Factors Influencing the Persistence of Salmonella Infantis in Broiler Litter During Composting and Stabilization Processes and Following Soil Incorporation
- 1Institute of Soil, Water and Environmental Sciences, Newe Ya'ar Research Center, Agricultural Research Organization - Volcani Institute, Ramat Yishay, Israel
- 2Faculty of Agriculture, Food and Environment, The Hebrew University of Jerusalem, Jerusalem, Israel
- 3Division of Avian Diseases, Kimron Veterinary Institute, Bet Dagan, Israel
- 4Institute of Postharvest and Food Sciences, Agricultural Research Organization - Volcani Institute, Rishon LeZion, Israel
Broiler litter (BL), a by-product of broiler meat production, is frequently contaminated with Salmonella and other zoonotic pathogens. To ensure the safety of crop production chains and limit pathogen spread in the environment, a pre-treatment is desired before further agricultural utilization. The objective of this study was to characterize the effect of physico-chemical properties on Salmonella persistence in BL during composting and stabilization and following soil incorporation, toward optimization of the inactivation process. Thirty-six combinations of temperature (30, 40, 50, and 60°C), water content (40, 55, and 70%; w/w), and initial pH (6, 7, and 8.5) were employed in static lab vessels to study the persistence of Salmonella enterica serovar Infantis (S. Infantis; a multidrug-resistant strain) during incubation of artificially-inoculated BL. The effect of aeration was investigated in a composting simulator, with controlled heating and flow conditions. Temperature was found to be the main factor significantly influencing Salmonella decay rates, while water content and initial pH had a secondary level of influence with significant effects mainly at 30 and 40°C. Controlled simulations showed faster decay of Salmonella under anaerobic conditions at mesophilic temperatures (<45°C) and no effect of NH3 emissions. Re-wetting the BL at mesophilic temperatures resulted in Salmonella burst, and led to a higher tolerance of the pathogen at increased temperatures. Based on the decay rates measured under all temperature, water content, and pH conditions, it was estimated that the time required to achieve a 7 log10 reduction in Salmonella concentration, ranges between 13.7–27.2, 6.5–15.6, 1.2–4.7, and 1.3–1.5 days for 30, 40, 50, and 60°C, respectively. Inactivation of BL indigenous microbial population by autoclaving or addition of antibiotics to which the S. Infantis is resistant, resulted in augmentation of Salmonella multiplication. This suggests the presence of microbial antagonists in the BL, which inhibit the growth of the pathogen. Finally, Salmonella persisted over 90 days at 30°C in a Vertisol soil amended with inoculated BL, presumably due to reduced antagonistic activity compared to the BL alone. These findings are valuable for risk assessments and the formulation of guidelines for safe utilization of BL in agriculture.
Introduction
The poultry sector is amongst the fastest growing agriculture-based meat production industries worldwide, due to the increasing demand for meat and egg products (Bolan et al., 2010). Broiler litter (BL) is a by-product of broiler meat production. It consists of a mixture of bedding (usually sawdust or shavings, rice hulls or straw), manure, and wasted feeds and feathers. BL can become a source of zoonotic pathogens such as Salmonella that are pathogenic to humans (Chinivasagam et al., 2010; Wilkinson et al., 2011; Gould et al., 2013). Often, Salmonella that are excreted from infected chickens, contaminate the litter and eventually, the poultry house environment and the entire flock (Jones et al., 1991; Bryan and Doyle, 1995; Corrier et al., 1999; Trampel et al., 2000). Thus, besides the immediate risk to public health through the consumption of contaminated eggs and broiler meat products, Salmonella can be transferred to the agricultural environment by contaminated litter.
BL is a valuable alternative fertilizer and soil additive that is used in conventional and organic farming. It has been proven in numerous studies to be an effective fertilizer for row crops, including corn, cotton, and soybean (Tewolde et al., 2013) and in some cases, it was shown to be more effective and valuable than synthetic fertilizers (Tewolde et al., 2011). A meta-analysis based on 116 studies showed positive effects of poultry litter compared to inorganic fertilizers regarding P and K plant uptake and other indicators of soil fertility (Lin et al., 2016). Yet, although BL may be contaminated by a variety of zoonotic pathogens, most farmers use it without processing or after partial stabilization by stockpiling (Ogejo and Collins, 2009; Wilkinson et al., 2011; Wiedemann, 2015). These common practices may facilitate pathogen spread in agricultural fields, which in turn may lead to crop contamination. Indeed, there are numerous reports on the contamination of fresh produce in the field due to soil contamination (Beuchat, 2002; Bell et al., 2015; Gu et al., 2018; Jechalke et al., 2019). In many such cases Salmonellosis outbreaks have been associated with consuming contaminated fresh produce, like tomatoes, cantaloupe, and leafy vegetables (Fatica and Schneider, 2011; Herman et al., 2015; Chaves et al., 2016). Salmonella enterica serovar Typhimurium (S. Typhimurium) was detected in soil up to 231 days after application of poultry and cattle manure composts that were artificially inoculated with the pathogen (Islam et al., 2004a,b). In these studies, Salmonella was also detected in vegetable crops grown in the tested soils, 203 and 84 days after seeding of carrot and radish, respectively (Islam et al., 2004a), or 231 and 63 days after seeding parsley and lettuce, respectively (Islam et al., 2004b). In spite of strict control measures and regulations, Salmonella outbreaks due to consumption of contaminated fresh produce are still a threat to public health.
Thermal processing is considered as a practical and effective approach for inactivating pathogens in BL or BL-based organic fertilizers prior to land application (Williams and Benson, 1978; Macklin et al., 2008; Wilkinson et al., 2011). Although thermal inactivation may occur partially by stockpiling the litter for some time before spreading, it cannot be effective as compared to controlled thermophilic composting. The first active stage of composting is an exothermic process governed by aerobic decomposition reactions. Typically, the efficiency of the composting process depends on proper aeration of the material (Sánchez et al., 2017; Alkoaik, 2019; Oazana et al., 2020), under which the degradation rates can be maximized. These conditions in turn yield high rates of heat emission and eventually lead to thermal inactivation of pathogens. Based on international regulations (USA and Canada; also adopted in Israel), to ensure effective pathogen elimination, all compost particles need to be exposed to a minimum temperature of 55°C for a period of at least 3 consecutive days (United States Environmental Protection Agency, 2003; Wichuk and McCartney, 2007). Yet, composting piles are often not extensively controlled, such that thermal inactivation is not efficient throughout the entire pile even after several turnings (i.e., temperatures do not reach a minimum of 55°C throughout the pile; Wilkinson et al., 2011; Avidov et al., 2017). Although thermophilic temperatures are reported in numerous composting studies, only average values are usually presented, while the spatial variability of the temperature in the pile is practically unknown. The situation is even more critical in static piles, where a minimal management regime is commonly applied (Avidov et al., 2019). Pathogens surviving the composting process or any phase of stabilization, may regrow during storage or following land application. Indeed, several studies have demonstrated the persistence of zoonotic pathogens in the finished compost at different levels of maturity and in compost-amended soils (Chen and Jiang, 2014; Reynnells et al., 2014; Hruby et al., 2018).
The combination of temperature with other environmental factors may have variable effects on Salmonella persistence in BL. Several studies have examined the effect of temperature and water content, generally showing that thermal susceptibility of the pathogen increases with increasing water content. At low water contents, desiccation may play a major role in pathogen inactivation. Yet, desiccation-adapted Salmonella spp. persisted longer in aged chicken litter compared to non-adapted cells (Chen et al., 2013). The increased persistence and temperature-tolerance of Salmonella at low water content was evident in a study by Liu et al. (1969), who showed that Salmonella Senftenberg strain 775 W in meat and bone meal of 5% water content remained relatively stable at temperatures as high as 50°C. Heat inactivation of Salmonella spp. in fresh poultry compost was faster at 50% water content compared to 40%, both at 50 and 55°C (Singh et al., 2012). Yet, an opposite trend was shown by Wilkinson et al. (2011) who found that at lower temperatures (35 and 45°C) more effective reduction of S. Typhimurium in poultry litter occurred at 30% than at 65% water content. Other co-factors may also play a role in bacterial inactivation, such as the combined and intensified effect of drying and NH3 emissions, shown by Himathongkham and Riemann (1999). Biological mechanisms, such as competition between indigenous microorganisms and pathogens (Wichuk and McCartney, 2007), and microbial antagonism (Millner et al., 1987; Erickson et al., 2010; Gurtler et al., 2018) may also affect pathogen inactivation.
Overall, beyond thermal inactivation, which is considered as the primary mechanism responsible for the inactivation of human pathogens in BL and other livestock manures, there is a lack of knowledge about the combined effect of different physico-chemical and biological factors. This study aimed at investigating various key factors and the interactions among them that influence the persistence of Salmonella in BL during composting and stabilization processes and following soil incorporation.
Materials and Methods
Broiler Litter (BL) and Soil
BL
Selected physical and chemical properties of the BL and the soil used in this study are presented in Tables 1A,B. Un-stabilized (fresh) BL was collected throughout the experimental period from tunnel-ventilated broiler houses of several farms located at the Jezreel Valley, northern Israel: Moshav Barak, Moshav Beit She'arim, Moshav Balfouria, and Kibbutz Yifat. The farms use raising protocols of the main poultry cooperatives in Israel, while some of the variability shown in Table 1 may represent spatial variation within any given poultry house and between different growing cycles at the same poultry house. In all cases, the BL was collected at the end of 6-weeks growing period. Stabilized BL was obtained from a static pile, representing a common practice in Israel. About 35 m3 of BL from the poultry farm of Moshav Balfouria were stockpiled in the open yard without any further treatment for a period of 8 months. This pile was closely monitored within the first 2 months, showing that 27 and 23% of the stockpile volume were below 45°C during the first and second weeks, respectively. Later, about 30% of the stockpile volume remained below that temperature (based on ca. 53 sampling points monitored on a weekly basis in the first month and then biweekly in the second month). Composted BL was prepared using a polyethylene sleeve with forced aeration (Avidov et al., 2017, 2018). For that, about 35 m3 of BL was pre-wetted to achieve a water content of ca. 50–55% (51.1 ± 1.71%), which is within the optimal range for composting (Christian et al., 2009; Zakarya et al., 2018), and then packed in a polyethylene sleeve that was sealed manually (Avidov et al., 2019). Controlled composting was processed for 56 days with blower settings of 2 min on and 30 min off. The entire compost material within the sleeve maintained thermophilic temperatures (>45°C) up to 69 and 66°C during the first and second weeks, respectively. Only ca. 2 and 4% of the volume was estimated to be below 45°C during the third and fourth weeks, respectively (based on ca. 48 sampling points monitored weekly in the first month and biweekly in the second month. Temperatures were measured using Type K thermocouples, constructed on 80 cm-long stainless steel rods; Elcon Ltd., Israel). The composted BL was left (stored) within the sleeve which was partly open for additional 6 months. Finally, the material of both the stockpile and the sleeve was sampled from 9 different locations each, unified and homogenized. All materials (un-stabilized, stabilized, or composted BL) were stored at 4°C until use, except for the experiments used to assess the potential of antagonistic indigenous populations against Salmonella, for which the un-stabilized BL was used without any storage. Before each experiment, the BL was acclimated for ca. 24 h at room temperature.
Soil
A Vertisol-type soil was collected from 0 to 30 cm depth at the Newe Ya'ar Research Center, Jezreel Valley; northern Israel.
Physical and Chemical Analyses
Dry based aqueous extracts (1:9 w/w) of BL or soil samples were prepared with distilled water by shaking the suspension for 1 h at 200 RPM on a reciprocal shaker. The pH was analyzed directly in the suspension (LL-Ecotrode Plus WOC; Metrohm, Herisau, Switzerland), while the electrical conductivity (EC) was determined in the supernatant after centrifugation at 6,000 RPM for 20 min at 25°C (CyberScan CON 11, Eutech Instruments, Thermo Fisher Scientific Inc., Waltham, Massachusetts, USA). Total C and N were determined after grinding sub-samples (mixer mill MM 400, Retsch, Haan, Germany) by FlashSmart 2000 Elemental Analyzer (Thermo Fisher Scientific Inc., Waltham, Massachusetts, USA).
Bacterial Strain and Inoculum Preparation
We used a clinical isolate of Salmonella enterica serovar Infantis; a multi-drug resistant strain containing a mega plasmid (pESI) that carries several antibiotics resistance genes (Aviv et al., 2014; courtesy of Prof. Ohad Gal-Mor, Sheba Medical Center, Tel-Ha'shomer, Israel). The culture was stored in phosphate-buffered saline (PBS) containing 15% glycerol (Duchefa biochemie, Netherlands) at −80°C. All growing media were prepared according to the manufacturer's instructions. Before each experiment, about 100 μl of the stored culture (PBS with 15% glycerol) were transferred into 100 ml of nutrient broth (NB; Oxoid, Basingstokes, UK) with tetracycline (20 μg ml−1) and incubated for 24–72 h at 37°C. The culture was then transferred into several sterile polypropylene (PP) tubes, and washed 3 times with 40 ml sterile PBS by centrifugation at 6,500 RPM for 10 min at 25°C. The final pellet was re-suspended in 40 ml PBS to achieve a final concentration of ca. 9 log10 colony forming units (CFU) ml−1. To inoculate the BL, the suspension was added together with the amount of water needed to adjust the BL to the desired water content. The final concentration of Salmonella was ca. 7 log10 CFU g−1 dry matter.
Salmonella Enumeration
The initial stock prepared for each experiment was enumerated by serial dilution in sterile PBS and plating triplicate aliquots of 100 μl on XLD agar (Oxoid Basingstokes, UK) amended with tetracycline (20 μg ml−1). The plates were incubated at 37°C for 48 h before counting. BL samples, before and following Salmonella inoculation, were analyzed by weighing 8 g of dry matter (based on a preliminary analysis of water content) and suspending it in a sterile stomacher bag after the addition of 80 ml PBS. The stomacher (STO-4, MRC, Israel) was operated at 10 pedals s−1 for 3 min, and then the suspension was transferred into a sterile PP tube and let to settle for ca. 5 min. A 1 ml sample was taken from the top suspension to prepare serial dilutions, from which 100 μl (or 200 μl in cases where we had to decrease the detection limit) were plated in triplicate plates, as described above. Typical black colonies were counted as presumptive S. Infantis. No black colonies were observed in un-inoculated BL samples. The detection limit was determined as 10–20 CFU g−1 dry matter. Salmonella enrichment was also performed to ensure complete bacterial inactivation. A 1 ml of the undiluted suspension was transferred into each of 5–10 tubes containing 9 ml of buffered peptone water (BPW, Oxoid, Basingstokes, UK) and tetracycline (20 μg ml−1). The tubes were incubated for 24 h at 37°C. Each of the enrichment tubes (undiluted) was checked for positive or negative growth on XLD agar amended with tetracycline to check for the presence of typical Salmonella colonies. The detection limit after enrichment was determined as 1–2 CFU g−1 dry matter.
Decay Rate Calculations
Exponential decay rates of Salmonella were calculated using Equation (1)
where C(t) is the concentration of Salmonella (CFU g−1 dry matter) at point in time t (d), C0 is the initial concentration of Salmonella, and k is the first-order decay constant (d−1). Decay rate constants were calculated using a linear curve fit between the natural log-transformed concentrations (ln C/C0) and time. The number of data points to be included in the linear correlation of each dataset, was selected to provide the highest R2, while excluding data points below the detection limit.
Laboratory Simulations of Temperature, Water Content, and pH Conditions
Thirty-six combinations of four temperatures (30, 40, 50, and 60°C), three water contents (40, 55, and 70%; w/w), and three initial pH (6, 7, and 8.5) were tested. For each combination, triplicate vessels were prepared with 200 g (dry based) of un-stabilized BL that was artificially inoculated with S. Infantis at a concentration of ca. 7 log10 CFU g−1 dry matter. The BL was first placed in a biohazard bag and adjusted to the desired pH by adding acid (1 M H2SO4) or base (1 M NaOH). The amount of required acid or base was pre-determined in preliminary titrations on parallel samples (at 55% water content). The pH-adjusted BL was thoroughly mixed by massaging the bag over several minutes and then inoculating it with S. Infantis, as described above, to achieve the desired initial concentration. The inoculated BL was thoroughly mixed by massaging the bag one more time and finally the bag was placed (loosely tied) in a 600 ml glass beaker. Aeration was not controlled in these experiments. The beakers were placed in an incubator (pre-verified over 4 days before each experiment) at the desired temperature (30, 40, 50, or 60°C) for 14 days, and then transferred to 30°C for additional 14 days of incubation to evaluate regrowth potential. The desired water content was adjusted during the incubation period, based on gravimetric analyses performed in a preliminary experiment on vessels with non-inoculated BL under identical conditions. Adjustments were made every sampling day during the first 2 weeks and every 3 days during the rest of the experiment. Salmonella enumerations were performed on sub-samples from each vessel, on days 0, 1, 3, 7, 14, and 28. A preliminary analysis showed that the percentage of inoculated S. Infantis that could be recovered from the BL after incubation for 24 h at 4°C was 75 and 71% on average, at 50 and 70% BL water content, respectively (data not shown).
Before each incubation series, the BL was checked for the presence of indigenous tetracycline-resistant Salmonella and was always found to be below the detection limit after enrichment. Triplicate non-inoculated vessels were incubated in each of the four temperatures and served as controls. In these samples, the BL was adjusted to a water content of 55% and initial pH 7 (the water content was adjusted using 10 ml of PBS plus the needed amount of deionized water and acid/base, as done for the inoculated samples). The aim of the control vessels was to negate any possible growth of indigenous Salmonella or cross-contamination between samples.
Controlled Simulations of Aerobic and Anaerobic Conditions
The fate of S. Infantis in un-stabilized BL under controlled aerobic and anaerobic conditions was evaluated using the Agricultural Research Organization Composting Simulator (ARO-CS) in which the temperature and aeration are controlled by a programmable logic controller (Oazana et al., 2018). The system includes six 9-liter reactors; each is mounted into a separate 80-liter bath and equipped with two temperature sensors: one thermocouple in each bath and one PT-100 in each reactor. The airflow was controlled by individual mass flow controllers (MFC) and transferred to the reactors through a humidifier mounted into the water bath, ensuring water-saturated inflow, and eliminating drying of the composting mixture. However, in cases of intense aerobic activity, during which it was difficult to restrain the heat evolved (Oazana et al., 2020), the air was transferred through a bypass to enable evaporative cooling.
Two simulation experiments were performed: In the first experiment the effect of aeration on the persistence of S. Infantis at 40, 50, and 60°C was evaluated during 38 days. Each of the six reactors was filled with 1.4 kg of un-stabilized BL (dry-based; 4.5 liters) that was adjusted to 40% water content, initial pH 7, and was artificially inoculated with S. Infantis at ca. 7 log10 CFU g−1 dry matter. Three of the reactors were maintained under aerobic conditions with a flow of 5 l min−1 (10 min on and 2 min off), and three reactors were kept under anaerobic conditions, without any aeration. The simulation was divided into three phases: (I) mesophilic phase: Temperature was maintained between 30 and 37°C for 9 days (aerobic reactors), or between 35 and 37°C for 7 days (anaerobic reactors). The humidifier bypass was used for the aerobic reactors to restrain the heat evolved during this phase. This in turn resulted in BL drying and required re-wetting, which delayed the move to phase II in 2 days. (II) Heating phase: Temperature was increased to 40, 50, and 60°C, under both aerobic and anaerobic conditions, one reactor for each temperature. These temperatures were maintained for 14 (aerobic) or 7 days (anaerobic). (III). A second mesophilic phase: The content of each reactor was transferred into a lab incubator for additional 14 days at 30°C, to explore regrowth potential. The BL was sampled during the simulations on days 0, 3, 7, 9, 10, 11, 12, 14, 16, 23, and 37 (aerobic), and on days 0, 3, 7, 9, 10, 14, 28, and 37 (anaerobic) and analyzed for Salmonella, water content, and pH. Emission of NH3 was measured on days 1, 3, 7, 9, 11, 16, and 23 (aerobic) or days 1, 3, 8, 10, and 14 (anaerobic). Air samples (ca. 25 liters) were collected in NalophanTM bags (polyethylene terephthalate; thickness 20 mm; Kalle GmbH, Wiesbaden, Germany) through a sampling port located on the cape of each reactor. Before sampling, the reactors were flushed for 20 min at 2.5 l min−1 of air (aerobic) or N2 (99.999% purity) (anaerobic). NH3 was analyzed in triplicates for each bag, using the spectrophotometric method of Willis et al. (1996) with slight modifications as described by Avidov et al. (2017).
In the second simulation experiment, the persistence of Salmonella in un-stabilized BL was evaluated during a more gradual increase of temperature under anaerobic conditions. Duplicate reactors were filled with 1.4 kg BL (dry-based; 4.7 liters) that was adjusted to 40% water content, initial pH 7, and artificially inoculated with S. Infantis, as described above. The reactors were heated to 45°C by increment steps of 1°C and a total heating time of 14 days. The BL was sampled on days 0, 1, 2, 3, 5, 7, 9, 11, and 14 and analyzed for Salmonella concentrations.
Modulation of Microbial Populations in BL
Two sets of experiments were conducted to check the possible involvement of antagonistic microorganisms in BL that inhibit the growth of Salmonella under mesophilic temperatures: (1) Heat inactivation of indigenous microbial populations of the BL. (2) Addition of antibiotics to which the S. Infantis strain is resistant. The effect of heat inactivation was explored both in liquid suspension and in the BL itself. For BL suspension—aliquots of 8 g (dry-based) of un-stabilized BL pre-adjusted to 60% water content, were placed in open 50 ml PP tubes and autoclaved for 1 h (Vertical pressure steam sterilizer LS-B50L-I, KWF, China; 121°C, 0.11 MPa). Autoclave performance was validated with 1 mL spore ampules (Crosstex, USA) placed within a BL sample of similar size. The autoclaved and non-autoclaved BL samples were inoculated with 100 μl of a Salmonella stock, and mixed by vortexing the tubes for a few seconds. The content of each tube was diluted 1:10 in PBS (mixing in a sterile stomacher bag, as described above), and 25 ml suspension were transferred into Erlenmeyer flasks containing 100 ml of sterile NB (a total volume of 125 ml). Control flasks (no BL suspension) contained 100 ml of sterile NB that was inoculated with 100 μl of the Salmonella stock. The initial concentration of Salmonella was ca. 2 log10 CFU ml−1 in EXP 1 (heat inactivation) and 4 log CFU ml−1 in EXP 2 (a second heat inactivation experiment and the addition of antibiotics). Multiplication of the pathogen was monitored over 24 h. For the BL itself—aliquots of 8 g (dry-based) of un-stabilized and stabilized BL were placed in 50 ml open PP tubes and autoclaved. Both autoclaved and non-autoclaved samples were inoculated with Salmonella as described above. The initial concentration of Salmonella in this experiment was ca. 8 log10 CFU g−1 dry matter. The tubes were placed inside a biological hood and incubated for 11 days at room temperature (20–25°C) under aerobic or anaerobic conditions. The tubes under aerobic conditions were left with untighten caps and those under anaerobic conditions were kept closed after replacing the headspace with N2 (purging for 30 s). Salmonella was enumerated by the end of the incubation period.
The effect of antibiotics was explored in a liquid suspension of un-stabilized BL as described above (without autoclaving). The suspension was amended with a cocktail of antibiotics [tetracycline, 20 μg ml−1; nitrofurantoin, 64 μg ml−1; trimethoprim, 50 μg ml−1; nalidixic acid, 20 μg ml−1; sulfamethoxazole, 50 μg ml−1; and rifampin, 100 μg ml−1 (Aviv et al., 2014); all from Sigma–Aldrich, St. Louis, MO; ≥97% purity]. Amended and un-amended NB medium were inoculated with the same Salmonella stock. The initial concentration of Salmonella in this experiment was ca. 4 log10 CFU ml−1 and multiplication of the pathogen was monitored over 120 h.
Persistence of S. Infantis in Soil-BL Mixtures
Batch of 1 kg soil was mixed with 5% (v/v) of un-stabilized or stabilized BL containing S. Infantis, at an initial concentration of ca. 6 log10 CFU g−1 dry matter of the final soil-BL mixture. The mixtures were brought to water field capacity of 30 or 70% (13.5 and 31.5% water content, respectively) and then divided into aliquots of 8 g (dry-based), which were transferred into 50 ml PP tubes and incubated at 30°C. Triplicate tubes were sacrificed for Salmonella counting on days 0, 4, 8, 11, 18, 31, 45, 60, 90, and 105. The content of each tube was suspended in PBS at 1:5 ratio, vortexed for 3 min, and Salmonella enumeration was performed as described above. Triplicate tubes containing non-inoculated soil-BL mixture at 70% water field capacity served as control on each sampling day, and were always found to be free of Salmonella.
Statistical Analyses
JMPIN software was used for all statistical analyses (SAS pro 14; SAS Institute Inc.). Mean values were compared by the Tukey-Kramer Honestly Significant Difference (HSD) test at p ≤ 0.05. Analyses of CFU counts were performed using log-transformed values.
Results
The Combined Effect of Temperature, Water Content, and pH on the Persistence of S. Infantis in BL During Static Incubation
The persistence of S. Infantis under the 36 combinations of temperature, water content, and pH is presented in Figures 1A–I. Each figure represents the results of four temperatures at one selected water content and initial pH. The BL was incubated under the selected temperature (30, 40, 50, or 60°C) for 2 weeks (phase I), during which Salmonella count was reduced below the detection limit after enrichment (1–2 CFU g−1 dry matter). Besides water content which was adjusted during incubation, the pH (Figure 2) and EC (Supplementary Figure 1) were dynamic and monitored in a parallel experiment of non-inoculated BL. Under lower temperatures (30 and 40°C), the pH typically increased during the first 2 weeks and stabilized at the range of 9–9.5. Different dynamics were observed under the higher temperatures (50 and 60°C), in which the pH tended to decrease during phase I and in certain cases to increase later during phase II. This effect of temperature on pH development was more evident at the lower water contents and the lower initial pH. Regarding the EC, a general increase was observed for all combinations during phase I, but it was more evident at a water content of 70% for which this increase was significantly higher in the lower (30 and 40°C) compared to the higher (50 and 60°C) temperatures.
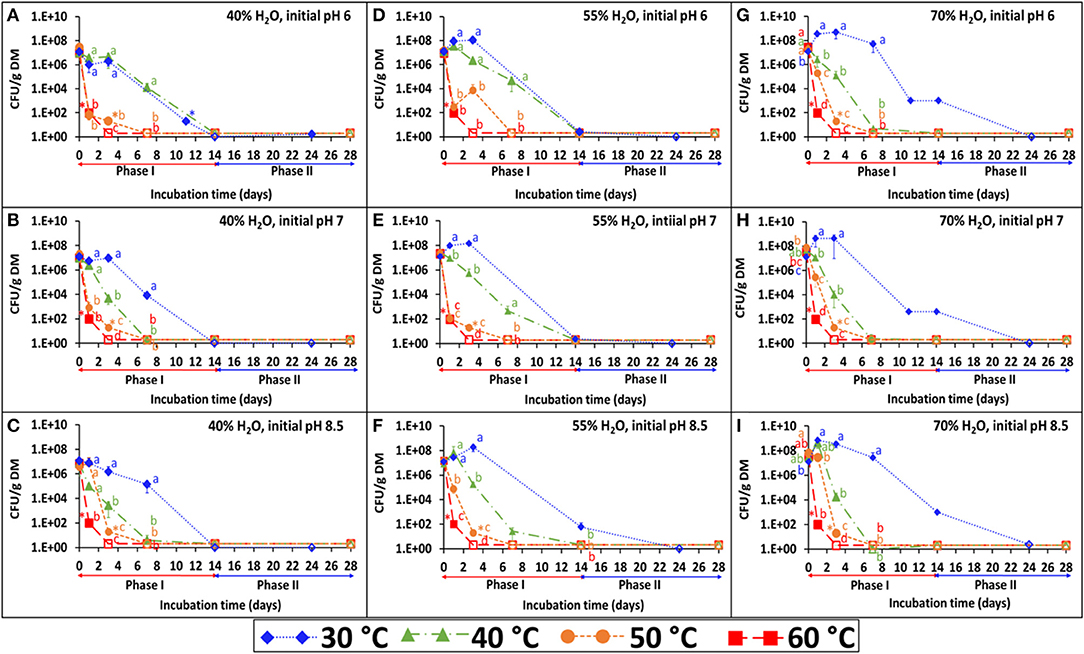
Figure 1. Persistence of S. Infantis in broiler litter (BL) incubated at different temperatures (30, 40, 50, and 60°C), water content (40, 55, and 70%) and initial pH (6, 7, and 8.5). The different incubation temperatures were applied during phase I (weeks 1–2), while at phase II (weeks 3–4) the incubation temperature was 30°C. Error bars represent the standard deviations of triplicate vessels. Letters denote significant differences between treatments at time intervals (log-transformed CFU values; Tukey-Kramer HSD; P ≤ 0.05). Empty symbols indicate values below the detection limit after enrichment (1–2 CFU g−1 dry matter); *values below the detection limit without enrichment (10–20 CFU g−1 dry matter). The figures are grouped according to water contents of 40% (A–C), 55% (D–F), and 70% (G–I). DM, dry matter.
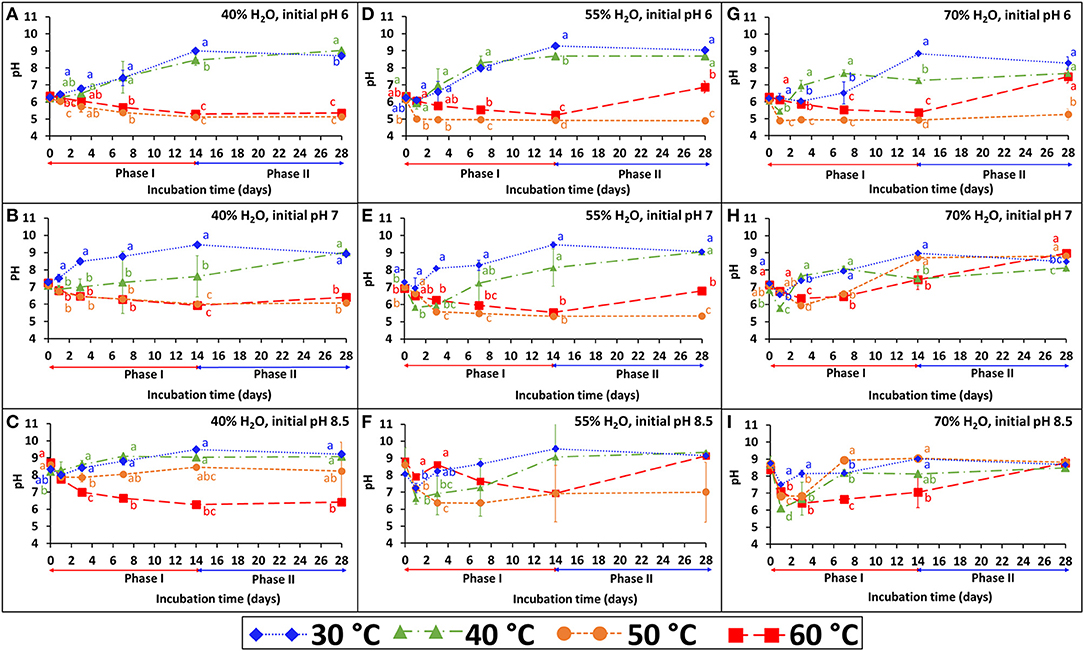
Figure 2. pH dynamics during incubation of broiler litter (BL) at different temperatures (30, 40, 50, and 60°C), water content (40, 55, and 70%) and initial pH (6, 7, and 8.5). The different incubation temperatures were applied during phase I (weeks 1–2), while at phase II (weeks 3–4) the incubation temperature was 30°C. Error bars represent the standard deviations of triplicate vessels. Letters denote significant differences between treatments at time intervals (Tukey-Kramer HSD; P ≤ 0.05). The figures are grouped according to water contents of 40% (A–C), 55% (D–F), and 70% (G–I).
The first-order decay constants in this experiment [–k (day−1)], are presented in Table 2. Based on these values, the time needed to reduce Salmonella below the detection limit after enrichment (<1 CFU g−1 dry matter) was calculated for initial concentrations of 7 and 3 log CFU g−1 dry matter. Temperature was shown to be the main factor influencing Salmonella decay rates, while water content and initial pH were found to be of secondary level of influence with significant effects mainly at 30 and 40°C. Under all water content and pH conditions, it was estimated that the time required to achieve 7 log reduction in Salmonella concentration ranges between 13.7–27.2, 6.5–15.6, 1.2–4.7, and 1.3–1.5 days for 30, 40, 50, and 60°C, respectively. Thus, the effect of temperature was most evident in the transition from mesophilic to thermophilic conditions, whereas increasing the temperature from 40 to 50°C was associated with increased decay rates by a factor of 4.6. Water content had a significant effect at 30°C, with the highest decay rates at a water content of 40% at all initial pH values. In contrast, at 40°C, the highest decay rates were observed at a water content of 70% at all pH values, and also at a water content of 40% and initial pH 7 and 8.5 only. Such effects were still significant at 50°C, with the highest decay rates at water contents of 40 and 55% and initial pH 6 and 7. These effects were negligable at 60°C.
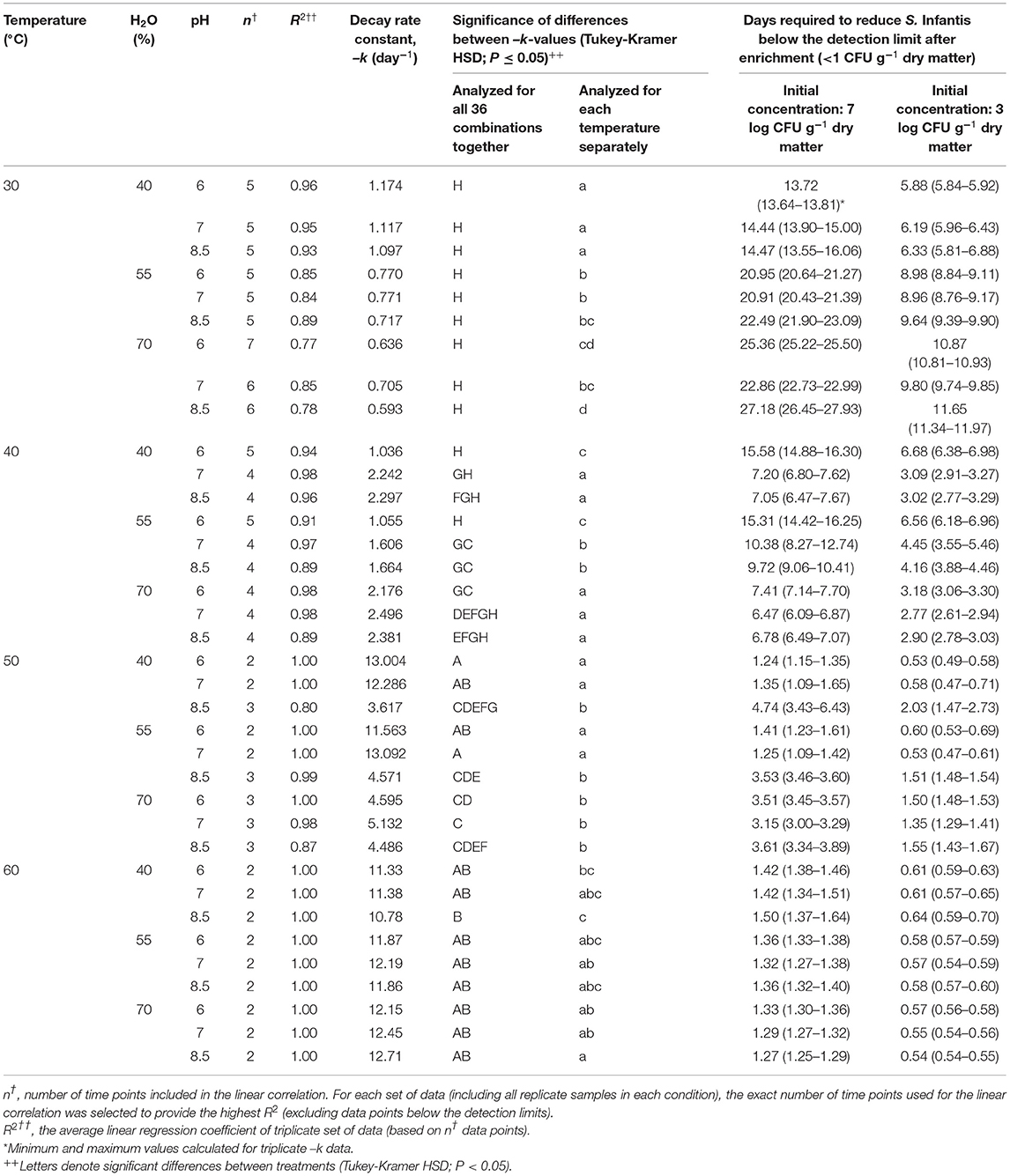
Table 2. First-order decay rate constants (–k; day−1) and the calculated time needed to reduce S. Infantis below the detection limit after enrichment, at initial concentrations of 7 and 3 log CFU g−1 dry matter.
Regrowth of Salmonella was not observed in phase II in any of the combinations after transferring the vessels to 30°C, neither in the vessels which were initially incubated at 30°C. Salmonella counts in non-inoculated control samples were below the detection limit throughout the experiment, negating the possibility that indigenous Salmonella were present in these samples or that cross-contamination occurred between samples.
Persistence of S. Infantis Under Controlled Aerobic and Anaerobic Conditions using Laboratory-Scale Simulations
The persistence of S. Infantis under controlled aerobic and anaerobic simulations of BL, pre-adjusted to a water content of 40% and initial pH 7, is demonstrated in Figures 3A,B. During phase I (mesophilic), Salmonella concentrations decreased substantially under both conditions, with 4–5 log10 reduction under aerobic conditions (Figure 3A) and 7 log10 reduction (below the detection limit) under anaerobic conditions (Figure 3B). During this phase, the water content decreased unintentionally in the aerobic reactors from 40 to 18.7% (Figure 3A–inset), since aeration was provided through a bypass instead of using the humidifier (see Materials and Methods) On day 7, the water content of the three aerobic reactors was re-adjusted to 40%, which in turn resulted in a 4–5 log10 increase of Salmonella concentration in one of the reactors; the one that was set to 50°C during phase II of the simulation. When phase II started (day 9), Salmonella concentrations increased even more in that reactor, but dropped below the detection limit after an additional 3 days. The effect of BL drying was also recognized at 60°C, where Salmonella showed a higher persistence and decreased below the detection limit only after 3 days, which is longer than expected based on the static vessels experiment (Figure 1 and Table 2). Moreover, following 2 days at 40°C in phase II, Salmonella increased by 4 log10 and then steadily increased, reaching a concentration similar to the initial conditions of the simulation. Under these conditions, Salmonella was not reduced below the detection limit, even after 2 more weeks of incubation at 30°C (Phase III). In contrast to aerobic conditions, Salmonella did not persist under anaerobic conditions, and no growth was observed during phases II and III of the simulation (Figure 3B).
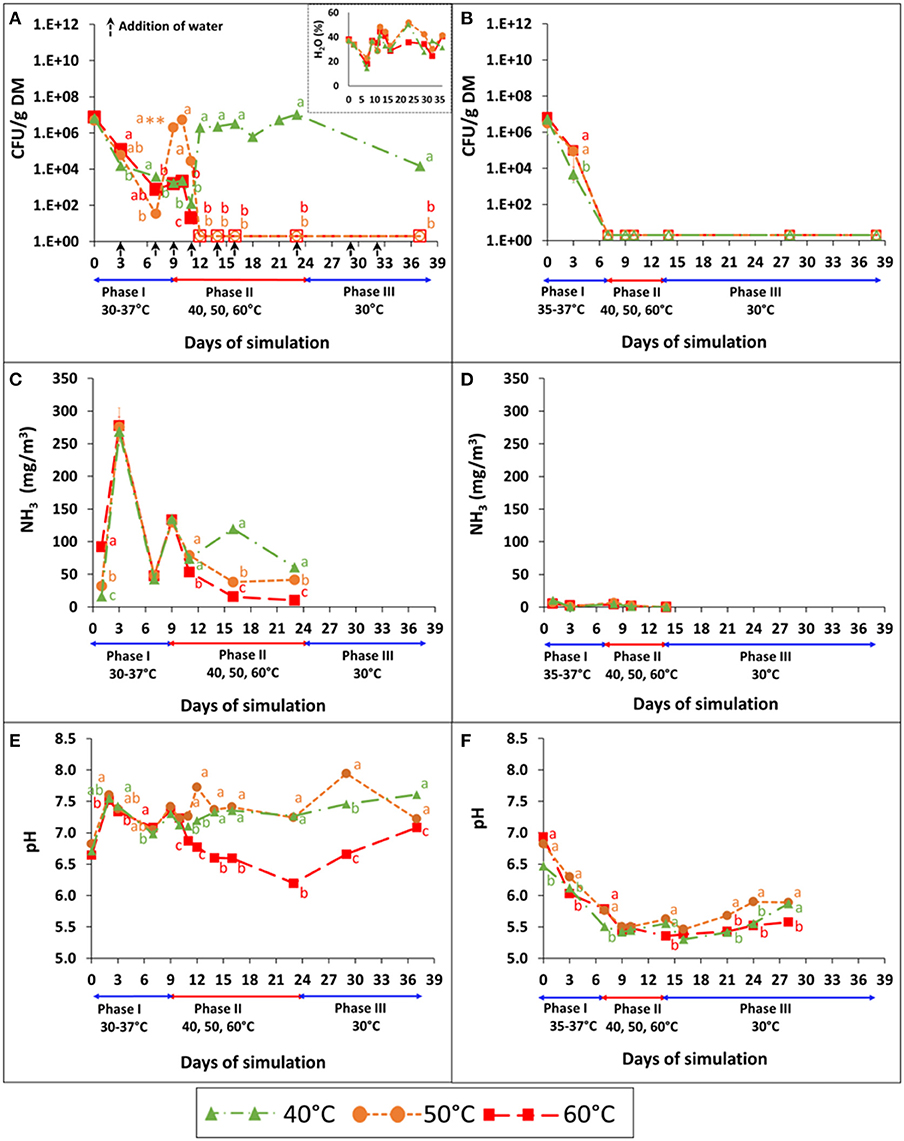
Figure 3. Persistence of S. Infantis (A,B), emissions of NH3 (C,D), and pH dynamics (E,F) in broiler litter (BL), during controlled aerobic and anaerobic simulations performed in six reactors (one reactor for each treatment). The water content was monitored and adjusted under aerobic conditions (A–inset). Mesophilic temperatures were maintained between 30 and 37°C in phase I and then increased to 40, 50, or 60°C in phase II until Salmonella was reduced below the detection limit. Finally, in phase III, the contents of each of the reactors were transferred to 30°C for 2 more weeks. The airflow was constant (5 l min−1) during the simulations of aerobic conditions. Error bars represent the standard deviations of triplicate analyses performed on a unified sample collected from each reactor. Empty symbols indicate values below the detection limit after enrichment (1–2 CFU g−1 dry matter); **a value above plate countability. Letters denote significant differences between treatments at time intervals (Tukey-Kramer HSD; P ≤ 0.05). DM, dry matter.
NH3 monitoring (Figures 3C,D) revealed a major difference between aerobic and anaerobic conditions, with no clear effect of temperature (phase II). Concentrations peaked on day 3 under aerobic conditions (ca. 270 mg m−3) while under anaerobic conditions they were mostly below 5 mg m−3. The respective pH values (Figures 3E,F) also showed a clear difference between aerobic and anaerobic conditions. The pH fluctuated or slightly increased during aerobic simulation, while under anaerobic conditions a distinct reduction (from 6.5–7 to 5.5) was observed in phase I.
The fate of S. Infantis in BL (pre-adjusted to a water content of 40% and initial pH 7) under anaerobic conditions was further investigated in another simulation, during which the mesophilic temperatures increased more gradually from 28 to 42°C (Figure 4). Salmonella concentration decreased from 7.5 log10 to <10 CFU g−1 dry matter (below detection limit) within 11 days, during which the temperature increased to 38°C only. In a similar manner to the other simulations under controlled anaerobic conditions (Figure 3F), the pH decreased from 7.04 (±0.1) to 6.09 (±0.04) over 14 days (data not shown).
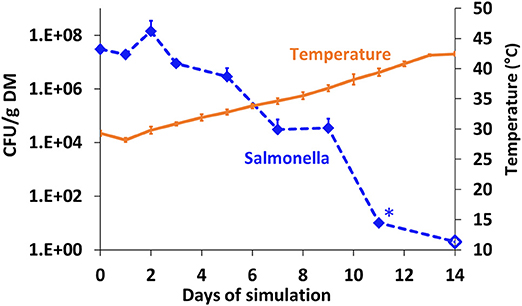
Figure 4. Persistence of S. Infantis in broiler litter (BL) during controlled anaerobic simulation in duplicate reactors maintained at mesophilic temperatures. The BL was pre-adjusted to a water content of 40% and initial pH 7, and the temperature increased to 42°C over a period of 14 days. Error bars represent the standard deviations of two reactors. Empty symbols indicate a value below the detection limit after enrichment (1–2 CFU g−1 dry matter); *values below the detection limit without enrichment (10–20 CFU g−1 dry matter). DM, dry matter.
Effect of Re-wetting on the Persistence of S. Infantis in BL
In addition to the effect of drying and re-wetting shown in the aerobic simulations (Figure 3), this effect was tested at 30°C in the static vessels for all water contents at pH 7 (Figures 1B,E,H) and for 40% water content only, also at pH 6 and 8.5 (Figures 1A,C). Regardless of the initial pH, adjusting the water content to 70% in BL that was previously incubated at 40 and 55% water content, resulted in a burst of Salmonella growth by 5–6 log10 CFU g−1 dry matter within the next 14 days (Figure 5–inset). During this period, no changes in Salmonella concentrations were observed in the BL samples which were initially incubated at a water content of 70%.
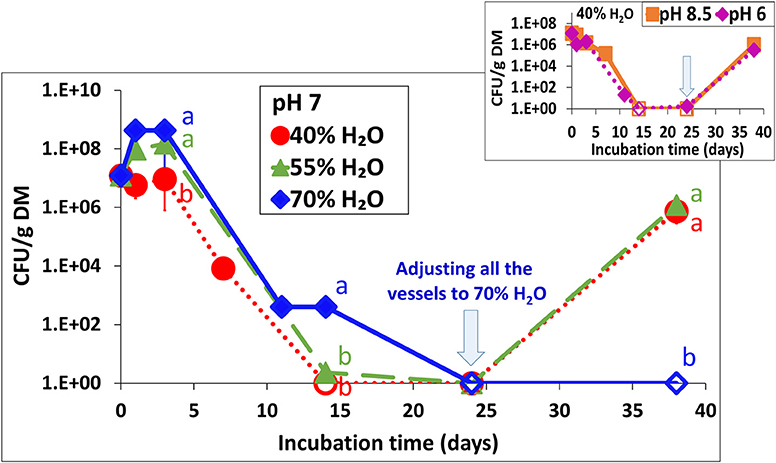
Figure 5. Persistence of S. Infantis in broiler litter (BL) following re-wetting of samples which were incubated for 24 days at 30°C and various water contents (as shown in Figure 1). The vessels were incubated for additional 14 days following adjustment of water content to 70% in BL that was initially incubated at 40 and 55% water content (initial pH 7) and in the BL that was initially incubated at 40% water content also for initial pH of 6 and 8.5 (inset). Error bars represent the standard deviations of triplicate vessels. Letters denote significant differences between treatments at time intervals (log-transformed CFU values; Tukey-Kramer HSD; P ≤ 0.05). Empty symbols indicate values below the detection limit after enrichment (1–2 CFU g−1 dry matter). DM, dry matter.
Potential Antagonistic Activity Against S. Infantis in BL
In suspensions containing autoclaved BL, Salmonella multiplied at a similar rate as in control medium without BL. From ca. 2 log10 CFU ml−1 it increased to 9.6 log10 CFU ml−1 within 20 h. On the other hand, Salmonella growth was completely inhibited in medium containing non-autoclaved BL (Figure 6A). These findings were repeated in another experiment using autoclaved BL from another farm (Figure 6A–inset). The effect of autoclaving was also observed in the BL itself (20–25°C; 60% water content). The concentration of Salmonella in the autoclaved BL increased from ca. 8 log10 to 9–10 log10 CFU g−1 dry matter within 11 days, both under aerobic and anaerobic conditions. On the other hand, Salmonella decreased to 3–6 log CFU g−1 dry matter in the non-autoclaved samples, with significantly more reduction under aerobic conditions for the un-stabilized BL (Figure 6B).
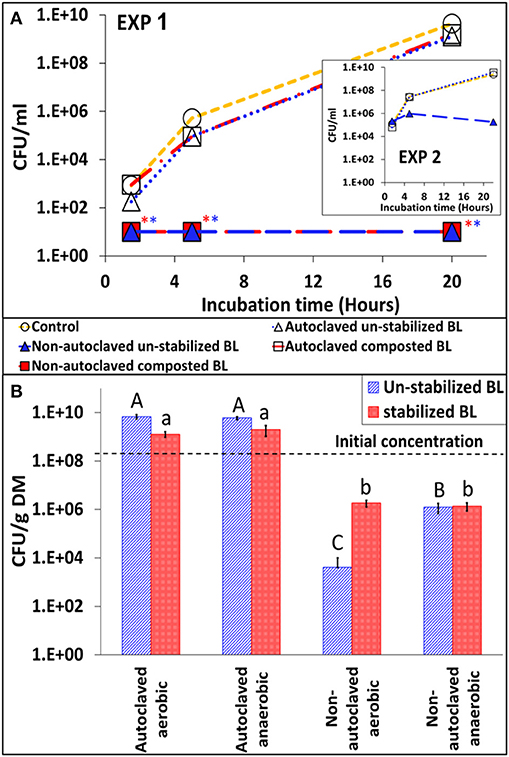
Figure 6. The efect of heat inactivation of indigenous microbial populations in broiler litter (BL) on S. Infantis multiplication and persistance. (A) Growth of Salmonella in nutrient broth suspension of autoclaved or non-autoclaved BL (un-stabilized and composted BL; initial Salmonella concentration ca. 2 log CFU ml−1). The inset figure shows the results of a similar experiment conducted with BL from another farm and at higher initial Salmonella concentration (ca. 4 log CFU ml−1). *Values below the detection limit without enrichment (10 CFU ml−1). (B) Persistence of Salmonella in the un-stabilized and stabilized BL itself, which were artificially contaminated with the pathogen and incubated for 11 days. Error bars represent the standard deviations of triplicate tubes. Letters denote significant differences between treatments by the end of the incubation period (log-transformed CFU values; Tukey-Kramer HSD; P ≤ 0.05). DM, dry matter.
The effect of BL amendment with antibiotics on the persistence of Salmonella is presented in Figure 7. In the un-amended treatment (no antibiotics), Salmonella multiplication was inhibited within 24 h, while no such inhibition occurred in the antibiotics-amended BL suspension and in the control media with or without antibiotics (no BL). After 24 h Salmonella concentration declined also in the antibiotics-amended BL (compared to the maximum value reached in the controls), yet the decline rate was lower than in the un-amended treatment.
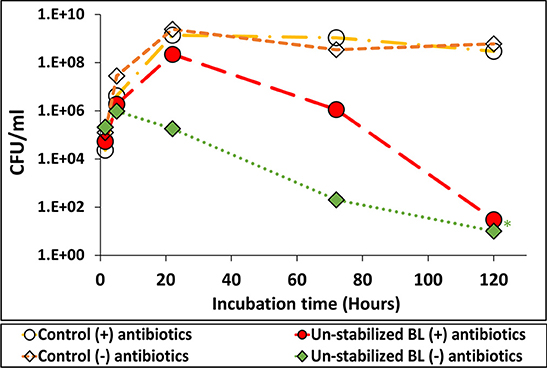
Figure 7. The efect of antibiotics-induced inactivation of indigenous microbial populations in broiler litter (BL) on S. Infantis multiplication. Growth of Salmonella in nutrient broth suspension of un-stabilized BL, which was amended or not amended with a cocktail of antibiotics to which the pathogen is resistant. *Values below the detection limit without enrichment (10 CFU ml−1).
Persistence of S. Infantis in BL-amended Soil
Persistence of Salmonella in soil amended with artificially-inoculated un-stabilized or stabilized BL is shown in Figure 8. Within 60 days, Salmonella concentration reduced by 2 log10 in the soil with stabilized BL and by 3–4 log10 in the soil with un-stabilized BL. Salmonella reduction was substantially slower at the higher water content (70 vs. 30% of field water capacity). After 90 days, the relatively dry soil samples (either with un-stabilized or stabilized BL) were also adjusted to 70% of water field capacity, which resulted in Salmonella increase by 2–3 log10 CFU g−1 dry matter, 15 days later. Control samples, without addition of S. Infantis, were free of Salmonella throughout the experiment.
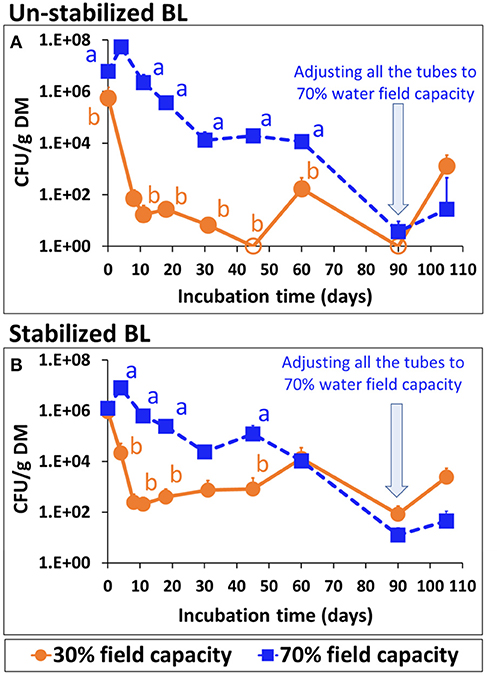
Figure 8. Persistence of S. Infantis in a vertisol soil amended with 5% (v/v) of un-stabilized or stabilized broiler liter (BL), adjusted to 30 or 70% water field capacity. (A) Un-stabilized BL. (B) Stabilized BL. Letters denote significant differences between treatments at time intervals (log-transformed CFU values; Tukey-Kramer HSD; P ≤ 0.05). Error bars represent the standard deviations of triplicate tubes. Empty symbols indicate values below the detection limit after enrichment (1–2 CFU g−1 DM). DM, dry matter.
Discussion
The persistence of Salmonella in poultry litter has been the subject of several studies that mainly focused on thermal inactivation (Williams and Benson, 1978; Wilkinson et al., 2011; Kim et al., 2012; Singh et al., 2012; Chen et al., 2013; Biswas et al., 2019). Most of them also demonstrated the effect of water content (Wilkinson et al., 2011; Kim et al., 2012; Singh et al., 2012; Chen et al., 2013), whereas the combined effect of multiple factors remained less understood. Beyond temperature, this study presents a more comprehensive investigation of various co-factors affecting the persistence of S. Infantis, an emerging pathogen associated with the poultry industry worldwide (Hindermann et al., 2017). These factors include water content, pH, drying and re-wetting, aerobic vs. anaerobic conditions, and potential antagonistic activity. The water content range was selected as being relevant for composting processes (Christian et al., 2009; Zakarya et al., 2018) and the range of initial pH was selected as being relevant for a spectrum of BL properties (Gordillo and Cabrera, 1997; Wood et al., 1999; Ekinci et al., 2000; Lopez-Mosquera et al., 2008). For practical reasons, four sources of BL were used in this comprehensive experimental scheme. Evidently, different sources may add additional factors to the already complex interactions among the co-factors examined in this study. Yet, these BL sources are from poultry growers in the same geographical region that use raising protocols of the biggest poultry cooperatives in Israel. Some of the variability shown in Table 1 regarding the main BL properties, may be related to the spatial variability within the poultry house, as well as the time of BL storage before each of the experiments which may vary as well.
Overall, the results indicate the significant role of several co-factors under mesophilic temperatures, in which Salmonella may persist longer, rather than under thermophilic temperatures (above 50°C in the present study) in which thermal inactivation is the main effective mechanism. The primary role of temperature is evident from the series of BL incubation experiments, in which 36 combinations of temperatures, water contents, and pH were tested in lab vessels (Figure 1). A 7 log10 reduction of Salmonella was achieved within 6.5–27.2 days at 30–40°C and within 1.2–4.7 days at 50–60°C (Table 2). Although the bags inside the vessels were loosely tied, we could not ensure aerobic conditions throughout the entire material. However, this setup is highly relevant for real scenarios since it is practically impossible to avoid anaerobic pockets during composting, especially in the core of windrow piles (Poulsen, 2011; Stegenta et al., 2019) and it is certainly relevant for static uncontrolled litter piles. Under thermophilic temperatures, the results of Singh et al. (2012) on poultry litter-based compost, are generally in agreement with the range of decay rates found in the present study. Thermal inactivation is anticipated to remain the primary factor affecting the persistence of Salmonella during stabilization and composting of BL. Yet, since most of the BL worldwide is still applied without controlled processing (Ogejo and Collins, 2009; Wilkinson et al., 2011; Wiedemann, 2015), the fate of Salmonella under mesophilic temperatures has more practical implications. Moreover, even composting may not ensure thermophilic conditions throughout the pile. In their study on poultry litter composting, Wilkinson et al. (2011) showed that only ca. 35–40% of the pile's cross-sectional area was exposed to temperatures higher than 55°C during the first 2 weeks. Similarly, in their study on composting of municipal sewage sludge in open piles, Stegenta et al. (2019) estimated that 69% of the pile volume did not reach a temperature of 60°C within 7–8 weeks. Also, Isobaev et al. (2014) showed that 24% of the temperature probes that were introduced randomly into a covered aerated static pile of biosolids, did not meet sanitation conditions within 4 weeks (55°C for 3 days). Such studies emphasize that composting treatment may not ensure exposure of all particles to thermophilic temperatures and durations that are needed for pathogen elimination.
A longer persistence, yet consistent decay of Salmonella in BL under mesophilic temperatures (a non-thermal inactivation), has been more scarcely reported. Previous studies suggested that thermal sensitivity of microorganisms increases with increasing water content (de Bertoldi, 1988; Wilkinson et al., 2011), but at a lower temperature, desiccation may play a greater role in pathogen inactivation (Wilkinson et al., 2011). After 24 h under mesophilic temperature (35°C), Wilkinson et al. (2011) found higher persistence of S. Typhimurium in poultry litter at a water content of 65% compared to 30%. The present study indicates that under mesophilic temperatures and favoring water contents, Salmonella may initially multiply in BL following inoculation (Figures 1, 5), as well as in BL-amended soil mixtures (Figure 8; 70% field water capacity), and then decay under all conditions. While the role of water content and initial pH was evident, there was no consistent effect with regards to these two factors. At 30°C, Salmonella decay rate was significantly higher at a lower water content. Likewise, Salmonella decayed substantially faster at 30 vs. 70% of water field capacity in soil-BL mixtures incubated at 30°C (Figure 8). In contrast, at 40°C, the effects of water content as well as initial pH were less consistent.
The varying effect of water content and pH and the dynamics of pH and EC during incubation (Figure 2 and Supplementary Figure 1), further emphasize the complication of predicting the role of co-factors that may synergistically affect Salmonella persistence. It may affect Salmonella directly by modifying the physico-chemical conditions favored by the pathogens, or indirectly by stimulating or inhibiting the activity of antagonistic populations. For example, high water content (70% in this study) may be associated with reduced air-filled porosity and the presence of anaerobic conditions. This, in turn, may affect the composition and density of antagonistic populations on one hand (biotic factor) and NH3 emissions on the other hand (abiotic factor). Nevertheless, although the release of NH3 was proposed as a factor that may inactivate Salmonella, the controlled simulations of the present study do not support this hypothesis. Salmonella decayed faster under anaerobic conditions (Figures 3A,B), during which NH3 emissions were negligible compared to those obtained under aerobic conditions (Figures 3C,D). The lower NH3 emissions under anaerobic conditions are expected due to the production of organic acids (Beffa et al., 1996; Naikwade et al., 2011), as evident also from the pH dynamics (Figures 3E,F). Thus, based on our findings, although aerobic processing of BL resulted in high levels of NH3, it was not a major factor in Salmonella inactivation under mesophilic temperatures. Notably, NH3 was shown to be effective as a process treatment designed to reduce enteric pathogens in livestock manure (Gurtler et al., 2018). Yet, this practice is based on the addition of high concentrations of liquid ammonia (Himathongkham and Riemann, 1999; Ottoson et al., 2008; Bolton et al., 2012) and not relied on the naturally associated NH3 emissions during manure stockpiling. Other studies also suggested the involvement of NH3 emission as a factor in the inactivation process (Kim et al., 2012; Chen et al., 2013, 2015). In these studies, inactivation was faster in more active samples (like fresh vs. aged manure), which also emitted more NH3; however, the factor of ammonia emission was not isolated.
The effect of BL drying and re-wetting on Salmonella persistence was evident from three different experiments. First, during composting simulations under aerobic conditions, the unintentional drying (from 40 to 14–23% water content) and the following re-wetting, resulted in rapid multiplication of Salmonella with 4 and 5 log10 increase in bacterial counts, at 40 and 50°C, respectively. This burst of growth was associated with increased thermal tolerance of the pathogen and a longer persistence. At 40°C Salmonella persisted more than 30 days following re-wetting (Figure 3A) compared to only 6–16 days in the static vessels experiment under equivalent temperature and water content (Figure 1 and Table 2). A second observation was obtained from the static vessels incubated at 30°C and water contents of 40 and 55%. Salmonella was reduced below the detection limit after enrichment; yet, re-wetting the BL to 70% water content, resulted in Salmonella increase by 5–6 log10 CFU within 14 days, irrespective to the initial pH (Figure 5). In the case of 55% water content, which is considered a suitable environment for the pathogen, the effect of water addition on Salmonella multiplication may suggest that the pathogen was dehydrated locally during the first phase of incubation. Finally, a third observation was obtained in soil-BL mixtures, in which the increase of field water capacity from 30 to 70% resulted in Salmonella multiplication within the following 15 days (Figure 8).
Our findings regarding the increased tolerance of Salmonella to high temperatures (50–60°C), are concurrent with previous studies. Increased tolerance of Salmonella to stress due to desiccation was investigated in pure culture (Gruzdev et al., 2011, 2012) as well as in poultry litter (Chen et al., 2013). In the study of Chen et al. (2013), a 5 log10 CFU reduction of desiccation-adapted cells in aged chicken litter of 20% moisture, required >6, >6, 4–5, and 3–4 h, compared to 1.5–2, 1–1.5, 0.5–1, and <0.5 h, for the non-adapted cells, at 70, 75, 80, and 85°C, respectively. Gruzdev et al. (2011) found that desiccated cells demonstrated high tolerance to a 1-h exposure to dry heat, with no substantial change in their viable counts at 60°C compared to their initial pre-challenge count and 1.5- and 3.1 log10 reductions at 80 and 100°C, respectively. In contrast, non-desiccated cells were highly susceptible to heat, with as much as a 3 log10 CFU reduction at 60°C and an 8 log10 reduction (below the detection limit) following 1-h incubation at 80 and 100°C. A reasonable mechanism to the increased tolerance of desiccated bacteria to high temperatures, is that very low water content in bacterial cells can inhibit or diminish the protein denaturation induced by high-temperature heating through vibration of water molecules to break S-S and hydrogen bonds of intracellular proteins. Thus, desiccation prevents the bacteria from denaturation of the membrane proteins and preserving their integrity even during exposure to a very high temperature (Earnshaw et al., 1995; Archer et al., 1998; Hiramatsu et al., 2005).
The increased thermal tolerance following re-wetting as observed in the present study has important implications. BL may dry before soil application and then be re-wetted by rain or field irrigation. The BL may also dry before composting, or any short phase of thermal stabilization, which may increase the chance of ineffective thermal inactivation. The increased stress-tolerance of desiccated cells may also be meaningful with regards to salinity. Due to organic matter oxidation (volatilization) and increased ash content, the salinity of BL increases during composting as shown in Supplementary Figure 1. Gruzdev et al. (2011) showed that desiccated Salmonella cells were able to maintain their viability in 1 to 5% bile salts and 0.1–0.5 M NaCl, while the number of the non-desiccated cells continuously declined in a dose-dependent manner. The increased tolerance to pH changes may also play a role, as the pH dynamics can vary substantially during BL processing (Figure 2). In this case, Gruzdev et al. (2012) showed that the survival of dehydrated Salmonella was maximal at pH 8.0 and decreased at lower or higher pH values, while that of the non-dehydrated cells was higher at the various pH values.
Out of the co-factors investigated in this study, the involvement of Salmonella antagonistic microorganisms (SAM) seems to play a major role in Salmonella inactivation by non-thermal mechanisms under mesophilic temperatures. Three different experiments provided indirect evidence that SAM activity was the primary mechanism for non-thermal inactivation of S. Infantis. Heat inactivation of the indigenous microbial populations of BL (Figure 6) or the addition of antibiotics to which the S. Infantis is resistant (Figure 7) resulted in augmentation of Salmonella multiplication, suggesting that raw BL contains microbial antagonists, susceptible to heat and antibiotics, which inhibit the growth of Salmonella. In the case of antibiotics addition, this effect was lessened in the following days, presumably due to degradation of the antibiotics. Notably, the potential effect of SAM inactivation was shown both in liquid suspensions of BL (Figures 6A, 7) and under aerobic and anaerobic conditions in the BL itself (Figure 6B), using different BL sources and degree of stabilization. Under anaerobic conditions, Salmonella may be inactivated also by the presence of volatile fatty acids (VFAs) that are produced during anaerobic digestion (Kunte et al., 1998; Salsali et al., 2006; Jiang et al., 2018). Yet, as shown in Figure 6B, Salmonella persisted longer in the non-autoclaved un-stabilized BL under anaerobic than under aerobic conditions, such that the effect of VFAs in this case seems unlikely. This varying magnitude of the potential effect of SAM can be related to the different experimental systems tested along this study but also due to the use of different BL sources and storage time. As suggested by Bucher et al. (2020), multiple factors and particularly physico-chemical variables are associated with litter microbiome succession. Yet, the authors emphasized the critical role of litter moisture and pH on bacterial diversity, which were pre-adjusted in all experiments of the present study. Moreover, heat inactivation by means of autoclaving was shown to facilitate the later proliferation of some heat-resistant bacterial populations in soil (Baker et al., 2020) and compost (Kim et al., 2011); yet the diversity of microbial populations after autoclaving is expected to be much lower before reaching a new equilibrium (Baker et al., 2020). Thus, although the water content and initial pH were pre-adjusted before each experiment, we cannot assess potential differences in Salmonella antagonistic populations. Moreover, we could not obtain specific information from the broiler growers about the possible use of antibiotics during the growing period, and we cannot assess the long-term impact of such use on BL microbiome.
The effect of antagonistic microorganisms on the fate of pathogens during composting of livestock manure and biosolids was suggested previously, based on a small number of observations (Millner et al., 1987; Sidhu et al., 2001; Jiang et al., 2002; Szala and Paluszak, 2008; Wilkinson et al., 2011; Weinberg et al., 2014). Similarly to the present study, Sidhu et al. (2001) showed the effect of sterilization of biosolids compost, in which S. Typhimurium multiplied rapidly as compared to non-sterilized compost in which Salmonella was suppressed. Likewise, Jiang et al. (2002) showed a longer persistence of E. coli in manure-soil mixtures, in which the soil was sterilized, compared to a mixture of non-sterilized soil. Following Salmonella decay in the present study, either by physico-chemical or presumably biological mechanisms, no regrowth was observed under mesophilic temperatures, besides those cases of BL re-wetting. These findings support the possibility that re-wetting is a major process inducing Salmonella regrowth. Then, if a Salmonella-contaminated BL or any BL-based organic fertilizer is applied, the pathogen may survive in the soil for several months, presumably due to a reduced antagonistic activity in the soil environment. Furthermore, multiplication of Salmonella may also be augmented upon soil wetting. Evidently, the fate of Salmonella will be further governed by horizontal and vertical transport mechanisms related to various soil properties, crop root systems, agricultural practice, and rainfall (Mawdsley et al., 1995; Islam et al., 2004a), as well as to the dynamics of SAM due to the same factors. Finally, the initial Salmonella concentrations used along this study (ca. 7 log10 g−1 dry mater) and the calculations regarding the number of days required to reduce S. Infantis below the detection limit, are relevant to real scenarios. Salmonella concentrations in the range of 3–5 log10 g−1 litter have been reported in the literature (Chinivasagam et al., 2009, 2010; Brooks et al., 2010); moreover, as shown in the present study, Salmonella may multiply in the litter or following soil application, and increase by several orders of magnitude.
Conclusions
Thermal inactivation is the primary mechanism of S. Infantis elimination in BL. Thus, composting or any thermal processing is expected to minimize the risk of Salmonella contamination upon soil application. In contrast, under mesophilic temperatures, other co-factors may play a significant role, including water content, pH, drying and re-wetting, aerobic vs. anaerobic conditions, and the presence of SAM. Although Salmonella persistence may be reduced at lower BL water content under mesophilic conditions, desiccation and re-wetting is critical, and increases the risk of Salmonella transfer from the poultry environment to soil and crops. Following soil application, Salmonella may persist for several months due to reduced antagonistic activity compared to the BL alone and retain its ability to multiply upon soil re-wetting. Desiccation and re-wetting of BL not only lead to augmentation of Salmonella multiplication, but may also increase its tolerance to thermophilic temperatures. The role of SAM under mesophilic temperatures is meaningful both under aerobic and anaerobic conditions with no clear effect of NH3 emissions. These effects, at varying magnitudes, are expected to appear in BL from different sources, both un-stabilized, as well as stabilized or composted BL in which Salmonella persisted after treatment. Future studies are needed to elucidate the mode of action of SAM in BL and soil. Such studies may ultimately assist with selecting the BL-processing conditions under which SAM activity is most effective or by modulation of the BL/soil microbiome toward augmentation of SAM activity.
Data Availability Statement
The original contributions presented in the study are included in the article/Supplementary Material, further inquiries can be directed to the corresponding author/s.
Author Contributions
RA: conceptualization, methodology, data analysis, and writing original draft. VV and AL: conceptualization and methodology. IS: methodology and resources. AH: methodology. SS: conceptualization and reviewing. YC: supervision and reviewing. YL: supervision, conceptualization, writing, reviewing, and editing. All authors: contributed to the article and approved the submitted version.
Funding
This study was supported by the Chief Scientist of the Israel Ministry of Agriculture and Rural Development (Project title: Mitigation of Salmonella and Campylobacter contamination in poultry, No. 20-14-0032); and the Division of Poultry at the Israel Ministry of Agriculture and Rural Development (Project title: Composting of poultry carcasses in closed sleeves inside the poultry house—a contingency solution for mass mortality events, No. 356-1111-17).
Conflict of Interest
The authors declare that the research was conducted in the absence of any commercial or financial relationships that could be construed as a potential conflict of interest.
Supplementary Material
The Supplementary Material for this article can be found online at: https://www.frontiersin.org/articles/10.3389/fsufs.2021.645721/full#supplementary-material
Supplementary Figure 1. Electrical conductivity (EC) dynamics during incubation of broiler litter (BL) at different temperatures (30, 40, 50, and 60°C), water content (40, 55, and 70%) and initial pH (6, 7, and 8.5). The different incubation temperatures were applied during phase I (weeks 1–2), while at phase II (weeks 3–4) the incubation temperature was 30°C. Error bars represent the standard deviations of triplicate vessels. Letters denote significant differences between treatments at time intervals (Tukey-Kramer HSD; P ≤ 0.05). The figures are grouped according to water contents of 40% (A–C), 55% (D–F), and 70% (G–I).
References
Alkoaik, F. N. (2019). Integrating aeration and rotation processes to accelerate composting of agricultural residues. PLoS ONE 14:e0220343. doi: 10.1371/journal.pone.0220343
Archer, J., Jervis, E. T., Bird, J., and Gaze, J. E. (1998). Heat resistance of Salmonella weltevreden in low-moisture environments. J. Food Prot. 61, 969–973. doi: 10.4315/0362-028X-61.8.969
Avidov, R., Saadi, I., Krasnovsky, A., Medina, S., Raviv, M., Chen, Y., et al. (2018). Using polyethylene sleeves with forced aeration for composting olive mill wastewater pre-absorbed by vegetative waste. Waste Manag. 78, 969–979. doi: 10.1016/j.wasman.2018.06.021
Avidov, R., Saadi, I., Krassnovsky, A., Hanan, A., Medina, S., Raviv, M., et al. (2017). Composting municipal biosolids in polyethylene sleeves with forced aeration: process control, air emissions, sanitary and agronomic aspects. Waste Manag. 67, 32–42. doi: 10.1016/j.wasman.2017.05.035
Avidov, R., Saadi, I., Varma, V. S., Khoury, O., Kfir, R., Hanan, A., et al. (2019). Composting of Poultry Carcasses in Closed Sleeves Inside the Chicken Coops - A “Contingency Solution” for Mass Mortality Events in Chicken Coops. Meshek Ha'Ofot. 82–88. http://snapcall.org/meshek/201904/index.html#p=82 (in Hebrew, accessed on May 18, 2020).
Aviv, G., Tsyba, K., Steck, N., Salmon-Divon, M., Cornelius, A., Rahav, G., et al. (2014). A unique megaplasmid contributes to stress tolerance and pathogenicity of an emergent Salmonella enterica serovar Infantis strain. Environ. Microbiol. 16, 977–994. doi: 10.1111/1462-2920.12351
Baker, C. A., Lee, S., De, J., Jeong, K. C., and Schneider, K. R. (2020). Survival of Escherichia coli O157 in autoclaved and natural sandy soil mesocosms. PLoS ONE 15:e0234562. doi: 10.1371/journal.pone.0234562
Beffa, T., Blanc, M., Marilley, L., Fischer, J. L., Lyon, P. F., and Aragno, M. (1996). “Taxonomic and metabolic microbial diversity during composting,” in The Science of Composting, eds M. de Bertoldi, P. Sequi, B. Lemmes, and T. Papi (Dordrecht: Springer), 149–161. doi: 10.1007/978-94-009-1569-5_16
Bell, R. L., Zheng, J., Burrows, E., Allard, S., Wang, C. Y., Keys, C. E., et al. (2015). Ecological prevalence, genetic diversity, and epidemiological aspects of Salmonella isolated from tomato agricultural regions of the Virginia Eastern Shore. Front. Microbiol. 6:415. doi: 10.3389/fmicb.2015.00415
Beuchat, L. R. (2002). Ecological factors influencing survival and growth of human pathogens on raw fruits and vegetables. Microb. Infect. 4, 413–423. doi: 10.1016/S1286-4579(02)01555-1
Biswas, S., Nazmi, A., Pitesky, M., Gallardo, R., and Pandey, P. (2019). Thermal inactivation of Escherichia coli and Salmonella typhimurium in poultry carcass and litter at thermophilic temperatures. J. Appl. Poult. Res. 28, 307–317. doi: 10.3382/japr/pfy072
Bolan, N. S., Szogi, A. A., Chuasavathi, T., Seshadri, B., Rothrock, M. J., and Panneerselvam, P. (2010). Uses and management of poultry litter. Worlds. Poult. Sci. J. 66, 673–698. doi: 10.1017/S0043933910000656
Bolton, D. J., Ivory, C., and McDowell, D. A. (2012). The effect of urea and ammonia treatments on the survival of Salmonella spp. and Yersinia enterocolitica in pig slurry. J. Appl. Microbiol. 114:134–140. doi: 10.1111/jam.12003
Brooks, J. P., McLaughlin, M. R., Scheffler, B., and Miles, D. M. (2010). Microbial and antibiotic resistant constituents associated with biological aerosols and poultry litter within a commercial poultry house. Sci. Total Environ. 408, 4770–4777. doi: 10.1016/j.scitotenv.2010.06.038
Bryan, F. L., and Doyle, M. P. (1995). Health risks and consequences of Salmonella and Campylobacter jejuni in raw poultry. J. Food Prot. 58, 326–344.
Bucher, M. G., Zwirzitz, B., Oladeinde, A., Cook, K., Plymel, C., Zock, G., et al. (2020). Reused poultry litter microbiome with competitive exclusion potential against Salmonella Heidelberg. J. Environ. Qual. 49, 869–881. doi: 10.1002/jeq2.20081
Chaves, R. D., Martinez, R. C. R., Rezende, A. C. B., Rocha, M. D., Oteiza, J. M., Sant'Ana, A., et al. (2016). “Salmonella and Listeria monocytogenes in ready-to-eat leafy vegetables,” in Food Hygiene and Toxicology in Ready to Eat Foods, ed P. Kotzekidou (New York, NY: Academic Press), 123–149. doi: 10.1016/B978-0-12-801916-0.00008-X
Chen, Z., Diao, J., Dharmasena, M., Ionita, C., Jiang, X., and Rieck, J. (2013). Thermal inactivation of desiccation-adapted Salmonella spp. in aged chicken litter. Appl. Environ. Microbiol. 79, 7013–7020. doi: 10.1128/AEM.01969-13
Chen, Z., and Jiang, X. (2014). Microbiological safety of chicken litter or chicken litter-based organic fertilizers: a review. Agriculture 4, 1–29. doi: 10.3390/agriculture4010001
Chen, Z., Wang, H., Ionita, C., Luo, F., and Jiang, X. (2015). Effects of chicken litter storage time and ammonia content on thermal resistance of desiccation-adapted Salmonella spp. Appl. Environ. Microbiol. 81, 6883–6889. doi: 10.1128/AEM.01876-15
Chinivasagam, H. N., Redding, M., Runge, G., and Blackall, P. J. (2010). Presence and incidence of food-borne pathogens in australian chicken litter. Br. Poult. Sci. 51, 311–318. doi: 10.1080/00071668.2010.499424
Chinivasagam, H. N., Tran, T., Maddock, L., Gale, A., and Blackall, P. J. (2009). Mechanically ventilated broiler sheds: a possible source of aerosolized Salmonella, Campylobacter, and Escherichia coli. Appl Environ Microbiol. 75, 7417–7425. doi: 10.1128/AEM.01380-09
Christian, A. H., Evanylo, G. K., and Pease, J. W. (2009). On Farm Composting - A Guide to Principles, Planning and Operations. Virginia Cooperative Extension Publications No. 452-232. Petersburg, VA: Virginia State University.
Corrier, D. E., Byrd, J. A., Hargis, B. M., Hume, M. E., Bailey, R. H., and Stanker, L. H. (1999). Presence of Salmonella in the crop and ceca of broiler chickens before and after preslaughter feed withdrawal. Poult. Sci. 78, 45–49. doi: 10.1093/ps/78.1.45
Earnshaw, R. G., Appleyard, J., and Hurst, R. M. (1995). Understanding physical inactivation processes: combined preservation opportunities using heat, ultrasound and pressure. Int. J. Food Microbiol. 28, 197–219. doi: 10.1016/0168-1605(95)00057-7
Ekinci, K., Keener, H. M., and Elwell, D. L. (2000). Composting short paper fiber with broiler litter and additives: part I: effects of initial pH and carbon/nitrogen ratio on ammonia emission. Compost Sci. Util. 8, 160–172. doi: 10.1080/1065657X.2000.10701761
Erickson, M. C., Liao, J., Boyhan, G., Smith, C., Ma, L., Jiang, X., et al. (2010). Fate of manure-borne pathogen surrogates in static composting piles of chicken litter and peanut hulls. Bioresour. Technol. 101, 1014–1020. doi: 10.1016/j.biortech.2009.08.105
Fatica, M. K., and Schneider, K. R. (2011). Salmonella and produce: survival in the plant environment and implications in food safety. Virulence 2, 573–579. doi: 10.4161/viru.2.6.17880
Gordillo, R. M., and Cabrera, M. L. (1997). Mineralizable nitrogen in broiler litter: I. Effect of selected litter chemical characteristics. J. Environ. Qual. 26, 1672–1679. doi: 10.2134/jeq1997.00472425002600060030x
Gould, L. H., Mungai, E. A., Johnson, S. D., Richardson, L. C., Williams, I. T., Griffin, P. M., et al. (2013). Surveillance for foodborne disease outbreaks—United States, 2009–2010. MMWR Morb. Mortal. Wkly. Rep. 62:41.
Gruzdev, N., Pinto, R., and Sela Saldinger, S. (2012). Persistence of Salmonella enterica during dehydration and subsequent cold storage. Food Microbiol. 32, 415–422. doi: 10.1016/j.fm.2012.08.003
Gruzdev, N., Pinto, R., and Sela, S. (2011). Effect of desiccation on tolerance of Salmonella enterica to multiple stresses. Appl Environ Microbiol. 77, 1667–1673. doi: 10.1128/AEM.02156-10
Gu, G., Strawn, L. K., Oryang, D. O., Zheng, J., Reed, E. A., Ottesen, A. R., et al. (2018). Agricultural practices influence Salmonella contamination and survival in pre-harvest tomato production. Front. Microbiol. 9:2451. doi: 10.3389/fmicb.2018.02451
Gurtler, J. B., Doyle, M. P., Erickson, M. C., Jiang, X., Millner, P., and Sharma, M. (2018). Composting to inactivate foodborne pathogens for crop soil application: a review. J. Food Prot. 81, 1821–1837. doi: 10.4315/0362-028X.JFP-18-217
Herman, K. M., Hall, A. J., and Gould, L. H. (2015). Outbreaks attributed to fresh leafy vegetables, United States, 1973–2012. Epidemiol. Infect. 143, 3011–3021. doi: 10.1017/S0950268815000047
Himathongkham, S., and Riemann, H. (1999). Destruction of Salmonella typhimurium, Escherichia coli O157:H7 and Listeria monocytogenes in chicken manure by drying and/or gassing with ammonia. FEMS Microbiol. Lett. 171, 179–182. doi: 10.1111/j.1574-6968.1999.tb13430.x
Hindermann, D., Gopinath, G., Chase, H., Negrete, F., Althaus, D., Zurfluh, K., et al. (2017). Salmonella enterica serovar Infantis from food and human infections, Switzerland, 2010–2015: poultry-related multidrug resistant clones and an emerging ESBL producing clonal lineage. Front. Microbiol. 8:1322. doi: 10.3389/fmicb.2017.01322
Hiramatsu, R., Matsumoto, M., Sakae, K., and Miyazaki, Y. (2005). Ability of Shiga toxin-producing Escherichia coli and Salmonella spp. to survive in a desiccation model system and in dry foods. Appl. Environ. Microbiol. 71, 6657–6663. doi: 10.1128/AEM.71.11.6657-6663.2005
Hruby, C. E., Soupir, M. L., Moorman, T. B., Pederson, C., and Kanwar, R. (2018). Salmonella and fecal indicator bacteria survival in soils amended with poultry manure. Water. Air. Soil Pollut. 229:32. doi: 10.1007/s11270-017-3667-z
Islam, M., Morgan, J., Doyle, M. P., Phatak, S. C., Millner, P., and Jiang, X. (2004a). Fate of Salmonella enterica serovar typhimurium on carrots and radishes grown in fields treated with contaminated manure composts or irrigation water. Appl. Environ. Microbiol. 70, 2497–2502. doi: 10.1128/AEM.70.4.2497-2502.2004
Islam, M., Morgan, J., Doyle, M. P., Phatak, S. C., Millner, P., and Jiang, X. (2004b). Persistence of Salmonella enterica serovar Typhimurium on lettuce and parsley and in soils on which they were grown in fields treated with contaminated manure composts or irrigation water. Foodborne Pathog. Dis. 1, 27–35. doi: 10.1089/153531404772914437
Isobaev, P., Bouferguene, A., Wichuk, K. M., and McCartney, D. (2014). An enhanced compost temperature sampling framework: case study of a covered aerated static pile. Waste Manag. 34, 1117–1124. doi: 10.1016/j.wasman.2014.03.016
Jechalke, S., Schierstaedt, J., Becker, M., Flemer, B., Grosch, R., Smalla, K., et al. (2019). Salmonella establishment in agricultural soil and colonization of crop plants depend on soil type and plant species. Front. Microbiol. 10:967. doi: 10.3389/fmicb.2019.00967
Jiang, X., Morgan, J., and Doyle, M. P. (2002). Fate of Escherichia coli O157: H7 in manure-amended soil. Appl. Environ. Microbiol. 68, 2605–2609. doi: 10.1128/AEM.68.5.2605
Jiang, Y., Dennehy, C., Lawlor, P. G., Hu, Z., Yang, Q., McCarthy, G., et al. (2018). Inactivation of Salmonella during dry co-digestion of food waste and pig manure. Waste Manag. 82, 231–240. doi: 10.1016/j.wasman.2018.10.037
Jones, F., Axtell, R., and Rives, D. (1991). A survey of Salmonella contamination in modern broiler production. J. Food Protect. 54, 502–507. doi: 10.4315/0362-028X-54.7.502
Kim, J., Diao, J., Shepherd, M. W., Singh, R., Heringa, S. D., Gong, C., et al. (2012). Validating thermal inactivation of Salmonella spp. in fresh and aged chicken litter. Appl. Environ. Microbiol. 78, 1302–1307. doi: 10.1128/AEM.06671-11
Kim, J., Miller, C. M., Shepherd, M. W. Jr., Liu, X., and Jiang, X. (2011). Impact of indigenous microorganisms on Escherichia coli O157: H7 growth in cured compost. Bioresour. Technol. 102, 9619–9625. doi: 10.1016/j.biortech.2011.07.055
Kunte, D. P., Yeole, T. Y., Chiplonkar, S. A., and Ranade, D. R. (1998). Inactivation of Salmonella typhi by high levels of volatile fatty acids during anaerobic digestion. J. Appl. Microbiol. 84, 138–142. doi: 10.1046/j.1365-2672.1997.00335.x
Lin, Y., van Santen, E., and Watts, D. (2016). “The effect of poultry litter application on agricultural production: a meta-analysis of crop yield, nutrient uptake and soil fertility,” in Proceedings of the 28th Annual Conference on Applied Statistics in Agriculture, 162–175. doi: 10.4148/2475-7772.1494
Liu, T. S., Snoeyenbos, G. H., and Carlson, V. L. (1969). The effect of moisture and storage temperature on a Salmonella Senftenburg 775W population in meat and bone meal. Poult. Sci. 48, 1628–1633. doi: 10.3382/ps.0481628
Lopez-Mosquera, M. E., Cabaleiro, F., Sainz, M. J., López-Fabal, A., and Carral, E. (2008). Fertilizing value of broiler litter: effects of drying and pelletizing. Bioresour. Technol. 99, 5626–5633. doi: 10.1016/j.biortech.2007.10.034
Macklin, K. S., Hess, J. B., and Bilgili, S. F. (2008). In-house windrow composting and its effects on foodborne pathogens. J. Appl. Poult. Res. 17, 121–127. doi: 10.3382/japr.2007-00051
Mawdsley, J. L., Bardgett, R. D., Merry, R. J., Pain, B. F., and Theodorou, M. K. (1995). Pathogens in livestock waste, their potential for movement through soil and environmental pollution. Appl. Soil Ecol. 2, 1–15. doi: 10.1016/0929-1393(94)00039-A
Millner, P. D., Powers, K. E., Enkiri, N. K., and Burge, W. D. (1987). Microbially mediated growth suppression and death of Salmonella in composted sewage sludge. Microb. Ecol. 14, 255–265. doi: 10.1007/BF02012945
Naikwade, P., Mogle, U., and Jadhav, B. (2011). Comparative study of aerobic and anaerobic composts prepared from autumn leaves on Zea mays L. Sci. Res. Rep. 1, 77–82.
Oazana, S., Naor, M., Grinshpun, J., Halachmi, I., Raviv, M., Saadi, I., et al. (2018). A flexible control system designed for lab-scale simulations and optimization of composting processes. Waste Manag. 72, 150–160. doi: 10.1016/j.wasman.2017.11.029
Oazana, S., Varma, V. S., Saadi, I., Sharma, D., Hanan, A., Medina, S., et al. (2020). High-rate stabilization and associated air emissions prospected during on-site in-vessel sewage sludge composting. Bioresour. Technol. Rep. 11:100543. doi: 10.1016/j.biteb.2020.100543
Ogejo, J. A., and Collins, E. R. (2009). Storing and Handling Poultry Litter. Virginia Cooperative Extension Publication, 442–054.
Ottoson, J., Nordin, A., von Rosen, D., and Vinnerås, B. (2008). Salmonella reduction in manure by the addition of urea and ammonia. Bioresour. Technol. 99, 1610–1615. doi: 10.1016/j.biortech.2007.04.009
Poulsen, T. G. (2011). Oxygen and carbon c distribution and movement in passively aerated compost piles. Compost Sci. Util. 19, 25–32. doi: 10.1080/1065657X.2011.10736973
Reynnells, R., Ingram, D. T., Roberts, C., Stonebraker, R., Handy, E. T., Felton, G., et al. (2014). Comparison of U.S. environmental protection agency and U.S. composting council microbial detection methods in finished compost and regrowth potential of Salmonella spp. and Escherichia coli O157:H7 in finished compost. Foodborne Pathog. Dis. 11, 555–567. doi: 10.1089/fpd.2013.1698
Salsali, H. R., Parker, W. J., and Sattar, S. A. (2006). Impact of concentration, temperature, and pH on inactivation of Salmonella spp. by volatile fatty acids in anaerobic digestion. Can. J. Microbiol. 52, 279–286. doi: 10.1139/W05-125
Sánchez, Ó. J., Ospina, D. A., and Montoya, S. (2017). Compost supplementation with nutrients and microorganisms in composting process. Waste Manag. 69, 136–153. doi: 10.1016/j.wasman.2017.08.012
Sidhu, J., Gibbs, R. A., Ho, G. E., and Unkovich, I. (2001). The role of indigenous microorganisms in suppression of Salmonella regrowth in composted biosolids. Water Res. 35, 913–920. doi: 10.1016/S0043-1354(00)00352-3
Singh, R., Kim, J., and Jiang, X. (2012). Heat inactivation of Salmonella spp. in fresh poultry compost by simulating early phase of composting process. J. Appl. Microbiol. 112, 927–935. doi: 10.1111/j.1365-2672.2012.05268.x
Stegenta, S., Sobieraj, K., Pilarski, G., Koziel, J. A., and Białowiec, A. (2019). Analysis of the spatial and temporal distribution of process gases within municipal biowaste compost. Sustain 11:2340. doi: 10.3390/su11082340
Szala, B., and Paluszak, Z. (2008). Validation of biodegradable waste composting process based on the inactivation of Salmonella Senftenberg W775. Polish J. Environ. Stud. 17, 79–85.
Tewolde, H., Adeli, A., Rowe, D. E., and Sistani, K. R. (2011). Cotton lint yield improvement attributed to residual effect of repeated poultry litter application. Agron. J. 103, 107–112. doi: 10.2134/agronj2010.0274
Tewolde, H., Sistani, K. R., and Adeli, A. (2013). Fall- and spring-applied poultry litter effectiveness as corn fertilizer in the mid-southern United States. Agron. J. 105, 1743–1748. doi: 10.2134/agronj2013.0137
Trampel, D. W., Hasiak, R. J., Hoffman, L. J., and Debey, M. C. (2000). Recovery of Salmonella from water, equipment, and carcasses in turkey processing plants. J. Appl. Poult. Res. 9, 29–34. doi: 10.1093/japr/9.1.29
United States Environmental Protection Agency (2003). Control of Pathogens and Vector Attraction in Sewage Sludge. United States Environmental Protection Agency (USEPA). doi: 10.1016/S0015-1882(99)80035-4
Weinberg, Z. G., Szakacs, G., Chen, Y., Pinto, R., Bernstein, S., Konya, B., et al. (2014). Inhibition of Escherichia coli in cultivated cattle manure. J. Anim. Sci. 92, 2336–2341. doi: 10.2527/jas.2013-7285
Wichuk, K. M., and McCartney, D. (2007). A review of the effectiveness of current time-temperature regulations on pathogen inactivation during composting. J. Environ. Eng. Sci. 6, 573–586. doi: 10.1139/S07-011
Wiedemann, S. G. (2015). Land Application of Chicken Litter: A Guide for Users. Canberra, ACT: Rural Industries Research and Development Corporation.
Wilkinson, K. G., Tee, E., Tomkins, R. B., Hepworth, G., and Premier, R. (2011). Effect of heating and aging of poultry litter on the persistence of enteric bacteria. Poult. Sci. 90, 10–18. doi: 10.3382/ps.2010-01023
Williams, J. E., and Benson, S. T. (1978). Survival of Salmonella typhimurium in poultry feed and litter at three temperatures. Avian Dis. 742–747.
Willis, R. B., Montgomery, M. E., and Allen, P. R. (1996). Improved method for manual, colorimetric determination of total kjeldahl nitrogen using salicylate. J. Agric. Food Chem. 44, 1804–1807. doi: 10.1021/jf950522b
Wood, B. H., Wood, C. W., Yoo, K. H., Yoon, K. S., and Delaney, D. P. (1999). Seasonal surface runoff losses of nutrients and metals from soils fertilized with broiler litter and commercial fertilizer. J. Environ. Qual. 28, 1210–1218. doi: 10.2134/jeq1999.00472425002800040023x
Keywords: poultry litter, poultry manure, antagonistic microorganisms, thermal inactivation, pathogen elimination, zoonotic microorganisms, fresh produce contamination, field crops contamination
Citation: Avidov R, Varma VS, Saadi I, Hanan A, Lublin A, Saldinger SS, Chen Y and Laor Y (2021) Factors Influencing the Persistence of Salmonella Infantis in Broiler Litter During Composting and Stabilization Processes and Following Soil Incorporation. Front. Sustain. Food Syst. 5:645721. doi: 10.3389/fsufs.2021.645721
Received: 23 December 2020; Accepted: 16 March 2021;
Published: 20 April 2021.
Edited by:
Jesus Fernandez Bayo, University of California, Davis, United StatesReviewed by:
Arturo F. Chica, University of Cordoba, SpainJuliano Toniato, University of California, Davis, United States
Copyright © 2021 Avidov, Varma, Saadi, Hanan, Lublin, Saldinger, Chen and Laor. This is an open-access article distributed under the terms of the Creative Commons Attribution License (CC BY). The use, distribution or reproduction in other forums is permitted, provided the original author(s) and the copyright owner(s) are credited and that the original publication in this journal is cited, in accordance with accepted academic practice. No use, distribution or reproduction is permitted which does not comply with these terms.
*Correspondence: Yael Laor, laor@volcani.agri.gov.il