- 1Faculty of Science and Technology, Free University of Bozen-Bolzano, Bolzano, Italy
- 2Department of Life Sciences and Biotechnology, University of Ferrara, Ferrara, Italy
- 3Department of Chemistry, Life Sciences and Environmental Sustainability, University of Parma, Parma, Italy
In ecological theory, it is currently unclear if intraspecific trait responses to environmental variation are shared across plant species. We use one of the strongest environmental variations in alpine ecosystems, i.e., advanced snowmelt due to climate warming, to answer this question for alpine snowbed plants. Snowbeds are extreme habitats where long-lasting snow cover represents the key environmental factor affecting plant life. Intraspecific variation in plant functional traits is a key to understanding the performance and vulnerability of species in a rapidly changing environment. We sampled snowbed species after an above-average warm winter to assess their phenotypic adjustment to advanced snowmelt, based on differences in the natural snowmelt dynamics with magnitudes reflecting predicted future warming. We measured nine functional traits related to plant growth and reproduction in seven vascular species, comparing snowbeds of early and late snowmelt across four snowbed sites in the southern Alps in Italy. The early snowbeds provide a proxy for the advanced snowmelt caused by climatic warming. Seed production was reduced under advanced snowmelt in all seed-forming snowbed species. Higher specific leaf area (SLA) and lower leaf dry matter content (LDMC) were indicative of improved growth potential in most seed-forming species under advanced snowmelt. We conclude, first, that in the short term, advanced snowmelt can improve snowbed species’ growth potential. However, in the long term, results from other studies hint at increasing competition in case of ongoing improvement of conditions for plant growth under continued future climate warming, representing a risk for snowbed species. Second, a lower seed production can negatively affect the seed rain. A reduction of propagule pressure can be crucial in a context of loss of the present snowbed sites and the formation of new ones at higher altitudes along with climate warming. Finally, our findings encourage using plant functional traits at the intraspecific level across species as a tool to understand the future ecological challenges of plants in changing environments.
Introduction
In the alpine environment, climate change has a dramatic influence on plant life. Rising temperatures are altering the timing of one of the most important determinants of plant distribution, i.e., the growing season and the related phenology (Walter and Breckle, 2002; Chuine, 2010; Matteodo et al., 2016). In this context, strong ecological differences are created by alterations in the timing of the melting of the snow cover because it triggers phenology at the verge of plant life. Responses of plants to environmental modifications are first visible in the adjustment of their functional traits within the reaction norm. However, it is not clear whether the adjustment of trait values is a key for trait-based predictions of species performance in plant communities (Roscher et al., 2018) as individual plant species may differ in their responses to environmental changes (Klanderud, 2005). Recently, the importance of intraspecific functional variability for better understanding species’ ecological fate and community dynamics has been recognized in the ecological literature (Ackerly and Cornwell, 2007; Albert et al., 2011; Violle et al., 2012; Wellstein et al., 2013; Jung et al., 2014; Sides et al., 2014; Escudero and Valladares, 2016; Chalmandrier et al., 2017; Roscher et al., 2018). Intraspecific trait variability can be genetically determined or be the result of the variation of trait phenotype under the direct effect of the environment. The phenotypic modification of a trait may result in different abilities of a plant to cope with new environmental conditions driving rapid plants’ reaction through morphological and physiological adjustment (Nicotra et al., 2010; Wellstein et al., 2013; Garnier et al., 2016). Defining magnitude and direction of phenotypic adjustments within a species will help to formulate hypotheses about the fate of species under climatic and environmental changes (Nicotra et al., 2010; Liancourt et al., 2015).
In this context, the particular vulnerability of alpine snowbeds to climate warming makes this ecosystem particularly suitable for the study of the effect of climate change on phenotypic variation of alpine plant species’ functional traits. Alpine snowbeds are ecologically extreme habitats, situated in areas covered with snow during most of the year. Snow cover is the main environmental factor controlling plant life in snowbeds. Indeed, long-lasting snow cover ensures stable temperatures during winter (Körner, 2003; Björk and Molau, 2007; Pignatti and Pignatti, 2014), protects plants from frost damage (Heegaard, 2002; Shimono and Kudo, 2003; Björk and Molau, 2007), controls soil water content, and determines the length of the growing season in relation to snowmelt date (Körner, 2003; Choler, 2005; Keller et al., 2005; Schöb et al., 2009). Snowbed plant species are highly specialized and well adapted to extreme environmental conditions (Billings and Mooney, 1968; Körner, 2003; Schöb et al., 2008; Hülber et al., 2011; Pignatti and Pignatti, 2014). They usually are very small in size and characterized by high relative growth rate and low carbon investment per unit leaf area to deal with the short growing season (Schöb et al., 2008). Phenology and growth in snowbed species are both closely related to snow persistence and snowmelt timing, that influence soil nutrient availability and soil water content (Venn and Morgan, 2007; Carbognani et al., 2012; Lluent et al., 2013; Livensperger et al., 2016). Snowbed vegetation is particularly sensitive to predicted changes in snow cover under climate warming (Schöb et al., 2008; Baptist et al., 2010; Carbognani et al., 2012, 2014a; Matteodo et al., 2016). Snow cover thickness and duration are strictly linked to air temperature during winter and spring, and to winter precipitation (Heegaard, 2002; Körner, 2003). The majority of future climate models forecasts rising temperatures during the twenty-first century which will imply a significant decrease in snow cover at all elevations in the mountains (Beniston et al., 2003; Watson and Haeberli, 2004; ACIA, 2005; Giorgi and Lionello, 2008; Kotlarski et al., 2012; Steger et al., 2013; Gobiet et al., 2014; Pachauri et al., 2014; Klein et al., 2016). Although some models forecast increasing precipitation in winter, higher fraction of winter precipitation is predicted to fall as rain instead of snow (Giorgi and Lionello, 2008; Gobiet et al., 2014), thus resulting in thinner and consequently less insulating snowpack (Wipf et al., 2009). Higher temperature and reduced snow cover will lead to an earlier snowmelt date that will anticipate the beginning of the growing season of snowbed species (Wipf et al., 2009; Sedlacek et al., 2015). Advanced snowmelt can trigger different mechanisms, i.e., (i) prolonging the duration of the growing season which could enhance plant growth (Theurillat and Guisan, 2001; Winkler et al., 2018), (ii) exposing vegetation to dangerous spring frost events (Inouye, 2008; Wipf et al., 2009; Baptist et al., 2010; Gerdol et al., 2013; Wheeler et al., 2015), (iii) improving fertility of nutrient-poor soils through enhanced mineralization rates (Billings and Bliss, 1959; Stanton et al., 1994; Körner, 2003) and (iv) reducing soil water content (Galen and Stanton, 1991; Giménez-Benavides et al., 2007; Venn et al., 2011; Winkler et al., 2018). Indeed, snowbed soils are subjected to high rates of water loss because of low field capacity coupled with high solar irradiance that enhances evaporation (Isard, 1986; Björk and Molau, 2007). Whereas snowbed vegetation may currently experience dry conditions at the middle or at the end of the growing season only, earlier snowmelt timing may extend drought stress toward the beginning of the growing season (Inouye, 2008; Venn and Morgan, 2009).
The effects of climate change on snowbeds have been extensively investigated in terms of changes in vegetation cover, community composition, species distribution (Galen and Stanton, 1995; Heegaard, 2002; Schöb et al., 2008, 2009; Hülber et al., 2011; Carbognani et al., 2012, 2014b; Sandvik and Odland, 2014; Matteodo et al., 2016) and phenological alterations (Walker et al., 1995; Sandvik and Totland, 2000; Hülber et al., 2010; Dorji et al., 2013; Petraglia et al., 2014a; Carbognani et al., 2016, 2018), sometimes through the analysis of functional diversity and community-weighted trait means (Venn et al., 2011; Pickering et al., 2014; Komac et al., 2015). Few studies have so far focused on the phenotypic variability of the functional traits of snowbed species (Kudo et al., 1999; Baptist et al., 2010; Komac et al., 2015; Sedlacek et al., 2015). In this study, we collected snowbed species after an above-average warm and dry winter (Colucci et al., 2017) to assess the effects of advanced snowmelt on seven representative snowbed plant species through the analysis of phenotypic variation of functional traits. The functional traits considered include both vegetative and reproductive traits that have been found to show plastic adjustment to environmental conditions (Nicotra et al., 2010; Wellstein et al., 2013; Jung et al., 2014; Liancourt et al., 2015; Chelli et al., 2017). These functional traits are related to important plant functions such as photosynthetic capacity, growth; and ultimately, competitive ability, reproduction and survival (Kudo et al., 1999; Baptist et al., 2010; Lluent et al., 2013; Pérez-Harguindeguy et al., 2013; Liancourt et al., 2015). Our study was directed to answer the following questions:
(i) Do snowbed species exhibit an intraspecific response to advanced snowmelt conditions in terms of functional traits?
(ii) Does this intraspecific traits adjustment show a common trend across different snowbed plant species and sites?
Materials and Methods
Study Areas and Species
Four snowbed sites located in the south-eastern Alps of Italy were investigated, two sites on calcareous bedrock and two on siliceous bedrock (Figure 1, see Supplementary Figure S1 for the detailed locations of the sites). The two calcareous sites were selected from snowbeds reported in Pignatti and Pignatti (2014), following the criteria of (i) representativeness of snowbed community and (ii) accessibility of the site. The selected calcareous sites are located at Forcella Travenanzes (46°31′ N, 12°1′ E, 2450 m, Province of Belluno) and on Pale di San Martino (46°16′ N, 11°50′ E, 2500 m, Province of Trento). These snowbeds are characterized by vegetation dominated by Salix retusa L. and Salix reticulata L. (Ellenberg, 1988; Grabherr and Mucina, 1993; Tomaselli et al., 2006; Pignatti and Pignatti, 2014). Less information about siliceous snowbed sites was available. Therefore, we selected siliceous sites with known representation of siliceous snowbed communities. These sites are located close to Passo Gavia (46°20′ N, 10°29′ E, 2651 m, Province of Sondrio) and in the upper Val Martello (46°28′ N, 10°40′ E, 2600 m, Province of Bolzano-Bozen). These snowbeds are characterized by vegetation dominated by Salix herbacea L. and by the moss Polytrichastrum sexangulare (Brid.) G. L. Sm. (Ellenberg, 1988; Grabherr and Mucina, 1993; Carbognani et al., 2014b).
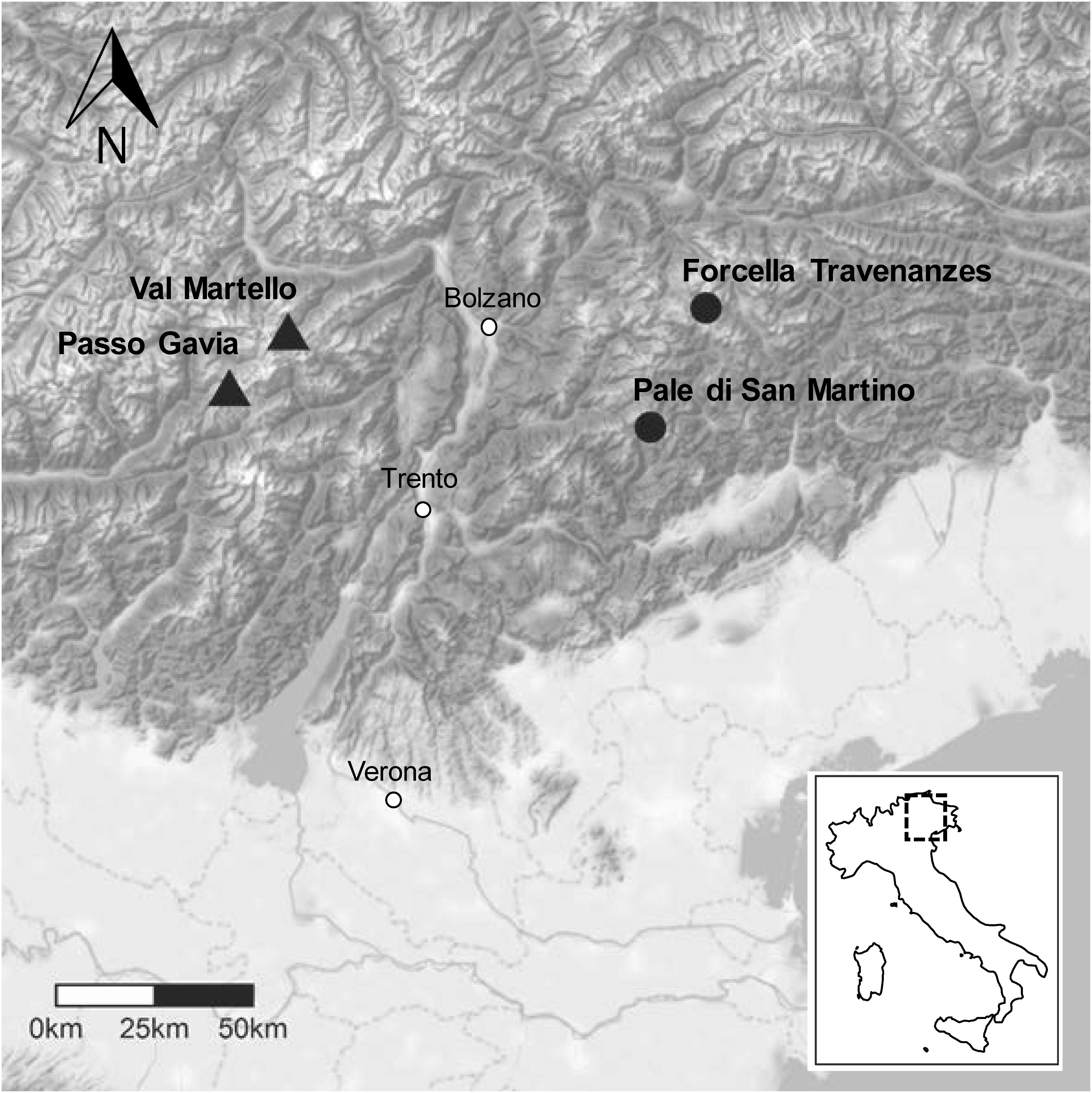
Figure 1. Location of the four snowbed sites in the Italian eastern Alps. Circles and triangles represent calcareous and siliceous sites, respectively.
Climatic data were obtained from weather stations close to the sites at almost the same elevation, with the exception of the Solda-Madriccio station located about 200 m above the Val Martello site. At Forcella Travenanzes, the mean annual temperature is -1°C, with an average minimum of -16°C and an average maximum of 13.5°C (data from Ra Valles station, 2592 m). At Pale di San Martino, mean annual temperature is 0.5°C. The average minimum and maximum are -19°C and 15.5°C, respectively (data from Rifugio Rosetta station, 2581 m). At Passo Gavia, mean annual temperature is -1.4°C. The average minimum is -10°C and the average maximum is 9.3°C (data from Passo Gavia, 2651 m, according to WorldClim datasets at a spatial resolution of 30 arc second; Hijmans et al., 2005). At Val Martello, mean annual temperature is -2°C with an average minimum of -23.7°C and a maximum of 15.9°C (data from Solda-Madriccio, 2825 m). Mean total annual precipitation is similar at the four sites, ranging from 1000 mm at Val Martello, to 1054 mm at Pale di San Martino, and 1150 mm at Passo Gavia. No data are available for Forcella Travenanzes. However, mean total annual precipitation at the Passo Falzarego station (2090 m, ca 350 m below the Forcella Travenanzes site) is 1230 mm.
From character and accompanying species of the snowbed communities (Ellenberg, 1988; Grabherr and Mucina, 1993; Pignatti and Pignatti, 2014) which are also the most frequent ones, we selected seven vascular plant species that were present in at least two of both of our calcareous and siliceous snowbed study sites: the two dwarf shrubs Salix herbacea and S. retusa as character species of the two associations of calcareous and siliceous bedrock, the grass Poa alpina L., and the forbs Veronica alpina L., Gnaphalium supinum L., Leucanthemopsis alpina (L.) Heywood and Polygonum viviparum L. [ = Bistorta vivipara (L.) S. Gray] (see Table 1 and Supplementary Table S1).
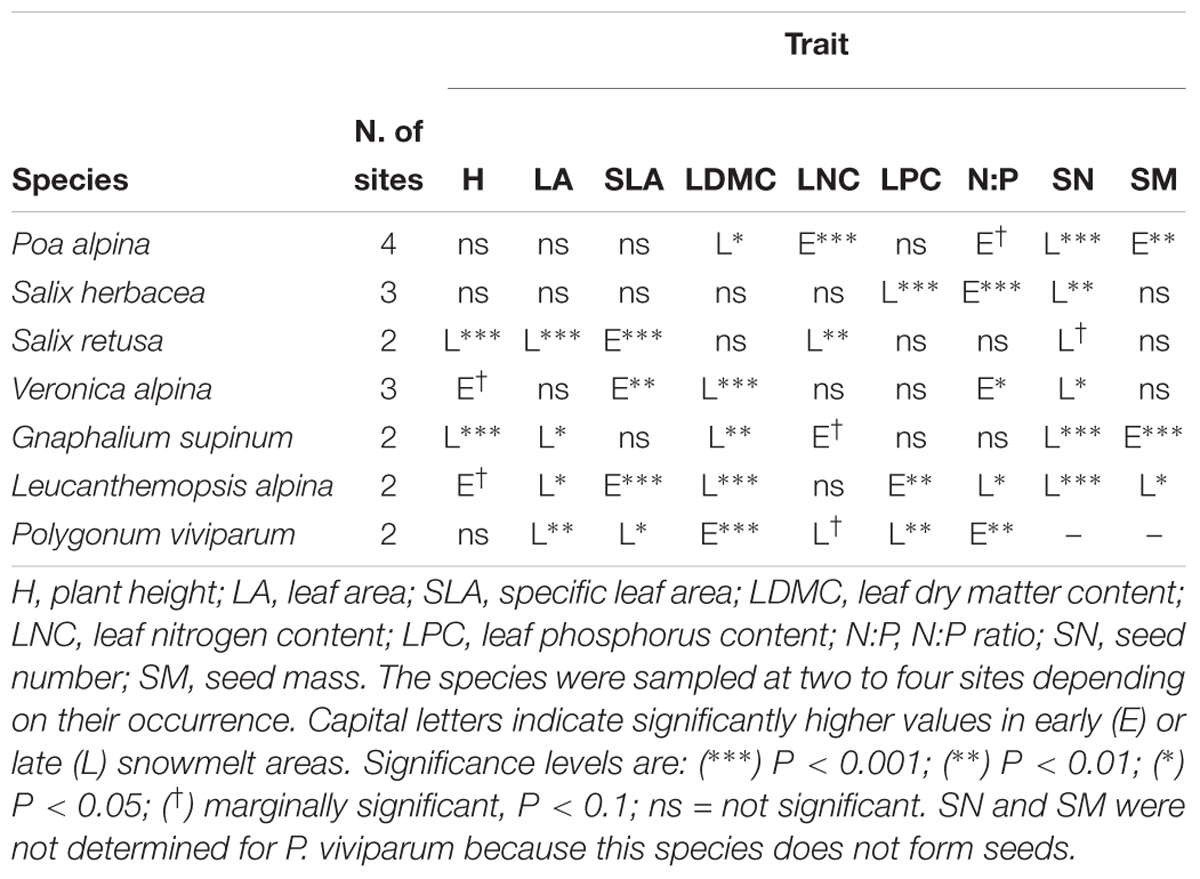
Table 1. Results of linear mixed effect models for differences between early and late snowmelt areas of single plant traits for each study species across the four study sites.
Study Design and Trait Data
At each of the four sites, one snowbed of early and one of late snowmelt (henceforth called early snowmelt area and late snowmelt area) were chosen resulting in four early snowbeds and four late snowbeds in total (detailed coordinates and location of each snowmelt area are reported in Supplementary Table S2). The early snowmelt areas represented areas of advanced snowmelt after the above-average warm winter of 2015/2016 characterized by a low amount of snowfall (Colucci et al., 2017, see Supplementary Figure S2 for inter-annual variability of temperature and precipitation obtained from climatic stations close to the study sites). The identification of the snowmelt areas was based on previous monitoring of snowmelt timing (Carbognani et al., 2016, personal observations). The shape of a snowbed area (15–25 m2) was consistent with snowmelt isolines that followed the microtopographic contour lines of the snowbed. We checked the precise snowmelt timing and hence the magnitude of snow-cover duration by continuously recording soil temperatures by data loggers. A data logger (Hobo, Onset Computer Corporation, Bourne, MA, United States) was placed in the ground at 5 cm depth in each area at each site. Soil temperatures were recorded at 1 h resolution starting before the first snowfall of 2015 until end August 2016. We compared the daily mean temperatures for a period of 6 weeks, starting from the date of snow-free conditions onwards, in early vs. late snowmelt areas across sites. This period corresponded to the growing season during which all vegetative and reproductive traits reached completion. Details on snowmelt timing and temperature at each site are reported in Figure 2.
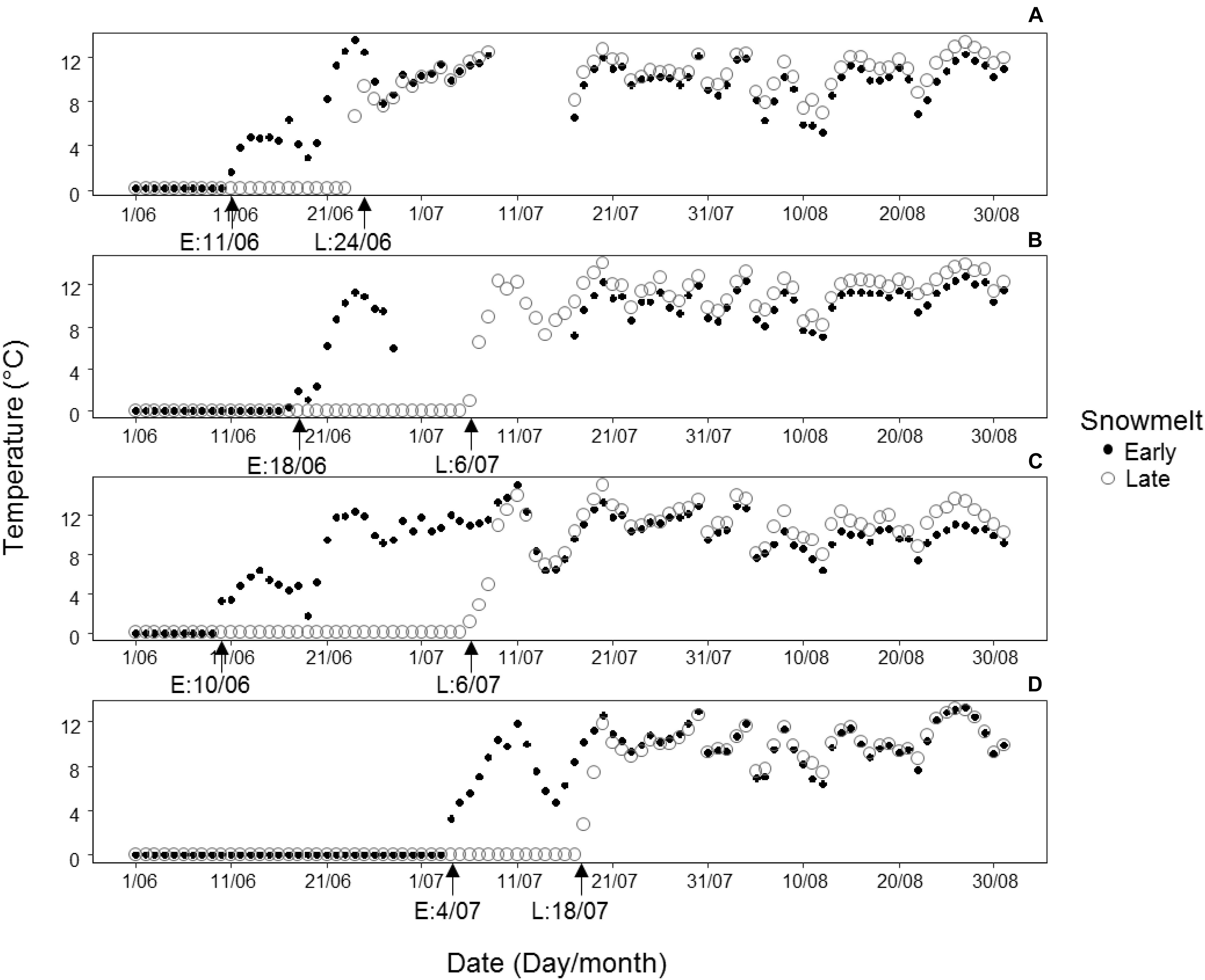
Figure 2. Patterns of daily mean soil temperatures (°C) registered at 5 cm soil depth during the growing season from melt-out date until the end of August in 2016 in areas of early (E, black points) and late (L, circles) snowmelt at each of the four study sites: (A) Forcella Travenanzes, (B) Pale di San Martino, (C) Val Martello, (D) Passo Gavia. The x axis represents the timescale in intervals of 10 days; the melt-out dates for the areas of early (E) and late (L) snowmelt are indicated by arrows on the x axis. For Forcella Travenanzes data from 8.07 to 16.07 are lacking because of loggers’ malfunction. For the same reason for Pale di San Martino data are lacking from 29.06 to 15.07 for early snowmelt area.
In total, seven vegetative and two reproductive traits were investigated in the selected seven snowbed species. The following vegetative key traits were determined in 30 randomly chosen individuals per snowbed area for each species: plant height (H), leaf area (LA), specific leaf area (SLA), leaf dry matter content (LDMC), leaf nitrogen content (LNC), leaf phosphorus content (LPC) and leaf N:P ratio. All of these traits are related to plants’ ecological strategies (for details see Supplementary Table S3), furthermore H and LA are also informative about competitive ability and plant persistence (Westoby, 1998; Díaz et al., 2016). SLA and LDMC are related to potential growth rate (Poorter and Remkes, 1990) while nutrient traits (LNC, LPC and N:P) can be considered as proxies of the soil nutrient status that can also assess the type of nutrient limitation (Güsewell, 2004; Pérez-Harguindeguy et al., 2013).
The two reproductive traits, number of seeds (SN) and seed mass (SM), were determined in 30 randomly chosen individuals per snowbed area for each species except P. viviparum which reproduces in a vegetative manner by bulbils. These traits are good indicators of the capacity of species to disperse and establish (Pierce et al., 2014; Rosbakh et al., 2014). More information about the functional significance of all of the assessed traits are provided in Supplementary Table S3.
The snowbed species have a different capacity of clonal growth. Five species, namely G. supinum, L. alpina, P. alpina, P. viviparum, and V. alpina, have very limited clonal growth (scale of few millimeters per year) using epigeous rhizomes, or in case of V. alpina hypogeous rhizomes (Klimešová et al., 2017)1. Thus, for these species, the 30 sampled plants in each snowbed area most likely represent distinct individuals. The two willow species, S. herbacea and S. retusa, have more extended clonal growth (scale of some centimeters per year) using the stem organ (Klimešová et al., 2017)1. For these species, we cannot exclude the possibility that some of the 30 sampled plants of a species in each snowbed area are ramets belonging to the same genet.
Data Collection
Sampling was carried out in 2016. We surveyed the four sites several times during the growing season and sampled plants for determining vegetative traits at the peak of the growing season, i.e., on different dates at each snowbed area. Before sampling, a preliminary survey for detecting herbivore damage was done. No visible signs of damage were found, except for few individuals of S. herbacea at the Val Martello site. According to the protocol (Pérez-Harguindeguy et al., 2013), the damaged leaves were not sampled. Since the two Salix spp. are dioecious species, to avoid biases due to sexual differences in functional traits, we always sampled female individuals of S. herbacea and S. retusa in every site. This was with the exception of S. herbacea growing in the early snowmelt area at Forcella Travenanzes, where there were no females. For each of the 30 randomly chosen healthy individuals (ramets for S. retusa and S. herbacea) of each snowbed area, the height was measured in the field using a caliper (Metrica, precision 0.05 mm) because of the small size of the plants. SLA and LDMC were determined on the same individuals used for measuring height. To this end, the plants were harvested and immediately sealed in a plastic bag wrapped with wet paper (Pérez-Harguindeguy et al., 2013).
We let the individuals rehydrate overnight and the day after we determined fresh weight and area of one fully expanded sound leaf for individual (CanoScan LiDE 120, Canon). The same leaves were weighed again after being dried at 70°C for 72 h (Pérez-Harguindeguy et al., 2013). To determine nutrient contents, several individuals were pooled randomly to obtain enough material for chemical analyses of five replicate samples. The material was oven-dried, grinded and digested at 420°C in 3 mL of selenous H2SO4. The digests were then processed through a continuous flow autoanalyzer (FlowSys; Systea, Anagni, Italy) to determine total N and P concentration by the salicylate method and molybdenum blue method, respectively.
Seeds were collected from another set of 30 randomly selected individuals per species per snowbed area. Seed collection was performed at the time of seed maturity before dispersal. The seeds were air-dried for 30 days and then counted to determine the total number of seeds per individual for G. supinum, L. alpina, and P. alpina, and the seed number per fruit for S. retusa, S. herbacea, and V. alpina. After counting, we determined seed mass using a balance with 0.001 mg accuracy.
It was not possible to collect seeds of P. alpina at Pale di San Martino because at this site almost all individuals of P. alpina belonged to the vivipara variety. Neither could seeds from the small population of S. herbacea at Forcella Travenanzes be sampled because there were no females in that population.
As another exception, slightly fewer numbers of individuals were sampled for reproductive traits in case of one site regarding L. alpina [Val Martello; early (n = 27) and late (n = 26)] and S. retusa [Pale di San Martino; early (n = 19)] as these areas harbored no further individuals.
Data Analysis
Differences in mean daily soil temperature during the growing season between early and late snowmelt areas were statistically assessed by a linear mixed effect model. We used temperature as the dependent variable, snowmelt (factor level k = 2: early or late) as fixed variable and snowbed site (factor level k = 4) as random variable.
Differences in functional trait attribute values between early and late snowmelt areas were assessed separately for each functional trait in each of the seven species. To this end, linear mixed effect models were applied using the functional trait value as the dependent variable, snowmelt (factor level k = 2: early or late) as fixed variable and snowbed site (factor level k = 2–4, depending on species occurrence in each site) as random variable. This allowed understanding how strongly each trait responded to snowmelt timing independent of the specific effects of the snowbed site.
In addition, to make the specific effect of snowbed sites apparent, linear models with each single functional trait as dependent variable and snowmelt and snowbed site as independent variables were applied. Subsequently, two-way ANOVAs were used to test for differences in functional trait values between snowbed sites and snowmelt timing.
The data were log-transformed prior to analysis whenever they did not meet the assumption of normality as assessed by the Shapiro test. All statistical analyses were performed in R (R Development Core Team, 2014 version 3.1.2). Linear mixed effect models were performed using the nlme package (Pinheiro et al., 2017).
Results
Snowmelt Timing and Soil Temperature
On average, snow-free conditions occurred 18 days earlier in the early snowmelt areas than in the late snowmelt areas (see Figure 2 for detailed information about snowmelt dates in each site). Daily mean soil temperature in the growing season was significantly lower in the early snowmelt areas (Figure 3).
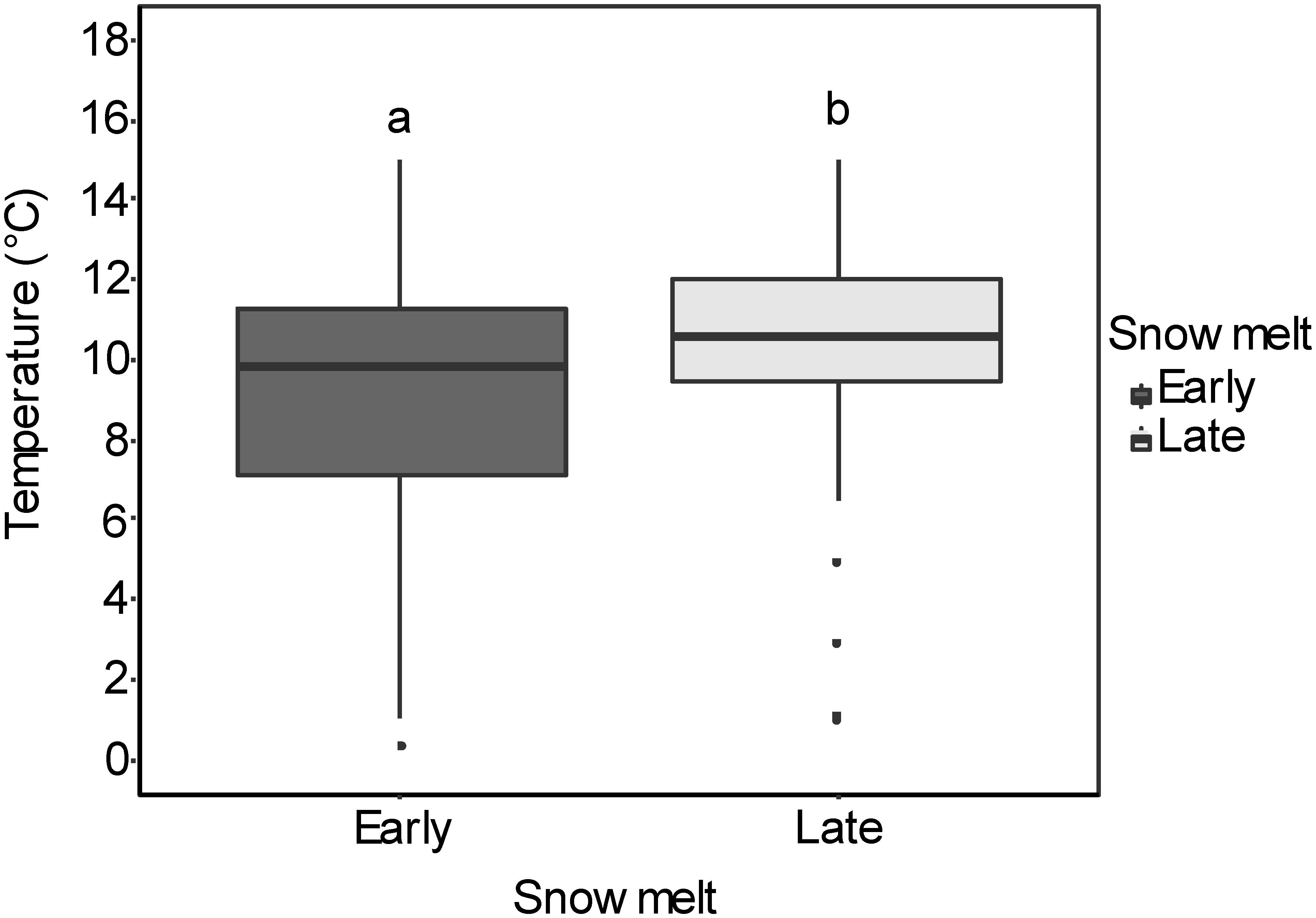
Figure 3. Box-whisker plots for soil temperatures in early and late snowmelt areas (number of sites = 4) for 6 weeks from melt-out date in 2016. The boxes comprise the 25th and 75th percentile with the upper and lower whiskers extending from the hinge to the upper and lower 1.5 inter-quartile ranges, respectively. The different letters indicate significant differences (P < 0.05) between early and late snowmelt areas. Detailed results of linear mixed effect models are given in Supplementary Table S4.
Vegetative Traits
The height of S. retusa and G. supinum was significantly lower in early snowmelt areas compared with late snowmelt areas (Figure 4 and Table 1). LA was lower in early snowmelt areas for S. retusa, G. supinum, L. alpina, and P. viviparum. SLA was higher in early snowmelt areas for S. retusa, V. alpina, and L. alpina (Figure 4 and Table 1). LDMC showed a reverse pattern compared with SLA with lower values in early snowmelt areas for V. alpina and L. alpina. S. retusa did not exhibit significant differences for these traits. Poa alpina and G. supinum also had lower LDMC in early snowmelt areas. Only P. viviparum did exhibit lower SLA and higher LDMC in early snowmelt areas (Figure 4 and Table 1). LNC in the seven species varied rather randomly in relation to snowmelt timing. While P. alpina presented higher LNC in early snowmelt areas, the reverse was true for S. retusa (Figure 4 and Table 1). LPC was lower in early snowmelt areas for S. herbacea and P. viviparum but higher in early snowmelt areas for L. alpina (Figure 4 and Table 1). The N:P ratio was higher in early snowmelt areas for most species: S. herbacea, V. alpina, P. viviparum and, marginally, for P. alpina. Only for L. alpina the N:P ratio was lower in early snowmelt areas (Figure 4 and Tables 1, 2).
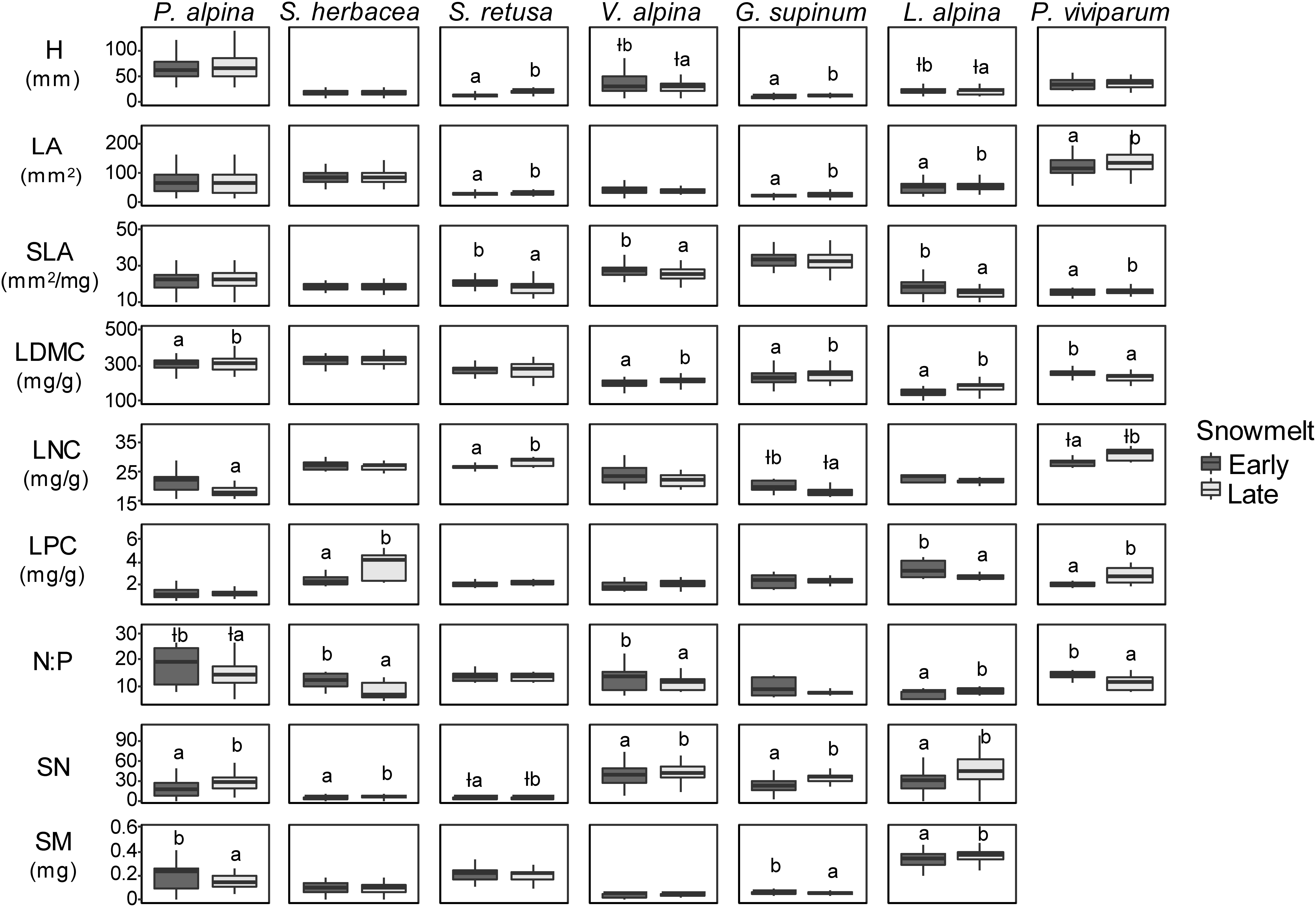
Figure 4. Box-whisker plots for plant traits in the seven snowbed species at early (dark gray boxes on the left-hand side of each panel) and late (light gray boxes on the right-hand side of each panel) snowmelt areas. H, plant height; LA, leaf area; SLA, specific leaf area; LDMC, leaf dry matter content; LNC, leaf nitrogen content; LPC, leaf phosphorus content; N:P, N:P ratio; SN, seed number; SM, seed mass. The species were sampled at two to four sites depending on their occurrence. P. alpina and S. herbacea were sampled for SN and SM at three and two sites, respectively, because they did not produce seeds at the other sites. The boxes comprise the 25th and 75th percentile with the upper and lower whiskers extending from the hinge to the upper and lower 1.5 inter-quartile ranges, respectively. Different letters indicate significant differences (P < 0.05) between early and late snowmelt areas. The symbol ł before the letters indicates a marginal significance (P < 0.10). See Supplementary Table S5 for values of median and first and third quartiles of early and late snowmelt groups for each species and each trait. Detailed results of linear mixed effect models are given in Supplementary Table S6.
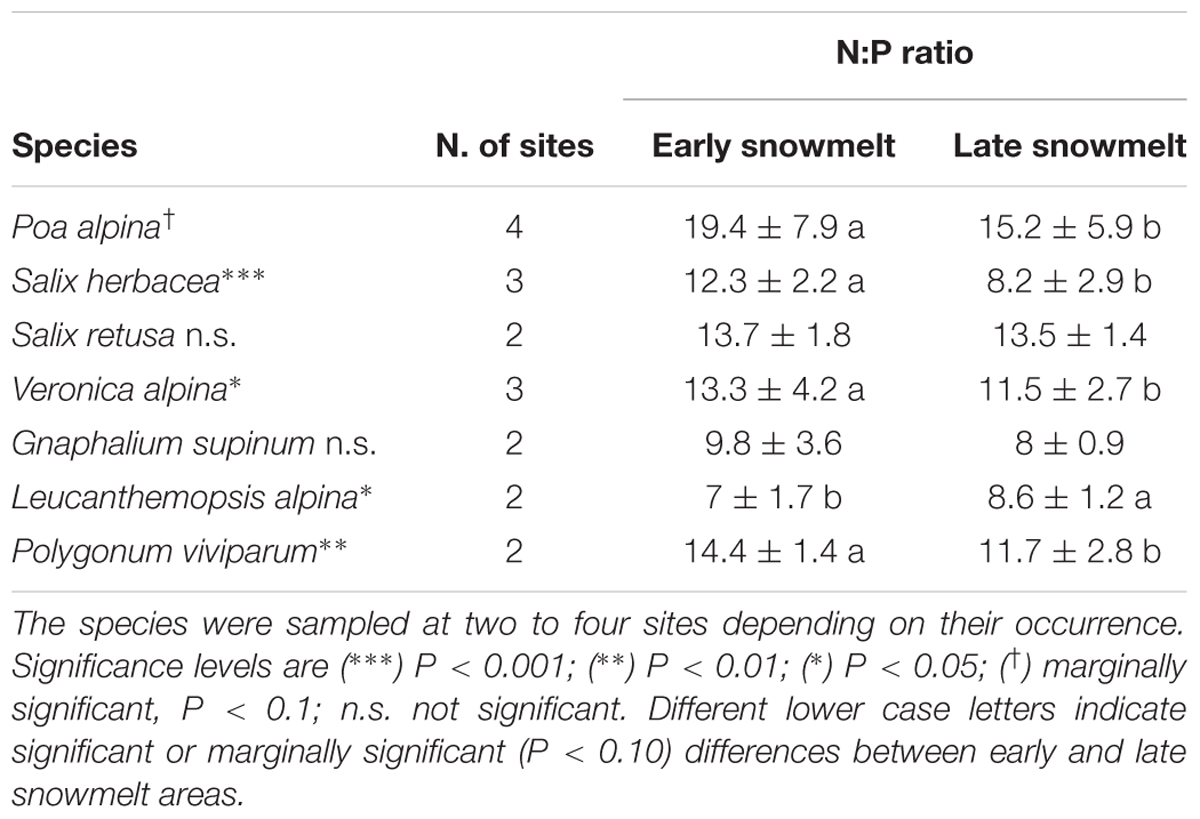
Table 2. Mean (±SD) N:P ratio for the seven species investigated in the early and late snowmelt areas across the four study sites.
Results of additional analyses of the same data based on linear models made the effect of sites apparent. In fact, results on trait-responses of species to advanced snowmelt held true also when the effect of the snowbed sites was added (see Supplementary Table S7).
Reproductive Traits
All seed-producing species produced less seeds in early snowmelt areas (Figure 4 and Table 1), with seed number being 40% lower for L. alpina, 35% lower for P. alpina, for G. supinum, 26% lower for S. herbacea and 13% lower for V. alpina in early vs. late snowmelt areas; in S. retusa the difference was only marginally significant (Figure 4 and Table 1). Poa alpina and G. supinum presented heavier seeds in early snowmelt areas (Figure 4 and Table 1). Conversely, L. alpina had lighter seeds in early snowmelt areas (Figure 4 and Table 1).
Discussion
Our findings demonstrate that advanced snowmelt triggers the adjustment of key functional traits related to reproduction and growth of snowbed species. In particular, seed number was reduced whereas SLA increased and LDMC and LA decreased under advanced snowmelt. As at least half of the seed-producing species exhibited the same direction in the intraspecific response of these four traits (SLA, LDMC, LA, seed number), while the other species kept the traits stable, we conclude that there was a robust trend in these phenotypic trait adjustments.
Another common trend was seen in an increase in the N:P ratio under advanced snowmelt. The other traits (height, LNC, LPC, SM) varied in opposing ways, meaning that they were not indicative of a straightforward plant trait-snowmelt response across species.
Reproductive Traits
Reduced seed production under advanced snowmelt probably depended on lower temperatures throughout the growing season in areas experiencing earlier emergence from snow. In fact, the soil temperatures during the vegetation period were significantly lower in the advanced snowmelt areas compared to the late snowmelt areas. It has already been demonstrated for another arctic-alpine species occurring at similar altitudes, i.e., Silene acaulis, that lowering the soil temperature by only 1°C during the maturation of flowers significantly reduced pollen tube length (Delph et al., 1997). Therefore, the temperature difference of more than 1°C in almost all of the early snowmelt areas (Forcella Travenanzes = 1.33°, Pale di San Martino = 1.35°, Val Martello = 2.01°, and Passo Gavia = 0.5°), could induce pollen limitation by reduced pollen tube growth and/or constrained pollen germination which will eventually result in lower fertilization success (Totland, 2001). A recent review confirms the importance of temperature on pollen performance and thus on seed quantity (Rosbakh et al., 2018). Reduced seed production by snowbed species under advanced snowmelt points toward negative effects on the seed rain. Fewer seeds would reduce the propagule pressure driving the establishment of new individuals and the colonization of novel sites (Lockwood et al., 2005). The need to establish on novel sites is a likely scenario under the effects of climate warming on high-altitude ecosystems (Hülber et al., 2011; Pickering et al., 2014; Komac et al., 2015). Grytnes et al. (2014) showed that novel snowbed sites at higher altitudes became available by the melting of ice and snow as a result of climate warming.
Contrary to seed number, seed mass varied little and not consistently in relation to snowmelt timing. Seed mass generally is a quite stable trait (Wellstein et al., 2013). Therefore, populations of alpine species growing over short distances are unlikely to produce seeds differing significantly in their mass (Pluess et al., 2005). In combination, less seeds without increased weight indicate less resources investment in sexual reproduction under advanced snowmelt.
Vegetative Traits
Snowbed species tended to reduce their LA under advanced snowmelt, as already found also by Domènech et al. (2016). At the same time, our results showed higher SLA and lower LDMC under advanced snowmelt for most of the seed-producing species. Because SLA is the ratio of LA to leaf dry weight, the decrease of LA under advanced snowmelt indicates less investment in leaf structural elements, as testified also by lower LDMC. As increased SLA and decreased LDMC are both positively related to relative growth rate (Poorter and Remkes, 1990; Hulshof et al., 2013), our findings indicate a likely positive short-term effect of longer growing season on growth of seed-producing snowbed species. Enhanced growth potential and reduced resource investment in leaf structural elements under advanced snowmelt weakens snowbed species in facing the risks associated with more frequent frost events earlier in the season (Inouye, 2008; Baptist et al., 2010; Wheeler et al., 2015). Whereas species adapted to snow-free exposed habitats such as Loiseleuria procumbens can profit from earlier start of the growing season (Wipf et al., 2009), snowbed species appear more prone to be damaged by frost events (Bannister et al., 2005; Wheeler et al., 2014). Our temperature records did not show occurrence of frost events at the study sites. Though, as the data loggers were not positioned aboveground, light frost events may not have been detected. However, visual inspection of the vegetation did not show any sign of frost damage in any species at any site. Thus, the tendency of the snowbed species to increase their SLA under advanced snowmelt conditions may indicate a relaxation of snowbeds’ environmental constrains in the year of observation (Rosbakh et al., 2014). Some of the species investigated presented a more conservative behavior in terms of leaf structural variation, as they did not exhibit any trait adjustment under advanced snowmelt. This is for example the case of G. supinum, S. herbacea and P. alpina for SLA. Interestingly, also Baptist et al. (2010) and Komac et al. (2015) reported no plastic responses to differential snowmelt timing in terms of SLA for G. supinum and P. alpina.
The LHS (leaf-height-seed) plant ecology strategy scheme (Westoby, 1998) states that resource allocation to one set of traits – i.e., L, H, or S – is only possible by deviating resources from other sets. If responding snowbed species in early snowmelt areas tend to invest more resources to leaf development, they will invest less for growth in height and/or for seed production. Interestingly, S. retusa grew shorter and tended to produce less seeds while increasing SLA under advanced snowmelt.
Polygonum viviparum, the only non-seed-producing species, showed an opposite direction of SLA and LDMC response in relation to snow-cover duration. This probably depended on the fact that P. viviparum was the only species reproducing in a vegetative manner through bulbils. Previous studies showed that the amount of resources stored in bulbils is significantly lower than that stored in seeds (Ceplitis and Bengtsson, 2004; Schnittler et al., 2009). Hence, the resources saved thanks to pseudo-viviparous reproduction could be used to improve leaf development also in the case of the short growing season.
Leaf nutrient contents varied overall little in relation to snowmelt timing while the N:P ratio varied more, both in relation to species identity, and snowmelt timing (Table 2). Leaf N:P ratio has often been used for assessing nutrient limitation in different ecosystems, with N:P ratio < 10 indicating N limitation and N:P ratio >20 indicating P limitation (Güsewell, 2004). As the leaf N:P ratio varies strongly because of intrinsic differences in foliar nutrient status among species and/or plant functional types (Gerdol et al., 2017), it does not seem appropriate to define absolute thresholds of N:P ratio to assess nutrient limitation. The overall low N:P ratios found in the present study suggest a general N-limitation or N-P co-limitation in snowbed habitats, in line with previous findings (Petraglia et al., 2013, 2014b). More interestingly, the leaf N:P ratio in most species was higher under advanced snowmelt. Koller and Phoenix (2017) observed soil P mineralization rates to be less responsive to environmental change than soil N mineralization rates. So, higher N:P ratios under advanced snowmelt may reflect stronger stimulation of N mineralization by shorter snow-cover duration.
Data Limitation
Our results are based on samples collected during the summer of 2016 after a particularly warm winter reflecting predicted magnitudes of climate warming (Supplementary Figure S2). However, even though there is a certain climatic variability between years, advanced snowmelt timing is predicted to be the norm in the future (ACIA, 2005; Pachauri et al., 2014). Regarding the snowbed species, they can be abundant within a snowbed but based on their distribution they do not always occur in every snowbed site (Pignatti and Pignatti, 2014). Therefore, four of the seven investigated species were present only in two sites. However, they showed the same trends detected for SLA, LDMC and seed number as the more replicated species.
Finally, this study investigated explicitly the phenotypic variation of the traits, without digging into the heritable component of intraspecific variability. Based on our findings of directed intraspecific functional trait adjustments, the present study opens the way to new interesting hypotheses, such as genetic differences and resulting ecotype differentiation in snowbed plant species.
Conclusion
Taken together, our findings on foliar trait adjustments suggest improved growth conditions for snowbed species under advanced snowmelt in a warmer climatic scenario. Therefore, advanced snowmelt can improve snowbed species’ growth potential in the short term. Though, in the long term it has been predicted that ongoing improvement of growth conditions under continued future climate warming would increase the competition among plants, leading to species replacement and consequent changes in plant community composition (Körner, 2003; Cannone et al., 2007; Gottfried et al., 2012; Rosbakh et al., 2017; Winkler et al., 2018). Moreover, lower seed production can negatively affect the seed rain. A reduction of propagule pressure can be crucial in a context of loss of the present snowbed sites and the formation of new ones at higher altitudes along with climate warming. However, hypotheses on long-term effects of climate change have always to be carefully formulated, given the possibility of unexpected effects of climate conditions acting in unpredictable ways on plant population dynamics (Cannone et al., 2007). Finally, our findings encourage using plant functional traits at the intraspecific level across species as a tool to understand the future ecological challenges of plants in changing environments.
Data Availability
All datasets generated for this study are included in the manuscript and/or the Supplementary Files.
Author Contributions
CW, RG, MT, and RT designed the study. RT collected the data of all sites, while AP and MC contributed to data collection at the Passo Gavia site. RT and CW analyzed the data and interpreted the results. RT wrote the first draft. All authors contributed to revisions.
Funding
RT had a Ph.D. grant from the Free University of Bolzano. This work was supported by the Open Access Publishing Fund provided by the Free University of Bozen-Bolzano.
Conflict of Interest Statement
The authors declare that the research was conducted in the absence of any commercial or financial relationships that could be construed as a potential conflict of interest.
Acknowledgments
We would like to thank the Meteotriveneto association, the Province of Bolzano and the Agenzia Regionale per la Prevenzione e Protezione Ambientale del Veneto for climatic data of the weather stations of Rifugio Rosetta, Solda-Madriccio and Ra Valles, respectively. We are grateful to Roberta Marchesini for her important technical support in laboratory, Arianna Guerreschi and Nicola Alessi for their help in fieldwork, and Andrew F. Speak for revising the English. We thank the Editor HH and the reviewers RV and RR for their helpful comments on a previous version of the manuscript.
Supplementary Material
The Supplementary Material for this article can be found online at: https://www.frontiersin.org/articles/10.3389/fpls.2019.00289/full#supplementary-material
Footnotes
References
ACIA (2005). Impacts on a Warming Arctic: Arctic Climate Impact Assessment. Cambridge: Cambridge University Press.
Ackerly, D. D., and Cornwell, W. K. (2007). A trait-based approach to community assembly: partitioning of species trait values into within- and among-community components. Ecol. Lett. 10, 135–145. doi: 10.1111/j.1461-0248.2006.01006.x
Albert, C. H., Grassein, F., Schurr, F. M., Vieilledent, G., and Violle, C. (2011). When and how should intraspecific variability be considered in trait-based plant ecology? Perspect. Plant Ecol. Evol. Syst. 13, 217–225. doi: 10.1016/j.ppees.2011.04.003
Bannister, P., Maegli, T., Dickinson, K. J. M., Halloy, S. R. P., Knight, A., Lord, J. M., et al. (2005). Will loss of snow cover during climatic warming expose New Zealand alpine plants to increased frost damage? Oecologia 144, 245–256. doi: 10.1007/s00442-005-0087-3
Baptist, F., Flahaut, C., Streb, P., and Choler, P. (2010). No increase in alpine snowbed productivity in response to experimental lengthening of the growing season. Plant Biol. 12, 755–764. doi: 10.1111/j.1438-8677.2009.00286.x
Beniston, M., Keller, F., Koffi, B., and Goyette, S. (2003). Estimates of snow accumulation and volume in the Swiss Alps under changing climatic conditions. Theor. Appl. Climatol. 76, 125–140. doi: 10.1007/s00704-003-0016-5
Billings, W. D., and Bliss, L. C. (1959). An alpine snowbank environment and its effects on vegetation, plant development, and productivity. Ecology 40, 388–397. doi: 10.2307/1929755
Billings, W. D., and Mooney, H. A. (1968). The ecology of arctic and alpine plants. Biol. Rev. 43, 481–529. doi: 10.1111/j.1469-185X.1968.tb00968.x
Björk, R. G., and Molau, U. (2007). Ecology of alpine snowbeds and the impact of global change. Arct. Antarct. Alp. Res. 39, 34–43. doi: 10.1657/1523-0430(2007)39[34:EOASAT]2.0.CO;2
Cannone, N., Sgorbati, S., and Guglielmin, M. (2007). Unexpected impacts of climate change on alpine vegetation. Front. Ecol. Environ. 5, 360–364. doi: 10.1890/1540-9295(2007)5[360:UIOCCO]2.0.CO;2
Carbognani, M., Bernareggi, G., Perucco, F., Tomaselli, M., and Petraglia, A. (2016). Micro-climatic controls and warming effects on flowering time in alpine snowbeds. Oecologia 182, 573–585. doi: 10.1007/s00442-016-3669-3
Carbognani, M., Petraglia, A., and Tomaselli, M. (2012). Influence of snowmelt time on species richness, density and production in a late snowbed community. Acta Oecol. 43, 113–120. doi: 10.1016/j.actao.2012.06.003
Carbognani, M., Petraglia, A., and Tomaselli, M. (2014a). Warming effects and plant trait control on the early-decomposition in alpine snowbeds. Plant Soil 376, 277–290. doi: 10.1007/s11104-013-1982-8
Carbognani, M., Tomaselli, M., and Petraglia, A. (2014b). Current vegetation changes in an alpine late snowbed community in the south-eastern Alps (N-Italy). Alp. Bot. 124, 105–113. doi: 10.1007/s00035-014-0135-x
Carbognani, M., Tomaselli, M., and Petraglia, A. (2018). Different temperature perception in high-elevation plants: new insight into phenological development and implications for climate change in the alpine tundra. Oikos 127, 1014–1023. doi: 10.1111/oik.04908
Ceplitis, A., and Bengtsson, B. O. (2004). Genetic variation, disequilibrium and natural selection on reproductive traits in Allium vineale. J. Evol. Biol. 17, 302–311. doi: 10.1046/j.1420-9101.2003.00678.x
Chalmandrier, L., Münkemüller, T., Colace, M. P., Renaud, J., Aubert, S., Carlson, B. Z., et al. (2017). Spatial scale and intraspecific trait variability mediate assembly rules in alpine grasslands. J. Ecol. 105, 277–287. doi: 10.1111/1365-2745.12658
Chelli, S., Wellstein, C., Campetella, G., Canullo, R., Tonin, R., Zerbe, S., et al. (2017). Climate change response of vegetation across climatic zones in Italy. Clim. Res. 71, 249–262. doi: 10.3354/cr01443
Choler, P. (2005). Consistent shifts in alpine plant traits along a mesotopographical gradient. Arct. Antarct. Alp. Res. 37, 444–453. doi: 10.1073/pnas.1713936115
Chuine, I. (2010). Why does phenology drive species distribution? Philos. Trans. R. Soc. B Biol. Sci. 365, 3149–3160. doi: 10.1098/rstb.2010.0142
Colucci, R. R., Giorgi, F., and Torma, C. (2017). Unprecedented heat wave in December 2015 and potential for winter glacier ablation in the eastern Alps. Sci. Rep. 7:7090. doi: 10.1038/s41598-017-07415-1
Delph, L. F., Johannsson, M. H., and Stephenson, A. G. (1997). How environmental factors affect pollen performance: ecological and evolutionary perspectives. Ecology 78, 1632–1639. doi: 10.1890/0012-9658(1997)078[1632:HEFAPP]2.0.CO;2
Díaz, S., Kattge, J., Cornelissen, J. H. C., Wright, I. J., Lavorel, S., Dray, S., et al. (2016). The global spectrum of plant form and function. Nature 529, 167–171. doi: 10.1038/nature16489
Domènech, M., Komac, B., Peñuelas, J., and Conesa, J. A. (2016). Site-specific factors influence the richness and phenology of snowbed plants in the Pyrenees. Plant Biosyst. 150, 741–749. doi: 10.1080/11263504.2014.990941
Dorji, T., Totland,Ø., Moe, S. R., Hopping, K. A., Pan, J., and Klein, J. A. (2013). Plant functional traits mediate reproductive phenology and success in response to experimental warming and snow addition in Tibet. Glob. Change Biol. 19, 459–472. doi: 10.1111/gcb.12059
Ellenberg, H. (1988). Vegetation Ecology of Central Europe, 4th Edn. Cambridge: Cambridge University Press.
Escudero, A., and Valladares, F. (2016). Trait-based plant ecology: moving towards a unifying species coexistence theory. Oecologia 180, 919–922. doi: 10.1007/s00442-016-3578-5
Galen, C., and Stanton, M. L. (1991). Consequences of emergence phenology for reproductive success in Ranunculus adoneus. Am. J. Bot. 78, 978–988. doi: 10.1002/j.1537-2197.1991.tb14502.x
Galen, C., and Stanton, M. L. (1995). Responses of snowbed plant species to changes in growing-season length. Ecology 76, 1546–1557. doi: 10.2307/1938156
Garnier, E., Navas, M. L., and Grigulis, K. (2016). Plant Functional Diversity: Organism Traits, Community Structure, and Ecosystem Properties. Oxford: Oxford University Press.
Gerdol, R., Marchesini, R., and Iacumin, P. (2017). Bedrock geology interacts with altitude in affecting leaf growth and foliar nutrient status of mountain vascular plants. J. Plant Ecol. 10, 839–850. doi: 10.1093/jpe/rtw092
Gerdol, R., Siffi, C., Iacumin, P., Gualmini, M., and Tomaselli, M. (2013). Advanced snowmelt affects vegetative growth and sexual reproduction of Vaccinium myrtillus in a sub-alpine heath. J. Veg. Sci. 24, 569–579. doi: 10.1111/j.1654-1103.2012.01472.x
Giménez-Benavides, L., Escudero, A., and Iriondo, J. M. (2007). Reproductive limits of a late-flowering high-mountain Mediterranean plant along an elevational climate gradient. New Phytol. 173, 367–382. doi: 10.1111/j.1469-8137.2006.01932.x
Giorgi, F., and Lionello, P. (2008). Climate change projections for the Mediterranean region. Glob. Planet. Change 63, 90–104. doi: 10.1016/j.gloplacha.2007.09.005
Gobiet, A., Kotlarski, S., Beniston, M., Heinrich, G., Rajczak, J., and Stoffel, M. (2014). 21st century climate change in the European Alps-A review. Sci. Total Environ. 493, 1138–1151. doi: 10.1016/j.scitotenv.2013.07.050
Gottfried, M., Pauli, H., Futschik, A., Akhalkatsi, M., Barancok, P., Alonso, J. L. B., et al. (2012). Continent-wide response of mountain vegetation to climate change. Nat. Clim. Change 2, 111–115. doi: 10.1038/nclimate1329
Grabherr, G., and Mucina, L. (eds). (1993). Die Pflanzengesellschaften Österreichs. Teil II. Natürliche Waldfreie Vegetation. Jena: Gustav Fischer Verlag.
Grytnes, J. A., Kapfer, J., Jurasinski, G., Birks, H. H., Henriksen, H., Klanderud, K., et al. (2014). Identifying the driving factors behind observed elevational range shifts on European mountains. Glob. Ecol. Biogeogr. 23, 876–884. doi: 10.1111/geb.12170
Güsewell, S. (2004). N: P ratios in terrestrial plants: variation and functional significance. New Phytol. 164, 243–266. doi: 10.1111/j.1469-8137.2004.01192.x
Heegaard, E. (2002). A model of alpine species distribution in relation to snowmelt time and altitude. J. Veg. Sci. 13, 493–504. doi: 10.1111/j.1654-1103.2002.tb02076.x
Hijmans, R. J., Cameron, S. E., Parra, J. L., Jones, P. G., and Jarvis, A. (2005). Very high resolution interpolated climate surfaces for global land areas. Int. J. Climatol. 25, 1965–1978. doi: 10.1002/joc.1276
Hülber, K., Bardy, K., and Dullinger, S. (2011). Effects of snowmelt timing and competition on the performance of alpine snowbed plants. Perspect. Plant Ecol. Evol. Syst. 13, 15–26. doi: 10.1016/j.ppees.2011.01.001
Hülber, K., Winkler, M., and Grabherr, G. (2010). Intraseasonal climate and habitat-specific variability controls the flowering phenology of high alpine plant species. Funct. Ecol. 24, 245–252. doi: 10.1111/j.1365-2435.2009.01645.x
Hulshof, C. M., Violle, C., Spasojevic, M. J., Mcgill, B., Damschen, E., Harrison, S., et al. (2013). Intra-specific and inter-specific variation in specific leaf area reveal the importance of abiotic and biotic drivers of species diversity across elevation and latitude. J. Veg. Sci. 24, 921–931. doi: 10.1111/jvs.12041
Inouye, D. W. (2008). Effects of climate change on phenology, frost damage, and floral abundance on montane wildflowers. Ecology 89, 353–362. doi: 10.1890/06-2128.1
Isard, A. S. (1986). Factors influencing soil moisture and plant community distribution on Niwot Ridge, Front Range, Colorado, U.S.A. Arct. Alp. Res. 18, 83–96. doi: 10.2307/1551216
Jung, V., Albert, C. H., Violle, C., Kunstler, G., Loucougaray, G., and Spiegelberger, T. (2014). Intraspecific trait variability mediates the response of subalpine grassland communities to extreme drought events. J. Ecol. 102, 45–53. doi: 10.1111/1365-2745.12177
Keller, F., Goyette, S., and Beniston, M. (2005). Sensitivity analysis of snow cover to climate change scenarios and their impact on plant habitats in alpine terrain. Clim. Change 72, 299–319. doi: 10.1007/s10584-005-5360-2
Klanderud, K. (2005). Climate change effects on species interactions in an alpine plant community. J. Ecol. 93, 127–137. doi: 10.1111/J.1365-2745.2004.00944.X
Klein, G., Vitasse, Y., Rixen, C., Marty, C., and Rebetez, M. (2016). Shorter snow cover duration since 1970 in the Swiss Alps due to earlier snowmelt more than to later snow onset. Clim. Change 139, 637–649. doi: 10.1007/s10584-016-1806-y
Klimešová, J., Danihelka, J., Chrtek, J., de Bello, F., and Herben, T. (2017). CLO-PLA: a database of clonal and bud-bank traits of the Central European flora. Ecology 98:1179. doi: 10.1002/ecy.1745
Koller, E. K., and Phoenix, G. K. (2017). Seasonal dynamics of soil and plant nutrients at three environmentally contrasting sites along a sub-Arctic catchment sequence. Polar Biol. 40, 1821–1834. doi: 10.1007/s00300-017-2105-4
Komac, B., Pladevall, C., Peñuelas, J., Conesa, J. V., and Domènech, M. (2015). Variations in functional diversity in snowbed plant communities determining snowbed continuity. Plant Ecol. 216, 1257–1274. doi: 10.1007/s11258-015-0506-4
Körner, C. (2003). Alpine Plant Life, Functional Plant Ecology of High Mountain Ecosystems, 2nd Edn. Berlin: Springer.
Kotlarski, S., Bosshard, T., Lüthi, D., Pall, P., and Schär, C. (2012). Elevation gradients of European climate change in the regional climate model COSMO-CLM. Clim. Change 112, 189–215. doi: 10.1007/s10584-011-0195-5
Kudo, G., Nordenhäll, U., and Molau, U. (1999). Effects of snowmelt timing on leaf traits, leaf production, and shoot growth of alpine plants: comparisons along a snowmelt gradient in northern Sweden. Écoscience 6, 439–450. doi: 10.1080/11956860.1999.11682543
Liancourt, P., Boldgiv, B., Song, D. S., Spence, L. A., Helliker, B. R., Petraitis, P. S., et al. (2015). Leaf-trait plasticity and species vulnerability to climate change in a mongolian steppe. Glob. Change Biol. 21, 3489–3498. doi: 10.1111/gcb.12934
Livensperger, C., Steltzer, H., Darrouzet-Nardi, A., Sullivan, P. F., Wallenstein, M., and Weintraub, M. N. (2016). Earlier snowmelt and warming lead to earlier but not necessarily more plant growth. AoB Plants 8, 1–15. doi: 10.1093/aobpla/plw021
Lluent, A., Anadon-Rosell, A., Ninot, J. M., Grau, O., and Carrillo, E. (2013). Phenology and seed setting success of snowbed plant species in contrasting snowmelt regimes in the Central Pyrenees. Flora 208, 220–231. doi: 10.1016/j.flora.2013.03.004
Lockwood, J. L., Cassey, P., and Blackburn, T. (2005). The role of propagule pressure in explaining species invasions. Trends Ecol. Evol. 20, 224–228. doi: 10.1016/j.tree.2005.02.004
Matteodo, M., Ammann, K., Verrecchia, E. P., and Vittoz, P. (2016). Snowbeds are more affected than other subalpine-alpine plant communities by climate change in the Swiss Alps. Ecol. Evol. 6, 6969–6982. doi: 10.1002/ece3.2354
Nicotra, A. B., Atkin, O. K., Bonser, S. P., Davidson, A. M., Finnegan, E. J., Mathesius, U., et al. (2010). Plant phenotypic plasticity in a changing climate. Trends Plant Sci. 15, 684–692. doi: 10.1016/j.tplants.2010.09.008
Pachauri, R. K., Allen, M. R., Barros, V. R., Broome, J., Cramer, W., Church, J. A., et al. (2014). Climate Change 2014: Synthesis Report, Contribution of Working Groups I, II and III to the Fifth Assessment Report of the Intergovernmental Panel on Climate Change, eds Core Writing Team, R. K. Pachauri, and L. A. Meyer (Geneva: IPCC), 151.
Pérez-Harguindeguy, N., Díaz, S., Garnier, E., Lavorel, S., Poorter, H., Jaureguiberry, P., et al. (2013). New handbook for standardised measurement of plant functional traits worldwide. Aust. J. Bot. 61, 167–234. doi: 10.1071/BT12225
Petraglia, A., Carbognani, M., and Tomaselli, M. (2013). Effects of nutrient amendments on modular growth, flowering effort and reproduction of snowbed plants. Plant Ecol. Divers. 6, 475–486. doi: 10.1080/17550874.2013.795628
Petraglia, A., Tomaselli, M., Mondoni, A., Brancaleoni, L., and Carbognani, M. (2014a). Effects of nitrogen and phosphorus supply on growth and flowering phenology of the snowbed forb Gnaphalium supinum L. Flora 209, 271–278. doi: 10.1016/j.flora.2014.03.005
Petraglia, A., Tomaselli, M., Petit Bon, M., Delnevo, N., Chiari, G., and Carbognani, M. (2014b). Responses of flowering phenology of snowbed plants to an experimentally imposed extreme advanced snowmelt. Plant Ecol. 215, 759–768. doi: 10.1007/s11258-014-0368-1
Pickering, C., Green, K., Barros, A. A., and Venn, S. (2014). A resurvey of late-lying snowpatches reveals changes in both species and functional composition across snowmelt zones. Alp. Bot. 124, 93–103. doi: 10.1007/s00035-014-0140-0
Pierce, S., Bottinelli, A., Bassani, I., Ceriani, R. M., and Cerabolini, B. E. L. (2014). How well do seed production traits correlate with leaf traits, whole-plant traits and plant ecological strategies? Plant Ecol. 215, 1351–1359. doi: 10.1007/s11258-014-0392-1
Pignatti, E., and Pignatti, S. (2014). Plant Life of the Dolomites. Berlin: Springer-Verlag. doi: 10.1007/978-3-642-31043-0
Pinheiro, J., Bates, D., DebRoy, S., Sarkar, D., and R Core Team. (2017). nlme: Linear and Nonlinear Mixed Effects Models. Available at: https://cran.r-project.org/web/packages/nlme/nlme.pdf
Pluess, A. R., Schütz, W., and Stöcklin, J. (2005). Seed weight increases with altitude in the Swiss Alps between related species but not among populations of individual species. Oecologia 144, 55–61. doi: 10.1007/s00442-005-0047-y
Poorter, H., and Remkes, C. (1990). Leaf area ratio and net assimilation rate of 24 wild species differing in relative growth rate. Oecologia 83, 553–559. doi: 10.1007/BF00317209
R Development Core Team (2014). R: A Language and Environment for Statistical Computing. Vienna: R Foundation for Statistical Computing.
Rosbakh, S., Bernhardt-Römermann, M., and Poschlod, P. (2014). Elevation matters: contrasting effects of climate change on the vegetation development at different elevations in the Bavarian Alps. Alp. Bot. 124, 143–154. doi: 10.1007/s00035-014-0139-6
Rosbakh, S., Leingärtner, A., Hoiss, B., Krauss, J., Steffan-Dewenter, I., and Poschlod, P. (2017). Contrasting effects of extreme drought and snowmelt patterns on mountain plants along an elevation gradient. Front. Plant Sci. 8:1478. doi: 10.3389/fpls.2017.01478
Rosbakh, S., Pacini, E., Nepi, M., and Poschlod, P. (2018). An unexplored side of regeneration niche: seed quantity and quality are determined by the effect of temperature on pollen performance. Front. Plant Sci. 9:1036. doi: 10.3389/fpls.2018.01036
Roscher, C., Gubsch, M., Lipowsky, A., Schumacher, J., Weigelt, A., Buchmann, N., et al. (2018). Trait means, trait plasticity and trait differences to other species jointly explain species performances in grasslands of varying diversity. Oikos 127:865. doi: 10.1111/oik.04815
Sandvik, M., and Totland, O. (2000). Short-term effects of simulated environmental changes on phenology, reproduction, and growth in the late-flowering snowbed herb Saxifraga stellaris L. Écoscience 7, 201–213. doi: 10.1080/11956860.2000.11682589
Sandvik, S. M., and Odland, A. (2014). Changes in alpine snowbed-wetland vegetation over three decades in northern Norway. Nord. J. Bot. 32, 377–384. doi: 10.1111/j.1756-1051.2013.00249.x
Schnittler, M., Pfeiffer, T., Harter, D., and Hamann, A. (2009). Bulbils contra seeds: reproductive investment in two species of Gagea (Liliaceae). Plant Syst. Evol. 279, 29–40. doi: 10.1007/s00606-008-0143-7
Schöb, C., Kammer, P. M., Choler, P., and Veit, H. (2009). Small-scale plant species distribution in snowbeds and its sensitivity to climate change. Plant Ecol. 200, 91–104. doi: 10.1007/s11258-008-9435-9
Schöb, C., Kammer, P. M., Kikvidze, Z., Choler, P., and Veit, H. (2008). Changes in species composition in alpine snowbeds with climate change inferred from small-scale spatial patterns. Web Ecol. 8, 142–159. doi: 10.5194/we-8-142-2008
Sedlacek, J., Wheeler, J. A., Cortés, A. J., Bossdorf, O., Hoch, G., Lexer, C., et al. (2015). The response of the alpine dwarf shrub Salix herbacea to altered snowmelt timing: lessons from a multi-site transplant experiment. PLoS One 10:e0122395. doi: 10.1371/journal.pone.0122395
Shimono, Y., and Kudo, G. (2003). Intraspecific variations in seedling emergence and survival of Potentilla matsumurae (Rosaceae) between alpine fellfield and snowbed habitats. Ann. Bot. 91, 21–29. doi: 10.1093/aob/mcg002
Sides, C. B., Enquist, B. J., Ebersole, J. J., Smith, M. N., Henderson, A. N., and Sloat, L. L. (2014). Revisiting Darwin’s hypothesis: does greater intraspecific variability increase species ecological breadth? Am. J. Bot. 101, 56–62. doi: 10.3732/ajb.1300284
Stanton, A. M. L., Rejmánek, M., and Galen, C. (1994). Changes in vegetation and soil fertility along a predictable snowmelt gradient in the Mosquito Gradient, Colorado, U. S. A. Arct. Alp. Res. 26, 364–374. doi: 10.2307/1551798
Steger, C., Kotlarski, S., Jonas, T., and Schär, C. (2013). Alpine snow cover in a changing climate: a regional climate model perspective. Clim. Dyn. 41, 735–754. doi: 10.1007/s00382-012-1545-3
Theurillat, J., and Guisan, A. (2001). Potential impact of climate change on vegetation in the European Alps: a review. Clim. Chang 50, 77–109. doi: 10.1023/a:1010632015572
Tomaselli, M., Lasen, C., Argenti, C., Gualmini, M., Petraglia, A., and Nascimbene, J. (2006). Studio geobotanico di due biotopi del Parco Naturale Regionale delle Dolomiti d’Ampezzo (Belluno-Italia Nordorientale). Gredleriana 6, 9–30.
Totland, Ø. (2001). Environment-dependent pollen limitation and selection on floral traits in an alpine species. Ecology 82, 2233–2244. doi: 10.1890/0012-9658(2001)082[2233:EDPLAS]2.0.CO;2
Venn, S. E., Green, K., Pickering, C. M., and Morgan, J. W. (2011). Using plant functional traits to explain community composition across a strong environmental filter in Australian alpine snowpatches. Plant Ecol. 212, 1491–1499. doi: 10.1007/s11258-011-9923-1
Venn, S. E., and Morgan, J. W. (2007). Phytomass and phenology of three alpine snowpatch species across a natural snowmelt gradient. Aust. J. Bot. 55, 450–456. doi: 10.1071/BT06003
Venn, S. E., and Morgan, J. W. (2009). Patterns in alpine seedling emergence and establishment across a stress gradient of mountain summits in south-eastern Australia. Plant Ecol. Divers. 2, 5–16. doi: 10.1080/17550870802691356
Violle, C., Enquist, B. J., McGill, B. J., Jiang, L., Albert, C. H., Hulshof, C., et al. (2012). The return of the variance: intraspecific variability in community ecology. Trends Ecol. Evol. 27, 244–252. doi: 10.1016/j.tree.2011.11.014
Walker, M. D., Ingersoll, R. C., and Webber, P. J. (1995). Effects of interannual climate variation on phenology and growth of two alpine forbs. Ecology 76, 1067–1083. doi: 10.2307/1940916
Walter, H., and Breckle, S.-W. (2002). Walter’s Vegetation of the Earth: the Ecological Systems of the Geo-Biosphere, 4th Edn. Berlin: Springer.
Watson, R. T., and Haeberli, W. (2004). Environmental threats, mitigation strategies and high-mountain areas. Ambio 13, 2–10. doi: 10.5167/uzh-62849
Wellstein, C., Chelli, S., Campetella, G., Bartha, S., Galiè, M., Spada, F., et al. (2013). Intraspecific phenotypic variability of plant functional traits in contrasting mountain grasslands habitats. Biodivers. Conserv. 22, 2353–2374. doi: 10.1007/s10531-013-0484-6
Westoby, M. (1998). A leaf-height-seed (LHS) plant ecology strategy scheme. Plant Soil 199, 213–227. doi: 10.1023/A:1004327224729
Wheeler, J. A., Hoch, G., Cortés, A. J., Sedlacek, J., Wipf, S., and Rixen, C. (2014). Increased spring freezing vulnerability for alpine shrubs under early snowmelt. Oecologia 175, 219–229. doi: 10.1007/s00442-013-2872-8
Wheeler, J. A., Schnider, F., Sedlacek, J., Cortés, A. J., Wipf, S., Hoch, G., et al. (2015). With a little help from my friends: community facilitation increases performance in the dwarf shrub Salix herbacea. Basic Appl. Ecol. 16, 202–209. doi: 10.1016/j.baae.2015.02.004
Winkler, D. E., Butz, R. J., Germino, M. J., Reinhardt, K., and Kueppers, L. M. (2018). Snowmelt timing regulates community composition, phenology, and physiological performance of alpine plants. Front. Plant Sci. 9:1140. doi: 10.3389/fpls.2018.01140
Keywords: climate change, plant functional traits, intraspecific trait variability, alpine environment, snowmelt
Citation: Tonin R, Gerdol R, Tomaselli M, Petraglia A, Carbognani M and Wellstein C (2019) Intraspecific Functional Trait Response to Advanced Snowmelt Suggests Increase of Growth Potential but Decrease of Seed Production in Snowbed Plant Species. Front. Plant Sci. 10:289. doi: 10.3389/fpls.2019.00289
Received: 21 November 2018; Accepted: 21 February 2019;
Published: 14 March 2019.
Edited by:
Heikki Hänninen, Zhejiang Agriculture and Forestry University, ChinaReviewed by:
Risto Virtanen, University of Oulu, FinlandRubén Retuerto, University of Santiago de Compostela, Spain
Copyright © 2019 Tonin, Gerdol, Tomaselli, Petraglia, Carbognani and Wellstein. This is an open-access article distributed under the terms of the Creative Commons Attribution License (CC BY). The use, distribution or reproduction in other forums is permitted, provided the original author(s) and the copyright owner(s) are credited and that the original publication in this journal is cited, in accordance with accepted academic practice. No use, distribution or reproduction is permitted which does not comply with these terms.
*Correspondence: Camilla Wellstein, camilla.wellstein@unibz.it