- 1The Genetic Engineering International Cooperation Base of Chinese Ministry of Science and Technology, Key Laboratory of Molecular Biophysics of Chinese Ministry of Education, College of Life Science and Technology, Huazhong University of Science and Technology, Wuhan, China
- 2School of Life Sciences and Engineering, Henan University of Urban Construction, Pingdingshan, China
- 3Key Laboratory of Herbage and Endemic Crop Biotechnology, Ministry of Education, College of Life Sciences, Inner Mongolia University, Hohhot, China
Sucrose non-fermenting 1-related protein kinase 2 (SnRK2) family members play crucial roles in plant abiotic stress response. However, the precise mechanism underlying the function of SnRKs has not been thoroughly elucidated in plants. In this research, a novel SnRK2 gene, TaSnRK2.9 was cloned and characterized from common wheat. The expression of TaSnRK2.9 was upregulated by polyethylene glycol (PEG), NaCl, H2O2, abscisic acid (ABA), methyl jasmonate (MeJA), and ethrel treatments. TaSnRK2.9 was mainly expressed in wheat young root, stamen, pistil, and lemma. Overexpressing TaSnRK2.9 in transgenic tobacco enhanced plants’ tolerance to drought and salt stresses both in young seedlings and mature plants with improved survival rate, seed germination rate, and root length. Physiological analyses suggest that TaSnRK2.9 improved antioxidant system such as superoxide dismutase (SOD), catalase (CAT), peroxidase (POD), and glutathione (GSH) to reduce the H2O2 content under drought or salt stress. Additionally, TaSnRK2.9 overexpression plants had elevated ABA content, implying that the function of TaSnRK2.9 may be ABA-dependent. Moreover, TaSnRK2.9 increased the expression of some ROS-related, ABA-related, and stress-response genes under osmotic or salt treatment. TaSnRK2.9 could interact with NtABF2 in yeast two-hybrid assay, and increased the expression of NtABF2 under mannitol or NaCl treatment in transgenic tobacco plants. In conclusion, overexpression of TaSnRK2.9 in tobacco conferred plants tolerance to drought and salt stresses through enhanced ROS scavenging ability, ABA-dependent signal transduction, and specific SnRK-ABF interaction.
Introduction
Abiotic stresses such as drought and high salinity severely affect plants growth, development, and crop productivity (Ruizlozano et al., 2012). To cope with such abiotic stresses, plants have evolved a series of complex physiological mechanisms such as ROS detoxification, ABA signaling pathway, ion balance, and osmosis regulation (Zhang et al., 2018). Previous researches showed that many genes, such as transcription factors and protein kinase genes, participated in response to abiotic stresses. And the SnRK2 plays a key role in plant stress signal transduction pathway.
The CDPK-SnRK superfamily includes seven families of serine-threonine protein kinases: CDPKs, CRKs, PPCKs, PEPRKs, CaMKs, CCaMKs, and SnRKs (Hrabak et al., 2003). In eukaryotic cells, yeast sucrose non-fermenting 1 (SNF1) protein kinase, animal AMPK, and plant SnRK protein kinase are highly conserved and take an active part in plants growth and development processes. Plant SnRKs are divided into three subgroups (SnRK1, SnRK2, and SnRK3) based on their structure difference (Hardie et al., 1998). SnRK1 mainly regulates carbon and nitrogen balance, while SnRK2 and SnRK3, which are plant-specific, play an essential role in stress signal transduction (Hrabak et al., 2003).
Increasing evidences suggest that PYR/PYL/RCARs-type 2C phosphatase (PP2C)-SnRK2 is an ABA-dependent stress signal pathway which is important in plant stress response (Rosenberger and Chen, 2018; Zhao et al., 2018). ABA is an indispensable phytohormone that plays a crucial role in plant stress response (Soma et al., 2017; Vishwakarma et al., 2017; Wang P. et al., 2018). Under normal condition, PP2C inhibited SnRK2s, while subjecting to abiotic stresses, accumulated ABA can be sensed by PYR/PYL/RCAR proteins, resulting in PP2Cs inhibition. SnRK2s are then activated by self-phosphorylation, which in turn induces the expression of a series of ABA-regulated genes (Melcher et al., 2009; Nishimura et al., 2009; Park et al., 2009; Umezawa et al., 2009; Vlad et al., 2009). Many researches show that SnRK2s are positively regulated by ABA and play key roles in plant abiotic stress (Umezawa et al., 2013).
In Arabidopsis, SnRK2s (AtSnRK2.2, AtSnRK2.3, AtSnRK2.6, AtSnRK2.7, and AtSnRK2.8) function redundantly in response to ABA and abiotic stresses (Fujii and Zhu, 2009; Fujita et al., 2009; Nakashima et al., 2009; Mizoguchi et al., 2010). In poplar, PtSnRK2.5 and PtSnRK2.7 positively regulate the plants response to salt stress (Song et al., 2016). In wheat, ten SnRK2s were identified by bioinformatic analyses (Zhang et al., 2016). Overexpressing TaSnRK2.3 or TaSnRK2.4 in Arabidopsis conferred plants enhanced tolerance to drought, salt, and freezing stresses through improved expression of stress-response genes and physiological indices such as decreased water loss and increased osmotic potential (Mao et al., 2010; Tian et al., 2013). Overexpressing TaSnRK2.7 or TaSnRK2.8 in Arabidopsis conferred plants tolerance to drought, salt, and cold stresses with longer primary roots and various physiological characteristics including strengthened cell membrane stability and improved photochemical efficiency (Zhang et al., 2010, 2011). The four identified genes all located in the cell membrane, cytoplasm, and nucleus. They all responded to PEG and NaCl. TaSnRK2.3, TaSnRK2.7, and TaSnRK2.8 responded to cold but TaSnRK2.4 did not. TaSnRK2.7 did not respond to ABA, suggesting that TaSnRK2.7 might be involved in non-ABA-dependent signal transduction pathways.
In this study, TaSnRK2.9 was cloned and functionally characterized from common wheat. To investigate the precise role of TaSnRK2.9 in plant stress response, TaSnRK2.9 was ectopically expressed in tobacco. Our results showed that TaSnRK2.9 overexpression plants exhibited enhanced tolerance to drought and high salinity in young seedlings and mature plants. Further investigation suggests that TaSnRK2.9 improved plant stress tolerance through detoxification of ROS, regulating the expression of ABA-related genes, and interacting with ABFs.
Materials and Methods
Wheat Growth Conditions and Stress Treatments
Common wheat (Triticum aestivum L., cv. Chinese spring) was used in this study. Wheat seeds were surface-sterilized with 75% (v/v) ethanol for 2 min followed by washing three times in double distilled water. Sterilized seeds were germinated and cultured with water in a climate chamber under a 12 h light/12 h dark cycle at 22°C with light intensity of 150 μmol m-2s-1. Two-week-old wheat seedlings with two leaves, were treated by multiple abiotic stresses or signaling molecules. Different stress treatments were carried out by submerging wheat seedling roots in solutions of 20% PEG6000, 200 mM NaCl, and 10 mM H2O2, respectively. For treatments with signaling molecules, seedlings were sprayed with 100 μM ABA, 100 μM MeJA, and 100 μM ethrel, respectively. Wheat seedlings cultivated in water were used as control. Leaves and roots of the seedlings were sampled at different time points (0, 1, 3, 6, 12, and 24 h) after treatments. Wheat plants grown in the field were used to determine organ specific expression of TaSnRK2.9. Young root, young stem, young leaf (at the four-leaf stage), flag leaf, mature stem, mature leaf (at anthesis stage), stamen, pistil, lemma, coleoptile, young spike, and mature spike were sampled. All obtained samples were immediately frozen in liquid nitrogen and stored at -80°C for RNA extraction and subsequent qRT-PCR analysis.
Cloning and Identification of TaSnRK2.9 Gene
To identify TaSnRKs in wheat, in silico cloning was used to predict putative TaSnRK genes. The Triticum urartu gene TuSAPK9 (GenBank: EMS68031.1) with high homology to OsSAPK9 was used as a query probe to blast the EST library of wheat. Then we obtained a wheat EST (HX198182) belonging to TaSnRK family. Since the EST did not show a clear 3′-end, SMART RACE cDNA amplification kit (Clontech, United States) was used to amplify the 3′ end of the gene. Then the full-length cDNA sequence was obtained. The PCR products were cloned into a pMD18-T vector (TaKaRa, Dalian, China) and transfected into E. coli TOP 10 competent cells (Tiangen, Beijing, China). Finally, the target gene in positive clone was sequenced (TSINGKE BioTech, Beijing, China) and analyzed by BLAST.
Bioinformatics Analysis of TaSnRK2.9
Protein sequences analysis was conducted with Clustal W. Then GeneDoc software was used to edit the alignment results. MEGA was selected to construct the phylogenetic tree using the neighbor-joining method (He et al., 2017). All the sequences were downloaded from NCBI database1.
qRT-PCR
Total RNA was extracted from different samples collected above according to Plant Tissue Total RNA Extraction kit (Zomanbio, Beijing, China). Three microliters RNA was used in agarose gel electrophoresis to check the quality and integrity of the obtained RNA samples. First strand cDNA was synthesized from mRNA using RevertAid First Strand cDNA Synthesis Kit (Thermo Fisher Scientific, Lithuania). Expression patterns of TaSnRK2.9 in different wheat organs and under different stress treatments, signal molecules were analyzed by qRT-PCR using the SuperReal PreMix Plus (SYBR Green) (Tiangen, Beijing, China) on a Fluorescence Real-time PCR detection system (Bio-Rad Laboratories, Hercules, CA, United States). PCR was conducted to test efficiency and specificity of the primer pairs, the amplified product was sequenced. TaActin (GenBank: AB181991.1) was used as the internal reference gene. Each sample was measured using three biological replicates. Appropriate negative controls without template were used to check any possible contamination or primer dimers in every single experiment. The relative expression of mRNA was calculated using the 2-ΔΔCt formula.
Subcellular Localization of TaSnRK2.9 Protein
To determine the subcellular localization of TaSnRK2.9 protein, a recombinant construct of pCAMBIA1304::TaSnRK2.9::GFP was transformed into onion epidermal cells using a gene gun (PDS-1000; BIO-RAD, Hercules, CA, United States) in accordance with the instruction manual. Restriction sites were added to the 5′ and 3′ ends of the complete coding sequence without the termination codon of TaSnRK2.9 by PCR using specific primer pairs. The product was subsequently inserted into pCAMBIA1304 vector to generate the TaSnRK2.9-GFP fusion protein under control of the CaMV 35S promoter. Then the pCAMBIA1304-TaSnRK2.9-GFP fusion protein construct was introduced into the onion epidermal cells with a gene gun. The pCAMBIA1304-GFP vector was used as a control. After incubation at 20°C for 24 h in the dark, the tissues were examined by fluorescence microscopy (IX71, Olympus, Japan).
Yeast Two-Hybrid Assay
Yeast two-hybrid assay was used to investigate the interaction between TaSnRK2.9 and NtABFs in vivo. The complete coding sequences of TaSnRK2.9, NtABF1, NtABF2, and NtABF4 were amplified by PCR using primers containing specific restriction sites. TaSnRK2.9 PCR products were inserted into pGADT7 vector. While NtABF1, NtABF2, and NtABF4 PCR products were cloned into pGBKT7 vector to create pGADT7-TaSnRK2.9, pGBKT7-NtABF1, pGBKT7-NtABF2, and pGBKT7-NtABF4 constructs. These recombinant constructs were transformed into yeast strain AH109 by the lithium acetate method. Plasmid pGADT7-T and pGBKT7-lam were used as a negative control, while pGADT7-T and pGBKT7-53 were used as a positive control. After confirmation by screening on selective medium plates (SD/-Trp-Leu), the positive colonies were transferred onto SD/-Trp-Leu-His-Ade plates with X-α-D-Galactosidase (X-α-gal). The protein interaction was determined according to the growth status on the plates after 3 days incubating under 30°C in the dark.
Bimolecular Fluorescence Complementation Assay
For the bimolecular fluorescence complementation (BiFC) assay, recombinant constructs TaSnRK2.9-YNE and NtABF2-YCE were constructed and transferred into Agrobacterium tumefaciens strain EHA105, respectively. Then they were co-transformed into tobacco leaves using transient expression method with a medical syringe. The tobacco plants were placed under normal growth condition after injection. Fluorescence was observed by fluorescence microscope (IX71, Olympus, Japan) 36 h after transformation.
Plant Transformation and Establishment of Transgenic Plants
To generate transgenic plants, the recombinant plasmids pCAMBIA1304-TaSnRK2.9 under the control of the CaMV 35S promoter was introduced into A. tumefaciens strain LBA4404. According to an Agrobacterium-mediated leaf disk transformation method, the pCAMBIA1304-TaSnRK2.9 and empty vector pCAMBIA1304 were transformed into tobacco leaves, respectively. Specific primers were used to confirm positive seedlings to amplify TaSnRK2.9 by PCR. Then positive seedlings were transferred to soil for further study. T1 seeds were germinated and grown on MS medium containing 100 mg/L kanamycin for 2 weeks, then the survived plants were transferred to soil to get T2 seeds. Depending on the transgene expression level, three independent transgenic T3 line seedlings (OE2, OE3, and OE5) were selected to conduct stress tolerance and all the following experiments.
Stress Tolerance Analysis of Transgenic Lines
Wild type (WT), VC, and TaSnRK2.9 overexpression (OE) lines were cultured in MS medium for 1 week and then transplanted into soil. For drought stress tolerance assay, 3-week-old seedlings similar in growth status were subjected to withhold water for 3 weeks. The control plants were watered regularly. Fifty plants from each line were used to detect survival rate after withholding water for 3 weeks. At the 2 week drought stress treatment stage, leaves from each line were collected to measure H2O2, SOD, CAT, POD, IL, RWC, MDA, proline, soluble sugar, and ABA contents. Three weeks after withholding water, photographs were taken. Then the plants were watered for 1 week for recovery.
For the salt stress treatment assay, the plants were irrigated with 300 mM NaCl solution three times a week for 4 weeks. Meanwhile, SOD, CAT, and POD activities, H2O2, GSH, MDA, proline, soluble sugar, and chlorophyll contents, and IL were determined after 2 weeks of salt treatment. The abiotic tolerance experiments were repeated three times.
Measurement of H2O2 Accumulation, Antioxidants, IL, RWC, MDA, Proline, Soluble Sugar, ABA, and Chlorophyll Content
The leaves from the same position of seedlings were collected to assay the physiological indices. For the assessment of H2O2, GSH and proline contents, the enzyme activities of SOD, CAT and POD, detection kits (Jiancheng, China) were used by spectrophotometric method. For the MDA content, thiobarbituric acid method was used according to the method described previously (Heath and Packer, 1968). For the IL, the samples were clipped into small pieces, and then were immersed in test tubes with 10 ml of distilled water at room temperature for 12 h. The initial conductivity (C1) was determined with a conductivity meter (DDBJ-350, Shanghai, China). Subsequently, the samples were boiled for 20 min to release all the ions. After cooling to the room temperature, the conductivity (C2) was determined again. IL was calculated as IL (%) = (C1/C2) × 100% (Hu et al., 2012). For the RWC, leaves were collected and weighed as fresh weight (FW), then they were covered with water for 12 h to measure turgid weight (TW). Subsequently, the leaves were put in the dry oven at 105°C for 15 min, then leaves were dried at 80°C to get dried weight (DW), such that RWC (%) = [(FW-DW)/(TW-DW)] × 100% (Luo et al., 2017). For the soluble sugar content, phenol reaction method was used as described before (Kong et al., 2011). For the ABA content, an ABA ELISA assay kit (Jiancheng, China) was used (Wang Y. et al., 2018). For the chlorophyll content, leaves were grinded into pounder, then soaked in 50 mL ethanol (90%, v/v) tube, and the samples were incubated in a shaker at low temperature for 3 h. The chlorophyll content was analyzed by a spectrophotometer using light at the wave lengths of 665 and 649 nm.
Expression Analyses of Stress-Related Genes
Two-week-old seedlings grown in the MS medium were transplanted to MS medium with 300 mM mannitol or 200 mM NaCl for 7 days. And the whole seedlings were sampled for RNA extraction after treatments. The qRT-PCR was used to analyze the expression of stress-related genes. Additionally, NtActin was used as a reference gene. All primers used in this study are listed in Supplementary Table S1.
Statistical Analysis
Statistical analyses were performed with Excel. All the experiments were carried out at least three times, and the Student’s t-test was applied to compare the difference, at a remarkable difference P < 0.05 or P < 0.01 (Wang X.et al., 2015).
Results
Identification of TaSnRK2.9 Gene From Wheat
A full length cDNA of SnRK was cloned from wheat (Chinese spring) using RACE method. Sequence alignment and phylogenetic analysis (Supplementary Figure S1) showed that it had high similarity to OsSAPK9. Therefore, it was designated TaSnRK2.9 (GenBank accession No. MH844552). TaSnRK2.9 consists a 1080 bp ORF which encodes a 360 amino acid protein with a predicted relative molecular mass of 40.36 kDa and theoretical pI of 4.94. Sequence blast using the Ensembl Plants database2 showed that TaSnRK2.9 is located on wheat 5A chromosome.
Expression Patterns of TaSnRK2.9 Under Various Stress Conditions and in Different Organs
The qRT-PCR was employed to examine the expression patterns of TaSnRK2.9. In the stress treatment assay, 1-week-old wheat seedlings were treated with PEG, NaCl, H2O2, ABA, MeJA, and ethrel, and were sampled at 0, 1, 3, 6, 9, 12, and 24 h, respectively. In organ-specific expression assay, young root, young stem, young leaf, flag leaf, mature stem, mature leaf, stamen, pistil, lemma, coleoptile, young spike, and mature spike from the wheat were collected to extract RNA. As shown in Figures 1A–F, TaSnRK2.9 was significantly upregulated by PEG, approximately 3.7-fold. TaSnRK2.9 expression reached the maximum of almost twofold at 6 h after NaCl treatment. With H2O2, ABA, MeJA, and ethrel treatments, the expression of TaSnRK2.9 was gradually elevated by 4.2-fold at 12 h, 2.2-fold at 12 h, 7.3-fold at 6 h, and 2.8-fold at 6 h, respectively. These results indicate that the expression of TaSnRK2.9 is responsive to PEG, NaCl, H2O2, ABA, MeJA, and ethrel, implying that TaSnRK2.9 may play a role in abiotic stress response. In addition, TaSnRK2.9 was expressed in all the organs examined in wheat (Figure 1G). TaSnRK2.9 expressed at higher levels in young root, stamen, pistil, and lemma, while its expressions were lower in young stem, young leaf, flag leaf, mature stem, mature leaf, coleoptile, young spike, and mature spike.
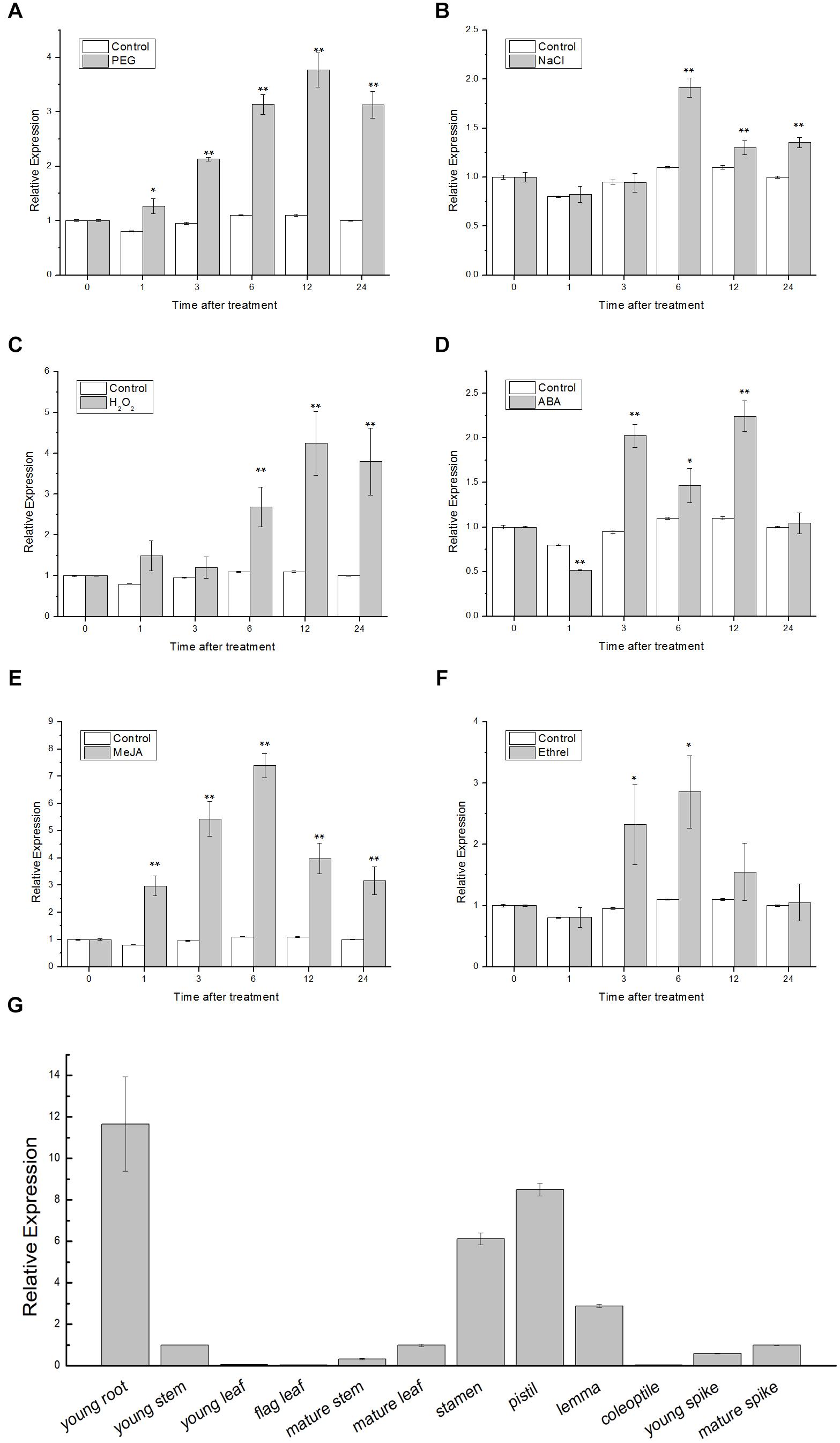
Figure 1. Expression pattern analyses of TaSnRK2.9 gene in wheat. Two-week-old wheat seedling leaves under different treatments including (A) 20% PEG6000, (B) 200 mM NaCl, (C) 10 mM H2O2, (D) 100 μM ABA, (E) 100 μM MeJA, (F) 100 μM ethrel. (G) Organ-specific expression of TaSnRK2.9 in young root, young stem, young leaf, flag leaf, mature stem, mature leaf, stamen, pistil, lemma, coleoptile, young spike, and mature spike. qRT-PCR was used to analyze the expression differences. Values are means ± SD from three independent replicates. Asterisks indicate significant difference between WT and the transgenic lines (Student’s t-test, ∗P < 0.05; ∗∗P < 0.01).
TaSnRK2.9 Protein Is Localized Throughout the Cell
To investigate the subcellular localization of TaSnRK2.9, a recombinant vector 35S::TaSnRK2.9::GFP was constructed. Then the recombinant plasmid and the control vector (pCAMBIA1304-GFP) were transformed into onion epidermal cells by a gene-gun method. The green fluorescence signal from the 35S::TaSnRK2.9::GFP was observed in the whole cell, just the same as the control vector 35S::GFP (Figure 2). The results showed that TaSnRK2.9 protein was localized throughout the cell.
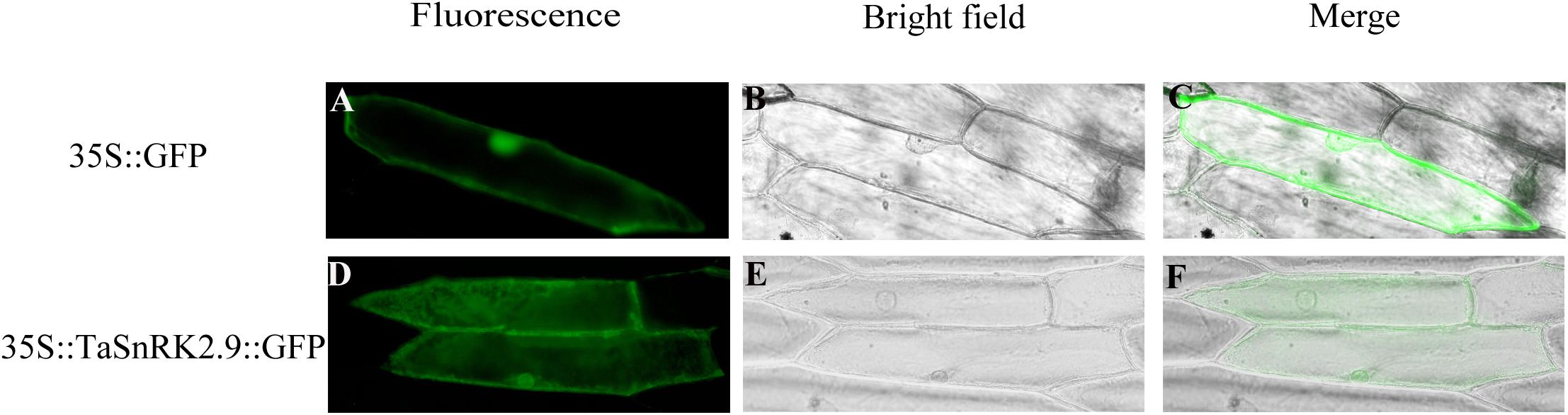
Figure 2. Subcellular localization of TaSnRK2.9 protein. The fusion protein TaSnRK2.9-GFP (pCAMBIA1304-TaSnRK2.9) and GFP (pCAMBIA1304-GFP) were transiently expressed in the onion epidermis cell by the gene-gun method. (A–C) Show the onion epidermis cell expressing pCAMBIA1304-GFP as the controls, (D–F) show the onion epidermis cell expressing pCAMBIA1304-TaSnRK2.9-GFP fusion protein. Pictures were taken in bright field and fluorescence field by fluorescence microscopy 24 h after bombardment. Three independent biological experiments were performed and produced similar results.
Overexpression of TaSnRK2.9 Enhances Drought and Salt Tolerance in Mature Transgenic Tobacco Plants
Since the expression of TaSnRK2.9 is responsive to PEG and NaCl treatments, whether TaSnRK2.9 participates in plant response to osmotic stress was examined. Transgenic tobacco plants overexpressing TaSnRK2.9 under the control of CaMV 35S promoter were generated, and the plants transformed by empty vector severed as vacant (VC). Among these lines, three T3 lines, OE2, OE3, and OE5 with higher TaSnRK2.9 transcript levels were selected for the further investigation.
Seeds germinated on MS medium containing 100 mg L-1 of kanamycin were transplanted in soil for 2 weeks under normal condition. Then the plants were subjected to drought or salt stress. After withholding water for 3 weeks, the control lines (WT, VC) almost died, the leaves were yellow and shriveled, some were wilted, while the transgenic lines (OE2, OE3, OE5) were still green and grew vigorously with only a few leaves dehydrated (Figure 3B). After re-watering for 1 week, more than 60% of the OE plants survived compared with only 18% of the control plants (Figures 3C,F). For the salt stress, plants were treated with NaCl solution for 4 weeks. After the treatment, transgenic plants grew better than the controls, while leaf wilting was more evident in the control plants (Figures 3D,E). Meanwhile, the survival rates of the TaSnRK2.9 overexpression tobacco plants (50%) were greatly higher than the control lines (20%) under salt stress (Figure 3F). These results showed that TaSnRK2.9 improved the drought and salt tolerance in mature transgenic tobacco plants.
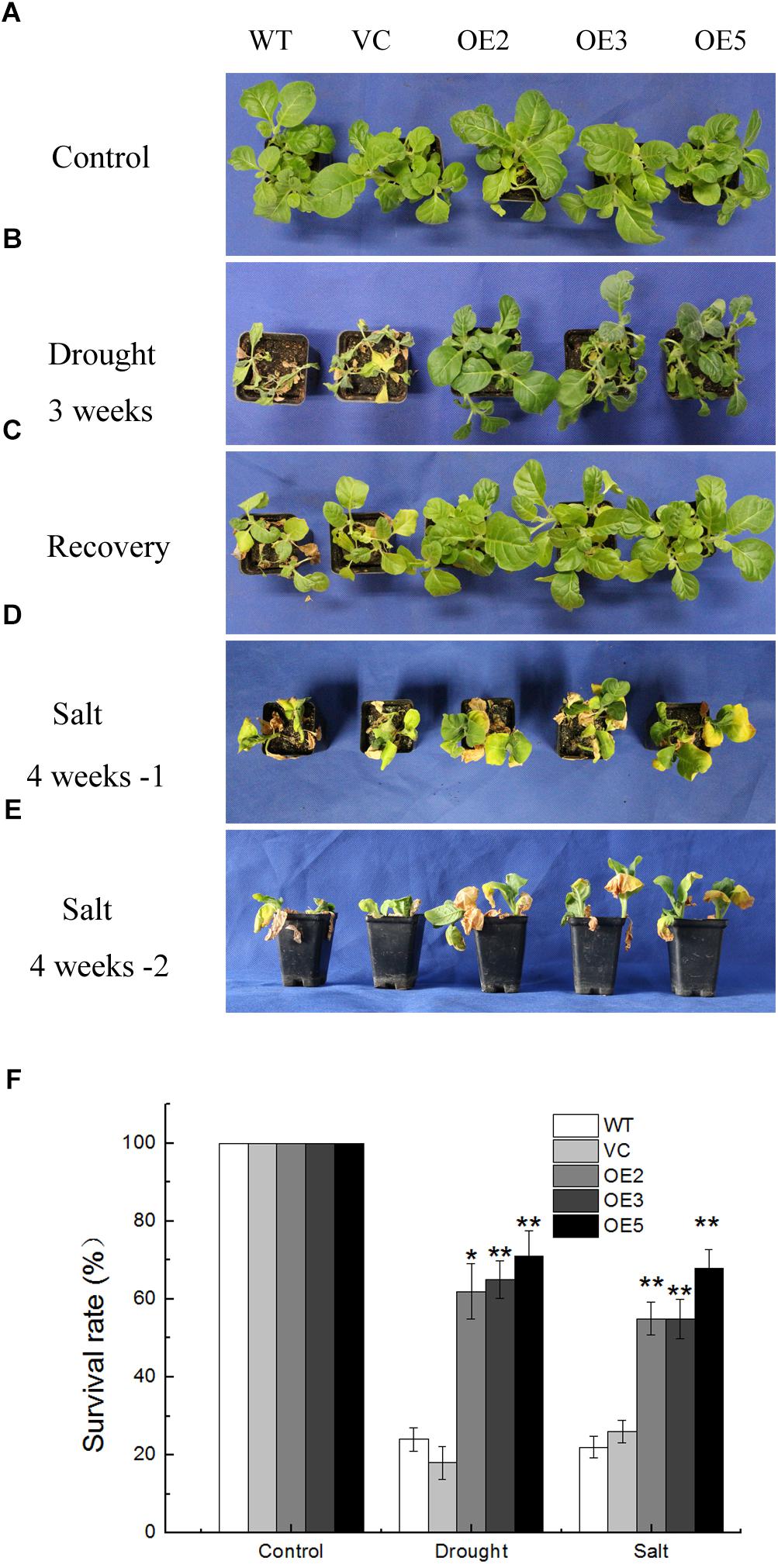
Figure 3. Phenotype and survival rate of the transgenic tobacco plants overexpressing TaSnRK2.9 under drought and salt stresses. (A) Control group of 4-week-old tobacco plants grown in the control environment. (B) Tobacco plants after water withholding for 3 weeks. (C) Tobacco plants in (B) were re-watered for 1 week. (D,E) Tobacco plants were subjected to salt condition for 4 weeks, pictures were taken from different orientations. (F) The survival rate of TaSnRK2.9 overexpressing plants and the control under drought or salt conditions. Data in (F) are means ± SD from three independent replicates (∗P < 0.05; ∗∗P < 0.01 by Student’s t-test).
Overexpression of TaSnRK2.9 Increases the Seeds Germination Rate and Root Length at Seedling Stage in Tobacco Under Mannitol or NaCl Conditions
To investigate the function of TaSnRK2.9 during seed germination and at young seedlings stage, the seeds germination rate and the root elongation were examined. For the germination rate, the seeds were sown on MS medium with 150 mM mannitol, or with 200 mM NaCl. Both control and TaSnRK2.9 overexpression lines grew similar on the MS medium (Figure 4A,a) under normal condition. When seeds were germinated on MS medium with 150 mM mannitol (Figure 4B,b) or with 200 mM NaCl (Figure 4C,c), the transgenic lines had a higher germination rate than the controls. For the root elongation experiment, the germinated seeds continued to grow on the MS medium until two cotyledons were fully appeared, then the seedlings were transferred to MS medium with mannitol or NaCl. The transgenic seedlings showed longer roots than the controls (WT, VC) under treatments (Figures 5B–D). The results suggested that the TaSnRK2.9 also increase the tolerance of the transgenic tobacco during seed germination and at seedlings stage under osmotic and salt stresses.
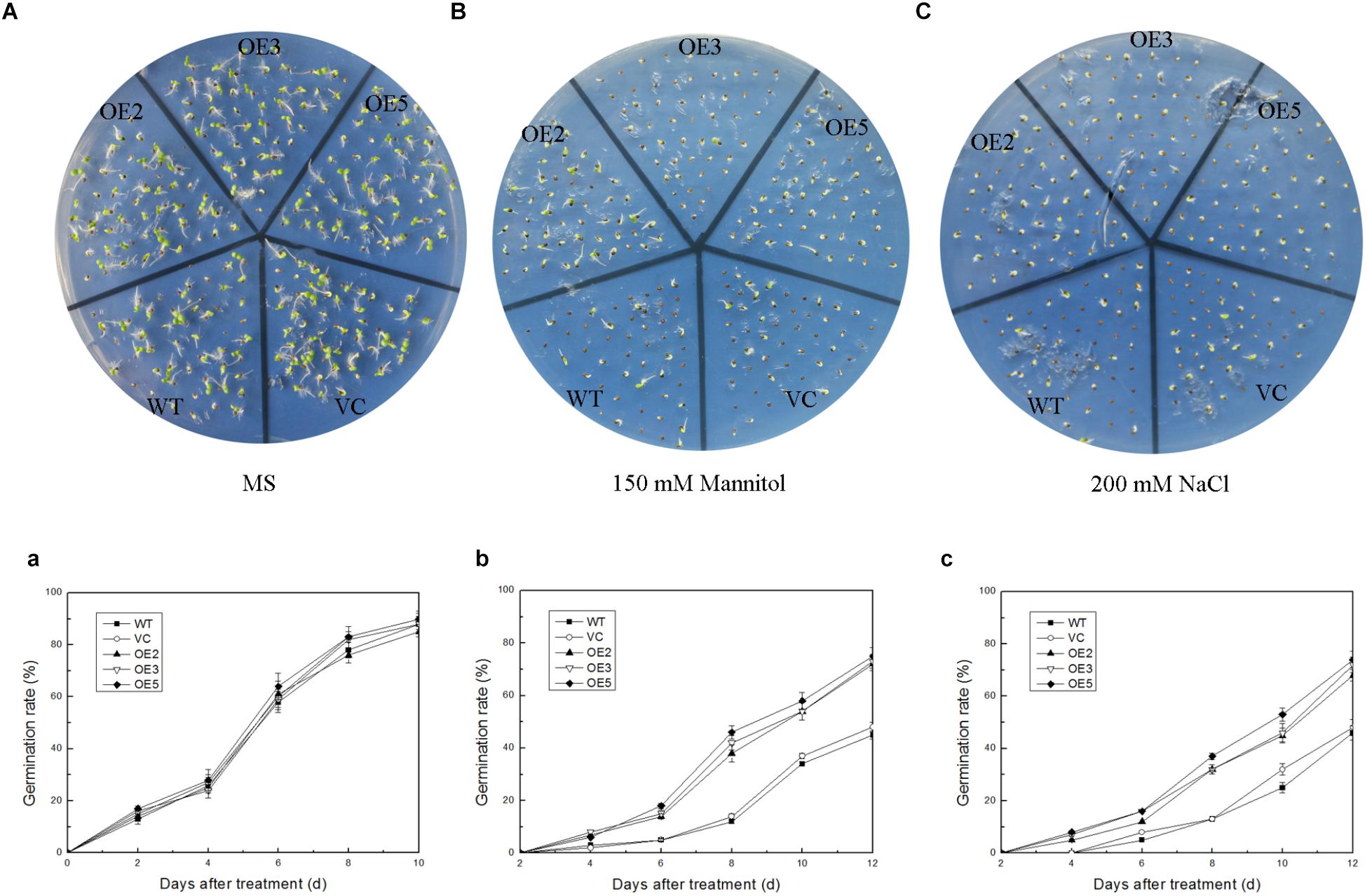
Figure 4. Germination rate of the transgenic tobacco seeds overexpressing TaSnRK2.9 under osmotic stress. TaSnRK2.9 overexpressing tobacco and the control (WT and VC) seeds were germinated on MS medium with no mannitol and NaCl (A,a), 150 mM mannitol (B,b), and 200 mM NaCl (C,c) and photographs were taken after 2 weeks. Plates (A–C) are the photographs of germination status on medium, chart (a–c) are calculated data of germination rate. Values are means ± SD (n = 3).
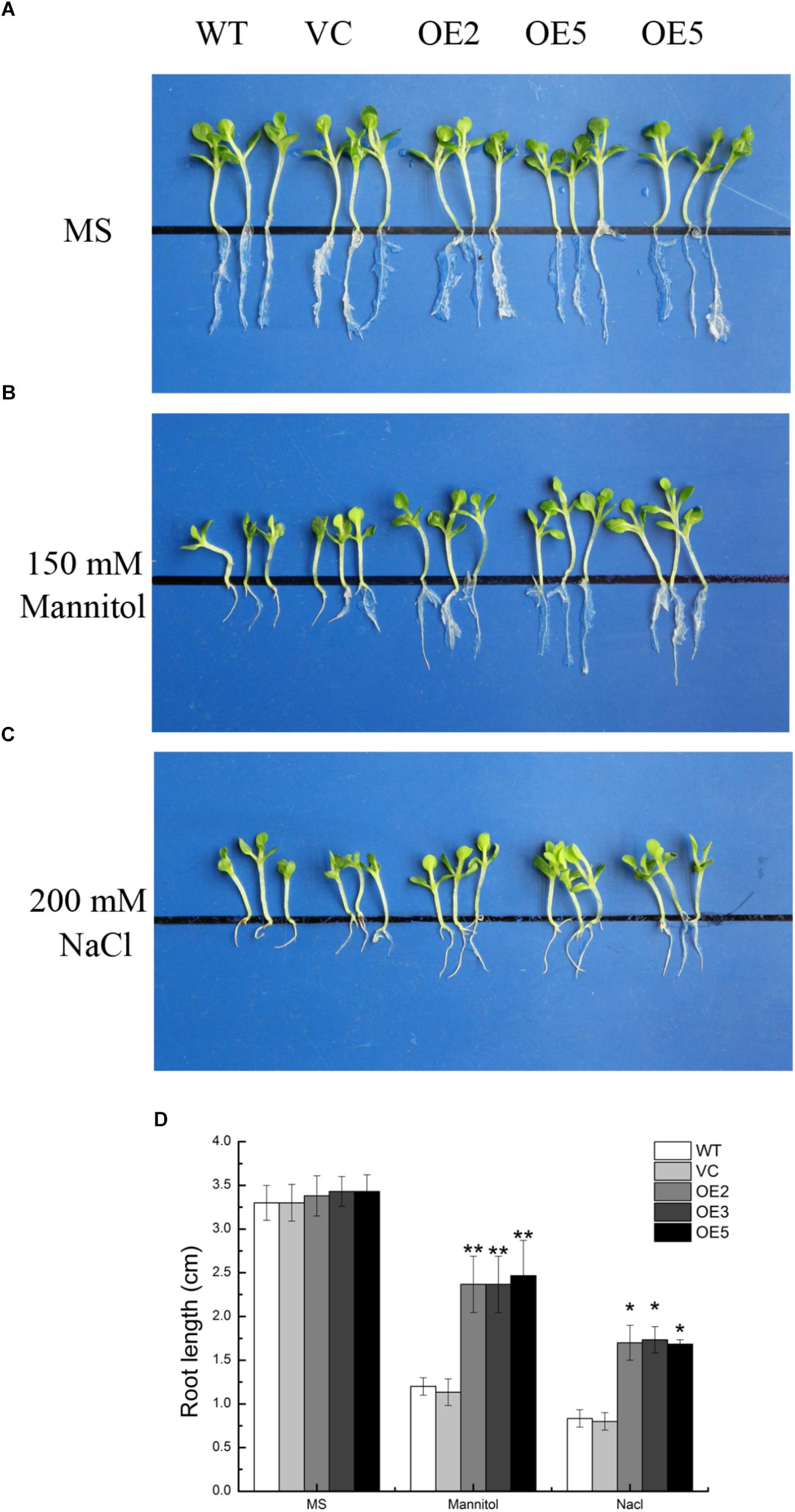
Figure 5. Root elongation of the transgenic tobacco seedlings overexpressing TaSnRK2.9 under osmotic stress. TaSnRK2.9 overexpressing tobacco and the control (WT and VC) seedlings were grown on (A) MS medium, (B) MS medium with 150 mM mannitol, (C) 200 mM NaCl for 2 weeks. (D) The root lengths were gauged and analyzed. Values are means ± SD (n = 3, ∗P < 0.05; ∗∗P < 0.01 by Student’s t-test).
Physiological Indices Comparison Between Transgenic and Control Plants Under Drought or Salt Conditions
That the overexpression of TaSnRK2.9 enhanced drought and salt tolerance in transgenic seeds, seedlings, and mature plants, prompted us to examine the physiological indices influenced by TaSnRK2.9 overexpression. Physiological indices were examined after drought or salt treatment. Abiotic stress such as drought and salt could lead to the accumulation of ROS, which results in plants oxidative damage, and ROS scavenging is essential in plant response to abiotic stress (Giraud et al., 2008; Hossain and Dietz, 2016).
For the drought treatment, the transgenic lines showed lower H2O2 content than the controls, and the activity of ROS-scavenging enzymes such as SOD, CAT, POD in the transgenic lines were higher (Figures 6A–D). Transgenic lines exhibited lower IL and more RWC than the WT and VC (Figures 6E,F), indicating that overexpression of TaSnRK2.9 could keep plants from water lose and retain ions after drought stress. MDA, an indicator of membrane lipid peroxidation, could damage structure and change the permeability of the plasma membrane (Moore and Roberts, 1998). The proline in the plant accumulates under osmotic stress conditions protects plant from adverse environment (Delauney and Verma, 1993). Transgenic lines exhibited lower MDA content than the controls, and the more proline was accumulated in the TaSnRK2.9 overexpression plants (Figures 6G,H). Meanwhile, the soluble sugar content in the transgenic lines was also higher than the controls (Figure 6I). As the expression of TaSnRK2.9 could be upregulated by ABA, the ABA content was also examined. The ABA content was increased in the transgenic lines after drought treatment (Figure 6J), which indicates that TaSnRK2.9 may participate in the ABA signal pathway. These results suggested that TaSnRK2.9 overexpressing plants could enhance the ability of ROS-scavenging, maintain structural integrity of cell membrane, and produce more osmotic substance to meliorate damage imposed by drought stress.
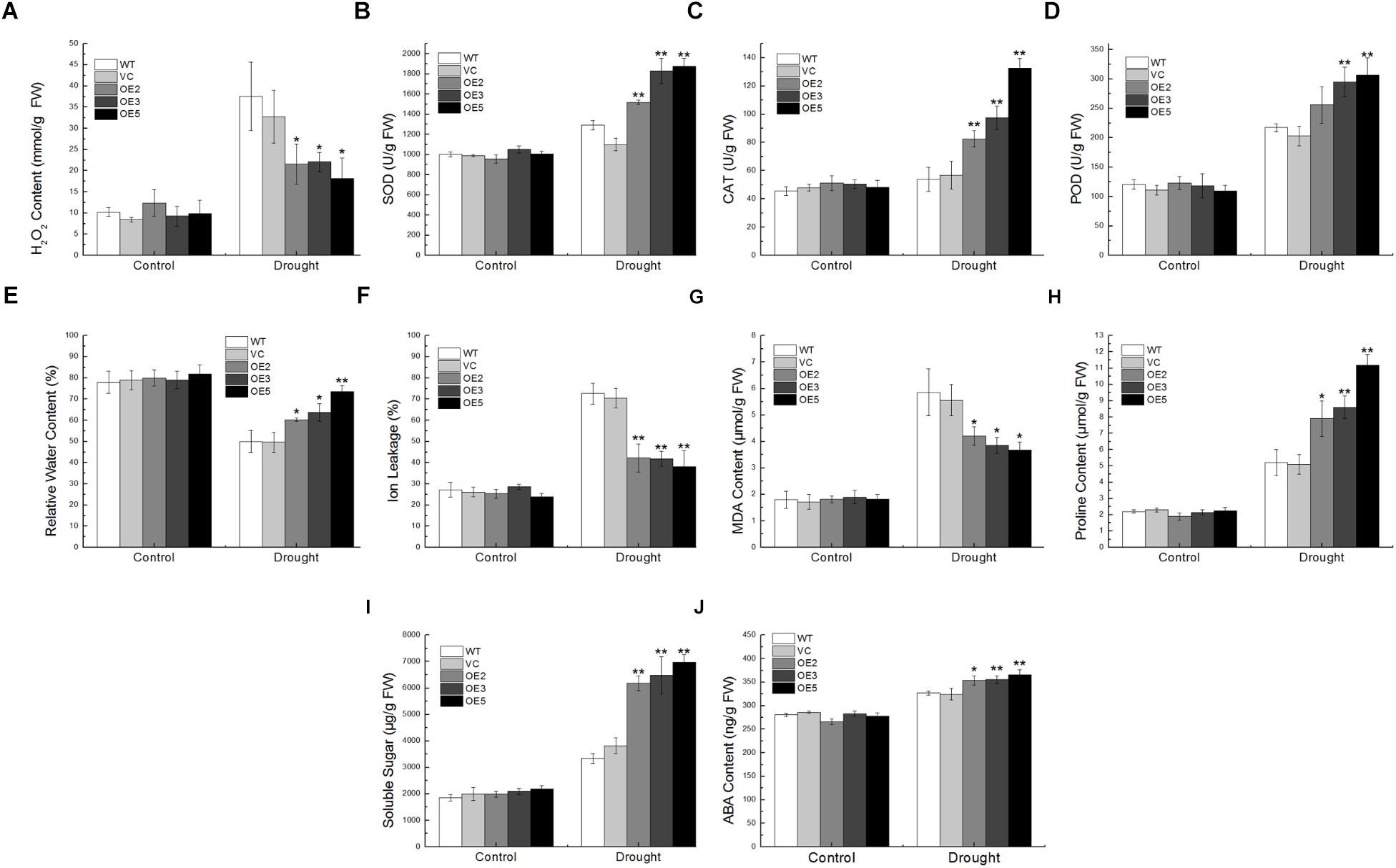
Figure 6. Physiological indices of the transgenic tobacco plants overexpressing TaSnRK2.9 under drought stress. Two-week-old tobacco plants grown on MS medium were transplanted in soil for 3 weeks. Plants were subjected to drought treatments while the controls were normally watered for 1 week. Leaves of the five lines were sampled for the assessment of (A) H2O2, (B) SOD, (C) CAT, (D) POD, (E) RWC, (F) IL, (G) MDA, (H) Proline, (I) Soluble sugar, (J) ABA. Data are means ± SD (n = 3, ∗P < 0.05; ∗∗P < 0.01 by Student’s t-test).
Under the salt treatment, the SOD, CAT, POD activities, and GSH content in the transgenic plants were remarkably higher than in the control plants, but the H2O2 content was lower, implying that TaSnRK2.9 could enhance the enzyme activities and antioxidants content to eliminate more ROS (Figures 7A–E). While the IL and MDA contents showed a lower level in the transgenic plants (Figures 7F,G), but the proline, soluble sugar, and the chlorophyll content were significantly higher than the controls (Figures 7H–J). These results indicate that TaSnRK2.9 could help plants produce more osmotic substances to regulate osmosis, to stabilize biopolymers’ structure, and to reduce the cell toxicity, which finally protect plants from seriously salt damage.
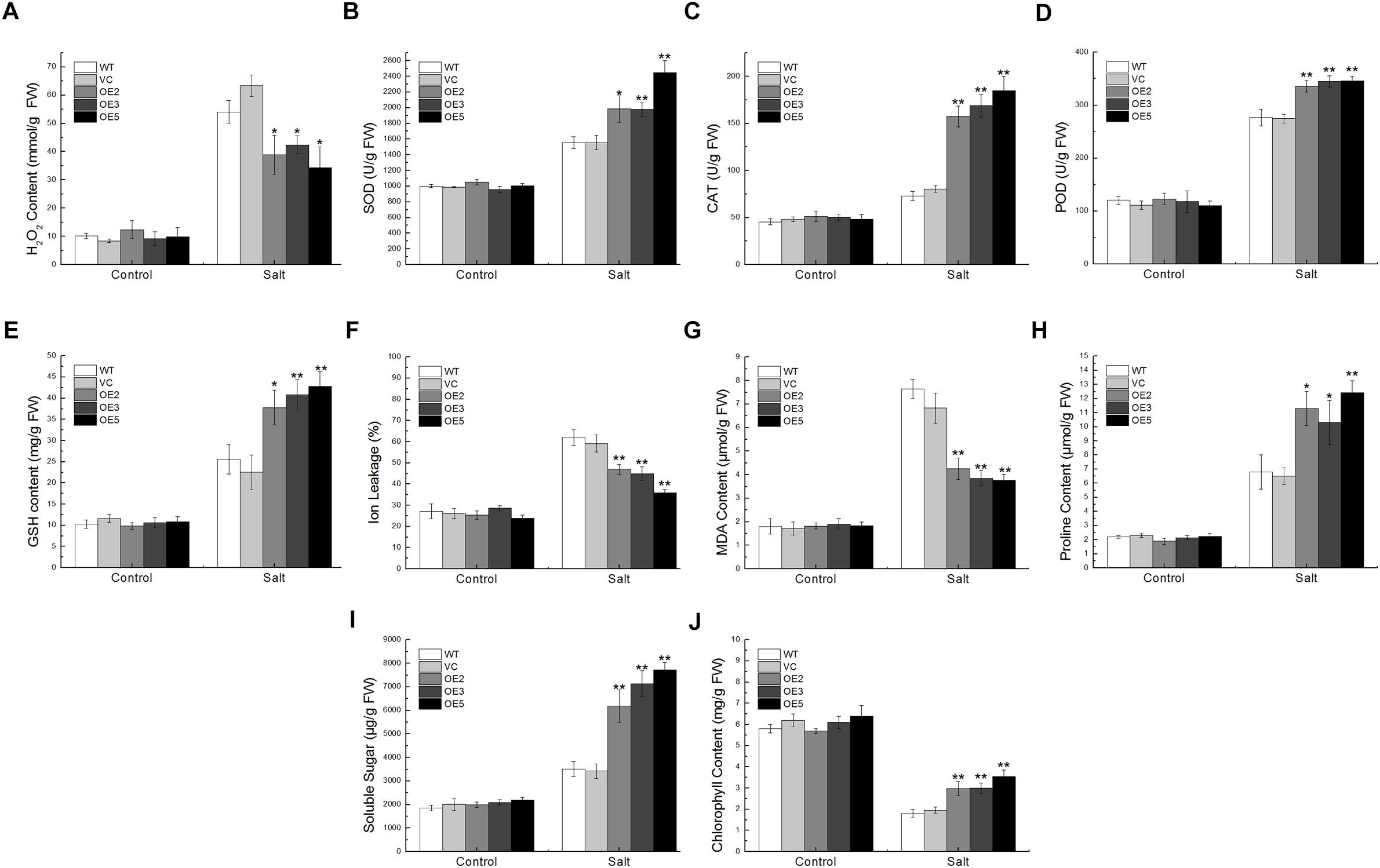
Figure 7. Physiological indices of the transgenic tobacco plants overexpressing TaSnRK2.9 under salt stress. Two-week-old tobacco plants grown on MS medium were transplanted in soil for 3 weeks, plants were watered with 400 mM NaCl solution for 2 weeks. Leaves of the five lines were sampled for the assessment of (A) H2O2, (B) SOD, (C) CAT, (D) POD, (E) GSH, (F) IL, (G) MDA, (H) Proline, (I) Soluble sugar, (J) Chlorophyll. Data are means ± SD (n = 3, ∗P < 0.05; ∗∗P < 0.01 by Student’s t-test).
TaSnRK2.9 Regulates ROS- and ABA-Related Gene Expressions Under Osmotic or NaCl Treatments
To further investigate the effects of TaSnRK2.9 in transgenic plants, we examined the expression levels of some ROS- and ABA-related genes in young seedlings with or without stress treatments. ROS-related genes including NtSOD, NtCAT, NtPOX2, NtAPX, and NtGSHI showed higher levels of expression after mannitol or NaCl treatment in the transgenic plants (Figures 8A–I). Expression of NtNCED1, which plays a vital role in ABA biosynthesis, NtRD29A, a downstream response gene in the ABA signal pathway, were also increased under mannitol stress (Figures 8J,K). These results demonstrate that TaSnRK2.9 confers tobacco plants abiotic stress tolerance through ROS detoxification, or take part in the ABA signal pathway.
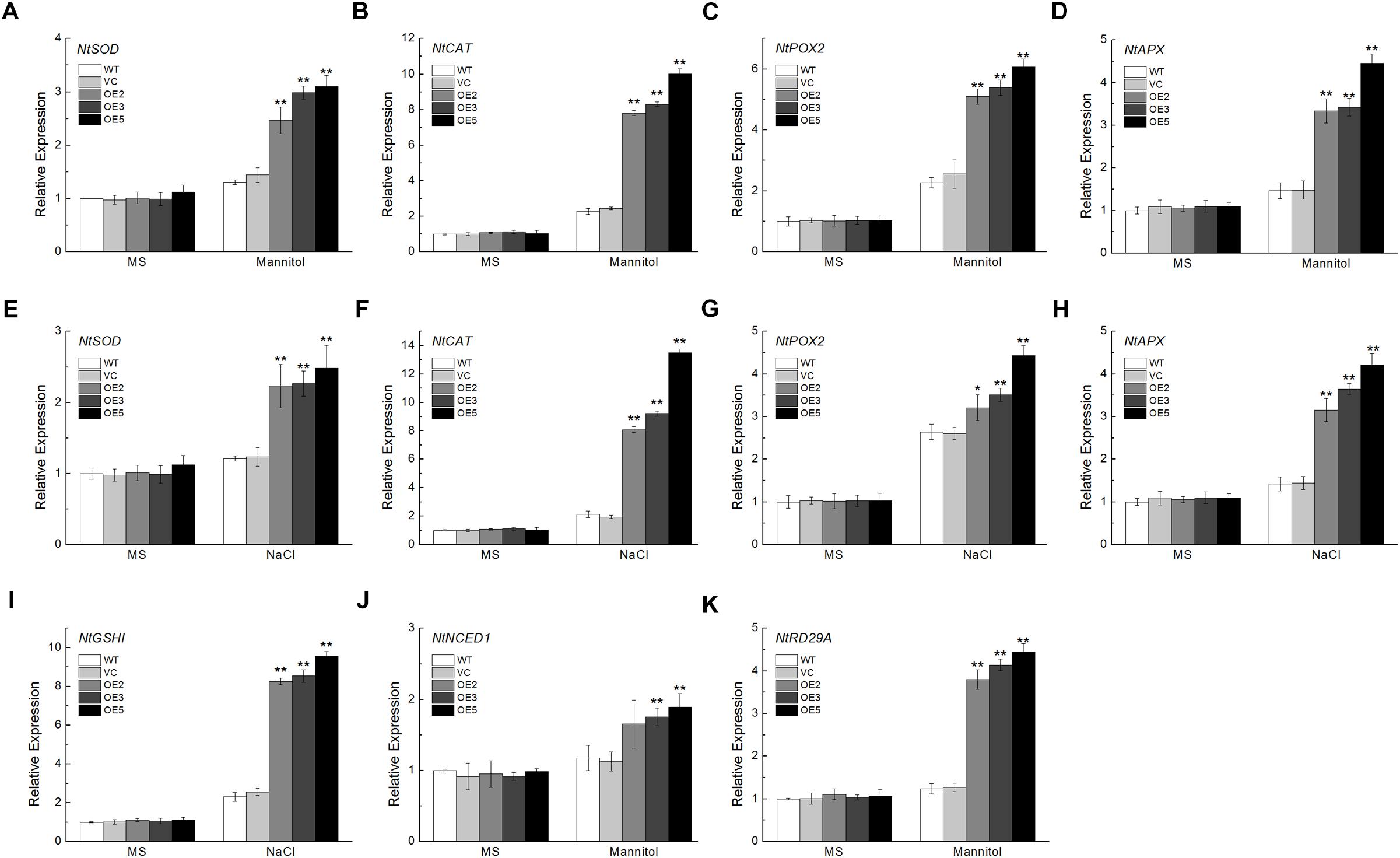
Figure 8. Expression analyses of the ROS-related and ABA-related genes in transgenic tobacco plants overexpressing TaSnRK2.9 under mannitol or NaCl stress by qRT-PCR. Two-week-old seedlings grown in the MS medium were transplanted to MS medium with 300 mM mannitol or 200 mM NaCl for 1 week. Whole seedlings were sampled for RNA extraction. Expression levels of (A,E) NtSOD, (B,F) NtCAT, (C,G) NtPOX2, (D,H) NtAPX, (I) NtGSHI, (J) NtNCED1, (K) NtRD29A. Data are means ± SD (n = 3, ∗P < 0.05; ∗∗P < 0.01 by Student’s t-test).
TaSnRK2.9 Regulates Stress-Responsive Gene Expressions Under Osmotic or NaCl Treatments
To get a deeper understanding of the molecular mechanism underlying the improved osmotic or NaCl tolerance in TaSnRK2.9 overexpression plants, the expression levels of eight stress-responsive genes were detected. These genes include NtERD10C, NtERD10D, and NtLEA5 which belong to LEA protein family (Figures 9A–C,a–c), NtLTP1, a lipid transfer protein (Figure 9D,d), NtSPSA, a sucrose-phosphate synthase (Figure 9E,e), NtADC1, a arginine decarboxylase (Figure 9F,f), NtSAMDC, a S-adenosyl-L-methionine decarboxylase (Figure 9G,g), NtP5CS1, a pyrroline-5-carhoxylate synthase (Figure 9H,h). These selected genes exhibited higher expression levels under osmotic or NaCl treatment than the controls, indicating that TaSnRK2.9 may regulate some stress-response genes to resist drought or salt stress, thus protecting plants from damage.
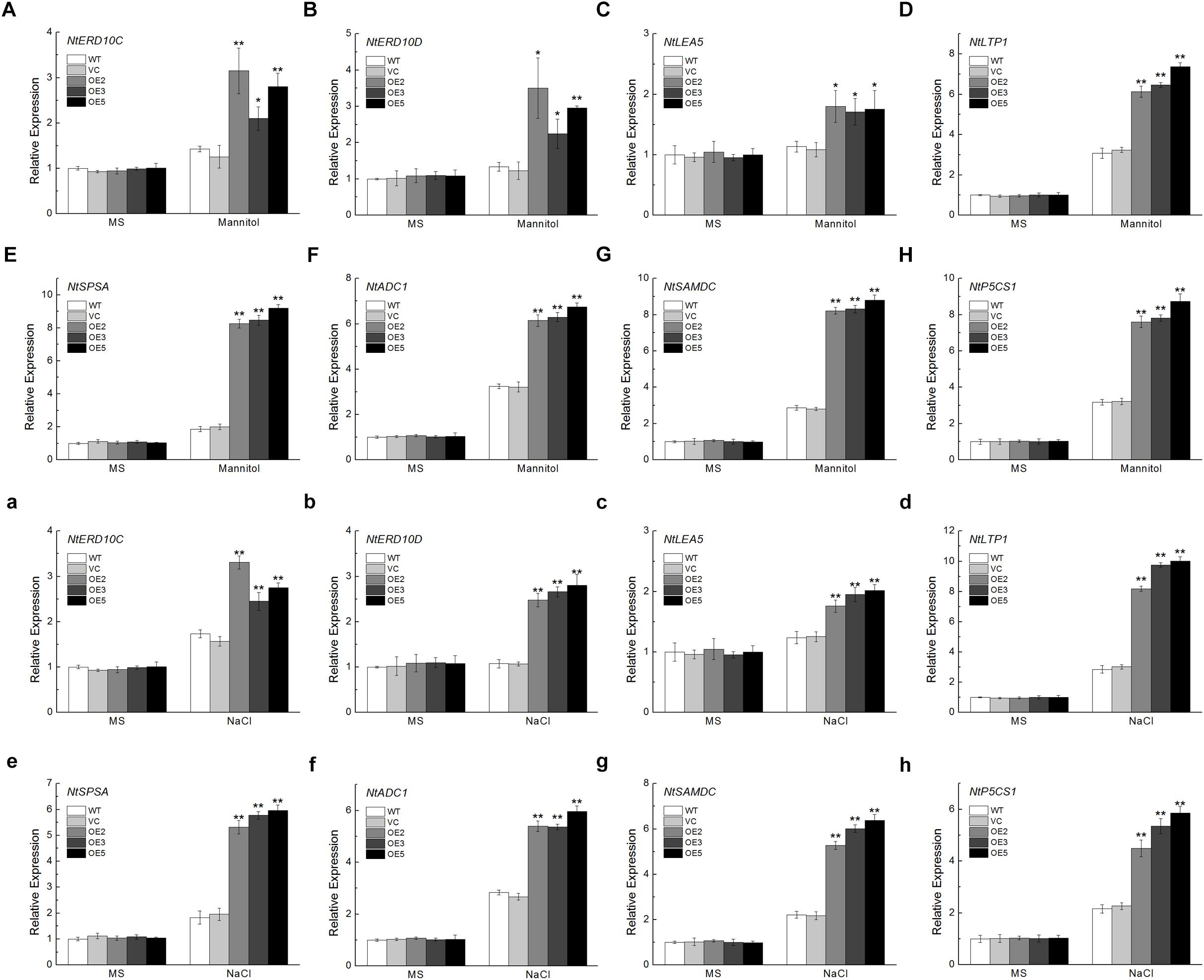
Figure 9. Expression analyses of the stress-responsive genes in transgenic tobacco plants overexpressing TaSnRK2.9 under normal environment and osmotic stresses. Expression levels of (A,a) NtERD10C, (B,b) NtERD10D, (C,c) NtLEA5, (D,d) NtLTP1, (E,e) NtSPSA, (F,f) NtADC1, (G,g) NtSAMDC, (H,h) NtP5CS1. Data are means ± SD (n = 3, ∗P < 0.05; ∗∗P < 0.01 by Student’s t-test).
TaSnRK2.9 Interacts With NtABF2 and Upregulates the Expression of ABF-Regulated Gene in Transgenic Tobacco Plants
To investigate the protein interaction of the TaSnRK2.9 and get a further understanding of the molecular mechanisms in the transgenic plants, yeast two-hybrid assay was used. As shown in Figure 10A, TaSnRK2.9 interacted with NtABF2 in the SD/-Trp-Leu-His-Ade-X-α-gal medium, while TaSnRK2.9 did not interact with NtABF1 or NtABF3. BiFC assay result confirmed this interaction (Supplementary Figure S2). Bright fluorescence was seen through the cell including cytoplasm and nucleus, indicating that TaSnRK 2.9 interacters with NtABF in the tobacco cell. We also tested the expression levels of NtABF2 and NtMYB102 (an ABF-regulated gene) under the mannitol or NaCl treatment. Results showed that the expressions of two genes were greatly increased in the TaSnRK2.9 overexpression plants than the controls (Figures 10B–E). These results suggested that TaSnRK2.9 conferred transgenic tobacco plants tolerance to mannitol or NaCl through interacting with NtABF2 and modulate the ABF-regulated gene expression.
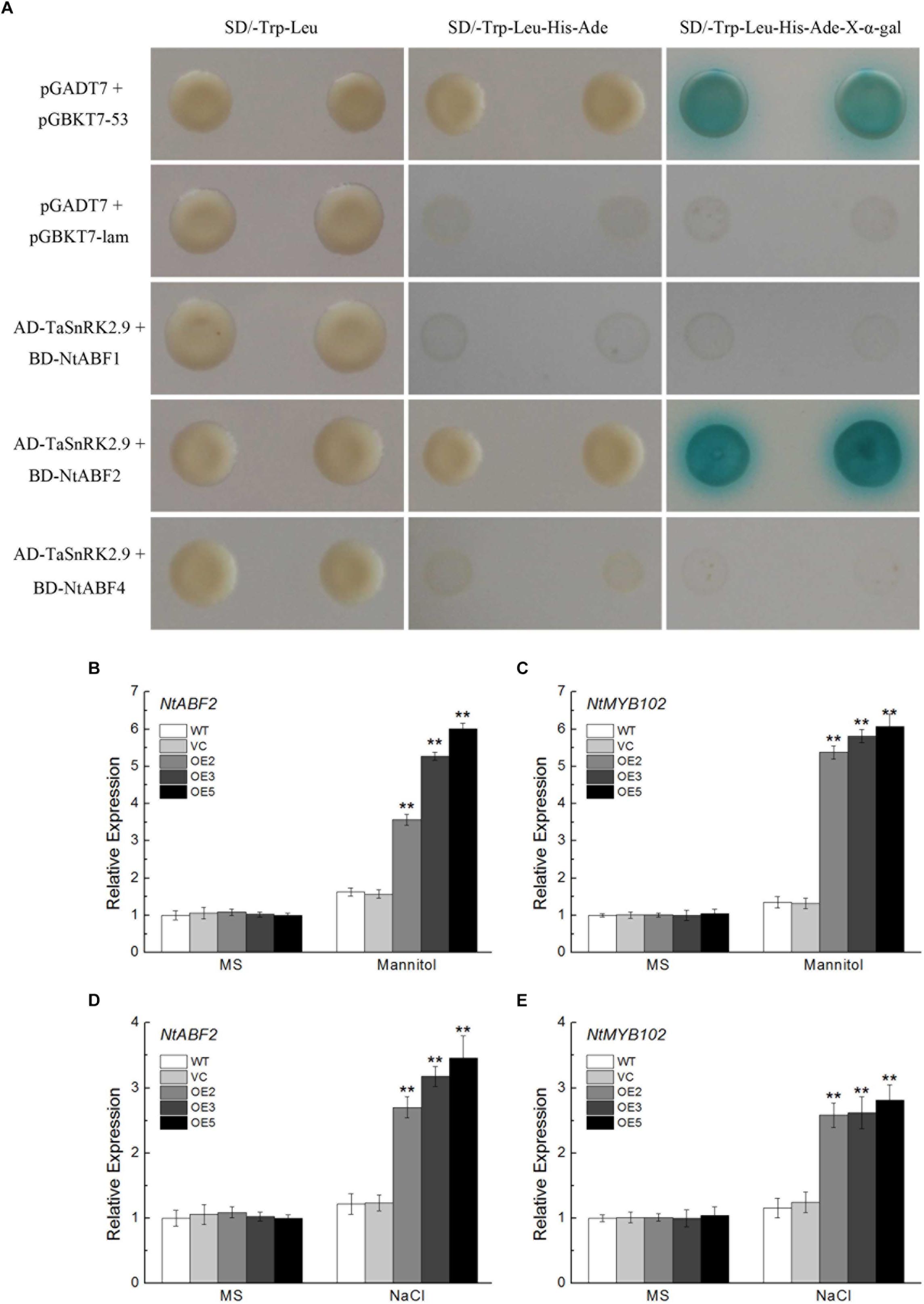
Figure 10. Protein interaction analyses between TaSnRK2.9 and NtABF2 by yeast two-hybrid assay and expression analyses of the related genes. (A) TaSnRK2.9 was cloned into the vector pGADT7, and three NtABFs were transferred to vector pGBKT7, then they were co-transformed into the yeast strain AH109. The transformants were grown on nutritional selective medium SD/-Trp-Leu, or SD/-Trp-Leu-His-Ade, or plus the chromogenic substrate X-α-Gal. The first and second lines indicate the positive and negative control, respectively. Three independent biological experiments were performed and produced similar results. (B,D) The relative expression level of the predicated interacting gene NtABF2 under drought or salt stress in tobacco. (C,E) The relative expression level of the related gene NtMYB102. Data in chart are means ± SD (n = 3, ∗P < 0.05; ∗∗P < 0.01 by Student’s t-test).
Discussion
As sessile organisms, plants are exposed to ever changing environments which are harmful to plants growth and development (Zhu, 2016; Vishwakarma et al., 2017). Abiotic stresses such as drought, high salinity, and temperature stresses severely affect cereal crops, and cause the reduction of productivity. The SnRK family members play an indispensable role in plants’ response to stresses. Previous studies of SnRKs mainly focused on ABA-dependent pathway in response to drought and salt stresses (Mao et al., 2010; Zhang et al., 2010, 2011, 2016; Tian et al., 2013). Although some SnRK genes in wheat have been identified and studied, the function of TaSnRK2.9 remains unclear.
In this study, we cloned and characterized a SnRK2 gene from common wheat, sequences analysis showed that it has a high similarity with OsSAPK9, thus designated TaSnRK2.9.
TaSnRK2.9 Is Responsive to Multiple Stresses and Signal Molecules
Numerous researches have shown that SnRKs are involved in multiple abiotic and biotic stresses. TaSnRKs mainly responded to PEG, NaCl, Cold, and ABA (Mao et al., 2010; Zhang et al., 2010, 2011; Tian et al., 2013). In our work, PEG, NaCl, and H2O2 rapidly increased the expression of TaSnRK2.9 (Figures 1A–C), which indicates that TaSnRK2.9 is sensitive to osmotic stress and reactive oxygen. The signal molecules ABA, MeJA, and ethrel also upregulated the TaSnRK2.9 expression (Figures 1D–F), suggesting that TaSnRK2.9 may participate in the related signaling pathway.
ABA is an important signal molecule involved in plant abiotic stress. When subjected to abiotic stress, plants produce more ABA, leading to ABA-dependent response (Zhao et al., 2018). Abiotic stress also causes ABA-independent response through SnRK2s (Fujii et al., 2011). In Arabidopsis, ten SnRK2s family members function redundantly in plant resisting abiotic stress (Fujii et al., 2011). SnRK2.2, SnRK2.3, SnRK2.6, SnRK2.7, and SnRK2.8 are ABA-dependent members (Furihata et al., 2006). MeJA modulates plant growth and defenses against to abiotic stress (Wasternack and Song, 2017). Therefore, understanding how TaSnRK2.9 functions in plant abiotic stress response is important.
TaSnRK2.9 Expresses in All Organs in Wheat and the TaSnRK2.9 Protein Locates Throughout the Cell
Unlike other TaSnRKs which mainly expressed in root, organ specific expression analysis shows that TaSnRK2.9 is mainly expressed in young root, stamen, pistil, and lemma (Figure 1G), indicating that TaSnRK2.9 may play a key role in plant growth and development. Consistent with other TaSnRK2s, TaSnRK2.9 also locates in the whole cell (Figure 2).
TaSnRK2.9 Enhances Transgenic Tobacco Plants to Drought and Salt Tolerance in Mature Plants and Seedlings
To further investigate the function of TaSnRK2.9 under abiotic stress, we generated transgenic tobacco plants overexpressing TaSnRK2.9 under the control of CaMV 35S promoter. Drought or salt treatment was applied to the young seedlings, mature transgenic plants as well as at seed germination stage. Results showed that TaSnRK2.9 overexpression tobacco plants exhibited enhanced tolerance to drought or salt treatment in comparison with WT and VC (Figure 3). Similar results were also found during seed germination and at young seedling stage. Previous studies showed the TaSnRK2.4 transgenic Arabidopsis had a delayed seed geimination (Mao et al., 2010). Our research indicated that seeds of TaSnRK2.9 overexpression tobacco germinated earlier and better than the controls on MS medium with mannitol or NaCl, the final germination rate was higher than the controls (Figure 4), suggesting that TaSnRK2.9 participates in regulating seed dormancy. Overexpression of TaSnRK2.9 also affected the root length in young tobacco seedlings. Transgenic plants showed longer roots contrast to WT and VC under mannitol or NaCl treatment (Figure 5). This implies that TaSnRK2.9 may help the roots grow longer to get more water and nutrients to tolerate osmotic stress. TaSnRK2.3, TaSnRK2.4, TaSnRK2.7, and TaSnRK2.8 had the similar effect on the primary root length(Mao et al., 2010; Zhang et al., 2010, 2011; Tian et al., 2013), indicating the SnRK2s have a typical characteristic to promote root elongation.
The Antioxidant Mechanism and ABA Signal Pathway Are Involved in TaSnRK2.9 Conferring Osmotic Stress Tolerance
The changes in physiological indices level always indicate the plants’ ability to cope with complex environments. Based on the plants’ phenotype results, physiological indices with or without drought and salt stresses were examined (Figures 6, 7). Abiotic stresses often cause the ROS accumulation, antioxidant enzymes such as SOD, CAT, POD and non-enzymatic antioxidants GSH play crucial roles in ROS scavenging. Plants have evolved a complex system to clear the excessive ROS (Miller et al., 2010). SOD is the first barrier to scavenge ROS by catalyzing the dismutation of O-2 to oxygen and H2O2 with the assistant of CAT and POD (Blokhina et al., 2003). GSH can eliminate free radicals to protect plants from ROS damage and it is a non-enzymatic antioxidant (Wang et al., 2016). Under drought stress, increased RWC indicates that TaSnRK2.9 overexpression improves plants’ ability to sustain water, helping the cell remain a proper volume and keep a balance to the transpiration rate. Lower IL implies that the cell membrane suffered less damage. MDA, a product of oxidative attack on membrane lipids, is an index of membrane injury, which could change membrane permeability. Proline not only regulates cell osmotic pressure but also modulates cell oxidation-reduction potential, thus safeguards plants from injuring. The chlorophyll content, an important factor of increased biomass and grain yield, increased under salt stress (Figure 7J), indicating the transgenic plants showed higher photosynthetic capacities compared to controls. ABA showed a higher content in the TaSnRK2.9 overexpression plants under drought stress, suggesting that the accumulated ABA affect the TaSnRK2.9 response to osmotic stress (Figure 6J).
In order to get further insights into how TaSnRK2.9 responds to drought or salt stress at the molecular level, the expressions of five ROS-related and two ABA-related genes were measured (Figure 8). The ROS-scavenging related genes NtSOD, NtCAT, NtPOX2, and NtAPX exhibited enhanced expression under mannitol or NaCl condition. The NtGSHI was upregulated after NaCl treatment. The expressions of NtNCED1 and NtRD29A, ABA-related genes, were greatly increased under mannitol treatment.
Reactive oxygen species accumulation induced by abiotic stress could damage the plants, so ROS scavenging becomes much more important to maintain the ROS balance (Mittler et al., 2004). Since each subcellular compartment has its own set of ROS production and ROS cleaning pathway, the steady-state level of ROS is not the same at the same time. ROS also acts as signal transduction molecules to modulate diverse pathways when accumulated under stresses (Choudhury et al., 2017). Plants defend abiotic stress via a temporal-spatial harmonizing between ROS and other signal molecules such as biological macromolecules, plant hormones (Baxter et al., 2014). When subjected to abiotic stress, the steady-state of the ROS was disturbed, so the plants have evolved a complex mechanism to keep the ROS balance. Overexpression of TaSnRK2.9 in tobacco plants could scavenge ROS via antioxidant enzymes (SOD, CAT, POD) and non-enzymatic antioxidant (GSH), thus sustaining ROS to a normal level to defend abiotic stress.
Abscisic acid signal pathway is widely studied, and plants defense against abiotic stress via ABA-dependent or ABA-independent pathway. SnRK2.2, SnRK2.3, and SnRK2.6 regulate the plant tolerance to abiotic stress through ABA-dependent way, but whether TaSnRK2.9 works in the same way remains unknown. In our present research, TaSnRK2.9 can be induced by ABA, overexpression of TaSnRK2.9 caused ABA accumulation, and elevated the expressions of ABA-related genes. These results indicate TaSnRK2.9 functions through ABA-dependent pathway when subjected to abiotic stress.
TaSnRK2.9 Regulates the Expressions of Some Stress-Response Genes in Transgenic Tobacco
To better understand the function of TaSnRK2.9 against abiotic stress, we examined the expressions of some stress-response genes. Eight such genes examined showed significantly higher expression than the controls under stress conditions. NtERD10C, NtERD10D, and NtLEA5, belonging to LEA family, could protect the structure of cell membranes and stabilize the biomacromolecules (Xiong and Zhu, 2002; Amara et al., 2012; Liu et al., 2013). The LEA protein family comprises a lot of multifunction proteins which is considered necessary in plants response to abiotic stress (Mertens et al., 2018). NtLTP1 encodes a lip transfer protein, which is involved in plant defending abiotic stress (Hu et al., 2013). As the cell membranes are mainly made up of lipid bilayer, the improved NtLTP1 expression indicates that plants produces more lip transfer protein, thus protect the cell membranes from severely damage. NtSPSA plays an important role in the biosynthesis of sucrose (Lin et al., 2008). The increased sucrose could provide more nutrients to plants when subjected to abiotic stress and supported plants growth and development (Chen et al., 2005) and could maintain osomatic balance. NtADC1 and NtSAMDC participate in the biosynthesis of polyamine, which could regulate cell osmotic pressure and stabilize the membrane structure (Jang et al., 2009). NtP5CS1 is a key regulator in proline biosynthesis (Dorothea and Ramanjulu, 2005). The expressions of stress-response genes were increased under mannitol or NaCl treatment in the TaSnRK2.9 overexpression plants, while the plants grown in the MS medium showed no difference (Figure 9). TaSnRK2.9 transgenic plants exhibited enhanced stress tolerance and produced more stress-response proteins when exposed to abiotic stress, which suggests that these proteins and sugar play essential roles in mediating plants against abiotic stress. In a word, TaSnRK2.9 regulates the expression of multiple stress-response genes and guarantees plants better adaption to the stress environment.
TaSnRK2.9 Is Involved in SnRK-ABF Pathway to Enhance Tolerance to Drought and Salt Stresses in Transgenic Tobacco Plants
To investigate the mechanism of TaSnRK2.9 action, we also conducted yeast two-hybrid assay. In the previous studies, interactions between AtSnRKs (SnRK2.2, SnRK2.3, SnRK2.6) and AtAREB1/ABF2 were identified, meanwhile many AtABFs and AtSnRK2s can be simultaneously regulated both by ABA and abiotic stresses (Kobayashi et al., 2010; Chan, 2012). Accumulated ABA modulates the expression of many genes controlled by ABA-responsive elements (ABREs) when subjected to abiotic stress. ABRE-binding protein/ABRE-binding factor (AREB/ABF) plays a key role in pants response to tolerance via regulating a series of gene expressions (Fujita et al., 2013). The SnRKs (SnRK2.2, SnRK2.3, SnRK2.6) protein could actively regulate the AREB/ABF transcription factors (Furihata et al., 2006; Fujii et al., 2007; Fujii and Zhu, 2009). AREB1, AREB2, and ABF3 are the master transcription factors that cooperatively regulate ABRE-dependent gene expression for ABA signaling under drought stress (Yoshida et al., 2010). So the SnRK-ABF pathway has a great importance in plants resisting abiotic stress (Wang L. et al., 2015). In our research, TaSnRK2.9 could interact with NtABF2, and upregulated the expression of NtABF2 under mannitol or NaCl treatment (Figures 10A,B,D). MYB102, a transcription factor containing a conserved MYB DNA-binding domain, which could be regulated by ABF, is involved in plants stress response (Stracke et al., 2001; Yoshida et al., 2010). The ABF-related gene NtMYB102 showed a great increase after mannitol or NaCl treatment (Figures 10C,E).
In the present study, we found that ABA could induce the expression of TaSnRK2.9 (Figure 1D), suggesting that the function of TaSnRK2.9 is connected with ABA. When plants were subjected to drought stress, TaSnRK2.9 overexpression caused more ABA accumulation (Figure 6J), and induced the expressions of ABA-related genes (Figures 8J,K), while the accumulated ABA caused a lot of downstream gene expressions and other physiological response so that to adjust to the abiotic stress response. These results indicate that TaSnRK2.9 may participate in the plants stress response via an ABA-dependent pathway. Furthermore, our result showed that TaSnRK2.9 interacted with NtABF2, and TaSnRK2.9 overexpression can upregulate the expressions of NtABF2 and ABF-related gene NtMYB102 under mannitol or NaCl (Figure 10). These implies that TaSnRK2.9 specifically interact with NtABF2 in response to ABA and osmotic stress.
Overexpression of TaSnRK2.9 enhanced tobacco plants tolerance to multiple abiotic stresses, thus making TaSnRK2.9 a candidate gene for molecular breeding and it is promising to improve crop plant stress resistance by using genetic engineering.
Conclusion
TaSnRK2.9 was responsive to various abiotic stress and different signaling molecules. Overexpression of TaSnRK2.9 enhanced tobacco plants tolerance to drought or salt with improved antioxidant system, RWC, proline, soluble sugar, chlorophyll, and ABA content, reduced H2O2, IL, and MDA. In summary, TaSnRK2.9 could enhance ROS scavenging ability through ABA-dependent signal transduction and specific SnRK-ABF interaction, resulting in enhanced plants tolerance to abiotic stress in transgenic tobacco plants.
Author Contributions
JF and LW designed all the experiments. JF analyzed the data and wrote the manuscript. LW conducted the research technology. YW carried out the stress tolerance experiments. QL and YZ help with making figures. DQ and JH participated in the bioinformatics analyses. PS, ZX, and JC conducted the PCR. GY and GH conceived the study and revised the manuscript. All the authors read and approved the final manuscript.
Funding
This work was supported by National Genetically Modified New Varieties of Major Projects of China (2016ZX08010004-004), the National Natural Science Foundation of China (Nos. 31771418 and 31570261), and Key Project of Hubei Province (2017AHB041).
Conflict of Interest Statement
The authors declare that the research was conducted in the absence of any commercial or financial relationships that could be construed as a potential conflict of interest.
Supplementary Material
The Supplementary Material for this article can be found online at: https://www.frontiersin.org/articles/10.3389/fpls.2018.02003/full#supplementary-material
Abbreviations
ABA, abscisic acid; ABF, ABA-responsive element-binding transcription factor; AMPK, AMP-activated protein kinase; CaMK, calmodulin-dependent protein kinases; CaMV, cauliflower mosaic virus; CAT, catalase; CCaMK, calcium and calmodulin-dependent protein kinases; CDPK, calcium-dependent protein kinases; CRK, CDPK-related kinases; EST, expressed sequence tag; GFP, green fluorescent protein; GSH, glutathione; IL, ion leakage; LEA, late embryogenesis-abundant; MDA, malondialdehyde; MeJA, methyl jasmonate; MS, Murashige and Skoog; OE, overexpression; ORF, open reading frame; PEG, polyethylene glycol; PEPRK, PEP carboxylase kinase-related kinases; POD, peroxidase; PP2C, type 2C phosphatase; PPCK, phosphoenolpyruvate carboxylase kinases; PYL, PYR-like; PYR, pyrabactin resistance; qRT-PCR, quantitative reverse-transcription PCR; RACE, rapid amplification of cDNA ends; RCAR, regulatory component of ABA receptor; ROS, reactive oxygen species; RWC, relative water content; SNF, sucrose non-fermenting; SnRK, sucrose non-fermenting 1-related protein kinase; SOD, superoxide dismutase; VC, vector control; WT, wild type.
Footnotes
References
Amara, I., Odena, A., Oliveira, E., Moreno, A., Masmoudi, K., Pages, M., et al. (2012). Insights into maize LEA proteins: from proteomics to functional approaches. Plant Cell Physiol. 53, 312–329. doi: 10.1093/pcp/pcr183
Baxter, A., Mittler, R., and Suzuki, N. (2014). ROS as key players in plant stress signalling. J. Exp. Bot. 65, 1229–1240. doi: 10.1093/jxb/ert375
Blokhina, O., Virolainen, E. A., and Fagerstedt, K. V. (2003). Antioxidants, oxidative damage and oxygen deprivation stress: a review. Ann. Bot. London 91, 179–194. doi: 10.1093/aob/mcf118
Chan, Z. (2012). Expression profiling of ABA pathway transcripts indicates crosstalk between abiotic and biotic stress responses in Arabidopsis. Genomics 100, 110–115. doi: 10.1016/j.ygeno.2012.06.004
Chen, S., Hajirezaei, M., and Börnke, F. (2005). Differential expression of sucrose-phosphate synthase isoenzymes in tobacco reflects their functional specialization during dark-governed starch mobilization in source leaves. Plant Physiol. 139, 1163–1174. doi: 10.1104/pp.105.069468
Choudhury, F. K., Rivero, R. M., Blumwald, E., and Mittler, R. (2017). Reactive oxygen species, abiotic stress and stress combination. Plant J. 90, 856–867. doi: 10.1111/tpj.13299
Delauney, A. J., and Verma, D. P. S. (1993). Proline biosynthesis and osmoregulation in plants. Plant J. Cell Mol. Bio. 4, 215–223. doi: 10.1046/j.1365-313X.1993.04020215.x
Dorothea, B., and Ramanjulu, S. (2005). Drought and salt tolerance in plants. Crit. Rev. Plant Sci. 24, 23–58. doi: 10.1080/07352680590910410
Fujii, H., Verslues, P. E., and Zhu, J. K. (2007). Identification of two protein kinases required for abscisic acid regulation of seed germination, root growth, and gene expression in Arabidopsis. Plant Cell 19, 485–494. doi: 10.1105/tpc.106.048538
Fujii, H., Verslues, P. E., and Zhu, J. K. (2011). Arabidopsis decuple mutant reveals the importance of SnRK2 kinases in osmotic stress responses in vivo. Proc. Natl. Acad. Sci. U.S.A. 108, 1717–1722. doi: 10.1073/pnas.1018367108
Fujii, H., and Zhu, J. K. (2009). Arabidopsis mutant deficient in 3 abscisic acid-activated protein kinases reveals critical roles in growth, reproduction, and stress. Proc. Natl. Acad. Sci. U.S.A. 106, 8380–8385. doi: 10.1073/pnas.0903144106
Fujita, Y., Nakashima, K., Yoshida, T., Katagiri, T., Kidokoro, S., Kanamori, N., et al. (2009). Three SnRK2 protein kinases are the main positive regulators of abscisic acid signaling in response to water stress in Arabidopsis. Plant Cell Physiol. 50, 2123–2132. doi: 10.1093/pcp/pcp147
Fujita, Y., Yoshida, T., and Yamaguchi-Shinozaki, K. (2013). Pivotal role of the AREB/ABF-SnRK2 pathway in ABRE-mediated transcription in response to osmotic stress in plants. Physiol. Plant. 147, 15–27. doi: 10.1111/j.1399-3054.2012.01635.x
Furihata, T., Maruyama, K., Fujita, Y., Umezawa, T., Yoshida, R., Shinozaki, K., et al. (2006). Abscisic acid-dependent multisite phosphorylation regulates the activity of a transcription activator AREB1. Proc. Natl. Acad. Sci. U.S.A. 103, 1988–1993. doi: 10.1073/pnas.0505667103
Giraud, E., Ho, L. H. M., Clifton, R., Carroll, A., Estavillo, G., Tan, Y. F., et al. (2008). The absence of ALTERNATIVE OXIDASE1a in Arabidopsis results in acute sensitivity to combined light and drought stress. Plant Physiol. 147, 595–610. doi: 10.1104/pp.107.115121
Hardie, D. G., Carling, D. A., and Carlson, M. (1998). The AMP-activated/SNF1 protein kinase subfamily: metabolic sensors of the eukaryotic cell? Annu. Rev. Biochem. 67, 821–855. doi: 10.1146/annurev.biochem.67.1.821
He, Y., Zhang, Y., Chen, L., Wu, C., Luo, Q., Zhang, F., et al. (2017). A member of the 14-3-3 gene family in Brachypodium distachyon, BdGF14d, confers salt tolerance in transgenic tobacco plants. Front. Plant Sci. 8:340. doi: 10.3389/fpls.2017.00340
Heath, R. L., and Packer, L. (1968). Photoperoxidation in isolated chloroplasts. I. Kinetics and stoichiometry of fatty acid peroxidation. Arch. Biochem. Biophys. 125, 189–198. doi: 10.1016/0003-9861(68)90654-1
Hossain, M. S., and Dietz, K. J. (2016). Tuning of redox regulatory mechanisms, reactive oxygen species and redox homeostasis under salinity stress. Front. Plant Sci. 7:548. doi: 10.3389/fpls.2016.00548
Hrabak, E. M., Chan, C. W., Gribskov, M., Harper, J. F., Choi, J. H., Halford, N., et al. (2003). The Arabidopsis CDPK-SnRK superfamily of protein kinases. Plant Physiol. 132, 666–680. doi: 10.1104/pp.102.011999
Hu, W., Huang, C., Deng, X., Zhou, S., Chen, L., Li, Y., et al. (2013). TaASR1, a transcription factor gene in wheat, confers drought stress tolerance in transgenic tobacco. Plant Cell Environ. 36, 1449–1464. doi: 10.1111/pce.12074
Hu, W., Yuan, Q., Wang, Y., Cai, R., Deng, X., Wang, J., et al. (2012). Overexpression of a wheat aquaporin gene, TaAQP8, enhances salt stress tolerance in transgenic tobacco. Plant Cell Physiol. 53, 2127–2141. doi: 10.1093/pcp/pcs154
Jang, E. K., Min, K. H., Kim, S. H., Nam, S. H., Zhang, S., Kim, Y. C., et al. (2009). Mitogen-activated protein kinase cascade in the signaling for polyamine biosynthesis in tobacco. Plant Cell Physiol. 50, 658–664. doi: 10.1093/pcp/pcp009
Kobayashi, Y., Murata, M., Minami, H., Yamamoto, S., Kagaya, Y., Hobo, T., et al. (2010). Abscisic acid-activated SNRK2 protein kinases function in the gene-regulation pathway of ABA signal transduction by phosphorylating ABA response element-binding factors. Plant J. 44, 939–949. doi: 10.1111/j.1365-313X.2005.02583.x
Kong, X., Pan, J., Zhang, M., Xing, X., Zhou, Y., Liu, Y., et al. (2011). ZmMKK4, a novel group C MAPK kinase in maize (Zea mays), confers salt and cold tolerance in transgenic Arabidopsis. Plant Cell Environ. 34, 1291–1303. doi: 10.1111/j.1365-3040.2011.02329.x
Lin, C., Zhang, Y. X., and Chai, T. Y. (2008). Arabidopsis DREB1A confers high salinity tolerance and regulates the expression of GA dioxygenases in Tobacco. Plant Sci. 174, 156–164. doi: 10.1016/j.plantsci.2007.11.002
Liu, Y., Wang, L., Xing, X., Sun, L. P., Pan, J. W., Kong, X. P., et al. (2013). ZmLEA3, a multifunctional group 3 LEA protein from Maize (Zea mays L.), is involved in biotic and abiotic stresses. Plant Cell Physiol. 54, 944–959. doi: 10.1093/pcp/pct047
Luo, Q., Wei, Q., Wang, R., Zhang, Y., Zhang, F., He, Y., et al. (2017). BdCIPK31, a calcineurin B-Like protein-interacting protein kinase, regulates plant response to drought and salt stress. Front. Plant Sci. 8:1184. doi: 10.3389/fpls.2017.01184
Mao, X., Zhang, H., Tian, S., Chang, X., and Jing, R. (2010). TaSnRK2.4, an SNF1-type serine/threonine protein kinase of wheat (Triticum aestivum L.), confers enhanced multistress tolerance in Arabidopsis. J. Exp. Bot. 61, 683–696. doi: 10.1093/jxb/erp331
Melcher, K., Ng, L. M., Zhou, X. E., Soon, F. F., Xu, Y., Suino-Powell, K. M., et al. (2009). A gate-latch-lock mechanism for hormone signalling by abscisic acid receptors. Nature 462, U602–U672. doi: 10.1038/nature08613
Mertens, J., Aliyu, H. A., and Cowan, D. A. (2018). LEA proteins and the evolution of the WHy domain. Appl. Environ. Microb. 84, 00539-18. doi: 10.1128/aem.00539-18
Miller, G., Suzuki, N., Ciftci-Yilmaz, S., and Mittler, R. (2010). Reactive oxygen species homeostasis and signalling during drought and salinity stresses. Plant Cell Environ. 33, 453–467. doi: 10.1111/j.1365-3040.2009.02041.x
Mittler, R., Vanderauwera, S., Gollery, M., and Van Breusegem, F. (2004). Reactive oxygen gene network of plants. Trends Plant Sci. 9, 490–498. doi: 10.1016/j.tplants.2004.08.009
Mizoguchi, M., Umezawa, T., Nakashima, K., Kidokoro, S., Takasaki, H., Fujita, Y., et al. (2010). Two closely related subclass II SnRK2 protein kinases cooperatively regulate drought-inducible gene expression. Plant Cell Physiol. 51, 842–847. doi: 10.1093/pcp/pcq041
Moore, K., and Roberts, L. J. (1998). Measurement of lipid peroxidation. Free Radic. Res. 28, 659–671. doi: 10.3109/10715769809065821
Nakashima, K., Fujita, Y., Kanamori, N., Katagiri, T., Umezawa, T., Kidokoro, S., et al. (2009). Three Arabidopsis SnRK2 protein kinases, SRK2D/SnRK2.2, SRK2E/SnRK2.6/OST1 and SRK2I/SnRK2.3, involved in ABA signaling are essential for the control of seed development and dormancy. Plant Cell Physiol. 50, 1345–1363. doi: 10.1093/pcp/pcp083
Nishimura, N., Hitomi, K., Arvai, A. S., Rambo, R. P., Hitomi, C., Cutler, S. R., et al. (2009). Structural mechanism of abscisic acid binding and signaling by dimeric PYR1. Science 326, 1373–1379. doi: 10.1126/science.1181829
Park, S. Y., Fung, P., Nishimura, N., Jensen, D. R., Fujii, H., Zhao, Y., et al. (2009). Abscisic acid inhibits type 2C protein phosphatases via the PYR/PYL family of START proteins. Science 324, 1068–1071. doi: 10.1126/science.1173041
Rosenberger, C. L., and Chen, J. (2018). To grow or not to grow: TOR and SnRK2 coordinate growth and stress response in Arabidopsis. Mol. Cell 69, 3–4. doi: 10.1016/j.molcel.2017.12.013
Ruizlozano, J. M., Porcel, R., Azcón, C., and Aroca, R. (2012). Regulation by arbuscular mycorrhizae of the integrated physiological response to salinity in plants: new challenges in physiological and molecular studies. J. Exp. Bot. 63:4033. doi: 10.1093/jxb/ers126
Soma, F., Mogami, J., Yoshida, T., Abekura, M., Takahashi, F., Kidokoro, S., et al. (2017). ABA-unresponsive SnRK2 protein kinases regulate mRNA decay under osmotic stress in plants. Nat. Plants 3:16204. doi: 10.1038/nplants.2016.204
Song, X., Yu, X., Hori, C., Demura, T., Ohtani, M. A., and Zhuge, Q. (2016). Heterologous overexpression of poplar SnRK2 genes enhanced salt stress tolerance in Arabidopsis thaliana. Front. Plant Sci. 7:612. doi: 10.3389/fpls.2016.00612
Stracke, R., Werber, M., and Weisshaar, B. (2001). The R2R3-MYB gene family in Arabidopsis thaliana. Curr. Opin. Plant Biol. 4, 447–456. doi: 10.1016/s1369-5266(00)00199-0
Tian, S., Mao, X., Zhang, H., Chen, S., Zhai, C., Yang, S., et al. (2013). Cloning and characterization of TaSnRK2.3, a novel SnRK2 gene in common wheat. J. Exp. Bot. 64, 2063–2080. doi: 10.1093/jxb/ert072
Umezawa, T., Sugiyama, N., Mizoguchi, M., Hayashi, S., Myouga, F., Yamaguchi-Shinozaki, K., et al. (2009). Type 2C protein phosphatases directly regulate abscisic acid-activated protein kinases in Arabidopsis. Proc. Natl. Acad. Sci. U.S.A. 106, 17588–17593. doi: 10.1073/pnas.0907095106
Umezawa, T., Sugiyama, N., Takahashi, F., Anderson, J. C., Ishihama, Y., Peck, S. C., et al. (2013). Genetics and phosphoproteomics reveal a protein phosphorylation network in the abscisic acid signaling pathway in Arabidopsis thaliana. Sci. Signal 6:rs8. doi: 10.1126/scisignal.2003509
Vishwakarma, K., Upadhyay, N., Kumar, N., Yadav, G., Singh, J., Mishra, R. K., et al. (2017). Abscisic acid signaling and abiotic stress tolerance in plants: a review on current knowledge and future prospects. Front. Plant Sci. 8:161. doi: 10.3389/fpls.2017.00161
Vlad, F., Rubio, S., Rodrigues, A., Sirichandra, C., Belin, C., Robert, N., et al. (2009). Protein phosphatases 2C regulate the activation of the Snf1-related kinase OST1 by abscisic acid in Arabidopsis. Plant Cell 21, 3170–3184. doi: 10.1105/tpc.109.069179
Wang, L., Hu, W., Feng, J., Yang, X., Huang, Q., Xiao, J., et al. (2016). Identification of the ASR gene family from Brachypodium distachyon and functional characterization of BdASR1 in response to drought stress. Plant Cell Rep. 35, 1221–1234. doi: 10.1007/s00299-016-1954-6
Wang, L., Hu, W., Sun, J., Liang, X., Yang, X., Wei, S., et al. (2015). Genome-wide analysis of SnRK gene family in Brachypodium distachyon and functional characterization of BdSnRK2.9. Plant Sci. 237, 33–45. doi: 10.1016/j.plantsci.2015.05.008
Wang, X., Zeng, J., Li, Y., Rong, X., Sun, J., Sun, T., et al. (2015). Expression of TaWRKY44, a wheat WRKY gene, in transgenic tobacco confers multiple abiotic stress tolerances. Front. Plant Sci. 6:615. doi: 10.3389/fpls.2015.00615
Wang, P., Zhao, Y., Li, Z., Hsu, C. C., Liu, X., Fu, L., et al. (2018). Reciprocal regulation of the TOR kinase and ABA receptor balances plant growth and stress response. Mol. Cell 69, 100.e6–112.e6. doi: 10.1016/j.molcel.2017.12.002
Wang, Y., Li, T., John, S. J., Chen, M., Chang, J., Yang, G., et al. (2018). A CBL-interacting protein kinase TaCIPK27 confers drought tolerance and exogenous ABA sensitivity in transgenic Arabidopsis. Plant Physiol. Biochem. 123, 103–113. doi: 10.1016/j.plaphy.2017.11.019
Wasternack, C., and Song, S. (2017). Jasmonates: biosynthesis, metabolism, and signaling by proteins activating and repressing transcription. J. Exp. Bot. 68, 1303–1321. doi: 10.1093/jxb/erw443
Xiong, L., and Zhu, J. K. (2002). Molecular and genetic aspects of plant responses to osmotic stress. Plant Cell Environ. 25, 131–139. doi: 10.1046/j.1365-3040.2002.00782.x
Yoshida, T., Fujita, Y., Sayama, H., Kidokoro, S., Maruyama, K., Mizoi, J., et al. (2010). AREB1, AREB2, and ABF3 are master transcription factors that cooperatively regulate ABRE-dependent ABA signaling involved in drought stress tolerance and require ABA for full activation. Plant J. 61, 672–685. doi: 10.1111/j.1365-313X.2009.04092.x
Zhang, H., Li, W., Mao, X., Jing, R. A., and Jia, H. (2016). Differential activation of the wheat SnRK2 family by abiotic stresses. Front. Plant Sci. 7:420. doi: 10.3389/fpls.2016.00420
Zhang, H., Mao, X., Jing, R., Chang, X., and Xie, H. (2011). Characterization of a common wheat (Triticum aestivum L.) TaSnRK2.7 gene involved in abiotic stress responses. J. Exp. Bot. 62, 975–988. doi: 10.1093/jxb/erq328
Zhang, H., Mao, X., Wang, C., and Jing, R. (2010). Overexpression of a common wheat gene TaSnRK2.8 enhances tolerance to drought, salt and low temperature in Arabidopsis. PLoS One 5:e16041. doi: 10.1371/journal.pone.0016041
Zhang, Y., Zhao, H., Zhou, S., He, Y., Luo, Q., Zhang, F., et al. (2018). Expression of TaGF14b, a 14-3-3 adaptor protein gene from wheat, enhances drought and salt tolerance in transgenic tobacco. Planta 248, 117–137. doi: 10.1007/s00425-018-2887-9
Zhao, Y., Zhang, Z., Gao, J., Wang, P., Hu, T., Wang, Z., et al. (2018). Arabidopsis duodecuple mutant of PYL-ABA receptors reveals PYL repression of ABA-independent SnRK2 activity. Cell Rep. 23, 3340.e5–3351.e5. doi: 10.1016/j.celrep.2018.05.044
Keywords: wheat, TaSnRK2.9, abiotic stress, antioxidant, ROS, ABA, SnRK-ABF
Citation: Feng J, Wang L, Wu Y, Luo Q, Zhang Y, Qiu D, Han J, Su P, Xiong Z, Chang J, Yang G and He G (2019) TaSnRK2.9, a Sucrose Non-fermenting 1-Related Protein Kinase Gene, Positively Regulates Plant Response to Drought and Salt Stress in Transgenic Tobacco. Front. Plant Sci. 9:2003. doi: 10.3389/fpls.2018.02003
Received: 02 October 2018; Accepted: 27 December 2018;
Published: 14 January 2019.
Edited by:
Lam-Son Tran, RIKEN, JapanReviewed by:
Chang-Jie Jiang, National Agriculture and Food Research Organization, JapanKazuo Nakashima, Japan International Research Center for Agricultural Sciences, Japan
Copyright © 2019 Feng, Wang, Wu, Luo, Zhang, Qiu, Han, Su, Xiong, Chang, Yang and He. This is an open-access article distributed under the terms of the Creative Commons Attribution License (CC BY). The use, distribution or reproduction in other forums is permitted, provided the original author(s) and the copyright owner(s) are credited and that the original publication in this journal is cited, in accordance with accepted academic practice. No use, distribution or reproduction is permitted which does not comply with these terms.
*Correspondence: Guangxiao Yang, ygx@hust.edu.cn Guangyuan He, hegy@hust.edu.cn
†These authors have contributed equally to this work