- 1College of Horticulture and Gardening, Yangtze University, Jingzhou, China
- 2Department of Chemistry, Faculty of Science, University of Hradec Kralove, Hradec Kralove, Czechia
A feature of arbuscular mycorrhiza is enhanced drought tolerance of host plants, although it is unclear whether host H+-ATPase activity and gene expression are involved in the physiological process. The present study aimed to investigate the effects of an arbuscular mycorrhizal fungus (AMF), Funneliformis mosseae, on H+-ATPase activity, and gene expression of trifoliate orange (Poncirus trifoliata) seedlings subjected to well-watered (WW) and drought stress (DS), together with the changes in leaf gas exchange, root morphology, soil pH value, and ammonium content. Soil drought treatment dramatically increased H+-ATPase activity of leaf and root, and AMF inoculation further strengthened the increased effect. A plasma membrane (PM) H+-ATPase gene of trifoliate orange, PtAHA2 (MW239123), was cloned. The PtAHA2 expression was induced by mycorrhization in leaves and roots and also up-regulated by drought treatment in leaves of AMF-inoculated seedlings and in roots of AMF- and non-AMF-inoculated seedlings. And, the induced expression of PtAHA2 under mycorrhization was more prominent under DS than under WW. Mycorrhizal plants also showed greater photosynthetic rate, stomatal conductance, intercellular CO2 concentration, and transpiration rate and better root volume and diameter than non-mycorrhizal plants under DS. AMF inoculation significantly increased leaf and root ammonium content, especially under DS, whereas it dramatically reduced soil pH value. In addition, H+-ATPase activity was significantly positively correlated with ammonium contents in leaves and roots, and root H+-ATPase activity was significantly negatively correlated with soil pH value. Our results concluded that AMF stimulated H+-ATPase activity and PtAHA2 gene expression in response to DS, which resulted in great nutrient (e.g., ammonium) uptake and root growth, as well as low soil pH microenvironment.
Introduction
Drought is one of the most serious environmental stresses, which severely restrains the growth and productivity of crop by destroying various physiological and biochemical processes such as nutrient absorption, photosynthesis, and cell metabolism (Zhang et al., 2020). As the initial site of damage after stress, changes in cell membrane structure negatively affect transport of various inorganic ions (Yoshida, 1991). Studies have demonstrated that cells regulated ion balances by changing the transmembrane transport of both ions and small molecules to maintain cell osmotic pressure under drought stress (DS) (Mak et al., 2014). Therefore, it is of profound significance to evaluate the transmembrane transport of key ions for revealing the mechanism of plants in response to DS.
Plasma membrane (PM) H+-ATPase is a kind of main membrane proteins widely existing in plant organelles (Małgorzata et al., 2018). Based on an electrical gradient inside and outside, H+ enters and exits the cell PM providing a driving force for the transport of small molecules, which is associated with mineral nutrient (NH4+) absorption, metabolite discharge, cytoplasmic pH regulation, cell growth, and stomata opening (Gaxiola et al., 2007). According to the “acid” growth theory, plant growth requires acidification of the cell wall space, and such acidification is derived by H+ effluxes of PM H+-ATPase, indicating that the H+ efflux is regulated by the proton pump, and thus, the acidification of the cell wall is important for root elongation (Staal et al., 2011). Similarly, optimal primary root growth and root hair development also require PM H+-ATPase to finely regulate H+ secretion of root tips (Haruta and Sussman, 2012). In Arabidopsis, a total of 11 PM H+-ATPase genes are identified and defined as AHA1–AHA11 (Arabidopsis PM H+-ATPase isoforms) (Palmgren, 2001). Among these isoforms, AHA2 is a housekeeping gene expressed at high levels and is the predominant proton pump in plant roots, contributing to the pH homeostasis and root growth and development (Młodziñska et al., 2014).
Arbuscular mycorrhizal fungi (AMF) form symbiotic association with roots of most terrestrial plants. Host plants transfer carbohydrates to AMF for mycelial growth and spore development; on the other hand, mycorrhizal hyphae enable to absorb nutrients from the soil to the host, thus promoting plant growth and nutrient acquisition (Aggarwal et al., 2011). It has been reported that AMF increased water absorption, nutrient absorption, photosynthesis, root structure, antioxidant defense systems, polyamine and fatty acid homeostasis, osmotic adjustment, aquaporin expression, soil structure, and hormone balance to resist soil water deficit (Wu et al., 2013, 2019; Fernández-Lizarazo and Moreno-Fonseca, 2016; He et al., 2020; Zou et al., 2020). These reactions are the result of a combination of nutritional, physical, and cellular effects. In addition, studies have shown that hyphal H+ effluxes and spores growth rate were stimulated during the presymbiotic development of Gigaspora margarita, which is related to phosphorus and sucrose deficiency (Ramos et al., 2008b). And, in the symbiosis of maize and mycorrhizal fungi, the H+ pump activity of the host plant was differently regulated by AMF (Ramos et al., 2009). Liu et al. (2020) reported that the tomato AM-specific H+-ATPase, SIHA8, was evolutionarily conserved in maintaining arbuscule development. In tobacco, Gianinazzi-Pearson et al. (2000) reported the expression of H+-ATPase located in cortical parenchyma of AMF-colonized cells only. In addition, two P-type HA genes GmHA5 and GmPMA1 were encoded in Glomus mosseae (Ferrol et al., 2000; Requena et al., 2003). However, the relationship between AMF and PM H+-ATPase gene (especially AHA2) expressions of host plants under water deficit is not yet known.
Here, we hypothesized that AMF potentially regulated PM H+-ATPase activity and AHA2 gene expression under DS, thus promoting root growth and nutrient (NH4+) acquisition to tolerate soil drought. To confirm the above hypothesis, trifoliate orange (Poncirus trifoliata L. Raf., a drought-sensitive citrus rootstock) was selected and inoculated with an AMF Funneliformis mosseae. Leaf gas exchange, root morphology, H+-ATPase activity and AHA2 gene expression in leaf and root, soil pH value, and ammonium content of trifoliate orange were determined under well-watered (WW) and DS.
Materials and Methods
Experimental Design
The experiment was a completely random design with two soil water regimes (WW and DS) and two AMF inoculations (+AMF and -AMF). Each treatment had eight replicates, in a total of 32 pots (three seedlings per pot).
Mycorrhizal Fungal Inoculants
Funneliformis mosseae (Nicol. and Gerd.) C. Walker & A. Schüβler [BGC XJ02] was selected. The arbuscular mycorrhizal fungal strain was provided by the Bank of Glomales in China (BGC) and propagated with white clover as its host for 3 months under greenhouse and potted conditions. At harvest time, the shoot of the white clover was removed, and roots and growth substrates were collected as the mycorrhizal inoculants, which contained the fungal spores (22 spores/g), sporocarps, mycorrhizal hyphae, and colonized roots.
Plant Culture
The four-leaf-old trifoliate orange seedling that was grown in autoclaved sand in an incubator at 26°C was transferred into a 1.4-L plastic pot containing 1.2 kg of autoclaved soil and sand (5:3, vol/vol). The soil properties had been described in detail in Cheng et al. (2021). At the time of transplanting the seedlings, 100 g of mycorrhizal inoculums was applied into trifoliate orange seedlings as the AMF treatment. The non-AMF treatment received 100 g autoclaved (0.11 MPa, 121°C, 1.5 h) mycorrhizal inoculums plus 2-mL filtrates (25-μm filters) of inoculums.
Before soil drought began, potted soil water maintained 75% maximum field water-holding capacity (corresponding to WW) by weighing the pots every day. After 11 weeks, half of the mycorrhizal and non-mycorrhizal plants were exposed to the 55% maximum field water-holding capacity (corresponding to DS) for 8 weeks, and the other half was continued to grow in soil with WW status for 8 weeks. Thereafter, these treated seedlings were harvested. During the experiment, positions of the pots were swapped weekly to reduce the environmental impact. The plants were grown in a greenhouse from March 24 to August 5, 2019, where the day/night temperature was 28°C/21°C, relative humidity 68%, and photon flux density 880 μmol/m2 per second.
Determination of Leaf Gas Exchange
On a sunny morning from 9:00 to 11:00 am before the plants were harvested, leaf gas exchange was determined using a Li-6400 Portable Photosynthesis System (Li-Cor Inc., Lincoln, NE, United States) in the fully extended leaf. Photosynthetic rate (Pn), stomatal conductance (gs), intercellular CO2 concentration (Ci), transpiration rate (Tr), and leaf temperature were recorded after steady state of gas exchange.
Determination of Root Mycorrhizal Colonization and Root Morphology
Root mycorrhizas were stained by 0.05% trypan blue as described by Phillips and Hayman (1970). Root mycorrhizal colonization degree was assessed as the percentage of root lengths colonized by mycorrhizal fungi against total observed root lengths. The intact roots were carefully scanned by the Epson Perfection V700 Photo Dual Lens System (J221A, Seiko Epson Corporation, Jakarta, Indonesia), and then the obtained root figures were analyzed with a WinRHIZO professional software (Regent Instruments Inc., Quebec, Canada) for root area, volume, and average diameter.
Determination of H+-ATPase Activity in Leaf and Root
The activity of H+-ATPase in leaf and root was determined by the enzyme-linked immunosorbent assay (ELISA). A 0.3-g fresh plant sample was ground under liquid nitrogen, incubated with phosphate buffer (pH 7.4), and centrifuged at 8,000g for 30 min. The supernatant was collected, and the H+-ATPase activity was assayed by the H+-ATPase ELISA kits (ml73629, Shanghai Enzyme-linked Biotechnology Co., Ltd., Shanghai, China) according to the user’s guide.
Polymerase Chain Reaction Amplification and Sequence Analysis of PtAHA2 (a PM H+-ATPase Gene)
Based on the known AHA2 (Arabidopsis PM H+-ATPase isoform 2) gene sequence (AT4G30190) in Arabidopsis thaliana and the BLAST result of sweet orange genome1, a pair of primers (F: 5′-CCCAACAAGCTAGAA GAGAAAAAG-3′; R: 5′-CGCGAGTAATGTTTTTTCCTTCTG-3′) were designed with the most homologous gene sequence (Cs4g03700.1) for open reading frame amplification.
Total RNA was extracted and purified from leaves and roots of trifoliate orange seedlings with the EASY spin Plus plant RNA kit (RN 38, Aidlab Biotecnolohies Co., Ltd., Beijing, China). After determining the concentration and purity of the isolated RNA by spectrophotometers at 260 and 280 nm, the RNA was reverse-transcribed into cDNA using the kit PrimeScriptTM RT reagent kit with a gDNA eraser (PK02006, TaKaRa Bio. Inc., Tokyo, Japan). The polymerase chain reaction (PCR) amplification was initiated using the Planta max super-fidelity DNA polymerase kit (Vazyme Biotech Co., Ltd., Nanjing, China) and recovered the destination fragment by the universal DNA purification kit (TianGen Biotech Beijing Co., Ltd., Beijing, China). The pEASY®-Blunt Zero Cloning Kit (Beijing TransGene Biotech Co., Ltd., Beijing, China) was used for the ligation and transformation of the target fragment. Afterward, it was screened on LB plates coated with kanamycin, and positive clones were isolated by PCR for sequencing. DNAMAN5.2.2 (Lynnon Biosoft, Quebec, Canada) and MEGA 72 were used to analyze the sequence alignment and the phylogenetic tree analysis, respectively.
Relative Expressions of PtAHA2
The primer was designed based on the predicted PM PtAHA gene sequence. Accumulation of transcript was measured by quantitative real-time PCR (Light Cycler480 System, Roche Diagnostics, Switzerland) using the TB Green premix ExTM TaqII for fluorescence quantitative analysis. Gene-specific primer sequences of PtAHA2 gene were as follows: F: 5′-GATGGTAAATGGAGTGAGGAAGAAG-3′; R: 5′-GGATTTTTGGTGACAGGAAGAGAT T-3′. The β-actin gene (Cs1g05000; forward: 5′-CCGACCGTATGAGCAAGGAAA-3′; reverse: 5′-TTCCTGTGGACAATGGATGGA-3′) was used as the housekeeping gene. The expression level of PtAHA2 was normalized to the expression level of non-AMF plants exposed to WW. The 2–ΔΔCT method was used to calculate the relative quantification (Livak and Schmittgen, 2001).
Determination of Soil pH Value
A 10-g air-dried soil sample through 2-mm sieves was mixed with 25 mL distilled water without CO2 (soil:water = 1:2.5) and shaken well with a magnetic stirrer. After 30 min, the pH value of the supernatant was determined using a pH meter (pH828+, Smart Sensor, Dongguan, China) after precalibration.
Determination of Ammonium Content in Leaf and Root
Ammonium content in leaf and root was determined by the ninhydrin method described by Tang (1999). A 0.5-g fresh sample was ground with 5 mL of 10% acetic acid and filtered. Afterward, 2-mL filtrate was mixed with 3 mL of the ninhydrin reagent (C9H4O3⋅H2O)and 0.1 mL of 1% ascorbic acid solution at 100°C for 15 min. After cooling, the absorbance of the solution was determined at 580 nm.
Statistical Analysis
All the data were analyzed with the analysis of variation according to SAS software (SAS Institute, Inc., Cary, NC, United States), and significant differences between treatments were compared by the Duncan multiple-range tests at p = 0.05 level. The Pearson correlation coefficient between two specified variables was analyzed with SAS software.
Results
Changes in Root Mycorrhizal Colonization and Root Morphology
Mycorrhizal fungal colonization was not found in the roots of uninoculated plants, whereas the root mycorrhizal colonization rate of the inoculated plants was 46.91% ± 1.86% under WW and 34.11% ± 7.10% under DS, respectively. The DS treatment significantly reduced root mycorrhizal colonization, compared with the WW treatment. Drought treatment also inhibited root morphology to a certain extent, and F. mosseae inoculation partly mitigated the inhibitive effect (Figures 1A–C). Compared with non-AMF treatment, AMF inoculation increased root average diameter and volume, respectively, by 23.53 and 119.78% under WW and by 15.87 and 172.11% under DS, respectively (Figures 1A,C). Mycorrhizal fungal treatment did not significantly affect root surface area, regardless of soil water regimes (Figure 1B).
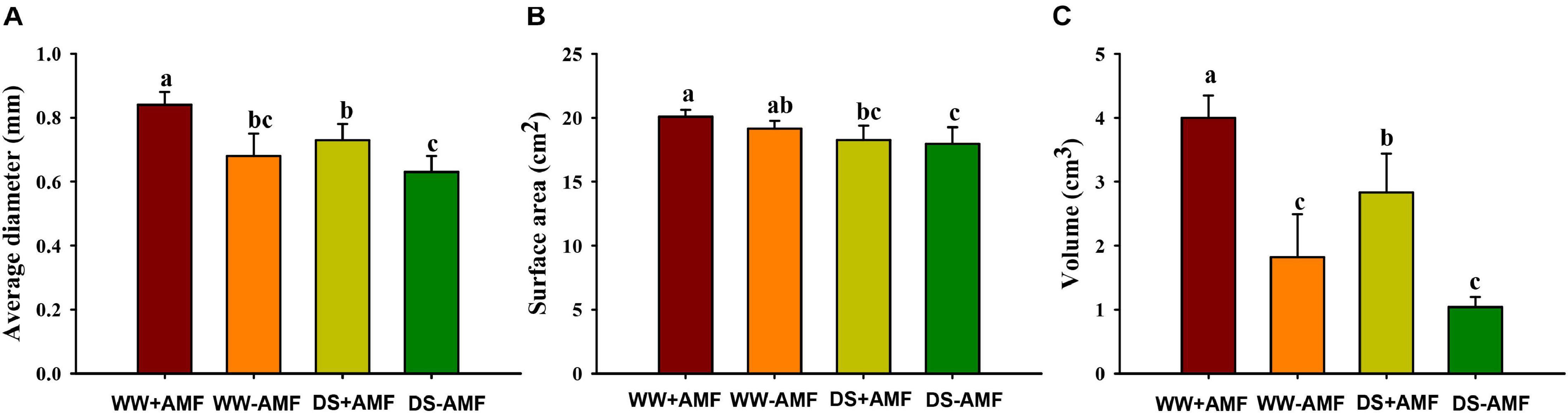
Figure 1. Effects of Funneliformis mosseae on root average diameter (A), root surface area (B), and root volume (C) of trifoliate orange (Poncirus trifoliata) seedlings subjected to well-watered (WW) and drought stress (DS). Data (means ± SD, n = 4) followed by different letters above the bars indicate significant differences (P < 0.05) between treatments.
Changes in Leaf Gas Exchange
Drought treatment significantly decreased leaf Pn, gs, Ci, and Tr, compared with WW treatment (Table 1). Compared with non-mycorrhizal fungal plants, F. mosseae–inoculated plants recorded significantly higher leaf Pn, gs, Ci, and Tr by 118.80, 237.93, 45.61, and 237.70% under WW and by 141.15, 103.49, 60.70, and 134.04% under DS, respectively. In addition, leaf temperature was markedly reduced by F. mosseae inoculation by 1.67% under WW only.

Table 1. Effects of Funneliformis mosseae on leaf gas exchange of trifoliate orange (Poncirus trifoliata) seedlings subjected to well-watered (WW) and drought stress (DS).
Changes in Leaf and Root H+-ATPase Activity
Soil drought treatment significantly increased H+-ATPase activity in leaf and root, irrespective of AMF inoculation or not (Figure 2). On the other hand, AMF colonization distinctly enhanced H+-ATPase activity in leaf by 27.37 and 26.06% and in root by 22.14 and 22.61% under WW and DS conditions, respectively, compared with non-AMF colonization.
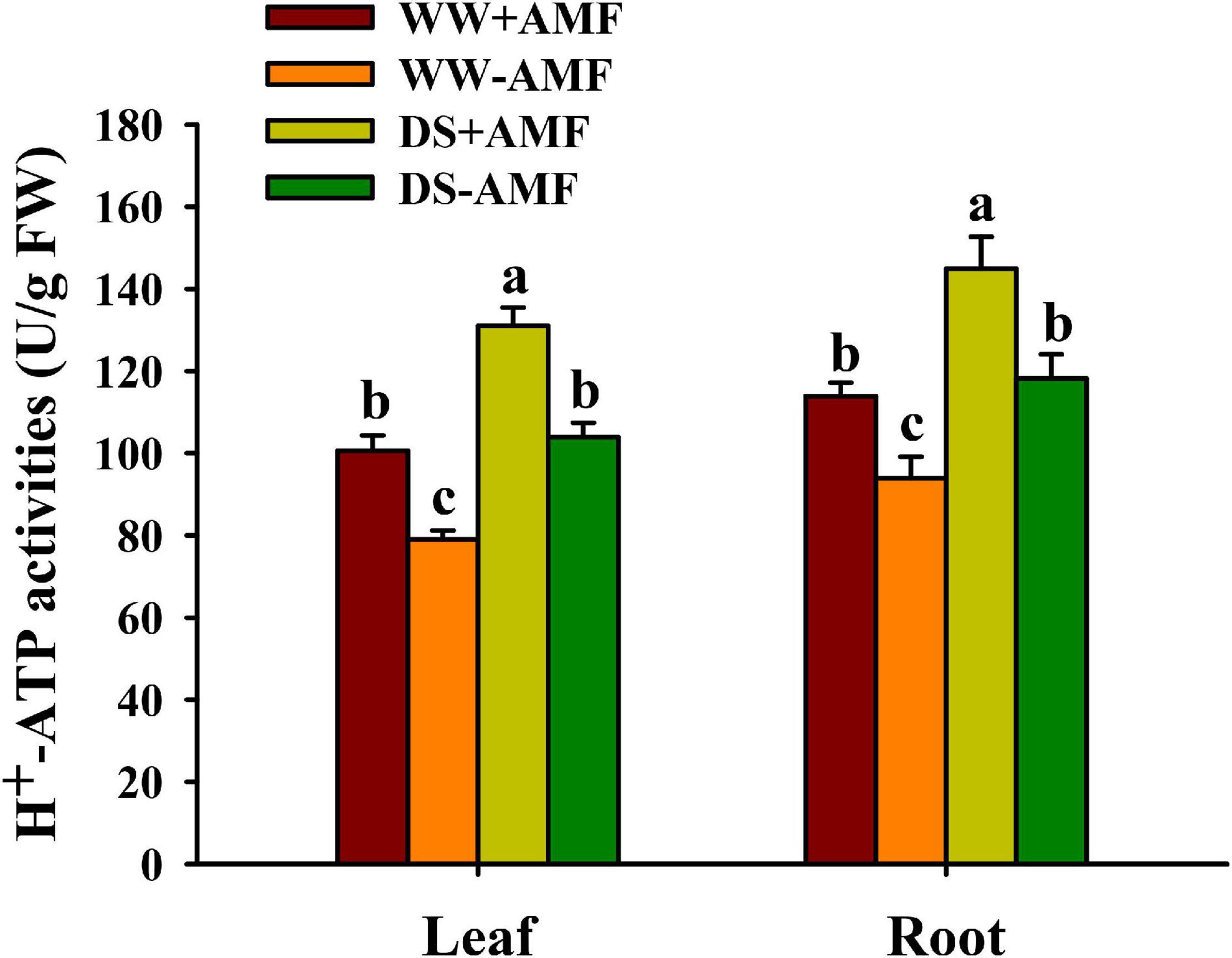
Figure 2. Effects of Funneliformis mosseae on leaf and root H+-ATP activities of trifoliate orange (Poncirus trifoliata) seedlings subjected to well-watered (WW) and drought stress (DS). Data (means ± SD, n = 4) followed by different letters above the bars indicate significant differences (P < 0.05) between treatments.
Sequence Analyses of PtAHA2
A total of 2,965 bp in PM H+-ATPase gene (PtAHA2) encoding 885 amino acids were cloned from trifoliate orange, based on sweet orange database and homologous cloning. A GenBank accession number of PtAHA2 was MW239123. The sequencing of the PtAHA2 showed that the protein sequence homology between trifoliate orange and sweet orange was 99.66% by DNAMAN (Supplementary Material 1), suggesting that trifoliate orange has a high homology with sweet orange. In addition, multiple sequence alignment indicated that the sequence homology of PtAHA2 proteins with other AHA2 families was 83.72% (Figure 3), indicating that HA2-type proteins have high sequence conservation. Based on the phylogenetic tree analysis of PtAHA2, five H+-ATPase genes in Arabidopsis (AtAHA2, AtAHA6, AtAHA7, AtAHA4, and AtAHA10) and five H+-ATPase genes in Oryza sativa (OsAHA7, OsAHA6, OsAHA8, OsAHA1, and OsAHA9) from families I to V by MEGA 7, PtAHA2 belonged to subfamily I, which was clustered with OsAHA7 and AtAHA2 (Supplementary Material 2).
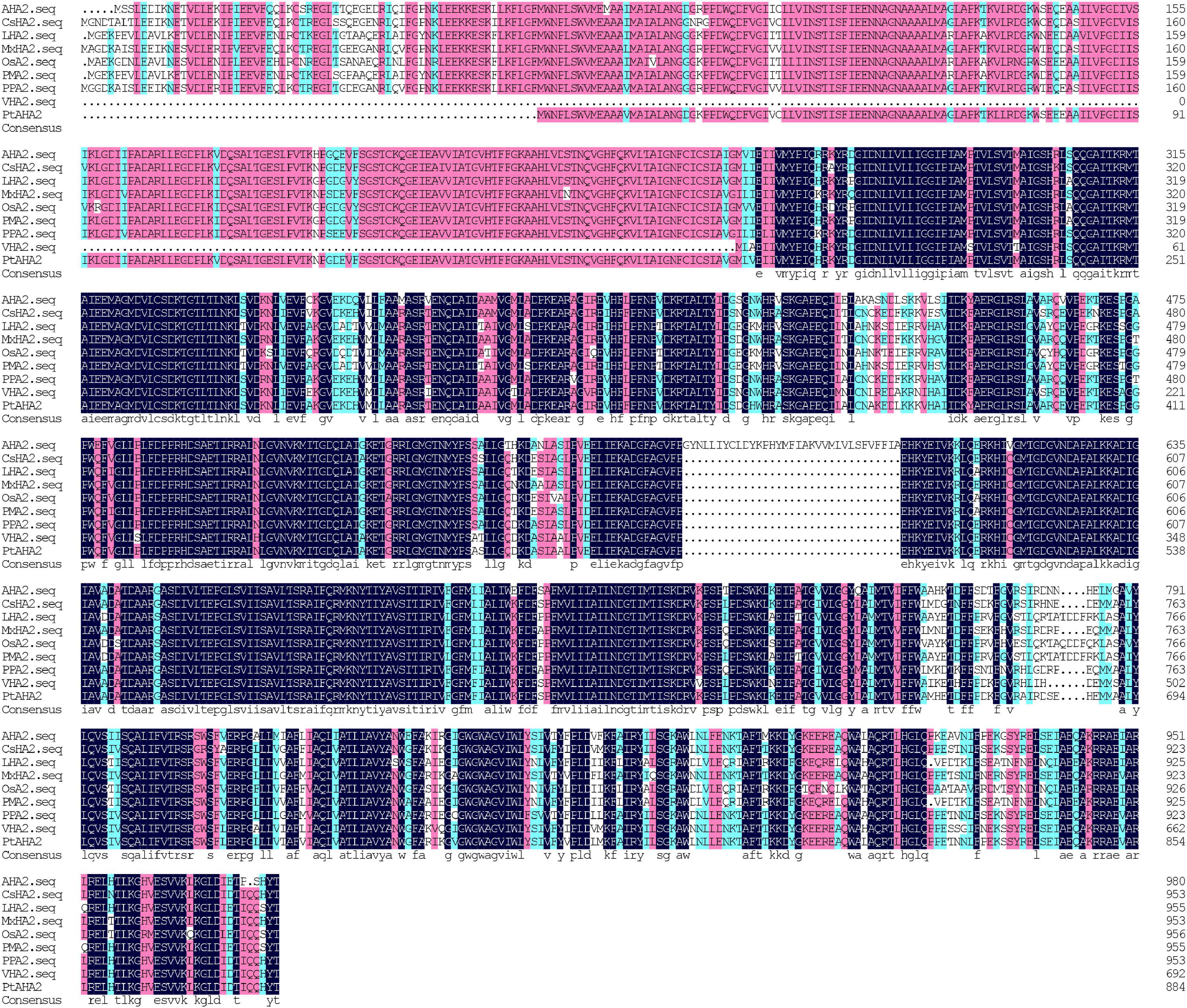
Figure 3. Sequence alignments of PtAHA2 with HA2 proteins from other plants. AHA2: Arabidopsis thaliana; CsHA2: Cucumis sativus; LHA2: Lycopersicon esculentum; MxHA2: Malus xiaojinensis; OSA2: Oryza sativa; PPA2: Prunus persica; VHA2: Vicia faba; PtAHA2: Poncirus trifoliata.
Changes in PtAHA2 Gene Expression
Compared with WW treatment, DS treatment did not affect the PtAHA2 expression in leaves of non-mycorrhizal plants, while it induced the expression of PtAHA2 in leaves of mycorrhizal seedlings and in roots of mycorrhizal and non-mycorrhizal seedlings (Figure 4). Moreover, the expression of PtAHA2 in roots was relatively higher than in leaves. In addition, leaf and root PtAHA2 gene expression was increased under mycorrhization by 1.62- and 9.50-fold under WW and by 5.62- and 20.92-fold under DS, respectively.
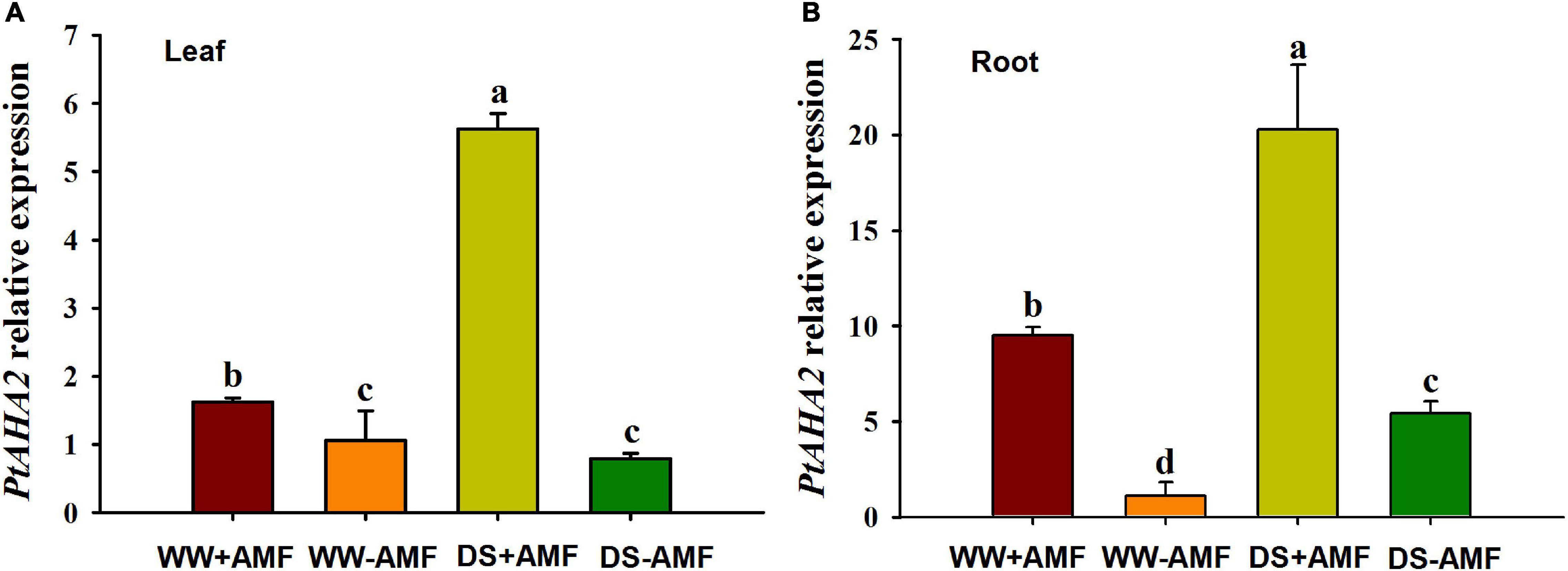
Figure 4. Effects of Funneliformis mosseae on relative expression of leaf (A) and root (B) plasma membrane H+-ATPase gene PtAHA2 in trifoliate orange (Poncirus trifoliata) seedlings subjected to well-watered (WW) and drought stress (DS). Data (means ± SD, n = 3) followed by different letters above the bars indicate significant differences (P < 0.05) between treatments.
Changes in Soil pH Value
Soil pH value was affected by mycorrhization, but not soil drought treatment (Figure 5). Compared with non-AMF inoculation, AMF inoculation significantly reduced soil pH value by 12.04 and 14.67% under WW and DS, respectively.
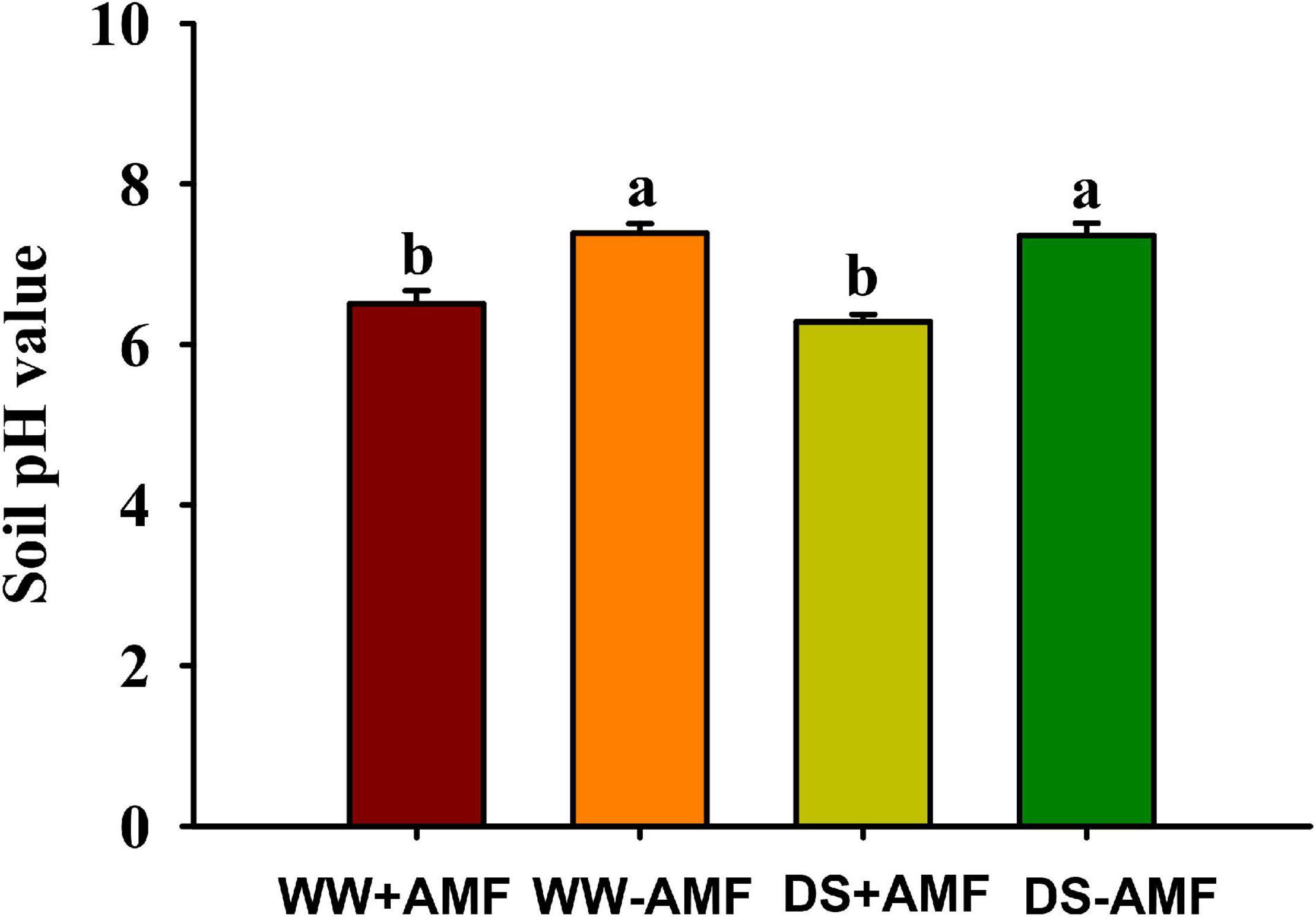
Figure 5. Effects of Funneliformis mosseae on soil pH value in trifoliate orange (Poncirus trifoliata) seedlings subjected to well-watered (WW) and drought stress (DS). Data (means ± SD, n = 4) followed by different letters above the bars indicate significant differences (P < 0.05) between treatment.
Changes in Leaf and Root Ammonium Content
Leaf and root ammonium contents were not significantly affected by soil DS treatment, irrespective of mycorrhizal and non-mycorrhizal plants (Figure 6). AMF colonization significantly enhanced the absorption of ammonium in leaf and root by 26.79 and 43.33% under DS, respectively, compared with non-AMF colonization. Under WW, AMF-inoculated plants recorded 17.65% significantly higher leaf ammonium content than non-AMF-inoculated plants, although no significant difference in root ammonium content was found between AMF- and non-AMF-inoculated plants.
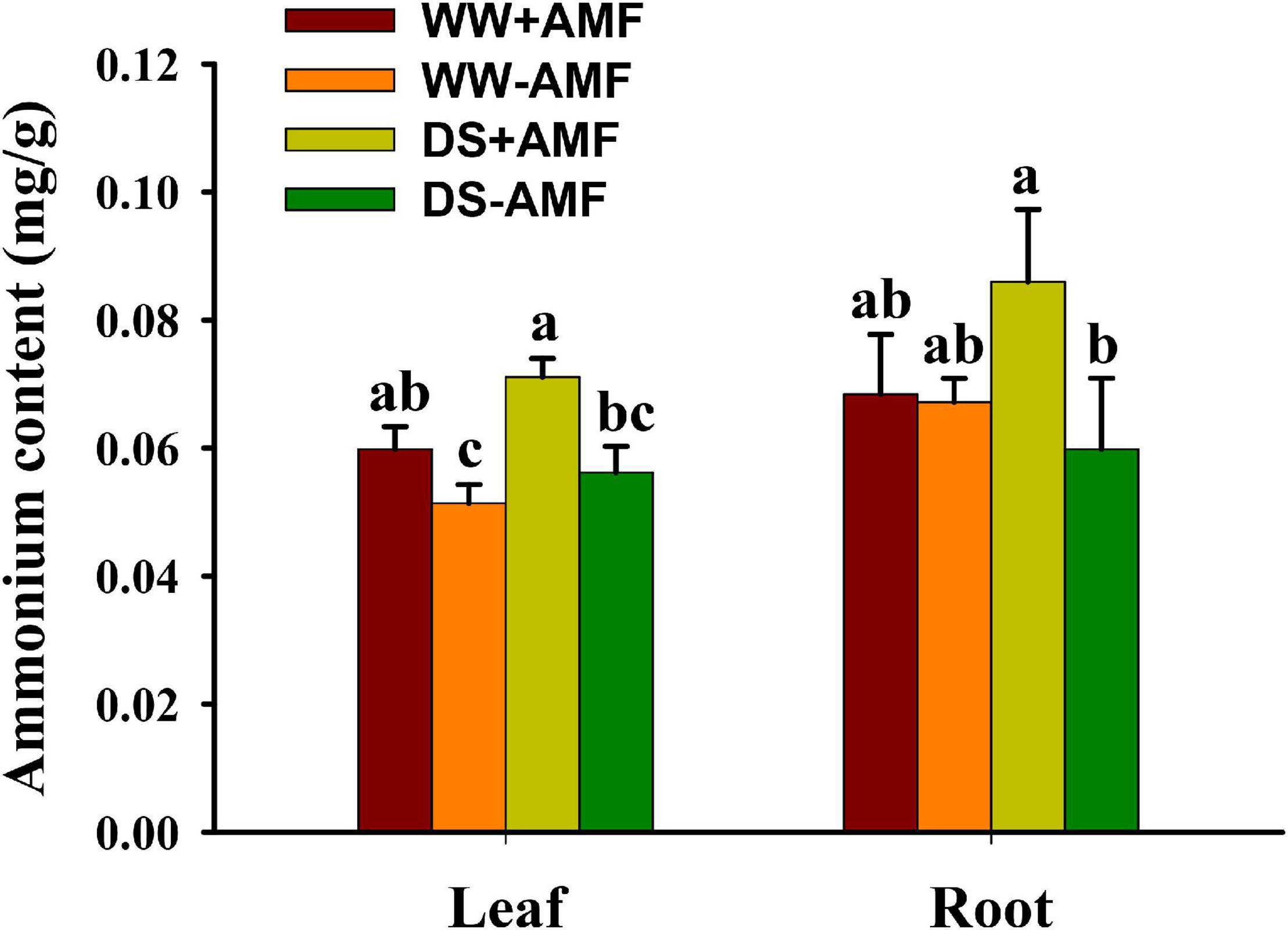
Figure 6. Effects of Funneliformis mosseae on leaf and root ammonium contents in trifoliate orange (Poncirus trifoliata) seedlings subjected to well-watered (WW) and drought stress (DS). Data (means ± SD, n = 4) followed by different letters above the bars indicate significant differences (P < 0.05) between treatment.
Correlation Studies
Based on the correlation analysis between H+-ATPase and selective variables, we found that leaf H+-ATPase activity was significantly (P < 0.01) positively correlated with leaf ammonium content (Figure 7A). Root H+-ATPase activity was significantly (P < 0.05) positively correlated with root ammonium content (Figure 7B) and negatively (P < 0.01) correlated with soil pH value (Figure 7C).
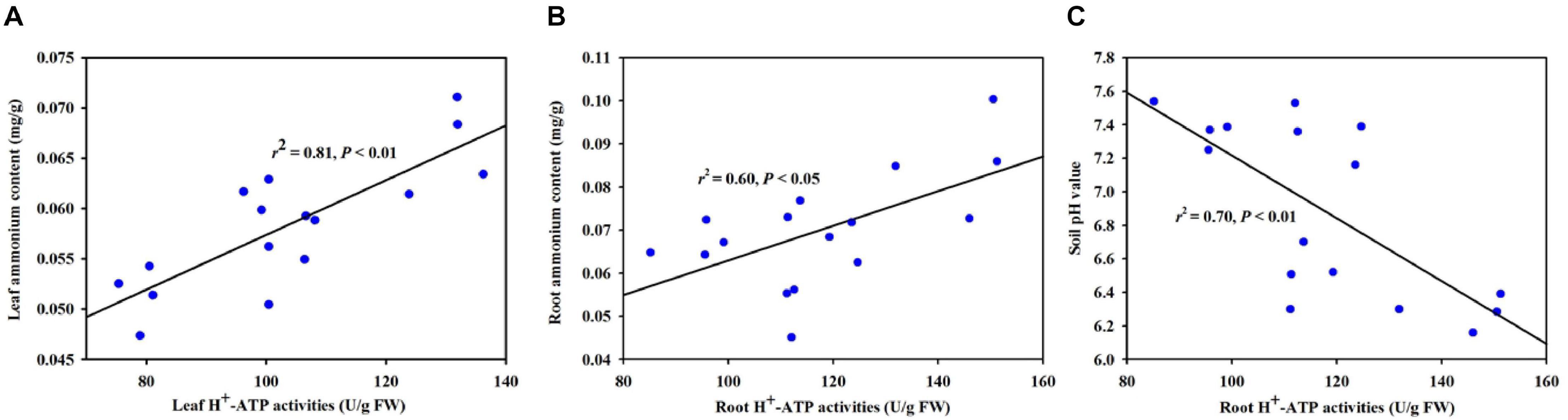
Figure 7. Linear regression between H+-ATPase activity and ammonium content in leaf (A) and root (B) and between root H+-ATPase activity and soil pH value (C) (n = 16).
Discussion
Earlier studies showed that mycorrhizal plants had greater leaf gas exchange capacity than non-mycorrhizal plants subjected to abiotic stress (e.g., DS and salt stress) (Porcel and Ruiz-Lozano, 2004; Xiong et al., 2007; Augé et al., 2015). Our study also confirmed that Pn, gs, Ci, and Tr of mycorrhizal trifoliate orange seedlings were significantly higher than that of non-mycorrhizal seedlings under both WW and DS, implying that mycorrhizal symbiosis has the ability to improve leaf gas exchange of the host under DS. As a result, mycorrhizal plants might produce more carbohydrates for the growth of both the host and the mycorrhizal fungus and also stimulate the transport of water exposed to DS (Hadian-Deljou et al., 2020). In addition, H+-ATPase genes such as AHA2 were highly expressed in guard cells of Arabidopsis for stomatal opening (Ueno et al., 2005). Overexpression of AHA2 in guard cells of Arabidopsis could manipulate stomatal opening, thereby improving plant growth (Wang et al., 2014). In the present study, we concluded that the up-regulated expression of PtAHA2 in leaf by mycorrhization may be associated with better leaf gas exchange of mycorrhizal plants, especially stomatal opening under DS.
Plant roots contact the soil directly to respond to environmental stress (Ingrain and Malamy, 2010; Franco et al., 2011). The present results showed that the inoculation of F. mosseae substantially improved root average diameter and volume under both WW and DS, compared with the non-AMF inoculation. Analogous results have been reported by Zou et al. (2017) in trifoliate orange. It is documented that PM AHA2 is very important for root growth and development, as shown by shorter and lower primary and lateral root length in aha2 mutant lines of A. thaliana versus wild-type plants (Młodziñska et al., 2014). Hence, under WW and DS, F. mosseae inoculation induced the activity and gene expression of PtAHA2 in roots, which potentially stimulates the growth of primary and lateral root, resulting in greater root volume in mycorrhizal plants. As shown in this study, the rhizosphere of mycorrhizal trifoliate orange plants had a low pH environment (Figure 7), and indoleacetic acid can be protonized to expand the membrane for transport into the cells, thereby accelerating root growth (Petrasek and Friml, 2009). Therefore, mycorrhizal plants have strong adaptability by optimizing the root morphology to potentially mitigate drought damage (Chu et al., 2014).
In this study, we observed that soil drought treatment dramatically increased leaf and root H+-ATPase activity in AM and non-AM plants, indicating that trifoliate orange seedlings could rapidly adapt to soil drought environment by enhancing the activity of H+-ATPase in leaf and root. Furthermore, mycorrhizal trifoliate orange seedlings recorded significantly higher H+-ATPase activity in leaf and root under both WW and DS conditions, suggesting that H+-ATPase activity of trifoliate orange could be induced by AMF.
The PM H+-ATPase is a large gene family that exhibits expression overlap and functional redundancy, but AHA1 and AHA2 are highly expressed in almost all tissues and organs (Haruta et al., 2010). Studies by Haruta et al. (2010) showed that single knockout of AHA1 or AHA2 did not represent an obvious phenotype, but double knockout is lethal. In our study, PtAHA2 expression was higher in roots than in leaves, possibly because AHA2 had a strong signal in epidermal and cortex cells of roots, as well as in phloem, xylem, and root hairs (Gianinazzi-Pearson et al., 2000; Fuglsang et al., 2007). The PtAHA2 expression was induced by soil drought, especially in roots. The AMF-inoculated seedlings recorded dramatically higher expression of PtAHA2 gene in leaf and root than the non-AMF-inoculated seedlings. And the induced expression of PtAHA2 under mycorrhization was more prominent under DS than under WW. Thus, PtAHA2 showed an AMF-specific expression profile. Liu et al. (2016) also analyzed the relative transcript of levels of four tomato H+-ATPase (HA) genes (SlHA1, SlHA2, SlHA4, and SlHA8) and found that SlHA1 and SlHA4 in leaf and root were not affected by mycorrhization, SlHA2 was up-regulated by mycorrhization only in root, and SlHA8 was expressed in root and activated by mycorrhization. This implied that plant HA expression could be regulated by mycorrhization, dependent on host plants and HA homologous genes. Further work should analyze the expression of HA genes in both host plants and mycorrhizal fungi (e.g., GmHA5 and GmPMA1) in response to DS, which is cooperative or competitive.
In our study, we found lower soil pH value in rhizosphere of mycorrhizal plants versus non-mycorrhizal plant, regardless of soil water regimes. Additionally, soil pH value was significantly negatively correlated with root H+-ATPase activity, because H+-ATPase releases protons into rhizosphere, resulting in a low pH environment (Chen et al., 2017). Acidic rhizosphere under environmental stress conditions is important to the nutrient availability in soil (Ramos et al., 2008a). The H+ electrochemical gradient generated by H+-ATPase provides driving forces for nutrients and solutes on the symbiotic membranes and participates in nutrient transfer (Garry et al., 2007). Liu et al. (2020) further analyzed the SlHA8 expression in tomato and found that the SlHA8 expression was essential for arbuscule development and symbiotic N uptake. In tobacco, H+-ATPase gene was expressed in AMF-colonized cortical cells only (Gianinazzi-Pearson et al., 2000). In Medicago truncatula, a ha1-2 mutant was isolated and impaired in arbuscule development, but not in root colonization of Rhizophagus irregularis (Krajinski et al., 2014). The AMF-induced HA gene was mainly localized in arbuscule-containing cells, where it is the nutrient unloading interface between AMF and hosts. When H+ ions are released into rhizosphere, plants also absorb ammonium ions into the root to maintain the charge balance (Motte and Beeckman, 2020). Our study also showed that the inoculation with F. mosseae dramatically improved ammonium content in leaf under WW and DS and in root under DS, as compared with non-AMF inoculation. Moreover, H+-ATPase activity was significantly positively correlated with ammonium content. Sun et al. (1999) observed that the activation of the proton pump in mycorrhizal hyphae under drought conditions regulated the rhizospheric microenvironment, resulting in inorganic ions accumulation in the soil. Therefore, the increase of ammonium ions in mycorrhizal plants is related to the induced expression of host H+-ATP gene by mycorrhizal fungi, in addition to mycorrhizal hyphal absorption (Johansen et al., 2010).
Conclusion
In short, we cloned a H+-ATPase gene of trifoliate orange, PtAHA2, which is evolutionarily conserved. The PtAHA2 expression distinctly increased under mycorrhization and soil DS, along with higher H+-ATPase activity in AM plants versus non-AM plants. The increase in PtAHA2 gene expression and H+-ATPase activity under mycorrhization was closely associated with root growth (e.g., root volume and average diameter), nutrient acquisition (ammonium), and leaf gas exchange (e.g., stomatal conductance), which are critical for enhanced drought tolerance of plants. Further analysis of mycorrhiza-specific PtAHA2 on mycorrhizal plants by molecular techniques such as gene knockout and screening for upstream transcription factors can help us understand the function of PtAHA2 in response to mycorrhization and DS.
Data Availability Statement
The original contributions presented in the study are included in the article/Supplementary Material, further inquiries can be directed to the corresponding authors.
Author Contributions
H-QC and Q-SW conceived and designed the experiments. H-QC performed the experiments. H-QC, Y-NZ, Q-SW, and KK analyzed the data. H-QC and Y-NZ prepared the figures. H-QC wrote the manuscript. Q-SW and KK revised the manuscript. All authors contributed to the article and approved the submitted version.
Funding
This study was supported by the National Key Research and Development Program of China (2018YFD1000303) and the Plan in Scientific and Technological Innovation Team of Outstanding Young Scientists, Hubei Provincial Department of Education, China (T201604). This work was also supported by the UHK project VT2019-2021.
Conflict of Interest
The authors declare that the research was conducted in the absence of any commercial or financial relationships that could be construed as a potential conflict of interest.
Supplementary Material
The Supplementary Material for this article can be found online at: https://www.frontiersin.org/articles/10.3389/fpls.2021.659694/full#supplementary-material
Footnotes
References
Aggarwal, A., Kadian, N., Tanwar, A., Yadav, A., and Gupta, K. (2011). Role of arbuscular mycorrhizal fungi (AMF) in global sustainable development. J. Appl. Nat. Sci. 3, 340–351. doi: 10.31018/jans.v3i2.211
Augé, R. M., Toler, H. D., and Saxton, A. M. (2015). Arbuscular mycorrhizal symbiosis alters stomatal conductance of host plants more under drought than under amply watered conditions: a meta-analysis. Mycorrhiza 25, 13–24. doi: 10.1007/s00572-014-0585-4
Chen, H., Zhang, Q., Cai, H., and Xu, F. (2017). Ethylene mediates alkaline-induced rice growth inhibition by negatively regulating plasma membrane H+-ATPase activity in roots. Front. Plant Sci. 8:1839. doi: 10.3389/fpls.2017.01839
Cheng, H. Q., Giri, B., Wu, Q. S., Zou, Y. N., and Kuča, K. (2021). Arbuscular mycorrhizal fungi mitigate drought stress in citrus by modulating root microenvironment. Arch. Agron. Soil Sci. doi: 10.1080/03650340.2021.1878497. [Epub ahead of print].
Chu, G., Chen, T. T., Wang, Z. Q., Yang, J. C., and Zhang, J. H. (2014). Morphological and physiological traits of roots and their relationships with water productivity in water-saving and drought-resistant rice. Field Crop Res. 162, 108–119. doi: 10.1016/j.fcr.2013.11.006
Fernández-Lizarazo, J. C., and Moreno-Fonseca, L. P. (2016). Mechanisms for tolerance to water-deficit stress in plants inoculated with arbuscular mycorrhizal fungi. A review. Agron. Colomb. 34, 179–189. doi: 10.15446/agron.colomb.v34n2.55569
Ferrol, N., Barea, J. M., and Azcón-Aguilar, C. (2000). The plasma membrane H+-ATPase gene family in the arbuscular mycorrhizal fungus Glomus mosseae. Curr. Genet. 37, 112–118. doi: 10.1007/s002940050017
Franco, J. A., Bañón, S., Vicente, M. M. J., Miralles, J., and Martínez-Sánchez, J. J. (2011). Review article:root development in horticultural plants grown under abiotic stress conditions-a review. J. Hort. Sci. Biotechnol. 86, 543–556. doi: 10.1080/14620316.2011.11512802
Fuglsang, A. T., Guo, Y., Cuin, T. A., Qiu, Q., Song, C., Kristiansen, K. A., et al. (2007). Arabidopsis protein kinase PKS5 inhibits the plasma membrane H+-ATPase by preventing interaction with 14-3-3 protein. Plant Cell 19, 1617–1634. doi: 10.1105/tpc.105.035626
Garry, M., Rosewarne, F., Andrew Smith, D. P., and Schachtman, S. E. (2007). Localization of proton-atpase genes expressed in arbuscular mycorrhizal tomato plants. Mycorrhiza 17, 249–258. doi: 10.1007/s00572-006-0101-6
Gaxiola, R. A., Palmgren, M. G., and Schumacher, K. (2007). Plant proton pumps. FEBS Lett. 581, 2204–2214. doi: 10.1016/j.febslet.2007.03.050
Gianinazzi-Pearson, V., Arnould, C., Oufattole, M., Arango, M., and Gianinazzi, S. (2000). Differential activation of H+-ATPase genes by an arbuscular mycorrhizal fungus in root cells of transgenic tobacco. Planta 211, 609–613. doi: 10.1007/s004250000323
Hadian-Deljou, M., Esna-Ashari, M., and Mirzaie-asl, A. (2020). Alleviation of salt stress and expression of stress-responsive gene through the symbiosis of arbuscular mycorrhizal fungi with sour orange seedlings. Sci. Hortic. 268:109373. doi: 10.1016/j.scienta.2020.109373
Haruta, M., Burch, H. L., Nelson, R. B., Barrett-Wilt, G., Kline, K. G., Mohsin, S. B., et al. (2010). Molecular characterization of mutant Arabidopsis plants with reduced plasma membrane proton pump activity. J. Biol. Chem. 285, 17918–17929. doi: 10.1074/jbc.m110.101733
Haruta, M., and Sussman, M. R. (2012). The effect of a genetically reduced plasma membrane protonmotive force on vegetative growth of Arabidopsis. Plant Physiol. 158, 1158–1171. doi: 10.1104/pp.111.189167
He, J. D., Zou, Y. N., Wu, Q. S., and Kuča, K. (2020). Mycorrhizas enhance drought tolerance of trifoliate orange by enhancing activities and gene expression of antioxidant enzymes. Sci. Hortic. 262:108745. doi: 10.1016/j.scienta.2019.108745
Ingrain, E. A., and Malamy, J. E. (2010). Root system architecture in avoiding chilling-induced water stress. Adv. Bot. Res. 55, 76–106.
Johansen, A., Finlay, R. D., and Olsson, P. A. (2010). Nitrogen metabolism of external hyphae of the arbuscular mycorrhizal fungus Glomus intraradices. New Phytol. 133, 705–712. doi: 10.1111/j.1469-8137.1996.tb01939.x
Krajinski, F., Courty, P. E., Sieh, D., Franken, P., Zhang, H. Q., Bucher, M., et al. (2014). The H+-ATPase HA1 of Medicago truncatula is essential for phosphate transport and plant growth during arbuscular mycorrhizal symbiosis. Plant Cell 26, 1808–1817. doi: 10.1105/tpc.113.120436
Liu, J. L., Chen, J. D., Xie, K., Tian, Y., Yan, A. N., Liu, J. J., et al. (2020). A mycorrhiza-specific H+-ATPase is essential for arbuscule development and symbiotic phosphate and nitrogen uptake. Plant Cell Environ. 43, 1069–1083. doi: 10.1111/pce.13714
Liu, J. L., Liu, J. J., Chen, A. Q., Ji, M. J., Chen, J. D., Yang, X. F., et al. (2016). Analysis of tomato plasma membrane H+-ATPase gene family suggests a mycorrhizal-mediated regulatory mechanism conserved in diverse plant species. Mycorrhiza 26, 645–656. doi: 10.1007/s00572-016-0700-9
Livak, K. J., and Schmittgen, T. D. (2001). Analysis of relative gene expression data using real-time quantitative PCR and the 2-Δ Δ CT method. Methods 25, 402–408. doi: 10.1006/meth.2001.1262
Mak, M., Babla, M., Xu, S. C., O’Carrigan, A., Liu, X. H., Gong, Y. M., et al. (2014). Leaf mesophyll K+, H+ and Ca2+ fluxes are involved in drought-induced decrease in photosynthesis and stomatal closure in soybean. Environ. Exp. Bot. 98, 1–12. doi: 10.1016/j.envexpbot.2013.10.003
Małgorzata, J., Wdowikowska, A., and Grażyna, K. (2018). Assay of plasma membrane H+-ATPase in plant tissues under abiotic stresses. Meth. Mol. Biol. 1696, 205–215. doi: 10.1007/978-1-4939-7411-5_14
Młodziñska, E., Kłobus, G., Christensen, M. D., and Fuglsang, A. T. (2014). The plasma membrane H+-ATPase AHA2 contributes to the root architecture in response to different nitrogen supply. Physiol. Plant 154, 270–282. doi: 10.1111/ppl.12305
Palmgren, M. G. (2001). Plant plasma membrane H+-ATPases: powerhouses for nutrient uptake. Annu. Rev. Plant Physiol. Plant Mol. Biol. 52, 817–845. doi: 10.1146/annurev.arplant.52.1.817
Petrasek, J., and Friml, J. (2009). Auxin transport routes in plant development. Development 136, 2675–2688. doi: 10.1242/dev.030353
Phillips, J. M., and Hayman, D. S. (1970). Improved procedures for clearing roots and staining parasitic and vesicular-arbuscular mycorrhizal fungi for rapid assessment of infection. Trans. Br. Mycol. Soc. 55, 158–161. doi: 10.1016/s0007-1536(70)80110-3
Porcel, R., and Ruiz-Lozano, J. M. (2004). Arbuscular mycorrhizal influence on leaf water potential, solute accumulation, and oxidative stress in soybean plants subjected to drought stress. J. Exp. Bot. 55, 1743–1750. doi: 10.1093/jxb/erh188
Ramos, A. C., Façanha, A. R., and José, F. (2008a). “Ion dynamics during the polarized growth ofarbuscular mycorrhizal fungi: from presymbiosis to symbiosis,” in Mycorrhiza: State of the Art, Genetics and Molecular Biology, Eco-function, Biotechnology, Eco-physiology, Structure and Systematics, ed. A. Varma (Berlin: Springer), 241–260. doi: 10.1007/978-3-540-78826-3_12
Ramos, A. C., Façanha, A. R., and José, F. (2008b). Proton (H+) flux signature for the presymbiotic development of the arbuscular mycorrhizal fungi. New Phytol. 178, 177–188. doi: 10.1111/j.1469-8137.2007.02344.x
Ramos, A. C., Martins, M. A., Okorokova-Façanha, A. L., Fábio, L. O., Okorokov, L. A., and Nuno, S. (2009). Arbuscular mycorrhizal fungi induce differential activation of the plasma membrane and vacuolar H+ pumps in maize roots. Mycorrhiza 19, 69–80. doi: 10.1007/s00572-008-0204-3
Requena, N., Breuninger, M., Franken, P., and Ocón, A. (2003). Symbiotic status, phosphate, and sucrose regulate the expression of two plasma membrane H+-ATPase genes from the mycorrhizal fungus Glomus mosseae. Plant Physiol. 132, 1540–1549. doi: 10.1104/pp.102.019042
Staal, M., De-Cnodder, T., Simon, D., Vandenbussche, F., Vander Straeten, D., Verbelen, J., et al. (2011). Apoplastic alkalinization is instrumental for the inhibition of cell elongation in the Arabidopsis root by the ethylene precursor 1-aminocyclopropane-1-carboxylic acid. Plant Physiol. 155, 2049–2055. doi: 10.1104/pp.110.168476
Sun, Y. P., Unestam, T., Lucas, S. D., Johanson, K. J., Kenne, L., and Finlay, R. (1999). Exudation-reabsorption in a mycorrhizal fungus, the dynamic interface for interaction with soil and soil microorganisms. Mycorrhiza 9, 137–144. doi: 10.1007/s005720050298
Tang, Z. C. (1999). A Guide to Modern Plant Physiology Experiments. Shanghai: Science Press, 138–139.
Ueno, K., Kinoshita, T., Inoue, S., Emi, T., and Shimazaki, K. (2005). Biochemical characterization of plasma membrane H+-ATPase activation in guard cell protoplasts of Arabidopsis thaliana in response to blue light. Plant Cell Physiol. 46, 955–963. doi: 10.1093/pcp/pci104
Wang, Y., Noguchi, K., Ono, N., Inoue, S., Terashima, I., and Kinoshita, T. (2014). Overexpression of plasma membrane H+-ATPase in guard cells promotes light-induced stomatal opening and enhances plant growth. Proc. Natl. Acad. Sci. U.S.A. 111, 533–538. doi: 10.1073/pnas.1305438111
Wu, Q. S., He, J. D., Srivastava, A. K., Zou, Y. N., and Kuča, K. (2019). Mycorrhizas enhance drought tolerance of citrus by altering root fatty acid compositions and their saturation levels. Tree Physiol. 39, 1149–1158. doi: 10.1093/treephys/tpz039
Wu, Q. S., Srivastava, A. K., and Zou, Y. N. (2013). AMF-induced tolerance to drought stress in citrus: a review. Sci. Hortic. 164, 77–87. doi: 10.1016/j.scienta.2013.09.010
Xiong, Y. C., Li, F. M., Zhang, T., and Xia, C. (2007). Evolution mechanism of non-hydraulic root-to-shoot signal during the anti-drought genetic breeding of spring wheat. Environ. Exp. Bot. 59, 193–205. doi: 10.1016/j.envexpbot.2005.12.003
Yoshida, S. (1991). Chilling-induced inactivation and its recovery of tonoplast H+-ATPase in mung bean cell suspension cultures. Plant Physiol. 95, 456–460. doi: 10.1104/pp.95.2.456
Zhang, F., Zou, Y. N., Wu, Q. S., and Kuča, K. (2020). Arbuscular mycorrhizas modulate root polyamine metabolism to enhance drought tolerance of trifoliate orange. Environ. Exp. Bot. 171:103962.
Zou, Y. N., Wang, P., Li, C. Y., Ni, Q. D., Zhang, D. J., and Wu, Q. S. (2017). Mycorrhizal trifoliate orange has greater root adaptation of morphology and phytohormones in response to drought stress. Sci. Rep. 7:41134.
Keywords: citrus, H+-ATPase, mycorrhiza, proton pump, water deficit
Citation: Cheng H-Q, Zou Y-N, Wu Q-S and Kuča K (2021) Arbuscular Mycorrhizal Fungi Alleviate Drought Stress in Trifoliate Orange by Regulating H+-ATPase Activity and Gene Expression. Front. Plant Sci. 12:659694. doi: 10.3389/fpls.2021.659694
Received: 28 January 2021; Accepted: 01 March 2021;
Published: 25 March 2021.
Edited by:
Italo F. Cuneo, Pontificia Universidad Católica de Valparaíso, ChileReviewed by:
Yong-ming Huang, Hubei Academy of Agricultural Sciences, ChinaXiancan Zhu, Anhui Normal University, China
Copyright © 2021 Cheng, Zou, Wu and Kuča. This is an open-access article distributed under the terms of the Creative Commons Attribution License (CC BY). The use, distribution or reproduction in other forums is permitted, provided the original author(s) and the copyright owner(s) are credited and that the original publication in this journal is cited, in accordance with accepted academic practice. No use, distribution or reproduction is permitted which does not comply with these terms.
*Correspondence: Qiang-Sheng Wu, wuqiangsh@163.com; Kamil Kuča, kamil.kuca@uhk.cz