- 1Key Laboratory of Grassland Ecosystem of Ministry of Education, and Sino-U.S. Centers for Grazingland Ecosystem Sustainability, College of Grassland Science, Gansu Agricultural University, Lanzhou, China
- 2College of Plant Protection, Gansu Agricultural University/Biocontrol Engineering Laboratory of Crop Diseases and Pests of Gansu Province, Lanzhou, China
- 3State Key Laboratory of Green Pesticide and Agricultural Bioengineering, Ministry of Education, Guizhou University, Guiyang, China
To study the physiological mechanisms of Neoseiulus barkeri in response to short-term heat stress, the eggs and the emerged adults were exposed to 38, 40, and 42°C, 85% ± 5%RH,16 h:8 h (L:D) for 2, 4, and 6 h. The activities of superoxide dismutase (SOD), peroxidase (POD), and catalase (CAT) as well as the protein content of N. barkeri were examined. All treatments caused significant different changes compared to the untreated control. The protein content increased as the temperature increased, while it showed different changing trends with the prolongation of exposure duration. The enzymatic activity of SOD, CAT, and POD was significantly affected by the temperature treatment. Both the maximum and minimum level of the three enzymes after a short-term heat stress differed significantly to the control group (p < 0.05). The highest values of three enzymatic activities were all obtained at 40°C-4 h. Person correlation analysis indicates that the high temperature was the primary factor affecting the enzymatic activity, while the exposure duration of the heat stress was the secondary factor. In general, the short-term heat stress increased the protein content of Neoseiulus barkeri and up-regulated the expression of SOD, CAT, and POD activities as well.
Introduction
Species of phytoseiid mites are important biological control agents against several mites and small insect pests of fruits, vegetables, and other crop species worldwide in the agriculture industry (Opit et al., 2004; Arthurs et al., 2009; Juan-Blasco et al., 2012; McMurtry et al., 2013). However, their growth and behavior are easily interfered by the ambient temperature (Hart et al., 2002; Palyvos and Emmanouel, 2009; Ghazy et al., 2012). In the context of global warming, the relationship between development rate and temperature has been used to forecast the geographical distribution of mite populations (Perring et al., 1984; Hoffmann and Sgrò, 2011). In the optimum temperature range, phytoseiid mites develop quickly, and have a high survival rate and lay more eggs (Emmert et al., 2008; Gadino and Walton, 2012). In contrary, the sudden rise of the ambient temperature will cause heat stress and exert some negative influence on the population dynamics, and lead to not only the rapid death of insects and mites, but also a decrease, even the loss, of reproduction ability (Michael and Whiting, 1996; Hoffmann et al., 2003; Cui et al., 2008).
Neoseiulus barkeri Hughes (Acari: Phytoseiidae), as the vital commercially produced phytoseiid mite in China, has played a key role in the control of many pest mites in recent years (Li et al., 2021). One of the deleterious consequences when N. barkeri are exposed to heat stress is the production of reactive oxygen species (ROS), i.e., hydroxyl radicals (HO•−) and superoxide anion radicals (O2 •−), as well as non-radicals, such as hydrogen peroxide (H2O2) and singlet oxygen (1O2) (Yang et al., 2010; Ray et al., 2012). In normal situations, a balance exists between the generation of ROS and the antioxidant processes. However, increased levels of ROS generated inside living organisms disrupt the oxidative balance and lead to the oxidative damage. (Gechev et al., 2006; Lopez-Martinez et al., 2008). Surplus ROS, which are highly reactive and deleterious, can result in lipid peroxidation disrupting cell membrane fluidity, nucleic acids, proteins, lipids, and finally leading to apoptosis (Green and Reed, 1998; Lu et al., 2017).
To prevent damage from the ROS, mites have developed antioxidant defense mechanisms and these systems have both enzymatic and non-enzymatic components (Felton and Summers, 1995; Sorensen et al., 2001). Major components of the antioxidant enzymes of insects include superoxide dismutase (SOD) (Yucel and Kayis, 2019), catalase (CAT) (Lu et al., 2014), peroxidase (POD) (Gill and Tuteja, 2010), glutathione-S-transferases (GST) (Dubovskiy et al., 2008), polyphenol oxidase (PPO) (Tamás et al., 2017) as well as ascorbate peroxidase (APX) (Emre et al., 2013). It was reported that SOD, CAT, and POD, which are used as primary antioxidant enzymes to eliminate the ROS so as to maintain free radicals at a low level to prevent the toxic effects to living organisms (Wang et al., 2001). SOD scavenges free radicals to form H2O2 and removes the superoxide anion radical (O2 •−) to prevent cells from being damaged. Whereas POD and CAT decompose H2O2, resulting in a low level of free radicals which are unable to exert a toxic effect and protecting the intact structure and function of cell membranes (Wang and Li, 2002). The change in the antioxidant enzyme activity is a significant bio indicator because of the role antioxidant enzymes play in maintaining the oxidative balance (Coskun et al., 2020).
The changes in antioxidant enzyme activity are closely associated with external stimulation and xenobiotics (e.g., pesticides, heavy metal, and high temperature) (Li et al., 2021). However, evolutionary adaptation can be rapid and may help species to counter stressful conditions or to realize ecological opportunities arising from the climate change (Bale, 2002). The evolution of insect and mite adaptability to extreme temperatures enables these organisms to withstand the effects of this environmental stress, thus supporting their survival and reproduction (Hoffmann et al., 2003).
We have found that the short-term heat stress does exert a negative effect on the longevity, reproduction characteristics, and functional response of N. barkeri. (Li et al., 2021; Li et al., 2022). In this study, the physiological mechanism of N. barkeri in the response to the short-term heat stress based on relevant antioxidant enzymes was conducted, among which the eggs and the emerged adults were treated at 38, 40, and 42°C for 2, 4, and 6 h, then the changes in the enzyme activity were tested, which aims to clarify the effects of different short-term heat stresses and exposure durations on three protective enzymes in the body of N. barkeri. The results would provide better understanding of revealing the physiological response mechanism of N. barkeri to the short-term heat stress and guidance for releasing as biological agents, and improvement in the practice of integrated control of spider mites as well.
Materials and methods
Mites
The initial predatory mite population of Neoseiulus barkeri and their prey, bran mites Aleuroglyphus ovatus (Acari: Sarcoptiformes), used in this study were all provided by the Institute of Fruit Tree Research in Changli, Hebei province, China. They were maintained in a climatic chamber at 25 ± 1°C, 75 ± 5% RH, L: D = 16 h: 8 h.
Newly emerged females (<24 h) were treated at 38, 40, and 42°C treated for 2, 4, and 6 h. There were 9 treatments in total (3 temperatures x 3 exposure durations). A total of six hundred survived females from each treatment were collected for the measurement of the enzyme activity. The control group was kept at room temperature (25 ± 1°C). Each treatment was replicated three times.
The assay kits
The assay kits of superoxide (SOD), catalase (CAT), and peroxidase (POD) used in this experiment were all provided by Sino Best Biological Technology Co., Ltd, Shanghai, China.
Preparation of the enzyme solution
The preparation of assay samples was based on the methods described by Lu et al. (2014) with some minor changes. After the short-term heat stress, 600 females of N. barkeri from each treatment were placed into a 2 ml centrifugal tube. A total of 1.5 ml of phosphate buffer (PBS, 0.05 M, pH 7.0) was added as an extract solution and rapidly triturated with the samples. The mixture was then centrifuged at 10,000 rpm for 15 min at 4°C. The supernatant was collected and stored at −80°C until used as the sample solution.
Protein content determination of adults
The protein contents of sample solution were determined with the Coomassie blue method described by Bradford (1976).
The regents were sequentially added to a 96-well plate. The plate was placed in 25°C-water bath for 10 min. Then, the mixture was oscillated 120 s in a full-wavelength spectrophotometer, and the OD values of each well were read at λ = 595 nm (OD595).
Superoxide dismutase activity assay
The regents supplied with the kit and the enzyme solution were sequentially added to a 1.5 ml glass cuvette. In the negative control, we replaced the enzyme solution with the same volume phosphate buffer (PBS, 0.05 M, pH 7.0). The cuvettes were placed in a 25°C-water bath for 30 min, and then the absorbance (OD560) was immediately measured and calculated using the amount of enzyme required for 50% inhibition as an enzyme activity unit (U). All the cuvettes were read at the same time. Enzyme activity was expressed as the change in the OD560 value per unit of time and unit of protein. This assay was replicated three times.
Catalase activity assay
Methods were performed according to the instruction in the CAT assay kit. In a 1.5 ml glass cuvette, regents supplied by the kit and enzyme solution were sequentially added and mixed. In the negative control, we replaced the enzyme solution with the same volume phosphate buffer (PBS, 0.05 M, pH 7.0). The absorbances (A1) at 5 s and (A2) at 1 min were obtained, respectively (OD240). All the cuvettes were read at the same time. The degradation of 1 μmol H2O2 in 1 mg histone per minute was defined as an enzyme activity unit. This assay was replicated three times.
Peroxidase activity assay
Methods were performed according to the instruction in the POD assay kit . The regents supplied by the kit were sequentially added to 1.5 ml glass cuvette. In the negative control, we replaced the enzyme solution with the same volume phosphate buffer (PBS, 0.05 M, pH 7.0). The instant absorbances (A1) at 30 s and A2 at 90 s were recorded (OD470). All the cuvettes were read at the same time. A change in absorbance at 470 nm of 0.01 per minute in 1 ml solution was defined as the enzyme activity unit. This assay was replicated three times.
Statistical analysis
We used the online software statistical product and service Software automatically (SPSSAU 22.0, Qingsi Technology Co., LTD, Beijing, China) to detect and handle outliers before starting data analysis. The range of the box-plots were made to calculate and automatically mark the minimum and maximum estimates of the raw data. If the data exceed this range, they were regarded as outliers and deleted.
All the data were performed using SPSS software (IBM SPSS Statistics 24, IBM Corporation, Somers, NY, United States). A two-way ANOVA analysis was conducted to determine the interaction between the temperature and heat stress duration (followed by Tukey HSD post-hoc tests, p < 0.05). The general linear model (GML) procedure was used to analyze the means when a significant interaction was shown between the temperature and duration. In addition, the one-way ANOVA (follwed by Duncan’s new multiple range method, p < 0.05) was applied to test the differences among the protein content and enzyme activity. The correlation procedure in SPSS software was used to calculate the coefficients between the dependent (enzyme activity) and independent factor (temperature and exposure duration). The standard curve of bovine serum albumin calculation and figure fabrication were both conducted by GraphPad Prism 5.
Results
The two-way ANOVA analysis indicated that the protein content was significantly affected by the temperature (F (2, 81) = 218.303, p < 0.001) and the treatment duration (F (2, 81) = 12.320, p = 0.032). The interaction between the temperature and duration was also significant (F (4, 81) = 6.298, p < 0.001).
The protein content of N. barkeri females after high temperatures generally presented the variation tendency of increasing with rise of temperature are shown in Table 1. At 38°C, the protein content first increased, then decreased and then increased again, which made the value reached 0.469 mg/g from 0.165 mg/g. At 40°C, it continued to rise and reached the maximum level of 0.553 mg/g at 40°C-4 h (F (1, 18) = 216.269, p < 0.001), which is more than three times higher than the control of 0.165 mg/g. Then, it descended to 0.354 mg/g but still had a significant difference with that of the control group (F (1, 18) = 151.124, p = 0.002).
Superoxide dismutase activity
Variance analysis showed that the SOD activity was significantly affected by temperature (F (2, 81) = 50.257, p < 0.001), treatment duration (F (2, 81) = 1.595, p = 0.006) as well as their interaction (F (4, 81) = 1.228, p < 0.001).
SOD activity changed differently at different temperature (Figure 1). At 38°C, it first increased to 30.55 U/mg·prot from 25.64 U/mg·prot. After slight decreases (F (1, 18) = 20.869, p = 0.019) it went up again, its value reached to 29.52 U/mg·prot. However, it continued rising in the first 4 h at 40°C and reached the maximum level 35.30 U/mg·prot at 40°C- 4 h (F (1, 18) = 56.201, p < 0.001), then, it dropped to 28.90 U/mg·prot but still had a significant difference from that of control (F (1, 18) = 13.623, p = 0.026).
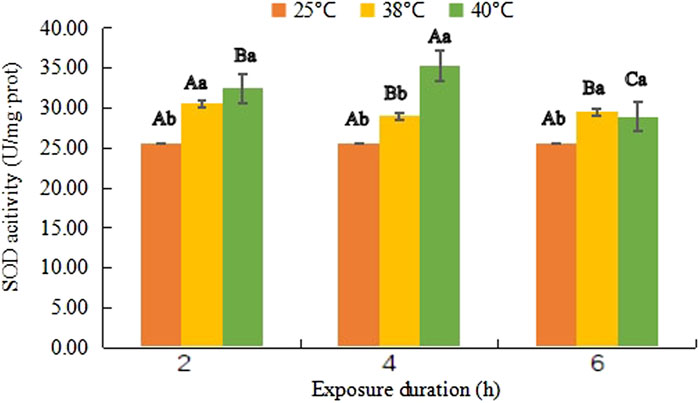
FIGURE 1. Superoxide dismutase (SOD) activity (mean activity value ± SD) in adult females of Neoseiulus barkeri after the short-term heat stress. Different capitals indicate significant differences within the different exposure duration at the same temperature, while different lowercases show significant differences within the same exposure duration at different temperature (Duncan’s new multiple range method, p < 0.05).
Table 2 showed the coefficients between the temperature and SOD activity, and the duration and SOD activity were 0.841 and -0.153, respectively. The correlation between the temperature and SOD activity was proved to be positive due to the positive value. On the contrary, the correlation between duration and SOD activity was negative. These results indicate that temperature was the major factor that affected the SOD activity in this experiment. No correlation was found between the two independent variables (temperature and duration), which indicated that they were mutually independent in this experiment.
Catalase activity
As shown in Figure 2, the CAT enzyme activity reached a maximum value (17.03 U/mg·prot) after 2 h of treatment at 38°C, and it decreased with the prolongation of treatment time, but was still higher than that of the control group after 6 h of heat treatment (F (1, 18) = 14.747, p = 0.031). However, at 40°C, it increased consistently from 14.01 U/mg·prot in the first 4 h and its maximum value 18.05 U/mg·prot was obtained at 40°C-4 h (F (1, 18) = 43.219, p < 0.001), and then, it dropped to 15.20 U/mg·prot (F (1, 18) = 25.214, p < 0.001).
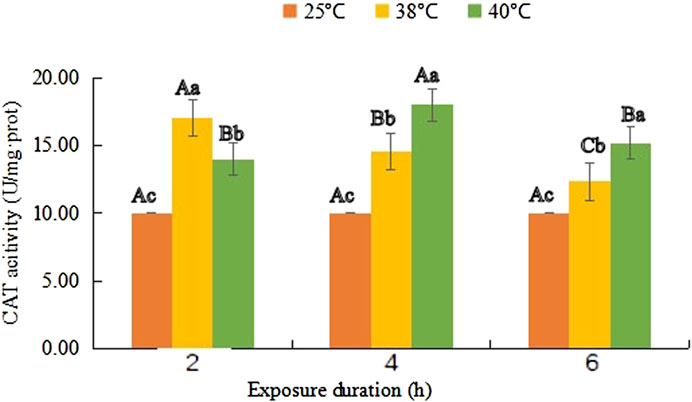
FIGURE 2. Catalase (CAT) activity (mean activity value ± SD) in adult females of Neoseiulus barkeri after the short-term heat stress. Different capitals indicate significant differences within the different exposure duration at the same temperature, while different lowercases show significant differences within the same exposure duration at different temperature (Duncan’s new multiple range method, p < 0.05). Variance analysis showed that the CAT activity was significantly affected by the temperature (F (2, 81) = 315.276, p < 0.001), treatment duration (F (2, 81) = 52.003, p < 0.001) as well as their interaction. (F (4, 81) = 124.198, p < 0.001).
Table 3 presented the coefficients between the temperature and CAT activity, and the duration and CAT activity were 0.710 and -0.069, respectively. The temperature was also the major factor influencing the CAT activity. The exposure duration contributed less to the CAT activity variation. Similarly, no correlation was found between the temperature and duration.
Peroxidase activity
POD activity shown in Figure 3 followed the trend of increasing, then slightly decreasing (F (1, 18) = 0.108, p = 0.309), and finally increasing to 6.05 U/mg·prot from 5.83 U/mg·prot, while it continued rising in the first 4 h at 40°C and reached the maximum level 7.38 U/mg·prot at 40°C- 4 h (F (1, 18) = 62.812, p < 0.001), and then it dropped to 5.41 U/mg·prot, but no significant difference was found with the control of 4.83 U/mg·prot (F (1, 18) = 1.268, p = 0.597).
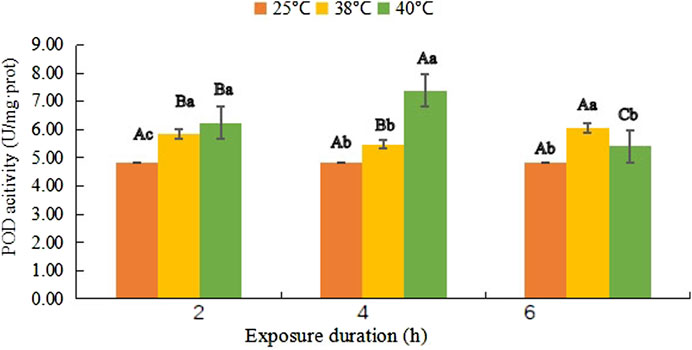
FIGURE 3. Peroxidase (POD) activity (mean activity value ± SD) in adult females of Neoseiulus barkeri after the short-term heat stress. Different capitals indicate significant differences within the different exposure duration at the same temperature, while different lowercases show significant differences within the same exposure duration at different temperature (Duncan’s new multiple range method, p < 0.05). Variance analysis showed that the POD activity was significantly affected by the temperature (F (2, 81) = 335.965, p < 0.001), treatment duration (F (2, 81) = 87.399, p < 0.001) as well as their interaction (F (4, 81) = 237.070, p < 0.001).
The coefficients between the temperature and POD activity, and the duration and POD activity were shown in table 4 with values of 0.764 and −0.105, respectively, which proved that temperature was much more related than duration to the POD activity variation in this experiment. Furthermore, the temperature and duration were still completely unrelated.
Discussion
Temperature is one of the most important environmental variables that induce physiological change in organisms (Jin et al., 2010; Jia et al., 2011). Arthropods have evolved a series of diverse behavioral and physiological strategies to avoid temperature impairments, such as seeking shelter, changing the fluidity of cell membranes, and accumulating sugars, polyols, anti-freeze proteins and amino acids (Storey, 1997; Wang and Kang, 2005; Zhang et al., 2014). Neoseiulus barkeri, is one of the poikilothermic organisms, whose body temperature is highly affected by the ambient temperature. Moreover, Neoseiulus barkeri has been playing a key role in controlling pest mites in natural filed. However, few studies of thermal stress on the N. barkeri were found. Our previous work and another study all showed that the thermal stress negatively affected the longevity, reproduction characteristics (Li et al., 2021), copulation (Zhang et al., 2016), and functional response (Li et al., 2022). Physiological mechanisms like the change of antioxidant enzymes of Neoseiulus barkeri, when faced thermal stress, were still not clear.
In the present study, the exposure of N. barkeri to high temperatures had significant effects on the activities of SOD, CAT, and POD. The effects were enhanced with the increasing temperature and longer exposure duration. At 38°C, the activity of three enzymes showed a sudden rise after 2 h-exposure, then the CAT continued decreasing while the SOD and POD decreased after 4 h-exposure but slightly increased after 6 h-exposure. The difference in activity between the CAT and other two enzymes could be explained that under normal physiological states, an interacting network of antioxidant enzymes effectively neutralizes the harmful effects of ROS (Davies, 1995; Kayis et al., 2015). This detoxification pathway is the result of multiple enzymes with SOD catalyzing the first step; and then, CAT, POD, and various others removing hydrogen peroxide (Sies, 1997). Specifically, SOD removes O2-through the process of dismutation to O2 and H2O2; and then, H2O2 is sequentially reduced to H2O and O2 by CAT and POD (Hoffmann et al., 1997; Kashiwagi et al., 1997).
However, the activity of the three enzymes presented the exact same variation tendency at 40°C. It consistently increased in the first 4 h and reached the maximum value, but it dropped after 6 h-exposure. It means that N. barkeri generated more substances like ROS; thus, enzymes with a higher level activity were needed to protect the body. Comparing with 38°C, 40°C enhanced the negative effect of thermal stress on N. barkeri. A similar pattern was reported by Li Z. M et al., 2010 for the activity of CAT in the adult Tetrastichus brontispae, a parasitoid of Brontispa longissimi. Significant change in POD and CAT activities were also found in the adult Mononychellus mcgregori after exposure to heat stress for 36–42°C (Lu et al., 2014). Thus, those activity changes of antioxidant enzymes might be the reason that N. barkeri could still survive at high temperature even their population characteristics were heavily affected (Coombs and Bale, 2013; Li et al., 2021).
It is known that SOD plays an important role as an antioxidant enzyme by reducing the high level of superoxide radical induced by high surrounding temperature. In this study, the SOD activity significantly increased in early exposure but decreased in the following stressing period, suggesting that SOD was induced by changes in surrounding temperatures and then protect the mites from thermal stress. In addition, N. barkeri might become acclimated to the thermal stress. This result is in consistent with the findings of An and Choi (2010), where early-stage of exposure to acute temperature changes resulted in the oxidative stress regulated by antioxidant enzymes, but continued stress caused by acute temperature exposure resulted in the decreased SOD activity (Yang et al., 2010). It is probably because the well-adapted characteristic that mites owned to their surrounding environment (Hangartner and Hoffmann, 2016; Xu et al., 2001).
In this study, the increased CAT and POD activity was observed together. It is believed that this increase has occurred to eliminate H2O2, which is the final product formed during the elimination of superoxide radicals via the increased SOD activity. Previous findings showed that CAT removed H2O2 only at high cellular concentrations, whereas it is inefficient for H2O2 removal at low concentrations (Ahmad et al., 1991). It is probably because the synergistic work of SOD and POD removed most of the ROS and H2O2, which made CAT remain at a low level. It has been reported that the CAT activity was too low to detect in the herbivorous citrus red mite P. citri when exposed to thermal stress (Yang et al., 2010). In the current study, a significant increase of CAT activities was observed in the adults of the carnivorous mite N. barkeri exposed to different thermal stress conditions. Apparently, it enhanced the removal of H2O2 and prevented damage by the oxidative stress. The difference in the CAT activity between the predatory mite N. barkeri and herbivorous mite P. citri suggests that the two species differ in the antioxidant defense mechanism though they both belong to the Acari (Zhang et al., 2014).
In this study, correlation analysis showed that the temperature was the primary factor affecting the enzymatic activity, while the exposure duration was the secondary factor. It is probably because the poikilotherms such as N. barkeri are generally well adapted to high temperatures, the longer they are exposed to a high temperature, the more adapted they would be (Hangartner and Hoffmann, 2016). The study by Feng et al. (2008) indicated that non-lethal heat pre-stimulation enhanced the ability of Tetranychus cinnabarinus to resist extremely high temperatures. Other similar studies have presented the same enzymatic change, but none of them pointed out which was more important between the temperature and exposure duration. These physiological changes could to some extent help the organism to resist the adverse environment, however in the meantime, they were also the processes of energy consumption at the expense of potential fertility or survival level, which might be one of the physiological explanations to their shortened longevity in our previous findings (Silbermann and Tatar, 2000; Roux et al., 2010; Zhang et al., 2013).
The present study mainly focused on the three types of primary antioxidant enzymes. Studies have shown that other substances scavenging H2O2 also exist when mites encounter heat stress, such as ascorbate peroxidase (APX) (Li et al., 2007), which has a strong affinity and binding force with H2O2, and may also be involved in the process of protecting the body. Other non-enzymes may also be used to protect the body from damage, such as ɑ -tocopherol, glutathione, trehalose, polyol, and ascorbic acid. (Ghiselli et al., 2000; Cumming et al., 2014). Antioxidant enzymes also work together with heat shock protein HSPs to reduce the ROS damage to mite bodies (Lopez-Martinez et al., 2008). These related works are expected to be conduct in the following study.
Data availability statement
The data presented in the study are deposited in the Figshare repository, accession number 10.6084/m9.figshare.19642347.
Ethics statement
Informed consent was obtained from all individual participants included in the study. The research project was conducted on invertebrate species that are not subjected to any specific ethical issue and legislation.
Author contributions
S-QS was the supervisor of this research work, who helped design the work. J-JZ helped revise the manuscript. W-ZL carried out the experiments, drafted the manuscript, and analyzed the data. TZ helped carry out the experiments and rear the experimental colonies of the Neoseiulus barkeri and their prey bran mites Aleuroglyphus ovatus.
Funding
This research was supported by the Fund for Key Course Construction of Postgraduate of Gansu Agricultural University (No. GSAUZDKC-2016) and the Program of Introducing Talents to Chinese Universities (111 Program No. D20023).
Acknowledgments
The authors would like to express their appreciation to Yu Lichen and her team member Xu Changxin, the Institute of Fruit Tree Research in Changli, Hebei Province, China, for their technical support, guidance, and providing the predatory mite population used in this study. They are grateful to Zhi-Qiang Zhang of Manaaki Whenua, Landcare Research and the University of Auckland (New Zealand), for his good guidance in data analysis.
Conflict of interest
The authors declare that the research was conducted in the absence of any commercial or financial relationships that could be construed as a potential conflict of interest.
Publisher’s note
All claims expressed in this article are solely those of the authors and do not necessarily represent those of their affiliated organizations, or those of the publisher, the editors, and the reviewers. Any product that may be evaluated in this article, or claim that may be made by its manufacturer, is not guaranteed or endorsed by the publisher.
References
Ahmad S., Duval D. L., Weinhold L. C., Pardini R. S. (1991). Cabbage looper antioxidant enzymes: tissue specificity. Insect Biochem. 21, 563–572. doi:10.1016/0020-1790(91)90111-q
An M. I., Choi C. Y. (2010). Activity of antioxidant enzymes and physiological responses in ark shell, Scapharca broughtonii, exposed to thermal and osmotic stress: effects on hemolymph and biochemical parameters. Comp. Biochem. Physiology Part B Biochem. Mol. Biol. 155, 34–42. doi:10.1016/j.cbpb.2009.09.008
Arthurs S., McKenzie C. L., Chen J., Dogramaci M., Brennan M., Houben K., et al. (2009). Evaluation of Neoseiulus cucumeris and Amblyseius swirskii (Acari: Phytoseiidae) as biological control agents of chilli thrips, Scirtothrips dorsalis (Thysanoptera: Thripidae) on pepper. Biol. Control 49, 91–96. doi:10.1016/j.biocontrol.2009.01.002
Bale J. S. (2002). Insects and low temperatures: from molecular biology to distributions and abundance. Phil. Trans. R. Soc. Lond. B 357, 849–862. doi:10.1098/rstb.2002.1074
Bradford M. M. (1976). A rapid and sensitive method for the quantitation of microgram quantities of protein utilizing the principle of protein-dye binding. Anal. Biochem. 72, 248–254. doi:10.1006/abio.1976.9999
Coombs M. R., Bale J. S. (2013). Comparison of thermal activity thresholds of the spider mite predators Phytoseiulus macropilis and Phytoseiulus persimilis (Acari: Phytoseiidae). Exp. Appl. Acarol. 59, 435–445. doi:10.1007/s10493-012-9619-9
Coskun M., Kayis T., Gulsu E., Alp A. L. P. (2020). Effects of selenium and vitamin E on enzymatic, biochemical, and immunological biomarkers in Galleria mellonella L. Sci. Rep. 10, 9953. doi:10.1038/s41598-020-67072-9
Cui X. H., Wan F. H., Xie M., Liu T. X. (2008). Effects of heat shock on survival and reproduction of two whitefly Species,Trialeurodes vaporariorumandBemisia tabaciBiotype B. J. Insect Sci. 8, 1–10. doi:10.1673/031.008.2401
Cumming K. T., Raastad T., Holden G., Bastani N. E., Schneeberger D., Paronetto M. P., et al. (2014). Effects of vitamin C and E supplementation on endogenous antioxidant systems and heat shock proteins in response to endurance training. Physiol. Rep. 2, e12142. doi:10.14814/phy2.12142
Davies K. (1995). Oxidative stress: the paradox of aerobic life. Biochem. Soc. Symp. 61, 1–31. doi:10.1042/bss0610001
Dubovskiy I. M., Martemyanov V. V., Vorontsova Y. L., Rantala M. J., Gryzanova E. V., Glupov V. V. (2008). Effect of bacterial infection on antioxidant activity and lipid peroxidation in the midgut of Galleria mellonella L. larvae (Lepidoptera, Pyralidae). Comp. Biochem. Phys. c 148, 1–5. doi:10.1016/j.cbpc.2008.02.003
Emmert C. J., Mizell R. F., Andersen P. C., Frank J. H., Stimac J. L. (2008). Effects of contrasting diets and temperatures on reproduction and prey consumption by Proprioseiopsis asetus (Acari: Phytoseiidae). Exp. Appl. Acarol. 44, 11–26. doi:10.1007/s10493-008-9130-5
Emre I., Kayis T., Coskun M., Dursun O., Cogun H. Y. (2013). Changes in antioxidative enzyme activity, glycogen, lipid, protein, and malondialdehyde content in cadmium-treated Galleria mellonella larvae. Ann. Entomol. Soc. Am. 106, 371–377. doi:10.1603/an12137
Felton G. W., Summers C. B. (1995). Antioxidant systems in insects. Arch. Insect Biochem. Physiol. 29, 187–197. doi:10.1002/arch.940290208
Feng H, Z., Liu Y. H., He L., Yang D. X., Ii M., Lu W. C. (2008). Heat shock response and HSPs of Tetranychus cinnabarinus (Acari: Tetranychidae) resistant to avermectin. Acta Entomol. Sin. 51, 1164–1169. doi:10.16380/J.KCXB.2008.11.011
Gadino A. N., Walton V. M. (2012). Temperature-related development and population parameters for Typhlodromus pyri (Acari: Phytoseiidae) found in Oregon vineyards. Exp. Appl. Acarol. 58, 1–10. doi:10.1007/s10493-012-9562-9
Gechev T. S., Van Breusegem F., Stone J. M., Denev I., Laloi C. (2006). Reactive oxygen species as signals that modulate plant stress responses and programmed cell death. Bioessays 28, 1091–1101. doi:10.1002/bies.20493
Ghazy N. A., Suzuki T., Amano H., Ohyama K. (2012). Effects of air temperature and water vapor pressure deficit on storage of the predatory mite Neoseiulus californicus (Acari: Phytoseiidae). Exp. Appl. Acarol. 58, 111–120. doi:10.1007/s10493-012-9556-7
Ghiselli A., Serafini M., Natella F., Scaccini C. (2000). Total antioxidant capacity as a tool to assess redox status: Critical view and experimental data. Free Radic. Biol. Med. 29, 1106–1114. doi:10.1016/s0891-5849(00)00394-4
Gill S. S., Tuteja N. (2010). Reactive oxygen species and antioxidant machinery in abiotic stress tolerance in crop plants. Plant Physiology Biochem. 48, 909–930. doi:10.1016/j.plaphy.2010.08.016
Green D. R., Reed J. C. (1998). Mitochondria and apoptosis. Science 281, 1309–1312. doi:10.1126/science.281.5381.1309
Hangartner S., Hoffmann A. A. (2016). Evolutionary potential of multiple measures of upper thermal tolerance inDrosophila melanogaster. Funct. Ecol. 30, 442–452. doi:10.1111/1365-2435.12499
Hart A. J., Bale J. S., Tullett A. G., Worland M. R., Walters K. F. A. (2002). Effects of temperature on the establishment potential of the predatory mite Amblyseius californicus McGregor (Acari: Phytoseiidae) in the UK. J. Insect Physiology 48 (6), 593–599. doi:10.1016/s0022-1910(02)00087-2
Hoffmann A. A., Dagher H., Hercus M., Berrigan D. (1997). Comparing different measures of heat resistance in selected lines of Drosophila melanogaster. J. Insect Physiology 43, 393–405. doi:10.1016/s0022-1910(96)00108-4
Hoffmann A. A., Sgrò C. M. (2011). Climate change and evolutionary adaptation. Nature 470, 479–485. doi:10.1038/nature09670
Hoffmann A. A., Sørensen J. G., Loeschcke V. (2003). Adaptation of Drosophila to temperature extremes: bringing together quantitative and molecular approaches. J. Therm. Biol. 28, 175–216. doi:10.1016/s0306-4565(02)00057-8
Jia F. X., Dou W., Hu F., Wang J. J. (2011). Effects of thermal stress on lipid peroxidation and antioxidant enzyme activities of oriental Fruit Fly,Bactrocera dorsalis(Diptera: Tephritidae). Fla. Entomol. 94, 956–963. doi:10.1653/024.094.0432
Jin H. L., Chen Q., Jin Q. A., Tang C., Wen H. B., Peng Z. Q. (2010). Effects of heat stress on the development and fecundity of Asecodes hispinarum (Bouceˇk). Chin. J. Trop. Crops. 31, 631–635. doi:10.3969/j.issn.1000-2561.2010.04.023
Juan-Blasco M., Qureshi J. A., Urbaneja A., Stansly P. A. (2012). Predatory Mite,Amblyseius swirskii(Acari: Phytoseiidae), for biological control of asian citrus Psyllid,Diaphorina citri(Hemiptera: Psyllidae). Fla. Entomol. 95, 543–551. doi:10.1653/024.095.0302
Kashiwagi A., Kashiwagi K., Takase M., Hanada H., Nakamura M. (1997). Comparison of catalase in diploid and haploid Rana rugosa using heat and chemical inactivation techniques. Comp. Biochem. Physiology Part B Biochem. Mol. Biol. 118, 499–503. doi:10.1016/s0305-0491(97)00216-2
Kayis T., Coskun M., Dursun O., Emre I. (2015). Alterations in antioxidant enzyme activity, lipid peroxidation, and ion balance induced by dichlorvos in Galleria mellonella L. Ann. Entomol. Soc. Am. 108, 570–574. doi:10.1093/aesa/sav038
Li W. Z., Li H. L., Guo Z. K., Shang S. Q. (2021). Effects of short-term heat stress on the development and reproduction of predatory mite Neoseiulus barkeri (Acari, Phytoseiidae). saa 26, 713–723. doi:10.11158/saa.26.4.5
Li W. Z., Zhu T., Li H. L., Shang S. Q. (2022). The effects of short‐term heat stress on functional response of Neoseiulus barkeri to Tetranychus urticae. J. Appl. Entomol. 146, 310–318. doi:10.1111/jen.12954
Li X., Schuler M. A., Berenbaum M. R. (2007). Molecular mechanisms of metabolic resistance to synthetic and natural xenobiotics. Annu. Rev. Entomol. 52, 231–253. doi:10.1146/annurev.ento.51.110104.151104
Li Z. M., Chen Q., Jin QiA., Tang C., Wen H. B., Peng Z. Q. (2010). Effect of high temperature on the protective enzymes of Tetrastichus brontispae. Chin. J. Trop. Crops. 31, 994–998. doi:10.3969/j.issn.1000-2561.2010.06.022
Lopez-Martinez G., Elnitsky M. A., Benoit J. B., Lee R. E., Denlinger D. L. (2008). High resistance to oxidative damage in the Antarctic midge Belgica antarctica, and developmentally linked expression of genes encoding superoxide dismutase, catalase and heat shock proteins. Insect Biochem. Mol. Biol. 38, 796–804. doi:10.1016/j.ibmb.2008.05.006
Lu F., Liang X., Lu H., Li Q., Chen Q., Zhang P., et al. (2017). Overproduction of superoxide dismutase and catalase confers cassava resistance to Tetranychus cinnabarinus. Sci. Rep. 7, 40179. doi:10.1038/srep40179
Lu F. P., Chen Q., Chen Z. S., Lu H., Xu X. L., Jing F. L., et al. (2014). Effects of heat stress on development, reproduction and activities of protective enzymes in Mononychellus mcgregori. Exp. Appl. Acarol. 63, 267–284. doi:10.1007/s10493-014-9784-0
Mcmurtry J. A., Moraes G. J., Sourassou N. F. (2013). Revision of the lifestyles of phytoseiid mites (Acari: Phytoseiidae) and implications for biological control strategies. Syst. Appl. Acarol. 18, 297–424. doi:10.11158/saa.18.4.1
Michael L. Y., Whiting D. C. (1996). Response of ‘Hayward’ kiwifruit to high-temperature controlled atmosphere treatments for control of two-spotted spider mite (Tetranychus urticae). Postharvest Biol. Technol. 7, 73–81. doi:10.1016/0925-5214(95)00035-6
Opit G. P., Nechols J. R., Margolies D. C. (2004). Biological control of twospotted spider mites, Tetranychus urticae koch (Acari: Tetranychidae), using phytoseiulus persimilis athias-henriot (Acari: Phytoseidae) on ivy geranium: assessment of predator release ratios. Biol. Control 29, 445–452. doi:10.1016/j.biocontrol.2003.08.007
Palyvos N. E., Emmanouel N. G. (2009). Temperature-dependent development of the predatory mite Cheyletus malaccensis (Acari: Cheyletidae). Exp. Appl. Acarol. 47, 147–158. doi:10.1007/s10493-008-9200-8
Perring T. M., Holtzer T. O., Kalisch J. A., Norman J. M. (1984). Temperature and humidity effects on ovipositional rates, fecundity, and longevity of adult female Banks grass mites (Acari: Tetranychidae). Ann. Entomol. Soc. Am. 77, 581–586. doi:10.1093/aesa/77.5.581
Ray P. D., Huang B. W., Tsuji Y. (2012). Reactive oxygen species (ROS) homeostasis and redox regulation in cellular signaling. Cell. Signal. 24, 981–990. doi:10.1016/j.cellsig.2012.01.008
Roux O., Le Lann C., van Alphen J. J. M., van Baaren J. (2010). How does heat shock affect the life history traits of adults and progeny of the aphid parasitoidAphidius avenae(Hymenoptera: Aphidiidae)? Bull. Entomol. Res. 100, 543–549. doi:10.1017/S0007485309990575
Sies H. (1997). Oxidative stress: oxidants and antioxidants. Exp. Physiol. 82 (2), 291–295. doi:10.1113/expphysiol.1997.sp004024
Silbermann R., Tatar M. (2000). Reproductive costs of heat shock protein in transgenic Drosophila melanogaster. Evolution 54, 2038–2045. doi:10.1111/j.0014-3820.2000.tb01247.x
Sørensen J. G., Dahlgaard J., Loeschcke V. (2001). Genetic variation in thermal tolerance among natural populations ofDrosophila buzzatii: down regulation of Hsp70 expression and variation in heat stress resistance traits. Funct. Ecol. 15, 289–296. doi:10.1046/j.1365-2435.2001.00525.x
Storey K. B. (1997). Organic solutes in freezing tolerance. Comp. Biochem. Physiology Part A Physiology 117, 319–326. doi:10.1016/s0300-9629(96)00270-8
Tamás L., Mistrík I., Zelinová V. (2017). Heavy metal-induced reactive oxygen species and cell death in barley root tip. Environ. Exp. Bot. 140, 34–40. doi:10.1016/j.envexpbot.2017.05.016
Wang H. S., Kang L. (2005). Effect of cooling rates on the cold hardiness and cryoprotectant profiles of locust eggs. Cryobiology 51, 220–229. doi:10.1016/j.cryobiol.2005.07.003
Wang M., Li Z. Z. (2002). Studies on the activities of enzymes of protective system during diapause of sawfly Chinolyda flagellicorni. For. Sci. 38, 100–104.
Wang Y., Oberley L. W., Murhammer D. W. (2001). Antioxidant defense systems of two lipidopteran insect cell lines. Free Radic. Biol. Med. 30, 1254–1262. doi:10.1016/s0891-5849(01)00520-2
Xu Z. B., Zou X. P., Zhang N., Feng Q. L., Zheng S. C. (2001). Detoxification of insecticides, allechemicals and heavy metals by glutathione S-transferase SlGSTE1 in the gut of Spodoptera litura. J. Insect. Sci. 22, 503–511. doi:10.1111/1744-7917.12142
Yang L. H., Huang H., Wang J. J. (2010). Antioxidant responses of citrus red mite, Panonychus citri (McGregor) (Acari: Tetranychidae), exposed to thermal stress. J. Insect Physiology 56, 1871–1876. doi:10.1016/j.jinsphys.2010.08.006
Yucel M. S., Kayis T. (2019). Imidacloprid induced alterations in oxidative stress, biochemical, genotoxic, and immunotoxic biomarkers in non-mammalian model organismGalleria mellonellaL. (Lepidoptera: Pyralidae). J. Environ. Sci. Health, Part B 54, 27–34. doi:10.1080/03601234.2018.1530545
Zhang G. H., Li Y. Y., Zhang K. J., Wang J. J., Liu Y. Q., Liu H., et al. (2016). Effects of heat stress on copulation, fecunditiy and longevity in newly-emerged adults of the predatory mite Neoseiulus barkeri (Acari: Phytoseiidae). Syst. Appl. Acarol. 21, 295. doi:10.11158/saa.21.3.5
Zhang G. H., Liu H., Wang J. J., Wang Z. Y. (2014). Effects of thermal stress on lipid peroxidation and antioxidant enzyme activities of the predatory mite, Neoseiulus cucumeris (Acari: Phytoseiidae). Exp. Appl. Acarol. 64, 73–85. doi:10.1007/s10493-014-9806-y
Keywords: Neoseiulus barkeri, short-term heat stress, protein content, antioxidant enzyme, activity
Citation: Li W-Z, Zhu T, Zhou J-J and Shang S-Q (2022) Effects of short-term heat stress on the activity of three antioxidant enzymes of predatory mite Neoseiulus barkeri (acari, phytoseiidae). Front. Physiol. 13:937033. doi: 10.3389/fphys.2022.937033
Received: 05 May 2022; Accepted: 05 July 2022;
Published: 17 August 2022.
Edited by:
Natraj Krishnan, Mississippi State University, United StatesReviewed by:
Claus Zebitz, University of Hohenheim, GermanyKai Jun Zhang, Southwest University, China
Copyright © 2022 Li, Zhu, Zhou and Shang. This is an open-access article distributed under the terms of the Creative Commons Attribution License (CC BY). The use, distribution or reproduction in other forums is permitted, provided the original author(s) and the copyright owner(s) are credited and that the original publication in this journal is cited, in accordance with accepted academic practice. No use, distribution or reproduction is permitted which does not comply with these terms.
*Correspondence: Su-Qin Shang, shangsq@gsau.edu.cn