- 1Department of Medical Biology, Faculty of Science, University of South Bohemia in České Budějovice, České Budějovice, Czechia
- 2Laboratory of Genomics and Proteomics of Disease Vectors, Biology Centre CAS, Institute of Parasitology, České Budějovice, Czechia
The last three decades of research into tick salivary components have revealed several proteins with important pharmacological and immunological activities. Two primary interests have driven research into tick salivary secretions: the search for suitable pathogen transmission blocking or “anti-tick” vaccine candidates and the search for novel therapeutics derived from tick salivary components. Intensive basic research in the field of tick salivary gland transcriptomics and proteomics has identified several major protein families that play important roles in tick feeding and overcoming vertebrate anti-tick responses. Moreover, these families contain members with unrealized therapeutic potential. Here we review the major tick salivary protein families exploitable in medical applications such as immunomodulation, inhibition of hemostasis and inflammation. Moreover, we discuss the potential, opportunities, and challenges in searching for novel tick-derived drugs.
Introduction
The data being generated in the high-throughput era are rapidly shifting research and development efforts toward novel and precise therapeutics that target specific pathological mechanisms and populations and diminish harmful side-effects (Paul et al., 2010; Jorgensen, 2011). Drug discovery pipelines can take a number of different forms including high-throughput screening of compound libraries or compounds similar to existing drugs, drug repurposing (Shim and Liu, 2014; Grammer and Lipsky, 2017), target-oriented molecular design, target searching using systems pharmacology approaches (Huang et al., 2014; Zhou et al., 2016), and searching for novel bioactive substances in various organisms. The latter approach is becoming increasingly attractive, as massive screening has proven to be less cost-effective than anticipated and, with rapid development in mass spectrometry, narrowing of active fractions to individual compounds is becoming easier (Wang et al., 2019). Apart from medicinal plants and/or herbal mixtures (Li and Weng, 2017), other sources – such as arthropods and parasites – have also proven to be useful (Cherniack, 2011; Ratcliffe et al., 2014; El-Tantawy, 2015). Unlike plant products that usually kill or repel herbivores and pests and have diverse effects on vital vertebrate physiology, parasitic proteins evolved to target specific vertebrate defense mechanisms. For example, the leech Hirudo officinalis produces the strongest known natural anti-coagulant hirudin, and hirudin and its artificial derivatives like bivalrudin are now used in clinical practice to treat coagulation disorders. Similarly, tick salivary proteins hold promise for the treatment of those processes they subvert, particularly immune-related diseases and hemostatic disorders.
Tick Salivary Secretions and Tick-Host-Pathogen Interactions
Ticks are obligate blood-feeding ectoparasites of vertebrates including lizards, birds, and mammals (Jongejan and Uilenberg, 2004). As such, they must cope with diverse host defense mechanisms (Ribeiro and Francischetti, 2003; Francischetti et al., 2009) triggered by the bite/injury itself and the concomitant infection. The infections transmitted by ticks can be passive, such as from pathogens like poxviruses or apicomplexa present in blood on the tick hypostome or regurgitated during feeding (Tuppurainen et al., 2011; Hammer et al., 2016), and/or active when ticks are vectors for pathogens. Ticks can transmit bacteria of the genera Borrelia, Anaplasma, Rickettsia, Francisella, and others; protozoan parasites of the genus Babesia; and several viruses, with tick-borne encephalitis virus a major tick-transmitted viral pathogen in humans (Swanson et al., 2006; Coipan et al., 2013; Berggoetz et al., 2014; Jahfari et al., 2016; Kazimirova et al., 2017; Dehhaghi et al., 2019). Early works on tick saliva (Wikel, 1982; Ribeiro et al., 1985) showed that ticks actively modulate and/or inhibit host defense mechanisms, thus enabling the tick to complete its blood meal and facilitate pathogen transmission, as reviewed elsewhere (Francischetti et al., 2009; Wikel, 2013; Kotal et al., 2015). The latter effect has been described as saliva-assisted (originally saliva-activated) transmission (SAT) (Nuttall and Labuda, 2004, 2008), and several molecules have been described as SAT factors (Kazimirova and Stibraniova, 2013). It has been hypothesized that targeting salivary factors using a vaccine could block pathogen transmission (Neelakanta and Sultana, 2015), but despite successful vaccination and RNA interference experiments, such vaccines have yet to be clinically effective for most tick species (Neelakanta and Sultana, 2015). This is at least in part due to high functional redundancy among salivary components (Chmelar et al., 2016) or because salivary proteins are often beneficial but not indispensable for pathogen transmission (Pospisilova et al., 2019). However, from another perspective, these proteins represent a diverse and abundant library of pharmacoactive molecules with potential for medical exploitation. The functions and activities of numerous salivary components have been described and reviewed elsewhere (Chmelar et al., 2012, 2017; Kazimirova and Stibraniova, 2013; Simo et al., 2017). Here we review tick salivary protein families with potential for medical use, and in doing so we highlight that tick salivary secretions represent a unique source of novel drugs that are only just starting to be exploited and translated for clinical benefit.
Tick Salivary Protein Families With Therapeutic Potential
Thanks to comprehensive transcriptomic and proteomic studies of tick salivary glands and saliva, 10s of protein families have been identified in ticks (Oleaga et al., 2007; Oliveira et al., 2013; Schwarz et al., 2013; Mudenda et al., 2014; Tirloni et al., 2014, 2015, 2017; Karim and Ribeiro, 2015; Xu et al., 2015; Yu et al., 2015; de Castro et al., 2016; Kim et al., 2016; Esteves et al., 2017; Ribeiro et al., 2017). Some have been experimentally proven to possess anti-inflammatory, anti-hemostatic, anti-complement, and/or immunomodulatory activities, as reviewed elsewhere (Chmelar et al., 2012; Kazimirova and Stibraniova, 2013). Several diverse but abundant secreted protein groups have been repeatedly identified in transcriptomes from tick salivary glands. Their experimentally evidenced activities are summarized in Figures 1, 2, and their potential medical uses are discussed in detail below.
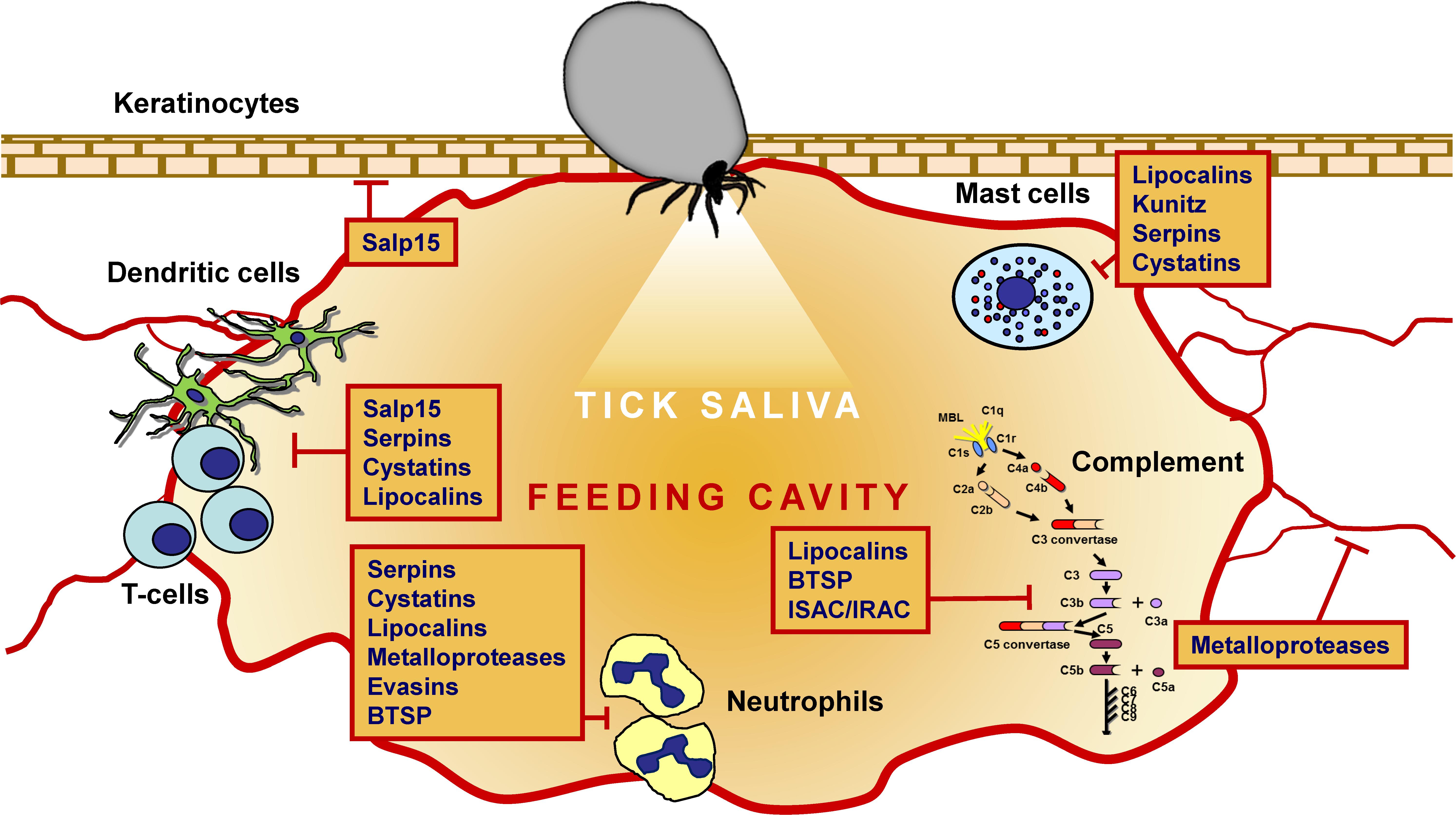
Figure 2. The immunomodulatory and anti-complement activities of the major tick-secreted protein families.
Lipocalins
Lipocalins form a large family of barrel-shaped proteins with specific folds creating pockets that usually bind hydrophobic molecules such as steroids or lipids. Lipocalins form the largest and most diverse protein family in ticks. They were originally described as histamine-binding proteins (Paesen et al., 1999) but were later found to bind other biogenic amines such as serotonin (Sangamnatdej et al., 2002; Mans et al., 2008b) and bioactive lipids (leukotrienes, thromboxanes, or cholesterol) (Keller et al., 1993; Beaufays et al., 2008; Mans and Ribeiro, 2008; Roversi et al., 2017). Apart from binding small hydrophobic molecules, tick lipocalins can also bind larger proteins such as the C5 component of the complement cascade (Nunn et al., 2005; Fredslund et al., 2008). The lipocalin from the tick Ornithodoros moubata (Coversin) displayed a therapeutic effect in disease models (Soltys et al., 2009; Romay-Penabad et al., 2014; Pischke et al., 2017) and is already being tested in clinical trials for the treatment of thrombotic microangiopathy (Brocklebank and Kavanagh, 2017; Goodship et al., 2017). The lipocalin Japanin from Rhipicephalus appendiculatus was found to modulate dendritic cell differentiation, thus altering subsequent T cell-dependent cellular responses (Preston et al., 2013). Several other tick lipocalins have been successfully tested in other disease models; for example, histamine binding lipocalin Ha24 from Hyalomma asiaticum inhibited cell recruitment and histamine secretion in a mouse experimental asthma model (Wang et al., 2016), and rEV131 and rEV504 from R. appendiculatus inhibited allergic asthma and acute respiratory distress syndrome by scavenging histamine (Couillin et al., 2004; Ryffel et al., 2005; Weston-Davies et al., 2005). Therefore, tick lipocalins are proven drug candidates that target hemostasis, complement, inflammation, and acquired immunity.
Protease Inhibitors
Endogenous protease inhibitors regulate many physiological processes in mammals, and their dysregulation leads to some serious diseases and even cancer development. Several protease inhibitor families have been identified in tick saliva. Serine protease inhibitors form four groups – Kunitz-domain inhibitors, serpins, trypsin inhibitor-like cysteine-rich domain inhibitors (TIL-domain inhibitors), and Kazal-domain inhibitors – while the cysteine protease inhibitors usually belong to the cystatin family. Tick protease inhibitors and their functions are reviewed elsewhere (Schwarz et al., 2012; Blisnick et al., 2017; Porter et al., 2017; Parizi et al., 2018), and the therapeutic potential of serpins and cystatins was outlined in our previous review (Chmelar et al., 2017). Here we discuss the therapeutic potential of the other two groups, Kunitz- and TIL-domain inhibitors.
Kunitz-Domain Protease Inhibitors
The Kunitz-domain protease inhibitors are the second largest family of secreted salivary proteins. Ticks possess Kunitz inhibitors with one to seven Kunitz domains, and most have been characterized as anti-coagulants that inhibit various proteases in the coagulation cascade (Corral-Rodriguez et al., 2009; Chmelar et al., 2012). Other family members possess anti-platelet activity due to integrin binding (see section “Disintegrins”). Their anti-platelet and anti-coagulatory properties make Kunitz inhibitors interesting as novel and target-specific drugs. Indeed, a Kunitz-domain protein Ir-CPI (Ixodes ricinus contact phase inhibitor) was shown to be a very efficient inhibitor of the contact phase of the coagulation cascade (Decrem et al., 2009), which is now being exploited in pre-clinical testing1. As well as hemostasis regulation, the Kunitz inhibitors Ixolaris and Amblyommin-X have displayed anti-cancer therapeutic potential (Carneiro-Lobo et al., 2009; Chudzinski-Tavassi et al., 2010; Barboza et al., 2015; de Souza et al., 2016).
Similar to lipocalins, Kunitz-domain tick inhibitors are a large and diverse group of proteins that could be used in drug development for human disease. Their highest potential lies in their anti-hemostatic properties, for which several Kunitz inhibitors have already been patented.
TIL-Domain Inhibitors
TIL-domain inhibitors represent another abundant group of tick salivary protease inhibitors. TIL-domain inhibitors are small (6–9 kDa), structurally conserved proteins with five disulfide bridges. Their inhibitory activity against trypsin was originally described in the parasitic nematode Ascaris lumbricoides. Proteins with the same cysteine scaffold were subsequently described as anti-coagulants in the helminth Ancylostoma caninum (Stassens et al., 1996) or in the skin secretions in the frog Bombina bombina (Mignogna et al., 1996). Moreover, they have also been identified in bee hemolymph (Bania et al., 1999) and as toxins in Scorpiops jendeki scorpion venom (Chen et al., 2013). The TIL-domain structure has been resolved, and the loop with the active P1 site is also recognized in non-tick proteins (Huang et al., 1994; Mignogna et al., 1996; Bania et al., 1999; Cierpicki et al., 2000). In addition to its anti-protease activity, scorpion TIL-domain inhibitor Sj7170 stimulated tumor growth and metastasis formation (Song et al., 2014). TIL-domain inhibitors play a regulatory role in Drosophila seminal fluid (Lung et al., 2002), and TIL domains are present in the large complex molecule of von Willebrand factor (Zhou et al., 2012), albeit with unknown function.
In tick salivary glands, TIL-domain inhibitors were the most abundant and diverse protein family with 108 members in R. appendiculatus and 64 in I. ricinus (Lieskovska et al., 2015; de Castro et al., 2016), suggesting that they are important in host defenses. Moreover, due to their conserved structure and known active site, TIL-domain inhibitors seem to be good candidates for protein engineering.
Disintegrins
Disintegrins form a large group of proteins that were originally described in snake venom as 60–80 kDa cysteine-rich proteins containing an integrin-binding motif Arg-Gly-Asp (RGD). This domain is also typical in extracellular matrix proteins and enables platelet binding via integrins and subsequent thrombus formation. RGD domains in soluble proteins such as disintegrins prevent platelet aggregation, which is crucial for the success of hematophagous parasites (Assumpcao et al., 2012). Three Kunitz domain-containing disintegrins have been functionally characterized: disagregin from O. moubata, which shows low similarity to Kunitz inhibitors and does not actually contain an RGD domain (Karczewski et al., 1994; Karczewski and Connolly, 1997); savignygrin from Ornithodoros savignyi (Mans et al., 2002a,b); and monogrins from Argas monolakensis (Mans et al., 2008a). Another group of tick disintegrins includes variabilin (Wang et al., 1996), which was the only group member characterized and later named ixodegrins (Ixodida + disintegrins) after their discovery in the Ixodes pacificus transcriptome (Francischetti et al., 2005). Variabilin was shown to block the binding of fibrinogen to integrin αIIbβ3 (Wang et al., 1996). The second tick protein characterized in the ixodegrin family was YY-39 from Ixodes scapularis, which was shown to bind integrin αIIbβ3 and thus compete with fibrinogen binding to platelets (Tang et al., 2015). Ixodegrins are structurally similar to snake venom disintegrins and usually contain RGD or KGD domains. Given their properties, they may be candidate therapeutics as novel anti-thrombotic agents.
Basic Tail Secreted Proteins (BTSPs)
The first BTSP family member was identified and described as one of 14 immunodominant antigens in I. scapularis and named Salp14 (Das et al., 2001). More homologs were subsequently found in the transcriptomes of I. scapularis (Valenzuela et al., 2002) and other tick species, where BTSP usually forms one of the most abundant and diverse putatively secreted groups (Francischetti et al., 2005; Schwarz et al., 2013; Karim and Ribeiro, 2015; de Castro et al., 2016). Salp14 and its smaller homolog Salp9Pac are anti-coagulants important for tick feeding, as evidenced by RNA interference studies in ticks (Narasimhan et al., 2002, 2004). Another BTSP family member, Ixonnexin, promoted fibrinolysis by accelerating plasminogen activation and inhibiting factor Xa (Assumpcao et al., 2018). An immunogen originally named P8 (Schuijt et al., 2011b) displayed lectin binding properties and acted as a complement inhibitor (Schuijt et al., 2011a), thus protecting Borrelia spirochetes from complement attack (Wagemakers et al., 2016).
The BTSP family contains 100s of members, some with modifications such as acidic tails or no tail instead of basic tails, which are usually composed of lysine and arginine residues (Francischetti et al., 2008). They work as anti-coagulants and specific complement inhibitors, both of which are very attractive drug targets.
Salp15
Wikel (1982) observed that tick infestation inhibited mitogen-induced guinea-pig T cell proliferation. Since, then, many effects of tick saliva on T cell function have been described (Kotal et al., 2015). The best characterized tick salivary protein with a direct effect on T cells is Salp15 from I. scapularis (Anguita et al., 2002), a member of a large family of heavily glycosylated proteins that seem to be unique to prostriate ticks such as Ixodes spp. (Wang et al., 2014). Salp15 binds specifically to the CD4 co-receptor on T cells, thus inhibiting cell signaling, reducing IL-2 production, and promoting CD4+ T cell activation (Anguita et al., 2002; Garg et al., 2006; Juncadella et al., 2007). The immunomodulatory potential of Salp15 has been tested in several in vivo models with promising therapeutic results, e.g., in inhibiting HIV infection (Juncadella et al., 2008), experimental autoimmune encephalomyelitis (Juncadella et al., 2010), transplantation rejection (Tomas-Cortazar et al., 2017), and asthma (Paveglio et al., 2007). In addition to its anti-CD4+ T cell effect, Salp15 is also a suppressor of dendritic cell function (Hovius et al., 2008; den Dunnen et al., 2009), suggesting another use in targeted therapy. In addition, Salp15 inhibited TLR2-dependent inflammation and production of antimicrobial peptides by keratinocytes (Marchal et al., 2011). Apart from its immunomodulatory activities (Juncadella and Anguita, 2009), it has also been associated with protection of Borrelia spirochetes from the immune system (Ramamoorthi et al., 2005; Dai et al., 2009).
Metalloproteases
Metalloproteases form large group of physiologically important enzymes that play a role in extracellular matrix degradation, protein shedding from the cell surface, and enzyme activation. Tick salivary metalloproteases accelerate fibrinolysis (Francischetti et al., 2003; Beaufays et al., 2008), thus their function is anti-hemostatic. Two proteins from I. scapularis from the ADAMTS family (a disintegrin and metalloprotease with thrombospondin motifs) significantly inhibited neutrophil function, broadening the range of possible metalloprotease activities (Guo et al., 2009). The abundancy and diversity of metalloproteases in tick saliva is high; however, using them as therapeutics can be problematic due to their stability and the long-term maintenance of their activity.
Evasins
Chemokines play a role in cell recruitment to inflammation sites and therefore play crucial roles in promoting inflammatory responses (Charo and Ransohoff, 2006). Evasins form a family of chemokine-binding proteins first identified in Rhipicephalus sanguineus (Frauenschuh et al., 2007; Deruaz et al., 2008). Homologs were also found in Amblyomma and Ixodes species; however, in Ixodes, the homology is very weak (Hayward et al., 2017). Evasin-1 and evasin-4 bind CC chemokine members, while evasin-3 is specific for CXC chemokines (Frauenschuh et al., 2007; Deruaz et al., 2008). Evasin-1 can inhibit neutrophil, T cell, and macrophage migration and the production of inflammatory cytokines, from which originates its therapeutic potential. Evasin-1 has shown benefit in the treatment of pulmonary fibrosis (Russo et al., 2011) and graft versus host disease (Castor et al., 2010). Evasin-4 can interact with at least 18 CC chemokines, inhibit eosinophil recruitment (Vieira et al., 2009; Deruaz et al., 2013), and was effective against post-infarction myocardial injury and remodeling (Braunersreuther et al., 2013). Evasin-3 effectively inhibited myocardial reperfusion (Montecucco et al., 2010) and neutrophil recruitment (Deruaz et al., 2008), thereby reducing atherosclerotic vulnerability for ischemic stroke (Copin et al., 2013). Evasin-3 was also shown to inhibit neutrophil-mediated inflammation in a mouse acute pancreatitis model (Montecucco et al., 2014). The therapeutic potential of evasins is reviewed in Bonvin et al. (2016).
Tick Proteins and Bioengineering
The preceding discussion highlights that tick salivary proteins can target every possible immune mechanism and therefore have the potential to be used as drugs, especially against disorders of hemostasis, immune-mediated inflammatory diseases, and also against tumor growth. Indeed, many tick molecules have been patented for these reasons (Nuttall and Paesen, 2000; Nunn, 2004; Chudzinski-Tavassi et al., 2005); moreover, Coversin has already been, and continues to be, tested in clinical trials (ClinicalTrials.gov: NCT03829449, NCT03427060, NCT02591862, NCT03588026) for paroxysmal nocturnal hemoglobinuria and microscopic and thrombotic microangiopathy (Brocklebank and Kavanagh, 2017; Goodship et al., 2017). However, the pharmacokinetics and pharmacodynamics of tick proteins can limit their therapeutic potential. Similar to any large molecule of exogenous origin, tick salivary proteins display strong immunogenicity. This can be overcome using several modifications such as PEGylation [attachment of polyethylene glycol (PEG) polymers], which should, in addition to decreasing immunogenicity, improve solubility (Veronese and Mero, 2008; Jokerst et al., 2011). Another possibility is humanization of the proteins, in which potential T cell epitopes are eliminated or changed. This approach has been used with great success for monoclonal antibodies designed predominantly for cancer treatment by the fusion of the hypervariable parts of mouse-derived monoclonal antibodies to human heavy chains (De Groot and Scott, 2007). A similar approach could be used for tick serpins, where the reactive center loop (RCL), which is responsible for serpin specificity, would be of tick origin, and the conserved serpin scaffold would be of human origin (Silverman et al., 2010; Whisstock et al., 2010). The concept of creating novel serpins by combining the RCL and scaffold from different serpins has been proven with the fusion of the furin inhibitor B8 and the mutant variant of α1-antitrypsin (Izaguirre et al., 2013, 2019). Furthermore, a novel extracellular inhibitor of human granzyme B was produced by combining mouse RCL and a human scaffold into a single chimera (Marcet-Palacios et al., 2015). Despite these promising results, there is no experimental evidence that the same approach would be successful for tick salivary molecules as well. Our understanding of the interaction between tick salivary secretion and host immune system is still incomplete, and immune tolerance is not the only obstacle in turning tick proteins into drugs.
In addition to immunogenicity, small proteins and peptides, which many tick salivary proteins are, usually have a short circulatory half-life. This problem can be solved by fusing the studied protein with the Fc fragment of human IgG. Such a fusion was performed with evasin-4 but without success (Bonvin et al., 2016). PASylation – protein conjugation with the polymeric sequence of Pro, Ala, and Ser – is a relatively new method similar to PEGylation that can prolong protein half-life in the circulation by several days (Schlapschy et al., 2013). There are ways to modify tick salivary proteins to improve their pharmacodynamics and pharmacokinetics, some of which have already been successfully used, such as PASylation of Coversin (see section “Lipocalins”), which inhibited its degradation in plasma (Kuhn et al., 2016).
Concluding Remarks
Based on experimental evidence and/or the membership of proteins to well-described families, the function of some tick proteins has been proven or at least inferred. For some families, functional knowledge is still lacking. Therefore, tick salivary proteins remain rather poorly explored with respect to their therapeutic applications. Most efforts aiming to develop new drugs from ticks focus on the two largest families, Kunitz-domain inhibitors and lipocalins, as these groups contain new members with novel functions via several genetic mechanisms (Mans et al., 2008b; Schwarz et al., 2014). However, other groups deserve attention as well, such as TIL-domain protease inhibitors, Salp15, evasins, and many others. This minireview summarizes the main protein groups to emphasize the therapeutic potential of tick salivary proteins and some of the problems faced with clinical translation.
Author Contributions
JC, JK, and AK wrote the manuscript. JC prepared the figures. MK reviewed and edited the manuscript.
Funding
This work was supported by the Grant Agency of the Czech Republic (19-14704Y to JC and 19-07247S to MK) and by the ERD Funds, projectCePaVip OPVVV (No. 384 CZ.02.1.01/0.0/0.0/16_019/0000759 to MK).
Conflict of Interest Statement
The authors declare that the research was conducted in the absence of any commercial or financial relationships that could be construed as a potential conflict of interest.
Acknowledgments
We thank the Nextgenediting for editorial support.
Footnotes
References
Anguita, J., Ramamoorthi, N., Hovius, J. W., Das, S., Thomas, V., Persinski, R., et al. (2002). Salp15, an ixodes scapularis salivary protein, inhibits CD4(+) T cell activation. Immunity 16, 849–859. doi: 10.1016/s1074-7613(02)00325-4
Assumpcao, T. C., Mizurini, D. M., Ma, D., Monteiro, R. Q., Ahlstedt, S., Reyes, M., et al. (2018). Ixonnexin from tick saliva promotes fibrinolysis by interacting with plasminogen and tissue-type plasminogen activator, and prevents arterial thrombosis. Sci. Rep. 8:4806. doi: 10.1038/s41598-018-22780-1
Assumpcao, T. C., Ribeiro, J. M., and Francischetti, I. M. (2012). Disintegrins from hematophagous sources. Toxins 4, 296–322. doi: 10.3390/toxins4050296
Bania, J., Stachowiak, D., and Polanowski, A. (1999). Primary structure and properties of the cathepsin G/chymotrypsin inhibitor from the larval hemolymph of Apis mellifera. Eur J. Biochem. 262, 680–687. doi: 10.1046/j.1432-1327.1999.00406.x
Barboza, T., Gomes, T., Mizurini, D. M., Monteiro, R. Q., Konig, S., Francischetti, I. M., et al. (2015). (99m)Tc-ixolaris targets glioblastoma-associated tissue factor: in vitro and pre-clinical applications. Thromb. Res. 136, 432–439. doi: 10.1016/j.thromres.2015.05.032
Beaufays, J., Adam, B., Menten-Dedoyart, C., Fievez, L., Grosjean, A., Decrem, Y., et al. (2008). Ir-LBP, an ixodes ricinus tick salivary LTB4-binding lipocalin, interferes with host neutrophil function. PLoS One 3:e3987. doi: 10.1371/journal.pone.0003987
Berggoetz, M., Schmid, M., Ston, D., Wyss, V., Chevillon, C., Pretorius, A. M., et al. (2014). Protozoan and bacterial pathogens in tick salivary glands in wild and domestic animal environments in South Africa. Ticks Tick Borne Dis. 5, 176–185. doi: 10.1016/j.ttbdis.2013.10.003
Blisnick, A. A., Foulon, T., and Bonnet, S. I. (2017). Serine protease inhibitors in ticks: an overview of their role in tick biology and tick-borne pathogen transmission. Front. Cell Infect. Microbiol. 7:199. doi: 10.3389/fcimb.2017.00199
Bonvin, P., Power, C. A., and Proudfoot, A. E. (2016). Evasins: therapeutic potential of a new family of chemokine-binding proteins from ticks. Front. Immunol. 7:208. doi: 10.3389/fimmu.2016.00208
Braunersreuther, V., Montecucco, F., Pelli, G., Galan, K., Proudfoot, A. E., Belin, A., et al. (2013). Treatment with the CC chemokine-binding protein Evasin-4 improves post-infarction myocardial injury and survival in mice. Thromb. Haemost. 110, 807–825. doi: 10.1160/TH13-04-0297
Brocklebank, V., and Kavanagh, D. (2017). Complement C5-inhibiting therapy for the thrombotic microangiopathies: accumulating evidence, but not a panacea. Clin. Kidney J. 10, 600–624. doi: 10.1093/ckj/sfx081
Carneiro-Lobo, T. C., Konig, S., Machado, D. E., Nasciutti, L. E., Forni, M. F., Francischetti, I. M., et al. (2009). Ixolaris, a tissue factor inhibitor, blocks primary tumor growth and angiogenesis in a glioblastoma model. J. Thromb. Haemost. 7, 1855–1864. doi: 10.1111/j.1538-7836.2009.03553.x
Castor, M. G., Rezende, B., Resende, C. B., Alessandri, A. L., Fagundes, C. T., Sousa, L. P., et al. (2010). The CCL3/macrophage inflammatory protein-1alpha-binding protein evasin-1 protects from graft-versus-host disease but does not modify graft-versus-leukemia in mice. J. Immunol. 184, 2646–2654. doi: 10.4049/jimmunol.0902614
Charo, I. F., and Ransohoff, R. M. (2006). The many roles of chemokines and chemokine receptors in inflammation. N. Engl. J. Med. 354, 610–621. doi: 10.1056/nejmra052723
Chen, Z., Wang, B., Hu, J., Yang, W., Cao, Z., Zhuo, R., et al. (2013). SjAPI, the first functionally characterized Ascaris-type protease inhibitor from animal venoms. PLoS One 8:e57529. doi: 10.1371/journal.pone.0057529
Cherniack, E. P. (2011). Bugs as drugs, part two: worms, leeches, scorpions, snails, ticks, centipedes, and spiders. Altern. Med. Rev. 16, 50–58.
Chmelar, J., Calvo, E., Pedra, J. H., Francischetti, I. M., and Kotsyfakis, M. (2012). Tick salivary secretion as a source of antihemostatics. J. Proteomics 75, 3842–3854. doi: 10.1016/j.jprot.2012.04.026
Chmelar, J., Kotal, J., Kopecky, J., Pedra, J. H. F., and Kotsyfakis, M. (2016). All for one and one for all on the tick-host battlefield. Trends Parasitol. 32, 368–377. doi: 10.1016/j.pt.2016.01.004
Chmelar, J., Kotal, J., Langhansova, H., and Kotsyfakis, M. (2017). Protease inhibitors in tick saliva: the role of serpins and cystatins in tick-host-pathogen interaction. Front. Cell Infect. Microbiol. 7:216. doi: 10.3389/fcimb.2017.00216
Chudzinski-Tavassi, A. M., De-Sa-Junior, P. L., Simons, S. M., Maria, D. A., De Souza Ventura, J., Batista, I. F., et al. (2010). A new tick Kunitz type inhibitor, Amblyomin-X, induces tumor cell death by modulating genes related to the cell cycle and targeting the ubiquitin-proteasome system. Toxicon 56, 1145–1154. doi: 10.1016/j.toxicon.2010.04.019
Chudzinski-Tavassi, A. M., Maria, D. A., Batista, I. F., and Ho, P. L. (2005). Kunitz-Type Recombinant Inhibitor. U.S. Patent No. WO2006029492A1.
Cierpicki, T., Bania, J., and Otlewski, J. (2000). NMR solution structure of Apis mellifera chymotrypsin/cathepsin G inhibitor-1 (AMCI-1): structural similarity with Ascaris protease inhibitors. Protein Sci. 9, 976–984. doi: 10.1110/ps.9.5.976
Coipan, E. C., Jahfari, S., Fonville, M., Maassen, C. B., Van Der Giessen, J., Takken, W., et al. (2013). Spatiotemporal dynamics of emerging pathogens in questing Ixodes ricinus. Front. Cell Infect. Microbiol. 3:36. doi: 10.3389/fcimb.2013.00036
Copin, J. C., Da Silva, R. F., Fraga-Silva, R. A., Capettini, L., Quintao, S., Lenglet, S., et al. (2013). Treatment with Evasin-3 reduces atherosclerotic vulnerability for ischemic stroke, but not brain injury in mice. J. Cereb. Blood Flow Metab. 33, 490–498. doi: 10.1038/jcbfm.2012.198
Corral-Rodriguez, M. A., Macedo-Ribeiro, S., Barbosa Pereira, P. J., and Fuentes-Prior, P. (2009). Tick-derived Kunitz-type inhibitors as antihemostatic factors. Insect. Biochem. Mol. Biol. 39, 579–595. doi: 10.1016/j.ibmb.2009.07.003
Couillin, I., Maillet, I., Vargaftig, B. B., Jacobs, M., Paesen, G. C., Nuttall, P. A., et al. (2004). Arthropod-derived histamine-binding protein prevents murine allergic asthma. J. Immunol. 173, 3281–3286. doi: 10.4049/jimmunol.173.5.3281
Dai, J., Wang, P., Adusumilli, S., Booth, C. J., Narasimhan, S., Anguita, J., et al. (2009). Antibodies against a tick protein, Salp15, protect mice from the Lyme disease agent. Cell Host Microbe. 6, 482–492. doi: 10.1016/j.chom.2009.10.006
Das, S., Banerjee, G., Deponte, K., Marcantonio, N., Kantor, F. S., and Fikrig, E. (2001). Salp25D, an Ixodes scapularis antioxidant, is 1 of 14 immunodominant antigens in engorged tick salivary glands. J. Infect. Dis. 184, 1056–1064. doi: 10.1086/323351
de Castro, M. H., De Klerk, D., Pienaar, R., Latif, A. A., Rees, D. J., and Mans, B. J. (2016). De novo assembly and annotation of the salivary gland transcriptome of Rhipicephalus appendiculatus male and female ticks during blood feeding. Ticks Tick Borne Dis. 7, 536–548. doi: 10.1016/j.ttbdis.2016.01.014
De Groot, A. S., and Scott, D. W. (2007). Immunogenicity of protein therapeutics. Trends Immunol. 28, 482–490. doi: 10.1016/j.it.2007.07.011
de Souza, J. G., Morais, K. L., Angles-Cano, E., Boufleur, P., De Mello, E. S., Maria, D. A., et al. (2016). Promising pharmacological profile of a Kunitz-type inhibitor in murine renal cell carcinoma model. Oncotarget 7, 62255–62266. doi: 10.18632/oncotarget.11555
Decrem, Y., Rath, G., Blasioli, V., Cauchie, P., Robert, S., Beaufays, J., et al. (2009). Ir-CPI, a coagulation contact phase inhibitor from the tick Ixodes ricinus, inhibits thrombus formation without impairing hemostasis. J. Exp. Med. 206, 2381–2395. doi: 10.1084/jem.20091007
Dehhaghi, M., Kazemi Shariat Panahi, H., Holmes, E. C., Hudson, B. J., Schloeffel, R., and Guillemin, G. J. (2019). Human tick-borne diseases in Australia. Front. Cell Infect. Microbiol. 9:3. doi: 10.3389/fcimb.2019.00003
den Dunnen, J., Gringhuis, S. I., and Geijtenbeek, T. B. (2009). Innate signaling by the C-type lectin DC-SIGN dictates immune responses. Cancer Immunol. Immunother. 58, 1149–1157. doi: 10.1007/s00262-008-0615-1
Deruaz, M., Bonvin, P., Severin, I. C., Johnson, Z., Krohn, S., Power, C. A., et al. (2013). Evasin-4, a tick-derived chemokine-binding protein with broad selectivity can be modified for use in preclinical disease models. FEBS J. 280, 4876–4887. doi: 10.1111/febs.12463
Deruaz, M., Frauenschuh, A., Alessandri, A. L., Dias, J. M., Coelho, F. M., Russo, R. C., et al. (2008). Ticks produce highly selective chemokine binding proteins with antiinflammatory activity. J. Exp. Med. 205, 2019–2031. doi: 10.1084/jem.20072689
El-Tantawy, N. L. (2015). Helminthes and insects: maladies or therapies. Parasitol. Res. 114, 359–377. doi: 10.1007/s00436-014-4260-7
Esteves, E., Maruyama, S. R., Kawahara, R., Fujita, A., Martins, L. A., Righi, A. A., et al. (2017). Analysis of the salivary gland transcriptome of unfed and partially fed amblyomma sculptum ticks and descriptive proteome of the saliva. Front. Cell Infect. Microbiol. 7:476. doi: 10.3389/fcimb.2017.00476
Francischetti, I. M., Mather, T. N., and Ribeiro, J. M. (2003). Cloning of a salivary gland metalloprotease and characterization of gelatinase and fibrin(ogen)lytic activities in the saliva of the Lyme disease tick vector Ixodes scapularis. Biochem. Biophys. Res. Commun. 305, 869–875. doi: 10.1016/s0006-291x(03)00857-x
Francischetti, I. M., Meng, Z., Mans, B. J., Gudderra, N., Hall, M., Veenstra, T. D., et al. (2008). An insight into the salivary transcriptome and proteome of the soft tick and vector of epizootic bovine abortion, Ornithodoros coriaceus. J. Proteomics 71, 493–512. doi: 10.1016/j.jprot.2008.07.006
Francischetti, I. M., My Pham, V., Mans, B. J., Andersen, J. F., Mather, T. N., Lane, R. S., et al. (2005). The transcriptome of the salivary glands of the female western black-legged tick Ixodes pacificus (Acari: Ixodidae). Insect. Biochem. Mol. Biol. 35, 1142–1161. doi: 10.1016/j.ibmb.2005.05.007
Francischetti, I. M., Sa-Nunes, A., Mans, B. J., Santos, I. M., and Ribeiro, J. M. (2009). The role of saliva in tick feeding. Front. Biosci. 14, 2051–2088. doi: 10.2741/3363
Frauenschuh, A., Power, C. A., Deruaz, M., Ferreira, B. R., Silva, J. S., Teixeira, M. M., et al. (2007). Molecular cloning and characterization of a highly selective chemokine-binding protein from the tick Rhipicephalus sanguineus. J. Biol. Chem. 282, 27250–27258. doi: 10.1074/jbc.m704706200
Fredslund, F., Laursen, N. S., Roversi, P., Jenner, L., Oliveira, C. L., Pedersen, J. S., et al. (2008). Structure of and influence of a tick complement inhibitor on human complement component 5. Nat. Immunol. 9, 753–760. doi: 10.1038/ni.1625
Garg, R., Juncadella, I. J., Ramamoorthi, N., Ashish Ananthanarayanan, S. K., Thomas, V., Rincón, M., et al. (2006). Cutting edge: CD4 is the receptor for the tick saliva immunosuppressor, Salp15. J. Immunol. 177, 6579–6583. doi: 10.4049/jimmunol.177.10.6579
Goodship, T. H. J., Pinto, F., Weston-Davies, W. H., Silva, J., Nishimura, J. I., Nunn, M. A., et al. (2017). Use of the complement inhibitor coversin to treat HSCT-associated TMA. Blood Adv. 1, 1254–1258. doi: 10.1182/bloodadvances.2016002832
Grammer, A. C., and Lipsky, P. E. (2017). Drug repositioning strategies for the identification of novel therapies for rheumatic autoimmune inflammatory diseases. Rheum. Dis. Clin. North Am. 43, 467–480. doi: 10.1016/j.rdc.2017.04.010
Guo, X., Booth, C. J., Paley, M. A., Wang, X., Deponte, K., Fikrig, E., et al. (2009). Inhibition of neutrophil function by two tick salivary proteins. Infect. Immun. 77, 2320–2329. doi: 10.1128/IAI.01507-08
Hammer, J. F., Jenkins, C., Bogema, D., and Emery, D. (2016). Mechanical transfer of Theileria orientalis: possible roles of biting arthropods, colostrum and husbandry practices in disease transmission. Parasit. Vectors 9:34. doi: 10.1186/s13071-016-1323-x
Hayward, J., Sanchez, J., Perry, A., Huang, C., Rodriguez Valle, M., Canals, M., et al. (2017). Ticks from diverse genera encode chemokine-inhibitory evasin proteins. J. Biol. Chem. 292, 15670–15680. doi: 10.1074/jbc.M117.807255
Hovius, J. W., De Jong, M. A., Den Dunnen, J., Litjens, M., Fikrig, E., Van Der Poll, T., et al. (2008). Salp15 binding to DC-SIGN inhibits cytokine expression by impairing both nucleosome remodeling and mRNA stabilization. PLoS Pathog. 4:e31. doi: 10.1371/journal.ppat.0040031
Huang, C., Zheng, C., Li, Y., Wang, Y., Lu, A., and Yang, L. (2014). Systems pharmacology in drug discovery and therapeutic insight for herbal medicines. Brief. Bioinform. 15, 710–733. doi: 10.1093/bib/bbt035
Huang, K., Strynadka, N. C., Bernard, V. D., Peanasky, R. J., and James, M. N. (1994). The molecular structure of the complex of Ascaris chymotrypsin/elastase inhibitor with porcine elastase. Structure 2, 679–689. doi: 10.1016/s0969-2126(00)00068-x
Izaguirre, G., Arciniega, M., and Quezada, A. G. (2019). Specific and selective inhibitors of proprotein convertases engineered by transferring serpin b8 reactive-site and exosite determinants of reactivity to the serpin alpha1PDX. Biochemistry 58, 1679–1688. doi: 10.1021/acs.biochem.8b01295
Izaguirre, G., Qi, L., Lima, M., and Olson, S. T. (2013). Identification of serpin determinants of specificity and selectivity for furin inhibition through studies of alpha1PDX (alpha1-protease inhibitor Portland)-serpin B8 and furin active-site loop chimeras. J. Biol. Chem. 288, 21802–21814. doi: 10.1074/jbc.M113.462804
Jahfari, S., Hofhuis, A., Fonville, M., Van Der Giessen, J., Van Pelt, W., and Sprong, H. (2016). Molecular detection of tick-borne pathogens in humans with tick bites and erythema migrans, in the Netherlands. PLoS Negl. Trop Dis. 10:e0005042. doi: 10.1371/journal.pntd.0005042
Jokerst, J. V., Lobovkina, T., Zare, R. N., and Gambhir, S. S. (2011). Nanoparticle PEGylation for imaging and therapy. Nanomedicine 6, 715–728. doi: 10.2217/nnm.11.19
Jorgensen, J. T. (2011). A challenging drug development process in the era of personalized medicine. Drug Discov. Today 16, 891–897. doi: 10.1016/j.drudis.2011.09.010
Juncadella, I. J., and Anguita, J. (2009). The immunosuppresive tick salivary protein, Salp15. Adv. Exp. Med. Biol. 666, 121–131. doi: 10.1007/978-1-4419-1601-3_10
Juncadella, I. J., Bates, T. C., Suleiman, R., Monteagudo-Mera, A., Olson, CM., Jr., Navasa, N., et al. (2010). The tick saliva immunosuppressor, Salp15, contributes to Th17-induced pathology during experimental autoimmune encephalomyelitis. Biochem. Biophys. Res. Commun. 402, 105–109. doi: 10.1016/j.bbrc.2010.09.125
Juncadella, I. J., Garg, R., Ananthnarayanan, S. K., Yengo, C. M., and Anguita, J. (2007). T-cell signaling pathways inhibited by the tick saliva immunosuppressor, Salp15. FEMS Immunol. Med. Microbiol. 49, 433–438. doi: 10.1111/j.1574-695x.2007.00223.x
Juncadella, I. J., Garg, R., Bates, T. C., Olivera, E. R., and Anguita, J. (2008). The Ixodes scapularis salivary protein, salp15, prevents the association of HIV-1 gp120 and CD4. Biochem. Biophys. Res. Commun. 367, 41–46. doi: 10.1016/j.bbrc.2007.12.104
Karczewski, J., and Connolly, T. M. (1997). The interaction of disagregin with the platelet fibrinogen receptor, glycoprotein IIb-IIIa. Biochem. Biophys. Res. Commun. 241, 744–748. doi: 10.1006/bbrc.1997.7881
Karczewski, J., Endris, R., and Connolly, T. M. (1994). Disagregin is a fibrinogen receptor antagonist lacking the Arg-Gly-Asp sequence from the tick, Ornithodoros moubata. J. Biol. Chem. 269, 6702–6708.
Karim, S., and Ribeiro, J. M. (2015). An insight into the sialome of the lone star tick, amblyomma americanum, with a glimpse on its time dependent gene expression. PLoS One 10:e0131292. doi: 10.1371/journal.pone.0131292
Kazimirova, M., and Stibraniova, I. (2013). Tick salivary compounds: their role in modulation of host defences and pathogen transmission. Front. Cell Infect. Microbiol. 3:43. doi: 10.3389/fcimb.2013.00043
Kazimirova, M., Thangamani, S., Bartikova, P., Hermance, M., Holikova, V., Stibraniova, I., et al. (2017). Tick-borne viruses and biological processes at the tick-host-virus interface. Front. Cell Infect. Microbiol. 7:339. doi: 10.3389/fcimb.2017.00339
Keller, P. M., Waxman, L., Arnold, B. A., Schultz, L. D., Condra, C., and Connolly, T. M. (1993). Cloning of the cDNA and expression of moubatin, an inhibitor of platelet aggregation. J. Biol. Chem. 268, 5450–5456.
Kim, T. K., Tirloni, L., Pinto, A. F., Moresco, J., Yates, J. R. III, Da Silva Vaz, I., et al. (2016). Ixodes scapularis tick saliva proteins sequentially secreted every 24 h during blood feeding. PLoS Negl. Trop Dis. 10:e0004323. doi: 10.1371/journal.pntd.0004323
Kotal, J., Langhansova, H., Lieskovska, J., Andersen, J. F., Francischetti, I. M., Chavakis, T., et al. (2015). Modulation of host immunity by tick saliva. J. Proteomics 128, 58–68. doi: 10.1016/j.jprot.2015.07.005
Kuhn, N., Schmidt, C. Q., Schlapschy, M., and Skerra, A. (2016). PASylated coversin, a C5-Specific complement inhibitor with extended pharmacokinetics, shows enhanced anti-hemolytic activity in vitro. Bioconjug. Chem. 27, 2359–2371. doi: 10.1021/acs.bioconjchem.6b00369
Li, F. S., and Weng, J. K. (2017). Demystifying traditional herbal medicine with modern approach. Nat. Plants 3:17109. doi: 10.1038/nplants.2017.109
Lieskovska, J., Palenikova, J., Sirmarova, J., Elsterova, J., Kotsyfakis, M., Campos Chagas, A., et al. (2015). Tick salivary cystatin sialostatin L2 suppresses IFN responses in mouse dendritic cells. Parasite Immunol. 37, 70–78. doi: 10.1111/pim.12162
Lung, O., Tram, U., Finnerty, C. M., Eipper-Mains, M. A., Kalb, J. M., and Wolfner, M. F. (2002). The Drosophila melanogaster seminal fluid protein Acp62F is a protease inhibitor that is toxic upon ectopic expression. Genetics 160, 211–224.
Mans, B. J., Andersen, J. F., Schwan, T. G., and Ribeiro, J. M. (2008a). Characterization of anti-hemostatic factors in the argasid, Argas monolakensis: implications for the evolution of blood-feeding in the soft tick family. Insect. Biochem. Mol. Biol. 38, 22–41. doi: 10.1016/j.ibmb.2007.09.002
Mans, B. J., Ribeiro, J. M., and Andersen, J. F. (2008b). Structure, function, and evolution of biogenic amine-binding proteins in soft ticks. J. Biol. Chem. 283, 18721–18733. doi: 10.1074/jbc.M800188200
Mans, B. J., Louw, A. I., and Neitz, A. W. (2002a). Disaggregation of aggregated platelets by savignygrin, a alphaIIbeta3 antagonist from Ornithodoros savignyi. Exp. Appl. Acarol. 27, 231–239.
Mans, B. J., Louw, A. I., and Neitz, A. W. (2002b). Savignygrin, a platelet aggregation inhibitor from the soft tick Ornithodoros savignyi, presents the RGD integrin recognition motif on the Kunitz-BPTI fold. J. Biol. Chem. 277, 21371–21378. doi: 10.1074/jbc.m112060200
Mans, B. J., and Ribeiro, J. M. (2008). Function, mechanism and evolution of the moubatin-clade of soft tick lipocalins. Insect. Biochem. Mol. Biol. 38, 841–852. doi: 10.1016/j.ibmb.2008.06.007
Marcet-Palacios, M., Ewen, C., Pittman, E., Duggan, B., Carmine-Simmen, K., Fahlman, R. P., et al. (2015). Design and characterization of a novel human Granzyme B inhibitor. Protein Eng. Des. Sel. 28, 9–17. doi: 10.1093/protein/gzu052
Marchal, C., Schramm, F., Kern, A., Luft, B. J., Yang, X., Schuijt, T. J., et al. (2011). Antialarmin effect of tick saliva during the transmission of Lyme disease. Infect. Immun. 79, 774–785. doi: 10.1128/IAI.00482-10
Mignogna, G., Pascarella, S., Wechselberger, C., Hinterleitner, C., Mollay, C., Amiconi, G., et al. (1996). BSTI, a trypsin inhibitor from skin secretions of Bombina bombina related to protease inhibitors of nematodes. Protein Sci. 5, 357–362. doi: 10.1002/pro.5560050220
Montecucco, F., Lenglet, S., Braunersreuther, V., Pelli, G., Pellieux, C., Montessuit, C., et al. (2010). Single administration of the CXC chemokine-binding protein Evasin-3 during ischemia prevents myocardial reperfusion injury in mice. Arterioscler. Thromb. Vasc. Biol. 30, 1371–1377. doi: 10.1161/ATVBAHA.110.206011
Montecucco, F., Mach, F., Lenglet, S., Vonlaufen, A., Gomes Quindere, A. L., Pelli, G., et al. (2014). Treatment with Evasin-3 abrogates neutrophil-mediated inflammation in mouse acute pancreatitis. Eur. J. Clin. Invest. 44, 940–950. doi: 10.1111/eci.12327
Mudenda, L., Pierle, S. A., Turse, J. E., Scoles, G. A., Purvine, S. O., Nicora, C. D., et al. (2014). Proteomics informed by transcriptomics identifies novel secreted proteins in Dermacentor andersoni saliva. Int. J. Parasitol. 44, 1029–1037. doi: 10.1016/j.ijpara.2014.07.003
Narasimhan, S., Koski, R. A., Beaulieu, B., Anderson, J. F., Ramamoorthi, N., Kantor, F., et al. (2002). A novel family of anticoagulants from the saliva of Ixodes scapularis. Insect. Mol. Biol. 11, 641–650. doi: 10.1046/j.1365-2583.2002.00375.x
Narasimhan, S., Montgomery, R. R., Deponte, K., Tschudi, C., Marcantonio, N., Anderson, J. F., et al. (2004). Disruption of Ixodes scapularis anticoagulation by using RNA interference. Proc. Natl. Acad. Sci. U.S.A. 101, 1141–1146. doi: 10.1073/pnas.0307669100
Neelakanta, G., and Sultana, H. (2015). Transmission-blocking vaccines: focus on anti-vector vaccines against tick-borne diseases. Arch. Immunol. Ther. Exp. 63, 169–179. doi: 10.1007/s00005-014-0324-8
Nunn, M. A., Sharma, A., Paesen, G. C., Adamson, S., Lissina, O., Willis, A. C., et al. (2005). Complement inhibitor of C5 activation from the soft tick Ornithodoros moubata. J. Immunol. 174, 2084–2091. doi: 10.4049/jimmunol.174.4.2084
Nuttall, P. A., and Labuda, M. (2004). Tick-host interactions: saliva-activated transmission. Parasitology 129, S177–S189.
Nuttall, P. A., and Labuda, M. (2008). “Saliva-assisted transmission of tick-borne pathogens,” in Ticks: Biology, Disease and Control, eds A. S. Bowman and P. A. Nuttall (Cambridge: Cambridge University Press), 205–219. doi: 10.1017/cbo9780511551802.011
Oleaga, A., Escudero-Poblacion, A., Camafeita, E., and Perez-Sanchez, R. (2007). A proteomic approach to the identification of salivary proteins from the argasid ticks Ornithodoros moubata and Ornithodoros erraticus. Insect. Biochem. Mol. Biol. 37, 1149–1159. doi: 10.1016/j.ibmb.2007.07.003
Oliveira, C. J., Anatriello, E., De Miranda-Santos, I. K., Francischetti, I. M., Sa-Nunes, A., Ferreira, B. R., et al. (2013). Proteome of Rhipicephalus sanguineus tick saliva induced by the secretagogues pilocarpine and dopamine. Ticks Tick Borne Dis. 4, 469–477. doi: 10.1016/j.ttbdis.2013.05.001
Paesen, G. C., Adams, P. L., Harlos, K., Nuttall, P. A., and Stuart, D. I. (1999). Tick histamine-binding proteins: isolation, cloning, and three-dimensional structure. Mol. Cell 3, 661–671. doi: 10.1016/s1097-2765(00)80359-7
Parizi, L. F., Ali, A., Tirloni, L., Oldiges, D. P., Sabadin, G. A., Coutinho, M. L., et al. (2018). Peptidase inhibitors in tick physiology. Med. Vet. Entomol. 32, 129–144. doi: 10.1111/mve.12276
Paul, S. M., Mytelka, D. S., Dunwiddie, C. T., Persinger, C. C., Munos, B. H., Lindborg, S. R., et al. (2010). How to improve R&D productivity: the pharmaceutical industry’s grand challenge. Nat. Rev. Drug Discov. 9, 203–214.
Paveglio, S. A., Allard, J., Mayette, J., Whittaker, L. A., Juncadella, I., Anguita, J., et al. (2007). The tick salivary protein, Salp15, inhibits the development of experimental asthma. J. Immunol. 178, 7064–7071. doi: 10.4049/jimmunol.178.11.7064
Pischke, S. E., Gustavsen, A., Orrem, H. L., Egge, K. H., Courivaud, F., Fontenelle, H., et al. (2017). Complement factor 5 blockade reduces porcine myocardial infarction size and improves immediate cardiac function. Basic Res. Cardiol. 112:20. doi: 10.1007/s00395-017-0610-9
Porter, L. M., Radulovic, Z. M., and Mulenga, A. (2017). A repertoire of protease inhibitor families in Amblyomma americanum and other tick species: inter-species comparative analyses. Parasit. Vectors 10:152. doi: 10.1186/s13071-017-2080-1
Pospisilova, T., Urbanova, V., Hes, O., Kopacek, P., Hajdusek, O., and Sima, R. (2019). Tracking of Borrelia afzelii transmission from infected Ixodes ricinus nymphs to mice. Infect. Immun. 87:e00896-18. doi: 10.1128/IAI.00896-18
Preston, S. G., Majtan, J., Kouremenou, C., Rysnik, O., Burger, L. F., Cabezas Cruz, A., et al. (2013). Novel immunomodulators from hard ticks selectively reprogramme human dendritic cell responses. PLoS Pathog. 9:e1003450. doi: 10.1371/journal.ppat.1003450
Ramamoorthi, N., Narasimhan, S., Pal, U., Bao, F., Yang, X. F., Fish, D., et al. (2005). The Lyme disease agent exploits a tick protein to infect the mammalian host. Nature 436, 573–577. doi: 10.1038/nature03812
Ratcliffe, N., Azambuja, P., and Mello, C. B. (2014). Recent advances in developing insect natural products as potential modern day medicines. Evid. Based Complement. Alternat. Med. 2014:904958. doi: 10.1155/2014/904958
Ribeiro, J. M., and Francischetti, I. M. (2003). Role of arthropod saliva in blood feeding: sialome and post-sialome perspectives. Annu. Rev. Entomol. 48, 73–88. doi: 10.1146/annurev.ento.48.060402.102812
Ribeiro, J. M., Makoul, G. T., Levine, J., Robinson, D. R., and Spielman, A. (1985). Antihemostatic, antiinflammatory, and immunosuppressive properties of the saliva of a tick, Ixodes dammini. J. Exp. Med. 161, 332–344. doi: 10.1084/jem.161.2.332
Ribeiro, J. M., Slovak, M., and Francischetti, I. M. (2017). An insight into the sialome of Hyalomma excavatum. Ticks Tick Borne Dis. 8, 201–207. doi: 10.1016/j.ttbdis.2016.08.011
Romay-Penabad, Z., Carrera Marin, A. L., Willis, R., Weston-Davies, W., Machin, S., Cohen, H., et al. (2014). Complement C5-inhibitor rEV576 (coversin) ameliorates in-vivo effects of antiphospholipid antibodies. Lupus 23, 1324–1326. doi: 10.1177/0961203314546022
Roversi, P., Johnson, S., Preston, S. G., Nunn, M. A., Paesen, G. C., Austyn, J. M., et al. (2017). Structural basis of cholesterol binding by a novel clade of dendritic cell modulators from ticks. Sci. Rep. 7:16057. doi: 10.1038/s41598-017-16413-2
Russo, R. C., Alessandri, A. L., Garcia, C. C., Cordeiro, B. F., Pinho, V., Cassali, G. D., et al. (2011). Therapeutic effects of evasin-1, a chemokine binding protein, in bleomycin-induced pulmonary fibrosis. Am. J. Respir. Cell Mol. Biol. 45, 72–80. doi: 10.1165/rcmb.2009-0406OC
Ryffel, B., Couillin, I., Maillet, I., Schnyder, B., Paesen, G. C., Nuttall, P., et al. (2005). Histamine scavenging attenuates endotoxin-induced acute lung injury. Ann. N. Y. Acad. Sci. 1056, 197–205. doi: 10.1196/annals.1352.034
Sangamnatdej, S., Paesen, G. C., Slovak, M., and Nuttall, P. A. (2002). A high affinity serotonin- and histamine-binding lipocalin from tick saliva. Insect. Mol. Biol. 11, 79–86. doi: 10.1046/j.0962-1075.2001.00311.x
Schlapschy, M., Binder, U., Borger, C., Theobald, I., Wachinger, K., Kisling, S., et al. (2013). PASylation: a biological alternative to PEGylation for extending the plasma half-life of pharmaceutically active proteins. Protein Eng. Des. Sel. 26, 489–501. doi: 10.1093/protein/gzt023
Schuijt, T. J., Coumou, J., Narasimhan, S., Dai, J., Deponte, K., Wouters, D., et al. (2011a). A tick mannose-binding lectin inhibitor interferes with the vertebrate complement cascade to enhance transmission of the lyme disease agent. Cell Host Microbe. 10, 136–146. doi: 10.1016/j.chom.2011.06.010
Schuijt, T. J., Narasimhan, S., Daffre, S., Deponte, K., Hovius, J. W., Van’t Veer, C., et al. (2011b). Identification and characterization of Ixodes scapularis antigens that elicit tick immunity using yeast surface display. PLoS One 6:e15926. doi: 10.1371/journal.pone.0015926
Schwarz, A., Cabezas-Cruz, A., Kopecky, J., and Valdes, J. J. (2014). Understanding the evolutionary structural variability and target specificity of tick salivary Kunitz peptides using next generation transcriptome data. BMC Evol. Biol. 14:4. doi: 10.1186/1471-2148-14-4
Schwarz, A., Valdes, J. J., and Kotsyfakis, M. (2012). The role of cystatins in tick physiology and blood feeding. Ticks Tick Borne Dis. 3, 117–127. doi: 10.1016/j.ttbdis.2012.03.004
Schwarz, A., Von Reumont, B. M., Erhart, J., Chagas, A. C., Ribeiro, J. M., and Kotsyfakis, M. (2013). De novo Ixodes ricinus salivary gland transcriptome analysis using two next-generation sequencing methodologies. FASEB J. 27, 4745–4756. doi: 10.1096/fj.13-232140
Shim, J. S., and Liu, J. O. (2014). Recent advances in drug repositioning for the discovery of new anticancer drugs. Int. J. Biol. Sci. 10, 654–663. doi: 10.7150/ijbs.9224
Silverman, G. A., Whisstock, J. C., Bottomley, S. P., Huntington, J. A., Kaiserman, D., Luke, C. J., et al. (2010). Serpins flex their muscle: I. Putting the clamps on proteolysis in diverse biological systems. J. Biol. Chem. 285, 24299–24305. doi: 10.1074/jbc.R110.112771
Simo, L., Kazimirova, M., Richardson, J., and Bonnet, S. I. (2017). The essential role of tick salivary glands and saliva in tick feeding and pathogen transmission. Front. Cell Infect. Microbiol. 7:281. doi: 10.3389/fcimb.2017.00281
Soltys, J., Kusner, L. L., Young, A., Richmonds, C., Hatala, D., Gong, B., et al. (2009). Novel complement inhibitor limits severity of experimentally myasthenia gravis. Ann. Neurol. 65, 67–75. doi: 10.1002/ana.21536
Song, Y., Gong, K., Yan, H., Hong, W., Wang, L., Wu, Y., et al. (2014). Sj7170, a unique dual-function peptide with a specific alpha-chymotrypsin inhibitory activity and a potent tumor-activating effect from scorpion venom. J. Biol. Chem. 289, 11667–11680. doi: 10.1074/jbc.M113.540419
Stassens, P., Bergum, P. W., Gansemans, Y., Jespers, L., Laroche, Y., Huang, S., et al. (1996). Anticoagulant repertoire of the hookworm Ancylostoma caninum. Proc. Natl. Acad. Sci. U.S.A. 93, 2149–2154. doi: 10.1073/pnas.93.5.2149
Swanson, S. J., Neitzel, D., Reed, K. D., and Belongia, E. A. (2006). Coinfections acquired from ixodes ticks. Clin. Microbiol. Rev. 19, 708–727. doi: 10.1128/cmr.00011-06
Tang, J., Fang, Y., Han, Y., Bai, X., Yan, X., Zhang, Y., et al. (2015). YY-39, a tick anti-thrombosis peptide containing RGD domain. Peptides 68, 99–104. doi: 10.1016/j.peptides.2014.08.008
Tirloni, L., Islam, M. S., Kim, T. K., Diedrich, J. K., Yates, J. R. III, Pinto, A. F., et al. (2015). Saliva from nymph and adult females of Haemaphysalis longicornis: a proteomic study. Parasit. Vectors 8:338. doi: 10.1186/s13071-015-0918-y
Tirloni, L., Kim, T. K., Pinto, A. F. M., Yates, J. R. III, and Da Silva Vaz, I. Jr., et al. (2017). Tick-host range adaptation: changes in protein profiles in unfed adult Ixodes scapularis and Amblyomma americanum saliva stimulated to feed on different hosts. Front. Cell Infect. Microbiol. 7:517. doi: 10.3389/fcimb.2017.00517
Tirloni, L., Reck, J., Terra, R. M., Martins, J. R., Mulenga, A., Sherman, N. E., et al. (2014). Proteomic analysis of cattle tick Rhipicephalus (Boophilus) microplus saliva: a comparison between partially and fully engorged females. PLoS One 9:e94831. doi: 10.1371/journal.pone.0094831
Tomas-Cortazar, J., Martin-Ruiz, I., Barriales, D., Pascual-Itoiz, M. A., De Juan, V. G., Caro-Maldonado, A., et al. (2017). The immunosuppressive effect of the tick protein, Salp15, is long-lasting and persists in a murine model of hematopoietic transplant. Sci. Rep. 7:10740. doi: 10.1038/s41598-017-11354-2
Tuppurainen, E. S., Stoltsz, W. H., Troskie, M., Wallace, D. B., Oura, C. A., Mellor, P. S., et al. (2011). A potential role for ixodid (hard) tick vectors in the transmission of lumpy skin disease virus in cattle. Transbound. Emerg. Dis. 58, 93–104. doi: 10.1111/j.1865-1682.2010.01184.x
Valenzuela, J. G., Francischetti, I. M., Pham, V. M., Garfield, M. K., Mather, T. N., and Ribeiro, J. M. (2002). Exploring the sialome of the tick Ixodes scapularis. J. Exp. Biol. 205, 2843–2864.
Veronese, F. M., and Mero, A. (2008). The impact of PEGylation on biological therapies. BioDrugs 22, 315–329. doi: 10.2165/00063030-200822050-00004
Vieira, A. T., Fagundes, C. T., Alessandri, A. L., Castor, M. G., Guabiraba, R., Borges, V. O., et al. (2009). Treatment with a novel chemokine-binding protein or eosinophil lineage-ablation protects mice from experimental colitis. Am. J. Pathol. 175, 2382–2391. doi: 10.2353/ajpath.2009.090093
Wagemakers, A., Coumou, J., Schuijt, T. J., Oei, A., Nijhof, A. M., Van ’t Veer, C., et al. (2016). An Ixodes ricinus tick salivary lectin pathway inhibitor protects borrelia burgdorferi sensu lato from human complement. Vector Borne Zoonotic Dis. 16, 223–228. doi: 10.1089/vbz.2015.1901
Wang, X., Coons, L. B., Taylor, D. B., Stevens, S. E. Jr., and Gartner, T. K. (1996). Variabilin, a novel RGD-containing antagonist of glycoprotein IIb-IIIa and platelet aggregation inhibitor from the hard tick Dermacentor variabilis. J. Biol. Chem. 271, 17785–17790. doi: 10.1074/jbc.271.30.17785
Wang, X., Huang, Y., Niu, S. B., Jiang, B. G., Jia, N., Van Der Geest, L., et al. (2014). Genetic diversity of Salp15 in the Ixodes ricinus complex (Acari: Ixodidae). PLoS One 9:e94131. doi: 10.1371/journal.pone.0094131
Wang, X. J., Ren, J. L., Zhang, A. H., Sun, H., Yan, G. L., Han, Y., et al. (2019). Novel applications of mass spectrometry-based metabolomics in herbal medicines and its active ingredients: current evidence. Mass Spectrom Rev. [Epub ahead of print]
Wang, Y., Li, Z., Zhou, Y., Cao, J., Zhang, H., Gong, H., et al. (2016). Specific histamine binding activity of a new lipocalin from Hyalomma asiaticum (Ixodidae) and therapeutic effects on allergic asthma in mice. Parasit. Vectors 9:506. doi: 10.1186/s13071-016-1790-0
Weston-Davies, W., Couillin, I., Schnyder, S., Schnyder, B., Moser, R., Lissina, O., et al. (2005). Arthropod-derived protein EV131 inhibits histamine action and allergic asthma. Ann. N. Y. Acad. Sci. 1056, 189–196. doi: 10.1196/annals.1352.009
Whisstock, J. C., Silverman, G. A., Bird, P. I., Bottomley, S. P., Kaiserman, D., Luke, C. J., et al. (2010). Serpins flex their muscle: II. Structural insights into target peptidase recognition, polymerization, and transport functions. J. Biol. Chem. 285, 24307–24312. doi: 10.1074/jbc.R110.141408
Wikel, S. (2013). Ticks and tick-borne pathogens at the cutaneous interface: host defenses, tick countermeasures, and a suitable environment for pathogen establishment. Front. Microbiol. 4:337. doi: 10.3389/fmicb.2013.00337
Wikel, S. K. (1982). Influence of Dermacentor andersoni infestation on lymphocyte responsiveness to mitogens. Ann. Trop Med. Parasitol. 76, 627–632. doi: 10.1080/00034983.1982.11687593
Xu, X. L., Cheng, T. Y., Yang, H., Yan, F., and Yang, Y. (2015). De novo sequencing, assembly and analysis of salivary gland transcriptome of Haemaphysalis flava and identification of sialoprotein genes. Infect. Genet. Evol. 32, 135–142. doi: 10.1016/j.meegid.2015.03.010
Yu, X., Gong, H., Zhou, Y., Zhang, H., Cao, J., and Zhou, J. (2015). Differential sialotranscriptomes of unfed and fed Rhipicephalus haemaphysaloides, with particular regard to differentially expressed genes of cysteine proteases. Parasit. Vectors 8:597. doi: 10.1186/s13071-015-1213-7
Zhou, W., Wang, Y., Lu, A., and Zhang, G. (2016). Systems pharmacology in small molecular drug discovery. Int. J. Mol. Sci. 17:246. doi: 10.3390/ijms17020246
Keywords: ticks, therapeutics, immunomodulation, salivary proteins, anti-inflammatory proteins, hemostasis
Citation: Chmelař J, Kotál J, Kovaříková A and Kotsyfakis M (2019) The Use of Tick Salivary Proteins as Novel Therapeutics. Front. Physiol. 10:812. doi: 10.3389/fphys.2019.00812
Received: 25 April 2019; Accepted: 11 June 2019;
Published: 26 June 2019.
Edited by:
Itabajara Da Silva Vaz Jr., Federal University of Rio Grande do Sul, BrazilReviewed by:
Nathalie Boulanger, Université de Strasbourg, FranceTae Kim, Texas A&M University, United States
Copyright © 2019 Chmelař, Kotál, Kovaříková and Kotsyfakis. This is an open-access article distributed under the terms of the Creative Commons Attribution License (CC BY). The use, distribution or reproduction in other forums is permitted, provided the original author(s) and the copyright owner(s) are credited and that the original publication in this journal is cited, in accordance with accepted academic practice. No use, distribution or reproduction is permitted which does not comply with these terms.
*Correspondence: Michail Kotsyfakis, mich_kotsyfakis@yahoo.com