- 1State Key Laboratory of Southwestern Chinese Medicine Resources, Chengdu, China
- 2School of Pharmacy, Chengdu University of Traditional Chinese Medicine, Chengdu, China
- 3Hospital of Chengdu University of Traditional Chinese Medicine, Chengdu, China
Natural products have a significant role in the prevention of disease and boosting of health in humans and animals. Stroke is a disease with high prevalence and incidence, the pathogenesis is a complex cascade reaction. In recent years, it’s reported that a vast number of natural products have demonstrated beneficial effects on stroke worldwide. Natural products have been discovered to modulate activities with multiple targets and signaling pathways to exert neuroprotection via direct or indirect effects on enzymes, such as kinases, regulatory receptors, and proteins. This review provides a comprehensive summary of the established pharmacological effects and multiple target mechanisms of natural products for cerebral ischemic injury in vitro and in vivo preclinical models, and their potential neuro-therapeutic applications. In addition, the biological activity of natural products is closely related to their structure, and the structure-activity relationship of most natural products in neuroprotection is lacking, which should be further explored in future. Overall, we stress on natural products for their role in neuroprotection, and this wide band of pharmacological or biological activities has made them suitable candidates for the treatment of stroke.
Introduction
Stroke is a disease with high prevalence and incidence (Benjamin et al., 2018). Strokes can be divided into two categories: ischemic and hemorrhagic stroke, and over 80% are ischemic stroke (Zhou et al., 2018). Ischemic stroke is a pathological condition characterized by blood vessels occlusion and insufficient of blood supply. Stroke which is one of the most common causes of disability and death worldwide, seriously endangers human health and brings heavy burden to society and family (Donnan et al., 2008; Kalogeris et al., 2016). The pathological mechanism of cerebral ischemia is a complex cascade reaction, and its severity is related to the time of cerebral ischemia and the depth of the ischemic site (Steliga et al., 2020). The occurrence and development of cerebral ischemia included neuron excitotoxicity, mitochondrial dysfunction, neuroinflammatory damage, oxidative stress, etc. It is generally believed that glutamate excitotoxicity, energy metabolism disorder, and Ca2+ overload happened within 24 h of the onset of stroke, accompanied by the generation of free radicals (Guo, Li and Wang, 2009; Manzanero, Santro, Arumugam, 2013). Apoptosis and necrosis also occurred within a few hours of ischemia (Wang, C. et al., 2015). In the subacute phase, brain edema and blood-brain barrier (BBB) destruction happened. Endothelial cells, pericytes, astrocytes, etc are activated and inflammatory factors are released, accompanied with the proliferation of reactive glial cells (Stanimirovic and Satoh, 2000; Liebner et al., 2018). There exists endogenous repair in the late stage of cerebral ischemia, and neurogenesis, glial scars, angiogenesis could be observed (Steliga et al., 2020). Therefore, the occurrence and development of stroke is complicated, which makes its treatment very difficult. Tissue plasminogen activator (t-PA) is the only therapeutic medicine for ischemic stroke approved by Food and Drug Administration (FDA). However, t-PA has a restricted time window of 6 h, and delayed t-PA infusion increases the risk of hemorrhagic transformation and carries high mortality (Group 1995; Hacke et al., 2008). Despite constantly increasing understanding of ischemic stroke pathophysiology through laboratory and clinical studies, the treatment strategy is still not ideal. Therefore, there is an urgent medical need to identify new molecules that can provide neuroprotection against cerebral ischemic or ischemic reperfusion (I/R) injury.
Natural products obtained from natural sources, including plants, animals, fungi and microorganisms. Natural products have played an important role in ancient traditional medicine systems, such as Unani, Chinese and Ayurveda which are still in common use today. Natural products are known to exert additive, synergistic or antagonistic effects on the body. With the pharmacology technology developing, the administration forms of natural products are various, which has drawn much attention for the treatment of stroke from drug discovery to drug delivery (Hermann et al., 2019; Long et al., 2020; Williams et al., 2020). The pathogenesis of stroke is a complex cascade reaction, including neuro-inflammatory damage, oxidative stress, mitochondrial dysfunction, neurotoxicity, blood-brain barrier (BBB) disruption, neurovascular unit (NVU) damage and other factors. In recent years, it’s reported that a vast number of natural products have demonstrated beneficial effects on stroke worldwide (Fei et al., 2020). The neuroprotection of natural products has been discovered to modulate activities via multiple targets and signaling pathways with direct or indirect effects on enzymes, such as kinases, receptors and proteins (Bagli et al., 2016). This wide band of pharmacological or biological activities has made them suitable candidates for the treatment of stroke (Cai et al., 2019; Zhang, C. et al., 2020; Zhong et al., 2020). As a requirement of novel drug development for stroke, there is a focus on the neuroprotection of natural products via multi-targets. Hence, we stress on natural products for their role in neuroprotection (Figure 1). This review provides a comprehensive summary of the pharmacological effects and multiple target mechanisms of natural products for cerebral ischemic or I/R injury.
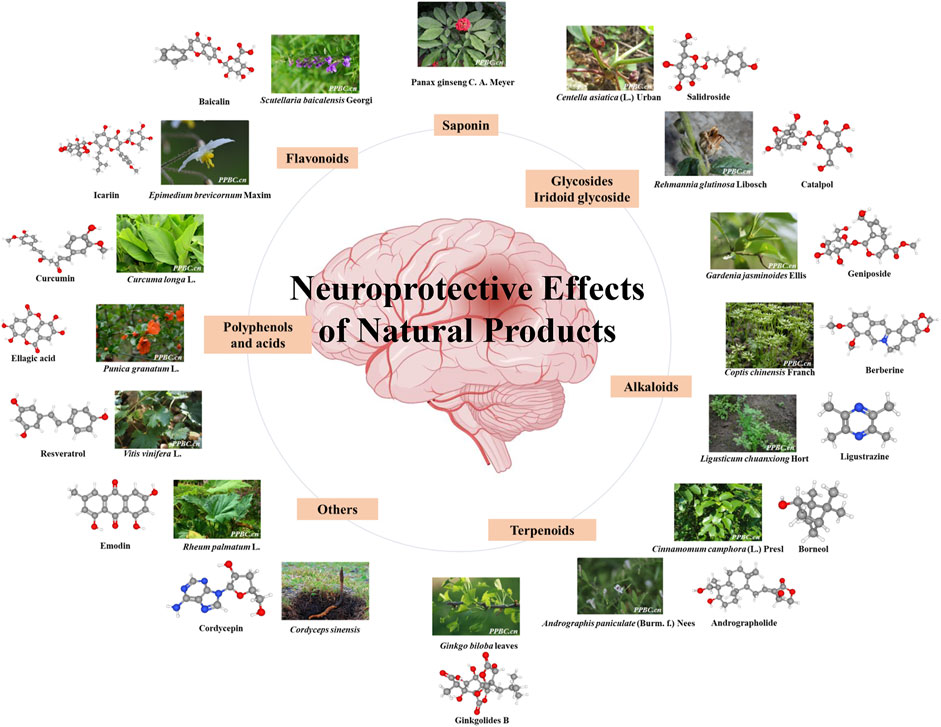
FIGURE 1. Neuroprotective effect of various natural products. The color figures of medicine are viewed in www.iplant.cn. The club model comes from PubChem.
Methodology
Database searches using Google scholar, PubMed, and China National Knowledge Internet (CNKI) were conducted until July 2020 to include up to date documented information in the present review. For data mining, the following words were used in the databases mentioned above: neuroprotection, natural product, phytoconstituents, natural products cerebral ischemic injury, natural products cerebral ischemic reperfusion injury, in vivo and in vitro studies for prevention and treatment of stroke. In almost all cases, the original articles or abstracts were obtained and the relevant data was extracted.
Neuroprotective Role of Flavonoids in Ischemic Brain Injury
Flavonoids, a natural bioactive compound found abundant in vegetables, fruits and traditional herbal medicine. Prevention and/or treatment with flavonoids such as baicalin, apigenin, vitexin, quercetin and other flavonoid compounds have shown promising neuroprotective effects against ischemic-induced injury by through increasing neuronal viability, cerebral blood flow and reducing ischemic-related apoptosis (Putteeraj et al., 2018).
Baicalin
Baicalin, mainly derived from the root of Scutellaria baicalensis Georgi, is one kind of a crucial flavonoid (Figure 2A). This medicinal plant is widely distributed in China, Russia, Mongolia, North Korea and Japan, and has the functions of clearing away heat and dampness, purging fire and detoxification (Zhao et al., 2019). In recent years, baicalin has also been found to have a wide range of neuroprotective effects (Liang et al., 2017; Sowndhararajan et al., 2018). Middle cerebral artery occlusion (MCAO) induced cerebral ischemic injury in vivo and oxygen–glucose deprivation (OGD) induced hypoxia injury in vitro were implemented. Baicalin at doses ranging from 50 to 200 mg/kg could significantly improve neurological deficit and reduce infarct volume via inhibiting apoptotic and oxidative pathway, including myeloid cell leukemia 1 (Mcl 1), B-cell lymphoma-2 (Bcl 2), superoxide dismutase (SOD), malondialdehyde (MDA), and so on (Cao et al., 2011; Zheng et al., 2015). Neuroprotective activity was also confirmed in another study. Baicalin could decrease nuclear factor kappa B (NF-κB) p 65 to exert neuroprotection (Xue et al., 2010). In addition, baicalin 100 mg/kg inhibits toll like receptor 2/4 (TLR2/4) signaling pathway in cerebral ischemia rats (Tu et al., 2011). Baicalin down-regulated nucleotide-binding oligomerization domain protein 2 (NOD2) receptor and tumor necrosis factor α (TNF α) expression of neurons with OGD in vitro and cerebral ischemia-reperfusion in vivo (Li, F et al., 2010). These findings suggested baicalin could afford neuroprotection through multiple pathways.
Scutellarin and Scutellarein
Scutellarin (Figure 2B) and scutellarein (Figure 2C), is the major active component extracted from Erigeron breviscapus (Vant.) Hand-Mazz, a Chinese herbal medicine (Zhang et al., 2009). Accumulated data have demonstrated the effectiveness and benefits of breviscapine and scutellarin in treating cerebrovascular disease and cardiovascular disease in clinic and in experimental study (Cao et al., 2008; Wang et al., 2015a; Gao et al., 2017). It has demonstrated that scutellarin is benefit to brain injury caused by cerebral ischemia/reperfusion due to its anti-oxidation and anti-inflammatory effects and ability to attenuate neuronal damage (Wang and Ma 2018). Scutellarin could effectively suppress the inflammatory response in activated microglia/brain macrophage in experimentally induced cerebral ischemia, whose underlying mechanism was associated with suppressing the phosphory c-Jun N-terminal kinase (p-JNK) and p-p38 mitogen-activated protein kinase (MAPK), increasing phosphorylation extracellular regulated kinase 1/2 (p-ERK1/2) (Chen H.-L et al., 2020). In MCAO rats given scutellarin 100 mg/kg treatment, neurological scores were significantly improved coupled with a marked decrease in infarct size, which was found that scutellarin had a neuroprotective effect, amplified TNC1 astrogliosis and activated microglia to remodel injured tissue via notch pathway (Fang et al., 2015; Fang et al., 2016). Pretreated with scutellarin 50 or 75 mg/kg has protective effects for cerebral injury through upregulating endothelial nitric oxide synthase (eNOS) expression and downregulating of vascular endothelial growth factor (VEGF), basic fibroblast growth factor (bFGF), and inducible nitric oxide synthase (iNOS) expression (Hu et al., 2005). Another study also proved scutellarin protected against ischemic injury through regulation of nicotinamide adenine dinucleotide phosphate oxidase 2 (NOX2) and connexin 43 to decrease oxidative damage and apoptotic cell death (Sun, J. B. et al., 2018; Zhang et al., 2009). Scutellarin and scutellarein could improve neuronal injury, and scutellarein had better protective effect than scutellarin through improving the Ca2+-ATPase and Na+, K+-ATPase activity in rat cerebral ischemia with bilateral common carotid arteries (BCCAO) (Tang et al., 2014). Scutellarin protects brain from acute ischemic injury probably through its inhibitory effect on the angiotensin-converting enzyme (ACE) and Ang II type 1 receptor (AT1R), also TNF-α, interleukin-6 (IL-6), and interleukin-1β (IL-1β) (Wang et al., 2016).
Apigenin and Vitexin
Apigenin (Figure 2D) is a natural flavonoid and vitexin (Figure 2E) is an apigenin flavone glycoside, which were found in several dietary plant foods as vegetables and fruits, and had a variety of pharmacological effects (Babaei et al., 2020). It has been reported that apigenin and vitexin played a protective role in diseases associated with the oxidative process, such as cardiovascular and neurological disorders (Ayoobi et al., 2017; Che et al., 2020; Li, S. et al., 2017). Angiogenesis is one of the ways to repair brain injury. It’s reported that apigenin could promote cell proliferation, tube formation, and cell migration while inhibiting apoptosis and autophagy by affecting caveolin-1/VEGF, Bcl-2, caspase-3, Beclin-1, and mechanistic target of rapamycin (mTOR) expression. What’s more, apigenin significantly reduced neurobehavioral scores and volume of cerebral infarction while promoting vascular endothelial cell proliferation by upregulating VEGFR2 to affect caveolin-1, VEGF, and eNOS expression in brain tissue of I/R rats (Pang et al., 2018). Vitexin could also play a protective role by suppressing oxidative stress (Cui et al., 2019). In addition, vitexin regulated cell apoptosis and autophagy via MAPK and mTOR/Ulk1 pathway to attenuate cerebral I/R injury (Wang et al., 2015b; Jiang et al., 2018). Apigenin also showed long-term therapeutic effect in cognitive impairments after cerebral I/R injury (Tu et al., 2017). Above all, it suggested apigenin and vitexin had neuroprotective effect through inflammation, angiogenesis, apoptosis and autophagy pathways with multi-targets.
Icariin
Icariin (Figure 2F), an active flavonoid extracted from Epimedium brevicornum Maxim, has been proven to possess a wide range of efficacy including anti-tumor, anti-oxidant, anti-bacterial and anti-inflammatory, etc (Luo et al., 2007; Zhou et al., 2011). It has been also reported to alleviate brain injury and attenuate cognitive deficits (Li, H. et al., 2010; Li et al., 2005). Xiong et al. and Deng et al. found that pretreatment with Icariin and Icariside II, a metabolite of icariin, could decrease neurological deficit score, diminish the infarct volume, and reduce IL-1β and transforming growth factor-β (TGF-β1). Moreover, Icariin suppressed inhibitory κB (IκB)-α degradation and NF-κB activation and up-regulated peroxisome proliferator-activated receptor α (PPARα) and peroxisome proliferator activated receptor γ (PPARγ) levels in I/R model (Deng et al., 2016; Xiong et al., 2016). Moreover, a study by Mo et al. found that Icariin can inhibit apoptosis and inflammatory in neurons after OGD/R through inositol requiring enzyme 1 (IRE1)- X-Box Binding Protein 1 (XBP1) signaling pathway (Mo et al., 2020).
Quercetin
Quercetin (Figure 2G), is a common flavonoid found in many fruits and vegetables (Lee et al., 2003). It has been reported that quercetin has an anti-oxidative property, anti - inflammation and immunity, anti-cancer, etc (Costa et al., 2016; Li, W. et al., 2016; Shafabakhsh and Asemi 2019; Xu, D. et al., 2019). A study by Jin et al. found that quercetin could increase the expression of ZO-1, Claudin-5, β-catenin, and LEF1, and decrease the expression of MMP-9, GSK-3β and axin to reduce brain edema and BBB leakage and improve BBB dysfunction, which suggested the neuroprotection of quercetin was related to Wnt/β-catenin pathway (Jin et al., 2019). Calcium acts as a second messenger that mediates physiologic functions. It’s reported that quercetin exerted a preventative effect through attenuation of intracellular calcium overload and restoration of down-regulated hippocalcin expression during ischemic injury (Park et al., 2020). Moreover, quercetin alleviated the increasement of protein tyrosine and serine/threonine phosphatase activity, along with the reduction of ERK and Akt phosphorylation in cerebral I/R injury (Wang, Y. Y. et al., 2020). In addition, iso-quercetin protected against oxidative stress and neuronal apoptosis via Nrf2-mediated inhibition of the NOX4/ROS/NF-κB signaling pathway in cerebral ischemic stroke (Dai et al., 2018).
Calycosin
Calycosin (Figure 2H), an isoflavone phytoestrogen isolated from Astragalus membranaceus, is found to possess various potential pharmacological activities, such as, anti-tumorigenesis, anti-oxidation, anti-virus and apoptosis-modulation effects (Chen, J et al., 2015; Wang and Zhao, 2016; Zhao, Y. et al., 2016). It was also demonstrated that calycosin 30 mg/kg could ameliorate both the neurological deficit and infarct volume in experimental cerebral ischemia reperfusion injury, and the mechanism might be attributed to its antioxidant effects (Guo et al., 2012). Post-stroke calycosin 30 mg/kg therapy increased brain-derived neurotrophic factor (BDNF)/TrkB to ameliorate the neurological injury due to switching the microglia from the activated ameboid state to the resting ramified state in ischemic stroke rats (Hsu et al., 2020). Calycosin exhibited a downregulation of dexamethasone-induced Ras-related protein 1 (RASD1), and an upregulation of estrogen receptor (ER)-α and Bcl-2 (Wang, X. et al., 2014). A study by Wang et al. found that calycosin pretreatment for 14 days dramatically upregulated p62, neighbor of BRCA1 gene 1 (NBR1) and Bcl-2, and downregulated TNF-α to ameliorate neurological scores in cerebral I/R rats (Wang, L. et al., 2018).
Neuroprotective Role of Alkaloids in Ischemic Brain Injury
Alkaloids are rated as among the most-investigated plant secondary metabolites with versatile biological activity (Qiu et al., 2014). Recent investigations have pointed out the dynamic role of alkaloids as antibacterial, antidepressant, antioxidant, anti-inflammation (Perviz et al., 2016; Li et al., 2020; Liu et al., 2020; Rosales et al., 2020). Accordingly, alkaloids are emerging as pharmaceutical tools for neuroprotective effects (An et al., 2020). Alkaloids were investigated on neuroprotective effects including berberine, ligustrazine, tetrahydropalmatine and others.
Berberine
Berberine (Figure 3A) is an isoquinoline alkaloid isolated from traditional herbal medicine. It is widely present in the roots, rhizomes, stems or bark of various natural plants, such as Coptis chinensis Franch., Phellodendron chinense Schneid., etc. These plants have been used for thousands of years (Wang et al., 2019a; Fan et al., 2019). Studies have shown that berberine has a variety of pharmacological activities, including anti-microbial, anti-tumor, lowering glucose, lowering cholesterol, anti-inflammatory, antioxidant, immunomodulatory and neuroprotective effects (Asemi et al., 2020; Song et al., 2020). So far, the neuroprotective effect of berberine has been involved (Lin and Zhang 2018). Berberine could improve the survival rate in OGD/R cells and improve the neurological deficiency in cerebral ischemic injury, which was involved in reducing the expression of cleave caspase 3 and up-regulating the expression of p-Bad to inhibit cell apoptosis via TrkB-PI3K/Akt pathway to promote neuron growth (Hu et al., 2012; Chai et al., 2013; Yang et al., 2018a). Other studies also showed berberine 40 mg/kg preconditioning had neuroprotective effects by promoting autophagy, reducing cell apoptosis via PI3K/Akt signaling pathway (Zhang et al., 2016a; Zhang et al., 2016b). In addition, berberine exerted the neuroprotective effects by regulating I/R-induced peripheral lymphocytes early immunoactivation (Song et al., 2012). A study by Zhang et al. found that neuroprotection of early and short-time applying berberine could upregulate p-Akt, p-GSK 3β and phosphor cAMP response element binding protein (p-CREB) and downregulate NF-κB expression to ameliorate BBB permeability in the acute phase of cerebral ischemia (Zhang et al., 2012).
Tetramethylpyrazine
Tetramethylpyrazine (Figure 3B), also called ligustrazine, is a kind of alkaloids identified in Ligusticum chuanxiong Hort, which has been used to treat cardiovascular and cerebrovascular diseases for hundreds of years in ancient China (Zhao, X. et al., 2016; Zheng, J. S. et al., 2018). In recent study, Ligusticum chuanxiong Hort and its main active compound ligustrazine have been studied and had anti-inflammatory, anti-oxidant and analgesic effect (Kao et al., 2006; Zou et al., 2018). Tetramethylpyrazine treatment with 20 mg/kg suppressed hypoxia inducible factor-1-alpha (HIF-1α), TNF-α, and activated caspase-3 expression in MCAO-induced brain ischemia in rats (Chang et al., 2007). Tetramethylpyrazine 20 mg/kg inhibited neutrophil activation via elevating nuclear related factor 2 (Nrf2) and heme oxygenase-1 (HO-1) expression and inhibiting high-mobility group box-1 protein (HMGB1)/toll-like receptor-4 (TLR4), Akt, and ERK pathway following cerebral ischemia rats (Chang et al., 2015). Tetramethylpyrazine analogues were also investigated to induce p-eNOS by activation of PI3K/Akt and TLR-4/NF-κB signaling pathway and regulate the expression of tight junction (TJ) proteins, matrix metalloprotein 9 (MMP-9) and aquaporin 4 (AQP4) under cerebral I/R injury (Yan et al., 2015; Xu et al., 2017; Zhou et al., 2019). The similar finds of PI3K/Akt pathway also were shown in another study (Ding et al., 2019). What’s more, the synergistic effect of drugs has also been shown to have good therapeutic effect. Ligustrazine accompanied with borneol could attenuate I/R injury by downregulating IL-1β, IL-6, and TNF-α, upregulating SOD, GSH-Px and regulating apoptosis and autophagy (Yu, B. et al., 2017; Yu et al., 2016).
Daurinoline
Daurinoline (Figure 3C) is a kind of dibenzyl tetrahydroisoquinoline alkaloid, isolated from menispermum dauricum DC., which has a variety of pharmacological activities, including neuroprotective effect (Zhang et al., 2011). Daurinoline 10 mg/kg and 20 mg/kg could enhance of SOD activity and the ability of scavenging oxygen free radicals (Lan and Lianjun 2020). Daurinoline reduced the level of MDA in brain tissue and increase the activity of SOD to prevent and ameliorated energy metabolism disturbance of cortex and mitochondria of brain induced by repeatedly cerebral ischemia reperfusion (Li and Gong 2004). In addition, daurinoline 5 and 10 mg/kg could inhibit cytochrome c (Cyt-C) release and caspase-3 and caspase- 9 to inhibit neuronal cells apoptosis in the penumbra after cerebral ischemia (Yang et al., 2009).
Levo-tetrahydropalmatine
Levo-tetrahydropalmatine (Figure 3D) are a series of alkaloids, isolated from a Chinese analgesic medicine, called Corydalis yanhusuo W. T. Wang. l-Tetrahydropalmatine, one of its main active ingredients, has been demonstrated to have potent analgesic effects and has been used in Chinese clinical practice for this purpose for many years (Chu et al., 2008; Han et al., 2012; Kang et al., 2016). Recent work showed that l-tetrahydropalmatine suppressed the central excitatory effects of amphetamine (Chang and Lin 2001), and played a protective role against neuronal injury in animal models (Liu et al., 2005; Mantsch et al., 2007; Chen et al., 2012). Levo-tetrahydropalmatine could regulate Src and c-Abl expression to inhibit neuron apoptosis and attenuate BBB injury in cerebral ischemic animals (Mao et al., 2015; Sun, J. B et al., 2018).
Peimine
Peimine (Figure 3E) is a major biologically active component of Fritillaria ussuriensis. Recent studies have shown that isosteroidal alkaloids in Fritillaria had a wide range of pharmacological activities besides its role in cough remedies (Li, J. et al., 2019; Li et al., 2020a; Zhang et al., 2020a; Zhao et al., 2018). It’s also reported that peimine had protective effects on cerebral I/R injury in rats. The mechanism of neuroprotective effect might be related to inhibition of apoptosis, oxidative stress and inflammatory response, reduction of pathological damage and neurological dysfunction through regulation of the PI3K/Akt/mTOR pathway (Guo et al., 2019; Duan et al., 2020).
Neuroprotective Role of Polyphenols and Acids in Ischemic Brain Injury
Polyphenols, a bioactive compound, were found abundant in vegetables, fruits and medical plant. Neuroprotection of polyphenols in medical plants is getting attention in the world. Curcumin, ellagic acid, resveratrol has been extensively studied and show multi-function. They are neuroprotectants, antioxidants, anti-inflammatory and antithrombic agents. It’s reported that the neuroprotective efficacy of these compounds was involved in mitigating brain infarction and global ischemia, improving cerebral blood circulation (Lin 2011).
Curcumin
Curcumin (Figure 3F), a polyphenolic compound extracted from Curcuma longa L., has potential anti-inflammatory, cardiovascular protective effect and neuroprotection. Recent studies demonstrated the neuroprotective effect of curcumin against cerebral ischemic injury through oxidation, apoptosis, autophagy pathways (Eghbaliferiz et al., 2020; Forouzanfar et al., 2020; Ułamek-Kozioł et al., 2020). Rats or mice with cerebral ischemic injury that received curcumin at 10–400 mg/kg exhibited significantly alleviated brain injury. Moreover, a more SOD, GSH-Px and glutathione (GSH) and a lower MDA, NO contents were found in curcumin administrated animals (Thiyagarajan and Sharma 2004; Li. Y. et al., 2016), which suggested the mechanism of curcumin was related to antioxidative activity. In addition, curcumin was proven to suppress the release of inflammatory cytokines via NF-κB, signal transducer and activator of transcription (STAT), Akt/mTOR, ERK signaling pathways (Huang et al., 2018; Li et al., 2015; Mukherjee et al., 2019; Xu, H. et al., 2018). Moreover, Bax, Bcl 2, caspase 3, LC3 II activity and other autophagy and apoptosis cytokines were also reversed by curcumin (Hou et al., 2019; Huang et al., 2018; Wang et al., 2005; Xie, C. J. et al., 2018; Xu, L. et al., 2019; Zhang et al., 2018a). Furthermore, curcumin promoted neuron survival in vitro to exert neuroprotective effects against ischemia injury (Lu et al., 2018; Xie, W. et al., 2018; Zhang, X. et al., 2018).
Resveratrol
Resveratrol (Figure 3G), also named 3,4,5-trihydroxy-trans-stilbene, a natural polyphenolic compound, occurs naturally in grapes and a variety of medicinal plants, and possesses multiple biological activities (Santos et al., 2019; Thapa et al., 2019). The effect of resveratrol on cerebral ischemic injury was also explored. Resveratrol dosage at 10–100 mg/kg marked improved neurological deficiency and protected against cerebral ischemic injury though PI3K/Akt, NF -κB and other pathways (Lei et al., 2020; Simao et al., 2012a; Simao et al., 2012a; Yu, P. et al., 2017). Resveratrol treatment could obviously upregulate nuclear factor erythroid 2-related factor 2 (Nrf2) and heme oxygenase-1 (HO-1) to ameliorate oxidative damage in cerebral ischemic injury (Ren et al., 2011; Yang et al., 2018b; Gao et al., 2018). Furthermore, MDA, NO, SOD could be reversed by resveratrol administration (Jie et al., 2020; Tsai et al., 2007; Xu, J. et al., 2018). Silent information regulator 1 (Sirt 1) is a NAD + dependent deacetylase, which plays an important role in cerebral I/R injury (She et al., 2017). It’s reported that resveratrol protected against cerebral ischemic injury by inhibiting NLRP3 inflammasome activation, improving alterations in mitochondrial and glycolytic function through Sirt1 pathway (Della-Morte et al., 2009; Koronowski et al., 2015; He et al., 2017; Koronowski et al., 2017). Moreover, resveratrol could inhibit inflammatory response, including prostaglandin E2 (PGE2), cyclooxygenase (COX)-2, NOS and MMP 9 to alleviate neurological deficits (Candelario-Jalil et al., 2007; Simao et al., 2012b; Wei et al., 2015). In addition, resveratrol could improve brain energy metabolism via inhibiting xanthine oxidase activity and preventing the production of hypoxanthine, xanthine and oxygen radicals (Li et al., 2011). These findings suggested resveratrol could reduce cerebral ischemia injury through multiple pathways.
Ellagic Acid
Ellagic acid (Figure 3H), an important cell protective and antioxidant compound, is a low molecular weight polyphenol derived from several fruits, vegetables and nuts (De Oliveira, 2016). Ellagic acid are recently more taken into accounts since their promising pharmacological effects, such as, anti-inflammation, anti-oxidant and anti-cancer (Ceci et al., 2018; Baradaran Rahimi et al., 2020). In recent study, ellagic acid is investigated as a potential endowed with multi-target pharmacological properties on central nervous system (Alfei et al., 2019). BCCAO was carried out to make global cerebral ischemic injury. Ellagic acid pretreatment with 100 mg/kg could reduce MDA level and restore the heart rate to normal level (Nejad et al., 2015). In addition, ellagic acid 100 mg/kg could activate PI3K/Akt/NOS pathway to alleviate brain injury and protect brain tissue in MCAO model (Kaihua and Jiyu 2020). Furthermore, ellagic acid administration with 10–90 mg/kg could improve brain injury outcomes and increase the proliferation of NSCs through the Wnt/β-catenin signaling pathway (Liu, Q. S. et al., 2017). The upregulation of zonula occludens-1 (ZO-1) and down-regulation of AQP 4 and MMP-9 in injured brain tissues after being treated ellagic acid 10–50 mg/kg (Wang et al., 2019b).
Neuroprotective Role of Glycosides in Ischemic Brain Injury
Asiaticoside
Asiaticoside (Figure 4A) is isolated from Centella asiatica (L.), which has been using as a memory enhancing and psychoactive drug for a long time in Asia (Zheng and Qin 2007). Many studies have shown that C. asiatica indeed has many biological activities in central nervous system (Mook-Jung et al., 1999; Zheng and Qin 2007; Dhanasekaran et al., 2009; Orhan 2012). A report demonstrated asiaticoside showed anti-inflammation effect via inhibiting overactivation of p38 MAPK pathway against cerebral I/R mice (Chen et al., 2014). Another study found asiaticoside showed a protective effect against cerebral I/R injury via the NOD2/MAPK/NF-kB signaling pathway (Zhang et al., 2020b).
Salidroside
Salidroside (Figure 4B), the major phenylpropanoid glycoside extract from medicinal Tibetan plant Rhodiola rosea L, has diverse pharmacological activities. Many recent reports and reviews have highlighted that salidroside may exert anti-inflammatory, neuroprotective effects and improve cognitive function (Li, M. et al., 2018; Xu, N. et al., 2019). Salidroside may involve the modulation of monoamine metabolism in the striatum and substantia nigra pars compacta (SNpc), which may be related to the function of the dopaminergic system in the cerebral I/R brain (Zhong et al., 2019). Many studies reported salidroside protected against cerebral I/R injury via PI3K/Akt pathway, accompanying with Nrf2/NF-κB pathway (Wei et al., 2017; Zhang, Y. et al., 2018; Zhang, J. et al., 2019; Zuo et al., 2018). Salidroside exhibited neuroprotective effects against hypoxia/reperfusion injury by activating the SIRT1/FOXO3α pathway (Xu, L. et al., 2018). In addition, salidroside is an effective treatment for ischemic stroke that functions via the fibroblast growth factor 2 (FGF2)-mediated cAMP/PKA/CREB pathway to promote dendritic and synaptic plasticity (Li et al., 2020b). Salidroside treatment reduced the expression of M1 microglia/macrophage markers and increased the exdslbpression of M2 microglia/macrophage markers after stroke and induced primary microglia from M1 phenotype to M2 phenotype (Liu et al., 2018). What’s more, salidroside reduced the markers of endothelial activation and neutrophilic infiltration after I/R injury by inhibition of complement, restoring an anti-inflammatory endothelial phenotype after oxidative stress and inhibiting classical complement activation, in association with anti-apoptotic effects (Wang, Y. et al., 2020).
Neuroprotective Role of Iridoid Glycosides in Ischemic Brain Injury
Iridoid glycosides are special glycosides. Iridoid glycoside exists broadly in plants of many families and has a wide variety of biological activities including purgative, liver protective, anti-microbial, analgesic, antitumor, sedative and anti-inflammatory activities (Ismailoglu et al., 2002). Geniposide, Catalpol and Picroside II were investigated in depth as iridoid glycosides, which had a neuroprotective effect.
Geniposide
Geniposide (Figure 4C), as an iridoid glycoside, was initially isolated from the herb Gardenia jasminoides Ellis (Wang et al., 1992), which has been noted for its variable pharmacological effects, including anti-oxidation, anti-inflammation, and anti-diabetes effects, among others (Koo et al., 2006; Wu et al., 2009). Previous studies showed that geniposide induced neuronal differentiation and attenuated cell injury (Liu et al., 2009; Yin et al., 2010). To date, there were some studies about the cerebral ischemia injury. Geniposide treatment after neonatal hypoxic-ischemia (HI) insult attenuated cell apoptosis, IgG leakage, microgliosis, astrogliosis, pericytes loss and junction protein degradation, which might be through the activation of PI3K/Akt pathway (Liu, F. et al., 2019). Geniposide attenuated inflammatory response by suppressing P2Y14 receptor and downstream ERK1/2 pathway in brain microvascular endothelial cells (BMECs) with OGD (Huang et al., 2017; Li, Y. et al., 2016). What’s more, geniposide in neuroprotection by activating autophagy and inhibiting NLRP3 inflammasome in microglial cells (Fu et al., 2020).
Catalpol
Catalpol (Figure 4D) is the main active component of the radix from Rehmannia glutinosa Libosch, and it belongs to the iridoid monosaccharide glycoside family (Ismailoglu et al., 2002; Zhang et al., 2008), which has pleiotropic protective effects on many diseases, including neurodegenerative diseases (Xia et al., 2012), ischemic stroke (Zhu et al., 2010), metabolic disorders (Zhu et al., 2010) and others. It’s reported that the efficacy of catalpol pretreatment on cerebral I/R injury may be attributed to reduction of free radicals and inhibition of lipid peroxidation and endothelin-1 (ET-1) production (Liu, H. et al., 2014). Additionally, a study by Li et al. found catalpol also exerted the most significant cytoprotective effect on astrocytes by suppressing the production of free radicals and elevating antioxidant capacity (Li et al., 2008). What’s more, catalpol significantly inhibited apoptosis by modulating Bcl-2 and Bax (Li et al., 2006). Catalpol affected angiogenesis via the JAK2/STAT3 signaling pathway and VEGF expression (Dong, W et al., 2016).
Picroside II
Picrorhiza scrophulariflora belongs to the plant family composed of picroside I, II and III, of which picroside II (Figure 4E) is one of the most effective components extracted from the dried rhizome and roots of Picrorhiza kurrooa Royle ex Benth and Picrorhiza scrophulariae flora Pennell (Stuppner and Wagner 1989; Wang et al., 1993). Current researches on picroside II are focused on its neuroprotective, anti-apoptotic, anti-cholestatic, anti-oxidant, anti-inflammation, immunomodulating activities (Cao et al., 2007; Li et al., 2007; He et al., 2009). It has been confirmed that picroside II 25 mg/ml could enhance nerve growth factor - induced PC12 cell axon growth, reduce H2O2 induced PC12 cell damage and improve cell survival in vitro (Cao et al., 2007). Picroside II attenuated cerebral I/R injury via inhibiting apoptosis and inflammation, included COX2, TLR4/NF-κB and MEK-ERK1/2 pathway (Guo et al., 2010; Wang, F. et al., 2015; Wang, L. Y. et al., 2015). Picroside II could protect BBB possibly through reducing oxidative stress factors (ROS, NOX2 ROCK, MLCK, and MMP-2) and enhancing BBB function factors, claudin-5 (Zhai et al., 2017). Furthermore, picroside II exerted a neuroprotective effect by inhibiting the mitochondria Cyt C signal pathway and decreasing the permeability of mitochondrial permeability transition pore (mPTP) following I/R injury in rats (Zhang et al., 2017; Li. Q et al., 2018).
Neuroprotective Role of Saponin in Ischemic Brain Injury
Ginsenoside Rg1 (Figure 5A) is the representative components in saponin. Ginsenoside Rg1 is one of the main active ingredients of ginseng (Zhang and Zhao 2014; Chuang et al., 2015). It has been shown that as a small molecular substance, ginsenoside Rg1 easily passes through the blood brain barrier. Moreover, ginsenoside Rg1 could promote stem cell orientation transformation, induce stem cell proliferation and played a neuroprotective role in brain repair (Cheng et al., 2005; Tang et al., 2017; Xie, C. J. et al., 2018). It’s reported that ginsenoside Rg1 could relieve the I/R injury through multiple pathways. Ginsenoside Rg1 could improve neurological injury, regulate BBB disruption and permeability and downregulate AQP 4 and protease-activated receptor-1 (PAR 1) (Zhou et al., 2014; Xie et al., 2015). Ginsenoside Rg1 alleviated oxidative stress after I/R through inhibiting miR-144 activity and subsequently promoting the Nrf2/ARE pathway (Chu et al., 2019). What’s more, a study by Li et al. found that ginsenoside Rg1 may exert its neuroprotective action on cerebral I/R injury through the activation of PPARγ signaling (Li et al., 2017). In addition, it’s also shown that ginsenoside Rg1 treatment obviously decreased cell apoptosis, while the transplanted cells could be differentiated into neurons and glial cells, which also improved cerebral ischemia (Bao et al., 2015). Other studies showed that ginsenoside Rg1 40 mg/kg was attributed to a decrease in ubiquitinated aggregates and a suppression of the inflammatory response and increased in the expression of BDNF in the hippocampal CA1 region after I/R insult (Wang, Y. et al., 2018; Zheng et al., 2019).
Astragaloside IV
Astragaloside IV (Figure 5B) is a triterpenoid saponin existing in the root of Astragalus membranaceus. Astragaloside IV also has a variety of pharmacological effects, such as anti-inflammatory, anti-cancer, anti-fibrosis, anti-oxidation stress, immunomodulatory through multiple signals (Ren et al., 2013; Zhang, X. et al., 2019). Meanwhile, it showed that astragaloside IV could inhibit neuroinflammation by reducing BBB permeability and lymphocyte infiltration, and played a neuroprotective role through mitochondrial pathway, antioxidant, anti-inflammatory and anti-apoptotic effects (Costa et al., 2018; Song et al., 2018), which mainly regulated JNK3, FAS, FasL, caspase-8, Bid, caspase-3 and cyto C, p62, Bax/Bcl-2, LC3II/LC3I (Li et al., 2019; Liu et al., 2013; Yin et al., 2020; Zhang, J. et al., 2019). In addition, astragaloside IV could also inhibit neutrophil adhesion related molecules (TNF-a, NF κB, IL-1β, etc.) to play an anti-inflammatory role, and had neuroprotective effect on cerebral I/R injury (Li et al., 2012).
Neuroprotective Role of Terpenoids in Ischemic Brain Injury
Andrographolide
Andrographolide (Figure 5C), a labdane diterpene lactone, is the most active and important constituent isolated from the leaves of Andrographis paniculata (Burm. f.) Nees (Acanthaceae) (Coon and Ernst 2004). Recent studies demonstrated that andrographolide possesses anticancer, anti-inflammatory and hepatoprotective activities, also neuroprotective effect (Negi et al., 2008; Bao et al., 2009). Andrographolide reduced NOX2 and iNOS expression possibly by modulating PI3K/AKT-dependent NF- κB and HIF-1 α activation, which mediated the protective effect in the cerebral I/R mice (Chern et al., 2011). Studies by Yen et al. found andrographolide could play an important role to cerebral endothelial cells (CECs). Furthermore, andrographolide increased Nrf2/HO-1 expression through p38 MAPK regulation, which provided protection against I/R injury (Yen et al., 2013; Yen et al., 2016).
Ginkgolides
Ginkgolide B (Figure 5D) were one of ten kinds of diterpene lactone compounds, which were isolated from Ginkgo biloba leaves. It’s reported that exact of Ginkgo biloba had a broad range of pharmacological effects, such as anti-inflammation, antioxidant, anti-depressant (Chen, C et al., 2017; Hu et al., 2018; Jin, G et al., 2014; Ran et al., 2014; Saini et al., 2014; Wan et al., 2016). Ginkgolides B upregulated the levels of antioxidant proteins through Akt/Nrf2 pathway to protect neurons from oxidative stress injury (Liu, Q. et al., 2019). In addition, ginkgolide B improved neurological function by promoting the proliferation and differentiation of neural stem cells in rats with cerebral I/R injury (Zheng et al., 2018b).
Borneol
Borneol (Figure 5E) is a terpene and bicyclic organic compound, a resin from Cinnamomum camphora (L.) Presl, a traditional Chinese medicine (Yin et al., 2017; Zheng et al., 2018a). As a traditional Chinese medicine, borneol has been used for thousands of years in the treatment of cardiovascular and cerebrovascular diseases. Modern pharmacological studies have shown that borneol had anti-inflammatory, antioxidant stress, anti-apoptosis and other pharmacological effects (Almeida et al., 2013). Borneol protected against cerebral I/R injury through multifunctional cytoprotective pathways, involving in the alleviation of intracellular ROS and iNOS/NO pathway, inhibition of inflammatory factor and depression of caspase-related apoptosis. Additionally, the inhibition of IκBα/NF-κB pathway might play a significant role in the neuroprotection of borneol (Liu et al., 2011). Another study by Dong et al. found that borneol could alleviate cerebral ischemic injury, most likely executed via anti-apoptosis and anti-inflammation effects and maintenance of the BBB stability and TJs to comprehensively improve NVU function (Dong et al., 2018). Additionally, the protection of OGD induced BMECs by tetramethylpyrazine phosphate and borneol combination involved anti-oxidation, apoptosis inhibition, and angiogenesis (Yu et al., 2019).
Neuroprotective Role of Other Compounds in Ischemic Brain Injury
Emodin
Emodin (Figure 5F), 1,3,8-trihydroxy-6-methylanthraquinone, is a naturally occurring anthraquinone derivative and an active component from Polygonum multiflorum Thunb. Rheum palmatum L. etc, which have been used widely in Asia in treatment of multiple diseases (Dong, X. et al., 2016). Emodin has been demonstrated to possess a wide spectrum of pharmacological effects, such as anti-viral, anti-bacterial, anti-allergic, anti-osteoporotic, immunosuppressive, neuroprotective activities (Dong, W. et al., 2016; Leung et al., 2020; Xue et al., 2020). In fact, the neuroprotective effect of Polygonum multiflorum Thunb was first published in 2000 (Gu et al., 2000) and the neuroprotective effect of emodin was published in 2005 when its ability to interfere with the release of glutamate was identified as a method of neuroprotection (Gu et al., 2005). Additionally, emodin might afford a significant neuroprotective effect against glutamate-induced apoptosis through the critical role including Bcl-2/Bax, active caspase-3, p-Akt, p-CREB, and mature BDNF for potent neuroprotective effects of emodin to subsequently enhance behavioral function in cerebral ischemia (Ahn et al., 2016). Another study by Leung et al. found emodin had neuroprotective effects against I/R or OGD injury both in vitro and in vivo, which may be increase Bcl-2 and glutamate transporter-1 (GLT-l) expression but suppress activated-caspase 3 levels through activating ERK1/2 pathway (Leung et al., 2020).
Cordycepin
Cordycepin (Figure 5G) is a derivative of nucleoside adenosine and one of the main active components of Cordyceps sinensis, which is mainly distributed in Europe, North America and Asia (Tuli et al., 2013; Wang, Y. et al., 2015). Cordycepin has a variety of pharmacological activities, such as anti-inflammatory, anti-tumor, antioxidant, anti-inflammatory microorganism, anti-hyperlipidemia, anti-hepatotoxicity, anti-depressant and neuroprotective activities (Ryu et al., 2014; Wang et al., 2015a; Qin et al., 2019). It was shown that cordycepin had an important neuroprotective effect on hypoxic injury by improving the electrophysiological function of neurons (Chen, K. et al., 2017; Liu, Z. B et althogenesis of lesions (Liu, G. et al., 2015). It is confirmed that cordycepin could significantly reduce glutamic acid and aspartic acid, increase SOD activity and down-regulate MDA and MMP-3 to alleviate cerebral ischemic injury or hypoxia injury, suggesting cordycepin could inhibit oxidative damage to exert neuroprotection (Hwang et al., 2008; Cheng et al., 2011). Cordycepin regulated adenosine A1 receptor to improve long-term potentiation formation and neuronal survival through p38/JNK/ERK pathway in BCCAO model and glutamate-induced HT22 neuronal cell death (Dong et al., 2019; Jin, M. L et al., 2014).
Polysaccharides
Polysaccharides are considered to have a wide range of pharmacological effects, such as scavenging free radicals, immune regulation, anti-tumor, anti-oxidation, anti-viral, anti-inflammatory, lowering blood sugar, anti-depression, liver protection, etc (Jin et al., 2012; Kwok et al., 2019; Fang et al., 2020). Panax notoginseng polysaccharide is a kind of heteroglycan derived from the medicinal plant Panax notoginseng, which could increase the ratio of Bcl-2/Bax and reduce caspase-3 in cerebral ischemic brain tissue (Jia et al., 2014). What’s more, it could enhance GSH-Px, SOD activity and IL 10 level, while downregulate MDA, TNF-α, IL-1β level to reduce cerebral infarction size and cell apoptosis to afford neuroprotective effect (Jia et al., 2014; Sy et al., 2015). Angelica polysaccharide is the main active ingredient of Angelica sinensis (Oliv.) Diels, which could also enhance the activities of SOD, GSH and GSH-PX, and reduce MDA, IL-1β, TNF-α and NF-κB in cerebral ischemia-reperfusion injury rats (Yan and Xie, 2018; Dai et al., 2020), in addition, enhance angiopoietin 1, angiopoietin 2, VEGF to promote angiogenesis (Hu et al., 2006; Li, J. et al., 2019). Ginkgo biloba polysaccharides could also play a neuroprotective effect by inhibiting oxidative stress and inflammation to improve neurological deficits in cerebral ischemic animals (Yang et al., 2013). Black fungus polysaccharide is the main active ingredient of Auricularia auricula (L.ex Hook.) Underw in China. Studies have found that fungus polysaccharides could reduce the production of ROS, MDA, and NO in rats with acute or chronic cerebral ischemia injury, enhance the activity of SOD and played an anti-oxidant effect. In addition, it can inhibit neuronal apoptosis and improve cerebral ischemia-reperfusion injury. (Lu et al., 2010; Qian and Min 2010; Ye et al., 2010). Fucose is a marine sulfated polysaccharide extracted from brown algae and some marine invertebrates (Apostolova et al., 2020; Oliveira et al., 2020). Experimental studies have shown that fucose can significantly reduce the levels of pro-inflammatory factors IL-1β, IL-6, MPO and TNF-α in cerebral ischemia model animals, reduce the levels of oxidative stress-related proteins SOD and MDA, and could also regulate cell apoptosis via inhibiting the MAPK pathway to play a neuroprotective effect in cerebral ischemia reperfusion study (Che et al., 2017). Other studies have shown that fucose could also play a neuroprotective effect by inhibiting neuronal apoptosis, glial cell activation and anti-oxidative stress properties (Ahn et al., 2019; Kim et al., 2019). Codonopsis pilosula polysaccharide is a kind of polysaccharide extracted from Codonopsis pilosula. Studies have shown that Codonopsis pilosula polysaccharide could reduce LDH, NO and MDA, increase the content of AChE, SOD and GSH-Px, and inhibit the expression of Beclin-1 to regulate autophagy in the cerebral ischemia-reperfusion injury model (Chen, W. et al., 2015; Ma et al., 2019). It could also inhibit the expression of GFAP protein (Li. S. et al., 2018). Codonopsis pilosula polysaccharides can also reduce the expression of Bax, up-regulate the expression of Bcl-2 and inhibit apoptosis to exert neuroprotective effects through mediating the Nrf2/HO-1 pathway (Ma et al., 2019). Lycium barbarum polysaccharide, astragalus polysaccharide, aloe polysaccharide, yam polysaccharide, etc. also have a certain protective effect on cerebral ischemia (Ge et al., 2017; Liu, H. et al., 2014; Lu et al., 2012; Peng et al., 2019; Wang, Y. et al., 2014; Yan and Zhou, 2012).
Structure-Activity Relationship Between Natural Products and Neuroprotection
Many studies have confirmed the neuroprotective effect of natural products on cerebral ischemia and clarified its preliminary mechanism of action. Different natural products have different mechanisms of anti-cerebral ischemia, which are closely related to the different structures of the compounds. Figure 6 shows the mechanism of cerebral ischemia and the role of different types of natural products in this process. The research on the structure-activity relationship of the biological activity of natural products is mainly related to the basic nucleus of the compound, the number and position of double bonds, and the position of functional groups. They have a great influence on the biological activity of the compound (Aljahdali et al., 2020; Zhou et al., 2021). The flavonoids are based on 2-phenylchromone as the skeleton, which has ortho-dihydroxyl in the structure. The hydroxyl substituent on the basic skeleton is the key functional group for scavenging oxygen free radicals. At the same time, a conjugated system is also the key active site to scavenging oxygen free radicals (Chen et al., 2013). Therefore, flavonoids have unique advantages in scavenging free radicals and inhibiting lipid peroxidation. In addition, flavonoids had anti-inflammatory effects. It is believed that when the C-5 and 7 positions of the A ring of flavonoids have hydroxyl groups at the same time, they will affect the secretion process, mitosis and cell interactions of cells, and then produce strong anti-inflammatory effect (Ying and Chi, 2007).
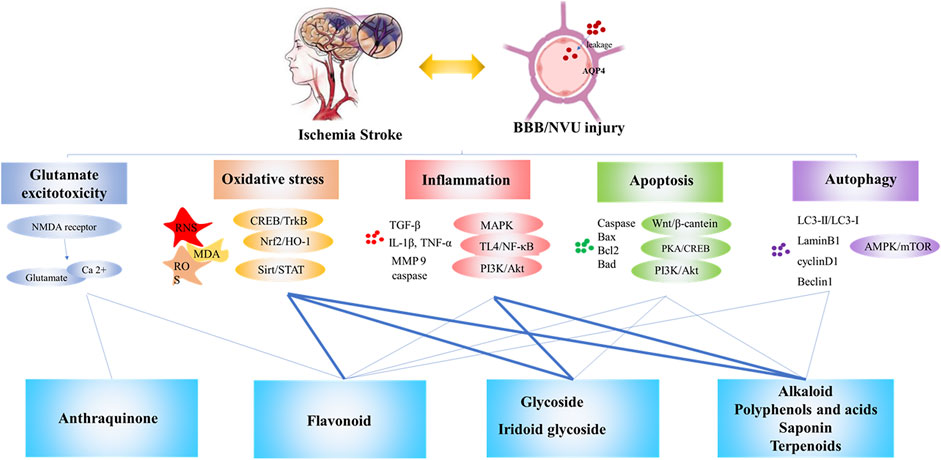
FIGURE 6. The mechanism of cerebral ischemia and the neuroprotective effect of natural products against cerebral ischemia. Note: Bold lines indicate a clear structure-activity relationship reported in the literature.
Alkaloids are quite different in structure. For example, berberine has a methylenedioxy ring structure and is the main functional group for anti-bacterial and hypoglycemic activity (Han et al., 2020; Wu and Fang, 2020). This may also be the key structure of its antioxidant effect, which needs further research to confirm. Ligustrazine has a simple structure and a wide range of pharmacological effects. Use it as a lead compound to synthesize various derivatives, which has a variety of biological activities (Wang et al., 2013), and the derivative is more stable than ligustrazine, such as ligustrazine hydrochloride injection has better curative effect in the treatment of ischemic cerebrovascular disease (Li and Zhang, 2020; You et al., 2020). Dauricine is a kind of bisbenzyltetrahydro isoquinoline alkaloid. It has a phenolic hydroxyl group and a conjugated system that is easy to form hydrogen bonds. It may be similar to flavonoids and is considered to be a key structure for antioxidant effects. Studies have shown that phenolic acids have a strong antioxidant effect, which is due to the existence of carboxyl, carbonyl and other structures (Bialecka-Florjanczyk et al., 2018; Wang, Y. et al., 2019). Glycoside components are compounds formed by non-sugar substances and sugars or sugar derivatives, which have the active characteristics both sugar substances and non-sugar substances. For example, flavonoid glycosides and flavonoid aglycones have anti-oxidative biological activity (Xue et al., 2001; Gao et al., 2008; Shao et al., 2013). In addition, most studies believe that glycoside compounds mainly exerted neuroprotective effects by improving the body’s immunity (Brito-Arias, 2007). Iridoid glycosides are a special glycoside chemical. The core of iridoid glycosides is hemiacetal-enether cyclopentane, which is an important structural basis. Anti-oxidant stress is the manifestation of its biological activity (Wang et al., 2019a). Some studies also believe that the double bond on the cyclopentane in iridoid glycosides, the C-11 substituent, and the way of forming the bond after ring opening also have important effects on the anti-inflammatory activity (Mao et al., 2019). For example, loganin, morroniside, and sorgenin all contain several electron-donating groups-hydroxyl groups. The hydroxyl groups release hydrogen atoms and combine with free radicals to block the chain reaction mediated by reactive oxygen species and reduce the body's oxidative stress. Stimulus levels, which weaken the phosphorylation of inflammatory signal pathway related factors, thereby inhibiting the inflammatory response, improving insulin resistance and liver damage (Li et al., 2014). Studies believe that tetracyclic triterpene saponins are the leading compounds for the treatment of neurological diseases, and can show strong neurological activity after proper structural modification and modification. Among them, C-7 and C-9 of lanolin triterpenoids The diene bond connected at the position may be the key group for nerve extension promoting activity (Liu, Y. et al., 2015). The research on the structure-activity relationship of tetracyclic triterpenes is mainly focused on the study of the binding sites related to the action of NMDAR antagonists, indicating that the structural modification and optimization of its aglycon and side chain sugar groups can obtain a new type of neuroactive monomer with high efficiency and low toxicity drug. Tetracyclic triterpene dammarane compounds as NMDAR antagonists will be an effective way to treat neurological diseases (Lee et al., 2006; Ryoo et al., 2020). Studies believe that ginkgolides can improve cerebral blood circulation and energy metabolism through specific inhibition of PAFR, and show a good market prospect in the prevention and treatment of cardiovascular and cerebrovascular diseases. The main differences in their effects are: the number and positions of hydroxyl groups contained in ginkgolides are different, PAF and ginkgolide ligands can be combined, and the introduction of benzyl derivatives at position C-10 is more beneficial for antagonistic activity; In addition, the presence of C, D ring and tert-butyl group also plays an important role in activity (Hui et al., 2013). Some scholars have studied the structure-activity relationship of andrographolide and found that the position of the double bond determines the anti-inflammatory effect of andrographolide and its derivatives, and the anti-inflammatory effects of compounds with double bonds in the ring are stronger than those with double bonds outside the ring (Tian et al., 2015). As a natural component of polycarbonyl and polyhydroxyl groups, emodin can coordinate and chelate with the zinc ions of type IV collagenase to inactivate type IV collagenase, thereby preventing type IV collagenase (MMP-2, MMP- 9) degradation of basement membrane and extracellular matrix of normal tissue cells, play a role in anti-invasion and anti-metastasis (Wang, 2006). As a nucleoside antibiotic, cordycepin has a good application prospect in regulating immunity and scavenging free radicals (Lee et al., 2020).
In summary, the activity of natural products has an important relationship with the structure of the substance itself. The active functional groups of natural products are the key to their functions. Moreover, the bioavailability of many natural products is very low, which limits their use, the structural modifications, changes in dosage forms or improvements in pharmacokinetic parameters can improve their bioavailability. Because the pathogenesis of cerebral ischemia is complex, and cascade reactions occur cross-over. The anti-cerebral ischemia effects of individual natural products are often weak. Therefore, a full understanding of the mechanism of the cerebral ischemia cascade can provide the possibility for the development of specific mechanisms or targeted compounds, and the combined use of different natural products to play a multi-target neuroprotective effect may be a promising therapy. However, it is very important to combine animal models with clinical practice (Ma et al., 2020). Choose appropriate experimental models according to actual conditions to provide a solid foundation for the clinical transformation of natural products.
Conclusions and Outlook
Stroke is one of the most common causes of disability and death worldwide, seriously endangers human health and brings heavy burden to society and family. Till now, there is no effective therapy which is available for the treatment of cerebral ischemia. Recombinant plasminogen activator is the only medicament utilized clinically and its use is restricted due to short therapy time windows and the risk of bleeding. Natural products from plants have been used to treat the cure of numerous disorders through the previous practice of therapeutics (Fan et al., 2020; Li Y. et al., 2017; Savopoulos et al., 2009; Chen, S. et al., 2020). Nowadays, many attempts have been made to explore the neuroprotective effects of natural products with the advance of technology. Hence, this review summarizes recent studies on the biological activities and mechanisms of recognized compounds for cerebral ischemia injury prevention or/and treatment (Table 1). Through the literatures, three aspects should be noted with special focus. First, multiple types of natural products, including flavonoids, alkaloids, saponin, terpene, iridoid glycosides, polyphenols and others, are proven to have a definite effect on cerebral ischemia injury. In fact, the clinical efficacy still requires further confirmation and to study. Second, animal models and cell experiments were used to investigate the neuroprotective effect of natural product on cerebral ischemia injury. Animal models including MCAO, BCCAO and other occlusion methods were used to induce cerebral ischemia injury. Due to the diversity of brain cells, a variety of cell lines are used in the study of neurological diseases, for example, HT22, BV2, BMEC and others. Due to the limitations of cell models, the neuroprotective effects of many natural products in cell models still need to be verified by in vivo experiments. Third, most of the compounds show neuroprotective effects through multiple targets or signaling pathways. These natural products work by comprehensive regulation, inhibiting excitotoxicity, influencing free radicals, anti-apoptosis, impairing blood-brain barrier disruption, anti-inflammation, influencing astrocytic activation and proliferation. The mechanism by which natural products has multiple targets and diverse signaling pathways. Therefore, natural products would be very valuable when seeking novel therapeutic agents for stroke.
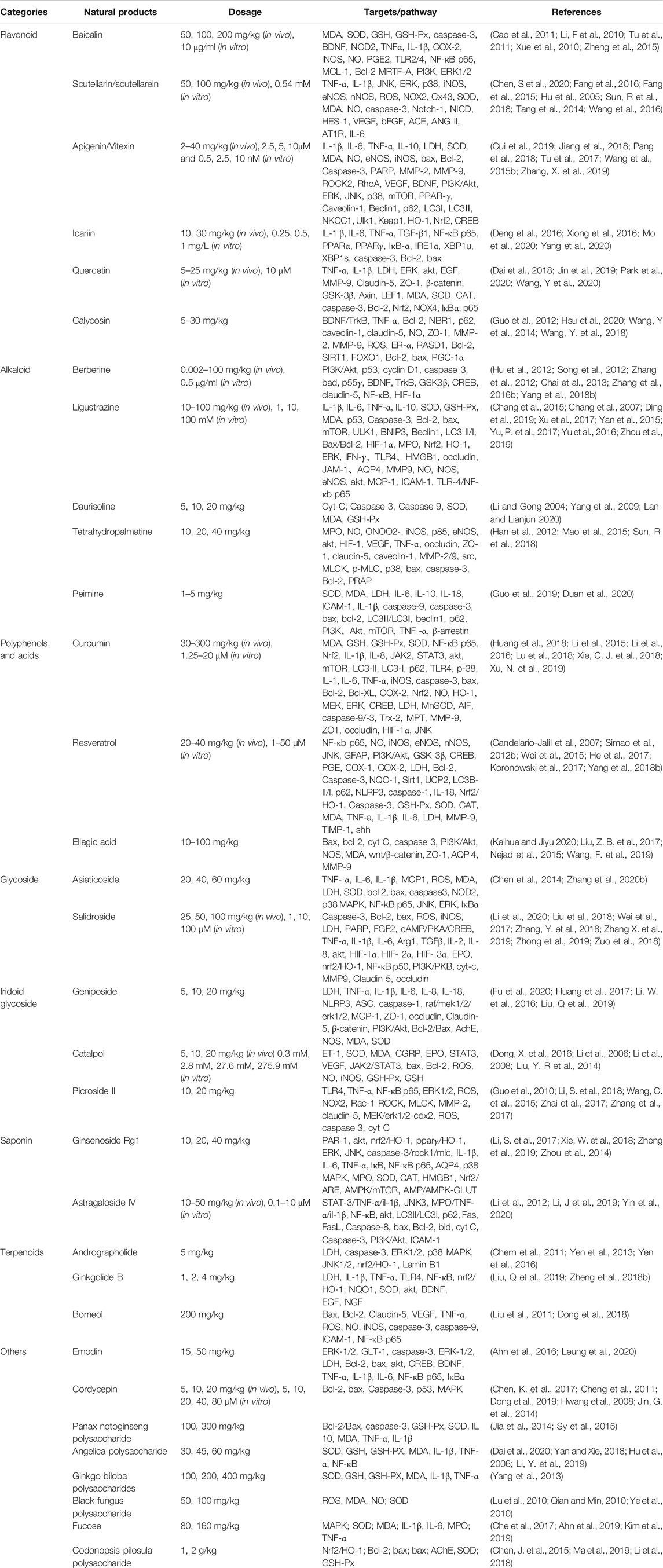
TABLE 1. Summary a few natural products and their targets of action imparting neuroprotective activity.
In conclusion, this review summarizes that the facts are comprehensive and deeply informative about the neuroprotective activities of natural products in vitro and in vivo experiment. For prophylactic and therapeutic management of stroke, they are promising candidates. Therefore, this review would provide as a reference for current advances in the study on natural products for neuroprotection.
Author Contributions
QX and HL are equally contributed to the article and supervised as a whole and finalized the manuscript. DL, JY, and DG carried out various literature survey studies. RM, JL, and JW prepared the initial draft and graphical representation for figures. MR, HC and YL provided significant input into the chemistry part.
Funding
The authors are grateful to National Natural Science Foundation of China (Grant Numbers 81873023, 81473371, 81873073), and Hundred Talents Program of the Hospital of Chengdu University of Traditional Chinese Medicine (Grant No. 20-Q18).
Conflict of Interest
The authors declare that the research was conducted in the absence of any commercial or financial relationships that could be construed as a potential conflict of interest.
Acknowledgments
The authors are grateful to National Natural Science Foundation of China (Grant Numbers: 81873023, 81473371, 81873073), and Hundred Talents Program of the Hospital of Chengdu University of Traditional Chinese Medicine (Grant No. 20-Q18).
Abbreviations
t-PA, tissue plasminogen activator; BBB, blood-brain barrier; NVU, neurovascular unit; CNKI, China National Knowledge Internet; FDA Food and Drug Administration; Mcl 1, myeloid cell leukemia 1; Bcl 2, B-cell lymphoma-2; SOD, superoxide dismutase, MDA, malondialdehyde; MCAO, middle cerebral artery occlusion; NF-κB, nuclear factor kappa B; TLR2/4, toll like receptor 2/4; NOD2, nucleotide-binding oligomerization domain protein 2; TNF α, tumor necrosis factor α; MAPK, mitogen-activated protein kinase; p-ERK1/2, phosphorylation extracellular regulated kinase 1/2; VEGF, vascular endothelial growth factor; bFGF, basic fibroblast growth factor, NOX2, nicotinamide adenine dinucleotide phosphate oxidase 2; BCCAO, bilateral common carotid arteries; iNOS, inducible nitric oxide synthase; eNOS, endothelial nitric oxide synthase; mTOR, mechanistic target of rapamycin; p-JNK, phosphorylation c-Jun N-terminal kinase; ACE, angiotensin-converting enzyme; AT1R, Ang II type 1 receptor, TNF-α, tumor necrosis factor -α; IL-6, interleukin-6; IL-1β, interleukin-1β; PARP, poly (ADPribose) polymerase; NAD, nicotinamide adenine dinucleotide; OGD, oxygen–glucose deprivation; I/R, ischemic reperfusion; HBMVECs, human brain microvessel endothelial cells; IκB, inhibitory κB; PPARα, peroxisome proliferator-activated receptor α; PPARγ, peroxisome proliferatoractivated receptor γ; IRE1, inositol requiring enzyme 1; XBP1 X-Box Binding Protein 1; BDNF, brain-derived neurotrophic factor; RASD1, dexamethasone-induced Ras-related protein 1; ER, estrogen receptor; p Akt, phospho-Akt; GSK 3β, glycogensynthase kinase 3-β; CREB, cAMP response element binding protein; HIF-1α, hypoxia inducible factor-1-alpha; GSH-Px, glutathione peroxidase; TJ, tight junction; MMP 9, matrix metalloprotein 9; AQP4, aquaporin 4; Cyt-C, cytochrome C; Sirt 1, silent information regulator 1; HI, hypoxic-ischemia; PAR 1, protease-activated receptor-1; SNpc, substantia nigra pars compacta; PGE2, prostaglandin E2; COX-2, cyclooxygenase; GSH, glutathione; STAT, signal transducer and activator of transcription; Nrf2, nuclear factor erythroid 2-related factor 2; HO-1, heme oxygenase-1; ET-1, endothelin-1; mPTP, mitochondrial permeability transition pore; PAR 1, protease-activated receptor-1; CECs, cerebral endothelial cells; GLT-l, glutamate transporter-1; ERK, extracellular signal-regulated kinase.
References
Ahn, J. H., Shin, M. C., Kim, D. W., Kim, H., Song, M., Lee, T. K., et al. (2019). Antioxidant properties of fucoidan alleviate acceleration and exacerbation of hippocampal neuronal death following transient global cerebral ischemia in high-fat diet-induced obese gerbils. Int. J. Mol. Sci. 20, 114. doi:10.3390/ijms20030554
Ahn, S. M., Kim, H. N., Kim, Y. R., Choi, Y. W., Kim, C. M., Shin, H. K., et al. (2016). Emodin from polygonum multiflorum ameliorates oxidative toxicity in HT22 cells and deficits in photothrombotic ischemia. J. Ethnopharmacology 188, 13–20. doi:10.1016/j.jep.2016.04.058
Alfei, S., Turrini, F., Catena, S., Zunin, P., Grilli, M., Pittaluga, A. M., et al. (2019). Ellagic acid a multi-target bioactive compound for drug discovery in CNS? A narrative review. Eur. J. Med. Chem. 183, 111724. doi:10.1016/j.ejmech.2019.111724
Aljahdali, A. Z., Foster, K. A., and O'Doherty, G. A. (2020). Synthesis and biological study of the phomopsolide and phomopsolidone natural products. Chem. Commun. 56, 12885–12896. doi:10.1039/d0cc04069j
Almeida, J. R., Souza, G. R., Silva, J. C., Saraiva, S. R., Júnior, R. G., Quintans, Jde. S., et al. (2013). Borneol, a bicyclic monoterpene alcohol, reduces nociceptive behavior and inflammatory response in mice. Sci. World J. 2013, 808460. doi:10.1155/2013/808460
An, J., Chen, B., Kang, X., Zhang, R., Guo, Y., Zhao, J., et al. (2020). Neuroprotective effects of natural compounds on LPS-induced inflammatory responses in microglia. Am. J. Transl. Res. 12, 2353–2378.
Apostolova, E., Lukova, P., Baldzhieva, A., Katsarov, P., Nikolova, M., Iliev, I., et al. (2020). Immunomodulatory and anti-inflammatory effects of fucoidan: a review. Polymers (Basel) 12. 2338. doi:10.3390/polym12102338
Asemi, Z., Behnam, M., Pourattar, M. A., Mirzaei, H., Razavi, Z. S., and Tamtaji, O. R. (2020). Therapeutic potential of berberine in the treatment of glioma: insights into its regulatory mechanisms. Cell Mol. Neurobiol. 11, 23. doi:10.1007/s10571-020-00903-5
Ayoobi, F., Shamsizadeh, A., Fatemi, I., Vakilian, A., Allahtavakoli, M., Hassanshahi, G., et al. (2017). Bio-effectiveness of the main flavonoids of Achillea millefolium in the pathophysiology of neurodegenerative disorders- a review. Iran J. Basic Med. Sci. 20, 604–612. doi:10.22038/IJBMS.2017.8827
Babaei, F., Moafizad, A., Darvishvand, Z., Mirzababaei, M., Hosseinzadeh, H., and Nassiri-Asl, M. (2020). Review of the effects of vitexin in oxidative stress-related diseases. Food Sci. Nutr. 8, 2569–2580. doi:10.1002/fsn3.1567
Bagli, E., Goussia, A., Moschos, M. M., Agnantis, N., and Kitsos, G. (2016). Natural compounds and neuroprotection: mechanisms of action and novel delivery systems. In Vivo 30, 535–547. doi:10.1002/9780470134931.ch3
Bao, C., Wang, Y., Min, H., Zhang, M., Du, X., Han, R., et al. (2015). Combination of ginsenoside Rg1 and bone marrow mesenchymal stem cell transplantation in the treatment of cerebral ischemia reperfusion injury in rats. Cell Physiol. Biochem. 37, 901–910. doi:10.1159/000430217
Bao, Z., Guan, S., Cheng, C., Wu, S., Wong, S. H., Kemeny, D. M., et al. (2009). A novel antiinflammatory role for andrographolide in asthma via inhibition of the nuclear factor-κb pathway. Am. J. Respir. Crit. Care Med. 179, 657–665. doi:10.1164/rccm.200809-1516oc
Baradaran Rahimi, V., Ghadiri, M., Ramezani, M., and Askari, V. R. (2020). Antiinflammatory and anti-cancer activities of pomegranate and its constituent, ellagic acid: evidence from cellular, animal, and clinical studies. Phytotherapy Res. 34, 685–720. doi:10.1002/ptr.6565
Benjamin, E. J., Virani, S. S., Callaway, C. W., Chamberlain, A. M., Chang, A. R., Cheng, S., et al. (2018). Heart disease and stroke statistics-2018 update: a report from the American heart association. Circulation 137, e67–e492. doi:10.1161/CIR.0000000000000558
Bialecka-Florjanczyk, E., Fabiszewska, A., and Zieniuk, B. (2018). Phenolic acids derivatives - biotechnological methods of synthesis and bioactivity. Curr. Pharm. Biotechnol. 19, 1098–1113. doi:10.2174/1389201020666181217142051
Brito-Arias, M. (2007). Synthesis and characterization of glycosides. Berlin, Germany: Springer Science+Business Media.
Cai, D., Qi, J., Yang, Y., Zhang, W., Zhou, F., Jia, X., et al. (2019). Design, synthesis and biological evaluation of diosgenin-amino acid derivatives with dual functions of neuroprotection and angiogenesis. Molecules 24, 113. doi:10.3390/molecules24224025
Candelario-Jalil, E., de Oliveira, A., Gräf, S., Bhatia, H. S., Hüll, M., Muñoz, E., et al. (2007). Resveratrol potently reduces prostaglandin E2 production and free radical formation in lipopolysaccharide-activated primary rat microglia. J. Neuroinflammation 4, 25. doi:10.1186/1742-2094-4-25
Cao, W., Liu, W., Wu, T., Zhong, D., and Liu, G. (2008). Dengzhanhua preparations for acute cerebral infarction. Cochrane Database Syst. Rev. 11, CD005568. doi:10.1002/14651858.CD005568.pub2
Cao, Y., Liu, J. W., Yu, Y. J., Zheng, P. Y., Zhang, X. D., Li, T., et al. (2007). Synergistic protective effect of picroside II and NGF on PC12 cells against oxidative stress induced by H2O2. Pharmacol. Rep. 59, 573–579. doi:10.7324/japs.2019.90403
Cao, Y., Mao, X., Sun, C., Zheng, P., Gao, J., Wang, X., et al. (2011). Baicalin attenuates global cerebral ischemia/reperfusion injury in gerbils via anti-oxidative and anti-apoptotic pathways. Brain Res. Bull. 85, 396–402. doi:10.1016/j.brainresbull.2011.05.002
Ceci, C., Lacal, P. M., Tentori, L., De Martino, M. G., Miano, R., and Graziani, G. (2018). Experimental evidence of the antitumor, antimetastatic and antiangiogenic activity of ellagic acid. Nutrients 10, 1756. doi:10.3390/nu10111756
Chai, Y.-S., Hu, J., Lei, F., Wang, Y.-G., Yuan, Z.-Y., Lu, X., et al. (2013). Effect of berberine on cell cycle arrest and cell survival during cerebral ischemia and reperfusion and correlations with p53/cyclin D1 and PI3K/Akt. Eur. J. Pharmacol. 708, 44–55. doi:10.1016/j.ejphar.2013.02.041
Chang, C.-K., and Lin, M.-T. (2001). DL-Tetrahydropalmatine may act through inhibition of amygdaloid release of dopamine to inhibit an epileptic attack in rats. Neurosci. Lett. 307, 163–166. doi:10.1016/s0304-3940(01)01962-0
Chang, C.-Y., Kao, T.-K., Chen, W.-Y., Ou, Y.-C., Li, J.-R., Liao, S.-L., et al. (2015). Tetramethylpyrazine inhibits neutrophil activation following permanent cerebral ischemia in rats. Biochem. Biophys. Res. Commun. 463, 421–427. doi:10.1016/j.bbrc.2015.05.088
Chang, Y., Hsiao, G., Chen, S.-h., Chen, Y.-c., Lin, J.-h., Lin, K.-h., et al. (2007). Tetramethylpyrazine suppresses HIF-1?, TNF-?, and activated caspase-3 expression in middle cerebral artery occlusion-induced brain ischemia in rats. Acta Pharmacologica Sinica 28, 327–333. doi:10.1111/j.1745-7254.2007.00514.x
Che, D. N., Cho, B. O., Kim, J. S., Shin, J. Y., Kang, H. J., and Jang, S. I. (2020). Luteolin and apigenin attenuate LPS-induced astrocyte activation and cytokine production by targeting MAPK, STAT3, and NF-kappaB signaling pathways. Inflammation 43, 1716–1728. doi:10.1007/s10753-020-01245-6
Che, N., Ma, Y., and Xin, Y. (2017). Protective role of fucoidan in cerebral ischemia-reperfusion injury through inhibition of MAPK signaling pathway. Biomolecules Ther. 25, 272–278. doi:10.4062/biomolther.2016.098
Chen, C., Liu, X.-P., Jiang, W., Zeng, B., Meng, W., Huang, L.-P., et al. (2017). Anti-effects of cordycepin to hypoxia-induced membrane depolarization on hippocampal CA1 pyramidal neuron. Eur. J. Pharmacol. 796, 1–6. doi:10.1016/j.ejphar.2016.12.021
Chen, H.-L., Jia, W.-J., Li, H.-E., Han, H., Li, F., Zhang, X.-L. -N., et al. (2020). Scutellarin exerts anti-inflammatory effects in activated microglia/brain macrophage in cerebral ischemia and in activated BV-2 microglia through regulation of MAPKs signaling pathway. Neuromol Med. 22, 264–277. doi:10.1007/s12017-019-08582-2
Chen, J., Lin, C., Yong, W., Ye, Y., and Huang, Z. (2015). Calycosin and genistein induce apoptosis by inactivation of HOTAIR/p-Akt signaling pathway in human breast cancer MCF-7 cells. Cel Physiol. Biochem. 35, 722–728. doi:10.1159/000369732
Chen, K., Sun, W., Jiang, Y., Chen, B., Zhao, Y., Sun, J., et al. (2017). Ginkgolide B suppresses TLR4-mediated inflammatory response by inhibiting the phosphorylation of JAK2/STAT3 and p38 MAPK in high glucose-treated HUVECs. Oxid Med. Cel Longev 2017, 9371602. doi:10.1155/2017/9371602
Chen, S., Yin, Z.-J., Jiang, C., Ma, Z.-Q., Fu, Q., Qu, R., et al. (2014). Asiaticoside attenuates memory impairment induced by transient cerebral ischemia-reperfusion in mice through anti-inflammatory mechanism. Pharmacol. Biochem. Behav. 122, 7–15. doi:10.1016/j.pbb.2014.03.004
Chen, W., Tang, Y., Liu, L., and Jiao, Y. (2015). Influence of Codonopsis pilosula polysaccharide on brain Tissues,Oxygen free radicals and beclin-1 level of rats with ischemia-reperfusion. Mil. Med. J. South China 29, 886–888+923. doi:10.1016/j.carbpol.2012.07.062
Chen, Y.-J., Liu, Y.-L., Zhong, Q., Yu, Y.-F., Su, H.-L., Toque, H. A., et al. (2012). Tetrahydropalmatine protects against methamphetamine-induced spatial learning and memory impairment in mice. Neurosci. Bull. 28, 222–232. doi:10.1007/s12264-012-1236-4
Chen, Y., Long, X., Pan, S., An, X., and Chen, S. L. (2013). Advances in pharmacodynamic mechanisms and SAR studies of flavonoids. Chin. J. Exp. Trad. Med. Formulae 19, 337–344. doi:10.1201/9781420041781.ch29
Cheng, Y., Shen, L.-h., and Zhang, J.-t. (2005). Anti-amnestic and anti-aging effects of ginsenoside Rg1 and Rb1 and its mechanism of action. Acta Pharmacologica Sinica 26, 143–149. doi:10.1111/j.1745-7254.2005.00034.x
Cheng, Z., He, W., Zhou, X., Lv, Q., Xu, X., Yang, S., et al. (2011). Cordycepin protects against cerebral ischemia/reperfusion injury in vivo and in vitro. Eur. J. Pharmacol. 664, 20–28. doi:10.1016/j.ejphar.2011.04.052
ChenS., , Chen, H., Du, Q., and Shen, J. (2020). Targeting myeloperoxidase (MPO) mediated oxidative stress and inflammation for reducing brain ischemia injury: potential application of natural compounds. Front. Physiol. 11 (undefined), 433. doi:10.3389/fphys.2020.00433
Chern, C.-M., Liou, K.-T., Wang, Y.-H., Liao, J.-F., Yen, J.-C., and Shen, Y.-C. (2011). Andrographolide inhibits PI3K/AKT-dependent NOX2 and iNOS expression protecting mice against hypoxia/ischemia-induced oxidative brain injury. Planta Med. 77, 1669–1679. doi:10.1055/s-0030-1271019
Chu, H., Jin, G., Friedman, E., and Zhen, X. (2008). Recent development in studies of tetrahydroprotoberberines: mechanism in antinociception and drug addiction. Cel Mol Neurobiol 28, 491–499. doi:10.1007/s10571-007-9179-4
Chu, S.-f., Zhang, Z., Zhou, X., He, W.-b., Chen, C., Luo, P., et al. (2019). Ginsenoside Rg1 protects against ischemic/reperfusion-induced neuronal injury through miR-144/Nrf2/ARE pathway. Acta Pharmacol. Sin 40, 13–25. doi:10.1038/s41401-018-0154-z
Chuang, J.-H., Tung, L. C., and Lin, Y. (2015). Neural differentiation from embryonic stem cellsin vitro: an overview of the signaling pathways. Wjsc 7, 437–447. doi:10.4252/wjsc.v7.i2.437
Coon, J. T., and Ernst, E. (2004). Andrographis paniculata in the treatment of upper respiratory tract infections: a systematic review of safety and efficacy. Planta Med. 70, 293–298. doi:10.1055/s-2004-818938
Costa, I. M., Vieira Lima, F., Fernandes, L., Norrara, B., Neta, F., Alves, R., et al. (2018). Astragaloside IV supplementation promotes A neuroprotective effect in experimental models of neurological disorders: a systematic review. Curr. Neuropharmacol. 17, 648–665. doi:10.2174/1570159X16666180911123341
Costa, L. G., Garrick, J. M., Roquè, P. J., and Pellacani, C. (2016). Mechanisms of neuroprotection by quercetin: counteracting oxidative stress and more. Oxid Med. Cel Longev 11, 2986796. doi:10.1155/2016/2986796
Cui, Y.-h., Zhang, X.-q., Wang, N.-d., Zheng, M.-d., and Yan, J. (2019). Vitexin protects against ischemia/reperfusion-induced brain endothelial permeability. Eur. J. Pharmacol. 853, 210–219. doi:10.1016/j.ejphar.2019.03.015
Dai, Y., Zhang, H., Zhang, J., and Yan, M. (2018). Isoquercetin attenuates oxidative stress and neuronal apoptosis after ischemia/reperfusion injury via Nrf2-mediated inhibition of the NOX4/ROS/NF-κB pathway. Chemico-Biol. Interact. 284, 32–40. doi:10.1016/j.cbi.2018.02.017
Dai, Z., Zhu, Y., and Wang, Z. (2020). Effects of Angelica polysaccharides on the expression of IL-1β, TNF-α, NF-κB in rats with cerebral ischemia reperfusion injury. Chin. Trad. Patent Med. 42, 2176–2178. doi:10.1016/b978-0-12-374530-9.00013-9
de Oliveira, M. R. (2016). The effects of ellagic acid upon brain cells: a mechanistic view and future directions. Neurochem. Res. 41, 1219–1228. doi:10.1007/s11064-016-1853-9
Della-Morte, D., Dave, K. R., DeFazio, R. A., Bao, Y. C., Raval, A. P., and Perez-Pinzon, M. A. (2009). Resveratrol pretreatment protects rat brain from cerebral ischemic damage via a sirtuin 1-uncoupling protein 2 pathway. Neuroscience 159, 993–1002. doi:10.1016/j.neuroscience.2009.01.017
Deng, Y., Xiong, D., Yin, C., Liu, B., Shi, J., and Gong, Q. (2016). Icariside II protects against cerebral ischemia-reperfusion injury in rats via nuclear factor-κB inhibition and peroxisome proliferator-activated receptor up-regulation. Neurochem. Int. 96, 56–61. doi:10.1016/j.neuint.2016.02.015
Dhanasekaran, M., Holcomb, L. A., Hitt, A. R., Tharakan, B., Porter, J. W., Young, K. A., et al. (2009). Centella asiatica extract selectively decreases amyloid β levels in hippocampus of Alzheimer’s disease animal model. Phytother. Res. 23, 14–19. doi:10.1002/ptr.2405
Ding, Y., Du, J., Cui, F., Chen, L., and Li, K. (2019). The protective effect of ligustrazine on rats with cerebral ischemia-reperfusion injury via activating PI3K/Akt pathway. Hum. Exp. Toxicol. 38, 1168–1177. doi:10.1177/0960327119851260
Dong, T., Chen, N., Ma, X., Wang, J., Wen, J., Xie, Q., et al. (2018). The protective roles of L- borneolum, D- borneolum and synthetic borneol in cerebral ischaemia via modulation of the neurovascular unit. Biomed. Pharmacother. 102, 874–883. doi:10.1016/j.biopha.2018.03.087
Dong, W., Xian, Y., Yuan, W., Huifeng, Z., Tao, W., Zhiqiang, L., et al. (2016). Catalpol stimulates VEGF production via the JAK2/STAT3 pathway to improve angiogenesis in rats' stroke model. J. Ethnopharmacology 191, 169–179. doi:10.1016/j.jep.2016.06.030
Dong, X., Fu, J., Yin, X., Cao, S., Li, X., Lin, L., et al. (2016). Emodin: a review of its pharmacology, toxicity and pharmacokinetics. Phytother. Res. 30, 1207–1218. doi:10.1002/ptr.5631
Dong, Z.-S. -W., Cao, Z.-P., Shang, Y.-J., Liu, Q.-Y., Wu, B.-Y., Liu, W.-X., et al. (2019). Neuroprotection of cordycepin in NMDA-induced excitotoxicity by modulating adenosine A1 receptors. Eur. J. Pharmacol. 853, 325–335. doi:10.1016/j.ejphar.2019.04.015
Donnan, G. A., Fisher, M., Macleod, M., and Davis, S. M. (2008). Stroke. The Lancet 371, 1612–1623. doi:10.1016/s0140-6736(08)60694-7
Duan, J., Kang, K., Huang, C.-r., Chen, L.-g., Wan, W.-f., Peng, T.-m., et al. (2020). Protective effect of peimine on cerebral ischemia-reperfusion injury in rats. Chin. J. New Drugs Clin. Rem 39, 240–246. doi:10.3724/sp.j.1008.2010.00238
Eghbaliferiz, S., Farhadi, F., Barreto, G. E., Majeed, M., and Sahebkar, A. (2020). Effects of curcumin on neurological diseases: focus on astrocytes. Pharmacol. Rep. 72, 769–782. doi:10.1007/s43440-020-00112-3
Fan, D., Liu, L., Wu, Z., and Cao, M. (2019). Combating neurodegenerative diseases with the plant alkaloid berberine: molecular mechanisms and therapeutic potential. Cn 17, 563–579. doi:10.2174/1570159x16666180419141613
Fan, Q., Zhou, J., Wang, Y., Xi, T., Ma, H., Wang, Z., et al. (2020). Chip-based serum proteomics approach to reveal the potential protein markers in the sub-acute stroke patients receiving the treatment of Ginkgo Diterpene Lactone Meglumine Injection. J. Ethnopharmacol. 260, 112964. doi:10.1016/j.jep.2020.112964
Fang, J., Wang, Z., Wang, P., and Wang, M. (2020). Extraction, structure and bioactivities of the polysaccharides from Ginkgo biloba: a review. Int. J. Biol. Macromol. 162, 1897–1905. doi:10.1016/j.ijbiomac.2020.08.141
Fang, M., Yuan, Y., Lu, J., Li, H. E., Zhao, M., Ling, E.-A., et al. (2016). Scutellarin promotes microglia-mediated astrogliosis coupled with improved behavioral function in cerebral ischemia. Neurochem. Int. 97, 154–171. doi:10.1016/j.neuint.2016.04.007
Fang, M., Yuan, Y., Rangarajan, P., Lu, J., Wu, Y., Wang, H., et al. (2015). Scutellarin regulates microglia-mediated TNC1 astrocytic reaction and astrogliosis in cerebral ischemia in the adult rats. BMC Neurosci. 16, 84. doi:10.1186/s12868-015-0219-6
Fei, F., Su, N., Li, X., and Fei, Z. (2020). Neuroprotection mediated by natural products and their chemical derivatives. Neural Regen. Res. 15, 2008–2015. doi:10.4103/1673-5374.282240
Forouzanfar, F., Read, M. I., Barreto, G. E., and Sahebkar, A. (2020). Neuroprotective effects of curcumin through autophagy modulation. IUBMB Life 72, 652–664. doi:10.1002/iub.2209
Fu, C., Zhang, X., Lu, Y., Wang, F., Xu, Z., Liu, S., et al. (2020). Geniposide inhibits NLRP3 inflammasome activation via autophagy in BV-2 microglial cells exposed to oxygen-glucose deprivation/reoxygenation. Int. Immunopharmacol. 84, 106547. doi:10.1016/j.intimp.2020.106547
Group T. N. I. O. N. D. A. S. r.-P. S. S. ( 1995). Tissue plasminogen activator for acute ischemic stroke. N. Engl. J. Med., 333, 1581–1587.doi:10.1056/NEJM199512143332401
Gao, J., Chen, G., He, H., Liu, C., Xiong, X., Li, J., et al. (2017). Therapeutic effects of breviscapine in cardiovascular diseases: a review. Front. Pharmacol. 8, 289. doi:10.3389/fphar.2017.00289
Gao, R., Zhao, X., Zheng, Y., and Liu, C. (2008). Study on immune function of soy isoflavone glycoside and aglycon. Cereals Oils, 14, 43–44. doi:10.1007/springerreference_135032
Gao, Y., Fu, R., Wang, J., Yang, X., Wen, L., and Feng, J. (2018). Resveratrol mitigates the oxidative stress mediated by hypoxic-ischemic brain injury in neonatal rats via Nrf2/HO-1 pathway. Pharm. Biol. 56, 440–449. doi:10.1080/13880209.2018.1502326
Ge, J. B., Lu, H. J., Song, X. J., Li, M., Chen, D. D., and Wu, F. (2017). [Protective effects of LBP on cerebral ischemia reperfusion injury in mice and mechanism of inhibiting NF-κB, TNF-α, IL-6 and IL-1β]. Zhongguo Zhong Yao Za Zhi 42, 326–331. doi:10.19540/j.cnki.cjcmm.20161222.016
Gu, J.-W., Hasuo, H., Takeya, M., and Akasu, T. (2005). Effects of emodin on synaptic transmission in rat hippocampal CA1 pyramidal neurons in vitro. Neuropharmacology 49, 103–111. doi:10.1016/j.neuropharm.2005.02.003
Gu, J., Zhang, X., Fei, Z., Wen, A., Qin, S., and Yi, S. (2000). [Rhubarb Extracts in Treating Complications of Severe Cerebral Injury]. Chin. Med. J. 113 (6), 529–531.
Guo, C., Tong, L., Xi, M., Yang, H., Dong, H., and Wen, A. (2012). Neuroprotective effect of calycosin on cerebral ischemia and reperfusion injury in rats. J. Ethnopharmacology 144, 768–774. doi:10.1016/j.jep.2012.09.056
Guo, S., Li, J., and Xiao, S. (2019). Effects of peimine a on inflammatory response and autophagy in mice with cerebral ischemia-reperfusion injury. Chin. J. Gerontol. 39, 5347–5350. doi:10.5353/th_b5328058
Guo, Y., Xu, X., Li, Q., Li, Z., and Du, F. (2010). Anti-inflammation effects of picroside 2 in cerebral ischemic injury rats. Behav. Brain Functions 6, 43. doi:10.1186/1744-9081-6-43
Guo, Z.-H., Li, F., and Wang, W.-Z. (2009). The mechanisms of brain ischemic insult and potential protective interventions. Neurosci. Bull. 25 (3), 139–152. doi:10.1007/s12264-009-0104-3
Hacke, W., Kaste, M., Bluhmki, E., Brozman, M., Dávalos, A., Guidetti, D., et al. (2008). Thrombolysis with alteplase 3 to 4.5 hours after acute ischemic stroke. N. Engl. J. Med. 359, 1317–1329. doi:10.1056/nejmoa0804656
Han, Y., Liu, H., Li, K., Wang, T., and Ge, X. (2020). Research progress on antifungal activity of berberine and synthesis of its molecular probes. Chem. World 61, 83–91. doi:10.31830/2348-7542.2019.107
Han, Y., Zhang, W., Tang, Y., Bai, W., Yang, F., Xie, L., et al. (2012). l-Tetrahydropalmatine, an active component of Corydalis yanhusuo W.T. Wang, protects against myocardial ischaemia-reperfusion injury in rats. PLoS One 7, e38627. doi:10.1371/journal.pone.0038627
He, L. J., Liang, M., Hou, F. F., Guo, Z. J., Xie, D., and Zhang, X. (2009). Ethanol extraction of Picrorhiza scrophulariiflora prevents renal injury in experimental diabetes via anti-inflammation action. J. Endocrinol. 200, 347–355. doi:10.1677/joe-08-0481
He, Q., Li, Z., Wang, Y., Hou, Y., Li, L., and Zhao, J. (2017). Resveratrol alleviates cerebral ischemia/reperfusion injury in rats by inhibiting NLRP3 inflammasome activation through Sirt1-dependent autophagy induction. Int. Immunopharmacol. 50, 208–215. doi:10.1016/j.intimp.2017.06.029
Hermann, D. M., Popa-Wagner, A., Kleinschnitz, C., and Doeppner, T. R. (2019). Animal models of ischemic stroke and their impact on drug discovery. Expert Opin. Drug Discov. 14, 315–326. doi:10.1080/17460441.2019.1573984
Hou, Y., Wang, J., and Feng, J. (2019). The neuroprotective effects of curcumin are associated with the regulation of the reciprocal function between autophagy and HIF-1α in cerebral ischemia-reperfusion injury. Dddt 13, 1135–1144. doi:10.2147/dddt.s194182
Hsu, C.-C., Kuo, T.-W., Liu, W.-P., Chang, C.-P., and Lin, H.-J. (2020). Calycosin preserves BDNF/TrkB signaling and reduces post-stroke neurological injury after cerebral ischemia by reducing accumulation of hypertrophic and TNF-α-containing microglia in rats. J. Neuroimmune Pharmacol. 15, 326–339. doi:10.1007/s11481-019-09903-9
Hu, J., Chai, Y., Wang, Y., Kheir, M. M., Li, H., Yuan, Z., et al. (2012). PI3K p55γ promoter activity enhancement is involved in the anti-apoptotic effect of berberine against cerebral ischemia-reperfusion. Eur. J. Pharmacol. 674, 132–142. doi:10.1016/j.ejphar.2011.11.014
Hu, Q., Shen, P., Bai, S., Dong, M., Liang, Z., Chen, Z., et al. (2018). Metabolite-related antidepressant action of diterpene ginkgolides in the prefrontal cortex. Ndt 14, 999–1011. doi:10.2147/ndt.s161351
Hu, X.-m., Zhou, M.-m., Hu, X.-m., and Zeng, F.-d. (2005). Neuroprotective effects of scutellarin on rat neuronal damage induced by cerebral ischemia/reperfusion. Acta Pharmacol. Sinica 26, 1454–1459. doi:10.1111/j.1745-7254.2005.00239.x
Hu, X., Liao, W., Yang, W., Jiang, C., Zhou, Q., Chen, M., et al. (2006). Effect of Angelica polysaccharide on the expression of angiopoietin after the ischemic brain injury in rats. Chin. J. Rehabil. Med. 11, 204–206+230. doi:10.26226/morressier.57d034cfd462b80292383365
Huang, B., Chen, P., Huang, L., Li, S., Zhu, R., Sheng, T., et al. (2017). Geniposide attenuates post-ischaemic neurovascular damage via GluN2A/AKT/ERK-dependent mechanism. Cel Physiol. Biochem. 43, 705–716. doi:10.1159/000480657
Huang, L., Chen, C., Zhang, X., Li, X., Chen, Z., Yang, C., et al. (2018). Neuroprotective effect of curcumin against cerebral ischemia-reperfusion via mediating autophagy and inflammation. J. Mol. Neurosci. 64, 129–139. doi:10.1007/s12031-017-1006-x
Hui, A., Wu, Z., Yuan, Y., Zhou, A., and Pan, J. (2013). Progress in synthesis and antagonistic activities towards PAFR and GlyR of ginkgolide derivatives and analogs. Chin. J. Org. Chem. 33, 1263–1272. doi:10.6023/cjoc201209028
Hwang, I., Lim, S., Yoo, K.-Y., Lee, Y., Kim, H., Kang, I.-J., et al. (2008). A Phytochemically characterized extract of Cordyceps militaris and cordycepin protect hippocampal neurons from ischemic injury in gerbils. Planta Med. 74, 114–119. doi:10.1055/s-2008-1034277
Ismailoglu, U. B., Saracoglu, I., Harput, U. S., and Sahin-Erdemli, I. (2002). Effects of phenylpropanoid and iridoid glycosides on free radical-induced impairment of endothelium-dependent relaxation in rat aortic rings. J. Ethnopharmacol. 79, 193–197. doi:10.1016/s0378-8741(01)00377-4
Jia, D., Deng, Y., Gao, J., Liu, X., Chu, J., and Shu, Y. (2014). Neuroprotective effect of Panax notoginseng plysaccharides against focal cerebral ischemia reperfusion injury in rats. Int. J. Biol. Macromol. 63, 177–180. doi:10.1016/j.ijbiomac.2013.10.034
Jiang, J., Dai, J., and Cui, H. (2018). Vitexin reverses the autophagy dysfunction to attenuate MCAO-induced cerebral ischemic stroke via mTOR/Ulk1 pathway. Biomed. Pharmacother. 99, 583–590. doi:10.1016/j.biopha.2018.01.067
Jie, L., Yu, R., Yue, C., Jing, L., Qin, X., and Qin, Y. (2020). Effects of resveratrol on microglia polarization after oxygen glucose deprivation/reoxygenation injury in vitro. Acta Anatomica Sinica 51, 320–325. doi:10.16098/j.issn.0529-1356.2020.03.002
Jin, M., Zhao, K., Huang, Q., Xu, C., and Shang, P. (2012). Isolation, structure and bioactivities of the polysaccharides from Angelica sinensis (Oliv.) Diels: a review. Carbohydr. Polym. 89, 713–722. doi:10.1016/j.carbpol.2012.04.049
Jin, Z., Ke, J., Guo, P., Wang, Y., and Wu, H. (2019). Quercetin improves blood-brain barrier dysfunction in rats with cerebral ischemia reperfusion via Wnt signaling pathway. Am. J. Transl Res. 11, 4683–4695. doi:10.1002/brb3.1911/v2/review1
JinG, , He, X. R., and Chen, L. P. (2014). The protective effect of ginko bilboa leaves injection on the brain dopamine in the rat model of cerebral ischemia/reperfusion injury. Afr. Health Sci. 14, 725–728. doi:10.4314/ahs.v14i3.31
JinM. L., , Park, S. Y., Kim, Y. H., Oh, J.-I., Lee, S. J., and Park, G. (2014). The neuroprotective effects of cordycepin inhibit glutamate-induced oxidative and ER stress-associated apoptosis in hippocampal HT22 cells. Neurotoxicology 41, 102–111. doi:10.1016/j.neuro.2014.01.005
Kaihua, Z., and Jiyu, L. (2020). Neuroprotective effect of ellagic acid on cerebral ischemia-reperfusion injury in rats based on PI3K/Akt/NOS pathway. Chin. J. Pract. Nervous Dis. 23,33–39. doi:10.1007/s12031-020-01703-8
Kalogeris, T., Baines, C. P., Krenz, M., and Korthuis, R. J. (2016). Ischemia/reperfusion. Compr. Physiol. 7, 113–170. doi:10.1002/cphy.c160006
Kang, D. W., Moon, J. Y., Choi, J. G., Kang, S. Y., Ryu, Y., Park, J. B., et al. (2016). Antinociceptive profile of levo-tetrahydropalmatine in acute and chronic pain mice models: role of spinal sigma-1 receptor. Sci. Rep. 6, 37850. doi:10.1038/srep37850
Kao, T.-K., Ou, Y.-C., Kuo, J.-S., Chen, W.-Y., Liao, S.-L., Wu, C.-W., et al. (2006). Neuroprotection by tetramethylpyrazine against ischemic brain injury in rats. Neurochem. Int. 48, 166–176. doi:10.1016/j.neuint.2005.10.008
Kim, H., Ahn, J. H., Song, M., Kim, D. W., Lee, T.-K., Lee, J.-C., et al. (2019). Pretreated fucoidan confers neuroprotection against transient global cerebral ischemic injury in the gerbil hippocampal CA1 area via reducing of glial cell activation and oxidative stress. Biomed. Pharmacother. 109, 1718–1727. doi:10.1016/j.biopha.2018.11.015
Koo, H.-J., Lim, K.-H., Jung, H.-J., and Park, E.-H. (2006). Anti-inflammatory evaluation of gardenia extract, geniposide and genipin. J. Ethnopharmacology 103, 496–500. doi:10.1016/j.jep.2005.08.011
Koronowski, K. B., Dave, K. R., Saul, I., Camarena, V., Thompson, J. W., Neumann, J. T., et al. (2015). Resveratrol preconditioning induces a novel extended window of ischemic tolerance in the mouse brain. Stroke 46, 2293–2298. doi:10.1161/strokeaha.115.009876
Koronowski, K. B., Khoury, N., Saul, I., Loris, Z. B., Cohan, C. H., Stradecki-Cohan, H. M., et al. (2017). Neuronal SIRT1 (silent information regulator 2 homologue 1) regulates glycolysis and mediates resveratrol-induced ischemic tolerance. Stroke 48, 3117–3125. doi:10.1161/strokeaha.117.018562
Kwok, S. S., Bu, Y., Lo, A. C., Chan, T. C., So, K. F., Lai, J. S., et al. (2019). A systematic review of potential therapeutic use of lycium barbarum polysaccharides in disease. Biomed. Res. Int. 2019, 4615745. doi:10.1155/2019/4615745
Lan, N., and Lianjun, G. (2020). Neuroprotective effect of daurinoline on focal cerebral ischemia in rats. China Pharmacist 23, 1029–1031. doi:10.1016/j.lfs.2003.06.042
Lee, C., Huang, K., Shaw, J., Chen, J., Kuo, W., Shen, G., et al. (2020). Cordyceps militarisTrends in the immunomodulatory effects of : total extracts, polysaccharides and cordycepin. Front. Pharmacol. 11, 575704. doi:10.3389/fphar.2020.575704
Lee, E., Kim, S., Chul Chung, K., Choo, M.-K., Kim, D.-H., Nam, G., et al. (2006). 20(S)-ginsenoside Rh2, a newly identified active ingredient of ginseng, inhibits NMDA receptors in cultured rat hippocampal neurons. Eur. J. Pharmacol. 536, 69–77. doi:10.1016/j.ejphar.2006.02.038
Lee, J.-C., Kim, J., Park, J.-K., Chung, G.-H., and Jang, Y.-S. (2003). The antioxidant, rather than prooxidant, activities of quercetin on normal cells:quercetin protects mouse thymocytes from glucose oxidase-mediated apoptosis. Exp. Cel Res. 291, 386–397. doi:10.1016/s0014-4827(03)00410-5
Lei, W., Huojun, H., Jinyang, M., Yuanxun, D., Song, H., Changtao, F., et al. (2020). Role of cell autophagy in protection of resveratrol against cerebral ischemia-reperfusion injury in rats. Chin. J. Clin. Neurosurg. 25, 303–307. doi:10.1016/j.jneuroim.2017.11.015
Leung, S. W., Lai, J. H., Wu, J. C., Tsai, Y. R., Chen, Y. H., Kang, S. J., et al. (2020). Neuroprotective effects of emodin against ischemia/reperfusion injury through activating ERK-1/2 signaling pathway. Int. J. Mol. Sci. 21, 114. doi:10.3390/ijms21082899
Li, D.-Q., Bao, Y.-M., Li, Y., Wang, C.-F., Liu, Y., and An, L.-J. (2006). Catalpol modulates the expressions of Bcl-2 and Bax and attenuates apoptosis in gerbils after ischemic injury. Brain Res. 1115, 179–185. doi:10.1016/j.brainres.2006.07.063
Li, F., Gong, Q.-H., Wu, Q., Lu, Y.-F., and Shi, J.-S. (2010). Icariin isolated from Epimedium brevicornum Maxim attenuates learning and memory deficits induced by d-galactose in rats. Pharmacol. Biochem. Behav. 96, 301–305. doi:10.1016/j.pbb.2010.05.021
Li, F., Lang, F., Zhang, H., Xu, L., Wang, Y., Zhai, C., et al. (2017). Apigenin alleviates endotoxin-induced myocardial toxicity by modulating inflammation, oxidative stress, and autophagy. Oxidative Med. Cell Longevity 2017, 1–10. doi:10.1155/2017/2302896
Li, F., Li, W., Li, X., Li, F., Zhang, L., Wang, B., et al. (2016). Geniposide attenuates inflammatory response by suppressing P2Y14 receptor and downstream ERK1/2 signaling pathway in oxygen and glucose deprivation-induced brain microvascular endothelial cells. J. Ethnopharmacology 185, 77–86. doi:10.1016/j.jep.2016.03.025
Li, H., Hu, J., Ma, L., Yuan, Z., Wang, Y., Wang, X., et al. (2010). Comprehensive study of baicalin down-regulating NOD2 receptor expression of neurons with oxygen-glucose deprivation in vitro and cerebral ischemia-reperfusion in vivo. Eur. J. Pharmacol. 649, 92–99. doi:10.1016/j.ejphar.2010.09.023
Li, H., Hung, A., Li, M., and Yang, A. W. H. (2019). Fritillariae thunbergii bulbus: traditional uses, phytochemistry, pharmacodynamics, pharmacokinetics and toxicity. Int. J. Mol. Sci. 20, 121. doi:10.3390/ijms20071667
Li, H., Yan, Z., Zhu, J., Yang, J., and He, J. (2011). Neuroprotective effects of resveratrol on ischemic injury mediated by improving brain energy metabolism and alleviating oxidative stress in rats. Neuropharmacology 60, 252–258. doi:10.1016/j.neuropharm.2010.09.005
Li, L., Li, H., and Li, M. (2015). Curcumin protects against cerebral ischemia-reperfusion injury by activating JAK2/STAT3 signaling pathway in rats. Int. J. Clin. Exp. Med. 8, 14985–14991. doi:10.1002/brb3.1911/v2/review1
Li, L., Zhou, Q. X., and Shi, J. S. (2005). Protective effects of icariin on neurons injured by cerebral ischemia/reperfusion. Chin. Med. J. 118, 1637–1643. doi:10.5353/th_b4414290
Li, L., and Zhang, H. (2020). Clinical study on Ligustrazine Hydrochloride Injection combined with ticagrelor in treatment of angina pectoris of coronary heart disease. Drugs Clinic 35, 1968–1972. doi:10.31525/ct1-nct04022031
Li, M., Liu, X., Wang, Y., and Ju, X. (2020). In vitro effects of peimine on the activity of cytochrome P450 enzymes. Xenobiotica 50, 1202–1207. doi:10.1080/00498254.2020.1761572
Li, M., Qu, Y. Z., Zhao, Z. W., Wu, S. X., Liu, Y. Y., Wei, X. Y., et al. (2012). Astragaloside IV protects against focal cerebral ischemia/reperfusion injury correlating to suppression of neutrophils adhesion-related molecules. Neurochem. Int. 60, 458–465. doi:10.1016/j.neuint.2012.01.026
Li, Q., Wang, J., Li, Y., and Xu, X. (2018). Neuroprotective effects of salidroside administration in a mouse model of Alzheimer's disease. Mol. Med. Rep. 7, 139. doi:10.3892/mmr.2018.8757
Li, S., Jiao, Y., Wang, H., Shang, Q., Lu, F., Huang, L., et al. (2017). Sodium tanshinone IIA sulfate adjunct therapy reduces high-sensitivity C-reactive protein level in coronary artery disease patients: a randomized controlled trial. Sci. Rep. 7, 17451. doi:10.1038/s41598-017-16980-4
Li, S., Liu, X., Chen, X., and Bi, L. (2020a). Research progress on anti-inflammatory effects and mechanisms of alkaloids from Chinese medical herbs. Evid. Based Complement. Alternat Med. 2020, 1303524. doi:10.1155/2020/1303524
Li, S., Lu, Y., Ding, D., Ma, Z., Xing, X., Hua, X., et al. (2020b). Fibroblast growth factor 2 contributes to the effect of salidroside on dendritic and synaptic plasticity after cerebral ischemia/reperfusion injury. Aging 12, 10951–10968. doi:10.18632/aging.103308
Li, S., Wang, T., Zhai, L., Ge, K., Zhao, J., Cong, W., et al. (2018). Picroside II exerts a neuroprotective effect by inhibiting mPTP permeability and EndoG release after cerebral ischemia/reperfusion injury in rats. J. Mol. Neurosci. 64, 144–155. doi:10.1007/s12031-017-1012-z
Li, T., Liu, J.-W., Zhang, X.-D., Guo, M.-C., and Ji, G. (2007). The neuroprotective effect of picroside II from hu-huang-lian against oxidative stress. Am. J. Chin. Med. 35, 681–691. doi:10.1142/s0192415x0700517x
Li, W., Suwanwela, N. C., and Patumraj, S. (2016). Curcumin by down-regulating NF-kB and elevating Nrf2, reduces brain edema and neurological dysfunction after cerebral I/R. Microvasc. Res. 106, 117–127. doi:10.1016/j.mvr.2015.12.008
Li, X., Ye, J., Zhao, L., Xia, P., Peng, X., and Yu, X. (2014). Effect of Gentiana officinalis from total secoiridoid glucoside on interleukin-1β (IL-1β)-induced rat chondrocytes. Chin. Pharm. J. 49, 1121–1125. doi:10.1016/j.biopha.2018.06.154
Li, Y., Guan, Y., Wang, Y., Yu, C. L., Zhai, F. G., and Guan, L. X. (2017). Neuroprotective effect of the ginsenoside Rg1 on cerebral ischemic injury in vivo and in vitro is mediated by pparγ-regulated antioxidative and anti-inflammatory pathways. Evid. Based Complement. Alternat Med. 2017, 7842082. doi:10.1155/2017/7842082
Li, Y., Bao, Y., Jiang, B., Wang, Z., Liu, Y., Zhang, C., et al. (2008). Catalpol protects primary cultured astrocytes from in vitro ischemia-induced damage. Int. J. Dev. Neurosci. 26, 309–317. doi:10.1016/j.ijdevneu.2008.01.006
Li, Y. H., and Gong, P. L. (2004). The protective effect of dauricine on cerebral ischemia in mice. Chin. Pharmacol. Bull. 20, 564–566. doi:10.4268/cjcmm20140626
Li, Y., Yang, Y., Zhao, Y., Zhang, J., Liu, B., Jiao, S., et al. (2019). Astragaloside IV reduces neuronal apoptosis and parthanatos in ischemic injury by preserving mitochondrial hexokinase-II. Free Radic. Biol. Med. 131, 251–263. doi:10.1016/j.freeradbiomed.2018.11.033
Li, Y., Yao, J., Han, C., Yang, J., Chaudhry, M., Wang, S., et al. (2016). Quercetin, inflammation and immunity. Nutrients 8, 167. doi:10.3390/nu8030167
Liang, W., Huang, X., and Chen, W. (2017). The effects of baicalin and baicalein on cerebral ischemia: a review. A&D 8, 850–867. doi:10.14336/ad.2017.0829
Liebner, S., Dijkhuizen, R. M., Reiss, Y., Plate, K. H., Agalliu, D., and Constantin, G. (2018). Plate karl H., agalliu dritan., constantin Gabriela.Functional morphology of the blood-brain barrier in health and disease. Acta Neuropathol. 135 (3), 311–336. doi:10.1007/s00401-018-1815-1
LiJ., , Lei, T., Lin, J., and Liao, W. (2019). Protective effects of Angelica polysaccharides on cerebral ischemia reperfusion injury in rats. Chin. Arch. Trad. Chin. Med. 37, 2272–2276+2317. doi:10.3724/sp.j.1008.2010.00238
LiM., , Li, J., Liu, S., and Li, W. (2018). Codonopsis pilosula polysaccharide on cerebral ischemia-reperfusion injury model mice brain protection and its effect on GFAP expression. Acta Neuropharmacologica 8, 42–43. doi:10.1016/j.carbpol.2012.07.062
Lin, B. (2011). Polyphenols and neuroprotection against ischemia and neurodegeneration. Mini Rev. Med. Chem. 11, 1222–1238. doi:10.2174/138955711804586784
Lin, X., and Zhang, N. (2018). Berberine: pathways to protect neurons. Phytotherapy Res. 32, 1501–1510. doi:10.1002/ptr.6107
Liu, F., Wang, Y., Yao, W., Xue, Y., Zhou, J., and Liu, Z. (2019). Geniposide attenuates neonatal mouse brain injury after hypoxic-ischemia involving the activation of PI3K/Akt signaling pathway. J. Chem. Neuroanat. 102, 101687. doi:10.1016/j.jchemneu.2019.101687
Liu, G., Song, J., Guo, Y., Wang, T., and Zhou, Z. (2013). Astragalus injection protects cerebral ischemic injury by inhibiting neuronal apoptosis and the expression of JNK3 after cerebral ischemia reperfusion in rats. Behav. Brain Functions 9, 36. doi:10.1186/1744-9081-9-36
Liu, G., Wu, X., and Tang, W. (2015). Study on the chemical constituents and physiological activity of Poria cocos. J. Qiqihar University(Natural Sci. Edition) 31, 50–54. doi:10.4268/cjcmm20140615
Liu, H., Bai, J., Tan, J., and Weng, X. (2014). Cerebral protective effect of astragalus polysaccharide pretreatment on focal cerebral ischemia-reperfusion injury in rats. J. Pract. Diabetology 10, 31–32. doi:10.3724/sp.j.1008.2010.00238
Liu, J.-h., Yin, F., Guo, L.-x., Deng, X.-h., and Hu, Y.-h. (2009). Neuroprotection of geniposide against hydrogen peroxide induced PC12 cells injury: involvement of PI3 kinase signal pathway. Acta Pharmacol. Sin 30, 159–165. doi:10.1038/aps.2008.25
Liu, Q., Jin, Z., Xu, Z., Yang, H., Li, L., Li, G., et al. (2019). Antioxidant effects of ginkgolides and bilobalide against cerebral ischemia injury by activating the Akt/Nrf2 pathway in vitro and in vivo. Cell Stress and Chaperones 24, 441–452. doi:10.1007/s12192-019-00977-1
Liu, R., Zhang, L., Lan, X., Li, L., Zhang, T.-T., Sun, J.-H., et al. (2011). Protection by borneol on cortical neurons against oxygen-glucose deprivation/reperfusion: involvement of anti-oxidation and anti-inflammation through nuclear transcription factor κappaB signaling pathway. Neuroscience 176, 408–419. doi:10.1016/j.neuroscience.2010.11.029
Liu, X., Wen, S., Yan, F., Liu, K., Liu, L., Wang, L., et al. (2018). Salidroside provides neuroprotection by modulating microglial polarization after cerebral ischemia. J. Neuroinflammation 15, 39. doi:10.1186/s12974-018-1081-0
Liu, Y.-l., Liang, J.-h., Yan, L.-d., Su, R.-b., Wu, C.-f., and Gong, Z.-h. (2005). Effects of l-tetrahydropalmatine on locomotor sensitization to oxycodone in mice. Acta Pharmacol. Sin 26, 533–538. doi:10.1111/j.1745-7254.2005.00101.x
Liu, Y.-r., Li, P.-w., Suo, J.-j., Sun, Y., Zhang, B.-a., Lu, H., et al. (2014). Catalpol provides protective effects against cerebral ischaemia/reperfusion injury in gerbils. J. Pharm. Pharmacol. 66, 1265–1270. doi:10.1111/jphp.12261
Liu, Y., Cui, Y., Lu, L., Gong, Y., Han, W., and Piao, G. (2020). Natural indole-containing alkaloids and their antibacterial activities. Arch. Pharm. (Weinheim) 23, e2000120. doi:10.1002/ardp.202000120
Liu, Y., Wang, J., Wang, W., Zhang, H., Zhang, X., and Han, C. (2015). The chemical constituents and pharmacological actions ofCordyceps sinensis. Evidence-Based Complement. Altern. Med. 2015, 1–12. doi:10.1155/2015/575063
LiuQ. S., , Li, S. R., Li, K., Li, X., Yin, X., and Pang, Z. (2017). Ellagic acid improves endogenous neural stem cells proliferation and neurorestoration through Wnt/beta-catenin signaling in vivo and in vitro. Mol. Nutr. Food Res. 61, 113. doi:10.1002/mnfr.201600587
LiuZ.-B., , Liu, C., Zeng, B., Huang, L.-P., and Yao, L.-H. (2017). Modulation effects of cordycepin on voltage-gated sodium channels in rat hippocampal CA1 pyramidal neurons in the presence/absence of oxygen. Neural Plasticity 2017, 1–9. doi:10.1155/2017/2459053
Long, Y., Yang, Q., Xiang, Y., Zhang, Y., Wan, J., Liu, S., et al. (2020). Nose to brain drug delivery - a promising strategy for active components from herbal medicine for treating cerebral ischemia reperfusion. Pharmacol. Res. 159, 104795. doi:10.1016/j.phrs.2020.104795
Lu, S., Sun, L., Shen, J., Su, F., Wang, H., Ye, Z., et al. (2010). Protective effects of auricularia auricular polysaccharide on chronic cerebral ischemia injury in rats. Chin. J. Pathophysiology 26, 721–724. doi:10.3892/mmr.2018.9579
Lu, Z.-Q., Deng, Y.-J., and Lu, J.-X. (2012). Effect of aloe polysaccharide on caspase-3 expression following cerebral ischemia and reperfusion injury in rats. Mol. Med. Rep. 6, 371–374. doi:10.3892/mmr.2012.927
Lu, Z., Liu, Y., Shi, Y., Shi, X., Wang, X., Xu, C., et al. (2018). Curcumin protects cortical neurons against oxygen and glucose deprivation/reoxygenation injury through flotillin-1 and extracellular signal-regulated kinase1/2 pathway. Biochem. Biophysical Res. Commun. 496, 515–522. doi:10.1016/j.bbrc.2018.01.089
Luo, Y., Nie, J., Gong, Q.-H., Lu, Y.-F., Wu, Q., and Shi, J.-S. (2007). Protective effects of icariin against learning and memory deficits induced by aluminium in rats. Clin. Exp. Pharmacol. Physiol. 34, 792–795. doi:10.1111/j.1440-1681.2007.04647.x
Ma, J., He, W., Gao, C., Yu, D., Xue, P., and Niu, Y. (2019). Antioxidant and neuroprotective effects of Codonopsis pilosula polysaccharides on hypoxic-ischemic brain injury induced by Nrf2 pathway. Chin. J. Clin. Anat. 37, 403–408. doi:10.4077/cjp.2011.amm059
Ma, R., Xie, Q., Li, Y., Chen, Z., Ren, M., Chen, H., et al. (2020). Animal models of cerebral ischemia: a review. Biomed. Pharmacother., 131, 110686. doi:10.1016/j.biopha.2020.110686
Mantsch, J. R., Li, S.-J., Risinger, R., Awad, S., Katz, E., Baker, D. A., et al. (2007). Levo-tetrahydropalmatine attenuates cocaine self-administration and cocaine-induced reinstatement in rats. Psychopharmacology 192, 581–591. doi:10.1007/s00213-007-0754-7
Manzanero, S., Santro, T., and Arumugam, T. V. (2013). Neuronal oxidative stress in acute ischemic stroke: sources and contribution to cell injury. Neurochem. Int. 62 (5), 712–718. doi:10.1016/j.neuint.2012.11.009
Mao, G., Zhang, L., Qian, F., Chen, K., Xu, J., Xu, J., et al. (2019). Research progress on anti-inflammatory activity of three kinds of iridoid glycosides in Chinese materia medica. Chin. Trad. Herbal Drugs 50, 225–233. doi:10.21203/rs.3.rs-58802/v1
Mao, X. W., Pan, C. S., Huang, P., Liu, Y. Y., Wang, C. S., Yan, L., et al. (2015). Levo-tetrahydropalmatine attenuates mouse blood-brain barrier injury induced by focal cerebral ischemia and reperfusion: involvement of Src kinase. Sci. Rep. 5, 11155. doi:10.1038/srep11155
Mo, Z.-t., Liao, Y.-l., Zheng, J., and Li, W.-n. (2020). Icariin protects neurons from endoplasmic reticulum stress-induced apoptosis after OGD/R injury via suppressing IRE1α-XBP1 signaling pathway. Life Sci. 255, 117847. doi:10.1016/j.lfs.2020.117847
Mook-Jung, I., Shin, J.-E., Yun, S. H., Huh, K., Koh, J. Y., Park, H. K., et al. (1999). Protective effects of asiaticoside derivatives against beta-amyloid neurotoxicity. J. Neurosci. Res. 58, 417–425. doi:10.1002/(sici)1097-4547(19991101)58:3<417::aid-jnr7>3.0.co;2-g
Mukherjee, A., Sarkar, S., Jana, S., Swarnakar, S., and Das, N. (2019). Neuro-protective role of nanocapsulated curcumin against cerebral ischemia-reperfusion induced oxidative injury. Brain Res. 1704, 164–173. doi:10.1016/j.brainres.2018.10.016
Negi, A. S., Kumar, J. K., Luqman, S., Shanker, K., Gupta, M. M., and Khanuja, S. P. S. (2008). Recent advances in plant hepatoprotectives: a chemical and biological profile of some important leads. Med. Res. Rev. 28, 746–772. doi:10.1002/med.20115
Nejad, K. H., Dianat, M., Sarkaki, A., Naseri, M. K., Badavi, M., and Farbood, Y. (2015). Ellagic acid improves electrocardiogram waves and blood pressure against global cerebral ischemia rat experimental models. Electron. Physician 7, 1153–1162. doi:10.14661/2015.1153-1162
Oliveira, C., Neves, N. M., Reis, R. L., Martins, A., and Silva, T. H. (2020). A review on fucoidan antitumor strategies: from a biological active agent to a structural component of fucoidan-based systems. Carbohydr. Polym. 239, 116131. doi:10.1016/j.carbpol.2020.116131
Orhan, I. E. (2012). Centella asiatica (L.) urban: from traditional medicine to modern medicine with neuroprotective potential. Evid. Based Complement. Alternat Med. 2012, 946259. doi:10.1155/2012/946259
Pang, Q., Zhao, Y., Chen, X., Zhao, K., Zhai, Q., and Tu, F. (2018). Apigenin protects the brain against ischemia/reperfusion injury via caveolin-1/VEGF in vitro and in vivo. Oxid Med. Cel Longev 2018, 7017204. doi:10.1155/2018/7017204
Park, D.-J., Jeon, S.-J., Kang, J.-B., and Koh, P.-O. (2020). Quercetin reduces ischemic brain injury by preventing ischemia-induced decreases in the neuronal calcium sensor protein hippocalcin. Neuroscience 430, 47–62. doi:10.1016/j.neuroscience.2020.01.015
Peng, X., Shi, Z., Liang, C., Ren, Y., and Tan, R. (2019). Protective effect of Chinese Yam polysaccharides on cerebral ischemia-reperfusion injury in mice. Pharmacol. Clin. Chin. Materia Med. 35, 60–63. doi:10.4268/cjcmm20140327
Perviz, S., Khan, H., and Pervaiz, A. (2016). Plant alkaloids as an emerging therapeutic alternative for the treatment of depression. Front. Pharmacol. 7, 28. doi:10.3389/fphar.2016.00028
Putteeraj, M., Lim, W., Teoh, S. L., and Yahaya, M. (2018). Flavonoids and its neuroprotective effects on brain ischemia and neurodegenerative diseases. Curr. Drug Targets 19, 115. doi:10.2174/1389450119666180326125252
Qian, G., and Min, L. (2010). Protective effect of black fungus polysaccharide on hypoxic-ischemic brain damage in neonatal rats. Chin. J. Trad. Med. Sci. Technol. 17, 129–130. doi:10.1109/hhbe.2011.6027979
Qin, P., Li, X., Yang, H., Wang, Z. Y., and Lu, D. (2019). Therapeutic potential and biological applications of cordycepin and metabolic mechanisms in cordycepin-producing fungi. Molecules 24, 2231. doi:10.3390/molecules24122231
Qiu, S., Sun, H., Zhang, A.-H., Xu, H.-Y., Yan, G.-L., Han, Y., et al. (2014). Natural alkaloids: basic aspects, biological roles, and future perspectives. Chin. J. Nat. Medicines 12, 401–406. doi:10.1016/s1875-5364(14)60063-7
Ran, K., Yang, D.-L., Chang, Y.-T., Duan, K.-M., Ou, Y.-W., Wang, H.-P., et al. (2014). Ginkgo biloba extract postconditioning reduces myocardial ischemia reperfusion injury. Genet. Mol. Res. 13, 2703–2708. doi:10.4238/2014.april.8.14
Ren, J., Fan, C., Chen, N., Huang, J., and Yang, Q. (2011). Resveratrol pretreatment attenuates cerebral ischemic injury by upregulating expression of transcription factor Nrf2 and HO-1 in rats. Neurochem. Res. 36, 2352–2362. doi:10.1007/s11064-011-0561-8
Ren, S., Zhang, H., Mu, Y., Sun, M., and Liu, P. (2013). Pharmacological effects of Astragaloside IV: a literature review. J. Trad. Chin. Med. 33, 413–416. doi:10.1016/s0254-6272(13)60189-2
Rosales, P. F., Bordin, G. S., Gower, A. E., and Moura, S. (2020). Indole alkaloids: 2012 until now, highlighting the new chemical structures and biological activities. Fitoterapia 143, 104558. doi:10.1016/j.fitote.2020.104558
Ryoo, N., Rahman, M. A., Hwang, H., Ko, S. K., Nah, S.-Y., Kim, H.-C., et al. (2020). Ginsenoside Rk1 is a novel inhibitor of NMDA receptors in cultured rat hippocampal neurons. J. Ginseng Res. 44, 490–495. doi:10.1016/j.jgr.2019.04.002
Ryu, E., Son, M., Lee, M., Lee, K., Cho, J. Y., Cho, S., et al. (2014). Cordycepin is a novel chemical suppressor of Epstein-Barr virus replication. Oncoscience 1, 866–881. doi:10.18632/oncoscience.110
Saini, A., Taliyan, R., and Sharma, P. (2014). Protective effect and mechanism of Ginkgo biloba extract-EGb 761 on STZ-induced diabetic cardiomyopathy in rats. Phcog Mag. 10, 172–178. doi:10.4103/0973-1296.131031
Santos, A. C., Pereira, I., Pereira-Silva, M., Ferreira, L., Caldas, M., Collado-González, M., et al. (2019). Nanotechnology-based formulations for resveratrol delivery: effects on resveratrol in vivo bioavailability and bioactivity. Colloids Surf. B: Biointerfaces 180, 127–140. doi:10.1016/j.colsurfb.2019.04.030
Savopoulos, C., Ntaios, G., and Hatzitolios, A. (2009). Is there a geographic variation in the seasonal distribution of acute myocardial infarction and sudden cardiac death?. Int. J. Cardiol. 135, 253–254. doi:10.1016/j.ijcard.2008.03.019
Shafabakhsh, R., and Asemi, Z. (2019). Quercetin: a natural compound for ovarian cancer treatment. J. Ovarian Res. 12, 55. doi:10.1186/s13048-019-0530-4
Shao, J., Jin, H., Zhu, H., and Liu, J. (2013). Optimization of the technological parameters for the alcohol extraction of ginkgo flavonoid glycosides and the in vitro antioxidant study. J. China Univ. Mining Technol. 42, 663–669. doi:10.1016/j.phytochem.2016.05.005
She, D. T., Jo, D. G., and Arumugam, T. V. (2017). Emerging roles of sirtuins in ischemic stroke. Transl Stroke Res. 12, 147. doi:10.1007/s12975-017-0544-4
Simão, F., Matté, A., Pagnussat, A. S., Netto, C. A., and Salbego, C. G. (2012b). Resveratrol preconditioning modulates inflammatory response in the rat hippocampus following global cerebral ischemia. Neurochem. Int. 61, 659–665. doi:10.1016/j.neuint.2012.06.009
Simão, F., Matté, A., Pagnussat, A. S., Netto, C. A., and Salbego, C. G. (2012a). Resveratrol prevents CA1 neurons against ischemic injury by parallel modulation of both GSK-3β and CREB through PI3-K/Akt pathways. Eur. J. Neurosci. 36, 2899–2905. doi:10.1111/j.1460-9568.2012.08229.x
Song, B., Tang, X., Wang, X., Huang, X., Ye, Y., Lu, X., et al. (2012). Bererine induces peripheral lymphocytes immune regulations to realize its neuroprotective effects in the cerebral ischemia/reperfusion mice. Cell Immunol. 276, 91–100. doi:10.1016/j.cellimm.2012.04.006
Song, D., Hao, J., and Fan, D. (2020). Biological properties and clinical applications of berberine. Front. Med. 4, 144. doi:10.5194/hess-2020-1-rc1
Song, M.-t., Ruan, J., Zhang, R.-y., Deng, J., Ma, Z.-q., and Ma, S.-p. (2018). Astragaloside IV ameliorates neuroinflammation-induced depressive-like behaviors in mice via the PPARγ/NF-κB/NLRP3 inflammasome axis. Acta Pharmacol. Sin 39, 1559–1570. doi:10.1038/aps.2017.208
Sowndhararajan, K., Deepa, P., Kim, M., Park, S. J., and Kim, S. (2018). Neuroprotective and cognitive enhancement potentials of baicalin: a review. Brain Sci. 8, 137. doi:10.3390/brainsci8060104
Stanimirovic, D., and Satoh, K. (2000). Inflammatory mediators of cerebral endothelium: a role in ischemic brain inflammation. Brain Pathol. 10 (1), 113–126. doi:10.1111/j.1750-3639.2000.tb00248.x
Steliga, A., Kowiański, P., Czuba, E., Waśkow, M., Moryś, J., and Lietzau, G. (2020). Neurovascular unit as a source of ischemic stroke biomarkers-limitations of experimental studies and perspectives for clinical application. Transl. Stroke Res. 11 (4), 553–579. doi:10.1007/s12975-019-00744-5
Stuppner, H., and Wagner, H. (1989). New cucurbitacin glycosides fromPicrorhiza kurrooa. Planta Med. 55, 559–563. doi:10.1055/s-2006-962095
Sun, J. B., Li, Y., Cai, Y. F., Huang, Y., Liu, S., Yeung, P. K., et al. (2018). Scutellarin protects oxygen/glucose-deprived astrocytes and reduces focal cerebral ischemic injury. Neural Regen. Res. 13, 1396–1407. doi:10.4103/1673-5374.235293
Sun, R., Song, Y., Li, S., Ma, Z., Deng, X., Fu, Q., et al. (2018). Levo-tetrahydropalmatine attenuates neuron apoptosis induced by cerebral ischemia-reperfusion injury: involvement of c-abl activation. J. Mol. Neurosci. 65, 391–399. doi:10.1007/s12031-018-1063-9
Sy, L., Xie, Y., and Yu, Z. (2015). Notoginseng polysaccharide on rat ischemia-reperfusion injury of protection. J. Apoplexy Nervous Dis. 32, 996–998. doi:10.1007/978-3-0348-8988-9_25
Tang, B., Wang, D., Li, M., Wu, Q., Yang, Q., Shi, W., et al. (2017). An in vivo study of hypoxia-inducible factor-1α signaling in ginsenoside Rg1-mediated brain repair after hypoxia/ischemia brain injury. Pediatr. Res. 81, 120–126. doi:10.1038/pr.2016.178
Tang, H., Tang, Y., Li, N., Shi, Q., Guo, J., Shang, E., et al. (2014). Neuroprotective effects of scutellarin and scutellarein on repeatedly cerebral ischemia-reperfusion in rats. Pharmacol. Biochem. Behav. 118, 51–59. doi:10.1016/j.pbb.2014.01.003
Thapa, S. B., Pandey, R. P., Park, Y. I., and Kyung Sohng, J. (2019). Biotechnological advances in resveratrol production and its chemical diversity. Molecules 24, 2571. doi:10.3390/molecules24142571
Thiyagarajan, M., and Sharma, S. S. (2004). Neuroprotective effect of curcumin in middle cerebral artery occlusion induced focal cerebral ischemia in rats. Life Sci. 74, 969–985. doi:10.1016/j.lfs.2003.06.042
Tian, Y., Liu, A., Su, J., Xu, S., Dai, G., and Xue, H. (2015). Theoretical study on the structure-activity relationship of andrographolide derivatives. J. Zhengzhou University(Natural Sci. Edition) 47, 103–106. doi:10.3109/14756361003724760
Tsai, S.-K., Hung, L.-M., Fu, Y.-T., Cheng, H., Nien, M.-W., Liu, H.-Y., et al. (2007). Resveratrol neuroprotective effects during focal cerebral ischemia injury via nitric oxide mechanism in rats. J. Vasc. Surg. 46, 346–353. doi:10.1016/j.jvs.2007.04.044
Tu, F., Pang, Q., Huang, T., Zhao, Y., Liu, M., and Chen, X. (2017). Apigenin ameliorates post-stroke cognitive deficits in rats through histone acetylation-mediated neurochemical alterations. Med. Sci. Monit. 23, 4004–4013. doi:10.12659/msm.902770
Tu, X. K., Yang, W. Z., Shi, S. S., Chen, Y., Wang, C. H., Chen, C. M., et al. (2011). Baicalin inhibits TLR2/4 signaling pathway in rat brain following permanent cerebral ischemia. Inflammation 34, 463–470. doi:10.1007/s10753-010-9254-8
Tuli, H. S., Sandhu, S., and Sharma, A. (2013). Pharmacological and therapeutic potential of Cordyceps with special reference to Cordycepin. Biotech 4, 1-12. doi:10.1007/s13205-013-0121-9
Ułamek-Kozioł, M., Czuczwar, S. J., Januszewski, S., and Pluta, R. (2020). Substantiation for the use of curcumin during the development of neurodegeneration after brain ischemia. Int. J. Mol. Sci. 21, 517. doi:10.3390/ijms21020517
Wan, W., Zhang, C., Danielsen, M., Li, Q., Chen, W., Chan, Y., et al. (2016). EGb761 improves cognitive function and regulates inflammatory responses in the APP/PS1 mouse. Exp. Gerontol. 81, 92–100. doi:10.1016/j.exger.2016.05.007
Wang, C., Li, Y., Gao, S., Cheng, D., Zhao, S., and Liu, E. (2015). Breviscapine injection improves the therapeutic effect of western medicine on angina pectoris patients. PLoS One 10, e0129969. doi:10.1371/journal.pone.0129969
Wang, D. Q., He, Z. D., Feng, B. S., and Yang, C. R. (1993). Chemical constituents from Picrorhiza scrophulariiflora. Acta Botanica Yunnanica 15, 83–88.
Wang, F., Zhang, Y., Zheng, X., Dai, Z., Liu, B., and Ma, S. (2019). Research progress of the structure and biological activities of iridoids compounds. Chin. Pharm. Aff. 33, 323–330. doi:10.1159/000391383
Wang, J., Wang, L., Lou, G.-H., Zeng, H.-R., Hu, J., Huang, Q.-W., et al. (2019a). Coptidis Rhizoma: a comprehensive review of its traditional uses, botany, phytochemistry, pharmacology and toxicology. Pharm. Biol. 57, 193–225. doi:10.1080/13880209.2019.1577466
Wang, J., Xu, J., Gong, X., Yang, M., Zhang, C., and Li, M. (2019b). Biosynthesis, chemistry, and pharmacology of polyphenols from Chinese salvia species: a review. Molecules 24, 59. doi:10.3390/molecules24010155
Wang, L.-Y., Liu, J., Li, Y., Li, B., Zhang, Y.-Y., Jing, Z.-W., et al. (2015). Time-dependent variation of pathways and networks in a 24-hour window after cerebral ischemia-reperfusion injury. BMC Syst. Biol. 9 (1), 11. doi:10.1186/s12918-015-0152-4
Wang, L., and Ma, Q. (2018). Clinical benefits and pharmacology of scutellarin: a comprehensive review. Pharmacol. Ther. 190, 105–127. doi:10.1016/j.pharmthera.2018.05.006
Wang, L., Wang, P., Xu, K., Chen, Y., Li, Q., and Lei, H. (2013). Research progress on structure-activity relationship of ligustrazine derivatives. Chin. Trad. Herbal Drugs 44, 2766–2771. doi:10.21873/anticanres.11635
Wang, L., Zhao, H., Zhai, Z.-z., and Qu, L.-x. (2018). Protective effect and mechanism of ginsenoside Rg1 in cerebral ischaemia-reperfusion injury in mice. Biomed. Pharmacother. 99, 876–882. doi:10.1016/j.biopha.2018.01.136
Wang, Q., Sun, A. Y., Simonyi, A., Jensen, M. D., Shelat, P. B., Rottinghaus, G. E., et al. (2005). Neuroprotective mechanisms of curcumin against cerebral ischemia-induced neuronal apoptosis and behavioral deficits. J. Neurosci. Res. 82, 138–148. doi:10.1002/jnr.20610
Wang, S.-W., Lai, C.-Y., and Wang, C.-J. (1992). Inhibitory effect of geniposide on aflatoxin B1-induced DNA repair synthesis in primary cultured rat hepatocytes. Cancer Lett. 65, 133–137. doi:10.1016/0304-3835(92)90157-q
Wang, T., Zhai, L., Zhang, H., Zhao, L., and Guo, Y. (2015a). Picroside II inhibits the MEK-ERK1/2-COX2 signal pathway to prevent cerebral ischemic injury in rats. J. Mol. Neurosci. 57, 335–351. doi:10.1007/s12031-015-0623-5
Wang, T., Zhao, L., Guo, Y., Zhang, M., and Pei, H. (2015b). Picroside II inhibits neuronal apoptosis and improves the morphology and structure of brain tissue following cerebral ischemic injury in rats. PLoS One 10, e0124099. doi:10.1371/journal.pone.0124099
Wang, W., Ma, X., Han, J., Zhou, M., Ren, H., Pan, Q., et al. (2016). Neuroprotective effect of scutellarin on ischemic cerebral injury by down-regulating the expression of angiotensin-converting enzyme and AT1 receptor. PLoS One 11, e0146197. doi:10.1371/journal.pone.0146197
Wang, X. (2006). Research on the design, synthesis, biological activity and quantitative structure-activity relationship of emodin derivatives targeting matrix metalloproteinases. Jinan, China: Shandong University.
Wang, X., Chen, L., Tan, C., and Xie, D. (2014). Protective effect of lycium barbarum polysaccharides on cerebral ischemia-reperfusion injury model rats. China Pharm. 25, 1365–1367. doi:10.5353/th_b5328058
Wang, X., and Zhao, L. (2016). Calycosin ameliorates diabetes-induced cognitive impairments in rats by reducing oxidative stress via the PI3K/Akt/GSK-3β signaling pathway. Biochem. Biophys. Res. Commun. 473, 428–434. doi:10.1016/j.bbrc.2016.03.024
Wang, Y., Dong, X., Li, Z., Wang, W., Tian, J., and Chen, J. (2014). Downregulated RASD1 and upregulated miR-375 are involved in protective effects of calycosin on cerebral ischemia/reperfusion rats. J. Neurol. Sci. 339, 144–148. doi:10.1016/j.jns.2014.02.002
Wang, Y., Ren, Q., Zhang, X., Lu, H., and Chen, J. (2018). Neuroprotective mechanisms of calycosin against focal cerebral ischemia and reperfusion injury in rats. Cel Physiol. Biochem. 45, 537–546. doi:10.1159/000487031
Wang, Y., Su, Y., Lai, W., Huang, X., Chu, K., Brown, J., et al. (2020). Salidroside restores an anti-inflammatory endothelial phenotype by selectively inhibiting endothelial complement after oxidative stress. Inflammation 43, 310–325. doi:10.1007/s10753-019-01121-y
Wang, Y., Wu, Y., Liang, C., Tan, R., Tan, L., and Tan, R. (2019). Pharmacodynamic effect of ellagic acid on ameliorating cerebral ischemia/reperfusion injury. Pharmacology 104, 320–331. doi:10.1159/000502401
Wang, Y., Zhen, Y., Wu, X., Jiang, Q., Li, X., Chen, Z., et al. (2015). Vitexin protects brain against ischemia/reperfusion injury via modulating mitogen-activated protein kinase and apoptosis signaling in mice. Phytomedicine 22, 379–384. doi:10.1016/j.phymed.2015.01.009
Wang, F., Yin, P., Lu, Y., Zhou, Z., Jiang, C., Liu, Y., et al. (2015). Cordycepin prevents oxidative stress-induced inhibition of osteogenesis. Oncotarget 6, 117. doi:10.18632/oncotarget.6072
Wang, Y.-Y., Chang, C.-Y., Lin, S.-Y., Wang, J.-D., Wu, C.-C., Chen, W.-Y., et al. (2020). Quercetin protects against cerebral ischemia/reperfusion and oxygen glucose deprivation/reoxygenation neurotoxicity. J. Nutr. Biochem. 83, 108436. doi:10.1016/j.jnutbio.2020.108436
Wei, H., Wang, S., Zhen, L., Yang, Q., Wu, Z., Lei, X., et al. (2015). Resveratrol attenuates the blood-brain barrier dysfunction by regulation of the MMP-9/TIMP-1 balance after cerebral ischemia reperfusion in rats. J. Mol. Neurosci. 55, 872–879. doi:10.1007/s12031-014-0441-1
Wei, Y., Hong, H., Zhang, X., Lai, W., Wang, Y., Chu, K., et al. (2017). Salidroside inhibits inflammation through PI3K/Akt/HIF signaling after focal cerebral ischemia in rats. Inflammation 40, 1297–1309. doi:10.1007/s10753-017-0573-x
Williams, E. I., Betterton, R. D., Davis, T. P., and Ronaldson, P. T. (2020). Transporter-mediated delivery of small molecule drugs to the brain: a critical mechanism that can advance therapeutic development for ischemic stroke. Pharmaceutics 12, 63. doi:10.3390/pharmaceutics12020154
Wu, L., and Fang, F. (2020). Advances in structural modification and structure-activity relationship of berberine. Chin. J. New Drugs 29, 1257–1264. doi:10.1001/archneur.1968.00470320053006
Wu, S.-y., Wang, G.-f., Liu, Z.-q., Rao, J.-j., Lü, L., Xu, W., et al. (2009). Effect of geniposide, a hypoglycemic glucoside, on hepatic regulating enzymes in diabetic mice induced by a high-fat diet and streptozotocin. Acta Pharmacol. Sin 30, 202–208. doi:10.1038/aps.2008.17
Xia, Z., Zhang, R., Wu, P., Xia, Z., and Hu, Y. (2012). Memory defect induced by beta-amyloid plus glutamate receptor agonist is alleviated by catalpol and donepezil through different mechanisms. Brain Res. 1441, 27–37. doi:10.1016/j.brainres.2012.01.008
Xie, C.-L., Li, J.-H., Wang, W.-W., Zheng, G.-Q., and Wang, L.-X. (2015). Neuroprotective effect of ginsenoside-Rg1 on cerebral ischemia/reperfusion injury in rats by downregulating protease-activated receptor-1 expression. Life Sci. 121, 145–151. doi:10.1016/j.lfs.2014.12.002
Xie, C. J., Gu, A. P., Cai, J., Wu, Y., and Chen, R. C. (2018). Curcumin protects neural cells against ischemic injury in N2a cells and mouse brain with ischemic stroke. Brain Behav. 8, e00921. doi:10.1002/brb3.921
Xie, W., Zhou, P., Sun, Y., Meng, X., Dai, Z., Sun, G., et al. (2018). Protective effects and target network analysis of ginsenoside Rg1 in cerebral ischemia and reperfusion injury: a comprehensive overview of experimental studies. Cells 7, 114. doi:10.3390/cells7120270
Xiong, D., Deng, Y., Huang, B., Yin, C., Liu, B., Shi, J., et al. (2016). Icariin attenuates cerebral ischemia-reperfusion injury through inhibition of inflammatory response mediated by NF-κB, PPARα and PPARγ in rats. Int. Immunopharmacology 30, 157–162. doi:10.1016/j.intimp.2015.11.035
Xu, D., Hu, M. J., Wang, Y. Q., and Cui, Y. L. (2019). Antioxidant activities of quercetin and its complexes for medicinal application. Molecules 24, 119. doi:10.3390/molecules24061123
Xu, H., Hua, Y., Zhong, J., Li, X., Xu, W., Cai, Y., et al. (2018). Resveratrol delivery by albumin nanoparticles improved neurological function and neuronal damage in transient middle cerebral artery occlusion rats. Front. Pharmacol. 9, 1403. doi:10.3389/fphar.2018.01403
Xu, L., Ding, L., Su, Y., Shao, R., Liu, J., and Huang, Y. (2019). Neuroprotective effects of curcumin against rats with focal cerebral ischemia-reperfusion injury. Int. J. Mol. Med. 43, 1879–1887. doi:10.3892/ijmm.2019.4094
Xu, N., Huang, F., Jian, C., Qin, L., Lu, F., Wang, Y., et al. (2019). Neuroprotective effect of salidroside against central nervous system inflammation-induced cognitive deficits: a pivotal role of sirtuin 1-dependent N rf-2/HO -1/NF -κ B pathway. Phytotherapy Res. 33, 1438–1447. doi:10.1002/ptr.6335
Xu, S.-H., Yin, M.-S., Liu, B., Chen, M.-L., He, G.-w., Zhou, P.-P., et al. (2017). Tetramethylpyrazine-2'-O-sodium ferulate attenuates blood-brain barrier disruption and brain oedema after cerebral ischemia/reperfusion. Hum. Exp. Toxicol. 36, 670–680. doi:10.1177/0960327116657401
Xue, D., Jiang, H., Xiao, K., and Xuan, L. (2001). Isolation, identification and antioxidant effect of soy isoflavones. J. Nanjing Agric. Univ., 89–92. doi:10.1186/isrctn98074661
Xue, X., Qu, X.-J., Yang, Y., Sheng, X.-H., Cheng, F., Jiang, E.-N., et al. (2010). Baicalin attenuates focal cerebral ischemic reperfusion injury through inhibition of nuclear factor κB p65 activation. Biochem. Biophysical Res. Commun. 403, 398–404. doi:10.1016/j.bbrc.2010.11.042
Xue, X., Quan, Y., Gong, L., Gong, X., and Li, Y. (2020). A review of the processed Polygonum multiflorum (Thunb.) for hepatoprotection: clinical use, pharmacology and toxicology. J. Ethnopharmacol. 261, 113121. doi:10.1016/j.jep.2020.113121
XuJ., , Kong, X., Xiu, H., Dou, Y., Wu, Z., and Sun, P. (2018). Combination of curcumin and vagus nerve stimulation attenuates cerebral ischemia/reperfusion injury-induced behavioral deficits. Biomed. Pharmacother. 103, 614–620. doi:10.1016/j.biopha.2018.04.069
XuL, , Jia, L., Wang, Q., Hou, J., Li, S., and Teng, J. (2018). Salidroside attenuates hypoxia/reoxygenation-induced human brain vascular smooth muscle cell injury by activating the SIRT1/FOXO3α pathway. Exp. Ther. Med. 15, 822–830. doi:10.3892/etm.2017.5446
Yan, A., and Xie, Y. (2018). Effect of angelicae sinensis radix polysaccharide on oxidative stress level and inflammatory cytokine expression of brain tissues in rats with cerebral ischemia reperfusion injury. Chin. J. Exp. Trad. Med. Formulae 24, 123–127. doi:10.1159/000418069
Yan, L., and Zhou, Q. H. (2012). Study on neuroprotective effects of astragalan in rats with ischemic brain injury and its mechanisms. Zhongguo Ying Yong Sheng Li Xue Za Zhi 28, 373–377. doi:10.1016/j.jalz.2010.05.551
Yan, S., Chen, L., Wei, X., Cheng, L., Kong, L., Liu, X., et al. (2015). Tetramethylpyrazine analogue CXC195 ameliorates cerebral ischemia-reperfusion injury by regulating endothelial nitric oxide synthase phosphorylation via PI3K/Akt signaling. Neurochem. Res. 40, 446–454. doi:10.1007/s11064-014-1485-x
Yang, J., Huang, J., Shen, C., Cheng, W., Yu, P., Wang, L., et al. (2018a). Resveratrol treatment in different time-attenuated neuronal apoptosis after oxygen and glucose deprivation/reoxygenation via enhancing the activation of nrf-2 signaling pathway in vitro. Cel Transpl. 27, 1789–1797. doi:10.1177/0963689718780930
Yang, J., Yan, H., Li, S., and Zhang, M. (2018b). Berberine ameliorates MCAO induced cerebral ischemia/reperfusion injury via activation of the BDNF-TrkB-PI3K/Akt signaling pathway. Neurochem. Res. 43, 702–710. doi:10.1007/s11064-018-2472-4
Yang, X., Zhang, L., Jiang, S., Gong, P., and Zeng, F. (2009). Effect of dauricine on apoptosis and expression of apoptogenic protein after transient focal cerebral ischemia-reperfusion injury in rats. Zhongguo Zhong Yao Za Zhi 34, 78–83. doi:10.31083/jin-170047
Yang, Y., Liu, P., Chen, L., Liu, Z., Zhang, H., Wang, J., et al. (2013). Therapeutic effect of Ginkgo biloba polysaccharide in rats with focal cerebral ischemia/reperfusion (I/R) injury. Carbohydr. Polym. 98, 1383–1388. doi:10.1016/j.carbpol.2013.07.045
Yang, Y., Liu, Y., Liu, M., Hu, W., Yang, X., Deng, Y., et al. (2020). The neuroprotective effects of icariin on focal cerebral ischemia reperfusion injured rats during early recovery period. Trad. Chin. Drug Res. Clin. Pharmacol. 31, 662–667. doi:10.3892/ijmm.2019.4094
Ye, T. M., Sun, L. N., Su, F., Shen, J., Wang, H. P., Ye, Z. G., et al. (2010). Study of the effects of Auricularia auricular polysaccharide on local ischemia/reperfusion injury in rat. Zhongguo Ying Yong Sheng Li Xue Za Zhi 26, 423–426. doi:10.14711/thesis-b878059
Yen, T.-L., Chen, R.-J., Jayakumar, T., Lu, W.-J., Hsieh, C.-Y., Hsu, M.-J., et al. (2016). Andrographolide stimulates p38 mitogen-activated protein kinase-nuclear factor erythroid-2-related factor 2-heme oxygenase 1 signaling in primary cerebral endothelial cells for definite protection against ischemic stroke in rats. Translational Res. 170, 57–72. doi:10.1016/j.trsl.2015.12.002
Yen, T.-L., Hsu, W.-H., Huang, S. K.-H., Lu, W.-J., Chang, C.-C., Lien, L.-M., et al. (2013). A novel bioactivity of andrographolide fromAndrographis paniculataon cerebral ischemia/reperfusion-induced brain injury through induction of cerebral endothelial cell apoptosis. Pharm. Biol. 51, 1150–1157. doi:10.3109/13880209.2013.782051
Yin, F., Liu, J., Zheng, X., Guo, L., and Xiao, H. (2010). Geniposide induces the expression of heme oxygenase-1 via PI3K/Nrf2-signaling to enhance the antioxidant capacity in primary hippocampal neurons. Biol. Pharm. Bull. 33, 1841–1846. doi:10.1248/bpb.33.1841
Yin, F., Zhou, H., Fang, Y., Li, C., He, Y., Yu, L., et al. (2020). Astragaloside IV alleviates ischemia reperfusion-induced apoptosis by inhibiting the activation of key factors in death receptor pathway and mitochondrial pathway. J. Ethnopharmacology 248, 112319. doi:10.1016/j.jep.2019.112319
Yin, Q., Wang, R., Yang, S., Wu, Z., Guo, S., Dai, X., et al. (2017). Influence of temperature on transdermal penetration enhancing mechanism of borneol: a multi-scale study. Int. J. Mol. Sci. 18, 195. doi:10.3390/ijms18010195
Ying, L., and Chi, Y. (2007). Research progress on the separation and structure-activity relationship of flavonoid glycosides from botanicals. Chin. J. Inf. Trad. Chin. Med., 100–102. doi:10.4268/cjcmm20131120
You, X., Yang, M., Ming, X., Li, Q., Qian, L., Liu, Y., et al. (2020). Efficacy of ligustrazine hydrochloride injection combined with butylphthalide capsule in the treatment of acute cerebral infarction and the effect of hemorheology. Shaanxi J. Trad. Chin. Med. 41, 743–745+757. doi:10.4314/tjpr.v17i9.28
Yu, B., Ruan, M., Zhang, Z. N., Cheng, H. B., and Shen, X. C. (2016). Synergic effect of borneol and ligustrazine on the neuroprotection in global cerebral ischemia/reperfusion injury: a region-specificity study. Evid. Based Complement. Alternat Med. 2016, 4072809. doi:10.1155/2016/4072809
Yu, B., Zhong, F. M., Yao, Y., Deng, S. Q., Xu, H. Q., Lu, J. F., et al. (2019). Synergistic protection of tetramethylpyrazine phosphate and borneol on brain microvascular endothelium cells injured by hypoxia. Am. J. Transl Res. 11, 2168–2180. doi:10.1093/chromsci/46.5.395
Yu, B., Ruan, M., Liang, T., Huang, S. W., Liu, S. J., Cheng, H. B., et al. (2017). Tetramethylpyrazine phosphate and borneol combination therapy synergistically attenuated ischemia-reperfusion injury of the hypothalamus and striatum via regulation of apoptosis and autophagy in a rat model. Am. J. Transl Res. 9, 4807–4820. doi:10.1007/978-3-7091-6837-0_3
Yu, P., Wang, L., Tang, F., Zeng, L., Zhou, L., Song, X., et al. (2017). Resveratrol pretreatment decreases ischemic injury and improves neurological function via sonic hedgehog signaling after stroke in rats. Mol. Neurobiol. 54, 212–226. doi:10.1007/s12035-015-9639-7
Zhai, L., Liu, M., Wang, T., Zhang, H., Li, S., and Guo, Y. (2017). Picroside II protects the blood-brain barrier by inhibiting the oxidative signaling pathway in cerebral ischemia-reperfusion injury. PLoS One 12, e0174414. doi:10.1371/journal.pone.0174414
Zhang, C., Chen, S., Zhang, Z., Xu, H., Zhang, W., Xu, D., et al. (2020). Asiaticoside alleviates cerebral ischemia-reperfusion injury via NOD2/mitogen-activated protein kinase (MAPK)/Nuclear factor kappa B (NF-kappaB) signaling pathway. Med. Sci. Monit. 26, e920325. doi:10.12659/msm.920325
Zhang, H.-F., Hu, X.-M., Wang, L.-X., Xu, S.-Q., and Zeng, F.-D. (2009). Protective effects of scutellarin against cerebral ischemia in rats: evidence for inhibition of the apoptosis-inducing factor pathway. Planta Med. 75, 121–126. doi:10.1055/s-0028-1088368
Zhang, H., Zhai, L., Wang, T., Li, S., and Guo, Y. (2017). Picroside II exerts a neuroprotective effect by inhibiting the mitochondria cytochrome C signal pathway following ischemia reperfusion injury in rats. J. Mol. Neurosci. 61, 267–278. doi:10.1007/s12031-016-0870-0
Zhang, J., Wu, C., Du, G., and Qin, X. (2019). Astragaloside IV derived from Astragalus membranaceus: a research review on the pharmacological effects. Adv. Pharmacol. 87, 89–112. doi:10.1016/bs.apha.2019.08.002
Zhang, J., Yang, L., and Guo, L. J. (2011). Effects od daurinoline on microcirculation of cerebral pia mater in mice. Med. J. Wuhan Univ. 32, 187. doi:10.1080/10739680601139153
Zhang, L., Cui, M., and Chen, S. (2020a). Identification of the molecular mechanisms of peimine in the treatment of cough using computational target fishing. Molecules 25, 1105. doi:10.3390/molecules25051105
Zhang, L., Li, F., Hou, C., Zhu, S., Zhong, L., Zhao, J., et al. (2020b). Design, synthesis, and biological evaluation of novel stachydrine derivatives as potent neuroprotective agents for cerebral ischemic stroke. Naunyn Schmiedebergs Arch. Pharmacol. 393, 2529–2542. doi:10.1007/s00210-020-01868-4
Zhang, Q., Bian, H., Guo, L., and Zhu, H. (2016b). Pharmacologic preconditioning with berberine attenuating ischemia-induced apoptosis and promoting autophagy in neuron. Am. J. Transl Res. 8, 1197–1207. doi:10.4016/14162.01
Zhang, Q., Bian, H., Guo, L., and Zhu, H. (2016a). Berberine preconditioning protects neurons against ischemia via sphingosine-1-phosphate and hypoxia-inducible factor-1α. Am. J. Chin. Med. 44, 927–941. doi:10.1142/s0192415x16500518
Zhang, Q., and Zhao, Y.-H. (2014). Therapeutic angiogenesis after ischemic stroke: Chinese medicines, bone marrow stromal cells (BMSCs) and their combinational treatment. Am. J. Chin. Med. 42, 61–77. doi:10.1142/s0192415x14500049
Zhang, R.-X., Li, M.-X., and Jia, Z.-P. (2008). Rehmannia glutinosa: review of botany, chemistry and pharmacology. J. Ethnopharmacology 117, 199–214. doi:10.1016/j.jep.2008.02.018
Zhang, X., Du, Q., Yang, Y., Wang, J., Liu, Y., Zhao, Z., et al. (2018). Salidroside alleviates ischemic brain injury in mice with ischemic stroke through regulating BDNK mediated PI3K/Akt pathway. Biochem. Pharmacol. 156, 99–108. doi:10.1016/j.bcp.2018.08.015
Zhang, X., Zhang, X., Wang, C., Li, Y., Dong, L., Cui, L., et al. (2012). Neuroprotection of early and short-time applying berberine in the acute phase of cerebral ischemia: up-regulated pAkt, pGSK and pCREB, down-regulated NF-κB expression, ameliorated BBB permeability. Brain Res. 1459, 61–70. doi:10.1016/j.brainres.2012.03.065
Zhang, Y., Fang, M., Sun, Y., Zhang, T., Shi, N., Li, J., et al. (2018). Curcumin attenuates cerebral ischemia injury in Sprague-Dawley rats and PC12 cells by suppressing overactivated autophagy. J. Photochem. Photobiol. B: Biol. 184, 1–6. doi:10.1016/j.jphotobiol.2018.05.010
ZhangX., , Lai, W., Ying, X., Xu, L., Chu, K., Brown, J., et al. (2019). Salidroside reduces inflammation and brain injury after permanent middle cerebral artery occlusion in rats by regulating PI3K/PKB/Nrf2/NFκB signaling rather than complement C3 activity. Inflammation 42, 1830–1842. doi:10.1007/s10753-019-01045-7
Zhao, B., Shen, C., Zheng, Z., Wang, X., Zhao, W., Chen, X., et al. (2018). Peiminine inhibits glioblastoma in Vitro and in Vivo through cell cycle arrest and autophagic flux blocking. Cel Physiol. Biochem. 51, 1566–1583. doi:10.1159/000495646
Zhao, T., Tang, H., Xie, L., Zheng, Y., Ma, Z., Sun, Q., et al. (2019). Scutellaria baicalensis Georgi. (Lamiaceae): a review of its traditional uses, botany, phytochemistry, pharmacology and toxicology. J. Pharm. Pharmacol. 71, 1353–1369. doi:10.1111/jphp.13129
Zhao, X., Li, X., Ren, Q., Tian, J., and Chen, J. (2016). Calycosin induces apoptosis in colorectal cancer cells, through modulating the ERβ/MiR-95 and IGF-1R, PI3K/Akt signaling pathways. Gene 591, 123–128. doi:10.1016/j.gene.2016.07.012
Zhao, Y., Liu, Y., and Chen, K. (2016). Mechanisms and clinical application of tetramethylpyrazine (an interesting natural compound isolated from Ligusticum wallichii): current status and perspective. Oxid Med. Cel Longev 2016, 2124638. doi:10.1155/2016/2124638
Zheng, C., and Qin, L.-P. (2007). Chemical components of Centella asiatica and their bioactivities. Zhong Xi Yi Jie He Xue Bao 5, 348–351. doi:10.3736/jcim20070324
Zheng, J.-S., Zheng, P.-D., Mungur, R., Zhou, H.-J., Hassan, M., and Jiang, S.-N. (2018). Ginkgolide B promotes the proliferation and differentiation of neural stem cells following cerebral ischemia/reperfusion injury, both in vivo and in vitro. Neural Regen. Res. 13, 1204–1211. doi:10.4103/1673-5374.232476
Zheng, Q., Chen, Z.-X., Xu, M.-B., Zhou, X.-L., Huang, Y.-Y., Zheng, G.-Q., et al. (2018a). Borneol, a messenger agent, improves central nervous system drug delivery through enhancing blood-brain barrier permeability: a preclinical systematic review and meta-analysis. Drug Deliv. 25, 1617–1633. doi:10.1080/10717544.2018.1486471
Zheng, Q., Huang, Y. Y., Zhu, P. C., Tong, Q., Bao, X. Y., Zhang, Q. H., et al. (2018b). Ligustrazine exerts cardioprotection in animal models of myocardial ischemia/reperfusion injury: preclinical evidence and possible mechanisms. Front. Pharmacol. 9, 729. doi:10.3389/fphar.2018.00729
Zheng, T., Jiang, H., Jin, R., Zhao, Y., Bai, Y., Xu, H., et al. (2019). Ginsenoside Rg1 attenuates protein aggregation and inflammatory response following cerebral ischemia and reperfusion injury. Eur. J. Pharmacol. 853, 65–73. doi:10.1016/j.ejphar.2019.02.018
Zheng, W.-x., Cao, X.-l., Wang, F., Wang, J., Ying, T.-z., Xiao, W., et al. (2015). Baicalin inhibiting cerebral ischemia/hypoxia-induced neuronal apoptosis via MRTF-A-mediated transactivity. Eur. J. Pharmacol. 767, 201–210. doi:10.1016/j.ejphar.2015.10.027
Zhong, Y., Gao, Y., Xu, Y., Qi, C., and Wu, B. (2020). Synthesis of novel aryloxyethylamine derivatives and evaluation of their in Vitro and in Vivo neuroprotective activities. Chem. Biodivers. 7, 33. doi:10.1080/10739680600777599
Zhong, Z. F., Han, J., Zhang, J. Z., Xiao, Q., Chen, J. Y., Zhang, K., et al. (2019). Neuroprotective effects of salidroside on cerebral ischemia/reperfusion-induced behavioral impairment involves the dopaminergic system. Front. Pharmacol. 10, 1433. doi:10.3389/fphar.2019.01433
Zhou, J., Wen, B., Xie, H., Zhang, C., Bai, Y., Cao, H., et al. (2021). Advances in the preparation and assessment of the biological activities of chitosan oligosaccharides with different structural characteristics. Food Funct. 12, 926–951. doi:10.1039/d0fo02768e
Zhou, J., Wu, J., Chen, X., Fortenbery, N., Eksioglu, E., Kodumudi, K. N., et al. (2011). Icariin and its derivative, ICT, exert anti-inflammatory, anti-tumor effects, and modulate myeloid derived suppressive cells (MDSCs) functions. Int. Immunopharmacology 11, 890–898. doi:10.1016/j.intimp.2011.01.007
Zhou, P., Du, S., Zhou, L., Sun, Z., Zhuo, L. H., He, G., et al. (2019). Tetramethylpyrazine2′Osodium ferulate provides neuroprotection against neuroinflammation and brain injury in MCAO/R rats by suppressing TLR-4/NF-κB signaling pathway. Pharmacol. Biochem. Behav. 176, 33–42. doi:10.1016/j.pbb.2018.08.010
Zhou, Y., Li, H.-q., Lu, L., Fu, D.-l., Liu, A.-j., Li, J.-h., et al. (2014). Ginsenoside Rg1 provides neuroprotection against blood brain barrier disruption and neurological injury in a rat model of cerebral ischemia/reperfusion through downregulation of aquaporin 4 expression. Phytomedicine 21, 998–1003. doi:10.1016/j.phymed.2013.12.005
Zhou, Z., Lu, J., Liu, W.-W., Manaenko, A., Hou, X., Mei, Q., et al. (2018). Advances in stroke pharmacology. Pharmacol. Ther. 191, 23–42. doi:10.1016/j.pharmthera.2018.05.012
Zhu, H.-F., Wan, D., Luo, Y., Zhou, J.-L., Chen, L., and Xu, X.-Y. (2010). Catalpol increases brain angiogenesis and up-regulates VEGF and EPO in the rat after permanent middle cerebral artery occlusion. Int. J. Biol. Sci. 6, 443–453. doi:10.7150/ijbs.6.443
Zou, J., Gao, P., Hao, X., Xu, H., Zhan, P., and Liu, X. (2018). Recent progress in the structural modification and pharmacological activities of ligustrazine derivatives. Eur. J. Med. Chem. 147, 150–162. doi:10.1016/j.ejmech.2018.01.097
Keywords: natural products, cerebral ischemia, neuroprotection, therapeutic application, mechanisms
Citation: Xie Q, Li H, Lu D, Yuan J, Ma R, Li J, Ren M, Li Y, Chen H, Wang J and Gong D (2021) Neuroprotective Effect for Cerebral Ischemia by Natural Products: A Review. Front. Pharmacol. 12:607412. doi: 10.3389/fphar.2021.607412
Received: 17 September 2020; Accepted: 08 March 2021;
Published: 22 April 2021.
Edited by:
Min Li, Hong Kong Baptist University, Hong KongReviewed by:
Hsu-Shan Huang, Taipei Medical University, TaiwanXiang Lin, The University of Hong Kong, Hong Kong
Copyright © 2021 Xie, Li, Lu, Yuan, Ma, Li, Ren, Li, Chen, Wang and Gong. This is an open-access article distributed under the terms of the Creative Commons Attribution License (CC BY). The use, distribution or reproduction in other forums is permitted, provided the original author(s) and the copyright owner(s) are credited and that the original publication in this journal is cited, in accordance with accepted academic practice. No use, distribution or reproduction is permitted which does not comply with these terms.
*Correspondence: Jian Wang, jianwang08@163.com; Daoyin Gong, 269095483@qq.com
†These authors have contributed equally to this work