Environmental Impacts of Pig and Poultry Production: Insights From a Systematic Review
- 1Department of Animal Science, Universidade Federal do Rio Grande do Sul, Porto Alegre, Brazil
- 2Département des Sciences Animales, Faculté des Sciences de l'Agriculture et de l'Alimentation, Université Laval, Québec, QC, Canada
- 3Sherbrooke Research and Development Centre, Agriculture and Agri-Food Canada, Sherbrooke, QC, Canada
- 4Elanco Animal Health, São Paulo, Brazil
Pig and poultry production systems have reached high-performance levels over the last few decades. However, there is still room for improvement when it comes to their environmental sustainability. This issue is even more relevant due to the growing demand for food demand since this surplus food production needs to be met at an affordable cost with minimum impact on the environment. This study presents a systematic review of peer-reviewed manuscripts that investigated the environmental impacts associated with pig and poultry production. For this purpose, independent reviews were performed and two databases were constructed, one for each production system. Previous studies published in peer-reviewed journals were considered for the databases if the method of life cycle assessment (LCA) was applied to pig (pork meat) or poultry (broiler meat or table eggs) production to estimate at least the potential effects of climate change, measured as CO2-eq. Studies considering the cradle-to-farm gate were considered, as well as those evaluating processes up to the slaughterhouse or processor gate. The pig database comprised 55 studies, while 30 publications were selected for the poultry database. These studies confirmed feeding (which includes the crop cultivation phase, manufacturing processes, and transportation) as the main contributor to the environmental impact associated with pig and poultry production systems. Several studies evaluated feeding strategies, which were indicated as viable alternatives to mitigate the environmental footprint associated with both production chains. In this study, precision feeding techniques are highlighted given their applicability to modern pig and poultry farming. These novel feeding strategies are good examples of innovative strategies needed to break paradigms, improve resource-use efficiency, and effectively move the current productive scenario toward more sustainable livestock systems.
Introduction
The increasing demand for food is an important challenge that society will face in the coming decades. The growing population will need more resources, leading to a relevant increase in food demand. The productive sector (including agriculture and livestock) needs to support the growing demands for food, however, without compromising the ability of the future generations to also meet their requirements. In other words, environmentally sustainable agri-food systems are mandatory requirements for a world with increasing urbanization and growing food demands.
In this context, the benefits of agri-food sectors for society need to be maximized (1), which can be achieved by improving the efficiency in which the resources are applied in the production chains. The current production methods will need to adapt to these new challenges (limited resources, increased production), with most surplus food production being supplied by innovative agri-food systems (2).
Pig and poultry production systems have reached high-performance levels over the last few decades. Together, these sectors provide a large amount of affordable and nutritious food, especially high-quality protein, contributing to food security worldwide. However, there is still room for improvement when it comes to their environmental sustainability. Feeding pigs and poultry requires tremendous amounts of feed resources, with several studies indicating it as an important source of environmental impact (3). In addition, pigs and broilers excrete annually large amounts of nitrogen and phosphorus to the environment, which conditions the production sustainability of these chains (4).
Conventionally, the impacts of pig and poultry production have been assessed by methodologies that used an “animal basis” approach (e.g., studies focusing on reducing nutrient excretion). These are very important studies; however, few mitigation strategies have focused on the efficiency of resource use, which is critical in a global context. Considering the relevance of the topic, it is important to investigate feeding practices that mitigate the environmental impacts associated with the entire production system. Thus, we carried out a systematic review to summarize, analyze, and compare studies that used life cycle assessment (LCA) to evaluate the environmental impacts associated with pig and poultry production systems.
Materials and Methods
This systematic review was based on structured and elaborated research performed using online search methods. The search strategy was planned and carried out to identify as many studies as possible on the subject. Papers were rigorously selected and those focusing on feeding practices were further evaluated.
Independent searches were performed for pig and poultry production systems. The strategy “PICo” was applied to build the research question by identifying “Population” (database 1: “pig”; database 2: “poultry”), “Interest” (“life cycle assessment”), and “Context” (“climate change”) for both searches. Alternative terms for population and interest were listed using synonymous words in English to compose the final search strategy. Context was applied later (through full-text reads) to avoid missing any study in which the response was not mentioned among the main terms (title, abstract, and keywords). The final search terms were:
Database 1:
(pig OR pigs OR swine) AND (“life cycle assessment” OR “life cycle” OR “carbon emission” OR “carbon footprint” OR “greenhouse gas*” OR “global warming” OR LCA)
Database 2:
(poultry OR broiler* OR chicken*OR hen) AND (“life cycle assessment” OR “life cycle” OR “carbon emission” OR “carbon footprint” OR “greenhouse gas*” OR “global warming” OR LCA).
The search was conducted in March 2020, considering only original peer-reviewed studies published in scientific journals available in PubMed, Scopus, and Web of Science. A snowball approach using forward (e.g., databases) and backward research methods (e.g., direct journal search, reference lists, studies listed in previously published reviews) was performed to increase the chance of including as many relevant studies as possible. No limitations on the geographic origin or year of publication were applied in both searches.
Each database was exported to the reference software (EndNote X9, Philadelphia, PA) used to organize references and manage part of the study selection. Duplicate references were identified and excluded. Studies were critically evaluated regarding their relevance and quality by examining titles and abstracts, followed by a complete review of the LCA study. Two reviewers performed a critical evaluation of the study eligibility. A study was not considered in the final database (removed) after mutual agreement, with a third reviewer reassessing studies that differed in terms of eligibility.
The selection criteria were stated as (i) original papers published in peer-reviewed journals, (ii) environmental impact evaluated using the LCA methodology; (iii) evaluation of pig (pork meat) or poultry (broiler meat or table eggs) production systems; (iv) scopes including cradle-to-farm, to the slaughterhouse, or to processor gate; (v) estimation of at least the potential impact of climate change, in CO2-eq. The quality of selected studies was further evaluated and information relevant to describe the proposed theoretical model was transferred to the pig and poultry spreadsheets. Finally, cross-study comparisons were performed considering the subject, scope, and main results observed.
Results
Studies Focusing on Pig Production
The research process until obtaining the final pig database is described in Figure 1A. Articles obtained by online searches (4,237 references) were critically evaluated and successive exclusions were performed. The main exclusions (more related to methodological aspects of the original studies) were performed when assessing the full-text, when 36 references were eliminated (criterium i and ii: 15 publications; criterium iii: 2 publications; criterium iv: 13 publications; and criterium v: 6 publications). The final list of 55 selected studies is described in Table 1.
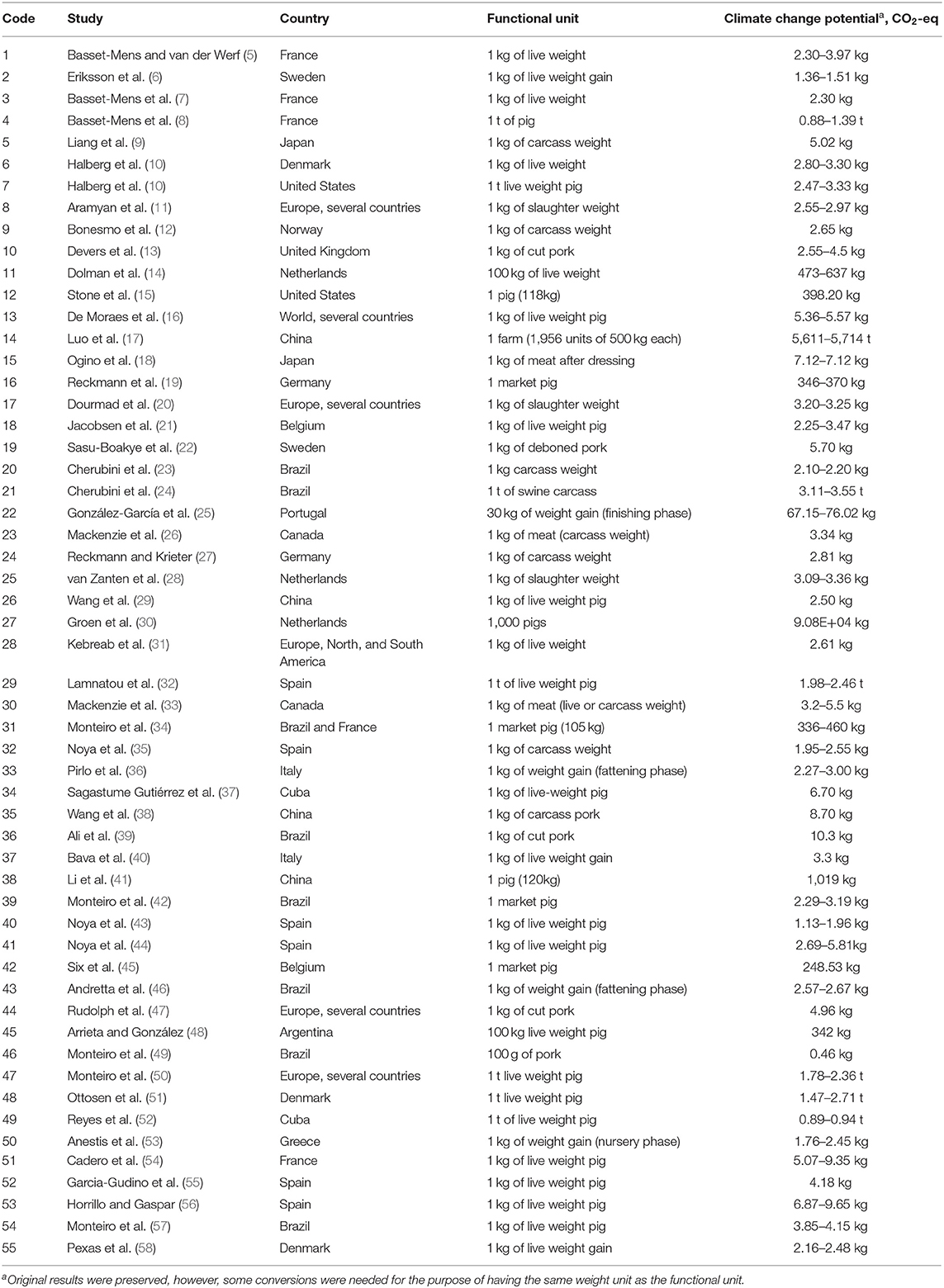
Table 1. Summary of the LCA studies on pig production in terms of location, functional unit, and climate change potential.
The first LCA study identified in the pig database was published in 2005. Considering the entire database, 26 journals reported publications, with 16 papers being published in Journal of Cleaner Production and 5 papers in Animal. Production scenarios located in Brazil (which was considered in eight studies), Spain (considered in six studies), France (considered in five studies), and China (considered in four studies) were assessed in the selected papers, as illustrated in Figure 2A. The frequency of studied countries is highly related to the location of the main research groups. However, it is important to highlight that the order of most studied countries is not in complete agreement with the pork production ranking (led by China). Another important aspect related to the geographical characteristics of the papers is that five studies were developed by researchers from countries different than the one (or at least one of the regions) considered in the simulations, with Brazil or South America being studied in three of them.
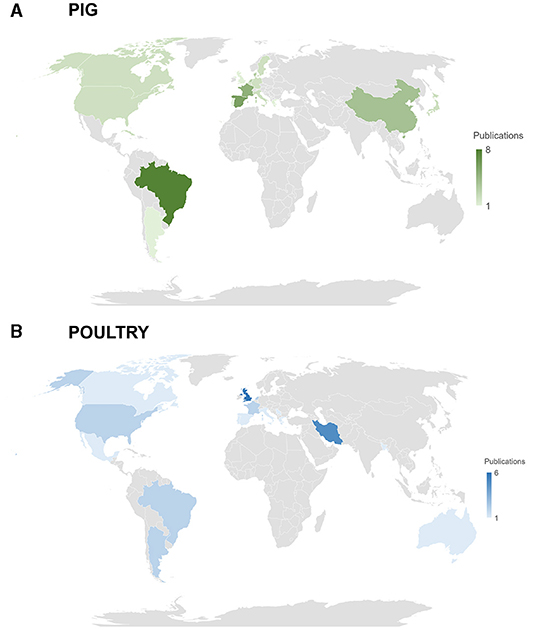
Figure 2. Location of the LCA studies focusing on pig (A) or poultry (B) production. Studies that simulated two or more countries, or even an entire continent, are not displayed in the figure.
A scope described as cradle-to-farm gate was used in the majority of the studies, which means that all phases comprised from the crop cultivation (and its inputs/outputs) up to the animal rearing phase were considered in these projects. The impacts associated with slaughtering and processing were considered in nine publications only.
Climate change was the focus of our study. However, the LCA studies also reported other impact categories (Figure 3A). From those variables, the most prevalent were eutrophication and acidification, followed by the use of energy and land.
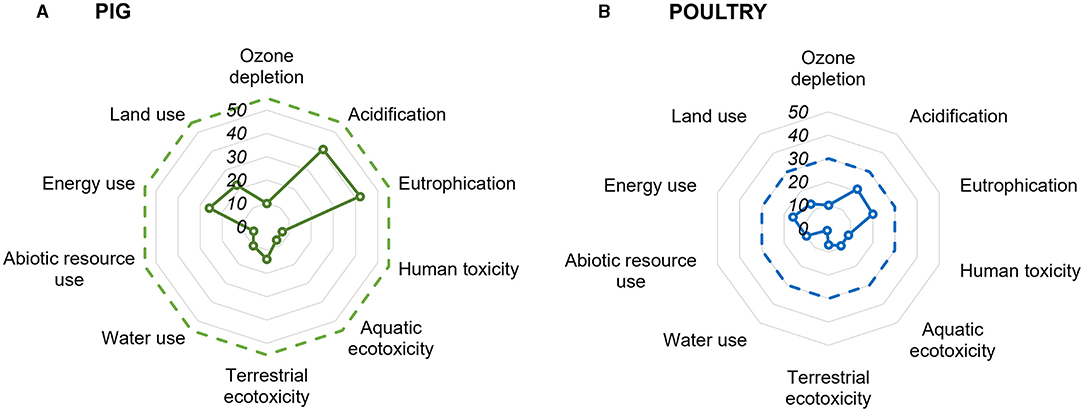
Figure 3. Environmental impact categories evaluated in the LCA studies focusing on pig (A) or poultry (B) production systems, with dashed lines indicating the total number of publications included in each database.
The main subjects under evaluation in the studies focusing on pig production are presented in Table 2. The characterization of pig production in the region or country was the main objective in 18 studies. Another important objective in the studies was the comparison of production systems (including organic or alternative housing systems), which was the main subject in nine papers.
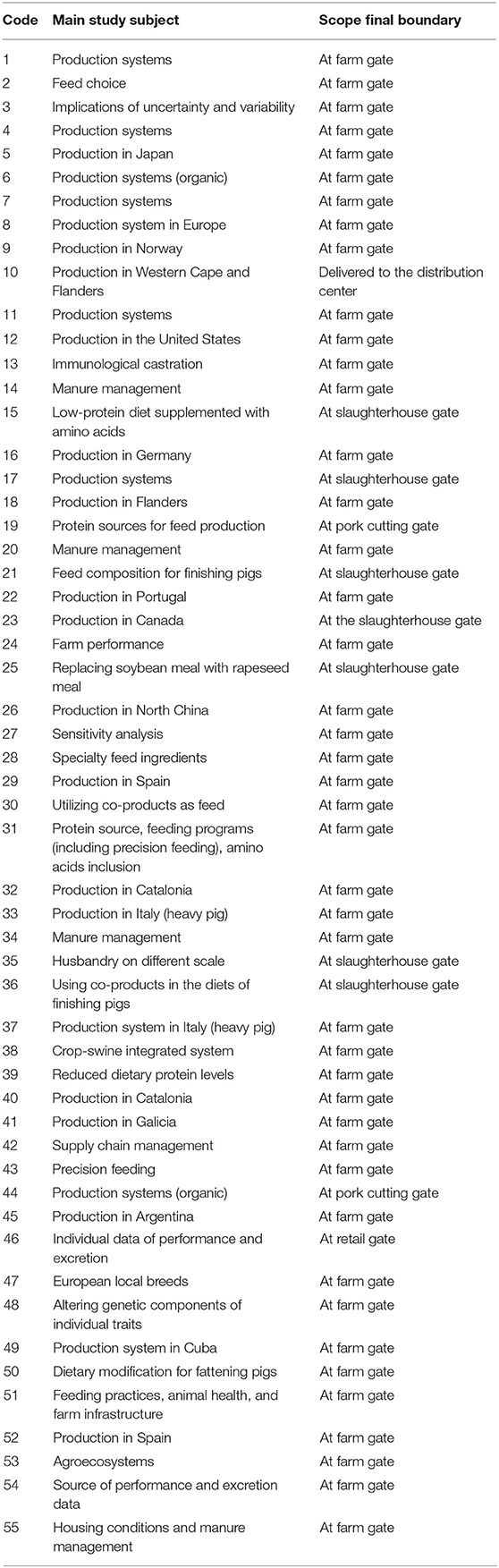
Table 2. Summary of the LCA studies on pig production in terms of main subject under analysis and scope boundary.
Changes in feeding practices (diet composition or feeding programs) were studied in 25% of the papers. The relative participation of feed production (which includes each ingredient's life cycle, fabrication, and transport) varied from 31 to 76% of the overall greenhouse gas (GHG) emissions in the pig database (Figure 4A). Despite the importance of feeding to the total pig production impact, the diet composition used in the inventory was described by the minority of the papers. Only 38% of the papers described the ingredient formulas, while only 29% of the studies showed any description for dietary nutritional composition, limited sometimes to crude protein. In addition, the environmental impacts related to the production of individual ingredients were presented in only 9% of the papers. The proportion of total impact associated with feed was highlighted in most of the studies. However, the impact of feed production (considering as a functional unit; e.g., 1 ton of feed) was presented in only 15% of the publications. These data would be of great value for further investigations on feeding practices that may mitigate the potential environmental impact of pig production. In addition, more information on feeding practices would allow a better comparison among studies, as great variability exists between the final results (impact of pig production) presented by the studies even for the same functional unit.
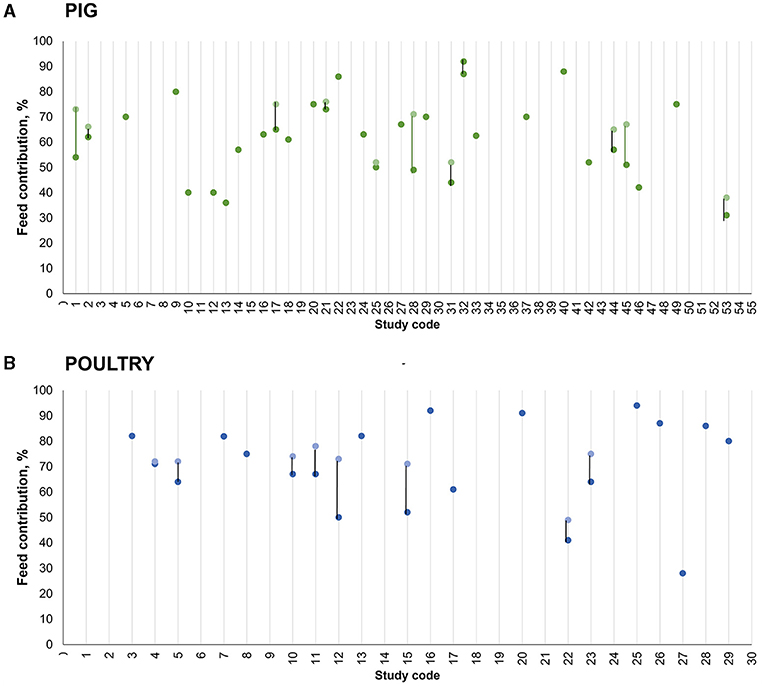
Figure 4. Feed contribution to the potential impact of climate change in LCA studies focusing on pig (A) or poultry (B) production. Study codes are the same as those presented in Tables 1, 2 for pigs and poultry, respectively. Blank lines were used for studies where the exact information was not presented in the original publication (text or tables, as the exact value could not be obtained when information was presented in figures).
As previously stated, crop production is a major contributor to the overall impacts of the pig production chain. The globalization of feed ingredient markets is relevant to LCA studies because it disconnects commodity production from its use/consumption. In a context in which most of the ingredients used for feed production are internationally traded, it is important to highlight that the impacts associated with a certain product are virtually shared with several countries involved in the international trade. The most frequent example of this intercontinental sharing was the use of soybean imported from South America, mainly from Brazil, in European countries. Considering the pig database, 49% of the studies mentioned the use of Brazilian soybean. For that reason, several papers also mentioned the inclusion of overseas transport during the inventory characterization.
Studies Focusing on Poultry Production
The research process until obtaining the final poultry database is described in Figure 1B. Articles obtained by online searches (6,502 references) were critically evaluated, which resulted in several exclusions. Seventeen references were excluded when assessing the full-text (criterium i and ii: 2 publications; criterium iii: 1 publication; criterium iv: 7 publications; and criterium v: 7 publications). The final list of 30 selected studies is described in Table 3.
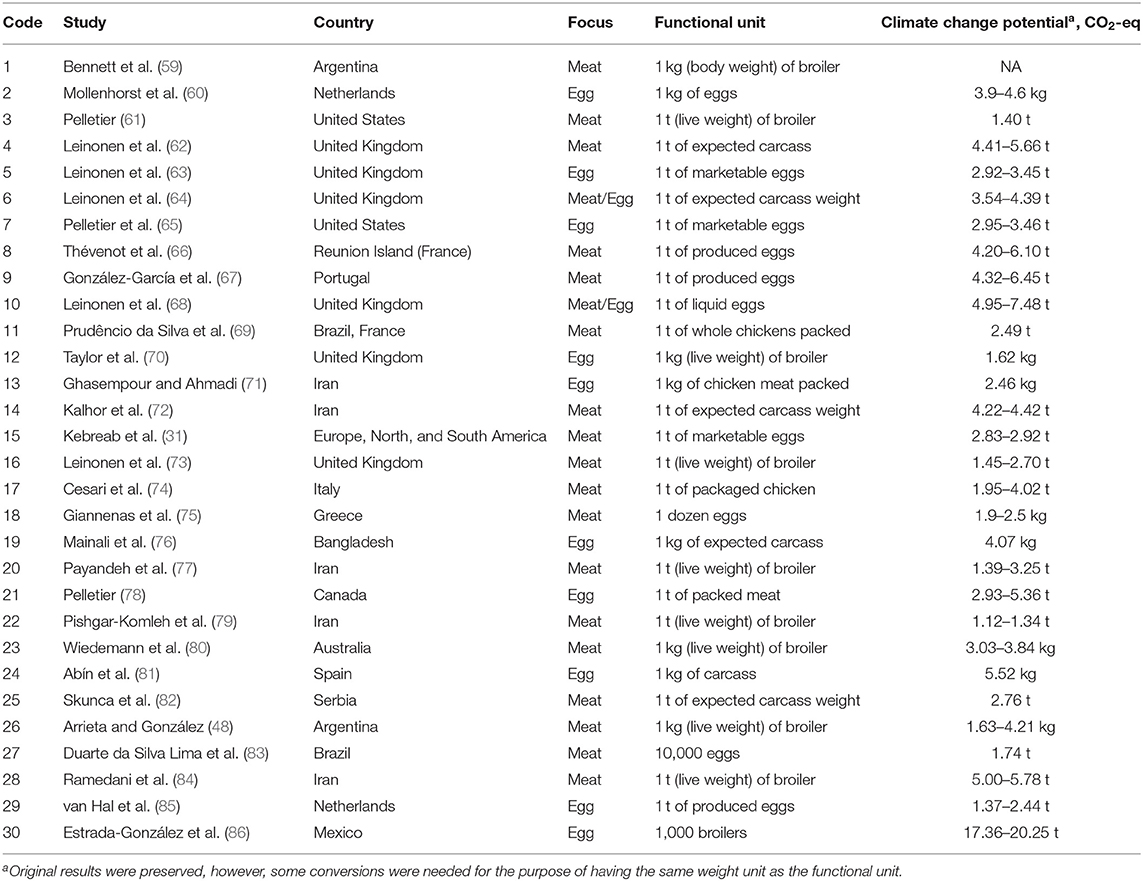
Table 3. Summary of the LCA studies on poultry production in terms of location, focus, functional unit and climate change potential.
The first study identified in the poultry database was published in 2006. Considering the entire database, 13 journals reported publications, with 10 papers being published in Journal of Cleaner Production and 5 papers in Poultry Science. Broiler production was evaluated in 18 studies, eggs were the main product evaluated in 10 studies, and both products were assessed in two papers. Production scenarios located in the United Kingdom and Iran (which were considered in 6 studies each); followed by Argentina, Brazil, France, Netherlands, and the USA, which were considered in two studies each; as illustrated in Figure 2B.
Likewise to the pig database, the scope described as cradle-to-farm gate was used in most studies focusing on poultry production. Impacts associated with slaughtering and processing were considered in 10 publications. Besides climate change, studies presented other impact categories (Figure 3B), such as acidification, eutrophication, and the use of energy and land. The characterization of the meat or egg production in the region or country was the main objective in 15 studies (Table 4).
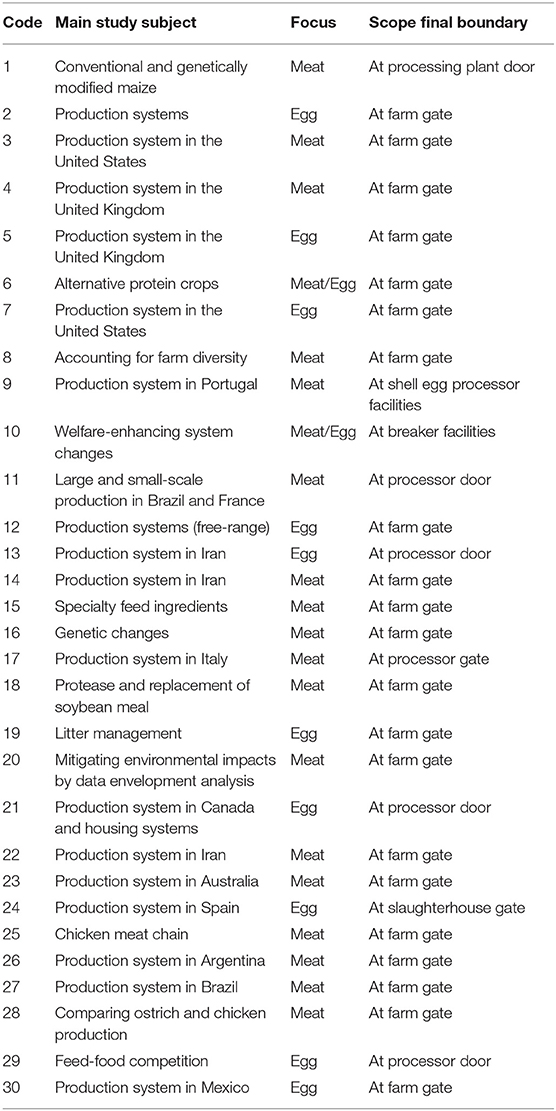
Table 4. Summary of the LCA studies on poultry production in terms of main subject under analysis and scope boundary.
Three papers described the environmental impacts of replacing ingredients in feed formulas, while one paper described the impacts of dietary supplementation with protease. Feeding was highlighted as the major source of environmental impact in most studies, accounting for 28–82% of the overall impact of climate change (Figure 4B). Despite the importance of feeding to the total impact, the diet composition used in the inventory was not described in most studies. Only 13% of the papers described the ingredient formulas, while only 10% of the studies showed any description for dietary nutritional composition. In addition, the environmental impacts related to the production of individual ingredients were presented in only 13% of the papers, with the impact of feed production (considering as a functional unit; e.g., 1 ton of feed) being presented in only 20% of the publications. The use of Brazilian soybean was reported by 30% of the studies, highlighting the importance of international trade also for the environmental impact of poultry production.
Discussion
The availability of peer-reviewed publications using LCA to assess the environmental impacts of pig and poultry production systems has increased over the years (Figure 5). The first studies of each database were published in close years for both pig (2005) and poultry (2006) production chains. However, the availability of studies focusing on pig production evolved greatly in the following years, mainly after 2014. In most research areas, the number of studies on poultry production is great than the number of publications available in a comparable topic in pigs. However, the opposite was found in this systematic review, probably due to the higher risk and concern with the environmental impacts of pig production compared to poultry systems.
The interest in using LCA to investigate the sustainability of a given production originates from its capability to quantify and evaluate the resources consumed and the emissions released at each phase needed for its production (8). Concerns about food safety and climate change have greatly increased in recent years. In response, the livestock industry must then reduce the utilization of resources by increasing its efficiency while reducing its environmental impact.
The impacts estimated for both production systems varied greatly across studies, mainly due to the heterogeneity of functional units and the amplitude of the considered life-cycle scopes. However, other attributes may also be listed as sources of variability when comparing publications. In particular, this heterogeneity may be related to the production systems under analysis (10, 62, 63), as well as to regional characteristics (31, 69). The conditions considered for housing (58), farm size (38, 79), level of intensification (20), and manure management (23) are also reported as important factors determining the final impact associated to the product. When focusing on animal aspects, some welfare (68) and genetic traits (50, 51, 73), as well as sanitary aspects (54) were reported.
Feed production was highlighted in several papers due to its relevant contribution to the total environmental impact. This phase was simulated including each ingredient's life cycle, fabrication, and transportation to the feed mill or to the farm in most studies. The reported contribution of the feed production phase relative to the overall GHG emissions varied from 31 to 76% in the pig database. In the poultry database, it accounted for 28–82% of the total climate change impact. Regardless of the exact environmental impact attributed to the feeding phase, almost all studies identified feeding as the production factor having the greatest environmental impact. These findings support the hypothesis that eco-friendly feeding practices can mitigate the environmental impacts of pig and poultry production.
Importance of Rearing System Scenarios
Even though a comparison between organic and conventional systems will not be deeply reviewed, it is important to highlight that several studies indicated the production system as one of the important aspects determining the relative contribution of feeding to the overall environmental impact (due to the feed ingredient composition, number of feeding phases, among others). Conventional production systems were considered in most simulations (i.e., conventional feed ingredients). However, some studies evaluated the environmental impacts of adopting alternative production systems (e.g., organic, free-range, certified labels). According to Leinonen et al. (62, 63), the global warming impact necessary to obtain a given functional unit of feed (e.g., 1 ton) can be low in organic farms in comparison to conventional farms. However, a higher feed amount is generally necessary for organic farms than in conventional production systems to obtain the same functional unit. Several reasons are indicated in the papers, as the impairment in feed conversion ratio, an increase in feed consumption, or even waste of feed or products. Thus, when the total cycle is analyzed, a greater global warming potential impact may be associated with feeding animals in organic than in conventional systems (10, 62, 63).
The environmental impacts of a given rearing system are highly correlated with animal performance, especially feed efficiency (27, 87). Thus, technologies that improve animal performance usually have great potential to mitigate life cycle environmental impacts. In this particular aspect, some technologies were assessed in the reviewed studies. Immunological castration and feed additives are some of these factors (16, 31, 53, 75), but probably many more aspects still need to be evaluated in future research.
Another important factor evaluated in some studies was the impact of innovative practices during the cultivation or processing of feed ingredients. The use of maize genetically modified (59), different processing methods for soybean (48), or crop-animal integrated systems (41) were evaluated, and impacts in the final product (i.e., functional unit) were reported.
Importance of Feeding Scenarios
The use of alternative feed ingredients is an important strategy in livestock systems. Some studies presented environmental advantages when using co-products in the assessed feeds (28, 33, 39). Other papers indicated that these advantages may be related to the calculation method, with favorable results being reported only when the impacts were not co-allocated between the main and the co-products (35, 88). The environmental cost to obtain co-products cannot be ignored in the LCA analysis, and this should be probably further evaluated in future research. Another limitation to be considered when comparing studies are the different ingredient choices given the difficulties in data acquisition, especially for local or limited ingredients as well as the great variability among processes used to obtain co-products (64, 88).
The distance between feedstuff production location and their place of use is an important argument in favor of using ingredient choice (or replacement) as a strategy to mitigate environmental impacts. Feed ingredients are products with cross-border flows, which are a consequence of globalization. Reducing the distance from producers to consumers means fewer transportation needs, and consequently fewer costs and emissions. Using this argument, several studies were developed proposing the use of locally grown ingredients instead of products cultivated in different countries or even continents (22, 43, 64). This is particularly important for local protein-ingredients that replace imported soybean and soybean meal (64). However, the use of local ingredients must not impair feed conversion (24, 66). Otherwise, the advantages may be lost caused by increased demand for feed to reach the same final weight.
Feed composition in terms of ingredients is also a way to reduce the excretion of nutrients and, consequently, manure composition. For that reason, the choice of ingredients needs to be made always with caution, focusing on the origin, but also on the nutritional quality of the product. Nitrogen excretion in manure is highly correlated with diet formulation. If an increase in nitrogen losses in the manure is related to a given ingredient choice, it is expected that this modification will lead to higher GHG emissions and probably other major consequences too (65). In this context, strategies that mitigate nutrient excretion, such as enzyme supplementation (75), synthetic amino acid partially replacing protein crops, or the use of low-protein diets (6, 42, 57), can potentially mitigate the environmental impacts of both pig and poultry production. The modification of the feed formulation method (89) and the adoption of precision feeding techniques (46, 90) are also very important and innovative tools. Due to its relevance for future animal production, precision feeding will be further discussed in the next section, with a focus on pig production.
Precision Feeding as an Eco-Friendly Strategy to Mitigate the Environmental Impacts of Pig Production
Feeding is a major source of environmental impacts, as previously discussed. When correctly applied, precision feeding is an efficient tool to decrease production and environmental costs (91). Pigs and poultry are usually fed according to group requirements, disregarding individual particularities. This means that all animals receive the same feed for an extended period, with part of the population receiving nutrients above their requirements (92). The animals that receive nutrients above their needs excrete this excess. An increased protein intake decreases protein efficiency utilization, resulting in larger nitrogen excretions (93). In many pig commercial systems, the nitrogen retention in conventional phase-feeding programs will rarely exceed 35%, being that the efficiency of nitrogen utilization used in many LCA studies (94). However, nitrogen efficiency varies depending on age, sanitary status, and crude protein levels (95, 96).
Precision feeding consists in providing the right amount of feed with the right balance composition to each animal at the right time. Thus, precision feeding can be defined as the technology that provides each animal the nutrients tailored to meet in real-time the animal requirements (91). Nitrogen and phosphorus excretions can be decreased by 40% and consequently reduce production costs by 10% when using an individual precision feeding program (93, 97).
In this context, precision feeding can improve the sustainability of pig production systems. Automatic feeding stations allow pigs to be fed individually with a diet whose composition is appropriate to their growth potential (91). This strategy is an important pattern shift in animal nutrition because at this point nutritional requirements are no longer consider static, but as dynamic processes that develop differently for each individual.
The use of precision feeding instead of conventional group feeding systems already demonstrated several benefits. The increased nutrient-use efficiency and the consequent reduction in the excretion of polluting substances to the environment, improving the overall sustainability of the production system, are the main advantages presented by this feeding system (91). In addition, studies have shown that it is possible to considerably reduce soybean meal and dicalcium phosphate in diet formulations compared to conventional programs. In validation studies (93, 97), individual feeding allowed a reduction in lysine intake by up to 26%, and nitrogen and phosphorus excretion by 30 and 14%, respectively, without affecting the productive pig performance.
Environmental Impacts of Applying Precision Feeding Techniques
Before applying precision feeding techniques, it is necessary to study the environmental impacts of adopting these techniques. An LCA study performed by Andretta et al. (46) intends to estimate the environmental impact of precision feeding techniques applied to pig production. Once again, in Brazilian scenarios, feeding was the largest source of environmental impact. In addition, the study showed that replacing conventional group feeding with daily group feeding (nutrient supply adjusted daily to meet the group requirements) could decrease the potential impact of eutrophication by 4% and acidification by 3%. The mitigation was even greater (up to 6% for the potential impact of climate change and 5% for eutrophication and acidification) when the program was applied to each animal individually (pigs received diets daily tailored to their requirements).
The study also highlighted a reduction over time in the potential impact of climate change associated with pig feed production related to reducing the expected dietary nutrient levels. In the simulated population, reducing the dietary standardized ileal digestible lysine level by one percentage point led to a reduction of up to 194.7 kg of CO2-eq per ton of feed, depending on the simulated scenario. Certainly, the main advantage of this method was the improved nutrient use efficiency. In other words, the same amount of product was produced using fewer resources. Monteiro et al. (34) performed a similar study considering Brazilian and French scenarios with simulated data (the previous study used data collected in vivo). In their study, a precision feeding system that fed pigs individually was able to reduce the impact of climate change by 7%.
Future Challenges
Animals are exposed to several conditions during their lives and these factors may impact directly their nutrient requirements (98). For example, sanitary challenges affect the way amino acids are used by the animal because the nutrients that would be used for protein deposition are directed to cope with the immune system (98). Sanitary challenges also impact the growth performance of pigs and broilers (99), reducing feed efficiency and consequently increasing the environmental impact associated with this production (27). Cadero et al. (54) reported a significant effect of impaired health status on the carbon footprint of pig production. This is only one of several topics that need to be more evaluated in the future, especially in a scenario with reduced use of antibiotics in animal production.
Several studies on the environmental impact of animal production have been published, but only a few have worked using precision feeding programs or considering sanitary challenges. More studies must be carried out to better understand their environmental impact on modern pig and poultry production. Despite all the variability found in livestock, precision systems can foster some eco-friendly solutions by the possibility of managing animals as an individual, having their diets tailored based on real-time data.
Important Aspects to Be Considered When Applying LCA to Animal Science
LCA is a well-known and established method to evaluate environmental impacts, particularly for complex production chains as those in the livestock sector. However, LCA has its limitation like any other scientific method. Some of these limitations have been described by Finkbeiner et al. (100). Some of these gaps may apply in the studies described in this systematic review.
One important limitation observed in the studies was the assessment of water use. Many studies did not include this impact category or they did not consider water consumption (water not returned to the system), which is very relevant for agriculture (100, 101).
The great variability in functional units is certainly another important limitation to be highlighted. The unit choice is a challenging task because it impacts directly on the results and is also related to the objective and scope (100). However, the variability among studies is a great limitation when comparing results since transformations are sometimes not possible or precise (e.g., results expressed for 1 ton of live pig are difficult to compare to those expressed for 1 ton of carcass because there are more processes included and sometimes the carcass yield is not fully known).
In addition, impacts on human health are probably insufficiently covered in LCA studies dealing with pig and poultry production. Soil contamination, noise, and odors are some of these impacts that are not commonly addressed in LCA studies. Additionally, the LCA method fails to consider other aspects, such as biodiversity, welfare, and social aspects (100). The positive impact of specific activities may be also disregarded.
Finally, the choice of a single scenario to represent the reality of an entire production chain is another important limitation of some reviewed LCA studies. The issue related to data gathering was previously highlighted (102). A single model (e.g., data collected in a single scenario) are not able to describe the pig and poultry production systems worldwide, and neither probably across regions. Even in integrated systems that are characterized by a higher level of uniformity, it is possible to observe a different performance in each producer (for the same genetic type, with the same feed, and similar management practices). Thus, variability is something that needs to be considered in future LCA studies.
Conclusion
This systematic review confirmed feeding as the largest source of environmental impact associated with pig and poultry production systems. This supports the hypothesis that novel feeding techniques may mitigate the environmental footprint associated with both production chains. Precision feeding is highlighted as a way to optimize nutrient-use efficiency and, for that reason, as a promising tool toward more sustainable animal production systems. It is still a challenging task to properly consider and compare the variability among LCA studies. Despite these issues, LCA is a comprehensive way to assess sustainability from a global perspective and its application on pig and poultry production systems is very encouraged in future research.
Data Availability Statement
The raw data supporting the conclusions of this article will be made available by the authors upon request.
Author Contributions
AR, CF, CO, MK, and IA searched articles for the systematic review and interpreted results. IA interpreted results, prepared figures, and tables, and wrote the first draft of the manuscript. M-PL-M and CP were involved in the interpretation and discussion of results. FH and AM contributed to manuscript revision, read, and drafted the final version of the manuscript. All authors read and approved the final version of the manuscript.
Conflict of Interest
The authors declare that the research was conducted in the absence of any commercial or financial relationships that could be construed as a potential conflict of interest.
Publisher's Note
All claims expressed in this article are solely those of the authors and do not necessarily represent those of their affiliated organizations, or those of the publisher, the editors and the reviewers. Any product that may be evaluated in this article, or claim that may be made by its manufacturer, is not guaranteed or endorsed by the publisher.
References
1. Tilman D, Cassman KG, Matson PA, Naylor R, Polasky S. Agricultural sustainability and intensive production practices. Nature. (2002) 418:671–7. doi: 10.1038/nature01014
2. Simmons J. Making safe, affordable and abundant food a global reality. Range Beef Cow Symposium. Lincoln (2011).
3. McAuliffe GA, Chapman DV, Sage CL. A thematic review of life cycle assessment (LCA) applied to pig production. Environ Impact Assess Rev. (2016) 56:12–22. doi: 10.1016/j.eiar.2015.08.008
4. Lovatto PA, Hauschild L, Hauptli L, Lehnen CR, Carvalho Ad'A. Modelagem da ingestão, retenção e excreção de nitrogênio e fósforo pela suinocultura Brasileira. J Rev Bras Zootecn. (2005) 34:2348–54. doi: 10.1590/S1516-35982005000700022
5. Basset-Mens C, van der Werf HMG. Scenario-based environmental assessment of farming systems: the case of pig production in France. Agric Ecosyst Environ. (2005) 105:127–44. doi: 10.1016/j.agee.2004.05.007
6. Eriksson IS, Elmquist H, Stern S, Nybrant T. Environmental systems analysis of pig production: the impact of feed choice. Int J Life Cycle Assess. (2005) 10:143–54. doi: 10.1065/lca2004.06.160
7. Basset-Mens C, van der Werf HMG, Durand P, Leterme P. Implications of uncertainty and variability in the Life Cycle Assessment of pig production systems. Int J Life Cycle Assess. (2006) 11:298–304. doi: 10.1065/lca2005.08.219
8. Basset-Mens C, van der Werf HMG, Robin P, Morvan T, Hassouna M, Paillat JM, et al. Methods and data for the environmental inventory of contrasting pig production systems. J Clean Prod. (2007) 15:1395–405. doi: 10.1016/j.jclepro.2006.03.009
9. Liang R, Taniguchi K, Kawashima H, Kikuchi E, Soma T. Estimation of global warming emissions associated with a pig production system by Life Cycle Assessment. J Life Cycle Assess. (2007) 3:178–83. doi: 10.3370/lca.3.178
10. Halberg N, Hermansen JE, Kristensen IS, Eriksen J, Tvedegaard N, Petersen BM. Impact of organic pig production systems on CO2 emission, C sequestration and nitrate pollution. Agron Sustain Dev. (2010) 30:721–31. doi: 10.1051/agro/2010006
11. Aramyan L, Hoste R, van den Broek W, Groot J, Soethoudt H, Nguyen TL, et al. Towards sustainable food production: a scenario study of the European pork sector. J Chain Netw Sci. (2011) 11:177–89. doi: 10.3920/JCNS2011.Qpork8
12. Bonesmo H, Little SM, Harstad OM, Beauchemin KA, Skjelvåg AO, Sjelmo O. Estimating farm-scale greenhouse gas emission intensity of pig production in Norway. Acta Agric Scand Sect A Anim Sci. (2012) 62:318–25. doi: 10.1080/09064702.2013.770913
13. Devers L, Kleynhans TE, Mathijs E. Comparative life cycle assessment of Flemish and Western Cape pork production. Agrekon. (2012) 51:105–28. doi: 10.1080/03031853.2012.741208
14. Dolman MA, Vrolijk HCJ, de Boer IJM. Exploring variation in economic, environmental and societal performance among Dutch fattening pig farms. Livest Sci. (2012) 149:143–54. doi: 10.1016/j.livsci.2012.07.008
15. Stone JJ, Dollarhide CR, Benning JL, Gregg Carlson C, Clay DE. The life cycle impacts of feed for modern grow-finish Northern Great Plains US swine production. Agric Syst. (2012) 106:1–10. doi: 10.1016/j.agsy.2011.11.002
16. De Moraes PJU, Allison J, Robinson JA, Baldo GL, Boeri F, Borla P. Life cycle assessment (LCA) and environmental product declaration (EPD) of an immunological product for boar taint control in male pigs. J Environ Assess Policy Manag. (2013) 15:1–26. doi: 10.1142/S1464333213500014
17. Luo Y, Stichnothe H, Schuchardt F, Li G, Huaitalla RM, Xu W. Life cycle assessment of manure management and nutrient recycling from a Chinese pig farm. Waste Manag Res. (2013) 32:4–12. doi: 10.1177/0734242X13512715
18. Ogino A, Osada T, Takada R, Takagi T, Tsujimoto S, Tonoue T, et al. Life cycle assessment of Japanese pig farming using low-protein diet supplemented with amino acids. Soil Sci Plant Nutr. (2013) 59:107–18. doi: 10.1080/00380768.2012.730476
19. Reckmann K, Traulsen I, Krieter J. Life Cycle Assessment of pork production: a data inventory for the case of Germany. Livest Sci. (2013) 157:586–96. doi: 10.1016/j.livsci.2013.09.001
20. Dourmad JY, Ryschawy J, Trousson T, Bonneau M, Gonzàlez J, Houwers HWJ, et al. Evaluating environmental impacts of contrasting pig farming systems with life cycle assessment. Animal. (2014) 8:2027–37. doi: 10.1017/S1751731114002134
21. Jacobsen R, Vandermeulen V, Van Huylenbroeck G, Gellynck X. Carbon footprint of pigmeat in Flanders. Int J Agric Sustain. (2014) 12:54–70. doi: 10.1080/14735903.2013.798896
22. Sasu-Boakye Y, Cederberg C, Wirsenius S. Localising livestock protein feed production and the impact on land use and greenhouse gas emissions. Animal. (2014) 8:1339–48. doi: 10.1017/S1751731114001293
23. Cherubini E, Zanghelini GM, Alvarenga RAF, Franco D, Soares SR. Life cycle assessment of swine production in Brazil: a comparison of four manure management systems. J Clean Prod. (2015) 87:68–77. doi: 10.1016/j.jclepro.2014.10.035
24. Cherubini E, Zanghelini GM, Tavares JMR, Belettini F, Soares SR. The finishing stage in swine production: influences of feed composition on carbon footprint. Environ Dev Sustain. (2015) 17:1313–28. doi: 10.1007/s10668-014-9607-9
25. González-García S, Belo S, Dias AC, Rodrigues JV, Costa RRD, Ferreira A, Andrade LPD, Arroja L. Life cycle assessment of pigmeat production: Portuguese case study and proposal of improvement options. J Clean Prod. (2015) 100:126–39. doi: 10.1016/j.jclepro.2015.03.048
26. Mackenzie SG, Leinonen I, Ferguson N, Kyriazakis I. Accounting for uncertainty in the quantification of the environmental impacts of Canadian pig farming systems1. J Anim Sci. (2015) 93:3130–43. doi: 10.2527/jas.2014-8403
27. Reckmann K, Krieter J. Environmental impacts of the pork supply chain with regard to farm performance. J Agric Sci. (2015) 153:411–21. doi: 10.1017/S0021859614000501
28. van Zanten HH, Bikker P, Mollenhorst H, Meerburg BG, de Boer IJM. Environmental impact of replacing soybean meal with rapeseed meal in diets of finishing pigs. Animal. (2015) 9:1866–74. doi: 10.1017/S1751731115001469
29. Wang X, Dadouma A, Chen Y, Sui P, Gao W, Jia L. Sustainability evaluation of the large-scale pig farming system in North China: an emergy analysis based on life cycle assessment. J Clean Prod. (2015) 102:144–64. doi: 10.1016/j.jclepro.2015.04.071
30. Groen EA, van Zanten HHE, Heijungs R, Bokkers EAM, de Boer IJM. Sensitivity analysis of greenhouse gas emissions from a pork production chain. J Clean Prod. (2016) 129:202–11. doi: 10.1016/j.jclepro.2016.04.081
31. Kebreab E, Liedke A, Caro D, Deimling S, Binder M, Finkbeiner M. Environmental impact of using specialty feed ingredients in swine and poultry production: a life cycle assessment1. J Anim Sci. (2016) 94:2664–81. doi: 10.2527/jas.2015-9036
32. Lamnatou C, Ezcurra-Ciaurriz X, Chemisana D, Plà-Aragonés LM. Environmental assessment of a pork-production system in North-East of Spain focusing on life-cycle swine nutrition. J Clean Prod. (2016) 137:105–15. doi: 10.1016/j.jclepro.2016.07.051
33. Mackenzie SG, Leinonen I, Ferguson N, Kyriazakis I. Can the environmental impact of pig systems be reduced by utilising co-products as feed? J Clean Prod. (2016) 115:172–81. doi: 10.1016/j.jclepro.2015.12.074
34. Monteiro A, Garcia-Launay F, Brossard L, Wilfart A, Dourmad JY. Effect of feeding strategy on environmental impacts of pig fattening in different contexts of production: evaluation through life cycle assessment. J Anim Sci. (2016) 94:4832–47. doi: 10.2527/jas.2016-0529
35. Noya I, Aldea X, Gasol CM, Gonzalez-Garcia S, Amores MJ, Colon J, et al. Carbon and water footprint of pork supply chain in Catalonia: From feed to final products. J Environ Manage. (2016) 171:133–43. doi: 10.1016/j.jenvman.2016.01.039
36. Pirlo G, Care S, Casa GD, Marchetti R, Ponzoni G, Faeti V, et al. Environmental impact of heavy pig production in a sample of Italian farms. A cradle-to-farm gate analysis. Sci Total Environ. (2016) 565:576–85. doi: 10.1016/j.scitotenv.2016.04.174
37. Sagastume Gutiérrez A, Cabello Eras JJ, Billen P, Vandecasteele C. Environmental assessment of pig production in Cienfuegos, Cuba: alternatives for manure management. J Clean Prod. (2016) 112:2518–28. doi: 10.1016/j.jclepro.2015.09.082
38. Wang X, Wu X, Yan P, Gao W, Chen Y, Sui P. Integrated analysis on economic and environmental consequences of livestock husbandry on different scale in China. J Clean Prod. (2016) 119:1–12. doi: 10.1016/j.jclepro.2016.01.084
39. Ali BM, van Zanten HHE, Berentsen P, Bastiaansen JWM, Bikker P, Lansink AO. Environmental and economic impacts of using co-products in the diets of finishing pigs in Brazil. J Clean Prod. (2017) 162:247–59. doi: 10.1016/j.jclepro.2017.06.041
40. Bava L, Zucali M, Sandrucci A, Tamburini A. Environmental impact of the typical heavy pig production in Italy. J Clean Prod. (2017) 140:685–91. doi: 10.1016/j.jclepro.2015.11.029
41. Li Z, Sui P, Wang X, Yang X, Long P, Cui J, et al. Comparison of net GHG emissions between separated system and crop-swine integrated system in the North China Plain. J Clean Prod. (2017) 149:653–64. doi: 10.1016/j.jclepro.2017.02.113
42. Monteiro ANTR, Bertol TM, de Oliveira PAV, Dourmad JY, Coldebella A, Kessler AM. The impact of feeding growing-finishing pigs with reduced dietary protein levels on performance, carcass traits, meat quality and environmental impacts. Livestock Sci. (2017) 198:162–9. doi: 10.1016/j.livsci.2017.02.014
43. Noya I, Aldea X, Gonzalez-Garcia S, Gasol CM, Moreira MT, Amores MJ, et al. Environmental assessment of the entire pork value chain in Catalonia - a strategy to work towards Circular Economy. Sci Total Environ. (2017) 589:122–9. doi: 10.1016/j.scitotenv.2017.02.186
44. Noya I, Villanueva-Rey P, Gonzalez-Garcia S, Fernandez MD, Rodriguez MR, Moreira MT. Life Cycle Assessment of pig production: a case study in Galicia. J Clean Prod. (2017) 142:4327–38. doi: 10.1016/j.jclepro.2016.11.160
45. Six L, De Wilde B, Vermeiren F, Van Hemelryck S, Vercaeren M, Zamagni A, et al. Using the product environmental footprint for supply chain management: lessons learned from a case study on pork. Int J Life Cycle Assess. (2017) 22:1354–72. doi: 10.1007/s11367-016-1249-8
46. Andretta I, Hauschild L, Kipper M, Pires P, Pomar C. Environmental impacts of precision feeding programs applied in pig production. Animal. (2018) 12:1990–−8. doi: 10.1017/S1751731117003159
47. Rudolph G, Hörtenhuber S, Bochicchio D, Butler G, Brandhofer R, Dippel S, et al. Effect of three husbandry systems on environmental impact of organic pigs. Sustainability. (2018) 10:3796. doi: 10.3390/su10103796
48. Arrieta EM, González AD. Energy and carbon footprints of chicken and pork from intensive production systems in Argentina. Sci Total Environ. (2019) 673:20–8. doi: 10.1016/j.scitotenv.2019.04.002
49. Monteiro A, Dourmad JY, Fachinello MR, Diaz-Huepa LM, Sitanaka NY, Partyka AVS, et al. Effect of observed individual data of performance and excretion on life cycle assessment of piglets. Sci Agric. (2019) 76:102–11. doi: 10.1590/1678-992x-2017-0094
50. Monteiro ANTR, Wilfart A, Utzeri VJ, Batorek Lukač N, TomaŽin U, Costa LN, et al. Environmental impacts of pig production systems using European local breeds: the contribution of carbon sequestration and emissions from grazing. J Clean Prod. (2019) 237:117843. doi: 10.1016/j.jclepro.2019.117843
51. Ottosen M, Mackenzie SG, Wallace M, Kyriazakis I. A method to estimate the environmental impacts from genetic change in pig production systems. Int J Life Cycle Assess. (2019) 25:523–37. doi: 10.1007/s11367-019-01686-8
52. Reyes YA, Barrera EL, Valle AS, Gil MP, Garcia OH, Dewulf J. Life Cycle Assessment for the Cuban pig production: case study in Sancti Spiritus. J Clean Prod. (2019) 219:99–109. doi: 10.1016/j.jclepro.2019.02.047
53. Anestis V, Papanastasiou DK, Bartzanas T, Giannenas I, Skoufos I, Kittas C. Effect of a dietary modification for fattening pigs on the environmental performance of commercial pig production in Greece. Sustain Prod Consum. (2020) 22:162–76. doi: 10.1016/j.spc.2020.03.002
54. Cadero A, Aubry A, Dourmad JY, Salaun Y, Garcia-Launay F. Effects of interactions between feeding practices, animal health and farm infrastructure on technical, economic and environmental performances of a pig-fattening unit. Animal. (2020) 14:s348–s359. doi: 10.1017/S1751731120000300
55. Garcia-Gudino J, Monteiro A, Espagnol S, Blanco-Penedo I, Garcia-Launay F. Life Cycle Assessment of Iberian traditional pig production system in Spain. Sustainability. (2020) 12:627. doi: 10.3390/su12020627
56. Horrillo A, Gaspar P. Organic farming as a strategy to reduce carbon footprint in dehesa agroecosystems: a case study comparing different livestock products. Animals. (2020) 10:162. doi: 10.3390/ani10010162
57. Monteiro ANTR, Dourmad JY, Moreira CA, Rossi RM, Ferreira LFM, Costa AER, et al. The source of performance and excretion data affects the environmental impact of pig rearing estimated by life cycle assessment. Can J Anim Sci. (2020) 100:184–92. doi: 10.1139/cjas-2019-0022
58. Pexas G, Mackenzie SG, Wallace M, Kyriazakis I. Environmental impacts of housing conditions and manure management in European pig production systems through a life cycle perspective: a case study in Denmark. J Clean Prod. (2020) 253:120005. doi: 10.1016/j.jclepro.2020.120005
59. Bennett RM, Phipps RH, Strange AM. The use of life cycle assessment to compare the environmental impact of production and feeding of conventional and genetically modified maize for broiler production in Argentina. J Anim Feed Sci. (2006) 15:71–82. doi: 10.22358/jafs/66843/2006
60. Mollenhorst H, Berentsen PBM, De Boer IJM. On-farm quantification of sustainability indicators: an application to egg production systems. Br Poult Sci. (2006) 47:405–17. doi: 10.1080/00071660600829282
61. Pelletier N. Environmental performance in the US broiler poultry sector: life cycle energy use and greenhouse gas, ozone depleting, acidifying and eutrophying emissions. Agric Syst. (2008) 98:67–73. doi: 10.1016/j.agsy.2008.03.007
62. Leinonen I, Williams AG, Wiseman J, Guy J, Kyriazakis I. Predicting the environmental impacts of chicken systems in the United Kingdom through a life cycle assessment: broiler production systems. Poult Sci. (2012) 91:8–25. doi: 10.3382/ps.2011-01634
63. Leinonen I, Williams AG, Wiseman J, Guy J, Kyriazakis I. Predicting the environmental impacts of chicken systems in the United Kingdom through a life cycle assessment: egg production systems. Poult Sci. (2012) 91:26–40. doi: 10.3382/ps.2011-01635
64. Leinonen I, Williams AG, Waller AH, Kyriazakis I. Comparing the environmental impacts of alternative protein crops in poultry diets: The consequences of uncertainty. Agric Syst. (2013) 121:33–42. doi: 10.1016/j.agsy.2013.06.008
65. Pelletier N, Ibarburu M, Xin H. A carbon footprint analysis of egg production and processing supply chains in the Midwestern United States. J Clean Prod. (2013) 54:108–14. doi: 10.1016/j.jclepro.2013.04.041
66. Thévenot A, Aubin J, Tillard E, Vayssières J. Accounting for farm diversity in Life Cycle Assessment studies - the case of poultry production in a tropical island. J Clean Prod. (2013) 57:280–92. doi: 10.1016/j.jclepro.2013.05.027
67. González-García S, Gomez-Fernández Z, Dias AC, Feijoo G, Moreira MT, Arroja L. Life Cycle Assessment of broiler chicken production: a Portuguese case study. J Clean Prod. (2014) 74:125–34. doi: 10.1016/j.jclepro.2014.03.067
68. Leinonen I, Williams AG, Kyriazakis I. The effects of welfare-enhancing system changes on the environmental impacts of broiler and egg production. Poult Sci. (2014) 93:256–66. doi: 10.3382/ps.2013-03252
69. Prudêncio da Silva V, van der Werf HMG, Soares SR, Corson MS. Environmental impacts of French and Brazilian broiler chicken production scenarios: An LCA approach. J Environ Manag. (2014) 133:222–31. doi: 10.1016/j.jenvman.2013.12.011
70. Taylor RC, Omed H, Edwards-Jones G. The greenhouse emissions footprint of free-range eggs. Poult Sci. (2014) 93:231–7. doi: 10.3382/ps.2013-03489
71. Ghasempour A, Ahmadi E. Assessment of environment impacts of egg production chain using life cycle assessment. J Environ Manage. (2016) 183:980–7. doi: 10.1016/j.jenvman.2016.09.054
72. Kalhor T, Rajabipour A, Akram A, Sharifi M. Environmental impact assessment of chicken meat production using life cycle assessment. Inf Process Agric. (2016) 3:262–71. doi: 10.1016/j.inpa.2016.10.002
73. Leinonen I, Williams AG, Kyriazakis I. Potential environmental benefits of prospective genetic changes in broiler traits. Poult Sci. (2016) 95:228–36. doi: 10.3382/ps/pev323
74. Cesari V, Zucali M, Sandrucci A, Tamburini A, Bava L, Toschi I. Environmental impact assessment of an Italian vertically integrated broiler system through a Life Cycle approach. J Clean Prod. (2017) 143:904–11. doi: 10.1016/j.jclepro.2016.12.030
75. Giannenas I, Bonos E, Anestis V, Filioussis G, Papanastasiou DK, Bartzanas T, et al. Effects of protease addition and replacement of soybean meal by corn gluten meal on the growth of broilers and on the environmental performances of a broiler production system in Greece. PLoS One. (2017) 12:e0169511. doi: 10.1371/journal.pone.0169511
76. Mainali B, Emran SB, Silveira S. Greenhouse gas mitigation using poultry litter management techniques in Bangladesh. Energy. (2017) 127:155–66. doi: 10.1016/j.energy.2017.03.103
77. Payandeh Z, Kheiralipour K, Karimi M, Khoshnevisan B. Joint data envelopment analysis and life cycle assessment for environmental impact reduction in broiler production systems. Energy. (2017) 127:768–74. doi: 10.1016/j.energy.2017.03.112
78. Pelletier N. Life cycle assessment of Canadian egg products, with differentiation by hen housing system type. J Clean Prod. (2017) 152:167–80. doi: 10.1016/j.jclepro.2017.03.050
79. Pishgar-Komleh SH, Akram A, Keyhani A, van Zelm R. Life cycle energy use, costs, and greenhouse gas emission of broiler farms in different production systems in Iran-a case study of Alborz province. Environ Sci Pollut Res. (2017) 24:16041–9. doi: 10.1007/s11356-017-9255-3
80. Wiedemann SG, McGahan EJ, Murphy CM. Resource use and environmental impacts from Australian chicken meat production. J Clean Prod. (2017) 140:675–84. doi: 10.1016/j.jclepro.2016.06.086
81. Abín R, Laca A, Laca A, Díaz M. Environmental assessment of intensive egg production: a Spanish case study. J Clean Prod. (2018) 179:160–8. doi: 10.1016/j.jclepro.2018.01.067
82. Skunca D, Tomasevic I, Nastasijevic I, Tomovic V, Djekic I. Life cycle assessment of the chicken meat chain. J Clean Prod. (2018) 184:440–50. doi: 10.1016/j.jclepro.2018.02.274
83. Duarte da Silva Lima N, de Alencar Nääs I, Garcia RG, Jorge de Moura D. Environmental impact of Brazilian broiler production process: evaluation using life cycle assessment. J Clean Prod. (2019) 237:117752. doi: 10.1016/j.jclepro.2019.117752
84. Ramedani Z, Alimohammadian L, Kheialipour K, Delpisheh P, Abbasi Z. Comparing energy state and environmental impacts in ostrich and chicken production systems. Environ Sci Pollut Res. (2019) 26:28284–93. doi: 10.1007/s11356-019-05972-8
85. van Hal O, Weijenberg AAA, de Boer IJM, van Zanten HHE. Accounting for feed-food competition in environmental impact assessment: Towards a resource efficient food-system. J Clean Prod. (2019) 240:118241. doi: 10.1016/j.jclepro.2019.118241
86. Estrada-González I, Taboada-González P, Guerrero-García-Rojas H, Marquez-Benavides L. Decreasing the environmental impact in an egg-producing farm through the application of LCA and lean tools. Appl Sci. (2020) 10:1352. doi: 10.3390/app10041352
87. Pelletier N, Lammers P, Stender D, Pirog R. Life cycle assessment of high- and low-profitability commodity and deep-bedded niche swine production systems in the Upper Midwestern United States. Agric Syst. (2010) 103:599–608. doi: 10.1016/j.agsy.2010.07.001
88. Stone J, Aurand K, Clay S, Thaler R. Life cycle assessment of tylosin and chlortetracycline antimicrobial use at swine production facilities. In: Rocky Mountain - 62nd Annual Meeting. Geological Society of America Abstracts with Programs. Denver, CO (2010) 42:17.
89. Jean dit Bailleul P, Rivest J, Dubeau F, Pomar C. Reducing nitrogen excretion in pigs by modifying the traditional least-cost formulation algorithm. Livest Prod Sci. (2001) 72:199–211. doi: 10.1016/S0301-6226(01)00224-X
90. Monteiro ANTR, Garcia-Launay F, Brossard L, Wilfart A, Dourmad JY. Effect of precision feeding on environmental impact of fattening pig production. In: European Conference on Precision Livestock Farming (ECPLF). Nantes (2017). p. 565–73.
91. Pomar C, van Milgen J, Remus A. Precision livestock feeding, principle and practice. In: Hendriks WH, Verstegen MWA, Babinszky L, editors. Poultry an Pig Nutrition Challenges of the 21st century. Wageningen: Wageningen Academic Publishers (2019). p. 397–418. doi: 10.3920/978-90-8686-884-1_18
92. Hauschild L, Pomar C, Lovatto PA. Systematic comparison of the empirical and factorial methods used to estimate the nutrient requirements of growing pigs. Animal. (2010) 4:714–23. doi: 10.1017/S1751731109991546
93. Andretta I, Pomar C, Rivest J, Pomar J, Radünz J. Precision feeding can significantly reduce lysine intake and nitrogen excretion without compromising the performance of growing pigs. Animal. (2016) 10:1137–47. doi: 10.1017/S1751731115003067
94. Dourmad J, Guingand N, Latimier P, Seve B. Nitrogen and phosphorus consumption, utilisation and losses in pig production: France. Livest Sci. (1999) 58:199–211. doi: 10.1016/S0301-6226(99)00009-3
95. Rakhshandeh A, Htoo JK, Karrow N, Miller SP, de Lange CF. Impact of immune system stimulation on the ileal nutrient digestibility and utilisation of methionine plus cysteine intake for whole-body protein deposition in growing pigs. Br J Nutr. (2013) 111:101–10. doi: 10.1017/S0007114513001955
96. Remus A, Hauschild L, Pomar C. Simulated amino acid requirements of growing pigs differ between current factorial methods. Animal. (2020) 14:725–30. doi: 10.1017/S1751731119002660
97. Andretta I, Pomar C, Rivest J, Pomar J, Lovatto P, Radünz Neto J. The impact of feeding growing-finishing pigs with daily tailored diets using precision feeding techniques on animal performance, nutrient utilization, and body and carcass composition. J Anim Sci. (2014) 92:3925–36. doi: 10.2527/jas.2014-7643
98. Le Floc'h N, Gondret F, Matte JJ, Quesnel H. Towards amino acid recommendations for specific physiological and patho-physiological states in pigs. Proc Nutr Soc. (2012) 71:425–32. doi: 10.1017/S0029665112000560
99. Pastorelli H, van Milgen J, Lovatto P, Montagne L. Meta-analysis of feed intake and growth responses of growing pigs after a sanitary challenge. Animal. (2012) 6:952–61. doi: 10.1017/S175173111100228X
100. Finkbeiner M, Ackermann R, Bach V, Berger M, Brankatschk G, Chang Y-J, et al. Challenges in life cycle assessment: an overview of current gaps and research needs. In: W Klöpffer, editor, Background and Future Prospects in Life Cycle Assessment. Dordrecht: Springer (2014). p. 207–58.
101. Shiklomanov I, Rodda JC. World Water Resources at the Beginning of the 21st Century. International Hydrology Series. Cambridge: Cambridge University Press (2003).
Keywords: sustainability, swine, broilers, environment, livestock, climate change, laying hens, precision feeding
Citation: Andretta I, Hickmann FMW, Remus A, Franceschi CH, Mariani AB, Orso C, Kipper M, Létourneau-Montminy MP and Pomar C (2021) Environmental Impacts of Pig and Poultry Production: Insights From a Systematic Review. Front. Vet. Sci. 8:750733. doi: 10.3389/fvets.2021.750733
Received: 31 July 2021; Accepted: 23 September 2021;
Published: 27 October 2021.
Edited by:
Emma Fàbrega Romans, Institute of Agrifood Research and Technology (IRTA), SpainReviewed by:
Siaka Seriba Diarra, University of the South Pacific, FijiPeter John White, The University of Sydney, Australia
Copyright © 2021 Andretta, Hickmann, Remus, Franceschi, Mariani, Orso, Kipper, Létourneau-Montminy and Pomar. This is an open-access article distributed under the terms of the Creative Commons Attribution License (CC BY). The use, distribution or reproduction in other forums is permitted, provided the original author(s) and the copyright owner(s) are credited and that the original publication in this journal is cited, in accordance with accepted academic practice. No use, distribution or reproduction is permitted which does not comply with these terms.
*Correspondence: Ines Andretta, ines.andretta@ufrgs.br; Aline Remus, aline.remus@agr.gc.ca