. Sci. Rep. 4, 5648. doi: 10.1038/srep05648
He, P., Li, P., Hua, Q., Liu, Y., Staufenbiel, M., Li, R. (2013). Chronic administration of anti-stroke herbal medicine TongLuoJiuNao reduces amyloidogenic processing of amyloid precursor protein in a mouse model of Alzheimer’s Disease. PloS One 8 (3), e58181. doi: 10.1371/journal.pone.0058181
Hofrichter, J., Krohn, M., Schumacher, T., Lange, C., Feistel, B., Walbroel, B. (2016). Sideritis spp. extracts enhance memory and learning in Alzheimer's β-Amyloidosis mouse models and aged C57Bl/6 Mice. J. Alzheimers Dis. 53 (3), 967–980. doi: 10.3233/JAD-160301
Hou, Y. C., Chao, P. D., Ho, H. J., Wen, C. C., Hsiu, S. L. (2003). Profound difference in pharmacokinetics between morin and its isomer quercetin in rats. J. Pharm. Pharmacol. 55 (2), 199–203. doi: 10.1211/002235702487
Hou, X. Q., Wu, D. W., Zhang, C. X., Yan, R., Yang, C., Rong, C. P. (2014). BushenYizhi formula ameliorates cognition deficits and attenuates oxidative stressrelated neuronal apoptosis in scopolamineinduced senescence in mice. Int. J. Mol. Med. 34 (2), 429–439. doi: 10.3892/ijmm.2014.1801
Hou, X. Q., Zhang, L., Yang, C., Rong, C. P., He, W. Q., Zhang, C. X. (2015). Alleviating effects of Bushen-Yizhi formula on ibotenic acid-induced cholinergic impairments in rat. Rejuvenation Res. 18 (2), 111–127. doi: 10.1089/rej.2014.1603
Huang, Y. D., Mucke, L. (2012). Alzheimer mechanisms and therapeutic strategies. Cell 148 (6), 1204–1222. doi: 10.1016/j.cell.2012.02.040
Huang, G. B., Zhao, T., Muna, S. S., Jin, H. M., Park, J. I., Jo, K. S. (2013). Therapeutic potential of Gastrodia elata Blume for the treatment of Alzheimer's disease. Neural Regener. Res. 8 (12), 1061–1070. doi: 10.3969/j.issn.1673-5374.2013.12.001
Huang, S. W., Wang, W., Zhang, M. Y., Liu, Q. B., Luo, S. Y., Peng, Y. (2016). The effect of ethyl acetate extract from persimmon leaves on Alzheimer’s disease and its underlying mechanism. Phytomedicine 23 (7), 694–704. doi: 10.1016/j.phymed.2016.03.009
Huayan, L., Jun, W., Takeshi, T. (2014). Juzen-Taiho-to, an herbal medicine, promotes the differentiation of transplanted bone marrow cells into microglia in the mouse brain injected with fibrillar amyloid β. Tohoku J. Exp. Med. 233 (2), 113–122. doi: 10.1620/tjem.233.113
Jeong, E. J., Lee, H. K., Lee, K. Y., Byung Ju, J., Dae Hyun, K., Jin-Ho, P. (2013). The effects of lignan-riched extract of Shisandra chinensis on amyloid-β-induced cognitive impairment and neurotoxicity in the cortex and hippocampus of mouse. J. Ethnopharmacol. 146 (1), 347–354. doi: 10.1016/j.jep.2013.01.003
Karakani, A. M., Riazi, G., Mahmood Ghaffari, S., Ahmadian, S., Mokhtari, F., Jalili Firuzi, M. (2015). Inhibitory effect of corcin on aggregation of 1N/4R human tau protein in vitro. Iran. J. Basic Med. Sci. 18 (5), 485–492.
Kaufmann, D., Kaur Dogra, A., Tahrani, A., Herrmann, F., Wink, M. (2016). Extracts from traditional chinese medicinal plants inhibit Acetylcholinesterase, a Known Alzheimer's Disease Target. Molecules 21 (9), 1-16. doi: 10.3390/molecules21091161
Lee, D. S., Cha, B. Y., Woo, J. T., Kim, Y. C., Jang, J. H. (2015). Acerogenin A from Acer nikoense maxim prevents oxidative stress-induced neuronal cell death through Nrf2-Mediated Heme Oxygenase-1 expression in mouse Hippocampal HT22 Cell Line. Molecules 20 (7), 12545–12557. doi: 10.3390/molecules200712545
Lee, D. S., Lee, M., Sung, S. H., Jeong, G. S. (2016). Involvement of heme oxygenase-1 induction in the cytoprotective and neuroinflammatory activities of Siegesbeckia Pubescens isolated from 5,3'-dihydroxy-3,7,4'-trimethoxyflavone in HT22 cells and BV2 cells. Int. Immunopharmacol. 40, 65–72. doi: 10.1016/j.intimp.2016.08.030
Li, L., Liu, J., Yan, X., Qin, K., Shi, M., Lin, T. (2011a). Protective effects of ginsenoside Rd against okadaic acid-induced neurotoxicity in vivo and in vitro. J. Ethnopharmacol. 138 (1), 135–141. doi: 10.1016/j.jep.2011.08.068
Li, X., Yuan, H. F., Quan, Q. K., Jian-Jun, W., Ning-Ning, W., Ming, L. (2011b). Scavenging effect of Naoerkang on amyloid beta-peptide deposition in the hippocampus in a rat model of Alzheimer's disease. Chin. J. Integr. Med. 17 (11), 847–853. doi: 10.1007/s11655-011-0896-7
Li, X., Zhao, X., Xu, X., Mao, X., Liu, Z., Li, H. (2014). Schisantherin A recovers Abeta-induced neurodegeneration with cognitive decline in mice. Physiol. Behav. 132, 10–16. doi: 10.1016/j.physbeh.2014.04.046
Li, N., Wang, J., Ma, J., Gu, Z., Jiang, C., Yu, L. (2015a). Neuroprotective effects of cistanches herba therapy on patients with moderate Alzheimer's Disease. Evid. Based Complement. Alternat. Med. 2015, 103985. doi: 10.1155/2015/103985
Li, Z., Li, H., Zhao, C., Lv, C., Zhong, C., Xin, W. (2015b). Protective effect of notoginsenoside R1 on an APP/PS1 mouse model of Alzheimer's Disease by up-regulating insulin degrading enzyme and inhibiting Aβ accumulation. CNS Neurological Disord. - Drug Targets 14 (3), 360–369. doi: 10.2174/1871527314666150225141521
Li, Z., Tong, Q., Xu, H., Hu, L., Zhao, R., Zhou, F. (2015c). Therapeutic Effects of TianDiJingWan on the Abeta 25-35-Induced Alzheimer’s Disease Model Rats. Evid. Based Complement. Alternat. Med. 2015, 307350. doi: 10.1155/2015/307350
Li, F., Han, G., Wu, K. (2016a). Tanshinone IIA Alleviates the AD Phenotypes in APP and PS1 Transgenic Mice. J. BioMed. Res. Int. 2016 (2), 1–8. doi: 10.1155/2016/7631801
Li, X., Cui, J., Yu, Y., Li, W., Hou, Y., Wang, X. (2016b). Traditional chinese nootropic medicine radix polygalae and its active constituent onjisaponin B reduce β-Amyloid production and improve cognitive impairments. PloS One 11 (3), e0151147. doi: 10.1371/journal.pone.0151147
Li, Y., Xu, J., Xu, P., Song, S., Liu, P., Chi, T. (2016c). Xanthoceras sorbifolia extracts ameliorate dendritic spine deficiency and cognitive decline via upregulation of BDNF expression in a rat model of Alzheimer’s disease. Neurosci. Lett. 629, 208–214. doi: 10.1016/j.neulet.2016.07.011
Liu, Q. F., Lee, J. H., Kim, Y.-M., Lee, S., Hong, Y. K., Hwang, S. (2015). In Vivo Screening of Traditional Medicinal Plants for Neuroprotective Activity against Aβ42 Cytotoxicity by Using Drosophila Models of Alzheimer’s Disease. Biol. Pharmaceutical Bull. 38 (12), 1891–1901. doi: 10.1248/bpb.b15-00459
Ma, Q., Ruan, Y., Xu, H., Shi, X., Wang, Z., Hu, Y. (2015). Safflower yellow reduces lipid peroxidation, neuropathology, tau phosphorylation and ameliorates amyloid beta-induced impairment of learning and memory in rats. BioMed. Pharmacother. 76, 153–164. doi: 10.1016/j.biopha.2015.10.004
Mahlke, J. D., Boligon, A. A., Machado, M. M., Athayde, M. L. (2012). In vitro toxicity, antiplatelet and acetylcholinesterase inhibition of Buddleja thyrsoides Lam. leaves. Nat. Prod. Res. 26 (23), 2223–2226. doi: 10.1080/14786419.2011.643884
Mao, X., Liao, Z., Guo, L., Xu, X., Wu, B., Xu, M. (2015). Schisandrin C ameliorates learning and memory deficits by Abeta1-42 -induced oxidative stress and neurotoxicity in mice. Phytother. Res. 29 (9), 1373–1380. doi: 10.1002/ptr.5390
McShane, R., Areosa Sastre, A., Minakaran, N. (2006). Memantine for dementia. Cochrane Database Syst. Rev. (2), Cd003154. doi: 10.1002/14651858.CD003154.pub5
Moon, M., Kim, H. G., Choi, J. G., Hyein, O., Lee, P. K. J., Keun, H. S. (2014). 6-Shogaol, an active constituent of ginger, attenuates neuroinflammation and cognitive deficits in animal models of dementia. Biochem. Biophys. Res. Commun. 449 (1), 8–13. doi: 10.1016/j.bbrc.2014.04.121
Nemetchek, M. D., Stierle, A. A., Stierle, D. B., Lurie, D. I. (2017). The Ayurvedic plant Bacopa monnieri inhibits inflammatory pathways in the brain. J. Ethnopharmacol. 197, 92–100. doi: 10.1016/j.jep.2016.07.073
O'Regan, J., Lanctot, K., Mazereeuw, G., Herrmann, N., O'Regan, J., Lanctot, K., Mazereeuw, G., Herrmann, N. (2015). Cholinesterase Inhibitor Discontinuation in Patients With Alzheimer's Disease. J. Clin. Pschiatry 76, e1424–e1431. doi: 10.4088/JCP.14r09237
Ohba, T., Yoshino, Y., Ishisaka, M., Abe, N., Tsuruma, K., Shimazawa, M. (2015). Japanese Huperzia serrata extract and the constituent, huperzine A, ameliorate the scopolamine-induced cognitive impairment in mice. Biosci. Biotechnol. Biochem. 79 (11), 1838–1844. doi: 10.1080/09168451.2015.1052773
Park, H., Oh, M. S. (2012). Houttuyniae Herba protects rat primary cortical cells from Abeta(25-35)-induced neurotoxicity via regulation of calcium influx and mitochondria-mediated apoptosis. Hum. Exp. Toxicol. 31 (7), 698–709. doi: 10.1177/0960327111433898
Park, S. J., Jung, J. M., Lee, H. E., Young Woo, L., Hyun, K. D., Jong Min, K. (2012). The memory ameliorating effects of INM-176, an ethanolic extract of Angelica gigas, against scopolamine- or Aβ(1-42)-induced cognitive dysfunction in mice. J. Ethnopharmacol. 143 (2), 611–620. doi: 10.1016/j.jep.2012.07.019
Park, H. R., Kim, J. Y., Lee, Y., Chun, H. J., Choi, Y. W., Shin, H. K. (2016). PMC-12, a traditional herbal medicine, enhances learning memory and hippocampal neurogenesis in mice. Neurosci. Lett. 617, 254–263. doi: 10.1016/j.neulet.2016.02.036
Qiu, J., Li, W., Feng, S. H., Wang, M., He, Z. Y. (2014). Ginsenoside Rh2 promotes nonamyloidgenic cleavage of amyloid precursor protein via a cholesterol-dependent pathway. Genet. Mol. Res. 13 (2), 3586–3598. doi: 10.4238/2014
Rivera, D., Allkin, R., Obon, C., Alcaraz, F., Verpoorte, R., Heinrich, M. (2014). What is in a name? The need for accurate scientific nomenclature for plants. J. Ethnopharmacol. 152 (3), 393–402. doi: 10.1016/j.jep.2013.12.022
Russo, P., Frustaci, A., Del Bufalo, A., Fini, M., Cesario, A. (2013). From traditional European medicine to discovery of new drug candidates for the treatment of dementia and Alzheimer's disease: acetylcholinesterase inhibitors. Curr. Med. Chem. 20 (8), 976–983.
Ryu, J. C., Zimmer, E. R., Rosa-Neto, P., Yoon, S. O. (2019). Consequences of metabolic disruption in Alzheimer's disease pathology. Neurother. 16, 600–610. doi: 10.1007/s13311-019-00755-y
Shao, H., Mi, Z., Ji, W. G., Zhang, C. H., Zhang, T., Ren, S. C. (2015). Rhynchophylline protects against the amyloid β-induced increase of spontaneous discharges in the hippocampal CA1 region of rats. Neurochemical Res. 40 (11), 2365–2373. doi: 10.1007/s11064-015-1730-y
Shu, S. Y., Yang, Q., Zhang, H. X. (2017). Effects of traditional Chinese medicine on the treatment of alzheimer's disease with Zishenhuoxuefang and its effects on the indexes of oxidative stress and amyloid beta protein levels. J. integrated traditional Chin. western Med. 26 (21), 2331–2333.
Singh, M., Kaur, M., Kukreja, H., Chugh, R., Silakari, O., Singh, D. (2013). Acetylcholinesterase inhibitors as Alzheimer therapy: from nerve toxins to neuroprotection. Eur. J. Med. Chem. 70, 165–188. doi: 10.1016/j.ejmech.2013.09.050
Singh, J. C., Kakalij, R. M., Kshirsagar, R. P., Kumar, B. H., Komakula, S. S., Diwan, P. V. (2015). Cognitive effects of vanillic acid against streptozotocin-induced neurodegeneration in mice. Pharm. Biol. 53 (5), 630–636. doi: 10.3109/13880209.2014.935866
Suganthy, N., Devi, K. P., Nabavi, S. F., Braidy, N., Nabavi, S. M. (2016). Bioactive effects of quercetin in the central nervous system: Focusing on the mechanisms of actions. BioMed. Pharmacother. 84, 892–908. doi: 10.1016/j.biopha.2016.10.011
Sun, Z. K., Yang, H. Q., Chen, S. D. (2013). Traditional Chinese medicine: a promising candidate for the treatment of Alzheimer's disease. Trans. Neurodegeneration 2 (1), 6. doi: 10.1186/2047-9158-2-6
Teng, Y., Zhang, M. Q., Wang, W., Liu, L. T., Zhou, L. M., Miao, S. K. (2014). Compound danshen tablet ameliorated abeta25-35-induced spatial memory impairment in mice via rescuing imbalance between cytokines and neurotrophins. BMC Complement. Altern. Med. 14, 23. doi: 10.1186/1472-6882-14-23
Verghese, P. B., Castellano, J. M., Holtzman, D. M. (2011). Apolipoprotein E in Alzheimer’s disease and other neurological disorders. Lancet Neurol. 10 (3), 241–252. doi: 10.1016/s1474-4422(10)70325-2
Wang, S. Y., Liu, J. P., Ji, W. W., Chen, W. J., Fu, Q., Feng, L. (2014). Qifu-Yin attenuates AGEs-induced Alzheimer-like pathophysiological changes through the RAGE/NF-kappaB pathway. Chin J. Nat. Med. 12 (12), 920–928. doi: 10.1016/s1875-5364(14)60135-7
Xu, W., Tan, L., Wang, H. F., Jiang, T., Tan, M. S., Tan, L. (2015). Meta-analysis of modifiable risk factors for Alzheimer's disease. J. Neurol. Neurosurg. Psychiatry 86 (12), 1299–1306. doi: 10.1136/jnnp-2015-310548
Yang, X. M., Liu, J. Z. (2012). β-amyloid peptide damage to mitochondria and its role in Alzheimer's disease. J. Clin. Pathol. 32 (5), 437–442.
Yang, L., Ye, C. Y., Huang, X. T., Tang, X. C., Zhang, H. Y. (2012). Decreased accumulation of subcellular amyloid-beta with improved mitochondrial function mediates the neuroprotective effect of huperzine A. J. Alzheimers Dis. 31 (1), 131–142. doi: 10.3233/jad-2012-120274
Yang, Y., Wang, Z., Wu, J., Chen, Y. (2016). Chemical constituents of plants from the Genus Phlegmariurus. Chem. Biodivers. 13 (3), 269–274. doi: 10.1002/cbdv.201500043
Yoshioka, T., Murakami, K., Ido, K., Hanaki, M., Yamaguchi, K., Midorikawa, S. (2016). Semisynthesis and structure-activity studies of uncarinic acid C isolated from Uncaria rhynchophylla as a specific inhibitor of the nucleation phase in Amyloid beta42 aggregation. J. Nat. Prod. 79 (10), 2521–2529. doi: 10.1021/acs.jnatprod.6b00392
Yu, X., Wang, L. N., Du, Q. M., Ma, L., Chen, L., You, R. (2012). Akebia Saponin D attenuates amyloid beta-induced cognitive deficits and inflammatory response in rats: involvement of Akt/NF-kappaB pathway. Behav. Brain Res. 235 (2), 200–209. doi: 10.1016/j.bbr.2012.07.045
Zhang, J. S., Zhou, S. F., Wang, Q., Guo, J. N., Liang, H. M., Deng, J. B. (2016a). Gastrodin suppresses BACE1 expression under oxidative stress condition via inhibition of the PKR/eIF2alpha pathway in Alzheimer's disease. Neurosci. 325, 1–9. doi: 10.1016/j.neuroscience.2016.03.024
Zhang, W., Zhi, D., Ren, H., Wang, D., Wang, X., Zhang, Z. (2016b). Shengmai Formula Ameliorates Pathological Characteristics in AD C. elegans. Cell. Mol. Neurobiol. 36 (8), 1291–1302. doi: 10.1007/s10571-015-0326-z
Keywords: Alzheimer's disease, β-amyloid, tau protein metabolism, free radical damage, natural drug
Citation: Ma Y, Yang M-w, Li X-w, Yue J-w, Chen J-z, Yang M-w, Huang X, Zhu L-l, Hong F-f and Yang S-l (2019) Therapeutic Effects of Natural Drugs on Alzheimer’s Disease. Front. Pharmacol. 10:1355. doi: 10.3389/fphar.2019.01355
Received: 30 May 2019; Accepted: 25 October 2019;
Published: 04 December 2019.
Edited by:
Ruiwen Zhang, University of Houston, United StatesReviewed by:
Longxuan Li, Secondary Military Medical University, ChinaAlessandro Martorana, University of Rome Tor Vergata, Italy
Copyright © 2019 Ma, Yang, Li, Yue, Chen, Yang, Huang, Zhu, Hong and Yang. This is an open-access article distributed under the terms of the Creative Commons Attribution License (CC BY). The use, distribution or reproduction in other forums is permitted, provided the original author(s) and the copyright owner(s) are credited and that the original publication in this journal is cited, in accordance with accepted academic practice. No use, distribution or reproduction is permitted which does not comply with these terms.
*Correspondence: Fen-fang Hong, hongfenfang@126.com; Shu-long Yang, slyang@ncu.edu.cn
†These authors have contributed equally to this work