- 1Department of Crop Science and Technology, College of Agriculture, South China Agricultural University, Guangzhou, China
- 2Scientific Observing and Experimental Station of Crop Cultivation in South China, Ministry of Agriculture, Guangzhou, China
Lead (Pb) caused interruptions with normal plant metabolism, crop yield losses and quality issues are of great concern. This study assessed the physio-biochemical responses, yield and grain quality traits and Pb distribution proportions in three different fragrant rice cultivars i.e., Meixiangzhan-2, Xinagyaxiangzhan and Basmati-385. Plants were exposed to 400, 800, and 1,200 ppm of Pb while pots without Pb were taken as control (0 ppm). Our results showed that Pb toxicity significantly (P < 0.05) reduced photosynthetic pigments (chlorophyll contents and carotenoids) and inducted oxidative stress with increased production of hydrogen peroxide (H2O2), malanodialdehyde (MDA) and leaves leachates; while such effects were more apparent in Xinagyaxiangzhan than other two rice cultivars. Pb stress differentially affected the production protein, proline and soluble sugars; however the production rates were higher at heading stage (HS) than maturity stage (MS). Furthermore, Pb stress altered superoxide dismutase (SOD), peroxidases (POD), catalases (CAT) and ascorbate peroxidases (APX) activities and glutathione (GSH) and oxidized glutathione (GSSG) production in all rice cultivars at both HS and MS. All Pb levels reduced the yield and yield components of all rice cultivars; nonetheless such reductions were observed highest in Xinagyaxiangzhan (69.12%) than Meixiangzhan-2 (58.05%) and Basmati-385 (46.27%) and resulted in grain quality deterioration. Significant and positive correlations among rice yields with productive tillers/pot and grains per panicle while negative with sterility percentage were also observed. In addition, all rice cultivars readily taken up the Pb contents from soil to roots and transported upward in different proportions with maximum in roots followed by stemss, leaves, ears and grains. Higher proportions of Pb contents in above ground plant parts in Xinagyaxiangzhan possibly lead to maximum losses in this cultivar than other two cultivars; while less damage in Basmati-385 might be related to strong anti-oxidative defense system and lower proportions of Pb contents in its aerial parts.
Introduction
Recent rates of soil contamination with different heavy metals (the non-essential elements for plants) and their entrance in to the agro-ecosystems and transference to human beings through food chain is an alarming situation over the globe (Abrahams, 2002; Anjum et al., 2016a,b). In terrestrial ecosystems, soil act as the main source of heavy metal transference to agricultural products. These metals enter in to the plant systems from soil or from external atmosphere surrounded by the plant and would have serious consequences in crop productivity and grain qualities.
Among different heavy metals, lead (Pb), is the second most harmful pollutant after arsenic and recently listed as “the chemical of great concern” according to the new European REACH regulations (Pourrut et al., 2011). It severely affects normal plant metabolism, morph-physiological features and crop growth and productivity (Sharma and Dubey, 2005; Ashraf et al., 2015). It often leads to diminished growth, deformation of cellular structures, ion homeostasis, reductions in chlorophyll biosynthesis, hormonal imbalance and induce over-production of reactive oxygen species (ROS) in plants (Shahid et al., 2011; Kumar et al., 2012). Pb, being non-redox metal, cause ROS production that led to oxidative stress within plant cells (Singh et al., 2010). Once produced, these ROS readily attacks to biological structures and biomolecules and results in metabolic dysfunction (Clemens, 2006).
Plants normally have three mechanisms of Pb-tolerance i.e., (a) passive mechanisms (plant develops different types of physical barriers against Pb uptake), (b) inducible mechanisms (metal detoxification and its excretion to extra-cellular spaces), and (c) activation of anti-oxidative defense system (which includes both enzymatic and non-enzymatic anti-oxidants) to scavenge ROS (Pourrut et al., 2011; Ashraf et al., 2015). Anti-oxidants, both enzymatic such as superoxide dismutase (SOD), peroxidase (POD), catalase (CAT), and ascorbate peroxidase (APX), and non-enzymatic such as reduced glutathione (GSH) and oxidized glutathione (GSSG) are involved in direct and/or indirect detoxification of ROS in plants (Mishra and Choudhary, 1998; Mittler, 2002). Except anti-oxidants, plants also accumulate various types of organic compounds or osmolytes proline and soluble sugars to shield essential cellular structures and to maintain cell osmotic potential (Chatterjee et al., 2004; Ali et al., 2014).
Scented rice is the most popular rice type among farmers and consumers over the globe due to its special aroma (Singh et al., 2000). Increasing demands and opportunities to get premium prices in world markets, yield maintenance and quality assurance are the main factors that would lead it to the international markets to meet consumer requirements. However, soil contaminated with toxic metals, especially Pb, would thus become the main hindrance in its way as it readily absorbed by the roots and transports to the edible plant parts which often lead to rice quality deterioration. Rice milling quality can be assessed by the rate of brown rice, milled rice, and head rice rates as well as milling recovery percentages which directly related to the market value while rice chalkiness percentage, chalkiness degree and grain protein and amylose contents are the appearance and cooking qualities of rice grains (Fahad et al., 2016a) that would greatly affected by Pb contaminated soils. Hence Pb effects on rice physiology and its mimicking effects on yield and quality as well as grain Pb concentrations are important to study. Although many previous studies reported the effects of Pb on rice growth and physio-biochemical responses but to our knowledge, a very few work has been done on its effects on yield and quality characters along with physio-biochemical responses especially in aromatic rice. Present study was planned with the objectives to (a) assess the Pb-induced changes in plant physio-biochemical mechanisms including anti-oxidant activities, (b) investigate the changes in yield and quality traits of aromatic rice, and to (c) estimate percentage proportions of accumulated Pb in different plant parts of rice.
Materials and Methods
Experimental Site, Soil, and Conditions
The experiment was carried out in open air conditions under rain-protected wire house located at Experimental Research Farm, College of Agriculture, South China Agricultural University, Guangzhou, China (23°09′ N, 113°22′ E and 11 m above the sea level) during April to July 2015 as early season rice in South China. During growing season, the mean monthly day and night temperatures lies between 23–29°C and 22–28°C, respectively with average humidity between 77 and 89%.
One month prior to nursery transplanting, the pots were filled with air-dried soil collected from uncontaminated paddy field with 10 kg soil in each pot (25 cm in height and 32 cm in diameter) containing total N 1.190 g kg−1, total P 0.952 g kg−1, total K 18.30 g kg−1, available N 90.38 mg kg−1, available P 9.45 mg kg−1, available K 130.29 mg kg−1, organic matter 21.83 g kg−1, soil pH 5.63, and 51.12 ppm initial soil Pb contents.
Treatment Application, Nursery Transplantation, and Crop Husbandry
After pot filling, soil within pots was supplemented with Pb by using Pb(NO3)2 in solution form. The required Pb concentrations i.e., 400, 800, and 1,200 ppm were prepared by dissolving the Pb(NO3)2 salt in deionized water, applied in respective pots and thoroughly mixed at the same time. The pots without any addition of external Pb were regarded as control (0 ppm). The pots were kept in submerged conditions by maintain a water layer above soil surface to create fully anaerobic and puddle like conditions within the pots.
Seeds of three aromatic rice cultivars i.e., “Meixiangzhan-2” (Lemont × Fengaozhan, indica type of aromatic rice cultivar with 145–147 days to maturity), “Xiangyaxiangzhan” (Xiangsimiao126 × Xiangyaruanzhan, indica type of aromatic rice cultivar with 112–114 days to maturity), and “Basmati-385” (early maturing, intermediate tall with very good cooking quality and aroma with 145–150 days to maturity) were collected from the College of Agriculture, South China Agricultural University, Guangzhou, China and soaked in tap water in wooden box at 25–30°C for 48 h to complete germination process. Healthy and germinated seeds were subsequently sown on soil containing plastic trays and placed on leveled paddy field and covered with a plastic sheet in early March. One month old seedlings were then transplanted to the pots with 3–4 seedlings per hill and 5 hills per pot (four seedlings aside and one in the middle of the pot). Till 1 week after transplanting, the damaged, broken and died seedlings were replaced with the healthy seedlings to get a good stand establishment. All pots were applied with 2.25 g N (Urea 46%), 3.33 g P (super phosphate 12% P2O5), and 1.35 g K (potassium chloride 60% K2O) with 70% as starter dose and 30% at tillering stage. The plants were regularly monitored and a water layer of 2–3 cm was maintained throughout the growth period with tap water.
Sampling and Observations
For physio-biochemical analyses and Pb determination, plants were sampled at heading stage (HS) and maturity stage (MS) and stored at −80°C (for biochemical assays) while yield and quality attribute were recorded at MS.
Photosynthetic Pigments and Biochemical Assays
To determine photosynthetic pigments, fresh leave samples (0.2 g) at HS and MS were extracted with 15 ml of 95% ethanol and the contents were estimated according to Arnon (1949). Hydrogen peroxide (H2O2) contents were estimated according to Velikova et al. (2000). The malondialdehyde (MDA) contents were determined by following the methods of Hodges et al. (1999) with the following formula: MDA content = {6.45(ΔOD532−600) − (0.56OD450)}. To estimate leachates percentage, the fresh leaf samples were thoroughly washed with deionized water (Milli-Q), cut in to small pieces. Leaf discs (0.3 g) were then placed in 10 ml deionized water and incubated at 25°C for 6 h and the electrical conductivity (EC) (EC1) was recorded with an EC meter (SX-650, Sansin, China). To record the second EC (EC2), the samples were then incubated at 90°C for 2 h and then cool down to 25°C. The leachates of the leaves samples were calculated as: leachates (%) = EC1/EC2 ×100) (Valentovic et al., 2006). Protein contents of fresh leaves samples were estimated according to Bradford (1976) at HS and MS using G-250. The Proline contents in fresh leaves samples (0.2 g) were determined according to Bates et al. (1973) by using ninhydrin at both HS and MS. For soluble sugars determination at HS and MS, methods of Zhang et al. (2009) were followed.
Fresh leaves samples (0.3 g) were crushed in liquid-N2 and homogenized in 6 ml of 50 ml sodium phosphate buffer (pH 7.8) with mortar and pestle in an ice bath and homogenate were centrifuged at 10,000 g for 20 min at 4°C and the aliquot of the supernatant was used to record the enzymatic activities.
Activities of superoxide dismutase (SOD, EC 1.15.1.1) were assayed according to Zhang et al. (2008) by following the inhibition of photochemical reduction due to nitro blue tetrazolium (NBT). SOD activity per unit was defined as the amount of enzyme required to inhibit NBT photochemical reduction to 50% as an activity unit (U). Peroxidase (POD, EC 1.11.1.7) activity was measured by using guaiacol method with minor modifications. The reactions mixture contained 1 ml of sodium phosphate buffer (pH 7.8), 0.2% of 0.95 ml guaiacol, 1 ml of 0.3% H2O2, and 0.05 ml aliquot of enzyme extract. The absorbance was read at 470 nm (Zhou and Leul, 1999). One unit of POD activity was the amount of enzyme that caused the decomposition of 1 μg substrate at 470 nm. Catalase activity (CAT, EC 1.11.1.6) was assayed according to the procedures of Aebi (1984) whereas one unit of enzyme activity (U) was the decomposition of 1 M H2O2 at A240 within 1 min in 1 g of fresh leaves samples. Ascorbate peroxidase (APX, EC 1.11.1.11) activity was estimated by using “APX determination kit” purchased from Nanjing Jiancheng Bioengineering Institute, China. The methods devised by the manufacturer were followed carefully and the absorbance was read at 290 nm. Reduced glutathione (GSH) and oxidized glutathione (GSSG) were also estimated by using the readymade build kits (A006-1 for GSH and A061-2 for GSSG), provided by Nanjing Jiancheng Bioengineering Institute, China (http://www.njjcbio.com). The instructions were strictly followed and the absorbance was read at 420 and 412 nm, respectively. Total glutathione was the addition of both GSH and GSSG (GSH+GSSG) contents.
Grain Yield and Related Attributes
To determine yield and related attributes, plants were harvested at MS and threshed manually for all rice cultivars and the grains were sun-dried till the grain moisture contents were lowered to 12–14%. Total tillers were calculated in each pot at tillering stage, while at reproductive stage; tillers containing panicles in each pot were counted to represent the productive tillers per pot. Spikelets were separated from the panicles of all rice cultivars and spikelets per panicle were calculated in each treatment. Number of partially filled and totally unfilled/empty grains on each panicle was counted and sterility percentage was calculated. Six random samples of 1,000-grains were taken from filled seed lot of all rice cultivars and weighed to get 1,000-grain weight and expressed in grams (g) while the total paddy weight from each pot was represented as grain yield pot−1. The harvest index of all rice cultivars was calculated as: 100 × (grain yield/plant dry biomass).
Grain Quality Characters
The harvested grains were taken from stored seed lot to determine rice quality attributes. A rice huller (Jingsu, China) was used to get brown rice and brown rice rate (BRR) was calculated as: (brown rice weight/paddy weight) × 100. The brown rice was milled with a Jingmi testing rice miller (Zhejiang, China) and milling recovery was recorded as: (milled rice weight/original weight) × 100. Milling degree was calculated as: milled rice weight/brown rice weight) × 100. To calculate head rice rate, whole milled grains were separated from 100 grains and head rice rate was calculated on percentage basis. Grain chalkiness percentage and chalkiness degree were assessed with the help of an SDE-A light box (Guangzhou, China). Grain chalkiness is the grains having opaque spots either on the dorsal and/or in center of the grain. Grain moisture, amylose, protein contents and grain alkali were determined by using an Infratec 1241 (FOSS-TECATOR) grain analyser.
Pb-Determination in Different Plant Parts
The sampled plants were separated into roots, stems, leaves, ears (at PH) and grains (at MS) stage, respectively of each rice cultivar and placed in oven at 80°C for drying. The oven dried ground samples (0.2 g) of each part were digested with di-acidic mixture of HNO3:HClO4 with a proportion of 4:1 v/v, and resultant solutions were diluted to 50 ml and then filtered by using Whatman filter paper no. 1. The Pb-concentrations in respective samples were estimated by using Atomic Absorption Spectrophotometer (AA6300C, Shimadzu, Japan) and the proportions Pb contents in different plant parts were calculated on percentage basis.
Experimental Design and Statistical Analyses
Pots were arranged in randomized complete block design (RCBD), having enough space among them to avoid shading, with 10 pots per treatment. The data were analyzed by using a statistical software “Statistix 8” (Analytical software, Tallahassee, Florida, USA) whilst difference amongst treatments were separated using least significant difference (LSD) test at P < 0.05. Correlation analyses among grain yield vs. other yield related attributes were computed and represented by using SigmaPlot 9.0 (Systat Software Inc., San Jose, CA, USA).
Results
Chlorophyll Contents and Carotenoids
Pb stress inhibited photosynthetic pigments, i.e., Chl a, Chl b, total chlorophyll contents (Chl a+b) and carotenoids significantly (P < 0.05) and the inhibition rates were increased with an increase in Pb-toxicity with highest at 1,200 ppm in all rice cultivar. For example, at heading stage (HS), the values for percentage reduction in Chl a, Chl b, and total chlorophyll contents (Chl a+b) were recorded as 54.80, 54.68, and 54.75% in Meixiangzhan-2, 54.41, 56.73, and 87.76% in Xiangyaxianzhan and 35.66, 37.07, and 36.21% in Basmati-385, respectively as compared with control. Similarly, at maturity stage (MS), the percentage reduction for Chl a, Chl b, and total chlorophyll contents (Chl a+b) were remained as 51.69, 39.63, and 34.56% for Meixiangzhan-2, 52.43, 59.69, and 55.46% for Xiangyaxianzhan, and 41.86, 50.33, and 45.11% for Basmati-385, when compared with control. Moreover, the ratio of Chl a/b were remained higher at higher doses of Pb-toxicity while chlorophyll contents were affected more severely due to Pb toxicity than carotenoids in all rice cultivars. Overall, the chlorophyll contents and carotenoids were recorded higher at HS as compare to MS while the magnitude of Pb stress on photosynthetic pigments across cultivars was recorded as: Xiangyaxianzhan > Meixiangzhan-2 > Basmati-385 (Table 1).
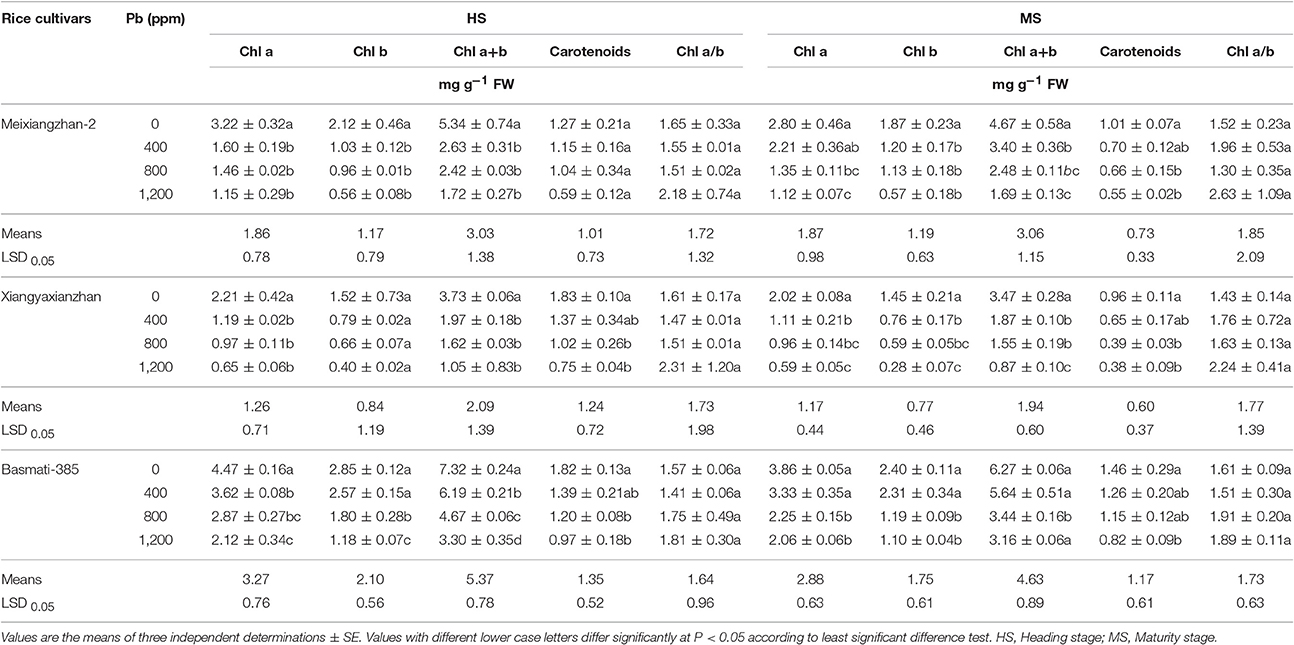
Table 1. Effect of different Pb concentrations on photosynthetic pigments of three scented rice cultivars at heading and maturity stages.
H2O2, MDA Contents, Leaf Leachates, and Osmo-Regulation
The degree of H2O2 production, lipid peroxidation (in terms of MDA production) and leaf leachates (an indicator of membrane damage) is associated with the Pb-toxicity level. Significant increase (P < 0.05) in MDA, H2O2 contents and leachates were recorded with an increase in soil Pb-concentrations in all three rice cultivars at both HS and MS. For Meixiangzhan-2, the values of percentage increase were 30.79–126.53% and 17.80–79.45%, 34.34–157.34% and 1.75–46.14% and 95.60–116.27% and 62.96–133.07% in H2O2, MDA and leaf leachates at HS and MS, respectively. Similarly, the values of percentage increase in H2O2 were in the range of 99.16–156.01% and 33.73–68.04% (in Xiangyaxianzhan), 16.43–24.71% and 7.65–20.05% (in Basmati-385), in MDA were in the range of 12.52–151.48% and 53.97–104.88% (in Xiangyaxianzhan) and 76.75–108.43% and 11.83–25.27% (in Basmati-385), and in leaf leachates were recorded in the range of 61.18–112.46% and 77.13–130.19 (in Xiangyaxianzhan) and 66.06–79.21% and 97.46–100.50% (in Basmati-385), at HS and MS, respectively. In general, the degree of oxidative stress was highest in Xiangyaxianzhan followed by Meixiangzhan-2 and Basmati-385 while the values of percentage increase for MDA and H2O2 contents and leaf leachates were higher at MS than HS (Table 2).
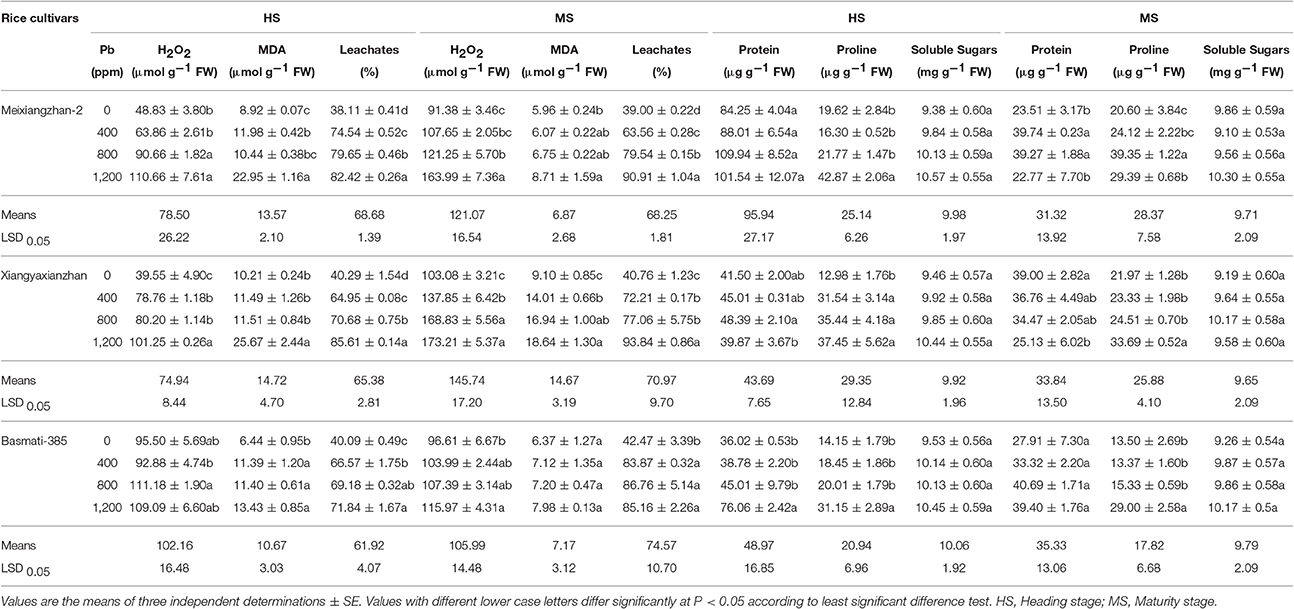
Table 2. Effect of different Pb concentrations on oxidative stress indicators, protein and osmolyte accumulation of three scented rice cultivars at heading and maturity stages.
Pb toxicity regulated the production protein, proline and soluble sugars in all rice cultivars at both HS and MS; however their concentrations were remained lower at MS than HS. Compared with control, the protein and proline contents of all rice cultivars (except protein contents in Meixiangzhan-2 at HS and in Basmati-385 at MS) were significantly affected (P < 0.05) by different Pb treatments, however, the contents of soluble sugars were remained statistically similar (P > 0.05) at both sampling stages. On average, at HS, Meixiangzhan-2 accumulated highest protein and proline contents while Basmati-385 accumulated higher soluble sugars at this stage whilst at MS, higher protein and soluble sugars were recorded in Basmati-385 (Table 2).
SOD, POD, CAT, and APX Activities
All Pb application levels affect variably to the enzymatic anti-oxidants in terms of SOD, POD, CAT, and APX activities in all rice cultivars at both HS and MS. At HS, the activity of SOD increased up to 25.53 and 32.66% at 400 ppm for Meixiangzhan-2 and Xiangyaxianzhan, respectively and then started to decline till 1,200 ppm; however for Basmati-385, the SOD activities were increased up to 49.41% till 800 ppm and then declined. Even though Pb toxicity changed SOD activities in Basmati-385 but the increase was not reached up to significance level (P > 0.05). The maximum POD activity was recorded at 400 ppm (63.46%) for Meixiangzhan-2, at 800 ppm (11.74%), and at 1,200 ppm (59.72%) for Basmati-385, compared with control. Similarly, the activities of CAT and APX were remained highest at 400 ppm (16.32 and 20.59%) for Meixiangzhan-2, at 800 and 1,200 ppm (4.62 and 134.78%) for Xiangyaxianzhan, and 5.38 and 58.33% for Basmati-385 at the same level of Pb as compared with control. At MS, the SOD activity decreased for Meixiangzhan-2, statistically similar (P > 0.05) for Xiangyaxianzhan and increased for Basmati-385 at all levels of Pb then control. The POD and CAT were recorded highest at 400 and 800 ppm (49.86 and 90.41%) and (2.04 and 57.29%) for Meixiangzhan-2 and Xiangyaxianzhan, respectively as well as at 1,200 ppm (18.53 and 44.76%) for Basmati-385. Similarly at HS, the activities of APX were also found statistically similar (P > 0.05) for all rice cultivars under in all Pb-treatments including control. Overall, the enzymatic activities were remained comparatively higher at HS than MS in all rice cultivars (Table 3).
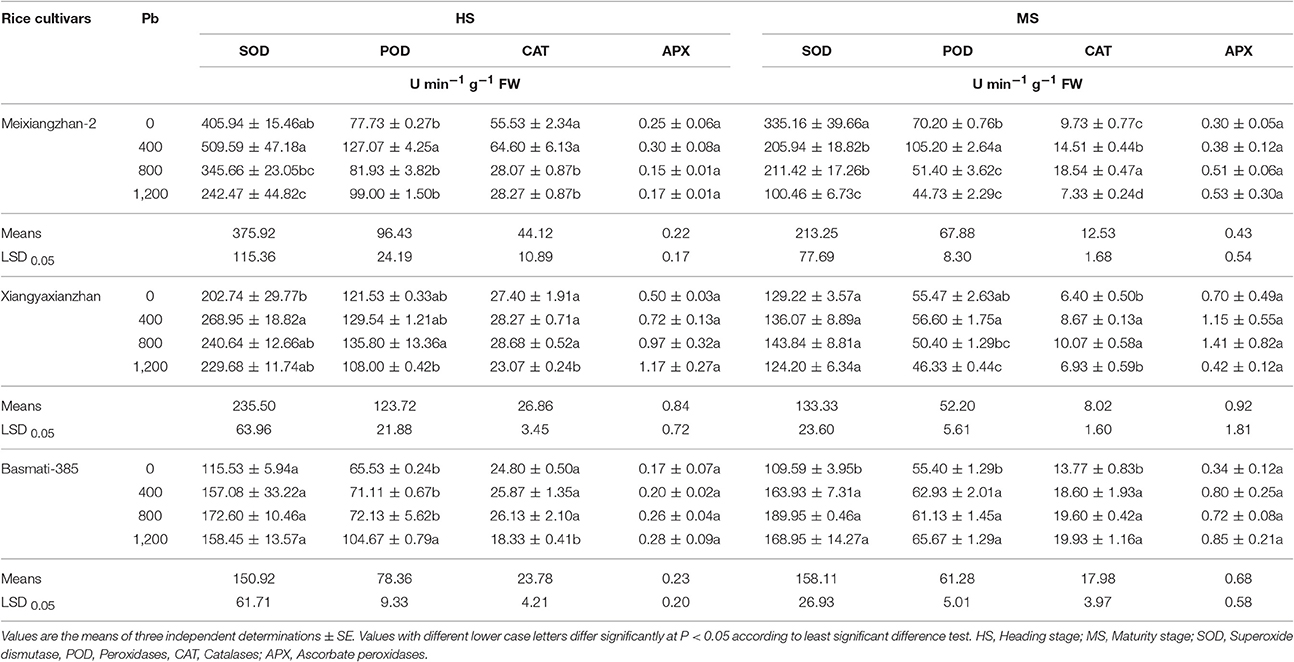
Table 3. Effect of different Pb concentrations on enzymatic anti-oxidants of three scented rice cultivars at heading and maturity stages.
GSH Contents and Reduced to Oxidized GSH (GSSG), Total Glutathione (GSH+GSSG) and GSH/GSH Ratio
In response to Pb, all rice cultivars showed substantial changes in GSH, GSSG, and the total glutathione (GSH+GSSG) at both HS and MS at different Pb-treatments. For instance, at HS, an increase of 67.47, 45.18, and 86.78% in GSH, 88.34, 57.28, and 117.54% in GSSG and 69.16, 39.74, and 88.59% in total glutathione (GSH+GSSG) levels were recorded in Meixiangzhan-2, Xiangyaxianzhan, and Basmati-385, respectively as compared with control. Moreover, at MS, the levels of GSH and GSSG and total GSH+GSSG were remained statistically similar (P > 0.05) for Meixiangzhan-2, and only GSSG for Xiangyaxianzhan for all the treatments including control while GSH and total GSH+GSSG decreased with higher levels of Pb toxicity. The GSH, total GSH+GSSG were found highest at 1,200 ppm and GSSG at 800 ppm with a percentage increase of 26.70, 21.49, and 91.37%, respectively. In addition, the ratio of GSH/GSSG was found statistically similar (P > 0.05) for Meixiangzhan-2 and Basmati-385, and not for Xiangyaxianzhan (highest at 1,200 ppm) at HS. At MS, no significant changes were observed for GSH/GSSG in Meixiangzhan-2 and Xiangyaxianzhan while varied significantly (P < 0.05) in Basmati-385 with an increase from 0 to 800 ppm and then decreased with further increase in Pb-toxicity. Overall, the GSH, GSSG and total GSH+GSSG were higher at MS than HS while the ratio of GSH/GSSG was found higher at HS than MS in all rice cultivars (Table 4).
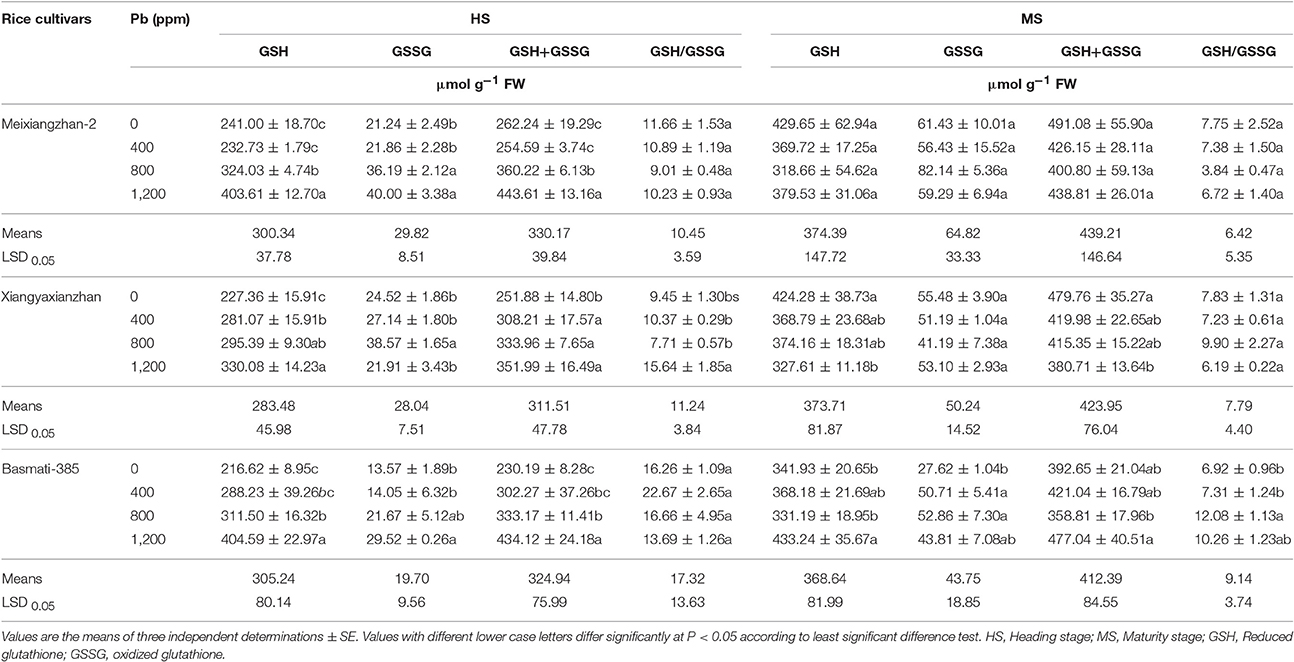
Table 4. Effect of different Pb concentrations on non-enzymatic anti-oxidants of three scented rice cultivars at heading and maturity stages.
Yield and Grain Quality Related Attributes
All Pb-treatments caused considerable reduction in yield and related attributes and resulted in deterioration of grain quality in all rice cultivars (Tables 5, 6), in dose dependent manner. Pb-stress reduced 15.70, 27.03, and 15.63% total tillers/pot, 24.10, 33.33, and 20.27% productive tillers/pot, 19.66, 21.02, and 12.03% grains/ panicle, 9.48, 15.36, and 11.03% 1,000-grain weight, 58.05, 69.12, and 46.27% grain yield pot−1, and 44.24, 61.11, and 39.30% harvest index while 111.95, 230.01, and 55.31% increase in sterility percentage were recorded in Meixiangzhan-2, Xiangyaxianzhan and Basmati-385, respectively. In addition, tillers/pot and 1,000-grain weight for Meixiangzhan-2 while productive tillers/pot, grains/panicle and sterility percentage for Basmati-385 were remained statistically similar (P > 0.05) (Table 5).
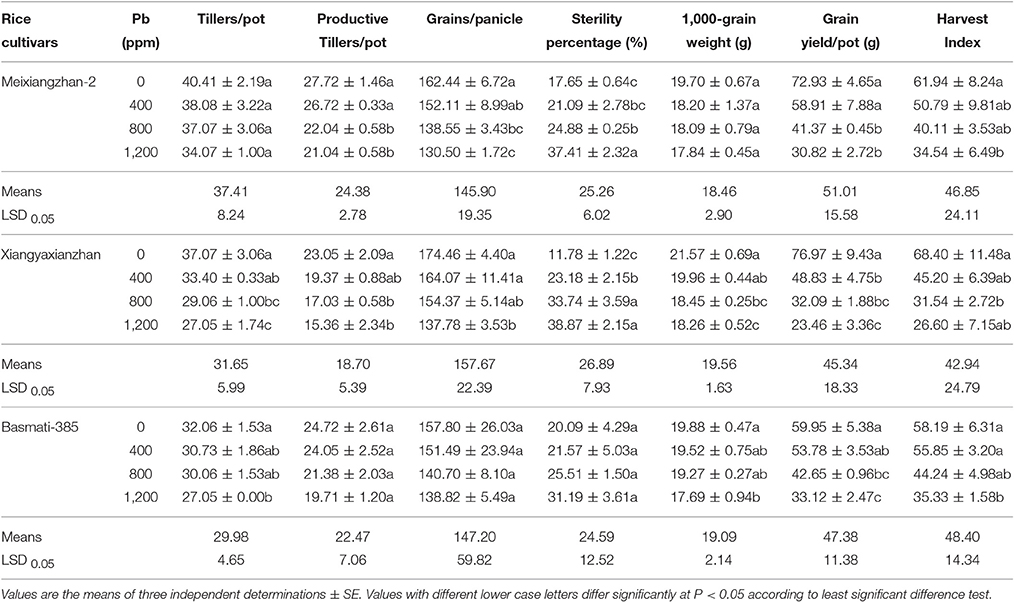
Table 5. Effect of different Pb concentrations yield and yield related components of three scented rice cultivars.
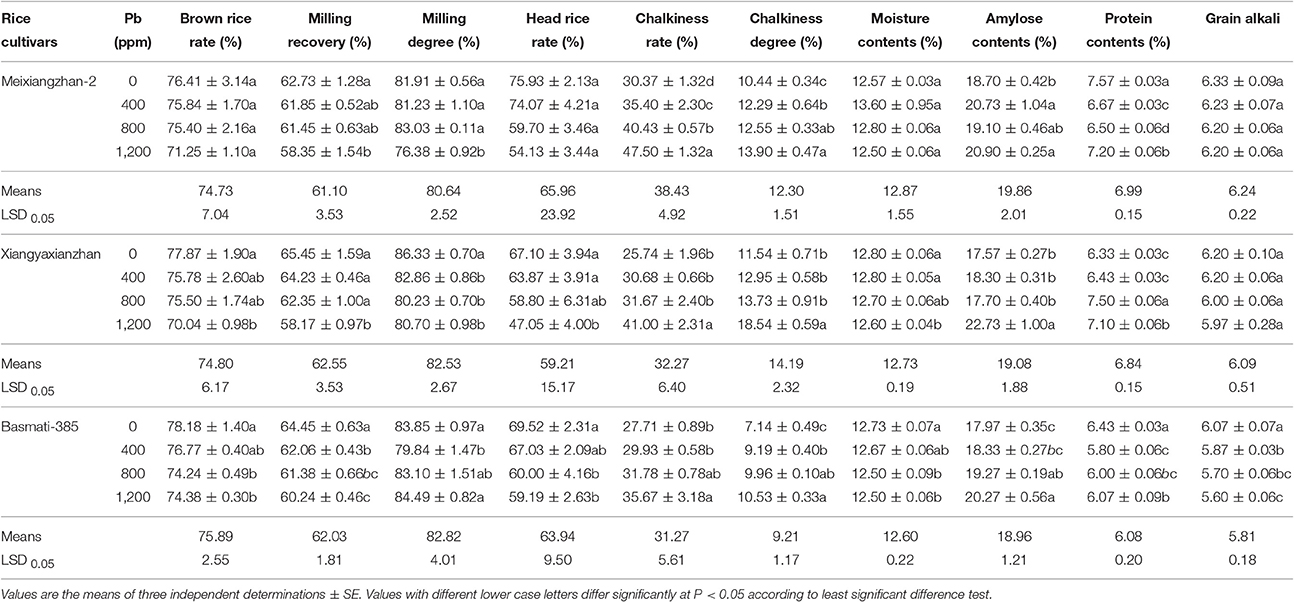
Table 6. Effect of different Pb concentrations on grain quality attributes of three scented rice cultivars.
Pb toxicity reduced brown rice rate (6.76, 10.06, and 4.87%), milling recovery (6.99, 11.12, and 6.53%), milling degree (6.76, 4.18, and 4.78%), head rice rate (28.71, 29.88, and 14.87%), while increased chalkiness rate (56.42, 59.30, and 28.72%) and rice chalkiness degree (33.17, 60.64, and 47.48%) in Meixiangzhan-2, Xiangyaxianzhan and Basmati-385, respectively. Moreover, moisture contents for Meixiangzhan-2 and grain alkali for Meixiangzhan-2 and Xiangyaxianzhan were found statistically similar (P > 0.05) while reduced significantly (P < 0.05) in Basmati-385. Further, reduced grain moisture contents i.e., 1.56 and 1.83% for Xiangyaxianzhan and Basmati-385, and 14.10, 18.42, and 9.84% in grain protein for Meixiangzhan-2, Xiangyaxianzhan and Basmati-385, respectively were recorded. Additionally, grain amylose contents increased under Pb stress conditions, and the values of increase percentage were reached at 11.76, 29.41, and 12.80% for Meixiangzhan-2, Xiangyaxianzhan and Basmati-385, respectively (Table 6).
Correlation Analyses among Yield and Yield Contributing Factors under Pb Toxicity
Significant correlations (P < 0.05) were recorded among grain yield with yield related attributes in all rice cultivars under Pb-treatments. Significant and positive correlations between rice yield with productive tillers/pot (r = 0.98, 0.99, and 0.99) and grains per panicle (0.99, 0.93, and 0.96) were observed in Meixiangzhan-2, Xiangyaxianzhan and Basmati-385, respectively while significant but negative with sterility percentage (r = −0.99 and −0.98) for Xiangyaxianzhan and Basmati-385, respectively; however relationships of grain yield with sterility percentage were remained non-significant (P > 0.05) for Meixiangzhan-2. Furthermore, the relationships of rice grain yield with 1,000-grain weight were only significant for Xiangyaxianzhan (r = 0.99), while remained non-significant (P > 0.05) for Meixiangzhan-2 and Basmati-385 under Pb-toxicity (Figure 1).
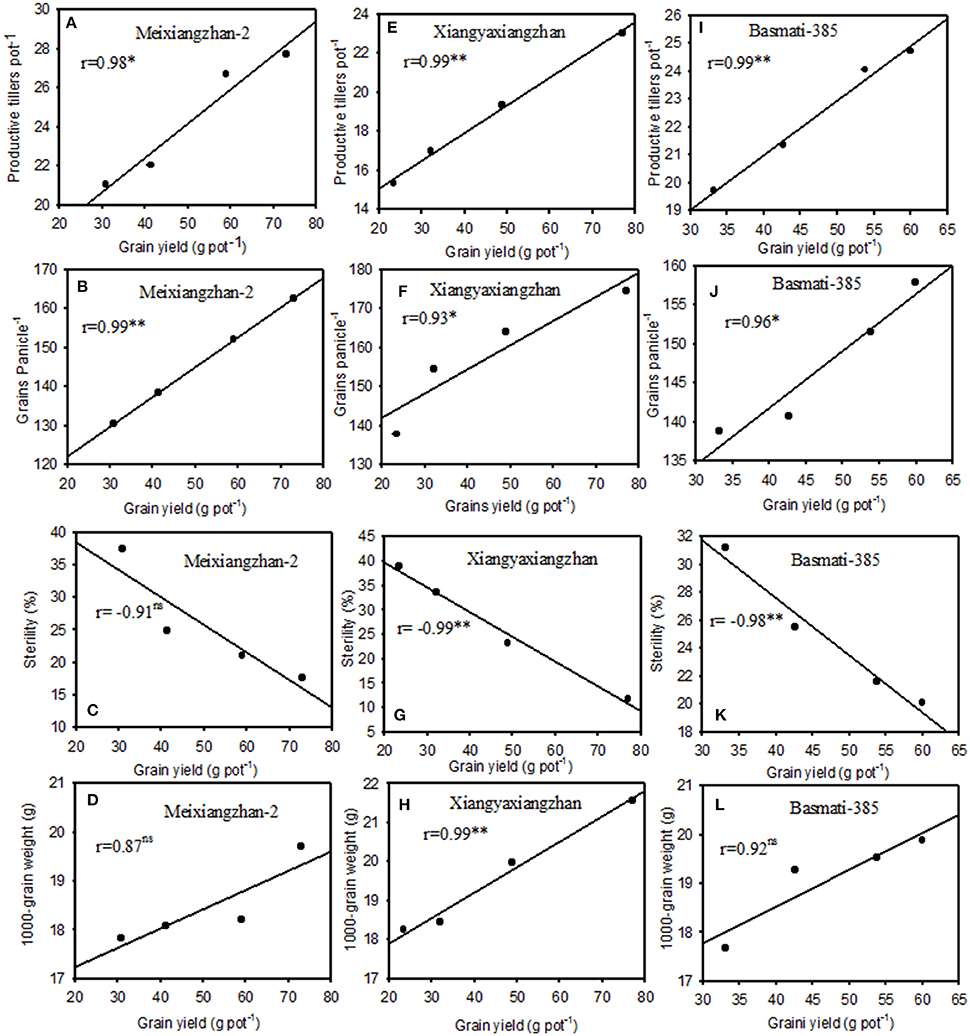
Figure 1. Relationships between grain yield per pot with productive tiller pot−1, grains panicle−1, sterility (%), and 1,000-grain weight for Meixiangzha-2 (A–D), for Xiangyaxiangzhan (E–H), and for Basmati-385 (I–L) under different Pb concentrations using polynomial linear regression analyses. *Significant at P < 0.05, **Significant at P < 0.05, ns, non-significant.
Pb Uptake and Percentage Accumulation in Different Plant Parts
The percentage Pb contents within plants parts are increased with an increase in the concentration of Pb applied. The roots stored the maximum proportions of Pb contents in all rice cultivars at all Pb-treatments. At HS, the root Pb proportions were remained up to 9.98, 6.82, and 4.65-fold higher for Meixiangzhan-2, 8.08, 4.44, and 3.76-fold higher for Xiangyaxianzhan and 6.78, 3.22, 3.13-fold higher for Basmati-385 than the combined/total Pb proportions (%) in stems, leaves and Meixiangzhan-2, Xiangyaxianzhan and Basmati-385, respectively at 400, 800, and 1,200 ppm soil Pb levels, respectively; however, the values were decreased as soil Pb level increased. Further stems accumulated 1.95, 1.87, and 3.76-fold higher Pb contents (%) than leaves and 13.60, 15.83, and 35.68-fold higher than ear Pb contents while the leaves Pb contents were remained 8.55, 8.45, and 9.49-fold higher than ears of Meixiangzhan-2, Xiangyaxianzhan and Basmati-385, respectively. At MS, root stored 7.53, 7.86, and 6.39-fold of Pb contents than all other plant parts whereas the stems Pb contents were 2.69, 1.95, and 3.78 times higher Pb stored than leaves of Meixiangzhan-2, Xiangyaxianzhan and Basmati-385, respectively. Moreover, grain Pb contents were 29.88, 31.99, and 32.64-fold lower than leaves Pb contents in Meixiangzhan-2, Xiangyaxianzhan and Basmati-385, respectively (Figure 2). Means across all three cultivars showed that the pattern of Pb accumulation in different plant parts as follows (mean values, mg kg−1): root (1,892, 2,916, and 4,606) > stem (140, 420, and 817) > leaves (76, 191, and 362) > ears (16, 26, and 41) at HS whist root (2,163, 3,125, and 4,763) > stem (470, 488, and 957) > leaves (216, 215, and 389) > grains (4.04, 8.44, and 12.39) at 400, 800, and 1,200 ppm Pb concentrations, respectively at MS. Hence, the Pb contents were decreased along the plant from root to ears/grains in all rice cultivars.
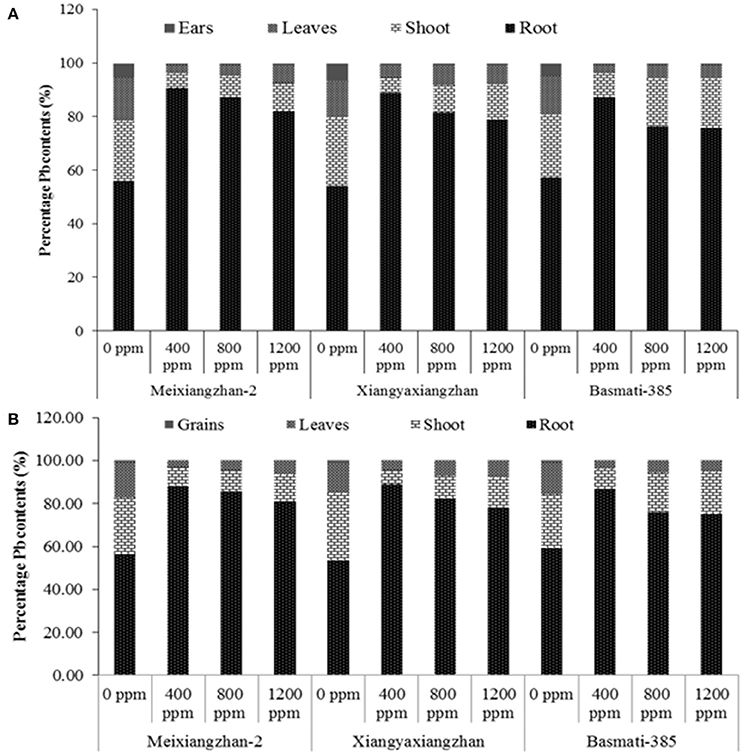
Figure 2. Percentage distribution of Pb contents in (A) root, stems, leaves and ears at heading stage (HS) and (B) root, stems, leaves and grains at maturity stage (MS) in Meixiangzhan-2, Xiangyaxiangzhan and Basmati-385. Each vertical column at each level of Pb represents the respective proportions of Pb contents in all parts under the same Pb level for a given rice cultivar.
Discussion
Present study revealed the toxic effects of Pb on photosynthetic pigments, plant metabolism, yield and quality and its bio-accumulation in three different scented rice cultivars. Pb-toxicity severely affected photosynthetic pigments in Meixiangzhan-2, Xiangyaxianzhan and Basmati-385 and the effects were become more intense with an increase in soil Pb-concentration at both HS and MS. (Table 1). Previous studies demonstrated the leaf chlorosis and/or leaf discoloration and chlorophyll destruction are the consequences of Pb-toxicity (Ernst et al., 2000; Srivastava et al., 2014), as depicted from our studies. Under Pb stress, the central Mg2+ is replaced by Pb2+, thus impaired chlorophyll molecules, caused chlorophyll ultrastructural changes and inhibit chlorophyll biosynthesis (Kupper et al., 1996). Further, Anjum et al. (2016c) also observed significant reduction in Chl a, Chl b, and total Chl a+b in maize facing individual and combined metal toxicity (Al and Cr). Increased chlorophyllase activities under metal toxicity often resulted destruction of chlorophyll production and carotenoids and/or may undergo to photo-oxidation (Luna et al., 1994; Gill et al., 2015).
Pb toxicity led to destructive changes at both HS and MS in scented rice in terms of H2O2 production, lipid peroxidation (MDA production) and membrane damage (electrolyte leakage) and the rate of destruction was highest in Xiangyaxianzhan followed by Meixiangzhan-2 and Basmati-385 (Table 2). Exposure of Pb stress in rice might resulted in over-production of reactive oxygen species (ROS) which oxidize essential cellular structures and cell membranes. MDA production due to peroxidation of polyunsaturated fatty acids is a key indicator of oxidative stress, while leaf leachates provide an estimated degree of membrane damage. Production of H2O2 up to a certain level can play a signaling role to activate the stress-tolerant related genes under abiotic stresses; however, its high rates of production and excess accumulation would lead to severe consequences in plants (Prasad et al., 1994). Pb-induced higher rates of H2O2 production were also reported by Chen et al. (2007) in 18-d old rice seedlings. Excessive rate of degradation in Xiangyaxianzhan and Meixiangzhan-2 would probably due to high-rate activities of ROS at higher Pb-levels which resulted in loss in membrane integrity and cellular damage than Basmati-385, and showed a Pb-sensitive behavior. Moreover, less ROS-induced injury in Basmati-385 than other two rice cultivars possibly due to reduced rate of production or its resilient abilities to scavenge ROS. Enhanced oxidative damage in terms of ROS production in Pb-sensitive rice seedlings were also described by Verma and Dubey (2003). Similarly, higher rates of oxidative damage and lipid peroxidation were also found in Ni-sensitive rice seedlings “Pant-12” under elevated Ni-levels (Maheshwari and Dubey, 2009).
Osmo-regulation through accumulation of various osmolytes is an important plant defensive response against multiple abiotic stresses (Farooq et al., 2009; Pourrut et al., 2011; Fahad et al., 2016b). In this study, Pb toxicity changed the production/accumulation of protein, proline and soluble sugars; however the rate of production was varied among all three rice cultivars and Pb-toxicity levels. Among three rice cultivars, Xiangyaxianzhan was found least effective regarding osmo-regulation under Pb-toxicity (Table 2). Biosynthesis and relative accumulation of these osmolytes are well-recognized due to a wide range of their functions, i.e., protection and stabilization of cellular membranes, protection of several enzymes, act as osmoticum for turgor maintenance, and scavenging roles against ROS (e.g., proline) in plants under heavy-metal stress (Rodriguez et al., 2012; Ali et al., 2014; Ashraf et al., 2015). Our results further revealed that the production and accumulation of these osmolytes may be reduced with higher rates of Pb-toxicity (Table 1). These dynamics in osmolyte accumulation possibly depends on comparative production and detoxification of ROS within plant cells/tissues, which can be explained that at higher Pb-levels, the production rates and activities of ROS might be too high to be quenched or the rate of osmolyte are not increased with respective to the ROS generated. Dynamic accumulation and roles of these osmolytes under Pb stress were previously reported by Chatterjee et al. (2004) and Piotrowska et al. (2009) who concluded that these osmolytes and protein pools may decrease at high Pb-levels, which may lead to severe oxidative stress. Furthermore, utilization of proteins in Pb detoxification and Pb-induced reduction in free amino acids may disturb N-metabolism in plants (Chatterjee et al., 2004; Gupta et al., 2009). However, increased proline contents which may play a significant role in Pb tolerance under Pb toxicity were recorded in Cassia angustifolia Vahl (Qureshi et al., 2007). Hence, any decrease in the concentrations of osmolytes would affect the Pb-tolerance mechanism in rice.
We have observed significant variations among defense system in terms of SOD, POD, CAT, and APX activities as enzymatic and accumulation of GSH, GSSG and total GSH+GSSG as non-enzymatic anti-oxidants in Meixiangzhan-2, Xiangyaxianzhan and Basmati-385 at both sampling stages; however, the activities of enzymatic and non-enzymatic anti-oxidants were Pb-concentration dependent. Moreover, at initial levels of Pb toxicity, the enzymatic anti-oxidants activities increased, but then decreased abruptly with further increase in Pb toxicity; however, the values of percentage decrease were recorded as Xiangyaxianzhan > Meixiangzhan-2 > Basmati-385 (Tables 3, 4). Upon lead exposure, plants activate their anti-oxidative defense mechanism to quench Pb-generated ROS to minimize oxidative stress; however, the activities of these enzymes can be reduced with metal intensification. Normally, increase or decrease in enzymatic activities depends on plant genotype, extent of stress to which plants are exposed, metal concentration, plant age, form of metal in rhizosphere, and duration of metal exposure (Islam et al., 2008; Singh et al., 2010). A specific Pb level might be inhibitory for one enzyme but promotive for the others; however these dynamics in their activities vary plant to plant. Some researchers declared Pb-induced increase in anti-oxidative defense system (Choudhury and Panda, 2004; Reddy et al., 2005) while other found significant reductions in their activities at higher Pb levels (Mishra et al., 2006; Chen et al., 2007). SOD, a metallo-enzyme, detoxifies to H2O2 and O2, hence play a crucial role to cleanse the ROS while CAT and APX are also key enzyme involved in the catabolism of H2O2 to H2O and O2 (Garg and Manchanda, 2009). Activities of POD provide protection to cell membranes through detoxification of free radicals that normally cause lipid peroxidation (Radotic et al., 2000). In our study, overall activities of these anti-oxidants were remain higher from low to medium Pb levels (400–800 ppm), and then reduced with further increase in Pb concentration; however, such a decrease was low in Basmati-385, which shows its ability to maintain anti-oxidative defense system more efficiently than other two rice cultivars. The possible reason of enhanced activities at lower Pb concentrations could be due to high detoxification rates of ROS produced in the plant tissues, however, at higher rate (1,200 ppm as in our case), the production and activity rate of ROS may be too high that the ROS scavenging activities of anti-oxidants and/or the production rate of these enzymes do not remain high enough to quench ROS at high-Pb concentrations. Both increased and decreased anti-oxidant activities under different heavy metals were previously reported (Guo et al., 2007; Anjum et al., 2016c).
Both GSH and GSSG are the most abundant non-enzymatic anti-oxidants in plants and also provide protection in plant cells from ROS-generated free radicals and serve as redox buffer (Sharma and Dietz, 2009). Our results showed a significant increase in both GSH and GSSG as well as total GSH+GSSG contents in all rice cultivars under Pb-stress, but such an increment (on percentage basis) were higher in Basmati-385 followed by Meixiangzhan-2 and Xiangyaxianzhan (Table 4). GSH has a direct role in Pb-detoxification and an essential component of Halliwell-Asada pathway where inter-converted into GSSG and GSH by two enzymes i.e., glutathione reductase (GR) and dehydroascorbate reductase (DHAR). Increased rates of GSH under Pb stress showed their ability to detoxify ROS by involving in H2O2-scavenging mechanism (Piechalak et al., 2002). Further, higher GSH/GSSG ratio in Basmati-385 was attributed to higher anti-oxidative capacity of this cultivar under Pb stress; while decline in GSH/GSSG ratio at MS than HS representing reduced anti-oxidant activities at MS. Contrary to our results, Srivastava et al. (2014) found decline in GSH contents in rice seedlings under interactive Pb and Cd stress, while both GSH and GSSG contents were increased in Brassica napus under Pb stress (Ali et al., 2014). Recent results of Mostofa et al. (2015) are in accordance with our studies who found a considerable increase in glutathione contents in rice seedlings under Cu stress.
Yield and quality components of all rice cultivars differentially affected by Pb-toxicity; however the reductions were more apparent in Xiangyaxiangzhan followed by Meixiangzhan-2 whilst Basmati-385 was least affected by Pb-stress (Tables 5, 6). External environment and genotypes interactions as well as crop management are the factors that are directly related to the yield formation and quality attributes of aromatic rice. Reductions in yield components under Pb toxicity would lead to reduced grain yield and harvest indices of all rice cultivars with maximum yield loss in Xiangyaxiangzhan. Previously, Pb concentration-related changes in rice yields were also observed, for example, soil Pb concentration at 1,000 mg kg−1 resulted in about 12% rice yield reduction (Gu et al., 1989), improved up to 2.54% (at 100 mg kg−1) and then decreased to 4.61% as soil Pb concentrations increased to 300 mg kg−1 of soil (Wang and Wu, 1997). Furthermore, Xie and Huang (1994) argued that low Pb levels could enhance rice tillering ability while would lead to significant reductions as soil Pb concentrations increased. Plant external factors led to significant changes in rice quality attributes especially in aromatic rice cultivars. Significant reductions in rice quality attributes i.e., brown and milled rice rates as well as head milled rice rate were found in two rice cultivars, “Yuxiangyouzhan” and “Tianyou998” exposed to individual (Pb and Cd) and combined metal (Pb+Cd) toxicity (Wang et al., 2010). Influences of external factors on rice quality attributes including rice chalkiness, amylose and grain protein contents grain amylose were also previously reported (Li et al., 2016; Mo et al., 2016). In addition, we have observed significant relationships of rice grain yield with most of the yield related attributes in all rice cultivars (Figure 1). Our results also corroborates with the findings of Liu et al. (2003) who concluded that rice yield components could have significant relationship with paddy yield under Pb toxic conditions; and any changes in yield components would have an ultimate effect of grain yield of rice.
In our study, all three aromatic rice cultivars accumulated different proportions of Pb contents in different plant parts; nonetheless the trend for Pb distribution proportion (from minimum to maximum) for all rice cultivars were recorded as: grain (at MS) < ear (at PH) < leaves < stems < root. Plant Pb contents were also affected by soil Pb-concentration (Figure 2). Higher Pb contents in above ground plant parts impart rice morphology and physiology, and would thus result in yield penalty. More transportation of Pb to grain may lead to quality deterioration and would raise grain quality issues. Among three rice cultivars, highest losses in Xiangyaxiangzhan may be related to higher Pb contents accumulated in above ground plant parts than Meixiangzhan-2 and Basmati-385. Plant characteristics and presence of Pb form in the soil solution would normally determine the fate of Pb in soil-plant-Pb interaction (Stoltz and Greger, 2002). Soil Pb concentration may possibly increase its accumulation in edible plant parts, regardless its mobilization rate from soil to plant (Li et al., 2007). Our results of increased soil Pb concentrations related increase in grain Pb contents are in accordance with Feng et al. (2011). On the other hand, Liu et al. (2015) reported that soil Pb contents and its respective accumulation in the grains has no one-to-one correlations and is not the only determinant for grain Pb accumulation in rice which suggests not only the soil Pb contents but also various other factors may also involve in accumulation and transportation of Pb in the plants. Hence, Pb transformation, transportation and accumulation mechanisms in soil-plant system are quite complex and would largely depends on plant characters, soil physicochemical properties and biological activities within the soil (Zhao et al., 2010). However, Pb uptake and its internal distribution with different plant parts would determine sensitivity and tolerance index in rice (Rout et al., 2001).
Conclusions
This study concluded that Pb toxicity may have severe consequences on rice yield and quality characters by changing its internal physio-biochemical mechanisms and inhibiting biosynthesis of photosynthetic pigments in scented rice. Among studied rice cultivars Basmati-358, Meixiangzhan-2 and Xiangyaxiangzhan proved tolerant, medium, sensitive to lead (Pb) with respect to percentage yield reduction and Pb accumulation behavior (higher in edible plant parts than other two rice cultivars). Moreover, higher Pb concentrations in the soil led to increased Pb proportions of Pb contents in grains of all rice cultivars; but the accumulation pattern from minimum to maximum was observed as: grain (at MS) < ear (at HS) < leaves < stems < root. In our case, although all Pb levels damaged the aromatic rice cultivars while soil Pb level exceeding 800 ppm resulted in significant losses in all rice cultivars. Hence, soil Pb level beyond 800 ppm could be toxic for fragrant rice cultivars and could bring significant yield losses along with rice quality deterioration.
Author Contributions
UA, ZM, and XT designed the research. UA, AK, and QD performed the experiments and collected the data. UA, AK, and QD performed the traits investigated and laboratory analyses. UA analyzed the data and wrote the manuscript. SP, HT, and XT provided guidance during experimentation. All authors approved the final version of the manuscript.
Conflict of Interest Statement
The authors declare that the research was conducted in the absence of any commercial or financial relationships that could be construed as a potential conflict of interest.
Acknowledgments
This work was financially supported by National Natural Science Foundation of China (31271646), Natural Science Foundation of Guangdong Province (8151064201000017), Agricultural Research Projects of Guangdong Province (2011AO20202001), and Agricultural Standardization Project of Guangdong Province (4100 F10003).
References
Abrahams, P. W. (2002). Soils: Their implications to human health. Sci. Total Environ. 291, 1–32. doi: 10.1016/S0048-9697(01)01102-0
Ali, B., Xu, X., Gill, R. A., Yang, S., Ali, S., Tahir, M., et al. (2014). Promotive role of 5-aminolevulinic acid on mineral nutrients and antioxidative defense system under lead toxicity in Brassica napus. Ind. Crop Prod. 52, 617–626. doi: 10.1016/j.indcrop.2013.11.033
Anjum, S. A., Ashraf, U., Khan, I., Tanveer, M., Ali, M., Hussain, I., et al. (2016b). Chromium and aluminum phytotoxicity in maize: morpho-physiological responses and metal uptake. Clean Soil Air Water 44, 1–10. doi: 10.1002/clen.201500532
Anjum, S. A., Ashraf, U., Khan, I., Tanveer, M., Saleem, M. F., and Wang, L. C. (2016a). Aluminum and chromium toxicity in maize: implications for agronomic attributes, net photosynthesis, physio-biochemical oscillations, and metal accumulation in different plant parts. Water Air Soil Pollut. 227, 326. doi: 10.1007/s11270-016-3013-x
Anjum, S. A., Ashraf, U., Saleem, M. F., and Wang, L. (2016c). Chromium toxicity induced alterations in growth, photosynthesis, gas exchange attributes and yield formation in maize. Pak. J. Agri. Sci. 53, 751–757. doi: 10.21162/PAKJAS/16.3824
Arnon, D. T. (1949). Copper enzyme in isolated chloroplasts polyphenoloxidase in Beta vulgaris. Plant Physiol. 24, 1–15. doi: 10.1104/pp.24.1.1
Ashraf, U., Kanu, A. S., Mo, Z. W., Hussain, S., Anjum, S. A., Khan, I., et al. (2015). Lead toxicity in rice; effects, mechanisms and mitigation strategies-a mini review. Environ. Sci. Pollut. Res. 22, 18318–18332. doi: 10.1007/s11356-015-5463-x
Bates, L. S., Waldren, R. P., and Teare, I. D. (1973). Rapid determination of free proline for water-stress studies. Plant Soil 39, 205–207. doi: 10.1007/BF00018060
Bradford, M. N. (1976). A rapid and sensitive method for the quantitation of microgram quantities of protein utilizing the principle of protein-dye binding. Anal. Chem. 72, 248–254. doi: 10.1016/0003-2697(76)90527-3
Chatterjee, C., Dube, B. K., Sinha, P., and Srivastava, P. (2004). Detrimental effects of lead phytotoxicity on growth, yield, and metabolism of rice. Commun. Soil Sci. Plant Anal. 35, 255–265. doi: 10.1081/CSS-120027648
Chen, J., Zhu, C., Li, L., Sun, Z., and Pan, X. (2007). Effects of exogenous salicylic acid on growth and H2O2-metabolizing enzymes in rice seedlings under lead stress. J. Environ. Sci. 19, 44–49. doi: 10.1016/S1001-0742(07)60007-2
Choudhury, S., and Panda, S. (2004). Toxic effects, oxidative stress and ultrastructural changes in moss Taxithelium nepalense (Schwaegr.) Broth. under chromium and lead phytotoxicity. Water Air Soil Pollut. 167, 73–90. doi: 10.1007/s11270-005-8682-9
Clemens, S. (2006). Toxic metal accumulation, response to exposure and mechanism of tolerance in plants. Biochimie 88, 1707–1719. doi: 10.1016/j.biochi.2006.07.003
Ernst, W. H. O., Nielssen, H. G. M., and Ten Bookum, W. M. (2000). Combination toxicology of metal-enriched soils: physiological responses of a Zn- and Cd-resistant ecotypes of Silene vulgaris on polymetallic soils. Environ. Exp. Bot. 43, 55–71. doi: 10.1016/S0098-8472(99)00048-9
Fahad, S., Hussain, S., Saud, S., Hassan, S., Chauhan, B. S., Khan, F., et al. (2016a). Responses of rapid viscoanalyzer profile and other rice grain qualities to exogenously applied plant growth regulators under high day and high night temperatures. PLoS ONE 11:e0159590. doi: 10.1371/journal.pone.0159590
Fahad, S., Hussain, S., Saud, S., Khan, F., Hassan, S., Nasim, W., et al. (2016b). Exogenously applied plant growth regulators affect heat-stressed rice pollens. J. Agron. Crop Sci. 202, 139–150. doi: 10.1111/jac.12148
Farooq, M., Wahid, A., Kobayashi, N., Fujita, D., and Basra, S. M. A. (2009). Plant drought stress: effects, mechanisms and management. Agron. Sustain. Dev. 29, 185–212. doi: 10.1051/agro:2008021
Feng, J., Wang, Y., Zha, J., Zhu, L., Bian, X., and Zhang, W. (2011). Source attributions of heavy metals in rice plant along highway in Eastern China. J. Environ. Sci. 23, 1158–1164. doi: 10.1016/S1001-0742(10)60529-3
Garg, N., and Manchanda, G. (2009). ROS generation in plants: boon or bane? Plant Biosys. 143, 8–96. doi: 10.1080/11263500802633626
Gill, R. A., Zang, L., Ali, B., Farooq, M. A., Cui, P., Yang, S., et al. (2015). Chromium-induced physio-chemical and ultrastructural changes in four cultivars of Brassica napus L. Chemosphere 120, 154–164. doi: 10.1016/j.chemosphere.2014.06.029
Gu, S. H., Zhu, J. Z., and Gu, Z. L. (1989). Study on the critical lead content of red paddy soil. Agro. Environ. Prot. 8, 17–22.
Guo, T. R., Zhang, G. P., and Zhang, Y. H. (2007). Physiological changes in barley plants under combined toxicity of aluminum, copper and cadmium. Colloids Surf B. Biointerfaces 57, 182–188. doi: 10.1016/j.colsurfb.2007.01.013
Gupta, D., Nicoloso, F., Schetinger, M., Rossato, L., Pereira, L., Castro, G., et al. (2009). Antioxidant defense mechanism in hydroponically grown Zea mays seedlings under moderate lead stress. J. Hazard. Mater. 172, 479–484. doi: 10.1016/j.jhazmat.2009.06.141
Hodges, D. M., DeLong, J. M., Forney, C. F., and Prange, R. K. (1999). Improving the thiobarbituric acid-reactive-substances assay for estimating lipid peroxidation in plant tissues containing anthocyanin and other interfering compounds. Planta 207, 604–611. doi: 10.1007/s004250050524
Islam, E., Liu, D., Li, T., Yang, X., Jin, X., Mahmood, Q., et al. (2008). Effect of Pb toxicity on leaf growth, physiology and ultrastructure in the two ecotypes of Elsholtzia argyi. J. Hazard. Mater. 154, 914–926. doi: 10.1016/j.jhazmat.2007.10.121
Kumar, A., Prasad, M. N. V., and Sytar, O. (2012). Lead toxicity, defense strategies and associated indicative biomarkers in Talinum triangulare grown hydroponically. Chemosphere 89, 1056–1065. doi: 10.1016/j.chemosphere.2012.05.070
Kupper, H., Kupper, F., and Spiller, M. (1996). Environmental relevance of heavy metal substituted chlorophylls using the example of water plants. J. Exp. Bot. 47, 259–266. doi: 10.1093/jxb/47.2.259
Li, J. X., Yang, X. E., He, Z. L., Jilani, G., Sun, C. Y., and Chen, S. M. (2007). Fractionation of lead in paddy soils and its bioavailability to rice plants. Geoderma 141, 174–180. doi: 10.1016/j.geoderma.2007.05.006
Li, M., Ashraf, U., Tian, H., Mo, Z. W., Pan, S., Anjum, S. A., et al. (2016). Manganese induced regulations in growth, yield formation, quality characters, rice aroma and enzyme involved in 2-acetyl-1-pyrroline biosynthesis in fragrant rice. Plant Physiol. Biochem. 103, 167–175. doi: 10.1016/j.plaphy.2016.03.009
Liu, J., Li, K., Xu, J., Zhang, Z., Ma, T., Lu, X., et al. (2003). Lead toxicity, uptake, and translocation in different rice cultivars. Plant Sci. 165, 793–802. doi: 10.1016/S0168-9452(03)00273-5
Liu, Z., Zhang, Q., Han, T., Ding, Y., Sun, J., Wang, F., et al. (2015). Heavy metal pollution in a soil-rice system in the Yangtze river region of China. Int. J. Environ. Res. Public Health 13:63. doi: 10.3390/ijerph13010063
Luna, C. M., Gonza'lez, C. A., and Trippi, V. S. (1994). Oxidative damage caused by an excess of copper in oat leaves. Plant Cell Physiol. 35, 11–15.
Maheshwari, R., and Dubey, R. S. (2009). Nickel-induced oxidative stress and the role of antioxidant defence in rice seedlings. Plant Growth Regul. 59, 37–49. doi: 10.1007/s10725-009-9386-8
Mishra, A., and Choudhary, M. A. (1998). Amelioration of lead and mercury effects on germination and rice seedling growth by antioxidants. Biol. Plantarum. 41, 469–473. doi: 10.1023/A:1001871015773
Mishra, S., Srivastava, S., Tripathi, R., Kumar, R., Seth, C., and Gupta, D. (2006). Lead detoxification by coontail (Ceratophyllum demersum L.) involves induction of phytochelatins and antioxidant system in response to its accumulation. Chemosphere 65, 1027–1039. doi: 10.1016/j.chemosphere.2006.03.033
Mittler, R. (2002). Oxidative stress, antioxidant and stress tolerance. Trends Plant Sci. 7, 841–851. doi: 10.1016/S1360-1385(02)02312-9
Mo, Z., Huang, J., Xiao, D., Ashraf, U., Duan, M., Pan, S., et al. (2016). Supplementation of 2-Ap, Zn and La improves 2-acetyl-1-pyrroline concentrations in detached aromatic rice panicles in vitro. PLoS ONE 11:e0149523. doi: 10.1371/journal.pone.0149523
Mostofa, M. G., Hossain, M. A., Fujita, M., and Tran, L. S. (2015). Physiological and biochemical mechanisms associated with trehalose-induced copper-stress tolerance in rice. Sci. Rep. 5:11433. doi: 10.1038/srep11433
Piechalak, A., Tomaszewska, B., Baralkiewicz, D., and Malecka, A. (2002). Accumulation and detoxification of lead ions in legumes. Phytochemistry 60, 153–162. doi: 10.1016/S0031-9422(02)00067-5
Piotrowska, A., Bajguz, A., Godlewska-Zylkiewicz, B., Czerpak, R., and Kaminska, M. (2009). Jasmonic acid as modulator of lead toxicity in aquatic plant Wolffia arrhiza (Lemnaceae). Environ. Exp. Bot. 66, 507–513. doi: 10.1016/j.envexpbot.2009.03.019
Pourrut, B., Shahid, M., Camille, D., Peter, W., and Eric, P. (2011). Lead uptake, toxicity, and detoxification in plants. Rev. Environ. Contam. Toxicol. 213, 113–136. doi: 10.1007/978-1-4419-9860-6_4
Prasad, T. K., Anderson, M. D., Martin, B. A., and Stewart, C. R. (1994). Evidence for chilling-induced oxidative stress in maize seedlings and a regulatory role for hydrogen peroxide. Plant Cell 6, 65–74. doi: 10.1105/tpc.6.1.65
Qureshi, M., Abdin, M., Qadir, S., and Iqbal, M. (2007). Lead-induced oxidative stress and metabolic alterations in Cassia angustifolia Vahl. Biol. Plantarum. 51, 121–128. doi: 10.1007/s10535-007-0024-x
Radotic, K., Ducic, T., and Mutavdzic, D. (2000). Changes in peroxidase activity and isoenzymes in spruce needles after exposure to different concentrations of cadmium. Environ. Exp. Bot. 44, 105–113. doi: 10.1016/S0098-8472(00)00059-9
Reddy, A. M., Kumar, S. G., Jyothsnakumari, G., Thimmanaik, S., and Sudhakar, C. (2005). Lead induced changes in antioxidant metabolism of horsegram (Macrotyloma uniflorum (Lam.) Verdc.) and bengalgram (Cicer arietinum L.). Chemosphere 60, 97–104. doi: 10.1016/j.chemosphere.2004.11.092
Rodriguez, C., Santos, E., Azevedo, R., Moutinho-Pereira, J., Correia, C., and Dias, M. C. (2012). Chromium (VI) induces toxicity at different photosynthetic levels in pea. Plant Physiol. Biochem. 53, 94–100. doi: 10.1016/j.plaphy.2012.01.013
Rout, G. R., Samantaray, S., and Das, P. (2001). Differential lead tolerance of rice and black gram genotypes in hydroponic culture. Rost. Výroba (Praha) 47, 541–548.
Shahid, M., Pinelli, E., Pourrut, B., Silvestre, J., and Dumat, C. (2011). Lead-induced genotoxicity to Vicia faba L. roots in relation with metal cell uptake and initial speciation. Ecotoxicol. Environ. Saf. 74, 78–84. doi: 10.1016/j.ecoenv.2010.08.037
Sharma, P., and Dubey, R. S. (2005). Lead toxicity in plants. Braz. J. Plant Physiol. 17, 35–52. doi: 10.1590/S1677-04202005000100004
Sharma, S. S., and Dietz, K. J. (2009). The relationship between metal toxicity and cellular redox imbalance. Trend Plant Sci. 14, 43–50. doi: 10.1016/j.tplants.2008.10.007
Singh, R. K., Singh, U. S., and Khush, G. S. (2000). Aromatic Rices. New Delhi: Oxford & IBH Publishing Co. Pvt. Ltd.
Singh, R., Tripathi, R. D., Dwivedi, S., Kumar, A., Trivedi, P. K., and Chakrabarty, D. (2010). Lead bioaccumulation potential of an aquatic macrophyte Najas indica are related to antioxidant system. Bioresour. Technol. 101, 3025–3032. doi: 10.1016/j.biortech.2009.12.031
Srivastava, R. K., Pandey, P., Rajpoot, R., Rani, A., and Dubey, R. S. (2014). Cadmium and lead interactive effects on oxidative stress and antioxidative responses in rice seedlings. Protoplasma 251, 1047–1065. doi: 10.1007/s00709-014-0614-3
Stoltz, E., and Greger, M. (2002). Accumulation properties of As, Cd, Cu, Pb and Zn by four wetland plant species growing on submerged mine tailings. Environ. Exp. Bot. 47, 271–280. doi: 10.1016/S0098-8472(02)00002-3
Valentovic, P., Luxova, M., Kolarovic, L., and Gasparikova, O. (2006). Effect of osmotic stress on compatible solutes content, membrane stability and water relations in two maize cultivars. Plant Soil Environ. 52, 186–191.
Velikova, V., Yordanov, I., and Edreva, A. (2000). Oxidative stress and some antioxidant systems in acid rain-treated bean plants: protective role of exogenous polyamines. Plant Sci. 151, 59–66. doi: 10.1016/S0168-9452(99)00197-1
Verma, S., and Dubey, R. S. (2003). Lead toxicity induces lipid peroxidation and alters the activities of antioxidant enzymes in growing rice plants. Plant Sci. 164, 645–655. doi: 10.1016/S0168-9452(03)00022-0
Wang, X., and Wu, Y. Y. (1997). Behavior property of heavy metals in soil-rice system. Chin. J. Ecol. 16, 10–14.
Wang, Y. Q., Xiao, L. Z., Li, S. B., Yang, G., and Cai, X. D. (2010). Effects of combined pollution of Pb and Cd on growth and yield of rice. J. Anhui Agric Sci. 38, 12653–12655. doi: 10.13989/j.cnki.0517-6611.2010.23.214
Xie, Z. M., and Huang, C. Y. (1994). Relationships between lead zinc arsenic contents and rice tillering in soil-rice system. J. Zhejiang Agric. Univ. 20, 67–71.
Zhang, G., Chen, M., Li, L., Xu, Z., Chen, X., Guo, J., et al. (2009). Overexpression of the soybean GmERF3 gene, an AP2/ERF type transcription factor for increased tolerances to salt, drought, and diseases in transgenic tobacco. J. Exp. Bot. 60, 3781–3796. doi: 10.1093/jxb/erp214
Zhang, W. F., Zhang, F., Raziuddin, R., Gong, H. J., Yang, Z. M., Lu, L., et al. (2008). Effects of 5-aminolevulinic acid on oilseed rape seedling growth under herbicide toxicity stress. J. Plant Growth Regul. 27, 159–169. doi: 10.1007/s00344-008-9042-y
Zhao, K., Liu, X. M., Xu, J. M., and Selim, H. M. (2010). Heavy metal contaminations in a soil-rice system: Identification of spatial dependence in relation to soil properties of paddy fields. J. Hazard. Mater. 181, 778–787. doi: 10.1016/j.jhazmat.2010.05.081
Keywords: antioxidant defense, grain yield, lead, oxidative stress, scented rice, quality characters
Citation: Ashraf U, Kanu AS, Deng Q, Mo Z, Pan S, Tian H and Tang X (2017) Lead (Pb) Toxicity; Physio-Biochemical Mechanisms, Grain Yield, Quality, and Pb Distribution Proportions in Scented Rice. Front. Plant Sci. 8:259. doi: 10.3389/fpls.2017.00259
Received: 03 October 2016; Accepted: 13 February 2017;
Published: 28 February 2017.
Edited by:
Zhenfei Guo, Nanjing Agricultural University, ChinaReviewed by:
Rudra Deo Tripathi, CSIR-National Botanical Research Institute, IndiaFahd Rasul, University of Agriculture Faisalabad (UAF), Pakistan
Copyright © 2017 Ashraf, Kanu, Deng, Mo, Pan, Tian and Tang. This is an open-access article distributed under the terms of the Creative Commons Attribution License (CC BY). The use, distribution or reproduction in other forums is permitted, provided the original author(s) or licensor are credited and that the original publication in this journal is cited, in accordance with accepted academic practice. No use, distribution or reproduction is permitted which does not comply with these terms.
*Correspondence: Xiangru Tang, tangxr@scau.edu.cn