Establishment of a Real-Time Recombinase Polymerase Amplification Assay for the Detection of Avian Reovirus
- 1School of Biotechnology and Food Engineering, Anyang Institute of Technology, Anyang, China
- 2Henan Provincial Engineering Laboratory of Insects Bio-reactor, China-UK-NYNU-RRes Joint Libratory of Insect Biology, Nanyang Normal University, Nanyang, China
- 3Guangdong Key Laboratory of Laboratory Animals, Guangdong Laboratory Animals Monitoring Institute, Guangzhou, China
- 4Henan Joint International Research Laboratory of Veterinary Biologics Research and Application, Academician Workstation of Animal Disease Control and Nutrition Immunity in Henan Province, Anyang, China
Avian reovirus (ARV) infection results in multiple disease manifestations in chicken. A rapid detection method will contribute to early diagnosis and control of the virus infection. The recombinase polymerase amplification (RPA) technology is a nucleic acid amplification method which is experiencing rapid development. In present study, a real-time reverse transcription (RT)-RPA assay was developed for the detection of ARV. The limit of detection of the real-time RT-RPA was 102 copies/μL of ARV genomic RNA standard in 95% of cases. The RT-RPA assay also exhibited remarkable specificity. When the nucleic acids of CRV and other common avian pathogens were subjected to the RT-RPA test, only ARV tested positive, all the other pathogens tested negative. Furthermore, the practicality of the RT-RPA assay in field was confirmed by testing 86 clinical samples. The clinical samples were also detected by qRT-PCR. The detection result by RT-RPA was 96.5% agreement with that of qRT-PCR. As a result of the simplicity and convenience of the assay with high sensitivity and specificity, the probe-based RT-RPA will be an alternative diagnostic assay for the detection of ARV in resource-limited settings.
Introduction
Avian reoviruses (ARVs) play significant roles in multiple disease manifestations in birds (1). ARV could infect various kinds of birds including chickens, turkeys, goslings, ducks and partridge, and leads to many kinds of disease syndromes including arthritis/tenosynovitis, intestinal diseases, respiratory diseases, diseases of the central nervous system (2–7). Since its first report in 1960s, ARV has been detected and isolated from domestic and wild birds across the world (6–15). Co-infections of ARV with other pathogens in commercial broiler chickens in intensive breeding farms lead to low feed conversion, poor weight gains and reduced marketability of affected chickens, resulting into huge economic losses. To enhance monitoring and diagnosis for avian reovirus (ARV) infection, a simple, sensitive and effective detection method would be essential for the effective control and prevention of ARV in poultry flocks.
Virus isolation is a classical detection method for the diagnosis of ARV in clinical samples (1). The virus culture in monolayer cells is time-consuming which takes a period of 5–7 days to develop viral cytopathic effects (CPE) (10). Furthermore, 2-3 passages are given to allow virus to grow as ARV are slow growing. Hence it is standard method to give 2-3 passages before reporting as negative (10). In comparison to culture method, molecular techniques are more sensitive and rapid, but it need sophisticated thermal cycling equipment and elaborate protocols (16–18). Thus, a user-friendly and roust diagnostic assay for the detection of ARV nucleic acids in clinical samples will make prevention and control program more effective. The isothermal amplification technique, which is carried out at an optimal single temperature, is faster and more convenient than PCR approach. Among the isothermal nucleic acid amplification methods, the loop-mediated isothermal amplification assay (LAMP) was commonly used (5), it still took about an hour to obtain the result. Although RPA method was a latterly invented technique, it has attracted much attention for the last few years (19). RPA reaction was initiated by three proteins, including a recombinase, a strand-displacing DNA polymerase and a single-stranded DNA-binding protein at a single optimum temperature between 37 and 42°C. Accumulating studies demonstrates that RPA technique has been successfully used in rapid detection of bacteria, viruses, and parasites (20–24). Endpoint detection of RPA products could be carried out by nucleic acid electrophoresis and lateral flow dipstick (LFD) (25). Compared to electrophoresis and LFD, a probe-based RPA assay can generate real-time amplification result in <20 min without an additional process after amplification (21).
In this study, we aim to establish a probe-based RT-RPA and evaluate its performance for the detection of ARV in field samples.
Materials and Methods
Viruses
The virus strains infectious bursal disease virus (IBDV) B87, reticuloendotheliosis virus (REV) C15 and the vaccine strain Marek's disease virus (MDV) 814 were purchased from Guangdong Animal Epidemic Prevention and Material Reserve Center. Chicken anemia virus (CAV) GD-2014, newcastle disease virus (NDV), ARV S1133, Avian influenza virus (AIV) H7N2 subtype, infectious bronchitis virus (IBV), infectious laryngotracheitis virus (ILTV), Mycoplasma gallisepticum (MG) and Mycoplasma synoviae (MS) were preserved in our laboratories.
Generation of RNA Molecule Standard
The target gene of the RT-RPA assay was the S1 fragment. S1 segment was amplified for the preparation of the RNA standard. A specified primer set (forward primer: 5′-ACTGTCATTGACTTCGAACG-3′, reverse primer: 5′-CTCGAGTACACCCCATACGC-3′) were designed to amplify the S1 conserved nucleotide sequence. Total nucleic acids of the cell suspension infected with ARV S1133 were isolated for RT-PCR reaction. The RT-PCR product was subjected to electrophoresis on a 2% agrose gel and purified. The purified amplicons were inserted into the pGEM-T vector (Promega, USA) and in vitro transcribed as the method previously reported (20). The transcribed RNA concentration was determined using Nano2000 (GE, USA). The RNA copy numbers were calculated as the method previously reported (26).
Primers and Probe Design
To establish the RT-RPA assay, the primer and probe were designed based on the nucleotide sequence of ARV S1133 (Genbank ID: MG822668.1) following the instruction manual provided by TwistDx Inc. Cambridge, UK, and synthesized by BGI Genomics (Shenzhen, China). The nucleotide sequences of the primers and probe were summarized in Table 1.
RT-RPA Procedure
The RT-RPA reaction was performed by TwistAmp® RT exo kit (TwistDx Inc. Cambridge, UK). The protocol for preparation of the RT-RPA assay was following the manufacturer's instructions. Real-time RPA reaction was performed in a 50 μL volume. In a reaction tube containing the dried enzyme pellets, 29.5 μL of rehydration buffer, 1 μL template, 2.1 μL of each primer (10 μm), 0.6 μL probe, 2.5 μL magnesium acetate and 12.2 μL water were added. The reaction tubes were inverted thoroughly and centrifuged briefly. Then the tubes were immediately inserted into the fluorescence detector Deaou-308C (DEAOU Biotechnology, Guangzhou, China) at 39°C. The tubes were firstly incubated for 4 min, and mixed thoroughly. The tubes were then incubated for 16 min at 39°C. The fluorescence was measured for 40 times at 30s internal. If a sample generated an amplification curve above the threshold of the negative control, it was considered positive and below threshold as negative.
Sensitivity and Specificity of the Assay
The minimal detection limit of the RT-RPA assay was analyzed by the 10-fold serially diluted RNA standards ranged from 100 copy/μL-106 copies/μL. Each RNA standard dilution was tested by the RT-RPA assay in 8 replicates. The cross-reaction of the assay also evaluated. The pathogens used for specificity test included IBDV, REV, MDV, CAV, AIV, NDV, IBV, ILTV, MG, and MS. ARV S1133 was used as positive control.
Clinical Samples
To evaluate the practicality of the developed real-time RT-RPA assay, 86 tendon tissue specimens were collected from the chickens suspected to be infected with ARV in a poultry farm in Guangdong province. All the animal procedures were approved by the Office of Laboratory Animal.
Management of Guangdong Laboratory Animals Monitoring Institute, Guangzhou, China. One μg tendon was placed in 1.5-mL Eppendorf tube. The tendon was cut by scissors and prepared as tissue homogenate with the disposable pestle (Axygen Inc., USA) by adding 250 μL phosphate buffered saline (PBS). The tendon homogenate was frozen and thawed twice and clarified by low-speed centrifugation, and the supernatants were collected for the extraction of nucleic acids. Total nucleic acids of the specimens were extracted using a QIAamp Viral RNA Mini Kit (Qiagen, Germany) and tested by both real-time fluorescence RT-PCR and RT-RPA. The real-time fluorescence RT-PCR was performed in accord with a previously reported protocol (17). A minimum of 39 copies/μL of ARV genomic RNA could be detected by the assay. RNA volume and total reaction volume used for real-time RT-PCR were the same as that for RT-RPA.
Results
Screening of the Primer-Probe Set
The most important thing for optimization of the RT-RPA assay is to screen an efficient primer-probe set. In present study, four primer combinations with one probe were evaluated by the real-time RT-RPA assay. As clearly illustrated in Figure 1, the primer pair 2F/2R took the least time to generate test result among all the primer combinations. The fluorescence signal of primers 2F/2R increased significantly from 4 min, whereas the other three primer pairs took about 5 min. Besides, the fluorescence amplitude of 2F/2R along with the time was consistently highest among all the primer pairs. Thus, the primers-probe set (2F/2R) was used to establish the RT-RPA assay for the detection of ARV.
Specificity Test
To determine the specificity of the assay, ARV and 10 other avian pathogens were detected by the assay. The remarkable fluorescence increase was only observed for ARV S1133 but not the other 10 avian pathogens or the negative control sample (Figure 2), indicating that the RT-RPA assay using the optimal primers-probe set was ARV-specific and had no cross-reactions with other pathogens.
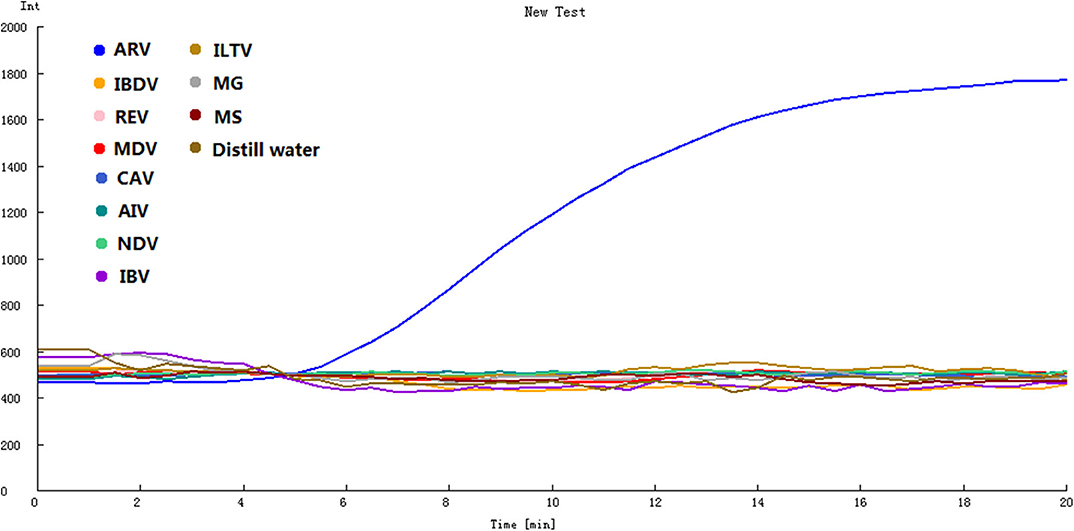
Figure 2. Specificity of the real-time RT-RPA assay. IBDV, REV, MDV, CAV, AIV, NDV, IBV, ILTV, MG, MS were tested by the assay. ARV was detected by the assay as the positive control, and distilled water was the negative control.
Detection Limit of the Assay
The transcribed RNA was 10-fold serially diluted (100 copy/μL-106 copies/μL) and detected by the real-time RT-RPA assay. The templates containing 106 −102 RNA molecules produced fluorescence curves, while other templates and the distilled water didn't produced fluorescence curve (Figure 3). The RNA dilutions were tested in 8 replicates by the RT-RPA assay to assess the repeatability of the assay (Supplementary Figures 1–6). The RNA dilutions ranging from 106-102 copies were all positive by RT-RPA in 8 replicates. However, only one test was positive when RNA concentration was 10 copies; and all the 8 replicates were negative when one copy per reaction was used. The exact detection limit of the assay was 102 copies molecules in 95% of cases determined by the probit regression analysis.
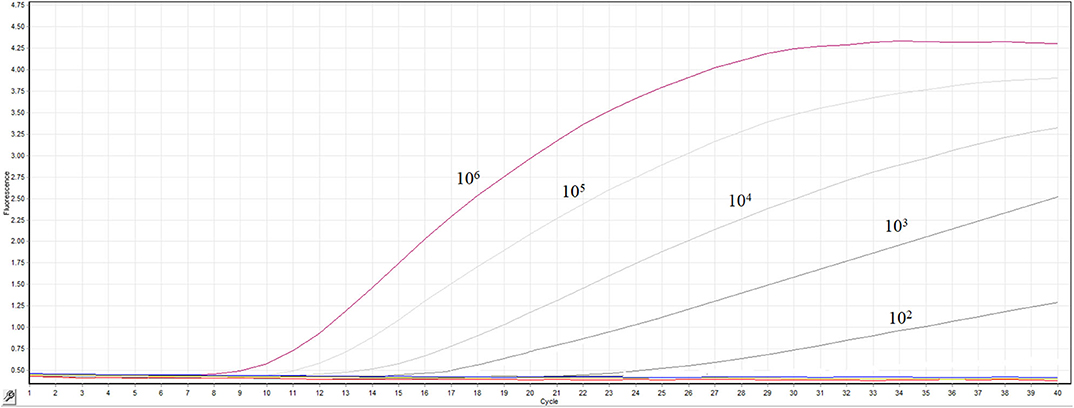
Figure 3. Sensitivity the real-time RT-RPA assay. A range of serially diluted RNA standards from 100 copy/μL-106 copies/μL were detected by the assay.
To verify the sensitivity of the method, more strains of ARV were tested. Blast analysis (https://blast.ncbi.nlm.nih.gov/Blast.cgi) showed that the sequences of the primer-probe set share 100% similarity with most of the ARV strains. Meanwhile, several point mutations were present on some ARV strains (ARV strain GX110058, ARV strain B-98 et.al) at the binding site of the primer-probe sequence. So ARV strain GX110058 segment S1 (GenBank: KF741742.1) and strain B-98 segment S1 (GenBank: DQ643974.1) were synthesized by BGI Genomics (Shenzhen, China) and cloned in pGEM-T vector. The sensitivity of the RT-RPA assay was evaluated by the above method. The result showed that the assay could detect 102 copies ARV strain GX110058 and ARV strain B-98 S1 molecules, respectively (Supplementary Figures 7, 8), confirming that several point mutations have little effect on the sensitivity of the RT-RPA assay which was reported previously (21).
Performance of the RT-RPA Assay on Clinical Samples
Eighty-six tendon tissue specimens which were collected from the chickens in a poultry farm were detected by the assay developed above. Of these samples, 38 ARV-positive samples and 48 ARV-negative samples were confirmed by RT-RPA. All the samples were detected by a previously reported qRT-PCR assay. The qRT-PCR result showed that 41 samples were ARV-positive and 45 samples were ARV-negative. The detailed test results were displayed in the Table 2. There were 3 positive samples determined by qRT-PCR, but negative by RT-RPA. The Ct values of the three samples were >35, indicating low viral loads. The minimum detection limit of the qRT-PCR assay was 39 copies/μL of ARV genomic RNA. Ct value was 34.9 when the input copy number was 100 copies RNA (17). The Ct values >35 suggested ARV genomic RNA of the three samples were less than the detection limit of RT-RPA. The coincidence rate was 96.5% between the test results of RT-RPA and RT-qPCR (Table 2). The threshold time (TT) values and CT values showed a poor correlation by linear regression analysis, suggesting the real-time RT-RPA assay could be used to qualitative detection of ARV (Figure 4). The poor correlation has been reported in several RPA studies (20, 21). Currently, there were few studies reporting the possible reasons. The possible explanation was that the extracts of the samples that contained the other irrelevant nucleic acids besides ARV may affect the efficiency of enzymes in the RPA reaction.
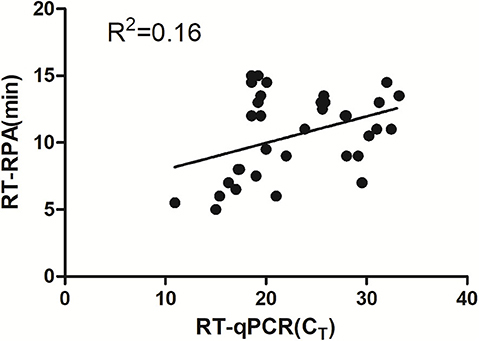
Figure 4. Validation of the RT-RPA assay on the clinical samples. RT-RPA threshold times (TT, y axis) and qRT-PCR cycle threshold values (CT, x axis) of the clinical samples were compared by Prism software. R2 value was 0.16.
Discussion
In present study, we established a probed-based isothermal amplification assay for rapid and sensitive diagnosis of ARV. The fluorescence-based RT-RPA assay was carried out at a consistent temperature of 39°C, and the amplification took about 20 min to finish (Figure 1). Fluorescence signals could be real-time monitored by a portable device. Only nucleic acid from ARV could be amplified by the assay. The other viruses which are common infectious pathogens in chicken were not amplified by the assay, suggesting remarkable specificity of the RT-RPA assay (Figure 2). The real-time RT-RPA assay could detect as few as 100 genomic copies per reaction. The assay was applied to detect 86 clinical samples. The detection result of the clinical samples by RT-RPA was 96.5% consistent with that of the qRT-PCR method. Therefore, the RT-RPA assay could feasibly be used to rapid detection of ARV and had the potential to apply in field.
Primer design was of vital importance in the establishment of a RT-RPA assay with high sensitivity and specificity. Four primer pairs combined with a probe were screened for the optimal performance of the assay. The primer pair 2F/2R took the least time to generate test result among all the primer combinations. The fluorescence signal of primers 2F/2R increased significantly from 4 min, whereas the other three primer pairs took about 5 min. Besides, the fluorescence amplitude of 2F/2R along with the time was consistently highest among all the primer pairs. Thus, the optimal primer pair 2F/2R was used in the RT-RPA assay.
RPA which was invented in 2006 has been experiencing rapid development (19, 27). The RPA method was simpler and faster compared to other detection methods. For example, ARV diagnosis by cell culture took at least 48 h to obtain the result. Real-time qRT-PCR was faster, but still took about 2 h to obtain the ultimate result (17), expensive instrument and professional team were indispensable. In the LAMP assay for the detection of ARV, six primers were required (5). Hence, compared to the previously reported methods, the advantages of the RPA method are obvious. The real-time RT-RPA assay developed in present study only required two primers and one probe, the test result could be obtained in <20 min. As illustrated in Figure 1, the template containing 106 copies RNA molecules took about 5 min to obtain the amplification curve in the RT-RPA assay. To our best knowledge, the RT-RPA assay is the most rapid among all the previously reported methods for the detection of ARV (17, 28). Low cost is another advantage of the RPA assay.
For rapid detection of ARV in field with limited resources, RPA method has huge advantages including its portability, simplicity, and short turnaround time. A RPA-based portable laboratory has been established for field detection of dengue virus (29). In our study, 86 specimens were simultaneously tested by the RT-RPA and qRT-PCR. The detection result of the RT-RPA assay was 96.5% in agreement with that of the qRT-PCR assay, indicating good performance of the assay and potential for detecting ARV in field. In summary, the highly sensitive RT-RPA is suitable for extensive applications in laboratories, which are specifically designed for ARV detection and surveillance.
Data Availability Statement
The original contributions presented in the study are included in the article/Supplementary Material, further inquiries can be directed to the corresponding author/s.
Ethics Statement
The animal study was reviewed and approved by Animal experiments were approved by the Office of Laboratory Animal Management of Guangdong Laboratory Animals Monitoring Institute, Guangzhou, China.
Author Contributions
LM, HS, and FC designed the study and drafted the manuscript. LM and MZ conducted the experiments, data analysis, and participated in drafting of the manuscript. YS and KZ helped with the experiments. All the authors read and approved the final manuscript.
Funding
This study was supported by grants from Ph.D. Research Start-up Foundation of Anyang Institute of Technology (BSJ2020013) and National Natural Science Foundation of China (31802170). This study was also supported by a grant from Guangzhou Municipal Science and Technology Project (201904010284).
Conflict of Interest
The authors declare that the research was conducted in the absence of any commercial or financial relationships that could be construed as a potential conflict of interest.
Supplementary Material
The Supplementary Material for this article can be found online at: https://www.frontiersin.org/articles/10.3389/fvets.2020.551350/full#supplementary-material
References
1. Sellers HS. Current limitations in control of viral arthritis and tenosynovitis caused by avian reoviruses in commercial poultry. Vet Microbiol. (2017) 206:152–6. doi: 10.1016/j.vetmic.2016.12.014
2. Petek M, Felluga B, Borghi G, Baroni A. The crawley agent: an avian reovirus. Arch Gesamte Virusforsc. (1967) 21:413–24. doi: 10.1007/BF01241740
3. Van de Zande S, Kuhn EM. Central nervous system signs in chickens caused by a new avian reovirus strain: a pathogenesis study. Vet Microbiol. (2007) 120:42–9. doi: 10.1016/j.vetmic.2006.10.024
4. Hieronymus DR, Villegas P, Kleven SH. Identification and serological differentiation of several reovirus strains isolated from chickens with suspected malabsorption syndrome. Avian Dis. (1983) 27:246–54. doi: 10.2307/1590390
5. Xie Z, Peng Y, Luo S, Wang Y, Liu J, Pang Y, et al. Development of a reverse transcription loop-mediated isothermal amplification assay for visual detection of avian reovirus. Avian Pathol. (2012) 41:311–6. doi: 10.1080/03079457.2012.686104
6. Yang J, Tian J, Chen L, Tang Y, Diao Y. Isolation and genomic characterization of a novel chicken-orign orthoreovirus causing goslings hepatitis. Vet Microbiol. (2018) 227:69–77. doi: 10.1016/j.vetmic.2018.10.017
7. Kugler R, Dandar E, Feher E, Jakab F, Mato T, Palya V, et al. Phylogenetic analysis of a novel reassortant orthoreovirus strain detected in partridge (perdix perdix). Virus Res. (2016) 215:99–103. doi: 10.1016/j.virusres.2015.11.018
8. Noh JY, Lee DH, Lim TH, Lee JH, Day JM, Song CS. Isolation and genomic characterization of a novel avian orthoreovirus strain in Korea, 2014. Arch Virol. (2018) 163:1307–16. doi: 10.1007/s00705-017-3667-8
9. Tang Y, Lu H. Genomic characterization of a novel avian arthritis orthoreovirus variant by next-generation sequencing. Arch Virol. (2015) 160:2629–32. doi: 10.1007/s00705-015-2547-3
10. Lu H, Tang Y, Dunn PA, Wallner-Pendleton EA, Lin L, Knoll EA. Isolation and molecular characterization of newly emerging avian reovirus variants and novel strains in Pennsylvania, USA, 2011-2014. Sci Rep. (2015) 5:14727. doi: 10.1038/srep14727
11. Tang Y, Lin L, Sebastian A, Lu H. Detection and characterization of two co-infection variant strains of avian orthoreovirus (ARV) in young layer chickens using next-generation sequencing (NGS). Sci Rep. (2016) 6:24519. doi: 10.1038/srep24519
12. Palomino-Tapia V, Mitevski D, Inglis T, van der Meer F, Abdul-Careem MF. Molecular characterization of emerging avian reovirus variants isolated from viral arthritis cases in Western Canada 2012-2017 based on partial sigma (sigma)C gene. Virology. (2018) 522:138–46. doi: 10.1016/j.virol.2018.06.006
13. Pradhan HK, Mohanty GC, Kataria JM, Pattnaik B, Verma KC. Antinuclear antibody in chickens with reoviral arthritis. Avian Dis. (1987) 31:249–53. doi: 10.2307/1590868
14. van der Heide L. The history of avian reovirus. Avian Dis. (2000) 44:638–41. doi: 10.2307/1593104
15. Lawson B, Dastjerdi A, Shah S, Everest D, Núñez A, Pocknell A, et al. Mortality associated with avian reovirus infection in a free-living magpie (Pica pica) in great Britain. BMC Vet Res. (2015) 11:20. doi: 10.1186/s12917-015-0329-5
16. Kort YH, Bourogaa H, Gribaa L, Hassen J, Ghram A. Genotyping and classification of tunisian strains of avian reovirus using RT-PCR and RFLP analysis. Avian Dis. (2015) 59:14–9. doi: 10.1637/10879-060414-Reg.1
17. Ke GM, Cheng HL, Ke LY, Ji WT, Chulu JL, Liao MH, et al. Development of a quantitative light cycler real-time RT-PCR for detection of avian reovirus. J Virol Methods. (2006) 133:6–13. doi: 10.1016/j.jviromet.2005.09.011
18. Tang Y, Lu H. Whole genome alignment based one-step real-time RT-PCR for universal detection of avian orthoreoviruses of chicken, pheasant and turkey origins. Infect Genet Evol. (2016) 39:120–6. doi: 10.1016/j.meegid.2016.01.018
19. Piepenburg O, Williams CH, Stemple DL, Armes NA. DNA detection using recombination proteins. PLoS Biol. (2006) 4:e204. doi: 10.1371/journal.pbio.0040204
20. Ma L, Cong F, Zhu Y, Wu M, Xu F, Huang R, et al. Development of a reverse transcription recombinase polymerase amplification assay for rapid detection of theiler's murine encephalomyelitis virus. Mol Cell Probes. (2018) 41:27–31. doi: 10.1016/j.mcp.2018.08.006
21. Ma L, Zeng F, Cong F, Huang B, Zhu Y, Wu M, et al. Development and evaluation of a broadly reactive reverse transcription recombinase polymerase amplification assay for rapid detection of murine norovirus. BMC Vet Res. (2018) 14:399. doi: 10.1186/s12917-018-1736-1
22. Geng Y, Liu G, Liu L, Deng Q, Zhao L, Sun XX, et al. Real-time recombinase polymerase amplification assay for the rapid and sensitive detection of campylobacter jejuni in food samples. J Microbiol Methods. (2018) 157:31–6. doi: 10.1016/j.mimet.2018.12.017
23. Gunaratna G, Manamperi A, Bohlken-Fascher S, Wickremasinge R, Gunawardena K, Yapa B, et al. Evaluation of rapid extraction and isothermal amplification techniques for the detection of leishmania donovani DNA from skin lesions of suspected cases at the point of need in Sri Lanka. Parasit Vectors. (2018) 11:665. doi: 10.1186/s13071-018-3238-1
24. Shahin K, Gustavo Ramirez-Paredes J, Harold G, Lopez-Jimena B, Adams A, Weidmann M. Development of a recombinase polymerase amplification assay for rapid detection of Francisella noatunensis subsp. orientalis. PLoS ONE. (2018) 13:e0192979. doi: 10.1371/journal.pone.0192979
25. Wang Z, Yang PP, Zhang YH, Tian KY, Bian CZ, Zhao J. Development of a reverse transcription recombinase polymerase amplification combined with lateral-flow dipstick assay for avian influenza H9N2 HA gene detection. Transbound Emerg Dis. (2018) 66:546–51. doi: 10.1111/tbed.13063
26. Yuan W, Wang J, Xu F, Huang B, Lian Y, Rao D, et al. Development of a duplex real-time RT-PCR for the simultaneous detection and differentiation of theiler's murine encephalomyelitis virus and rat theilovirus. J Virol Methods. (2016) 236:139–46. doi: 10.1016/j.jviromet.2016.07.004
27. Ma L, Zeng F, Huang B, Zhu Y, Wu M, Xu F, et al. Point-of-care diagnostic assay for rapid detection of porcine deltacoronavirus using the recombinase polymerase amplification method. Transbound Emerg Dis. (2019) 66:546–51. doi: 10.1111/tbed.13155
28. Kumar D, Chauhan TK, Agarwal RK, Dhama K, Goswami PP, Mariappan AK, et al. A double-stranded probe coupled with isothermal amplification for qualitative and quantitative detection of avian reovirus. Arch Virol. (2017) 162:979–85. doi: 10.1007/s00705-016-3184-1
Keywords: avian reovirus, recombinase polymerase amplification, rapid detection, specific, sensitive
Citation: Ma L, Shi H, Zhang M, Song Y, Zhang K and Cong F (2020) Establishment of a Real-Time Recombinase Polymerase Amplification Assay for the Detection of Avian Reovirus. Front. Vet. Sci. 7:551350. doi: 10.3389/fvets.2020.551350
Received: 13 April 2020; Accepted: 17 August 2020;
Published: 22 September 2020.
Edited by:
Yashpal S. Malik, Indian Veterinary Research Institute (IVRI), IndiaReviewed by:
Naresh Jindal, Lala Lajpat Rai University of Veterinary and Animal Sciences, IndiaRajeev Kaul, University of Delhi, India
Copyright © 2020 Ma, Shi, Zhang, Song, Zhang and Cong. This is an open-access article distributed under the terms of the Creative Commons Attribution License (CC BY). The use, distribution or reproduction in other forums is permitted, provided the original author(s) and the copyright owner(s) are credited and that the original publication in this journal is cited, in accordance with accepted academic practice. No use, distribution or reproduction is permitted which does not comply with these terms.
*Correspondence: Feng Cong, congfeng521@126.com
†These authors have contributed equally to this work